- 1School of Geography and Remote Sensing, Guangzhou University, Guangzhou, China
- 2Rural Non-point Source Pollution Comprehensive Management Technology Center of Guangdong Province, Guangzhou, China
- 3Centre for Climate and Environmental Changes, Guangzhou University, Guangzhou, China
- 4National-Regional Joint Engineering Research Center for Soil Pollution Control and Remediation in South China, Guangdong Key Laboratory of Integrated Agro-Environmental Pollution Control and Management, Institute of Eco-Environmental and Soil Sciences, Guangdong Academy of Sciences, Guangzhou, China
Urbanization has induced substantial changes in soil physicochemical characteristic, which plays an important role in regulating soil fauna biodiversity in forests and grasslands. However, less is known about the urbanization effect on soil fauna biodiversity and how soil physicochemical changes mediate this effect. Along an urbanization gradient in the city of Guangzhou, we established four sites with different urbanization intensities, including an urban site, two suburban sites, and a rural site, and then studied their soil physicochemical characteristic and soil fauna biodiversity. The soil physicochemical characteristic dramatically changed along the urbanization gradient. In contrast, the soil fauna biodiversity exhibited a very different pattern. Soil fauna abundance was highest in the suburban sites. Moreover, there were significant changes of Pielou’s evenness and community structure in the suburban sites. Soil fauna biodiversity property in the urban site was similar to that in the rural site, except that the rural site was characterized by Enchytraeidae while the urban site was not characterized by any taxa. Our linear and canonical correspondence analysis models suggested that soil physicochemical characteristic only contributed a little to the variance of soil fauna abundance (19%), taxa number (27%), and community structure (12%). In contrast, soil physicochemical characteristic explained about half of the variance in Shannon’s diversity and Pielou’s evenness. However, with urbanization intensity increasing, soil physicochemical changes could both increase and decrease the diversity and evenness. Thus, our results revealed an inconsistent pattern between soil fauna biodiversity and soil physicochemical characteristic along an urbanization gradient. This study suggested that soil physicochemical change was less important as expected in regulating soil fauna biodiversity pattern under an urbanization context. To elucidate the effect of urbanization on soil fauna biodiversity, further studies should take other urbanization agents into account.
Introduction
Urbanization is among the most dramatic changes in terrestrial ecosystem surface, resulting in a series of substantial abiotic and biotic changes (McIntyre et al., 2001; Veresoglou et al., 2015; Eisenhauer et al., 2019). Soil fauna is a megadiverse kingdom providing an array of ecosystem services (Wardle et al., 2004) and has been harnessed as a tool in monitoring ecosystem health (Fountain and Hopkin, 2005; Bispo et al., 2009; Gerlach et al., 2013). Soil fauna biodiversity is generally regulated by population dispersal limitation, environmental influence, and biotic interaction (Vellend, 2010; Gao M. et al., 2020). Urbanization has induced substantial changes in soil physicochemical characteristic, but less is known about its effects on soil fauna biodiversity. In natural and semi-natural ecosystems, soil physicochemical characteristic plays a vital role in regulating soil fauna biodiversity (Coleman et al., 2004). However, it is unclear that in human-dominant urban ecosystems, how soil physicochemical changes will mediate the effects of urbanization on soil fauna biodiversity.
There are many studies on urban soil physicochemical characteristic changes, drawing a conclusion that urbanization substantially altered soil physicochemical characteristic. In urban areas, soils are usually served as a supporting for green space and embedded in the context of city constructions such as buildings, roads, and public squares. Such landscape background would increase soil temperature (Shi et al., 2012) and exacerbate soil moisture fluctuation (Butler and Davies, 2011). Urban soils may be trampled, covered, dug, or removed and thus losing its natural structure and texture, showing higher bulk density, incomplete vertical structure, and broken texture (Gilbert, 1989; Banat et al., 2005; Wei and Yang, 2010). Artificial product wastes, such as broken bricks, glass and china, and kitchen refuse, are buried into soils during city constructions and residential daily life in urban areas. In addition, artificial wastes could also enter into soils in the form of dust, through rainfall water flow and dry deposition. As a result, increased soil pH (Jim, 1998; Pouyat et al., 2015; Asabere et al., 2018) and changed element contents (e.g., C, N, P, and Ca) (Pouyat et al., 2002; Trammell et al., 2020) in urban areas are widely reported. Notably, traffic, industry, and garden management may produce wastes rich in metal elements, such as Cd, Cr, Cu, Pb, and Zn, which are harmful to most lives and hard to be removed from soils once being polluted (Wei and Yang, 2010).
In contrast, the effects of urbanization on soil fauna biodiversity are far from a conclusion. There are some studies suggesting that soil fauna biodiversity is higher in less disturbed ecosystems under an urbanization context (Fiera, 2009; Szlavecz et al., 2018; Liu et al., 2019), while some studies find contradictory results (Sterzynska et al., 2018; Joimel et al., 2019). For example, by summarizing 758 studies, Joimel et al. (2017) concluded that there are higher soil fauna biodiversity in downtown and industrial areas. We still knew little why results of urbanization effects on soil fauna contradict.
At present, there are more studies suggesting that urban soil fauna biodiversity is influenced by factors other than soil physicochemical characteristic under an urbanization context. Habitat landscape characteristic, such as habitat area, connectivity, and diversity, are important in determining soil fauna biodiversity (Bolger et al., 2000; Milano et al., 2018; Xie et al., 2018; Gao M. et al., 2020), mostly because they could affect extinction rate, individual and gene exchanges, and taxa co-occurrence, thus shaping community assembly processes (LaPoint et al., 2015; Lepczyk et al., 2017). Transport and cross-region tourism would help soil fauna overcoming geographic isolation and entering into potential urban habitats, resulting in direct changes in soil fauna taxa composition (Gray, 1989; Tothmeresz et al., 2011; Horvath et al., 2012; Chatzinikolaou et al., 2018). In urban green space, garden management and visits of residents will expose soil fauna under continuous anthropogenic disturbances (Norton, 2011; Tresch et al., 2018, 2019). These facts raised a question that how important the soil physicochemical property change is in mediating urbanization effect on soil fauna biodiversity.
In this article, by studying the soil fauna communities and soil physicochemical characteristics in four urbanization gradient sites, we aimed at exploring the following: (1) whether soil fauna biodiversity changes consistently with soil physicochemical characteristic as urbanization intensity increases and (2) how soil physicochemical characteristic regulates soil fauna biodiversity across the urbanization gradient.
Materials and Methods
Sites Along an Urbanization Gradient
Four urbanization gradient sites were established in Guangzhou City (22°26′ to 23°56′N and 112°57′ to 114°03′E), which is the capital of Guangdong Province in South China. The climate is a subtropical, marine monsoon, with an annual average temperature of 21.5–22.2°C and an annual average precipitation of 1,623.6–1,899.8 mm. Natural vegetation covering the city is tropical monsoon forest. The typical soil in natural ecosystems is latosolic red soil.
With urbanization intensity decreasing, the four sites are urban site (URB), suburban site 1 (SUB1), suburban site 2 (SUB2), and rural site (RUR), located at Yuexiu Park, Tianlu Lake Forest Park, Maofeng Mountain Forest Park, and Shimen Forest Reserve, respectively (Figure 1A). The Yuexiu Park (where URB is located) developed from the Sun Yat-sen Memorial Park established in 1920s. At present, Yuexiu Park is embedded in Guangzhou downtown and surrounded by residential buildings and business districts with a dense human population. The vegetations in the park are either native woodland or managed garden. Soil ecosystems in Yuexiu Park were highly fragmented by impervious roads and highly disturbed by tourists. The Tianlu Lake Forest Park (where SUB1 is located) is about 19 km away from the Yuexiu Park. The Tianlu Lake Forest Park is at the edge of Guangzhou downtown, laying between natural hilly forests in the north and residential areas in the south. The vegetations are integrated secondary broadleaf forests and plantations. The Maofeng Mountain Forest Park (where SUB2 is located) is about 27 km away to the Yuexiu Park and 9.4 km to the Tianlu Lake Forest Park. The park is surrounded by hilly forests and only has a roadway to connect with Guangzhou downtown. The vegetations are also secondary broadleaf forests and plantations. The Shimen Forest Reserve (where RUR is located) is at the Northeastern Guangzhou, about 75 km away from the Yuexiu Park. The Reserve is mainly covered by subtropical evergreen broadleaf forests and, in the mountain feet, by plantations.
