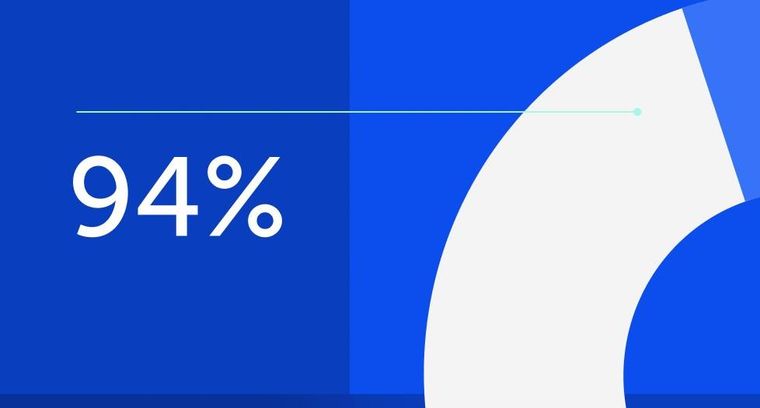
94% of researchers rate our articles as excellent or good
Learn more about the work of our research integrity team to safeguard the quality of each article we publish.
Find out more
ORIGINAL RESEARCH article
Front. Ecol. Evol., 13 September 2022
Sec. Paleoecology
Volume 10 - 2022 | https://doi.org/10.3389/fevo.2022.1000168
Serows and gorals (Bovidae, Caprinae) are emblematic antelopes distributed in Southeast Asia. They all are nearly threaten or vulnerable species nowadays despite having a more widespread distribution during the Pleistocene. Fossils of three native caprine species, i.e., a Sumatran serow Capricornis sumatraensis, Chinese goral Naemorhedus griseus, and Himalayan goral Naemorhedus goral, were recovered from four Pleistocene paleontological and archeological sites in Thailand, namely Pha Bong, Khok Sung, Tham Wiman Nakin, and Tham Lod Rockshelter. To investigate dietary changes of these Southeast Asian serows and gorals through the Quaternary, differences in feeding habits and habitat preferences between the Pleistocene and extant populations were examined using the hypsodonty index (HI) together with the mesowear II method and the dental microwear texture analysis (DMTA). The HI and mesowear-II results showed that Pleistocene and extant caprines were mixed feeders. For the Pleistocene caprine populations, the DMTA results suggested more browsing signals for C. sumatraensis than both Naemorhedus species that were mixed feeders similar to their extant populations. The DMTA demonstrated a considerable dietary overlap among the Pleistocene sympatric caprine populations. The dental microwear results also revealed that the extant C. sumatraensis is a leaf-dominant browser, while the extant N. griseus possibly feeds on seeds or tough food items. These data combined with previous stable carbon isotope analyses reinforce the idea that the restricted ranges of habitats for these extant serow and goral populations have possibly been driven by the Holocene climatic and environmental changes as well as the negative effects of human activities.
Serows (Capricornis) and gorals (Naemorhedus), the family Bovidae and subfamily Caprinae, are ruminants with a goat-like or antelope-like appearance. Members of these two genera are present today in Southeast Asia, South Asia, and China (Lekagul and McNeely, 1988; Wilson and Mittermeier, 2011). Although taxonomic status and the existing number of species in these two genera have been matters for debate so far, four recognized species, i.e., Sumatran serows (Capricornis sumatraensis), red serows (Capricornis rubidus), Chinese gorals (Naemorhedus griseus), and Himalayan gorals (Naemorhedus goral), are likely to be valid based on most recent studies of morphological and molecular phylogenetic analyses (Bover et al., 2019; Mori et al., 2019; Joshi et al., 2022). These present-day serows and gorals are found in high-altitude areas such as steep limestone mountains, cliffs, and caves, at the elevation ranging from 200 to 3,000 m for Sumatran serows (Lekagul and McNeely, 1988; Wilson and Mittermeier, 2011) and from 900 to 4,000 m for those gorals (Lekagul and McNeely, 1988; Chaiyarat et al., 1999; Bhattacharya et al., 2012). These three caprine taxa are listed as endangered or near-threatened species and are protected by CITES Appendix I (Duckworth et al., 2008; Duckworth and MacKinnon, 2008; Phan et al., 2020).
However, only two caprine species, C. sumatraensis and N. griseus, are nowadays distributed in Thailand (Figure 1). The former species is found in the southern, western, and northern parts of Thailand (Chairat, 2007; Wilson and Mittermeier, 2011; Nakasathien, 2017), whereas N. griseus is present only in the northern region where the coexistence of these two species occurs (Buranapim et al., 2014; Safoowong, 2015; Jangtarwan et al., 2020). Fossils of caprines were recovered from four paleontological and archeological sites of which the faunal ages range from the middle Pleistocene to the late Pleistocene (Suraprasit et al., 2016, 2021a; Bocherens et al., 2017; Wattanapituksakul et al., 2018). Fossil evidence indicates that N. goral coexisted with C. sumatraensis and N. griseus in low-altitude areas of Thailand at the elevation below 800 m above mean sea level during the Pleistocene (Tougard, 1998, 2001; Shoocongdej et al., 2007; Bocherens et al., 2017; Wattanapituksakul et al., 2018; Suraprasit et al., 2020). Based on the stable carbon isotope analysis of mammalian tooth enamel, it has been suggested that environmental changes in Southeast Asia from the Pleistocene savannah to the Holocene rainforest have contributed to the extinction of some grassland-dwelling mammals (Louys and Robert, 2020). For instance, the extinction of N. goral in Thailand might have been driven by the reduction of open habitats during the Holocene (Suraprasit et al., 2020). In addition to the replacement of open grassland landscapes by more closed rainforests, the decreasing number of wild caprine populations is mostly caused by anthropogenic activities such as overhunting and deforestation for timber trading or rice field expansion (Ellen et al., 2000; Sodhi et al., 2004; for more detailed information of caprines in Thailand, see in Supplementary material 1). This raises the importance of paleoecological studies to test how environmental changes and anthropogenic activities have driven the present-day species’ range contractions.
Figure 1. The distribution of extant and Pleistocene caprines in Southeast Asia, the eastern region of South Asia, and South China [modified after Suraprasit et al. (2020)]. Six paleontological and archeological sites in Southeast Asia mentioned in this study: 1 = Pha Bong, Mae Hong Son (Bocherens et al., 2017); 2 = Khok Sung, Nakhon Ratchasima (Suraprasit et al., 2016); 3 = Tham Wiman Nakin, Chaiyaphum (Suraprasit et al., 2021a); 4 = Tham Lod Rockshelter, Mae Hong Son (Wattanapituksakul et al., 2018); 5 = Boh Dambang, Cambodia (Bacon et al., 2018b); and 6 = Nam Lot, Laos (Bacon et al., 2018a). Extant populations of C. sumatraensis and N. griseus have overlapping distribution areas at the northern part of Thailand, Myanmar, and China.
The paleoecology of bovids has been extensively investigated during the past two decades, using several methods (e.g., analyses of the stable carbon/nitrogen isotopes in animals’ tissues, limb ecomorphometrics, and dental wear patterns), allowing paleontologists to have access to individual and populational life history traits (Green and Croft, 2018). Nonetheless, information on diet and habitat preferences of Pleistocene caprines in Thailand remains scarce and has been only obtained from the stable carbon isotope measurements of mammalian tooth enamel (Pushkina et al., 2010; Bocherens et al., 2017; Suraprasit et al., 2018, 2020, 2021b) and the dental mesowear-I analysis (Isarankura Na Ayudhya et al., 2021).
The Southeast Asian Pleistocene C. sumatraensis has been identified as a generalist foraging chiefly on either pure C3 or C4 plants in both closed forest and open grassland landscapes, in response to local environmental conditions based on stable carbon isotope studies (e.g., Pushkina et al., 2010; Bacon et al., 2018a,b; Suraprasit et al., 2018, 2020). In extant populations from Thailand, Laos, and Indonesia, C. sumatraensis has been considered to be a pure C3 feeder (Pushkina et al., 2010; Suraprasit et al., 2020). However, the δ13C results showed that Pleistocene N. griseus and N. goral in Thailand were more specialized feeders focusing on either pure C4 or mixed C3/C4 plants (Suraprasit et al., 2020). One specimen of extant N. griseus shows a mixed C3/C4 diet, while the extant N. goral feeds on pure C3 or mixed C3/C4 plants (Suraprasit et al., 2020). It is inferred from this isotope evidence that the extant caprine populations live in more restricted habitats than the Pleistocene ones. As all grasslands in the tropics are mostly covered by C4 grasses and sedge today (Sage, 2004; Edwards and Still, 2008), the dichotomy between C3 and C4 diets may reflect the habitats used by animals (open vs. closed) but not the types of vegetation that they consumed (Cerling and Harris, 1999). Thus, the question is raised whether the extant gorals mainly graze on C3 monocots, or otherwise browse in C3 bushes, trees, and shrubs. In addition, the mesowear I analysis suggested a more browsing signal for C. sumatraensis but similar feeding habits for both species of Naemorhedus through the Pleistocene and Holocene (Isarankura Na Ayudhya et al., 2021), unlike the achievement of previously analyzed carbon isotope data (Suraprasit et al., 2020).
