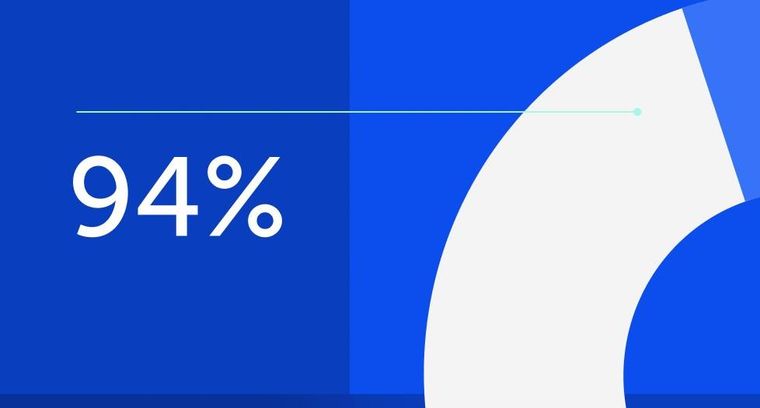
94% of researchers rate our articles as excellent or good
Learn more about the work of our research integrity team to safeguard the quality of each article we publish.
Find out more
MINI REVIEW article
Front. Ecol. Evol., 23 December 2021
Sec. Conservation and Restoration Ecology
Volume 9 - 2021 | https://doi.org/10.3389/fevo.2021.816374
This article is part of the Research TopicEcology, Conservation, and Restoration of Grazing Ecosystems in the AnthropoceneView all 12 articles
Regenerative grazing management (ReGM) seeks to mimic natural grazing dynamics to restore degraded soils and the ecological processes underpinning sustainable livestock production while enhancing biodiversity. Regenerative grazing, including holistic planned grazing and related methods, is an adaptive, rotational stocking approach in which dense livestock herds are rotated rapidly through multiple paddocks in short bouts of grazing to defoliate plants evenly and infrequently, interspersed with long recovery periods to boost regrowth. The concentrated “hoof action” of herds in ReGM is regarded vital for regenerating soils and ecosystem services. Evidence (from 58 studies) that ReGM benefits biodiversity is reviewed. Soils enriched by ReGM have increased microbial bioactivity, higher fungal:bacteria biomass, greater functional diversity, and richer microarthropods and macrofauna communities. Vegetation responds inconsistently, with increased, neutral, or decreased total plant diversity, richness of forage grasses and invasive species under ReGM: grasses tend to be favored but shrubs and forbs can be depleted by the mechanical action of hooves. Trampling also reduces numerous arthropods by altering vegetation structure, but creates favorable habitat and food for a few taxa, such as dung beetles. Similarly, grazing-induced structural changes benefit some birds (for foraging, nest sites) while heavy stocking during winter and droughts reduces food for seedeaters and songbirds. With herding and no fences, wildlife (herbivores and predators) thrives on nutritious regrowth while having access to large undisturbed areas. It is concluded that ReGM does not universally promote biodiversity but can be adapted to provide greater landscape habitat heterogeneity suitable to a wider range of biota.
Regenerative Agriculture is a broad movement that has rapidly gained prominence in the agricultural community as well as in the popular discourse and academic literature over the last 5–6 years (Massy, 2017; Lal, 2020; Giller et al., 2021). Though not formally or consistently defined (Newton et al., 2020), regenerative farming aims to provide more environmentally benign alternatives to conventional agricultural approaches to meet growing food needs while addressing critical global problems of stagnating crop yields, diminishing food quality and security, and widespread soil degradation and increased desertification (Rhodes, 2017; LaCanne and Lundgren, 2018). Restoring soil health and multifunctionality, particularly microbial activity, nutrient recycling, and carbon and water retention, is regarded as critical for developing sustainable and resilient farming systems (Sherwood and Uphoff, 2000; Schreefel et al., 2020). Sequestering atmospheric carbon into soils to mitigate climate change as well as nurturing and promoting biodiversity are also stated goals of regenerative agriculture (Gosnell et al., 2019; Newton et al., 2020). A variety of agronomic practices are considered regenerative, including mainstream and alternative agronomic practices such as minimum-till or conservation agriculture, cover- and rotational cropping, integrated crop-livestock and agroforestry systems, permaculture, organic farming, etc. (Francis et al., 1986; White, 2020). Domestic livestock can also be employed using regenerative grazing management (ReGM) to revitalize soils and grazing ecosystems (Lal, 2020).
Regenerative grazing management (hereafter abbreviated as ReGM) is an adaptive form of intensive grazing management (Teague and Kreuter, 2020; Spratt et al., 2021) in which the timing and distribution (density) of livestock grazing is carefully planned, managed, and monitored with the aim of improving rangeland productivity and overall ranching system resilience (Garnett et al., 2017; Teague and Barnes, 2017). In essence, ReGM represents a rebranding of Holistic Planned Grazing (HPG) developed by Alan Savory in southern Africa more than half-a-century ago (Savory and Parsons, 1980; Gosnell et al., 2020) and which is now applied on rangeland in many parts of the world (Teague and Kreuter, 2020). Holistic Planned Grazing, and hence ReGM, is characterized by the stocking of large herds of livestock on multiple small permanent or temporary paddocks for short periods (less than 1 day to few days) followed by long periods of recovery rest (many weeks to months) to mimic the rapid movement of concentrated ungulate herds being chased across the African landscape by predators (Savory and Butterfield, 2016). The fundamental principles of ReGM are to limit the duration of grazing to avoid regrazing of forage plants and to employ the “herd effect” to trample down dead plants, break up hard soil crusts, and incorporate dung, urine, and plant organic matter into soils to improve soil carbon, increase water infiltration and retention, and accelerate nutrient flow for grass regrowth (Savory and Butterfield, 2016; Teague and Barnes, 2017). Other grazing practices that employ a similar intensive, adaptive rotational stocking approach to regenerate rangeland or cultivated pastures include adaptive multi-paddock grazing (AMP), high-density-short-duration grazing, [ultra] high intensity grazing, mob grazing, cell grazing, time-controlled grazing, and management intensive [planned] grazing. Also common to HPG and related regenerative grazing methods is the consistent claim that they have a significantly greater potential than conventional, less intensive, grazing systems to improve rangeland and livestock condition, productivity, and biodiversity, and slow or even reverse climate change by the sequestration of large amounts of carbon into soils, even at much higher stocking rates than usually prescribed (Savory, 20131; Savory and Butterfield, 2016).