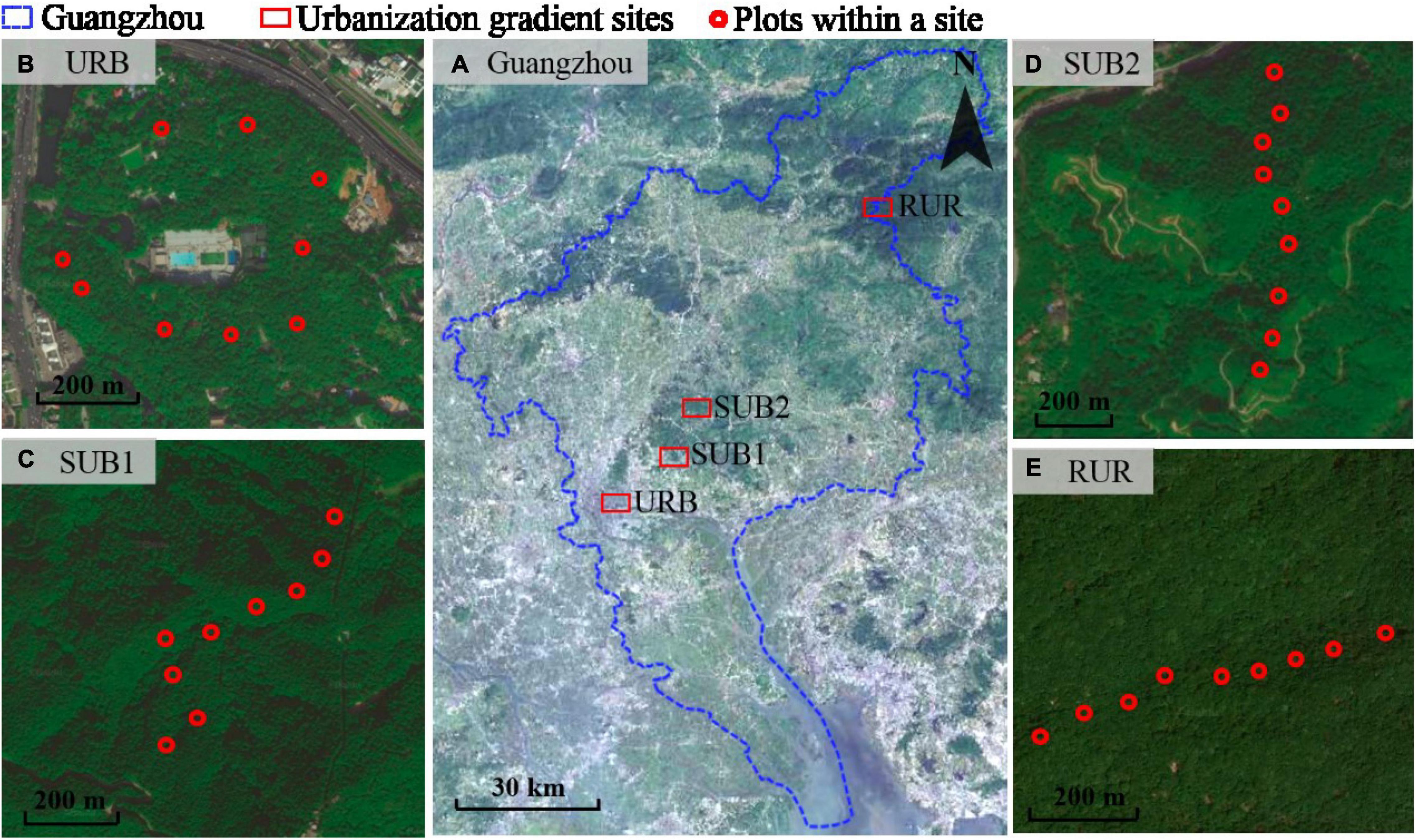
Figure 1. (A) The site location in Guangzhou City along an urbanization gradient. (B–E) The locations of nine plots within each site. The urbanization gradient sites are urban (URB), suburban 1 (SUB1), suburban 2 (SUB2), and rural (RUR) sites.
Soil Fauna Samplings and Identifications
To obtain represented soil fauna samples, we made samples in a 1,000-m-long transect in each urbanization gradient site to cover as much environmental habits as possible in plant-covered areas (Figures 1B–E). We planned to take samples in 9 plots lying at a straight line. However, it was hard to make it in these subtropical hilly forests, where there were so many ridges and valleys, while the Yuexiu Park was not large enough. Soil fauna sampling was performed only once in each plot of the four sites during November 2020 to January 2021. We established three subplots in each plot, and the subplots were 5 m away from each other. To acquire a sample, plant litter was first removed from the surface. Then a soil core was taken in a subplot with a 5 cm diameter cylinder, to 10 cm depth. Soils collected in a plot were pooled and mixed to generate a soil sample. Immediately after collections, the samples were transported to our laboratory, and soil fauna were extracted using Tullgren dry funnels for 48 h. All specimens were sorted mainly according to Yin (1998) and counted using a dissecting microscope (Leica, German) and a Nikon Eclipse 80i microscope (Nikon, Tokyo, Japan).
In each plot, soil fauna abundance (individual number from a sample) and taxa number (taxa number from a sample, S) were recorded and used to calculate Shannon’s diversity index (H) as follows (Shannon, 1948):
where Pi is the ratio between the abundance of group i and the total number of soil fauna. Evenness was evaluated according to the Pielou’s evenness index (J) as follows (Pielou, 1969):
Soil Samplings and Physicochemical Analyses
Similar to the soil fauna sampling, another three soil corers were taken using a 3 cm diameter steel corer in each plot to generate a soil sample for soil physicochemical characteristic analysis. Soil bulk density (BD) was measured following the methodology described in Maynard and Curran (2007). In brief, the soil samples were dried at 105°C for 48 h and were weighed. BD (in g/cm3) corresponds to the dry weight divided by the volume of samples. Soil pH was measured using a 1:2.5 soil-water suspension with the potentiometric method. Gravimetric soil water content (SWC) was measured on 20 g soil dried at 105°C for 48 h. Soil organic matter (SOM) content was determined using H2SO4-K2Cr2O7 oxidation method. Soil total nitrogen content (TN) was quantified by the Kjeldahl acid digestion method. Soil total phosphorus content (TP) was quantified using the molybdate blue method after acid digestion (Hou et al., 2014). Soil heavy metal concentration (i.e., Cd, Cr, Cu, Pb, and Zn) was analyzed with graphite furnace atomic absorption spectrophotometry method after digestion in a mixture of nitric acid, perchloric acid, and hydrogen peroxide.
Statistical Analysis
Since species richness tends to vary with sampling intensity and fauna individual number, we used rarefaction to compare species richness among the urbanization gradient sites. We randomly sampled 41 individuals (the lowest value in the soil fauna sampling) from each plot to estimate taxa richness. One-way ANOVA was used to assess the differences in soil fauna abundance, taxa richness, H, and J among the urbanization gradient sites. Where the overall analysis was significant (p < 0.050), we used Tukey’s HSD post hoc tests for pairwise comparisons. The same procedures were also used to test the differences in BD, SWC, pH, SOC, TN, TP, Cd, Cr, Cu, Pb, and Zn among the sites.
We assessed the changes in soil fauna community structure using multivariate analysis. Using taxa and abundance data, principal coordinate analysis (PCoA) with Jaccard’s dissimilarity was employed to visualize the separation of communities among the four urbanization gradient sites. Furthermore, we tested the differences in soil fauna communities among the sites using permutational multivariate analysis of variance (PERMANOVA). The p-value was obtained based on 999 permutations. The same procedure was used to assess the overall differences in soil physicochemical characteristic among the sites using PCoA and PERMANOVA. To examine the heterogeneity of soil fauna biodiversity and soil physicochemical characteristic within each site, the homogeneity of multivariate dispersions was calculated. Then the homogeneities were compared using one-way ANOVA and Tukey’s HSD post hoc tests (Anderson, 2006). Indicator species of each gradient site were determined using Dufrene-Legendre indicator (Dufrêne and Legendre, 1997).
To determine the relationships between soil physicochemical variables and soil fauna biodiversity (i.e., taxa number, abundance, H, and J), we used linear models with all-subset selection of a priori explanatory variables (i.e., BD, SWC, pH, SOC, TN, TP, Cd, Cr, Cu, Pb, and Zn). We then explored the influence of soil physicochemical characteristic on soil fauna community structure using canonical correspondence analysis (CCA) with backward stepwise selection of a priori explanatory variables (i.e., BD, SWC, pH, SOC, TN, TP, Cd, Cr, Cu, Pb, and Zn).
All analyses were performed using R version 4.02 (R Core Team, 2020). One-way ANOVA, Tukey’s HSD, and linear regression model were performed using the stats package (R Core Team, 2020). All-subset selection for the best linear model was performed using the leap package (Lumley and Miller, 2020). Rarefaction, PCoA, PERMANOVA, CCA, backward stepwise selection for the best CCA model, and homogeneity of multivariate dispersions were conducted using the vegan package (Oksanen et al., 2017). Indicator species analysis was done using the labdsv package (Roberts, 2013).
Results
Soil Physicochemical Characteristics Along the Urbanization Gradient
There were substantial differences among the urbanization gradient sites in soil BD, soil pH, SOC, TN, TP, gravimetric SWC, and total soil Cd, Cu, Cr, Pb, and Zn content (ANOVA: all p < 0.050) (Figure 2). With urbanization intensity increasing, gradual changes were observed in BD (Figure 2A), SWC (Figure 2F), Cu (Figure 2H), and Pb (Figure 2J). Soil physicochemical characteristic in the site URB was very different from other sites and had the highest BD (1.33 ± 0.04 g/cm3), soil pH (5.04 ± 0.29), TP (0.584 ± 0.060 g/kg), Cu (18.2 ± 1.3 mg/kg), Cr (49.1 ± 7.7 mg/kg), and Pb (162.8 ± 46.3 mg/kg), but lowest SWC (13.1 ± 1.6%). In contrast, the site RUR had the lowest BD (0.78 ± 0.04 g/cm3), TP (0.299 ± 0.055 g/kg), Cu (4.15 ± 0.17 mg/kg), and Pb (32.8 ± 1.14 mg/kg), but the highest SOC (36.8 ± 2.2 g/kg), TN (2.72 ± 0.20 g/kg), and SWC (25.6 ± 1.2%). Apart from total soil Zn, differences between RUR and URB in all measured soil physicochemical characteristics were significantly different (Tukey’s HSD: p < 0.050). Notably, total soil Pb, Cu, and Cr in the site URB were as high as 5.0 (Tukey’s HSD: p = 0.002), 4.4 (Tukey’s HSD: p < 0.001), and 3.2 (Tukey’s HSD: p = 0.037) times higher than those in the site RUR. Values of soil physicochemical characteristic in the two suburban sites, namely, SUB1 and SUB2, were usually between the sites RUR and SUB. The SUB1 and SUB2 had similar soil physicochemical characteristic values and only significantly differed in BD (Tukey’s HSD: p = 0.017).