While stable carbon isotope ratios can identify the photosynthetic pathways (C3 or C4) of the food vegetation resources, other proxies such as hypsodonty index (HI) together with mesowear II and dental microwear texture analysis (DMTA) indicate mechanical properties and inner compositions of foods in relation to ungulate feeding habits. The HI is a morphological study of a dental phenotype related to feeding habits and habitats through the evolutionary adaptation (Janis, 1988; Janis, 2008). Ungulates that feasibly live in open habitats tend to have high hypsodonty because of high consumption of silica-rich grasses and food contaminated with grits. Typically, the HI is often used to analyze in parallel with another proxy called mesowear for indicating feeding habits in a long temporal scale (Kaiser et al., 2013; Semprebon et al., 2019). Mesowear analysis refers to macroscopic tooth wear of attrition (tooth to tooth contact) and abrasion (tooth to food contact), according to the primary definition by Fortelius and Solounias (2000). The mesowear indicates a lifelong habit of species represented by its average diet from a specific location in space and time (Fortelius and Solounias, 2000; Ackermans, 2020). Because of an easy accessibility, the mesowear analysis has been applied to the study of diets and habitats in several herbivores using various scoring systems (e.g., Kaiser et al., 2009; Mihlbachler et al., 2010; Taylor et al., 2013). One of the mesowear scoring systems used for illustrating dietary feeding habits of ungulates is a mesowear II method (Rivals and Semprebon, 2006; Kaiser et al., 2009). Mesowear-II examines the attrition-abrasion signal through two variables: occlusal relief (OR) and cusp shape (CS), combining into a single variable named as a mesowear score (MS) (Kaiser et al., 2009). Grass phytoliths as well as dust in diets result in more abrasion than soft foliage (Baker et al., 1959; Damuth and Janis, 2011). Therefore, teeth of grazing ungulates that mainly consume grasses or nearby ground-level vegetation are more prone to abrasions than those of browsing animals. Unlike mesowear, dental microwear can provide more detailed information about food in a short-term period and can demonstrate directly the nature of meals of the last few days to weeks of an individual before death (Teaford and Oyen, 1989; Winkler and Kaiser, 2011; Davis and Pineda-Munoz, 2016; Teaford et al., 2017). Dental microwear textures can track differences in abrasive particles such as biosilica in herbaceous monocots and/or test the tissue composition such as lignin in food items (Walker et al., 1978; Scott et al., 2006; Scott, 2012; Barron-Ortiz et al., 2014). The dental microwear texture is then characterized by using the scale-sensitive fractal analysis (SSFA) (Scott et al., 2006). Microwear analysis might provide additional information on short-time dietary patterns such as dietary seasonal switch, sex differences, and niche partitioning (Calandra and Merceron, 2016; DeSantis, 2016). Therefore, the combination between mesowear and microwear has often been used to fill the gap between short- and long-term scales for dietary reconstructions (e.g., Rivals et al., 2011; Barron-Ortiz et al., 2014; Mihlbachler et al., 2018). In the present study, we investigated dietary and habitat preferences of Pleistocene fossil caprines and their extant populations through the combination of the HI (evolutionarily related dietary proxies) together with the mesowear-II (long-term dietary proxies) and dental microwear texture analyses (short-term dietary proxies; Davis and Pineda-Munoz, 2016). Our results are compared with previous stable carbon isotope data and field observation studies of extant wild populations. The information on diets and feeding habits of these caprines is useful to deepen the understanding of their dietary adaptations through the Quaternary and may provide some clues to propose appropriate ecological guidelines for future conservation biology politics.
Sixty-three fossil specimens were collected from four paleontological and archeological sites in Thailand: Pha Bong, Khok Sung, Tham Wiman Nakin, and Tham Lod Rockshelter, all of which cover the ages ranging from the middle to latest Pleistocene (for more detailed information, see Supplementary material 2). The number of fossil specimens in each locality is given in Table 1.
Table 1. General information of four paleontological and archeological sites and the number of specimens in this study.
In this study, a total of 39 extant caprine specimens include C. sumatraensis (n = 22), N. griseus (n = 12), and N. goral (n = 5) issued from ten collections: (1) Khao Nang Ram Wildlife Research Center, Uthai Thani (KNR), (2) Om Koi Wildlife Breeding Center, Chiang Mai (OK), (3) Natural History Museum of Chulalongkorn University, Bangkok (CUMNH), (4) Zoological Museum of Kasetsart University, Bangkok (ZMKU), (5) Forestry Museum of Kasetsart University, Bangkok (FMKU), (6) National Science Museum, Pathum Thani (THNHM), (7) Natural History Museum (British Museum collection), United Kingdom (BM), (8) Naturhistorisches Museum Basel, Switzerland (NMB), (9) Muséum National d’Histoire Naturelle, France (MNHN), and (10) Zoologische Staatssammlung München, Germany (ZSM). Specimens from the zoo were excluded from this study because the diet and habitat of animals are not naturally controlled. This research has been approved for the use of wild specimens by the Department of National Park, Wildlife and Plant Conservation, Thailand (Permission number 0907.4/6178).
To calculate the HI, the crown height of the lower third molar (m3) was divided by its width (Janis, 1988). As most of the m3 of extant individuals have remained embedded in the jaw bone, measurements of a tooth crown height might be underestimated. Therefore, the HI of isolated teeth of Pleistocene caprines C. sumatraensis (n = 7), N. griseus (n = 7), and N. goral (n = 6) was calculated and used as representatives of extant individuals.
The HI of mammals represents dental morphological characteristics throughout lifetime linked to their evolutionary adaptation (Janis, 2008). At the evolutionary time scales, the HI is commonly regarded as a species-specific parameter for interpreting progressively wear-inducing diets and environments for herbivorous mammals (Kaiser et al., 2013). In principle, high hypsodonty caused by the ingestion of abrasive dietary bolus (silica-bearing plant/grit digestion) is usually associated with mammals that occupy open habitats (Janis, 1988; Damuth and Janis, 2011). However, the combination of HI and mesowear has often been used to evaluate the accuracy of dietary interpretations (e.g., Fortelius and Solounias, 2000; Kaiser et al., 2009; Frazer and Theodor, 2011).
Samples for the mesowear II analysis include three extant species: C. sumatraensis (n = 22), N. griseus (n = 12), and N. goral (n = 5). Pleistocene specimens of the same taxa: C. sumatraensis (n = 19), N. griseus (n = 22), and N. goral (n = 22) were analyzed. The results obtained from mesowear II were compared with Kaiser et al. (2013)’s datasets including 26 species of browsers, 20 species of grazers, and 29 species of mixed feeders (see Supplementary material 3 for the full list of name and the number of extant specimens used in this study).
The OR and cusp(id) shape (CS), which were observed on the cutting edge of either the buccal cusp of an upper second molar or the lingual cuspid of a lower second molar, were used for mesowear analysis. Both anterior and posterior cusp(id)s were examined and only the sharper one was scored (Fortelius and Solounias, 2000). Unworn teeth, heavily worn teeth, and those with broken cusp(id)s were excluded from the analysis. The OR was classified as high or low, depending on how high the cusp(id)s uplift from the valley between them (Merceron et al., 2007; Blondel et al., 2010) (Figure 2). The obtained value was calculated using the ImageJ program (v. 1.51k). The CS was recorded by characterizing the cusp(id) apices as sharp, round, or blunt, using a 10 × magnification hand lens (Figure 2). The OR and CS were combined into a single parameter, so-called the MS (Kaiser et al., 2009). To investigate diets based on the MS, five different levels ranging from 0 to 4 have so far been recognized (Kaiser et al., 2009). A score of 0 is defined as the most attrition-dominated wear commonly found in leaf browsers, whereas a score of 4 reflects the most abrasion-dominated signal associated with grazing behavior (Table 2). The MS of each species was calculated based on the average values of all dental individuals (Kaiser et al., 2013).
Figure 2. Criteria of occlusal reliefs (OR) and cusp shapes (CS) for caprine teeth based on the mesowear II method.
Table 2. Interpretations of mesowear scores following Kaiser et al. (2009).
The MS is more related to intrinsic factors and dietary feeding habits than environmental conditions (Kaiser et al., 2009). According to the dataset of Kaiser et al. (2013), browsers have a low value of MS, while grazers have a high value of MS.