Claims about the ubiquitous superiority of ReGM are controversial, divisive, and strongly contested (see Briske et al., 2013; Sherren and Kent, 2019; Gosnell et al., 2020), spurring numerous studies, reviews (Skovlin, 1987; Holechek et al., 2000; Briske et al., 2008; Nordborg, 2016; Hawkins et al., 2022) and a meta-analysis (Hawkins, 2017). These studies and syntheses have revealed varied ecosystem and agronomic responses to ReGM with little compelling empirical evidence that ReGM will improve grass and animal production and vegetation condition wherever it is applied. What has seldom been examined, however, is the assertion that the stimulation of soil and vegetation productivity by ReGM will also improve the availability and quality of resources and habitats for multifarious flora and fauna, thereby promoting multi-taxa biodiversity; only two instances of soil biodiversity responses were reported in reviews. Biodiversity (species richness and diversity indices) is included as key indicator for verifying the success of ReGM (Savory Institute, 2019) and is valued by regenerative ranchers as a fundamental driver of the ecological and economic sustainability of their farm (Stinner et al., 1997) but little information is available on the effects of intensive, infrequent grazing on different biota (Carter et al., 2014) and what synergies exist and possible trade-offs will be required to simultaneously achieve high livestock production and biodiversity conservation (Lawrence, 2019). To start filling this gap, this review examined 58 studies (see Supplementary Data Sheet 1—Search strategy) on the positive, negative, or neutral effects on the diversity of soil microbes, plants, invertebrates, birds, and mammals done in North America (26), Africa (17), Australia (10), South America (3), and New Zealand (2). Studies included temporal changes under ReGM, comparisons with less intensive rotational, continuous, or deferred grazing systems, or ungrazed plots or properties or nature reserves (see study details—Supplementary Table 1). The effects on biodiversity of dense herds of livestock penned in moveable corrals used for rehabilitating areas and as a home base for ReGM were also reviewed. Mechanism whereby different disturbances exerted by intense grazing (trampling, grazing, nutrient addition) directly or indirectly affect biodiversity are summarized followed by a brief consideration of how ReGM could be improved to better benefit biodiversity.
The number of positive, negative, and neutral responses of various biota to ReGM is presented in Figure 1.
Figure 1. Count of positive, negative, and neutral responses of soil organisms (A), plants (B), large mammals (C), invertebrates (D), and birds (E) to regenerative grazing.
Herpetofaunal diversity was unaffected by ReGM in Africa (Fabricius et al., 2003) and Australia (Dorrough et al., 2012; Kay et al., 2017), and is not discussed further.
Regenerative grazing generally promoted soil microbial activity. Compared to soils in ungrazed or less intensively grazed areas, soils under ReGM had larger and more active microbial biomass, especially of fungi (Beukes and Cowling, 2003; Teague et al., 2011; Girard-Cartier and Kleppel, 2017; Kleppel, 2019), and similar (Girard-Cartier and Kleppel, 2017) or higher microbe group functional diversity (Kleppel, 2019). A higher fungi : bacteria ratio would enhance the retention and availability of soil water and nutrients for microbes and plant growth (Teague et al., 2011). In contrast, Dormaar et al. (1989) recorded lower soil fungal biomass than in exclosures after more than 10 years of ReGM, in concert with poorer soil water functionality and reduced vegetation condition.
Improved soil health under ReGM had positive or neutral effects on soil micro- and macrofauna. Teutscherová et al. (2021) found that a single year of ReGM increased overall macrofaunal diversity and the abundance of earthworms, beetles, and other invertebrates such as ants, spiders, woodlice, and earwigs, which enhanced soil structure through bioturbation. In turn, improved soil structure (e.g., greater porosity) and chemistry (e.g., more organic matter) under intensive stocking benefited soil-dwelling and epigeic arthropods (Tom et al., 2006; Moulin et al., 2016) but not nematodes and protozoa (Teague et al., 2011).
The influence of ReGM on the diversity of plant species varied widely in direction and magnitude. Species richness and diversity was unaffected by ReGM in sown pastures (Scott, 2001), semi-arid grassland (Weigel et al., 1989; Hillenbrand et al., 2019; Oliva et al., 2021), semi-arid shrubland (Beukes and Cowling, 2000), and mesic (>650 mm a–1 MAP) grassland (Jacobo et al., 2006; Dorrough et al., 2012; Chamane et al., 2017a; Kurtz et al., 2018). Reduction in species richness under ReGM ranged from 10% (Hall et al., 2014) to over 80% (Scott-Shaw and Morris, 2015), with grasses (Allington and Vallone, 2011), forbs (Lawrence, 2019) and shrubs (McManus et al., 2018) negatively affected by intense grazing and trampling, especially during droughts (Souther et al., 2020). Regenerative grazing increased plant species (Earl et al., 2003) and life-form diversity (Barnes and Howell, 2013) over time compared to ungrazed areas (Paine and Ribic, 2002; Girard-Cartier and Kleppel, 2017) as well as to rangeland grazed continuously (Lalampaa, 2016; Odadi et al., 2017; Rantso et al., 2021; Wang et al., 2021) or rotationally at a lower intensity (Laliberté and Tylianakis, 2012).
In general, ReGM promoted the diversity and abundance of perennial, productive forage grasses (Stinner et al., 1997; Chamane et al., 2017a; Huruba et al., 2018) over perennial forbs (Loeser et al., 2007; Morris and Scott-Shaw, 2019), and exotic over native species (Ruthven, 2007), although intense stocking can be employed to target invasive weeds (Girard-Cartier and Kleppel, 2015).