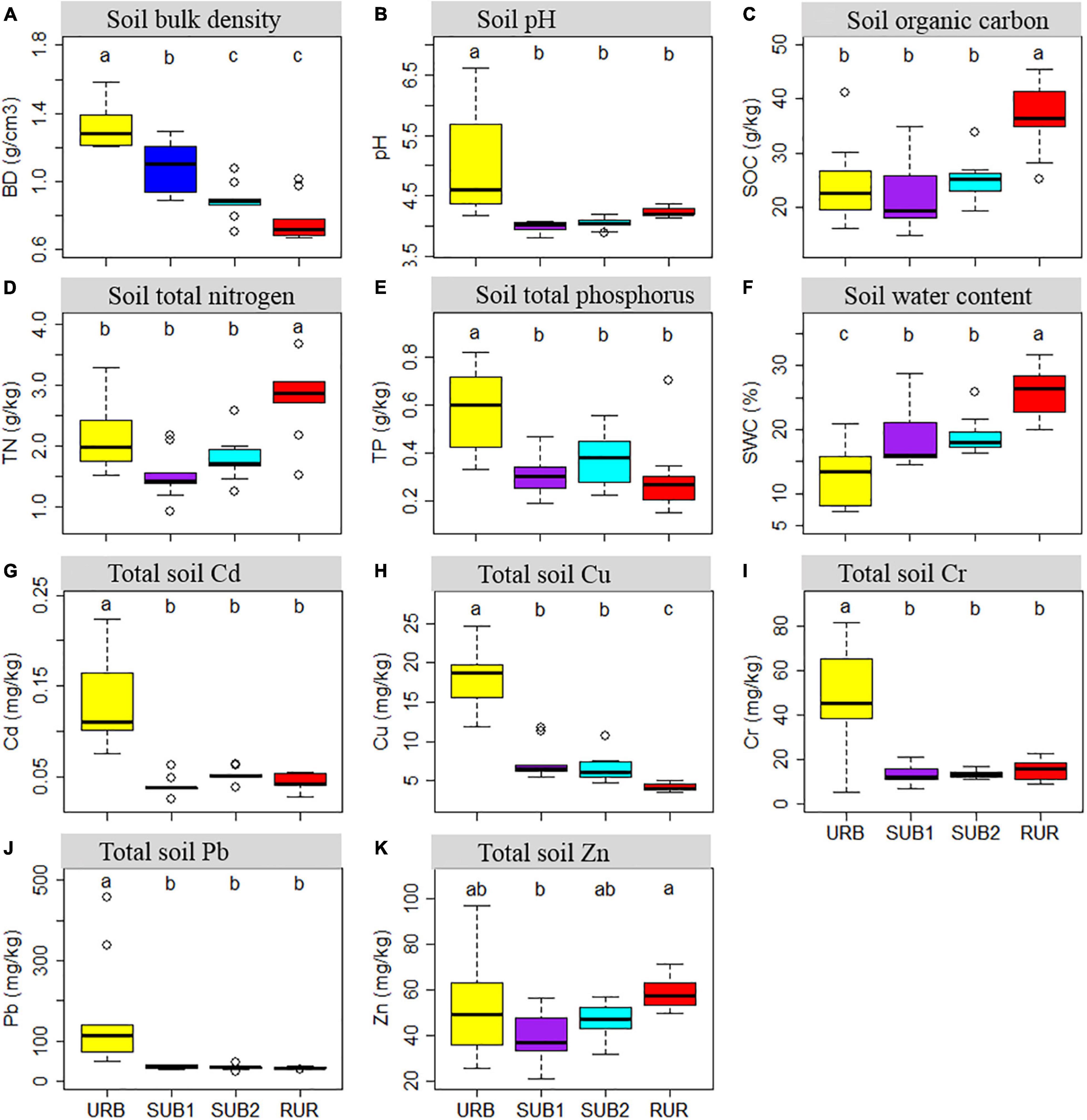
Figure 2. Boxplots showing soil physicochemical characteristic in urbanization gradient sites: (A) Soil bulk density; (B) Soil pH; (C) Soil organic carbon content; (D) Soil total nitrogen content; (E) Soil total phosphorus content; (F) Gravimetric soil water content; (G) Total soil Cd content; (H) Soil total Cu content; (I) Soil total Cr content; (J) Soil total Pb content; (K) Total soil Zn content. The urbanization gradient sites are urban (URB), suburban 1 (SUB1), suburban 2 (SUB2), and rural (RUR) sites. Each boxplot is shown for nine samples. Different letters represent statistically significant differences at p = 0.050.
The results of PCoA on soil physicochemical characteristic based on Jaccard’s dissimilarity were consistent with the variance analysis. All plots could be grouped into three categories with a clear separation (Figure 3A). Plots within URB were quite dissimilar with each other, and betadisper analysis showed that heterogeneity within the URB was the highest (Figure 4A). However, the ordination ellipses of URB did not overlap on any other sites. Pairwise PERMANOVA showed that soil physicochemical characteristic of URB significantly differed with SUB1, SUB2, and RUR (all adjusted p = 0.006) (Supplementary Table 1). Plots within the site RUR were highly clustered and did not overlap with any other sites either. Pairwise PERMANOVA showed that RUR was significantly different from other sites (all adjusted p = 0.006). In contrast, SUB1 and SUB2 had similar soil physicochemical characteristic, and the plots of these two sites were highly blended (pairwise PERMANOVA: p = 0.996).
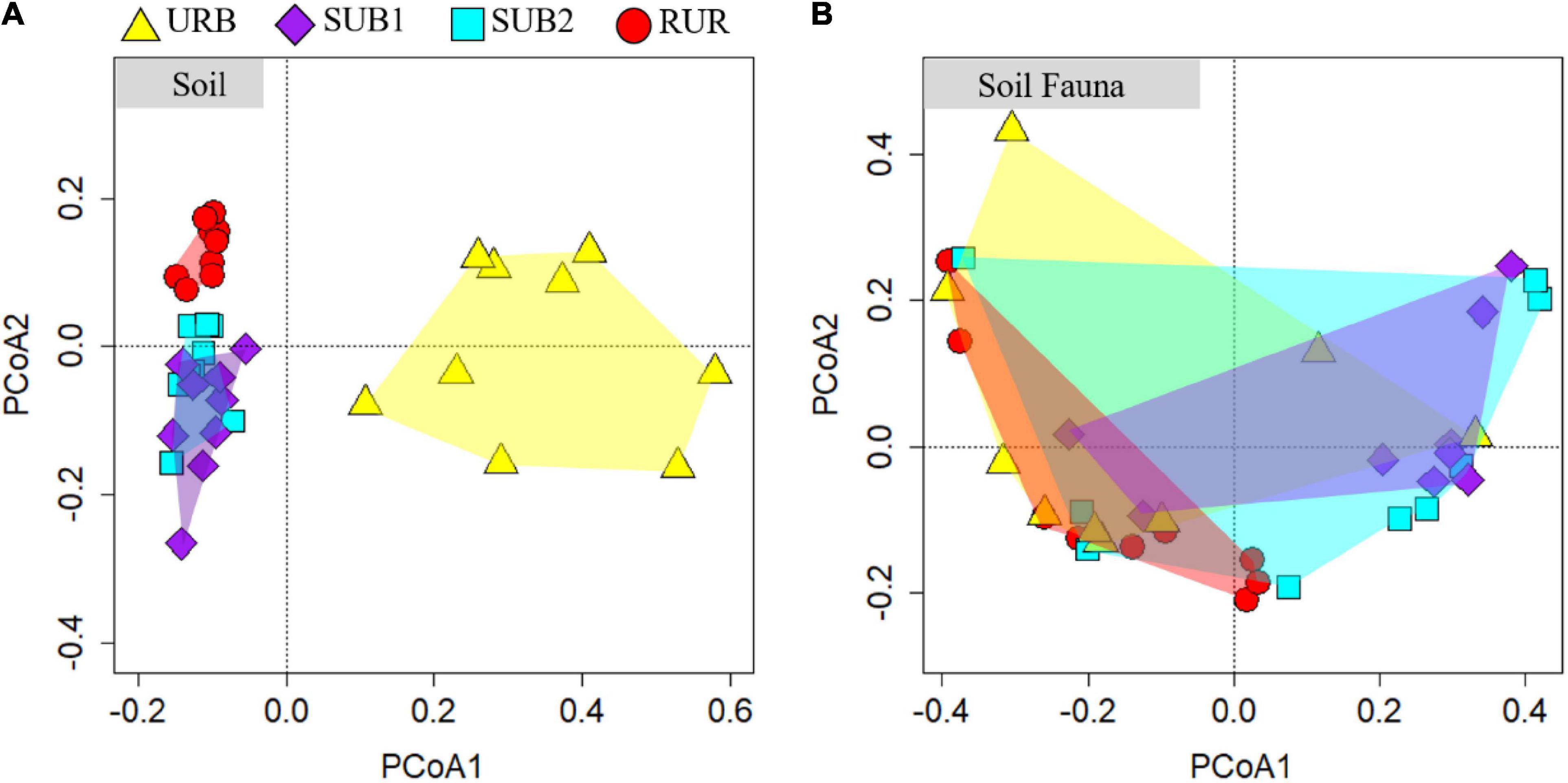
Figure 3. Principal coordinate analysis plots of (A) soil physicochemical characteristic and (B) soil fauna community structure in urbanization gradient sites. The urbanization gradient sites are urban (URB), suburban 1 (SUB1), suburban 2 (SUB2), and rural (RUR) sites.
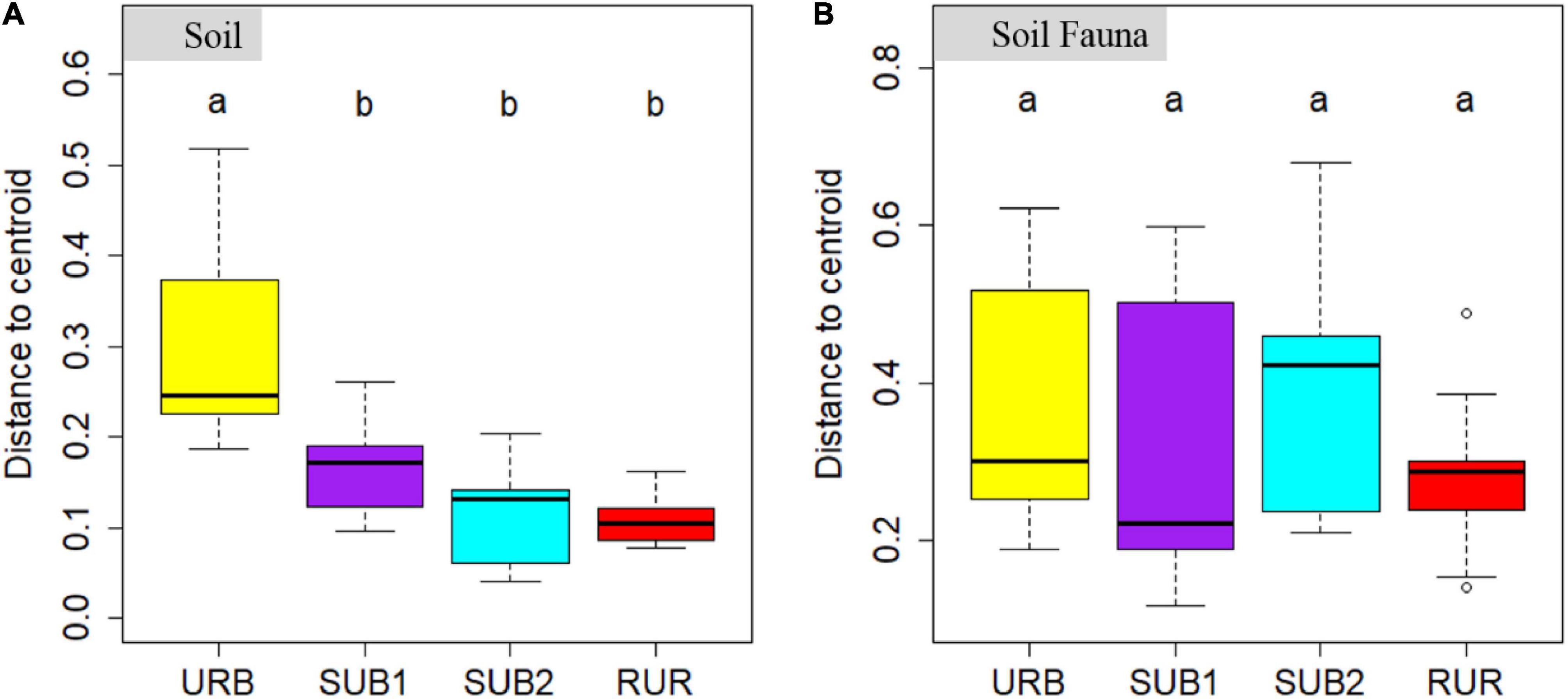
Figure 4. Boxplots showing the Jaccard’s dissimilarity of (A) soil physicochemical characteristic and (B) soil fauna community in urbanization gradient sites. The urbanization gradient sites are urban (URB), suburban 1 (SUB1), suburban 2 (SUB2), and rural (RUR) sites. Each boxplot is shown for nine samples. Different letters represent statistically significant differences at p = 0.050.