Caprine specimens for DMTA include extant C. sumatraensis (n = 15), N. griseus (n = 3), and N. goral (n = 3) as well as Pleistocene C. sumatraensis (n = 16), N. griseus (n = 22), and N. goral (n = 10). In complement with extant species of serows (Capricornis) and gorals (Naemorhedus), we also include a comparative dataset of 119 specimens belonging to three extant species of ruminants with known differences in feeding habits for our DMTA. These comparative specimens include a semi-wild cattle Bos taurus (n = 44), moose Alces alces (n = 50), and European bison Bison bonasus (n = 25). The semi-wild cattle from Camargue, Rhone delta, France, have dental microwear textures supporting the dominance of a grazing habit, whereas the moose from Biebrza in Poland is a browser (Merceron et al., 2021). The European bison from Białowieza in Poland is identified as having a mixed feeder pattern (Borowski and Kossak, 1972; Bowyer et al., 2003; Kowalczyk et al., 2011). A full list of name and the number of extant specimens used for DMTA is given in Supplementary material 3.
Dental microwear analysis was preferentially performed on unbroken upper second molars, mesio-buccal facets of protocones; or metaconules if the former is broken or altered, or lower second molars, disto-buccal facets of protoconids; or hypoconids if the protoconid is broken or altered (Ramdarshan et al., 2017). Teeth were cleaned with acetone and molds were made using polyvinylsiloxane (Coltène/Whaledent AG, Altstätten, Switzerland, ref 6015-ISO, Regular Body President, medium consistency). The silicone casts were cut and scanned with “TRIDENT,” a Leica DCM8 white confocal stereoscope with the 100 × objective lens (numerical aperture = 0.90, working distance = 0.90 mm) at the laboratory of PALEVOPRIM, University of Poitiers, France. The surfaces of 200 × 200 micrometers were extracted from the occlusal surfaces and aberrant peaks erased, using a template shown on the Leica Map software version 7.4.8737 (Figure 3), following the protocol in Merceron et al. (2016). Dental surfaces were analyzed through the SSFA using the Sfdx version 1.0.11 and Toothfrax version 1.0 beta 1.7 software (Scott et al., 2006). According to Scott et al. (2006), four microwear texture variables were extracted from dental surfaces: complexity (Asfc), anisotropy (epLsar), heterogeneity (HAsfc), and textural fill volume (Tfv) (Figure 4; Table 4).
Figure 3. Dental microwear textures from the second molars of (A) extant C. sumatraensis, (B) Pleistocene C. sumatraensis, (C) extant N. griseus, (D) Pleistocene N. griseus, (E) extant N. goral, and (F) Pleistocene N. goral. Scale bars = 50 μm.
Figure 4. Schematic illustration of microwear surfaces showing high and low values for four variables including complex textures, anisotropic textures, heterogeneous textures, and textural fill volume [modified from Scott et al. (2006) and Merceron et al. (2014)].
To investigate dietary differences between extinct and extant caprines, we ran an analysis of variance (ANOVA) (after box-cox transformation), using SPSS version 22.0, together with the three reference species as mentioned above. Post-hoc tests, run after ANOVA, were done using Tukey’s honest significant difference (HSD) and Fisher’s least significant difference (LSD) (Bonferroni adjustment) tests to balance the type I and II errors (Cook and Farewell, 1996) (Table 5). Browsers usually have higher complexity and textural fill volume values, resulting from their consumption of hard objects (e.g., lignin-bearing woody tissues such as twigs, shoots, bark but also fruits with seeds) (Scott et al., 2006; Scott, 2012). Grazers mainly feed on herbaceous monocotyledons (mostly grasses, sedges and rushes), which contain higher silica phytolith content than dicots (Scott et al., 2006; Scott, 2012). The shearing of this foliage results in higher anisotropy and lower complexity for grazing species, while the crushing cation on hard food objects seen in browsers is considered to generate complex and isotropic textures on enamel surfaces (Scott et al., 2006; Scott, 2012).
However, it is noteworthy that leaf-browsing species may have a combination of low anisotropy with low to intermediate complexity such as Giraffa camelopardalis or A. alces or high anisotropy with low to intermediate complexity such as Capreolus capreolus (Merceron et al., 2018, 2021). Analyzing the heterogeneity of complexity reflects a large diversity in feeding resources. Hence, leaf browsers or grazers would have lower heterogeneity than mixed feeders or browsers with a wide spectrum of food items and various mechanical properties (Scott et al., 2006; Scott, 2012).
The Pleistocene C. sumatraensis has the lowest HI of 2.77 and the lowest MS of 0.89 (Figure 5). The Pleistocene N. goral has the highest HI of 3.95 and the Pleistocene N. griseus displayed the highest MS of 1.50. The dental morphology of C. sumatraensis that has lower molar crowns than other two goral species possibly suggests its dietary adaptation for more browsing habits.
Figure 5. A bivariate plot of hypsodonty index (HI) against mesowear score (MS). The comparative dataset of extant ungulates is derived from Kaiser et al. (2013). Cs, extant C. sumatraensis, Ngr, extant N. griseus, Ngo, extant N. goral, CsP, Pleistocene C. sumatraensis, NgrP, Pleistocene N. griseus, and NgoP, Pleistocene N. goral.
In extant populations, C. sumatraensis has the lowest MS of 0.54 and N. goral shows the highest MS of 1.40 (Table 3). Both HI and MS have shown that the Pleistocene and extant C. sumatraensis falls within a 95% confidence ellipse for browsers, while Naemorhedus is out of it. All analyzed caprine taxa tend to be assembled in a 95% confidence ellipse for mixed feeders, overlapping with grazers for N. griseus and N. goral (Figure 5). The degree of hypsodonty and mesowear-II results has shown the trend that the Pleistocene Southeast Asian caprines preferentially occupied open habitats, although C. sumatraensis has the lower HI and MS than the other two goral species. This probably reflects less grit digestion from the surrounding areas or living in a more closed habitat than Naemorhedus.
Table 3. Hypsodonty index (HI) for species and mesowear score (MS) of extant and Pleistocene caprines.
Table 4. Descriptive statistics (mean and standard error, SE) of microwear textural parameters for Pleistocene and extant caprines and reference species.
Descriptive statistics of fossil and extant caprines and post-hoc tests using the ANOVA are shown in Tables 4, 5, respectively. According to the results of ANOVA, complexity, anisotropy, and heterogeneity of complexity have showed significant differences between species (with p < 0.001, p < 0.001, and p = 0.027, respectively). The browsing moose A. alces has the highest complexity of 4.02 and the lowest anisotropy of 2.36 × 10–3. Conversely, the semi-wild grazing B. taurus has the lowest complexity of 1.57 and the highest anisotropy of 5.17 × 10–3. The intermediate values of complexity and anisotropy are found in extant B. bonasus. The heterogeneity of complexity between extant B. taurus and B. bonasus displays a similar range of values, whereas A. alces has the highest value of 0.73. The textural fill volume of B. taurus shows the highest value, followed by B. bonasus and A. alces (Tables 4, 5 and see Supplementary material 4 for both HSD and LSD tests including extinct and extant species). In comparison with the comparative species, the extant C. sumatraensis showed a significantly lower anisotropy than B. taurus (Tables 4, 5). Both extant Naemorhedus species had a higher anisotropy than A. alces and B. bonasus, although a significant difference was found only in N. goral. The Pleistocene C. sumatraensis and N. griseus have significantly lower anisotropy, compared to those of extant B. taurus. The complexity of Pleistocene N. griseus is significantly higher than that of B. taurus and B. bonasus.
The DMTA has provided more detailed information for caprine diets in shorter temporal extent. Three extant Eurasian ruminant species whose dietary differences are recognized serve us as a standard comparison for the extinct species and some extant taxa. The high complexity and low anisotropy of extant A. alces are characteristic of browsing species, which include woody lignin-enriched elements in their diet (Berlioz et al., 2022). Conversely, the grazing B. taurus shows low complexity and high anisotropy, similar to several other African and Eurasian grazers (Ungar et al., 2007; Schulz et al., 2010; Scott, 2012; Berlioz et al., 2017). The anisotropy is related to the folivory, notably the ingestion of tough foliages requiring high slicing motion (Scott et al., 2006; Scott, 2012). When associated with low complexity, this evidence indicates the consumption of herbaceous silica-bearing monocots and the absence of woody material rich in lignin, which requires crushing to extract energy from cell content (Scott, 2012; Merceron et al., 2016). The intermediate values of complexity and anisotropy present in B. bonasus represent mixed feeding habits.