Increased plant species richness under ReGM may not be agronomically or ecologically desirable if unpalatable and less productive forage grasses and non-native ruderal species are favored (e.g., Chamane et al., 2017a; Souther et al., 2020).
The response of insects and arachnids to ReGM varies between taxa, season, and habitat quality (Lindsay and Cunningham, 2009; Barton et al., 2016). For example, various insect (e.g., ants, crickets, beetles, flies; grasshoppers, parasitoid hymenopterans) and arachnids were less abundant and speciose under ReGM but Hemiptera benefited from reduced vegetation cover (Fabricius et al., 2003; Debano, 2006; Lawrence et al., 2015). Fire ants invaded intensively grazed sites but did not reduce arthropod diversity (Schmid and Lundgren, 2020). Regenerative grazing increased habitat heterogeneity and food for dung beetles (Wagner et al., 2020) but destroyed spider webs and the grass structure upon which they are built; ground-dwelling arachnids were unaffected (Sebata, 2020). Invertebrates thrived where the biomass and cover of shrubs increased because of restricted grazing in riparian paddocks, resulting in higher inputs of invertebrate food into streams for trout, doubling their mass (Saunders and Fausch, 2007).
Regenerative grazing indirectly affects bird populations by modifying habitat structure and food availability. Ground-foraging birds such as quail can more successfully forage, and thus flourish, where ReGM reduces standing herbage, litter, and cover (Schulz and Guthery, 1988; Wilkins and Swank, 1992). In contrast, intense grazing reduced food reserves and cover for overwintering birds, especially during drought, decreasing their numbers and diversity (Bock and Bock, 1999). Timing is important: heavy stocking in the dormant season when food is scare reduced songbird diversity and species richness (Sliwinski et al., 2020). Applying a [shifting] range of grazing intensities across the landscape to diversify vegetation structure, including trees for birds (Dorrough et al., 2012), is key for promoting avian diversity (Davis et al., 2020) but ReGM as well as other grazing systems do not create sufficient habitat heterogeneity to suite a wide variety of birds species (Sliwinski et al., 2019).
The abundance, diversity of mammalian herbivores is increased at nutrient hotspots created by overnight corralling (Huruba et al., 2021a,b) and on the wider rangeland managed under ReGM because of improved grass and browse quality (Lalampaa et al., 2016; Odadi et al., 2017; Crawford et al., 2019). Similarly, applying ReGM by herding (from temporary corrals) rather than by fencing, combined with a cessation of lethal predator control led to a remarkable increase over 4 years in mammalian species richness (by 24%), particularly herbivores (+33%), and the abundance and distribution range of wildlife in a semi-arid shrubland in South Africa (Schurch et al., 2021).
The only study on small mammals revealed no general effect of ReGM on rodent diversity but that some species were sensitive to intensive grazing in particular habitats (Lemos, 2014).
The three central tenets of ReGM practices regarded essential for enhanced ecosystem function and profitable livestock production—(1) the “hoof” effect on soils and vegetation, (2) even grazing for a short duration with long recovery rests, and (3) recycling and redistribution of nutrients through animal excreta (Savory and Butterfield, 2016)—can indirectly or directly favor or harm biodiversity (Table 1). The same type of disturbance can have contrasting effects on different taxa and even on species within taxa.
Table 1. A summary of the positive and negative effects of regenerative grazing practices on biodiversity.
Excessive trampling, especially by cattle, generally degrades soil structure and function, although the effects are contingent on numerous site-specific factors such as soil texture and moisture, terrain, and vegetation cover (Trimble and Mendel, 1995; Bilotta et al., 2007; Byrnes et al., 2018). The contrasting reported improvements in soil health under ReGM that indirectly benefit soil biota (see Soil Organisms) could owe to the limited duration of trampling that soils experience, the breaking of impermeable hardened soil caps, and the incorporation of organic material from plants and animal excreta into the topsoil (Mwendera et al., 1997; Teague and Kreuter, 2020; Mor-Mussery et al., 2021). Trampling can also create suitable seedbeds (Huruba et al., 2018) and open the sward for ground-foraging birds (see Birds).
Plants exposed to trampling can be severely and extensively impacted; for example, fewer than 10% of forb species escaped mechanical damage to their above-ground tissues under ReGM (Chamane et al., 2017b). Tall plants, particularly soft-leaved forbs with elevated growing points are most vulnerable to trampling injury (Sun and Liddle, 1993; Morris and Scott-Shaw, 2019), which reduces their vigor and potential persistence (Morris, 2021). Grass canopies reduced or modified by trampling offer inferior habitat for arthropods, birds, and mammals that require tall swards for nesting or shelter (Fuhlendorf et al., 2006; Schieltz and Rubenstein, 2016; Oyarzabal and Guimarães, 2021) and herbivores can also inadvertently ingest instars and adult arthropods (Van Noordwijk et al., 2012; Wang and Tang, 2019). Hooves directly impact and reduce the cover of biological soil crusts (Eldridge, 1998).
In ReGM system, the long uninterrupted periods afforded plants and animals to recover from defoliation and trampling probably contribute more to the observed neutral and positive responses of individuals and populations than the restricted periods of stocking of small paddocks or areas employed to minimize selective grazing of palatable forage plants and to prevent regrazing of grass (Ferraro and Oesterheld, 2002; Barton et al., 2016; Porensky et al., 2021). Importantly, confining livestock to a small proportion of the grazing area at any one time minimizes the spatial extent and duration of their disturbance of other animals on the property (Schurch et al., 2021).
Despite high stocking densities and grazing pressures, a uniform defoliation intensity across plant species and vegetation patches is not easily achieved using ReGM (Venter et al., 2019). Selective grazing of species and areas can favor less palatable and more grazing resistant plants, altering vegetation composition (Anderson and Briske, 1995; Augustine and McNaughton, 1998). Increased paddock subdivision does not alter grazing selectivity to the degree expected (Gammon and Twiddy, 1990) nor can it prevent the ingress of unpalatable grasses that are selectively avoided by livestock even under intense stocking (Morris and Tainton, 1996).