Soil Fauna Biodiversity Along the Urbanization Gradient
There were not significant differences in taxa number, H, and J (Figure 5). Interestingly, the SUB1 with intermediate urbanization intensity had the highest soil fauna abundance (272.8 ± 47.0 ind.), which was significantly higher than that of the URB (121.1 ± 24.1 ind.) (Tukey’s HSD: p = 0.042) and the RUR (122.6 ± 13.4 ind.) (Tukey’s HSD: p = 0.039), but similar to the SUB2 (250.9 ± 53.5 ind.) (Tukey’s HSD: p = 0.977) (Figure 5C). The SUB1 also had the highest taxa number (10.8 ± 1.2) (Figure 5A) and lowest J (0.51 ± 0.02) (Figure 5E). However, the differences in taxa number (ANOVA: p = 0.156) or Pielou’s evenness index (ANOVA: p = 0.131) among the four sites were not significant. There was only minor difference in Shannon’s diversity index among the four sites (3.51–3.67) (ANOVA: p = 0.963).
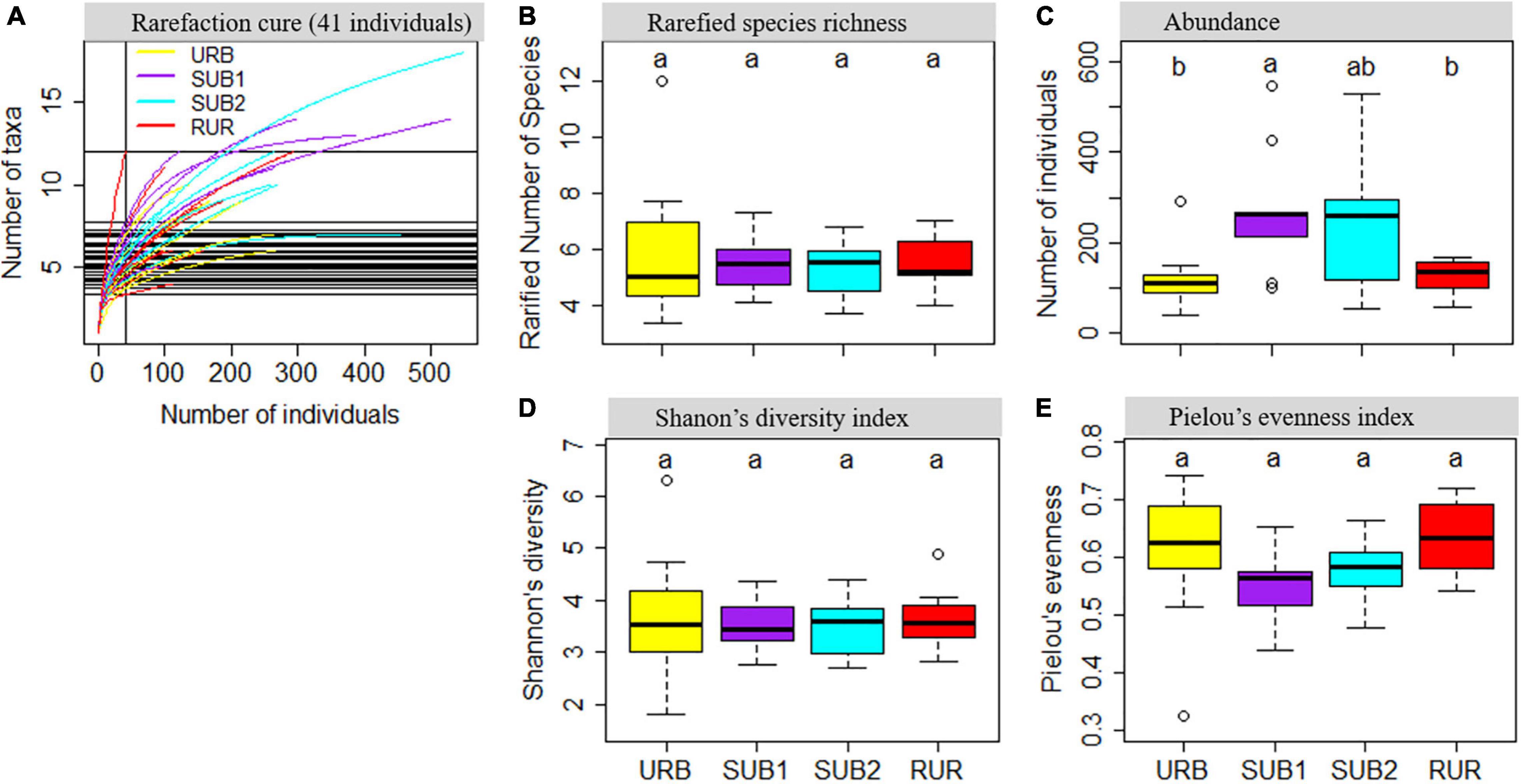
Figure 5. Soil fauna biodiversity properties in urbanization gradient sites. (A) Species rarefaction curve based on 41 individuals (the lowest value in the soil fauna sampling). Boxplots showing (B) the rarefied number of species, (C) the number of collected individuals, (D) Shannon’s diversity index, and (E) Pielou’s evenness index. The urbanization gradient sites are urban (URB), suburban 1 (SUB1), suburban 2 (SUB2), and rural (RUR) sites. Each boxplot is shown for nine samples. Different letters represent statistically significant differences at p = 0.050.
Principal coordinate analysis based on Jaccard’s dissimilarity showed that soil fauna community structure did not vary substantially among the urbanization gradient sites. Generally, soil fauna community structure of the four urbanization gradient sites was blended, and the ordination ellipses for each site were largely overlapped (Figure 3B). Soil fauna community structure was highly dissimilar within the URB and SUB2. The heterogeneity within each urbanization gradient site could also be seen in betadisper analysis, showing that average distance to centroids was 0.370, 0.331, 0.387, and 0.283 for URB, SUB1, SUB2, and RUR, respectively (ANOVA: p = 0.503) (Figure 4B). The plots within the SUB1 were slightly clustered, while two plots were very similar to the RUR plots. Pairwise PERMANOVA showed that soil fauna community structure in the site SUB1 significantly differed from RUR (adjusted p = 0.018) and tended to be differed from URB (adjusted p = 0.072) (Supplementary Table 1).
Indicator species analysis revealed that SUB1 was characterized by Pauropoda, SUB2 was characterized by Diplura, and RUR was characterized by Enchytraeidae (Figure 6). The analysis did not identify any taxa with high indicator value for the URB.