The extant C. sumatraensis has a browsing habit, as indicated by low complexity and anisotropy (Table 4). The extant N. goral shows a grazing signal based on microwear results but the low sample size prevents us to make definitive judgments. In any case, these dietary reconstructions are congruent with other existing ecological evidence. Although the dental microwear textures of extant N. griseus (n = 3) show a similar pattern of feeding habits with N. goral, the highest value of complexity and textural fill volume may represent more browsing habits for the former (Table 4). The small sample sizes of extant gorals for DMTA (n = 3 per species) should be taken into consideration and may require additional analyses.
The Pleistocene C. sumatraensis and N. griseus have significantly lower anisotropy than the extant B. taurus (Table 5), whereas the Pleistocene N. goral has slightly higher anisotropy than the extant A. alces and B. bonasus (Table 4). We infer that the Pleistocene C. sumatraensis and N. griseus likely fed on fewer herbaceous monocotyledons than N. goral. The Pleistocene C. sumatraensis has the lowest complexity, compared to other Pleistocene species (Table 4). In addition, the Pleistocene N. griseus has significantly higher complexity than the extant A. alces (Table 4), indicating the consumption of hard objects for the former (Ramdarshan et al., 2016). The Pleistocene N. griseus might have chiefly fed on harder food items such as seeds and lignified plant tissues, which require highly efficient crushing actions. However, the Pleistocene C. sumatraensis and two goral species were likely to be mixed feeders, but C. sumatraensis tended to feed on softer objects.
Regarding the previous studies of extant caprine diets, C. sumatraensis in Thailand has high intakes of dicotyledons, especially soft shoots of trees, as exemplified by analyses of feces and stomach contents as well as by field observations, notably through bite marks on plants (Chairat, 2007; Junshum et al., 2010; Nakasathien, 2017). In contrast, N. griseus in Thailand (previously assigned to N. goral) mainly feeds on a higher percentage of monocotyledon grasses than C. sumatraensis does (Chaiyarat et al., 1999), suggesting mixed feeding habits for the former. Other dietary observations on the living caprine populations from northern Thailand have indicated that N. griseus feeds on fallen dry leaves and grasses (Nimitsin, 2012). N. goral from Kashmir, India feeds more frequently on herbaceous monocotyledons (grasses, sedges, rushes) than on dicotyledon shrubs and trees (Junaid et al., 2012), like N. griseus in Thailand. Moreover, N. goral from Binsar Wildlife Sanctuary in India contains the high ratio of grasses in feces (Ilyas and Khan, 2004), implying a grass-dominated mixed feeding habit.
The HI and mesowear II results have shown mixed feeding habits for both extant and Pleistocene caprines, congruent with the HI and mesowear I interpretations mentioned by Isarankura Na Ayudhya et al. (2021). For the extant populations of Capricornis and Naemorhedus, our results based on the HI and MS are congruent with several previous studies on dietary interpretations of caprines (e.g., Ilyas and Khan, 2004; Chairat, 2007, Nimitsin, 2012). The results showed two possibilities of dietary interpretations in Pleistocene and extant C. sumatraensis, either mixed-feeding or browsing habits.
Dietary interpretations based on the DMTA results probably reflect inter- and intraspecific competition among the Pleistocene caprine populations. Based on the Tukey’s HSD tests, there are no significant differences in microwear variables between the Pleistocene serows and gorals (Table 5), suggesting dietary overlaps among sympatric populations, at least in terms of food properties. When focusing on the less conservative Fisher’s LSD tests, the Pleistocene N. griseus from Tham Lod Rockshelter shows significantly higher complexity and heterogeneity than the other Pleistocene C. sumatraensis in Thailand (Tables 4, 5), reflecting more browsing habits for the former. Both Pleistocene and extant N. griseus has also the highest heterogeneity, compared to other analyzed caprines in this study (Tables 4, 5), indicating more browsing habits. Small differences in dietary investigations using DMTA suggest that three Pleistocene caprine species might have shared food resources if they had coexisted.
However, as seen in their extant populations from western Sichuan in China, C. sumatraensis occupies steep slopes with high shrub density, while N. griseus tends to dwell in a high-altitude meadow (Chen et al., 2009). Although extant C. sumatraensis and N. griseus may have different habitat preferences, the interspecific competition in habitat use between both taxa is observed from the mountainous areas of Chiang Mai, northern Thailand (Chaiyarat et al., 1999; Nakasathien, 2017). The larger-sized and aggressive C. sumatraensis usually forces N. griseus out of coexisting areas (Chaiyarat et al., 1999; Nakasathien, 2017). For instance, these authors mentioned that one to six serow individuals that form herd (Nimitsin, 2012; Nakasathien, 2017) usually overcome a single or couple of N. griseus and successfully occupy the coexistence areas. The competition of natural resources between two extant caprine species might have led to the decreasing populations of a smaller-sized goral N. griseus. In contrast, studies on temporal activity patterns observed in extant caprine communities have revealed that gorals are likely to be active at the beginning of daytime from dawn to late morning, while serows are energetic after late afternoon to night (Bhattacharya et al., 2012; Lovari et al., 2020). It is presumable that although the dental microwear textures suggest small differences in dietary preferences between Capricornis (soft foliage) and Naemorhedus (harder food object), niche partitioning might have occurred in other different ways.
As the degree of HI combined with mesowear II results showed similarities in feeding habits between these three Pleistocene and extant caprine taxa (Table 6), comparisons between stable carbon isotope data and DMTA provide more detailed ecological interpretations of each species through time.
Table 6. Summary of dietary interpretations of Pleistocene and extant caprines based on dental wear pattern and stable carbon isotope studies.
The Pleistocene C. sumatraensis had enamel δ13C results congruent with the DMTA analysis. The δ13C values of tooth enamel of C. sumatraensis from the late middle Pleistocene of Tham Wiman Nakin [range: −12.8‰ to +1.7‰ and median: −11.0‰; Suraprasit et al. (2020)] and from the late middle Pleistocene of Khok Sung [range: −15.1‰ to −11.8‰ and median: −13.9‰; Suraprasit et al. (2018)] suggested that this species was a pure C3 feeder and probably seasonally fed on C4 plants. During the late Pleistocene, stable carbon isotopes in tooth enamel of C. sumatraensis from Tham Lod Rockshelter in northern Thailand [range: −14.3‰ to +1.9‰ and median: −12.2‰; Suraprasit et al. (2021b)], from Boh Dambang in Cambodia [range: −13.2‰ to +1.6‰ and median: −11.5‰; Bacon et al. (2018b)], and Nam Lot in northern Laos (range: −14.6‰ to −13.0‰ and median: −13.8‰; Bacon et al., 2018a) suggested similar patterns in feeding habits between the middle and late Pleistocene C. sumatraensis. All the isotope evidence has implied that the Pleistocene C. sumatraensis mainly fed on C3 plants and occasionally on C4 plants in open canopy areas. The DMTA results of Pleistocene C. sumatraensis are congruent with the stable carbon isotope data. The lowest of complexity and heterogeneity of Pleistocene C. sumatraensis, compared to the other two gorals (Table 4), supported its feeding habit on soft plants with low heterogeneity of diets.
All the recent evidence (stable carbon isotopes, DMTA, and field observations) has suggested browsing habits for extant C. sumatraensis (e.g., Chairat, 2007; Junshum et al., 2010; Nakasathien, 2017; Faiznur et al., 2020; Suraprasit et al., 2020). There are pure C3 signals of tooth enamel δ13C values of extant C. sumatraensis populations from Thailand (−17.8‰ to −8.8‰ and median: −15.9‰; Suraprasit et al., 2020) and from Sumatra (range: −16.6‰ to −12.3‰ and median: −14.1‰; Pushkina et al., 2010; Suraprasit et al., 2020). The DMTA analysis showed that the extant C. sumatraensis is a soft leaf-dominated browser and has intermediate heterogeneity of diets, as deduced from the lowest complexity and heterogeneity compared with other extant goral species (Table 4). The feeding habits of extant C. sumatraensis based on the DMTA and stable carbon isotope interpretations are relatively similar to those of Pleistocene populations (Table 6). Evidence from field observations such as nibble traces and feces (Chairat, 2007; Junshum et al., 2010; Nakasathien, 2017; Faiznur et al., 2020) supports the browsing habit in a closed forest for C. sumatraensis, congruent with the DMTA results in this study. Both of Pleistocene and extant C. sumatraensis might have had similar dietary feeding habits in browsing on soft foliage. However, the Pleistocene C. sumatraensis might have occupied a wider range of habitats than the extant one and have seasonally or occasionally fed on C4 grasses when open canopies were available.
The δ13C of tooth enamel of N. griseus from the late Pleistocene of Tham Lod Rockshelter (range: −7.1‰ to +1.9‰ and median: −0.4‰) suggested the consumption of either mixed C3/C4 and pure C4 plants in open mixed habitats (Suraprasit et al., 2020). The DMTA results of Pleistocene N. griseus had the highest complexity and heterogeneity compared to other Pleistocene caprine species (Table 4), indicating a mixed feeding habit with several dietary types of hard food items. During the Pleistocene, N. griseus tended to feed on more various and tougher plants and lived in a more open habitat canopy than C. sumatraensis.