Controlled rotation of livestock combined with intense trampling is predicted to distribute their excreta evenly across the landscape and enrich soils with carbon and nutrients recycled from plant tissues (Savory and Butterfield, 2016). Microbial and coprophagous invertebrate populations benefit from the modest levels of carbon sequestration (see Soils) achievable under ReGM (Hawkins et al., 2022), and plants are better able to compensate for defoliation on, fertile, organic-rich soils (Maschinski and Whitham, 1989; Venter et al., 2021). Nutrients imported by penned livestock create fertility hotspots (Huruba et al., 2018) which can kickstart restoration of a species-rich, palatable grass sward (Sibanda et al., 2016) but the potential for creating loci for non-native species invasion requires investigation. Also unknown is whether increased enrichment and grass productivity under ReGM (Teague and Kreuter, 2020) could eventually dimmish plant species diversity by favoring dominant over subordinate grasses and broadleaf species (Harpole et al., 2016).
Given the variable responses within and between taxa, it is concluded that ReGM is not universally beneficial to all biodiversity, as claimed. The assumption that any bottom-up stimulation of soil ecosystem processes and plant production by ReGM will inevitably cascade positively through the whole rangeland ecosystem to support larger and more diverse populations of all fauna and fauna is not supported. Furthermore, some direct and indirect impacts of ReGM, particularly trampling, can be pernicious and persistent for some biota. Relentless application of a uniform disturbance comprising short bouts of intense grazing and trampling across the whole property could homogenize vegetation communities and landscapes (Loeser et al., 2007; Sliwinski et al., 2020) reducing the spatial habitat heterogeneity required to sustain high biodiversity (Fuhlendorf and Engle, 2001; Benton et al., 2003). Nonetheless, the multi-paddock, controlled rotational stocking system employed in ReGM could be adapted (Teague et al., 2013) to both mitigate negative disturbances on plants and animals by applying more moderate stocking densities over most of the property (Joubert et al., 2017; Barzan et al., 2021) while at the same time creating a more heterogenous grazed landscape with habitats and living conditions suitable to a broader range of species and communities.
To engender greater landscape heterogeneity at all scales (Toombs et al., 2010), stocking density and duration and timing of grazing would need to be deliberately varied over space and time to provide a shifting mosaic of disturbance intensities that includes extremes (Sliwinski et al., 2020; Porensky et al., 2021) ranging from “overgrazed” bare patches to lightly grazed or protected paddocks especially in sensitive riparian zones (Paine and Ribic, 2002; Saunders and Fausch, 2007) and on steep slopes (Trimble and Mendel, 1995). Also important to include are other ecosystem management tools often eschewed by regenerative grazers (Savory and Butterfield, 2016), notably planned burning and long rests. Fire is essential to maintain the vegetation and of fire-dependant ecosystems such as mesic grassland and savanna (Bond, 2019; Gordijn and O’Connor, 2021), and when coupled with grazing can increase structural heterogeneity (Lituma et al., 2022) and contribute toward achieving the dual goals of biodiversity conservation and profitable cattle production (Limb et al., 2011; Bowman et al., 2016). Extended rests (of a year or longer) increase rangeland production (McDonald et al., 2019) and could create suitable habitat and grassbanks for particular bird species (Davis et al., 2020) while being restorative for animal and plant individuals and populations impacted by intense herbivory (Kirkman and Moore, 1995; Morris, 2021).
A carefully planned strategic approach would be required when adapting ReGM to better nurture and sustain diverse biological communities on ranches and in other grazing areas (Wang and Tang, 2019). The grazing strategy could consider approaches noted above that could generally promote biodiversity as well as specific management tactics targeting species and communities on the property of special conservation concern (Barry and Huntsinger, 2021).
The author confirms being the sole contributor of this work and has approved it for publication.
The author declares that the research was conducted in the absence of any commercial or financial relationships that could be construed as a potential conflict of interest.
All claims expressed in this article are solely those of the authors and do not necessarily represent those of their affiliated organizations, or those of the publisher, the editors and the reviewers. Any product that may be evaluated in this article, or claim that may be made by its manufacturer, is not guaranteed or endorsed by the publisher.
The Supplementary Material for this article can be found online at: https://www.frontiersin.org/articles/10.3389/fevo.2021.816374/full#supplementary-material
Allington, G. R., and Vallone, T. J. (2011). Long-term livestock exclusion in an arid grassland alters vegetation and soil. Range. Ecol. Manage. 64, 424–428. doi: 10.2111/REM-D-10-00098.1
Anderson, V. J., and Briske, D. D. (1995). Herbivore-induced species replacement in grasslands: is it driven by herbivory tolerance or avoidance? Ecol. Appl. 5, 1014–1024. doi: 10.2307/2269351
Augustine, D. J., and McNaughton, S. J. (1998). Ungulate effects on the functional species composition of plant communities: herbivore selectivity and plant tolerance. J. Wildl. Manage. 62, 1165–1183. doi: 10.2307/3801981
Barnes, M., and Howell, J. (2013). Multiple-paddock grazing distributes utilization across heterogeneous mountain landscapes: a case study of strategic grazing management. Rangelands 35, 52–61. doi: 10.2111/RANGELANDS-D-13-00019.1
Barry, S., and Huntsinger, L. (2021). Rangeland land-sharing, livestock grazing’s role in the conservation of imperiled species. Sustainability 13:4466. doi: 10.3390/su13084466
Barton, P. S., Sato, C. F., Kay, G. M., Florance, D., and Lindenmayer, D. B. (2016). Effects of environmental variation and livestock grazing on ant community structure in temperate eucalypt woodlands. Insect Conserv. Divers. 9, 124–134. doi: 10.1111/icad.12151
Barzan, F. R., Bellis, L. M., and Dardanelli, S. (2021). Livestock grazing constraints bird abundance and species richness: a global meta-analysis. Basic Appl. Ecol. 56, 289–298. doi: 10.1016/j.baae.2021.08.007
Benton, T. G., Vickery, J. A., and Wilson, J. D. (2003). Farmland biodiversity: is habitat heterogeneity the key? Trends Ecol. Evol. 18, 182–188. doi: 10.1016/S0169-5347(03)00011-9
Beukes, P. C., and Cowling, R. M. (2000). Impacts of non-selective grazing on cover, composition, and productivity of Nama-karoo grassy shrubland. Afr. J. Range Forage Sci. 17, 27–35. doi: 10.2989/10220110009485736
Beukes, P. C., and Cowling, R. M. (2003). Non-selective grazing impacts on soil-properties of the Nama Karoo. Range. Ecol. Manage. 56, 547–552. doi: 10.2307/4003849
Bilotta, G. S., Brazier, R. E., and Haygarth, P. M. (2007). The impacts of grazing animals on the quality of soils, vegetation, and surface waters in intensively managed grasslands. Adv. Agron. 94, 237–280. doi: 10.1016/S0065-2113(06)94006-1
Bock, C. E., and Bock, J. H. (1999). Response of winter birds to drought and short-duration grazing in southeastern Arizona. Conserv. Biol. 13, 1117–1123. doi: 10.1046/J.1523-1739.1999.98313.X
Bond, W. J. (2019). Open Ecosystems: Ecology and Evolution Beyond the Forest Edge. Oxford: Oxford University Press.