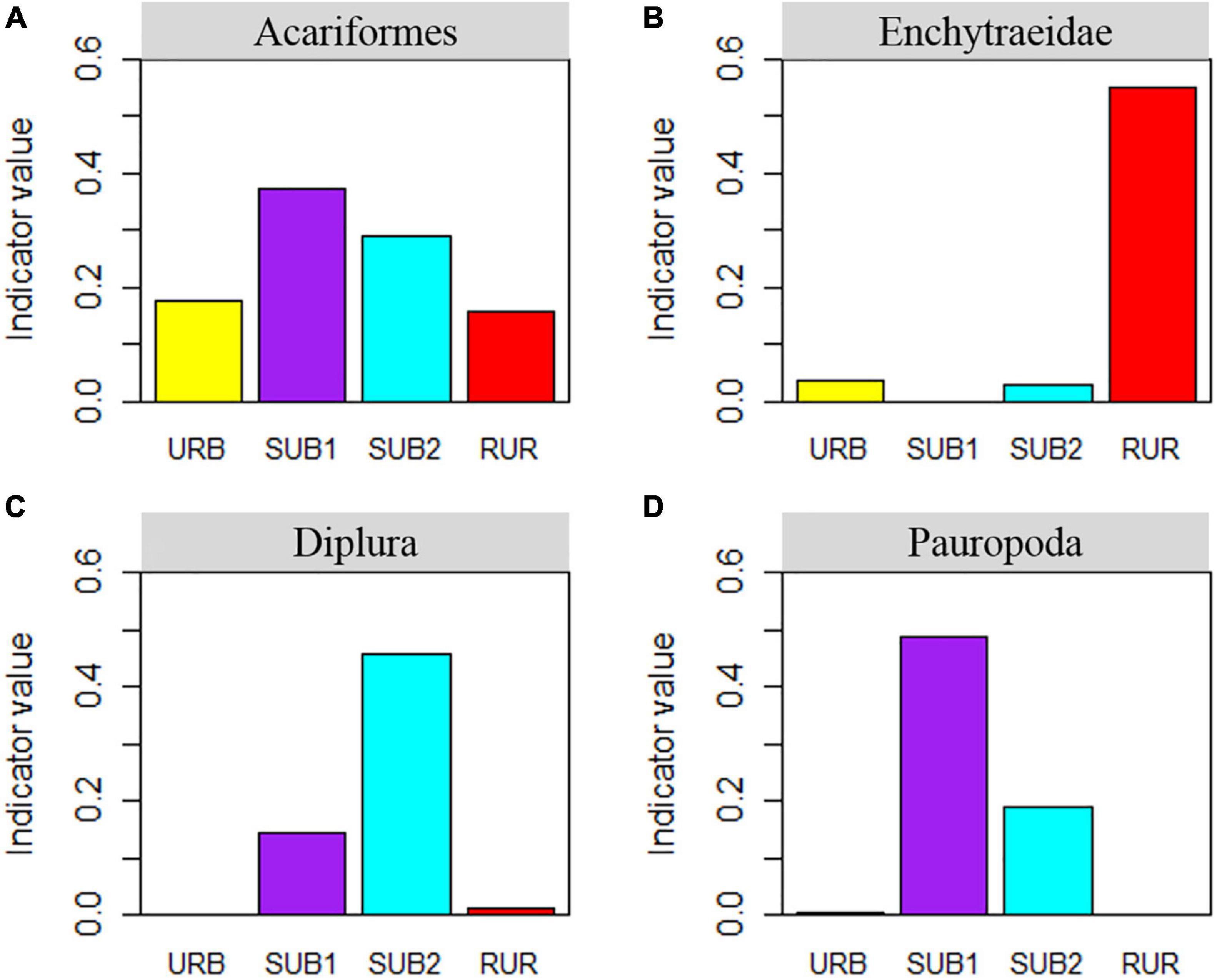
Figure 6. Significant indicator taxa for each urbanization gradient site as determined by the Dufrene-Legendre indicator species analysis. The significant indicator taxa are (A) Acariformes, (B) Enchytaeidae, (C) Diplura, (D) Pauropoda. The urbanization gradient sites are urban (URB), suburban 1 (SUB1), suburban 2 (SUB2), and rural (RUR) sites.
Relationships Between Soil Physicochemical Characteristics and Soil Fauna Biodiversity Across the Urbanization Gradient Site
Linear model analysis suggested that across the 36 plots, soil physicochemical characteristic contributed little to the variation in soil fauna abundance and taxa number, but played an important role in regulating both H and J. The best linear model for soil fauna abundance explained only 18.5% of the variance across all plots (p = 0.046) (Table 1). The independent variables included in the model were Cr (r = 0.62), SOC (r = –0.41), TN (r = –0.29), BD (r = –0.47), and Cd (r = –0.58) (all p > 0.050). A set of variables including Cu, SOC, and Cr explained 26.8% of the variance in soil fauna taxa number (p = 0.004). Total soil Cr content had an extremely negative effect on taxa number (r = –0.92, p = 0.002), while Cu had a significant positive effect (r = 0.63, p = 0.032). Linear model analysis also suggested that soil physicochemical characteristic played an important role in regulating H (R2 = 0.535, p < 0.001) and J (R2 = 0.456, p < 0.001). Contents of heavy metal, i.e., Cr (r = –0.86, p = 0.005), Pb (r = –0.28, p = 0.097), and Zn (r = –0.48, p = 0.026), had negative effects on H, while soil pH (r = 0.69, p = 0.014) and TN (r = 0.56, p < 0.001) had positive effects on H. The soil Pb content (r = –0.64, p = 0.009) was negatively correlated with J, while Cd (r = 0.76, p < 0.001) and TN (r = 0.63, p < 0.001) were positively correlated with J.
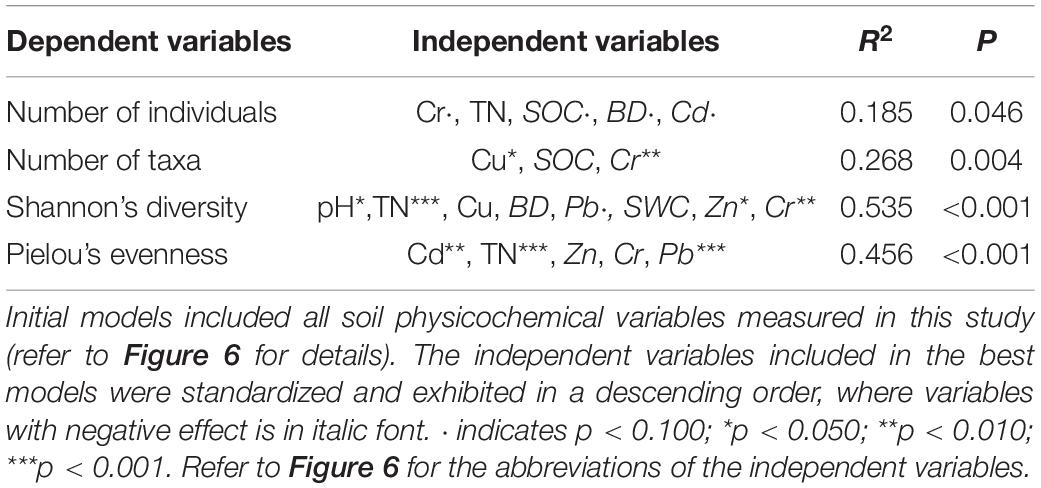
Table 1. Results from all-subset linear regression analysis relating soil fauna biodiversity to soil physicochemical characteristic.
The best CCA model indicated that soil physicochemical characteristic included SWC (p = 0.001), Cu (p = 0.013), and Cr (p = 0.018), explaining only 12.1% variance of the soil fauna community structure (p = 0.021) (Figure 7).
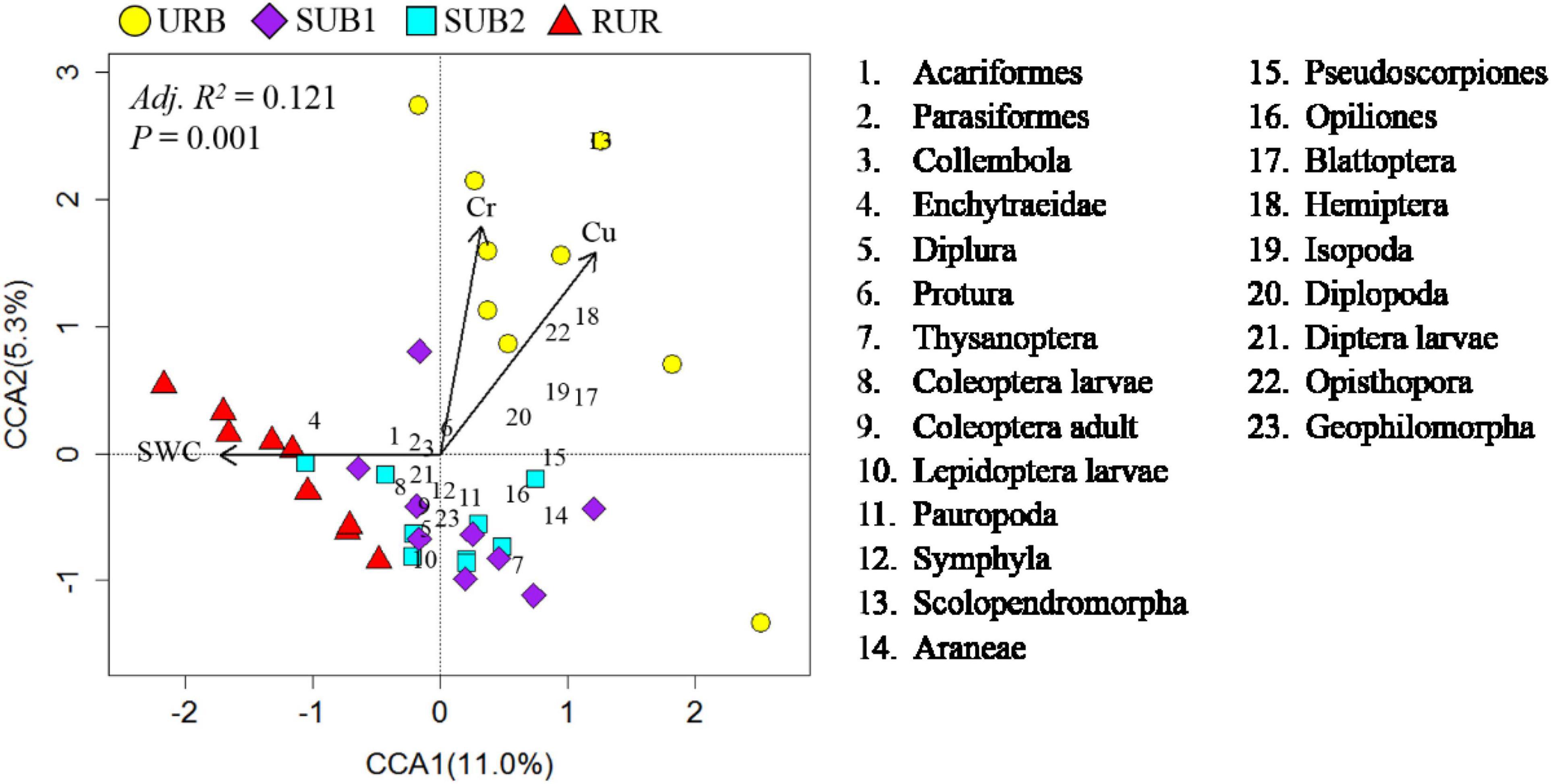
Figure 7. Canonical correspondence analysis (CCA) ordination plot using soil physicochemical characteristic as the environmental data matrix and the abundance of soil fauna taxa as response variable. The CCA model is the best model selected by a backward stepwise procedure. Explained variance (R2) is the sum of all canonical eigen values and adjusted by Bonferroni method (Zuur et al., 2007). The P-values are based on a permutation test (999 permutations). The urbanization gradient sites are urban (URB), suburban 1 (SUB1), suburban 2 (SUB2), and rural (RUR) sites.