In extant populations, one stable carbon isotope sample of N. griseus was only analyzed (−3.3‰) and fell within the range of a mixed C3/C4 feeder (Suraprasit et al., 2020). The highest complexity and heterogeneity of three extant N. griseus individuals thus provide further information regarding the possibility of a tough plant-dominated mixed feeder for the species (Table 4). Moreover, the DMTA results suggested that the Pleistocene N. griseus probably lived in more open habitats than the extant populations, although additional samples are required to confirm our interpretation.
The enamel δ13C values of N. goral from the middle Pleistocene of Pha Bong (range: −2.6‰ to +3.0‰ and median: −0.5‰), from the late middle Pleistocene of Tham Wiman Nakin (range −3.5‰ to +0.4‰ and median: −1.2‰), and from the late Pleistocene of Tham Lod Rockshelter (range: −5.3‰ to −0.1‰ and median: −3.3‰) implied a C4-dominated grazer living in open grasslands (with sometimes a small amount of C3 plants in diet) (Bocherens et al., 2017; Suraprasit et al., 2020). The DMTA results showed that the Pleistocene N. goral had high anisotropy with intermediate complexity and heterogeneity compared to the other two contemporaneous caprine taxa (Table 4). This probably indicates that the Pleistocene N. goral was a C4-dominated grazer with intermediate heterogeneity of diets occupying an open habitat. Based on the dental microwear textures, our analysis has shown that extant N. goral has a mixture of plant-based diets with several food types, similar to the Pleistocene populations. The apparent discrepancy between stable carbon isotopes and DMTA might be the consequences of the consumption of fresh growing non-abrasive grasses with ingestion of grits or browsing behavior in response to local environmental conditions that occurred few weeks before death. However, several months to a year after the amelogenesis process could have had a chance to record such behavior in the stable carbon isotope composition of enamel prisms.
The extant populations of N. goral from Himalaya in Nepal and from western China (range: −15.2‰ to −6.0‰ and median: −12.2‰) suggested the consumption of pure C3 (possibly C3 grasses) or mixed C3 and C4 plants with the habitat preference of a closed canopy (Suraprasit et al., 2020). The DMTA results of extant N. goral are congruent with the interpretation obtained from the stable carbon isotope records (Table 6). The highest anisotropy and the lowest complexity of extant N. goral indicate a grass feeding habit. Taking into account the low sample size, the extant populations of N. goral possibly feed on a higher amount of C3 grasses or various food types and/or lived in more closed habitats than the Pleistocene ones.
The DMTA results have suggested that the dietary preferences for these three caprine species might have not changed much over the Pleistocene. According to the location of paleontological and archeological sites where the caprine fossils were analyzed in this study (Figure 1), there is no discrepancy in dietary preferences observed among the Pleistocene populations across the latitudinal gradient. The Pleistocene caprines in the coexistence areas possessed mixed feeding behavior, although probably having differences in physical characteristics of foods (e.g., hardness). Isotopic, botanical, and faunal evidence revealed that, during the Pleistocene, mainland Southeast Asia was covered by forest-grassland mosaics with a variety of plant resources available for both grazers and browsers (e.g., Chaimanee, 1998; Pushkina et al., 2010; White et al., 2004; Bacon et al., 2018a,b; Yang and Grote, 2018; Suraprasit et al., 2018, 2019, 2020, 2021a,b). During the Holocene, wetter and warmer conditions have contributed to the spread of rainforests and the dominance of C3 plants in relation to the decline of C4 grassland landscapes (Kershaw et al., 2001; White et al., 2004; Morley, 2012; Louys and Robert, 2020; Suraprasit et al., 2020, 2021b).
Our dental microwear texture results supported the idea that the mosaic habitats of closed forests and open grasslands were present in Thailand during the Pleistocene. Differences in climatic and environmental conditions between the Pleistocene and Holocene have resulted in the ecological adaptation of grass-feeding mammals such as caprines, as it is the case for C. sumatraensis. However, we suggest that the anthropogenic activities and especially the agricultural development have had severe impacts on species distribution, spatial behavior, and home ranges of Southeast Asian caprines. Thus, the decreasing number of these present-day caprine populations should be taken into concern for the future conservation plans and management.
This study provides insights into diets and feeding habits of Pleistocene and extant caprines in Southeast Asia based on the HI, mesowear II method, and DMTA. The first two approaches have indicated similar dietary preferences and feeding habits between Pleistocene and extant caprine populations, with notice of browsing signals in C. sumatraensis. Although the DMTA pointed out that each caprine species has some differences in dietary preferences (food hardness), this approach fails to clearly discriminate the feeding habits between Pleistocene and extant caprine populations. The Pleistocene and extant C. sumatraensis has a soft-plant browsing habit, while both goral species are mixed feeders (but N. griseus preferring harder food items than the other two species). Previous studies including the stomach content, mesowear I, and stable carbon isotope analyses, together with our DMTA have suggested that mosaic habitats of closed forests and open grasslands were widespread in Thailand during the Pleistocene, but became more limited and were replaced by the expansion of rainforests during the Holocene. This study advocates that the combination of applying HI with the mesowear II methods, stable carbon approaches, and DMTA to the paleoecological study of past and living caprine populations through time is useful for proposing their habitat conservation plans and this aspect should be further extended to other Southeast Asian endangered mammal species in the future.
The original contributions presented in this study are included in the article/Supplementary material, further inquiries can be directed to the corresponding author.
The animal study was reviewed and approved by Department of National Parks, Wildlife and Plant Conservation of Thailand (DNP).
JI, GM, TW, and KS conceived the research project, raised funds, and wrote the manuscript. J-JJ, YC, and RS conducted the field excavations and provided the fossil samples. JI, GM, and KS performed and directed the sampling, preparation, and analysis of the material. JI and KS were principal investigators for the project. All authors discussed the results and commented on the manuscript.
This project was funded by the 90th Anniversary of Chulalongkorn University Scholarship and the Overseas Research Experience Scholarship for Graduate Student, Graduate School, Chulalongkorn University.
We would like to thank the Department of National Parks, Wildlife and Plant Conservation of Thailand (DNP) and to the Department of Mineral Resources (DMR) for giving us access and permissions to the specimens. We also extend our thanks to Athiwat Wattanapituksakul for providing helpful information on archeological sites in Pang Mapha and for all facilities during our sample collection process. We further thank curators and members from institutes and museums: Chulalongkorn University Museum of Natural History (CUMNH), Zoological Museum of Kasetsart University (ZMKU), Forestry Museum of Kasetsart University (FMKU), National Science Museum of Thailand (NSM), and Zoologische Staatssammlung München (ZSM), for permitting us access to extant caprine specimens and for facilitating our sample collection. We are grateful to all members of the PALEVOPRIM laboratory (CNRS and University of Poitiers, France) for training JI at the University of Poitiers. We appreciate the feedback and valuable comments from the editor and reviewers, which indeed helped us to improve the quality of the manuscript.
The authors declare that the research was conducted in the absence of any commercial or financial relationships that could be construed as a potential conflict of interest.
All claims expressed in this article are solely those of the authors and do not necessarily represent those of their affiliated organizations, or those of the publisher, the editors and the reviewers. Any product that may be evaluated in this article, or claim that may be made by its manufacturer, is not guaranteed or endorsed by the publisher.
The Supplementary Material for this article can be found online at: https://www.frontiersin.org/articles/10.3389/fevo.2022.1000168/full#supplementary-material
Bacon, A. M., Bourgon, N., Dufour, E., Zanolli, C., Duringer, P., and Ponche, J. L. (2018a). Nam Lot (MIS 5) and Duoi U’Oi (MIS 4) Southeast Asian sites revisited: Zooarchaeological and isotopic evidences. Palaeogeogr. Palaeoclimatol. Palaeoecol. 512, 132–144. doi: 10.1016/j.palaeo.2018.03.034
Bacon, A. M., Duringer, P., Westaway, K., Joannes-Boyau, R., Zhao, J., and Bourgon, N. (2018b). Testing the savannah corridor hypothesis during MIS2: The Boh Dambang hyena site in Southern Cambodia. Quat. Int. 464, 417–439. doi: 10.1016/j.quaint.2017.10.047
Baker, G., Jones, L. H. P., and Wardrop, I. D. (1959). Cause of wear in sheeps’ teeth. Nature 184, 1583–1584. doi: 10.1038/1841583b0
Barron-Ortiz, C. R., Theodor, J. M., and Arroyo-Cabrales, J. (2014). Dietary resource partitioning in the Late Pleistocene horses from Cedral, north-central Mexico: Evidence from the study of dental wear. Rev. Mex. Cienc. Geol. 31, 260–269.