Bowman, D. M., Perry, G. L., Higgins, S. I., Johnson, C. N., Fuhlendorf, S. D., and Murphy, B. P. (2016). Pyrodiversity is the coupling of biodiversity and fire regimes in food webs. Philos. Trans. R. Soc. Lond B Biol. Sci. 371:20150169. doi: 10.1098/rstb.2015.0169
Briske, D. D., Bestelmeyer, B. T., Brown, J. R., Fuhlendorf, S. D., and Polley, H. W. (2013). The savory method cannot green deserts or reverse climate change. Rangelands 35, 72–74.
Briske, D. D., Derner, J. D., Brown, J. R., Fuhlendorf, S. D., Teague, W. R., Havstad, K. M., et al. (2008). Rotational grazing on rangelands: reconciliation of perception and experimental evidence. Range. Ecol. Manage. 61, 3–17. doi: 10.2111/06-159R.1
Byrnes, R. C., Eastburn, D. J., Tate, K. W., and Roche, L. M. (2018). A global meta-analysis of grazing impacts on soil health indicators. J. Environ. Qual. 47, 758–765. doi: 10.2134/jeq2017.08.0313
Carter, J., Jones, A., O’Brien, M., Ratner, J., and Wuerthner, G. (2014). Holistic management: misinformation on the science of grazed ecosystems. Int. J. Biodivers. 2014, 163431. doi: 10.1155/2014/163431
Chamane, S., Kirkman, K. P., Morris, C., and O’Connor, T. (2017a). Does high-density stocking affect perennial forbs in mesic grassland? Afr. J. Range Forage Sci. 34, 133–142. doi: 10.2989/10220119.2017.1323008
Chamane, S., Kirkman, K. P., Morris, C., and O’Connor, T. (2017b). What are the long-term effects of high-density, short-duration stocking on the soils and vegetation of mesic grassland in South Africa? Afr. J. Range Forage Sci. 34, 111–121. doi: 10.2989/10220119.2017.1364295
Crawford, C. L., Volenec, Z. M., Sisanya, M., Kibet, R., and Rubenstein, D. I. (2019). Behavioral and ecological implications of bunched, rotational cattle grazing in East African Savanna ecosystem. Range. Ecol. Manage. 72, 204–209. doi: 10.1016/j.rama.2018.07.016
Davis, K. P., Augustine, D. J., Monroe, A. P., Derner, J. D., and Aldridge, C. L. (2020). Adaptive rangeland management benefits grassland birds utilizing opposing vegetation structure in the shortgrass steppe. Ecol. Appl. 30:e02020. doi: 10.1002/eap.2020
Debano, S. J. (2006). Effects of livestock grazing on aboveground insect communities in semi-arid grasslands of southeastern Arizona. Biodivers. Conserv. 15, 2547–2564. doi: 10.1007/978-1-4020-5283-5_12
Dormaar, J. F., Smoliak, S., and Willms, W. D. (1989). Vegetation and soil responses to short-duration grazing on fescue grasslands. Range. Ecol. Manage 42, 252–256. doi: 10.2307/3899484
Dorrough, J., McIntyre, S., Brown, G., Stol, J., Barrett, G., and Brown, A. (2012). Differential responses of plants, reptiles and birds to grazing management, fertilizer and tree clearing. Austral Ecol. 37, 569–582. doi: 10.1111/j.1442-9993.2011.02317.x
Earl, J. M., Kahn, L., and Nicholls, M. (2003). “Changes in grassland composition with grazing management in the mid-north of South Australia: continuous, rotational and pulse grazing,” in Proceedings of the 3rd National Conference on the Management of Native Grasses and Pastures, eds C. O’Dwyer and S. Hamilton (Dookie: University of Melbourne), 161–163.
Eldridge, D. J. (1998). Trampling of microphytic crusts on calcareous soils, and its impact on erosion under rain-impacted flow. Catena 33, 221–239. doi: 10.1016/S0341-8162(98)00075-7
Fabricius, C., Burger, M., and Hockey, P. A. R. (2003). Comparing biodiversity between protected areas and adjacent rangeland in xeric succulent thicket, South Africa: arthropods and reptiles. J. Appl. Ecol. 40, 392–403. doi: 10.1046/j.1365-2664.2003.00793.x
Ferraro, D. O., and Oesterheld, M. (2002). Effect of defoliation on grass growth: a quantitative review. Oikos 98, 125–133. doi: 10.1034/j.1600-0706.2002.980113.x
Francis, C. A., Harwood, R. R., and Parr, J. F. (1986). The potential for regenerative agriculture in the developing world. Am. J. Altern. Agric. 1, 65–74. doi: 10.1017/S0889189300000904
Fuhlendorf, S. D., and Engle, D. M. (2001). Restoring heterogeneity on rangelands: ecosystem management based on evolutionary grazing patterns. BioScience 51, 625–632.