Discussion
Effects of Urbanization on Soil Physicochemical Characteristic
In accordance with previous studies, our findings suggested that soil physicochemical characteristic substantially changed along the urbanization gradient. With urbanization proceeding, soil habitats in urban areas may be patched, disturbed, and polluted (Forman, 2009). Changes in urban soil physicochemical characteristic are reported worldwide. The higher soil pH in our urban site could be attributed to crowded buildings (Jim, 1998; Pouyat et al., 2015; Asabere et al., 2018). Busy traffic in urban areas may account for the heavy metal accumulation in urban soils (Wei and Yang, 2010). Soil structure and texture destroy induced by topsoil cover and removal, heavy equipment compaction, or walking could result in the higher soil BD (Kissling et al., 2009). Kitchen refuse and other organic waste being buried into soil, as well as fertilizer application in garden areas, will induce an increase in SOM content and thus the increases of soil organic carbon, nitrogen, and phosphorus (Pouyat et al., 2002; Trammell et al., 2020). The soil moisture decline in the urban soils maybe due to aboveground litter removal and soil texture destruction (Butler and Davies, 2011). This study clearly showed the changes of soil physicochemical characteristic in urban soils, which is happening in cities around the world.
Effects of Urbanization on Soil Fauna Biodiversity
Our results did not support the intermediate disturbance hypothesis, in which the suburban sites failed to indicate a significant increase in taxa richness and diversity. Indeed, it is not clear whether the hypothesis is valid in urban ecosystems, as studies reported contradictory results. While some studies find intermediate urbanization levels favor soil fauna biodiversity (Bogyo et al., 2015; Yu et al., 2021), some others suggest soil fauna biodiversity decreases with increasing urbanization intensity (Gray, 1989; Nagy et al., 2018; Lovei et al., 2019). Interestingly, soil fauna abundance in this study peaked under a medium urbanization intensity, as showed in both sites SUB1 and SUB2, which was in accordance with our previous study in the downtown and surrounding region of Guangzhou (Yu et al., 2021) and some other studies (Papastefanou et al., 2015).
We still did not know much why these results contradicted. However, studies on aboveground organisms suggest that urbanization may either facilitate or inhibit biodiversity. Urbanization could be harmful to organism as it induced direct habit loss, soil pollution, and strong disturbance (McKinney, 2006; Knop, 2016; Sanchez-Bayo and Wyckhuys, 2019). In contrast, urban habitats provide many resources for soil fauna, such as water from irrigation, food from garbage, and refuge sites in gardens and under paving stones (Jones and Leather, 2012; Hornung et al., 2018; Szlavecz et al., 2018). Soil fauna biodiversity response to urbanization maybe largely a tradeoff between the negative and positive effects of urbanization. Therefore, there should be efforts to investigate the soil fauna biodiversity response to each specific urban environmental change on soil fauna in order to improve the understanding on the urbanization effects on soil fauna biodiversity.
The response to urbanization is usually taxonomic-dependent, which may contribute to decreasing Pielou’s evenness index in the suburban sites, and is also a potential mechanism to maintain taxa number and diversity. Urbanization could be destructive to organisms specialized to inner and continuous habitats, but favorable to opportunists and cosmopolitan species (Knop, 2016; Diego Ibanez-Alamo et al., 2017). Soil biodiversity is especially diverse, and even functional and taxonomic redundant (Wolters, 2001; Allison and Martiny, 2008). What is more, global human footprint has dramatically accelerated species dispersal and thus alien species invasion, favoring opportunists and cosmopolitan species, which could flourish in human-dominant urban areas (Gray, 1989; Tothmeresz et al., 2011; Horvath et al., 2012; Chatzinikolaou et al., 2018). These would easily cause changes in soil fauna community structure and taxa evenness (Ge et al., 2019; Tresch et al., 2019; Abrego et al., 2020). However, under such a situation, taxa number and diversity could remain unchanged as some species may fill blank niches or occupy new niches created by urbanization (Morelli et al., 2016; Nielsen et al., 2019).
Indicator taxa for the four urbanization sites were different (the urban site did not have significant indicator species). Diplurans are small, elongate, delicate, primitive invertebrates, predating other small arthropods or ingest fungal mycelia and detritus (Ferguson, 1990). Most diplurans are euedaphic, but some are nocturnal cryptozoans, hiding under stones or bark during the day. Pauropods are tiny, true euedaphic terrestrial myriapods (Scheller, 2002). They inhabit in the lower litter layers, F-layers, and mineral soil in forests. It is generally assumed that pauropods are fungus feeders, but they may also be predaceous. In contrast, Enchytraeidae are typically 10–20 mm in length, bigger than both diplurans and pauropods. Enchytraeidae are commonly found in moist soils rich in organic matter (van Vliet, 2000), which probably made them to be the indicator species for the rural forest soil fauna community in this study. More efforts should be made to understand why they could be indicator species for the soil fauna communities in these ecosystems. Nevertheless, these taxa may have a potential to indicate urbanization effects on soil ecosystem.
Roles Soil Physicochemical Characteristics Play in Mediating Urbanization Effects on Soil Fauna Biodiversity
Across the 36 plots in this study, soil physicochemical characteristic only contributed little to the variances in fauna abundance, taxa number, and community structure, but is quite important in regulating Shannon’s diversity and Pielou’s evenness.
We found soil heavy metal contents, including Cd, Cr, Cu, Pb, and Zn, were very important factors in influencing soil fauna biodiversity. Negative relationship between soil fauna biodiversity and heavy metal content may be attributed to the universal heavy metal toxicity to organism growth and reproduction (Crommentuijn et al., 1993; Didden and Rombke, 2001; Herbert et al., 2004). In urban field studies whether or not heavy metals could inhibit soil fauna biodiversity (Fiera, 2009; Santorufo et al., 2012; Sterzynska et al., 2018), the results may depend on the resistance of study organism and the range of heavy metal content. The metals Cr and Cu were the key factors contributing to the divergence of urban soil fauna community structure from other sites, indicating that the accumulated heavy metals in urban soils played an important role in mediating urbanization effects on soil fauna community structure. In contrast, soil water availability was important in maintaining the rural soil fauna community structure and was strongly correlated with Enchytraeidae, the indicator species for the soil fauna community in the rural site. Soil in rural area was less disturbed, and with a higher SOM and lower BD, thus having a better water holding capacity, which may be important to resist to the negative effect of urbanization on soil fauna biodiversity.
Soil fauna biodiversity is not only affected by soil physicochemical characteristic but also regulated by habitat landscape, aboveground vegetation, direct disturbance, and so on. Soil physicochemical characteristic is very important in regulating soil fauna biodiversity in natural forests, grasslands, and farmlands (van Straalen, 1998; Bispo et al., 2009; Gerlach et al., 2013). In urban ecosystems, habitat landscape properties, including area, connectivity, and landscape heterogeneity, can significantly affect soil fauna biodiversity (Braaker et al., 2014; Milano et al., 2018; Xie et al., 2018). In addition, garden management (Byrne and Bruns, 2004) and land use history (Smetak et al., 2007; Francini et al., 2018) can also influence soil fauna biodiversity. These environmental factors that influence soil fauna biodiversity may not vary consistently, thus decoupling the apparent correlation between soil fauna biodiversity and physicochemical characteristic, which should be taken into account in further studies.
This study suggested that soil physicochemical changes due to urbanization could either increase or decrease soil fauna biodiversity, which was similar to studies on aboveground organisms suggesting urbanization could either facilitate or inhibit biodiversity through different urbanization agents (McKinney, 2006; Jones and Leather, 2012; Hornung et al., 2018). Consequently, though soil physicochemical characteristic explained about half of the variances in Shannon’s diversity and Pielou’s evenness across the plots, neither of them exhibited a pattern similar to the soil physicochemical characteristic along the gradient. These results suggested that under an urbanization context, the effects of soil physicochemical changes on soil fauna biodiversity may be indirect and complicated.
Limitations of This Study
It should be noted that sampling was performed only once in this study. Traditionally, in subtropical ecosystems, soil fauna biodiversity is different between wet and dry seasons, which is found in Guangzhou City (Qin et al., 2009; Wang and Tong, 2012) and other subtropical ecosystems (Zhao et al., 2011). Soil fauna biodiversity responses to environmental changes could also differ between wet and dry seasons. For example, Gao D. et al. (2020) found the response pattern of soil nematodes to land use changes in wet season is different from that in dry season in a subtropical forest. Therefore, there is a possibility that soil fauna biodiversity pattern along the urbanization gradient in wet season will be different from the one shown in this study. Moreover, soil fauna could be substantially influenced by low soil water availability (Liu et al., 2015) and low air temperature (Meyer-Wolfarth et al., 2021), thus less sensitive to other environmental changes in the mid-dry season. Nevertheless, during the sampling period, the air temperature ranged from 10 to 27°C with a mean of 18°C, which may not depress soil fauna growth and reproduction. The SWC ranged from 7 to 31% during the experiment. However, SWCs lower than 10% were only observed in the urban site, which could be attributed to the urbanization effect. Therefore, this study should produce reliable results on the relationships between soil fauna biodiversity and soil physicochemical characteristics.
Data Availability Statement
The original contributions presented in the study are included in the article/Supplementary Material, further inquiries can be directed to the corresponding author.
Author Contributions
GX and ZFW designed the experiment. CL, ZJW, ZL, ML, XF, and YL contributed to the field samples and analyzed the soil physicochemical properties. XC identified the soil fauna. SY and ZJW conducted the statistical analysis and wrote the manuscript. GX, ZFW, and CL revised the manuscript. All authors contributed to the article and approved the submitted version.
Funding
This work was supported by the National Natural Science Foundation of China (42071061 and 42071235) and the Innovation training program for college students of Guangzhou University (202011078001).
Conflict of Interest
The authors declare that the research was conducted in the absence of any commercial or financial relationships that could be construed as a potential conflict of interest.
Publisher’s Note
All claims expressed in this article are solely those of the authors and do not necessarily represent those of their affiliated organizations, or those of the publisher, the editors and the reviewers. Any product that may be evaluated in this article, or claim that may be made by its manufacturer, is not guaranteed or endorsed by the publisher.