Berlioz, E., Concepción, A., Cecile, B., María, S. T. R., and Gildas, M. (2017). Deer in an arid habitat: Dental microwear textures track feeding adaptability. Hystrix 28, 222–230.
Berlioz, E., Leduc, C., Hofman-Kamińska, E., Bignon-Lau, O., Kowalczyk, R., and Merceron, G. (2022). Dental microwear foraging ecology of a large browsing ruminant in Northern Hemisphere: The European moose (Alces alces). Palaeogeogr. Palaeoclimatol. Palaeoecol. 586:110754. doi: 10.1016/j.palaeo.2021.110754
Bhattacharya, T., Bashir, T., Poudyal, K., Sathyakumar, S., and Saha, G.K. (2012). Distribution, occupancy and activity patterns of goral (Nemorhaedus goral) and serow (Capricornis thar) in Khangchendzonga Biosphere Reserve, Sikkim, India. Mamm. Study 37, 173–181. doi: 10.3106/041.037.0302
Blondel, C., Merceron, G., Andossa, L., Taisso, M. H., Vignaud, P., and Brunet, M. (2010). Dental mesowear analysis of the late Miocene Bovidae from Toros- Menalla (Chad) and early hominid habitats in Central Africa. Palaeogeogr. Palaeoclimatol. Palaeoecol. 292, 184–191. doi: 10.1016/j.palaeo.2010.03.042
Bocherens, H., Schrenk, F., Chaimanee, Y., Kullmer, O., Morike, D., Pushkina, D., et al. (2017). Flexibility of diet and habitat in Pleistocene South Asian mammals: Implications for the fate of the giant fossil ape Gigantopithecus. Quat. Int. 434, 148–155. doi: 10.1016/j.quaint.2015.11.059
Borowski, S., and Kossak, S. (1972). The natural food preferences of the European bison in seasons free of snow cover. Acta Theriol. 17, 151–169. doi: 10.4098/AT.arch.72-13
Bover, P., Llamas, B., Mitchell, K. J., Thomson, V. A., Alcover, J. A., Lalueza-Fox, C., et al. (2019). Unraveling the phylogenetic relationships of the extinct bovid Myotragus balearicus Bate 1909 from the Balearic Islands. Quat. Int. 215, 185–195. doi: 10.1016/j.quascirev.2019.05.005
Bowyer, R. T., Van Ballengerghe, V., and Kie, J. G. (2003). Wild Mammals of North America: Biology, Management, and Conservation. Baltimore: The Johns Hopkins University Press, 931–964.
Buranapim, N., Sitasuwan, N., Kongprempoon, A., Korkusol, K., Siriaroonrat, B., and Kamolnorranath, S. (2014). Reintroduction and behavioral observations of Chinese gorals (Naemorhedus griseus) in natural conditions. Thai J. Vet. Med. 44, 75–83
Calandra, I., and Merceron, G. (2016). Dental microwear texture analysis in mammalian ecology. Mamm. Rev. 46, 215–228. doi: 10.1111/mam.12063
Cerling, T. E., and Harris, J. M. (1999). Carbon isotope fractionation between diet and bioapatite in ungulate mammals and implications for ecological and paleoecological studies. Oecologia 120, 347–363. doi: 10.1007/s004420050868
Chaimanee, Y. (1998). Plio-Pleistocene Rodents of Thailand. Thai Studies in Biodiversity No. 3 J. Mammal. 81, 277–278. doi: 10.1644/1545-1542(2000)081<0277:R>2.0.CO;2
Chairat, R. (2007). Habitat and Distribution of Serow (Capricornis sumatraensis). Phukhieo –EU Project. Bangkok: Wildlife and Plant Conservation.
Chaiyarat, R., Laohajinda, W., Kutintara, U., and Nabhitabhata, J. (1999). Ecology of the goral (Naemorhedus goral) Mon Chong: Om Koi wildlife sanctuary.
Chen, W., Hu, J.C., and Lu, X. (2009). Habitat use and separation between the Chinese serow (Capricornis milneedwardsii) and the Chinese goral (Naemorhedus griseus) in winter. Mammalia 73, 249-252. doi: 10.1515/MAMM.2009.035
Cook, R. J., and Farewell, V. T. (1996). Multiplicity considerations in the design and analysis of clinical traits. J. R. Statist. Soc. Ser. A 159, 93–110. doi: 10.2307/2983471
Damuth, J., and Janis, C. (2011). On the relationship between hypsodonty and feeding ecology in ungulate mammals, and its utility in palaeoecology. Biol. Rev. 86, 733–758. doi: 10.1111/j.1469-185X.2011.00176.x
Davis, M., and Pineda-Munoz, S. (2016). The temporal scale of diet and dietary proxies. Ecol. Evol. 6, 1883–1897. doi: 10.1002/ece3.2054
DeSantis, L. R. G. (2016). Surface topography: Metrology and properties. Surf. Topogr. Metrol. Prop. 4, 1–12. doi: 10.1088/2051-672X/4/2/023002
Duckworth, J. W., and MacKinnon, J. (2008). Naemorhedus goral. The IUCN Red List of Threatened Species 2008:e.T14296A4430073. Available online at: https://dx.doi.org/10.2305/IUCN.UK.2008.RLTS.T14296A4430073.en (accessed January 04. 2018).
Duckworth, J. W., Steinmetz, R., and Chaiyarat, R. (2008). Naemorhedus griseus. The IUCN Red List of Threatened Species 2008: e.T14303A4430834. Available online at: http://dx.doi.org/10.2305/IUCN.UK.2008.RLTS.T14303A4430834.en (accessed January 04, 2018).
Duval, M., Fang, F., Suraprasit, K., Jaeger, J.-J., Benammi, M., Chaimanee, Y., et al. (2019). Direct ESR dating of the Pleistocene vertebrate assemblage from Khok Sung locality, Nakhon Ratchasima province, northeastern Thailand. Palaeontolo. Electron. 22.3.69, 1–15. doi: 10.26879/941
Edwards, E. J., and Still, C. J. (2008). Climate, phylogeny and the ecological distribution of C4 grasses. Ecol. Lett. 11, 266–276. doi: 10.1111/j.1461-0248.2007.01144.x
Ellen, R., Parkes, P., and Bickers, A. (2000). Indigeneous Environmental Knowledge and Its Transformations. London: Harwood academic publishers.
Esposito, M., Chaimanee, Y., Jaeger, J. J., and Reyss, J. L. (1998). Datation des concrétions carbonatées de la Grotte du Serpent (Thaïlande) par la méthode Th/U. C. R. Acad. Sci. Ser. IIA 326, 603–608. doi: 10.1016/S1251-8050(98)80250-4
Esposito, M., Reyss, J. L., Chaimanee, Y., and Jaeger, J. J. (2002). U-series Dating of Fossil Teeth and Carbonates from Snake Cave. Thailand. J. Archaeol. Sci. 29, 341–349. doi: 10.1006/jasc.2002.0718
Faiznur, A., Yasuda, M., Maryati, M., Adlil, I. S., and Halid, M. S. (2020). The first record of Sumatran Serow, Capricornis sumatraensis (Bovidae, Cetartiodactyla), in Gunung Ledang Johor National Park, a tropical forest remnant on the Southern Malay Peninsula. Mammal. Study 45, 259–264. doi: 10.3106/ms2020-0022
Fortelius, M., and Solounias, N. (2000). Functional characterization of ungulate molars using the abrasion-attrition wear gradient: A new method for reconstructing paleodiets. Am. Mus. Novit. 3301, 1–36. doi: 10.1206/0003-0082(2000)301<0001:FCOUMU>2.0.CO;2
Frazer, D., and Theodor, J. M. (2011). Comparing ungulate dietary proxies using discriminant function analysis. J. Morphol. 272, 1513–1526. doi: 10.1002/jmor.11001
Green, J. L., and Croft, D. A. (2018). “Using dental mesowear and microwear for dietary inference: A review of current techniques and application,” in Methods in Paleoecology, eds D. A. Croft, D. F. Su, and S. W. Simpson (Berlin: Springer), 53–74. doi: 10.1007/978-3-319-94265-0_5
Ilyas, O., and Khan, J. A. (2004). Food habits of barking deer (Muntiacus muntjak) and goral (Naemorhedus goral) in Binsar Wildlife Sanctuary. India. Mammalia 67, 521–532. doi: 10.1515/mamm-2003-0406
Isarankura Na Ayudhya, J., Wannaprasert, T., Shoocongdej, R., Chaimanee, Y., and Suraprasit, K. (2021). Palaeoecological reconstruction of serows and gorals (Bovidae: Caprinae) from the Pleistocene of Thailand using dental mesowear and hypsodonty: Implications for species conservation. Thailand Nat. Hist.Mus. J. 15, 137–146.