Fuhlendorf, S. D., Harrell, W. C., Engle, D. M., Hamilton, R. G., Davis, C. A., and Leslie, D. M. Jr. (2006). Should heterogeneity be the basis for conservation? Grassland bird response to fire and grazing. Ecol. Appl. 16, 1706–1716.
Gammon, D. M., and Twiddy, D. R. (1990). Patterns of defoliation in four-and eight-paddock grazing systems. J. Grassl. Soc. South. Afr. 7, 29–35. doi: 10.1080/02566702.1990.9648201
Garnett, T., Godde, C., Muller, A., Röös, E., Smith, P., de Boer, I., et al. (2017). Grazed and Confused. Oxford: Food Climate Research Network.
Giller, K. E., Hijbeek, R., Andersson, J. A., and Sumberg, J. (2021). Regenerative agriculture: an agronomic perspective. Outlook Agric. 50, 13–25. doi: 10.1177/0030727021998063
Girard-Cartier, C. B., and Kleppel, G. S. (2015). Grazing as a control for the spread of mile-a-minute (persicaria perfoliata) and the restoration of biodiversity in plant communities in a lower New York state parkland. Ecol. Restor. 33, 82–89. doi: 10.3368/er.33.1.82
Girard-Cartier, C. B., and Kleppel, G. S. (2017). Grazing and the coupling of biodiversity in vascular plant and soil microbial communities. Northeast. Nat. 24, 67–85. doi: 10.1656/045.024.0sp806
Gordijn, P. J., and O’Connor, T. G. (2021). Multidecadal effects of fire in a grassland biodiversity hotspot: does pyrodiversity enhance plant diversity? Ecol. Appl. 31, e02391. doi: 10.1002/eap.2391
Gosnell, H., Gill, N., and Voyer, M. (2019). Transformational adaptation on the farm: processes of change and persistence in transitions to ‘climate-smart’ regenerative agriculture. Global Environ. Change 59:101965. doi: 10.1016/j.gloenvcha.2019.101965
Gosnell, H., Grimm, K., and Goldstein, B. E. (2020). A half century of Holistic management: what does the evidence reveal? Agric. Hum. Values 37, 849–867. doi: 10.1007/s10460-020-10016-w
Hall, T. J., McIvor, J. G., Reid, D. J., Jones, P., MacLeod, N. D., McDonald, C. K., et al. (2014). A comparison of stocking methods for beef production in northern Australia: pasture and soil surface condition responses. Rangel 36, 161–174. doi: 10.1071/RJ13075
Harpole, W. S., Sullivan, L. L., Lind, E. M., Firn, J., Adler, P. B., Borer, E. T., et al. (2016). Addition of multiple limiting resources reduces grassland diversity. Nature 537, 93–96. doi: 10.1038/nature19324
Hawkins, H. J. (2017). A global assessment of holistic planned grazing™ compared with season-long, continuous grazing: meta-analysis findings. Afr. J. Range Forage Sci. 34, 65–75. doi: 10.2989/10220119.2017.1358213
Hawkins, H. J., Venter, Z. S., and Cramer, M. D. (2022). A holistic view of holistic management: what do farm-scale, carbon, and social studies tell us? Agric. Ecosyst. Environ. 323:107702. doi: 10.1016/j.agee.2021.107702
Hillenbrand, M., Thompson, R., Wang, F., Apfelbaum, S., and Teague, R. (2019). Impacts of holistic planned grazing with bison compared to continuous grazing with cattle in South Dakota shortgrass prairie. Agric. Ecosyst. Environ. 279, 156–168. doi: 10.1016/j.agee.2019.02.005
Holechek, J. L., de Souza Gomes, H., Molinar, F., Galt, D., and Valdez, R. (2000). Short-duration grazing: the facts in 1999. Rangelands 22, 18–22. doi: 10.2458/azu_rangelands_v22i1_holechek
Huruba, R., Mlambo, T., Mundy, P. J., Sebata, A., and MacFadyen, D. N. (2018). Short duration overnight cattle kraaling in natural rangelands: implications for grass composition, quality, above ground biomass, species diversity and basal cover. Agric. Ecosyst. Environ. 257, 144–151. doi: 10.1016/j.agee.2018.02.004
Huruba, R., Ndlovu, C., Mundy, P. J., Sebata, A., and MacFadyen, D. N. (2021b). Short duration overnight cattle kraaling in natural rangelands leads to increased tree damage by elephants. J. Trop. Ecol. 2021, 1–8. doi: 10.1017/S0266467421000353
Huruba, R., Nemera, S., Ngute, F., Sahomba, M., Mundy, P. J., Sebata, A., et al. (2021a). Short duration overnight cattle kraaling in natural rangelands: does time after kraal use affect their utilization by wildlife and above ground grass parameters? bioRxiv [Preprint]. doi: 10.1101/2021.03.08.434375
Jacobo, E. J., Rodríguez, A. M., Bartoloni, N., and Deregibus, V. A. (2006). Rotational grazing effects on rangeland vegetation at a farm scale. Rangel. Ecol. Manage. 59, 249–257. doi: 10.2458/azu_jrm_v59i3_jacobo
Joubert, L., Pryke, J. S., and Samways, M. J. (2017). Moderate grazing sustains plant diversity in Afromontane grassland. Appl. Veg. Sci. 20, 340–351. doi: 10.1111/avsc.12310
Kay, G. M., Mortelliti, A., Tulloch, A., Barton, P., Florance, D., Cunningham, S. A., et al. (2017). Effects of past and present livestock grazing on herpetofauna in a landscape-scale experiment. Conserv. Biol. 31, 446–458. doi: 10.1111/cobi.12779
Kirkman, K. P., and Moore, A. (1995). Perspective: towards improved grazing management recommendations for sourveld. Afr. J. Range Forage Sci. 12, 135–144. doi: 10.1080/10220119.1995.9647883
Kleppel, G. S. (2019). Microbial community structure in pasture and hayfield soils of the helderberg region of New York State: a comparison of management strategies. Agroecol. Sustainable Food Syst. 43, 1031–1053. doi: 10.1080/21683565.2019.1591564
Kurtz, D. B., Giese, M., Asch, F., Windisch, S. H., and Goldfarb, M. C. (2018). Effects of high impact grazing on species diversity and plant functional groups in grasslands of northern Argentina. Sustainability 10:3153. doi: 10.3390/su10093153
LaCanne, C. E., and Lundgren, J. G. (2018). Regenerative agriculture: merging farming and natural resource conservation profitably. PeerJ 6:e4428. doi: 10.7717/peerj.4428
Lal, R. (2020). Regenerative agriculture for food and climate. J. Soil Water Conserv. 75, 123A–124A. doi: 10.2489/jswc.2020.0620A
Lalampaa, K. P. (2016). Influence of Holistic Grazing Management on Herbaceous Species Diversity, Range Use Pattern and Livestock Productivity in Laikipia County, Kenya Ph. D, Thesis. Nairobi: University of Nairobi.