Supplementary Material
The Supplementary Material for this article can be found online at: https://www.frontiersin.org/articles/10.3389/fevo.2021.824004/full#supplementary-material
References
Abrego, N., Crosier, B., Somervuo, P., Ivanova, N., Abrahamyan, A., Abdi, A., et al. (2020). Fungal communities decline with urbanization-more in air than in soil. ISME J. 14, 2806–2815. doi: 10.1038/s41396-020-0732-1
Allison, S. D., and Martiny, J. B. H. (2008). Resistance, resilience, and redundancy in microbial communities. Proc. Natl. Acad. Sci. U.S.A. 105, 11512–11519. doi: 10.1073/pnas.0801925105
Anderson, M. J. (2006). Distance-based tests for homogeneity of multivariate dispersions. Biometrics 62, 245–253. doi: 10.1111/j.1541-0420.2005.00440.x
Asabere, S. B., Zeppenfeld, T., Nketia, K. A., and Sauer, D. (2018). Urbanization leads to increases in pH, carbonate, and soil organic matter stocks of arable soils of Kumasi, Ghana (West Africa). Front. Environ. Sci. 6:119. doi: 10.3389/fenvs.2018.00119
Banat, K. M., Howari, F. M., and Al-Hamad, A. A. (2005). Heavy metals in urban soils of central Jordan: should we worry about their environmental risks? Environ. Res. 97, 258–273. doi: 10.1016/j.envres.2004.07.002
Bispo, A., Cluzeau, D., Creamer, R., Dombos, M., Graefe, U., Krogh, P. H., et al. (2009). Indicators for monitoring soil biodiversity. Integr. Environ. Assess. Manag. 5, 717–719. doi: 10.1897/IEAM-2009-064.1
Bogyo, D., Magura, T., Simon, E., and Tothmeresz, B. (2015). Millipede (Diplopoda) assemblages alter drastically by urbanisation. Landsc. Urban Plann. 133, 118–126. doi: 10.1016/j.landurbplan.2014.09.014
Bolger, D. T., Suarez, A. V., Crooks, K. R., Morrison, S. A., and Case, T. J. (2000). Arthropods in urban habitat fragments in southern California: area, age, and edge effects. Ecol. Applic. 10, 1230–1248. doi: 10.1890/1051-0761(2000)010[1230:aiuhfi]2.0.co;2
Braaker, S., Ghazoul, J., Obrist, M. K., and Moretti, M. (2014). Habitat connectivity shapes urban arthropod communities: the key role of green roofs. Ecology 95, 1010–1021. doi: 10.1890/13-0705.1
Byrne, L. B., and Bruns, M. A. (2004). The effects of lawn management on soil microarthropods. J. Agric. Urban Entomol. 21, 150–156.
Chatzinikolaou, E., Mandalakis, M., Damianidis, P., Dailianis, T., Gambineri, S., Rossano, C., et al. (2018). Spatio-temporal benthic biodiversity patterns and pollution pressure in three Mediterranean touristic ports. Sci. Total Environ. 624, 648–660. doi: 10.1016/j.scitotenv.2017.12.111
Coleman, D. C., Crossley, D. A., and Hendrix, P. F. (2004). “4 – secondary production: activities of heterotrophic organisms—the soil fauna,” in Fundamentals of Soil Ecology, 2nd Edn, eds D. C. Coleman, D. A. Crossley, and P. F. Hendrix (Burlington, MA: Academic Press), 79–185. doi: 10.1016/b978-012179726-3/50005-8
Crommentuijn, T., Brils, J., and Vanstraalen, N. M. (1993). Influence of cadmium on life-history characteristics of Folsomia candida (Willem) in an artificial soil substrate. Ecotoxicol. Environ. Saf. 26, 216–227. doi: 10.1006/eesa.1993.1051
Didden, W., and Rombke, J. (2001). Enchytraeids as indicator organisms for chemical stress in terrestrial ecosystems. Ecotoxicol. Environ. Saf. 50, 25–43. doi: 10.1006/eesa.2001.2075
Diego Ibanez-Alamo, J., Rubio, E., Benedetti, Y., and Morelli, F. (2017). Global loss of avian evolutionary uniqueness in urban areas. Glob. Chang. Biol. 23, 2990–2998. doi: 10.1111/gcb.13567
Dufrêne, M., and Legendre, P. (1997). Species assemblages and indicator species:the need for a flexible asymmetrical approach. Ecol. Monogr. 67, 345–366. doi: 10.2307/2963459
Eisenhauer, N., Bonn, A., and Guerra, C. A. (2019). Recognizing the quiet extinction of invertebrates. Nat. Commun. 10:50. doi: 10.1038/s41467-018-07916-1
Ferguson, L. M. (1990). “Insecta: diplura,” in Soil Biology Guide, ed. D. L. Dindal (New York, NY: Wiley), 951–963.
Fiera, C. (2009). Biodiversity of Collembola in urban soils and their use as bioindicators for pollution. Pesqui. Agropecu. Brasil. 44, 868–873.
Fountain, M. T., and Hopkin, S. P. (2005). Folsomia candida (Collembola): a “standard” soil arthropod. Annu. Rev. Entomol. 50, 201–222. doi: 10.1146/annurev.ento.50.071803.130331
Francini, G., Hui, N., Jumpponen, A., Kotze, D. J., Romantschuk, M., Allen, J. A., et al. (2018). Soil biota in boreal urban greenspace: responses to plant type and age. Soil Biol. Biochem. 118, 145–155. doi: 10.1016/j.soilbio.2017.11.019
Gao, D., Wang, F., Li, J., Yu, S., Li, Z., and Zhao, J. (2020). Soil nematode communities as indicators of soil health in different land use types in tropical area. Nematology 22, 595–610. doi: 10.1163/15685411-00003325
Gao, M., Qiao, Z., Hou, H., Jin, G., and Wu, D. (2020). Factors that affect the assembly of ground-dwelling beetles at small scales in primary mixed broadleaved-Korean pine forests in north-east China. Soil Ecol. Lett. 2, 47–60. doi: 10.1007/s42832-019-0018-6
Ge, B., Mehring, A. S., and Levin, L. A. (2019). Urbanization alters belowground invertebrate community structure in semi-arid regions: a comparison of lawns, biofilters and sage scrub. Landsc. Urban Plann. 192:103664. doi: 10.1016/j.landurbplan.2019.103664
Gerlach, J., Samways, M., and Pryke, J. (2013). Terrestrial invertebrates as bioindicators: an overview of available taxonomic groups. J. Insect Conserv. 17, 831–850. doi: 10.1007/s10841-013-9565-9
Gray, J. S. (1989). Effects of environmental-stress on species rich assemblages. Biol. J. Linn. Soc. 37, 19–32. doi: 10.1371/journal.pone.0250725
Herbert, I. N., Svendsen, C., Hankard, P. K., and Spurgeon, D. J. (2004). Comparison of instantaneous rate of population increase and critical-effect estimates in Folsomia candida exposed to four toxicants. Ecotoxicol. Environ. Saf. 57, 175–183.
Hornung, E., Kasler, A., and Toth, Z. (2018). The role of urban forest patches in maintaining isopod diversity (Oniscidea). Zookeys 3, 371–388. doi: 10.3897/zookeys.801.22829
Horvath, R., Magura, T., and Tothmeresz, B. (2012). Ignoring ecological demands masks the real effect of urbanization: a case study of ground-dwelling spiders along a rural-urban gradient in a lowland forest in Hungary. Ecol. Res. 27, 1069–1077. doi: 10.1007/s11284-012-0988-7
Hou, E., Chen, C., Wen, D., and Liu, X. (2014). Relationships of phosphorus fractions to organic carbon content in surface soils in mature subtropical forests, Dinghushan, China. Soil Res. 52, 55–63. doi: 10.1071/sr13204
Jim, C. Y. (1998). Urban soil characteristics and limitations for landscape planting in Hong Kong. Landsc. Urban Plann. 40, 235–249. doi: 10.1016/s0169-2046(97)00117-5
Joimel, S., Schwartz, C., Hedde, M., Kiyota, S., Krogh, P. H., Nahmani, J., et al. (2017). Urban and industrial land uses have a higher soil biological quality than expected from physicochemical quality. Sci. Total Environ. 584, 614–621. doi: 10.1016/j.scitotenv.2017.01.086
Joimel, S., Schwartz, C., Maurel, N., Magnus, B., Machon, N., Bel, J., et al. (2019). Contrasting homogenization patterns of plant and collembolan communities in urban vegetable gardens. Urban Ecosyst. 22, 553–566. doi: 10.1007/s11252-019-00843-z
Jones, E. L., and Leather, S. R. (2012). Invertebrates in urban areas: a review. Eur. J. Entomol. 109, 463–478. doi: 10.14411/eje.2012.060
Kissling, M., Hegetschweiler, K. T., Rusterholz, H.-P., and Baur, B. (2009). Short-term and long-term effects of human trampling on above-ground vegetation, soil density, soil organic matter and soil microbial processes in suburban beech forests. Appl. Soil Ecol. 42, 303–314. doi: 10.1016/j.apsoil.2009.05.008
Knop, E. (2016). Biotic homogenization of three insect groups due to urbanization. Glob. Chang. Biol. 22, 228–236. doi: 10.1111/gcb.13091
LaPoint, S., Balkenhol, N., Hale, J., Sadler, J., and van der Ree, R. (2015). Ecological connectivity research in urban areas. Funct. Ecol. 29, 868–878.
Lepczyk, C. A., Aronson, M. F. J., Evans, K. L., Goddard, M. A., Lerman, S. B., and Macivor, J. S. (2017). Biodiversity in the city: fundamental questions for understanding the ecology of urban green spaces for biodiversity conservation. Bioscience 67, 799–807. doi: 10.1093/biosci/bix079
Liu, K.-L., Peng, M.-H., Hung, Y.-C., and Neoh, K.-B. (2019). Effects of park size, peri-urban forest spillover, and environmental filtering on diversity, structure, and morphology of ant assemblages in urban park. Urban Ecosyst. 22, 643–656. doi: 10.1007/s11252-019-00851-z
Liu, S., Chen, J., Gan, W., Schaefer, D., Gan, J., and Yang, X. (2015). Spider foraging strategy affects trophic cascades under natural and drought conditions. Sci. Rep. 5:12396. doi: 10.1038/srep12396
Lovei, G. L., Horvath, R., Elek, Z., and Magura, T. (2019). Diversity and assemblage filtering in ground-dwelling spiders (Araneae) along an urbanisation gradient in Denmark. Urban Ecosyst. 22, 345–353. doi: 10.1007/s11252-018-0819-x
Maynard, D. G., and Curran, M. P. (2007). “Bulk density measurement in forest soils,” in Soil Sampling and Methods of Analysis, eds M. R. Carter and E. G. Gregorich (Boca Raton, FL: CRC Press), 863–869.