Jangtarwan, K., Kamsongkram, P., Subpayakom, N., Sillapaprayoon, S., Muangmai, N., and Kongphoemph, A. (2020). Predictive genetic plan for a captive population of the Chinese goral (Naemorhedus griseus) and perscriptive action for ex situ and in situ conservation management in Thailand. PLoS One. 15:e0234064. doi: 10.1371/journal.pone.0234064
Janis, C. M. (1988). An estimation of tooth volume and hypsodonty ndices in ungulate mammals, and the correlation of these factors with dietary preference. proceedings of the VII International Symposium on Dental Morphology. (Paris) 53, 367–387.
Janis, C. M. (2008). “An evolutionary history of browsing and grazing ungulates,” in the Ecology of Browsing and Grazing, eds I. J. Gordon and H. H. T. Prins (Berlin: Springer), 21–45. doi: 10.1007/978-3-540-72422-3_2
Joshi, B. D., Singh, V. K., Singh, H., Bhattacharjee, S., Singh, A., Singh, S. K., et al. (2022). Revisiting taxonomic disparities in the genus Naemorhedus: New insights from Indian Himalayan Region. Mammalia 86, 373–379. doi: 10.1515/mammalia-2021-0152
Junaid, M., Ahmad, F., Saxena, R. C., and Bansal, S. K. (2012). Botanical composition determination of goral Naemorhedus goral (Artiodactyla: Bovidae): Goral rescue centre. Pahalgam, Jammu & Kashmir, India. Eur. Zool. J. 1, 99–104.
Junshum, P., Joomwong, A., and Kamtubtim, S. (2010). A Survey and Estimate Density of Serow in Khao Somphot Non-Hunting Area, Lopburi Province. Thale Chup Son: Thepsatri Rajabhat University.
Kaiser, T. M., Brasch, J., Castell, J. C., Schulz, E., and Clauss, M. (2009). Tooth wear in captive wild ruminant species differs from that of free-ranging conspecifics. Mammal. Biol. 74, 425–427. doi: 10.1016/j.mambio.2008.09.003
Kaiser, T. M., Muller, D. W. H., Fortelius, M., Schulz, E., Codron, D., and Clauss, M. (2013). Hypsodonty and tooth facet development in relation to diet and habitat in herbivorous ungulates: Implications for understanding tooth wear. Mammal. Rev. 43, 34–46. doi: 10.1111/j.1365-2907.2011.00203.x
Kershaw, A. P., Penny, D., van der Kaars, S., Anshari, G., and Thamotherampillai, A. (2001). “Vegetation and climate in lowland Southeast Asia at the Last Glacial Maximum,” in Faunal and Floral Migrations and Evolution in SE Asia–Australasia, eds I. Metcalfe, M. B. S. Jeremy, M. Morwood, and I. Davidson (Lisse: Balkema), 227–236.
Kowalczyk, R., Taberlet, P., Coissac, E., Valentini, A., Miquel, C., Kaminski, T., et al. (2011). Influence of management practices on large herbivore diet – case of European bison in Bialowwieza Primeval Forest (Poland). For. Ecol. Manag. 261, 821–828. doi: 10.1016/j.foreco.2010.11.026
Lekagul, B., and McNeely, J. A. (1988). Mammals of Thailand. Bangkok: Association for the Conservation of Wildlife.
Louys, J., and Robert, P. (2020). Environmental drivers of megafauna and hominin extinction in Southeast Asia. Nature 586, 402–406. doi: 10.1038/s41586-020-2810-y
Lovari, S., Mori, E., and Procaccio, E. L. (2020). On the Behavioural Biology of the Mainland Serow: A Comparative Study. Animals 10:1669. doi: 10.3390/ani10091669
Marwick, B., and Gagan, M. K. (2011). Late Pleistocene monsoon variability in northwest Thailand: An oxygen isotope sequence from the bivalve Margaritanopsis laosensis excavated in Mae Hong Son province. Quat. Sci. Rev. 30, 3088–3098. doi: 10.1016/j.quascirev.2011.07.007
Merceron, G., Berlioz, E., Vonhof, H., Green, D., Garel, M., and Tutken, T. (2021). Tooth tales told by dental diet proxies: An alpine community of sympatric ruminants as a medel to decipher the ecology of fossil fauna. Palaeogeogr. Palaeoclimatol. Palaeoecol. 562, 1–14. doi: 10.1016/j.palaeo.2020.110077
Merceron, G., Colyn, M., and Geraads, D. (2018). Browsing and non-browsing extant and extinct giraffids: Evidence from dental microwear textural analysis. Palaeogeogr. Palaeoclimatol. Palaeoecol. 505, 128–139. doi: 10.1016/j.palaeo.2018.05.036
Merceron, G., Ellen, S., Laszl, K., and Thomas, M. K. (2007). Paleoenvironment of Dryopithecus brancoi at Rudabanya, Hungary: Evidence from dental meso- and micro-wear analyses of large vegetarian mammals. J. Hum. Evol. 53, 331–349. doi: 10.1016/j.jhevol.2007.04.008
Merceron, G., Hofman-Kaminska, E., and Kowalczyk, R. (2014). 3D dental microwear texture analysis of feeding habits of sympatric ruminants in the Bialowieza Primeval Forest. Poland. For. Ecol. Manag. 328, 262–269. doi: 10.1016/j.foreco.2014.05.041
Merceron, G., Ramdarshan, A., Francisco, A., Gautier, D., Boisserie, J., Milhet, X., et al. (2016). Untangling the environmental from the dietary: Dust does not matter. Proc. R. Soc. B. 283:20161032. doi: 10.1098/rspb.2016.1032
Mihlbachler, M. C., Campbell, D., Chen, C., Ayoub, M., and Kaur, P. (2018). Microwear-mesowear congruence and mortality bias in rhinoceros mass-death assemblages. Paleobiology 44, 131–154. doi: 10.1017/pab.2017.13
Mihlbachler, M. C., Rivals, F., Solounias, N., and Semprebon, G. M. (2010). Dietary change and evolution of horses in North America. Science 331, 1178–1181. doi: 10.1126/science.1196166
Mori, E., Nerva, L., and Lovari, S. (2019). Reclassification of the serows and gorals: The end of a neverending story? Mamm. Rev. 49, 256–262. doi: 10.1111/mam.12154
Morley, R. J. (2012). A review of the Cenozoic palaeoclimate history of Southeast Asia. Biotic evolution and environmental change in Southeast Asia. doi: 10.1017/CBO9780511735882.006
Nakasathien, S. (2017). the Serow in Thailand: Distribution, Habitats and Some Behaviors. Nonthaburi: Seub Nakhasathien foundation.
Nimitsin, C. (2012). Behavior of goral (Naemorhedus griseus) in semi-natural condition at Omkoi Wildlife Breeding Center. Ph. D thesis. Chiang Mai: Chiang Mai University.
Phan, T. D., Nijhawan, S., Li, S., and Xiao, L. (2020). Capricornis sumatraensis. The IUCN Red List of Threatened Species 2020: e.T162916735A162916910. Available online at: https://dx.doi.org/10.2305/IUCN.UK.2020-2.RLTS.T162916735A162916910.en (accessed February 18, 2020)
Pushkina, D., Bocherens, H., Chaimanee, Y., and Jaeger, J. J. (2010). Stable carbon isotope reconstuctions of diet and paleoenvironment from the late Middle Pleistocene snake cave in Northeastern Thailand. Naturwissenschaften 97, 299–309. doi: 10.1007/s00114-009-0642-6
Ramdarshan, A., Blondel, C., Brunetiere, N., Francisco, A., Gautier, D., Surault, J., et al. (2016). Seeds, browse, and tooth wear: A sheep perspective. Ecol. Evol. 6, 5559–5569. doi: 10.1002/ece3.2241
Ramdarshan, A., Cécile, B., Denis, G., Jérôme, S., and Merceron, G. (2017). Overcoming sampling issues in dental tribology: Insights from an experimentation on sheep. Palaeontol. Electron 20, 1-19. doi: 10.26879/762
Rivals, F., and Semprebon, G. M. (2006). A comparison of the dietary habits of a large sample of the Pleistocene pronghorn Stockoceros onusrosagris from the Papago Springs Cave in Arizona to the extant Antilocapra americana. J. Vertebr. Paleontol. 26, 495–500. doi: 10.1671/0272-4634(2006)26[495:ACOTDH]2.0.CO;2
Rivals, F., Solounias, N., and Schaller, G. B. (2011). Diet of Mongolian gazelles and Tibetan antelopes from steppe habitats using premaxillary shape, tooth mesowear and microwear analyses. Mamm. Biol. 76, 358–364. doi: 10.1016/j.mambio.2011.01.005
Safoowong, M. (2015). Population, Distribution and Habitat of Goral in Protected area. Wildlife Yearbook 15, 167–185.