Lalampaa, P. K., Wasonga, O. V., Rubenstein, D. I., and Njoka, J. T. (2016). Effects of holistic grazing management on milk production, weight gain, and visitation to grazing areas by livestock and wildlife in Laikipia County, Kenya. Ecol. Proc. 5, 1–12. doi: 10.1186/s13717-016-0061-5
Laliberté, E., and Tylianakis, J. M. (2012). Cascading effects of long-term land-use changes on plant traits and ecosystem functioning. Ecology 93, 145–155. doi: 10.2307/23144029
Lawrence, R. (2019). Ecological, Biophysical and Animal Production Responses to Strategic-Rest Grazing in Australia and Worldwide. Ph. D, Thesis. Armidale: University of New England.
Lawrence, R., Reid, N., and Rader, R. (2015). “High intensity, short duration livestock grazing management improves landscape function, and increases species richness and abundance of ground-layer insects on grazing properties of the northern slopes and tablelands of NSW, Australia,” in Proceedings of the 17th Australian Agronomy Conference, eds T. B. Acuña, T. C. Moeller, D. Parsons, and D. Hamilton (Hobart: Australian Society of Agronomy), 191–194.
Lemos, N. M. (2014). Assessing Rodent Species Counts and Diversity in the Not-Grazed Montana De Oro State Park and the Rotationally Grazed Pecho Ranch. Ph. D, Thesis]. San Luis Obispo (CA): California Polytechnic State University.
Limb, R. F., Fuhlendorf, S. D., Engle, D. M., Weir, J. R., Elmore, R. D., and Bidwell, T. G. (2011). Pyric–herbivory and cattle performance in grassland ecosystems. Rangel. Ecol. Manag. 64, 659–663. doi: 10.2307/41319082
Lindsay, E. A., and Cunningham, S. A. (2009). Livestock grazing exclusion and microhabitat variation affect invertebrates and litter decomposition rates in woodland remnants. For. Ecol. Manage. 258, 178–187. doi: 10.1016/j.foreco.2009.04.005
Lituma, C. M., Buckley, B. R., Keyser, P. D., Holcomb, E., Smith, R., Morgan, J., et al. (2022). Effects of patch-burn grazing and rotational grazing on grassland bird abundance, species richness, and diversity in native grassland pastures of the Midsouth USA. Agric. Ecosyst. Environ. 324:107710. doi: 10.1016/j.agee.2021.107710
Loeser, M. R., Sisk, T. D., and Crews, T. E. (2007). Impact of grazing intensity during drought in an Arizona grassland. Conserv. Biol. 21, 87–97. doi: 10.1111/j.1523-1739.2006.00606.x
Maschinski, J., and Whitham, T. G. (1989). The continuum of plant responses to herbivory: the influence of plant association, nutrient availability, and timing. Am. Nat. 134, 1–19. doi: 10.1086/284962
Massy, C. (2017). Call of the Reed Warbler: A New Agriculture–a New Earth. Brisbane: University of Queensland Press.
McDonald, S. E., Lawrence, R., Kendall, L., and Rader, R. (2019). Ecological, biophysical and production effects of incorporating rest into grazing regimes: a global meta-analysis. J. Appl. Ecol. 56, 2723–2731. doi: 10.1111/1365-2664.13496
McManus, J., Goets, S. A., Bond, W. J., Henschel, J. R., Smuts, B., and Milton, S. J. (2018). Effects of short-term intensive trampling on Karoo vegetation. Afr. J. Range Forage Sci. 35, 311–318. doi: 10.2989/10220119.2018.1529706
Mor-Mussery, A., Abu-Glaion, H., Shuker, S., and Zaady, E. (2021). The influence of trampling by small ruminants on soil fertility in semi-arid rangelands. Arid Land Res. Manage. 35, 189–197. doi: 10.1080/15324982.2020.1827083
Morris, C. D. (2021). Buried but unsafe - defoliation depletes the underground storage organ (USO) of the mesic grassland geophyte, Hypoxis hemerocallidea. Afr. J. Bot. 141, 265–272. doi: 10.1016/j.sajb.2021.05.017
Morris, C. D., and Scott-Shaw, R. (2019). Potential grazing indicator forbs for two mesic grasslands in South Africa. Ecol. Indic. 107:105611. doi: 10.1016/j.ecolind.2019.105611
Morris, C. D., and Tainton, N. M. (1996). Long-term effects of different rotational grazing schedules on the productivity and floristic composition of Tall Grassveld in KwaZulu-Natal. Afr. J. Range Forage Sci. 13, 24–28. doi: 10.1080/10220119.1996.9647889
Moulin, M., Lopez, J., Raman, A., Hodgkins, D., Adams, A., and Mannix, S. (2016). Measurement of biological diversity of arthropods and respiration in soils managed under time-controlled and set-stocked grazing practices in central-west new South Wales. Australia. Pol. J. Soil Sci. 45, 17–28. doi: 10.17951/pjss.2012.45.1.17
Mwendera, E. J., Saleem, M. M., and Woldu, Z. (1997). Vegetation response to cattle grazing in the Ethiopian highlands. Agric. Ecosyst. Environ. 64, 43–51. doi: 10.1016/S0167-8809(96)01128-0
Newton, P., Civita, N., Frankel-Goldwater, L., Bartel, K., and Johns, C. (2020). What is regenerative agriculture? A review of scholar and practitioner definitions based on processes and outcomes. Front. Sustainable Food Syst. 4:577723. doi: 10.3389/fsufs.2020.577723
Nordborg, M. (2016). Holistic ManagementA Critical Review of Allan Savory’s Grazing Method. Uppsala: SLU/EPOKCentre for Organic Food & Farming & Chalmers.