McIntyre, N. E., Rango, J., Fagan, W. F., and Faeth, S. H. (2001). Ground arthropod community structure in a heterogeneous urban environment. Landsc. Urban Plann. 52, 257–274. doi: 10.1016/s0169-2046(00)00122-5
McKinney, M. L. (2006). Urbanization as a major cause of biotic homogenization. Biol. Conserv. 127, 247–260. doi: 10.1016/j.biocon.2005.09.005
Meyer-Wolfarth, F., Oldenburg, E., Meiners, T., Munoz, K., and Schrader, S. (2021). Effects of temperature and soil fauna on the reduction and leaching of deoxynivalenol and zearalenone from Fusarium graminearum-infected maize stubbles. Mycotoxin Res. 37, 249–263. doi: 10.1007/s12550-021-00434-y
Milano, V., Maisto, G., Baldantoni, D., Bellino, A., Bernard, C., Croce, A., et al. (2018). The effect of urban park landscapes on soil Collembola diversity: a Mediterranean case study. Landsc. Urban Plann. 180, 135–147. doi: 10.1016/j.landurbplan.2018.08.008
Morelli, F., Benedetti, Y., Diego Ibanez-Alamo, J., Jokimaki, J., Mand, R., Tryjanowski, P., et al. (2016). Evidence of evolutionary homogenization of bird communities in urban environments across Europe. Glob. Ecol. Biogeogr. 25, 1284–1293. doi: 10.1111/geb.12486
Nagy, D. D., Magura, T., Horvath, R., Debnar, Z., and Tothmeresz, B. (2018). Arthropod assemblages and functional responses along an urbanization gradient: a trait-based multi-taxa approach. Urban For. Urban Green. 30, 157–168. doi: 10.1016/j.ufug.2018.01.002
Nielsen, T. F., Sand-Jensen, K., Dornelas, M., and Bruun, H. H. (2019). More is less: net gain in species richness, but biotic homogenization over 140 years. Ecol. Lett. 22, 1650–1657. doi: 10.1111/ele.13361
Norton, B. A. (2011). The Sanitisation of Urban Ecosystems: Simplification of the Ground Layer in Eucalypt Woodlands and the Effects on Arthropod Communities. Melbourne, VIC: University of Melbourne.
Oksanen, J., Blanchet, F. G., Friendly, M., Kindt, R., Legendre, P., McGlinn, D., et al. (2017). Vegan: Community Ecology Package. R package version 2.4-0.
Papastefanou, G., Panayiotou, E., Mylonas, M., and Simaiakis, S. M. (2015). Centipede assemblages along an urbanization gradient in the city of Heraklion, Crete (Greece). Zookeys 30, 163–179. doi: 10.3897/zookeys.510.8414
Pouyat, R., Groffman, P., Yesilonis, I., and Hernandez, L. (2002). Soil carbon pools and fluxes in urban ecosystems. Environ. Pollut. 116, S107–S118. doi: 10.1016/s0269-7491(01)00263-9
Pouyat, R. V. I, Yesilonis, D., Dombos, M., Szlavecz, K., Setala, H., Cilliers, S., et al. (2015). A global comparison of surface soil characteristics across five cities: a test of the urban ecosystem convergence hypothesis. Soil Sci. 180, 136–145. doi: 10.1097/ss.0000000000000125
Qin, Z., Zhang, J., and Li, Q. (2009). Community structure of soil meso- and micro-fauna in different habitats of urbanized region. J. Appl. Ecol. 20, 3049–3056.
R Core Team (2020). R: A Language and Environment for Statistical Computing. Vienna: R Foundation for Statistical Computing.
Sanchez-Bayo, F., and Wyckhuys, K. A. G. (2019). Worldwide decline of the entomofauna: a review of its drivers. Biol. Conserv. 232, 8–27. doi: 10.1016/j.biocon.2019.01.020
Santorufo, L., Van Gestel, C. A. M., Rocco, A., and Maisto, G. (2012). Soil invertebrates as bioindicators of urban soil quality. Environ. Pollut. 161, 57–63. doi: 10.1016/j.envpol.2011.09.042
Scheller, U. (2002). “Pauropoda,” in Soil Biology Guide, ed. D. L. Dindal (New York, NY: Wiley), 861–890.
Shi, B., Tang, C.-S., Gao, L., Liu, C., and Wang, B.-J. (2012). Observation and analysis of the urban heat island effect on soil in Nanjing, China. Environ. Earth Sci. 67, 215–229. doi: 10.1007/s12665-011-1501-2
Smetak, K. M., Johnsn-Maynard, J. L., and Lloyd, J. E. (2007). Earthworm population density and diversity in different-aged urban systems. Appl. Soil Ecol. 37, 161–168. doi: 10.1016/j.apsoil.2007.06.004
Sterzynska, M., Nicia, P., Zadrozny, P., Fiera, C., Shrubovych, J., and Ulrich, W. (2018). Urban springtail species richness decreases with increasing air pollution. Ecol. Indic. 94, 328–335. doi: 10.1016/j.ecolind.2018.06.063
Szlavecz, K., Vilisics, F., Toth, Z., and Hornung, E. (2018). Terrestrial isopods in urban environments: an overview. Zookeys 2018, 97–126. doi: 10.3897/zookeys.801.29580
Tothmeresz, B., Mathe, I., Balazs, E., and Magura, T. (2011). Responses of carabid beetles to urbanization in Transylvania (Romania). Landsc. Urban Plann. 101, 330–337. doi: 10.1016/j.landurbplan.2011.02.038
Trammell, T. L. E., Pataki, D. E., Pouyat, R. V., Groffman, P. M., Rosier, C., Bettez, N., et al. (2020). Urban soil carbon and nitrogen converge at a continental scale. Ecol. Monogr. 90:e01401.
Tresch, S., Frey, D., Bayon, R.-C. L., Mäder, P., Stehle, B., Fliessbach, A., et al. (2019). Direct and indirect effects of urban gardening on aboveground and belowground diversity influencing soil multifunctionality. Sci. Rep. 9:9769. doi: 10.1038/s41598-019-46024-y
Tresch, S., Moretti, M., Le Bayon, R. C., Mader, P., Zanetta, A., Frey, D., et al. (2018). A Gardener’s influence on urban soil quality. Front. Environ. Sci. 6:25. doi: 10.3389/fenvs.2018.00025
van Straalen, N. M. (1998). Evaluation of bioindicator systems derived from soil arthropod communities. J. Appl. Soil Ecol. 9, 429–437. doi: 10.1016/s0929-1393(98)00101-2
van Vliet, P. C. J. (2000). “Enchytraeids,” in Handbook of Soil Science, ed. M. Sumner (Boca Raton, FL: CRC Press), 70–77.
Vellend, M. (2010). Conceptual synthesis in community ecology. Q. Rev. Biol. 85, 183–206. doi: 10.1086/652373
Veresoglou, S. D., Halley, J. M., and Rillig, M. C. (2015). Extinction risk of soil biota. Nat. Commun. 6:8862. doi: 10.1038/ncomms9862
Wang, J., and Tong, X. (2012). Species diversity, seasonal dynamics, and vertical distribution of litter-dwelling thrips in an urban forest remnant of South China. J. Insect Sci. 12:67. doi: 10.1673/031.012.6701
Wardle, D. A., Bardgett, R. D., Klironomos, J. N., Setala, H., van der Putten, W. H., and Wall, D. H. (2004). Ecological linkages between aboveground and belowground biota. Science 304, 1629–1633. doi: 10.1126/science.1094875
Wei, B., and Yang, L. (2010). A review of heavy metal contaminations in urban soils, urban road dusts and agricultural soils from China. Microchem. J. 94, 99–107. doi: 10.1016/j.microc.2009.09.014
Wolters, V. (2001). Biodiversity of soil animals and its function. Eur. J. Soil Biol. 37, 221–227. doi: 10.1016/s1164-5563(01)01088-3
Xie, T., Wang, M., Chen, W., and Uwizeyimana, H. (2018). Impacts of urbanization and landscape patterns on the earthworm communities in residential areas in Beijing. Sci. Total Environ. 626, 1261–1269. doi: 10.1016/j.scitotenv.2018.01.187
Yu, S., Qiu, J., Chen, X., Luo, X., Yang, X., Wang, F., et al. (2021). Soil mesofauna community changes in response to the environmental gradients of urbanization in Guangzhou City. Front. Ecol. Evol. 8:546433. doi: 10.3389/fevo.2020.546433
Zhao, J., Wang, X. L., Shao, Y. H., Xu, G. L., and Fu, S. L. (2011). Effects of vegetation removal on soil properties and decomposer organisms. Soil Biol. Biochem. 43, 954–960. doi: 10.1016/j.soilbio.2011.01.010
Keywords: soil biodiversity, urban biodiversity, soil invertebrate, global change, urbanization
Citation: Yu S, Wu Z, Xu G, Li C, Wu Z, Li Z, Chen X, Lin M, Fang X and Lin Y (2022) Inconsistent Patterns of Soil Fauna Biodiversity and Soil Physicochemical Characteristic Along an Urbanization Gradient. Front. Ecol. Evol. 9:824004. doi: 10.3389/fevo.2021.824004
Received: 28 November 2021; Accepted: 27 December 2021;
Published: 31 January 2022.
Edited by:
Tian Zhao, Key Laboratory of Mountain Ecological Rehabilitation and Biological Resource Utilization, Chengdu Institute of Biology, CAS, ChinaReviewed by:
Shengjie Liu, Xishuangbanna Tropical Botanical Garden, CAS, ChinaSanjukta Manna, University of Calcutta, India
Copyright © 2022 Yu, Wu, Xu, Li, Wu, Li, Chen, Lin, Fang and Lin. This is an open-access article distributed under the terms of the Creative Commons Attribution License (CC BY). The use, distribution or reproduction in other forums is permitted, provided the original author(s) and the copyright owner(s) are credited and that the original publication in this journal is cited, in accordance with accepted academic practice. No use, distribution or reproduction is permitted which does not comply with these terms.
*Correspondence: Guoliang Xu, xugl@gzhu.edu.cn