Sage, R. F. (2004). The evolution of C4 photosynthesis. New Phytol. 161, 341–370. doi: 10.1111/j.1469-8137.2004.00974.x
Schulz, E., Calandra, I., and Kaiser, T. M. (2010). Applying tribology to teeth of hoofed mammals. Scanning 32, 162–182. doi: 10.1002/sca.20181
Scott, J. R. (2012). Dental microwear texture analysis of extant African Bovidae. Mammalia 76, 157–174. doi: 10.1515/mammalia-2011-0083
Scott, R. S., Ungar, P. S., Bergstrom, T. S., Brown, C. A., Childs, B. E., Teaford, M. F., et al. (2006). Dental microwear texture analysis: Technical considerations. J. Hum. Evol. 51, 339–349. doi: 10.1016/j.jhevol.2006.04.006
Semprebon, G. M., Rivals, F., and Janis, C. M. (2019). The role of grass vs. exogenous abrasive in the paleodietary patterns of North American ungulates. Front. Ecol. Evol. 7:65. doi: 10.3389/fevo.2019.00065
Shoocongdej, R., Phumijumnong, N., Chintakanon, K., Pureepatpong, N., Hoontrakul, U., and Treerayapiwat, C. (2007). Final report of highland archaeology project in Pang Mapha district, Mae Hong Son province Phase 2, Vol. 2. Bangkok: Thailand Research Fund (TRF).
Sodhi, N. S., Koh, L. P., Brook, B. W., and Peter, N. L. (2004). Southeast Asian biodiversity: An impending disaster. Ecol. Evol. 19, 654–660. doi: 10.1016/j.tree.2004.09.006
Suraprasit, K., Bocherens, H., Chaimanee, Y., Panha, S., and Jaeger, J. J. (2018). Late Middle Pleistocene ecology and climate in Northeastern Thailand inferred from the stable isotope analysis of Khok Sung herbivore tooth enamel and the land mammal cenogram. Quat. Sci. Rev. 193, 24–42. doi: 10.1016/j.quascirev.2018.06.004
Suraprasit, K., Jaeger, J. J., Chaimanee, Y., and Sutcharit, J. (2021a). Taxonomic reassessment of large mammals from the Pleistocene Homo-bearing site of Tham Wiman Nakin (Northeast Thailand): Relevance for faunal patterns in mainland Southeast Asia. Quat. Int. 603, 90–112. doi: 10.1016/j.quaint.2020.06.050
Suraprasit, K., Jaeger, J. J., Chaimanee, Y., Benammi, M., Chavasseau, O., Yamee, C., et al. (2015). A complete skull of Crocuta crocuta ultima indicates a late Middle Pleistocene age for the Khok Sung (northeastern Thailand) vertebrate fauna. Quat. Int. 374, 34–45. doi: 10.1016/j.quaint.2014.12.062
Suraprasit, K., Jaeger, J. J., Chaimanee, Y., Chavasseau, O., Yamee, C., Tian, P., et al. (2016). The Middle Pleistocene vertebrate fauna from Khok Sung (Nakhon Ratchasima, Thailand): Biochronological and paleobiogeographical implications. ZooKeys 613, 1–157. doi: 10.3897/zookeys.613.8309
Suraprasit, K., Jaeger, J. J., Shoocongdej, R., Chaimanee, Y., Wattanapituksakul, A., and Bocherens, H. (2020). Long-term isotope evidence on the diet and habitat breath of Pleistocene to Holocene caprines in Thailand: Implications for the extirpation and conservation of Himalayan gorals. Front. Ecol. Evol. 8:67. doi: 10.3389/fevo.2020.00067
Suraprasit, K., Jongautchariyakul, S., Yamee, C., Pothichaiya, C., and Bocherens, H. (2019). New fossil and isotope evidence for the Pleistocene zoogeographic transition and hypothesized savanna corridor in peninsular Thailand. Quat. Sci. Rev. 221:105861. doi: 10.1016/j.quascirev.2019.105861
Suraprasit, K., Shoocongdej, R., Chintakanon, K., and Bocherens, H. (2021b). Late Pleistocene human paleoecology in the highland savanna ecosystem of mainland Southeast Asia. Sci. Rep. 11:16756. doi: 10.1038/s41598-021-96260-4
Taylor, L. A., Kaiser, T. M., Schwitzer, C., Muller, D. W. H., Codron, D., Clauss, M., et al. (2013). Detecting inter-cusp and inter-tooth wear patterns in Rhinocerotids. PLoS One 8:e80921. doi: 10.1371/journal.pone.0080921
Teaford, M. F., and Oyen, O. J. (1989). In vivo and in vitro turnover in dental microwear. Am. J. Phys. Anthropol. 80, 447–460. doi: 10.1002/ajpa.1330800405
Teaford, M. F., Ungar, P. S., Taylor, A. B., Ross, C. F., and Vinyard, C. J. (2017). In vivo rates of dental microwear formation in laboratory primates fed different food items. Biosurf. Biotribol. 3, 166–173. doi: 10.1016/j.bsbt.2017.11.005
Tougard, C. (1998). Les faunes de grands mammifères du Pléistocène moyen terminal de Thaïlande dans leur cadre phylogénétique, paléoécologique et biochronologique. Ph.D thesis Montpellier: University of Montpellier II.
Tougard, C. (2001). Biogeography and migration routes of large mammal faunas in South–East Asia during the Late Middle Pleistocene: Focus on the fossil and extant faunas from Thailand. Palaeogeogr. Palaeoclimatol. Palaeoecol. 168, 337–358. doi: 10.1016/S0031-0182(00)00243-1
Ungar, P. S., Merceron, G., and Scott, R. S. (2007). Dental microwear texture analysis of varswater bovids and Early Pliocene paleoenvironments of Langebaanweg, Western Cape province, South Africa. J. Mammal. Evol. 14, 163–181. doi: 10.1007/s10914-007-9050-x
Walker, A., Hoeck, H. N., and Perez, L. (1978). Microwear of mammalian teeth as an indicator of diet. Science 201, 908–910. doi: 10.1126/science.684415
Wattanapituksakul, A., Filoux, A., Amphansri, A., and Tumpeesuwan, S. (2018). Late Pleistocene Caprinae assemblages of Tham Lod Rockshelter (Mae Hong Son province. Northwest Thailand). Quat. Int. 493, 212–226. doi: 10.1016/j.quaint.2018.06.003
White, J. C., Penny, D., Kealhofer, L., and Maloney, B. (2004). Vegetation changes from the late Pleistocene through the Holocene from three areas of archaeological significance in Thailand. Quat. Int. 113, 111–132. doi: 10.1016/j.quaint.2003.09.001
Winkler, D. E., and Kaiser, T. M. (2011). A case study of seasonal, sexual and ontogenetic divergence in the feeding behavior of the moose (Alces alces Linné, 1758). Verh. Naturwiss. Verh. Hamburg. 46, 331–348.
Keywords: Caprinae, hypsodonty index, mesowear, DMTA, paleoecology, Pleistocene, Thailand
Citation: Isarankura Na Ayudhya J, Merceron G, Wannaprasert T, Jaeger J-J, Chaimanee Y, Shoocongdej R and Suraprasit K (2022) Dental mesowear and microwear for the dietary reconstruction of Quaternary Southeast Asian serows and gorals. Front. Ecol. Evol. 10:1000168. doi: 10.3389/fevo.2022.1000168
Received: 21 July 2022; Accepted: 24 August 2022;
Published: 13 September 2022.
Edited by:
G. Lynn Wingard, United States Geological Survey (USGS), United StatesReviewed by:
Tzu-Ruei Yang, National Museum of Natural Science, TaiwanCopyright © 2022 Isarankura Na Ayudhya, Merceron, Wannaprasert, Jaeger, Chaimanee, Shoocongdej and Suraprasit. This is an open-access article distributed under the terms of the Creative Commons Attribution License (CC BY). The use, distribution or reproduction in other forums is permitted, provided the original author(s) and the copyright owner(s) are credited and that the original publication in this journal is cited, in accordance with accepted academic practice. No use, distribution or reproduction is permitted which does not comply with these terms.
*Correspondence: Kantapon Suraprasit, S2FudGFwb24uU0BjaHVsYS5hYy50aA==
Disclaimer: All claims expressed in this article are solely those of the authors and do not necessarily represent those of their affiliated organizations, or those of the publisher, the editors and the reviewers. Any product that may be evaluated in this article or claim that may be made by its manufacturer is not guaranteed or endorsed by the publisher.
Research integrity at Frontiers
Learn more about the work of our research integrity team to safeguard the quality of each article we publish.