Odadi, W. O., Fargione, J., and Rubenstein, D. I. (2017). Vegetation, wildlife, and livestock responses to planned grazing management in an African pastoral landscape. Land Degrad. Dev. 28, 2030–2038. doi: 10.1002/ldr.2725
Oliva, G., Ferrante, D., Cepeda, C., Humano, G., and Puig, S. (2021). Holistic versus continuous grazing in patagonia: a station-scale case study of plant and animal production. Rangel. Ecol. Manag. 74, 63–71. doi: 10.1016/j.rama.2020.09.006
Oyarzabal, G., and Guimarães, M. (2021). Friend and foe? The effects of grassland management on global patterns of spider diversity. Ecol. Entomol. 46, 1195–1204. doi: 10.1111/een.13065
Paine, L. K., and Ribic, C. A. (2002). Comparison of riparian plant communities under four land management systems in southwestern Wisconsin. Agric. Ecosyst. Environ. 92, 93–105. doi: 10.1016/S0167-8809(01)00269-9
Porensky, L. M., Augustine, D. J., Derner, J. D., Wilmer, H., Lipke, M. N., Fernández-Giménez, M. E., et al. (2021). Collaborative adaptive rangeland management, multipaddock rotational grazing, and the story of the regrazed grass plant. Rangel. Ecol. Manag. 78, 127–141. doi: 10.1016/j.rama.2021.06.008
Rantso, T., Odenya, W., and Chatanga, P. (2021). The Efficacy of Holistic Grazing Management in Communal Grazing System: A Case Study from Mokhotlong District, Lesotho. Beau Bassin, Mauritius: Lambert Academic Publishing.
Rhodes, C. J. (2017). The imperative for regenerative agriculture. Sci. Prog. 100, 80–129. doi: 10.3184/003685017X14876775256165
Ruthven, D. C. (2007). Grazing effects on forb diversity and abundance in a honey mesquite parkland. J. Arid. Environ. 68, 668–677. doi: 10.1016/j.jaridenv.2006.08.003
Saunders, W. C., and Fausch, K. D. (2007). Improved grazing management increases terrestrial invertebrate inputs that feed trout in Wyoming rangeland streams. Trans. Am. Fish. Soc. 136, 1216–1230. doi: 10.1577/T06-260.1
Savory, A., and Butterfield, J. (2016). Holistic Management: A Commonsense Revolution to Restore Our Environment. Washington, DC: Island Press.
Schieltz, J. M., and Rubenstein, D. I. (2016). Evidence based review: positive versus negative effects of livestock grazing on wildlife. What do we really know? Environ. Res. Lett. 11:113003. doi: 10.1088/1748-9326/11/11/113003
Schmid, R., and Lundgren, J. (2020). The effect of pasture management on fire ant, solenopsis invicta (hymenoptera: formicidae), abundance and the relationship to arthropod community diversity. bioRxiv [Preprint]. doi: 10.1101/2020.12.04.398214v2
Schreefel, L., Schulte, R. P. O., de Boer, I. J. M., Schrijver, A. P., and van Zanten, H. H. E. (2020). Regenerative agriculture–the soil is the base. Global Food Secur. 26:100404. doi: 10.1016/j.gfs.2020.100404
Schulz, P. A., and Guthery, F. S. (1988). Effects of short duration grazing on northern bobwhites: a pilot study. Wildl. Soc. Bull. 16, 18–24.
Schurch, M. P., McManus, J., Goets, S., Pardo, L. E., Gaynor, D., Samuels, I., et al. (2021). Wildlife-friendly livestock management promotes mammalian biodiversity recovery on a semi-arid Karoo farm in South Africa. Front. Conserv. Sci. 2:6. doi: 10.3389/fcosc.2021.652415
Scott, D. (2001). Sustainability of New Zealand high-country pastures under contrasting development inputs. 7. environmental gradients, plant species selection, and diversity. N. Z. J. Agric. Res. 44, 59–90. doi: 10.1080/00288233.2001.9513463
Scott-Shaw, R., and Morris, C. D. (2015). Grazing depletes forb species diversity in the mesic grasslands of KwaZulu-Natal, South Africa. Afr. J. Range Forage Sci. 32, 21–31. doi: 10.2989/10220119.2014.901418
Sebata, S. (2020). Spider Ecology in Southwestern Zimbabwe, with Emphasis on the Impact of Holistic Planned Grazing Practices. Ph. D, Thesis. Bloemfontein: University of the Free State.
Sherren, K., and Kent, C. (2019). Who’s afraid of allan savory? Scientometric polarization on holistic management as competing understandings. Renewable Agric. Food Syst. 34, 77–92. doi: 10.1017/S1742170517000308
Sherwood, S., and Uphoff, N. (2000). Soil health: research, practice and policy for a more regenerative agriculture. Appl. Soil Ecol. 15, 85–97. doi: 10.1016/S0929-1393(00)00074-3
Sibanda, P., Sebata, A., Mufandaedza, E., and Mawanza, M. (2016). Effect of short-duration overnight cattle kraaling on grass production in a southern African savanna. Afr. J. Range Forage Sci. 33, 217–223. doi: 10.2989/10220119.2016.1243580
Skovlin, J. (1987). Southern Africa’s experience with intensive short duration grazing. Rangelands 9, 162–167.
Sliwinski, M. S., Powell, L. A., and Schacht, W. H. (2020). Similar bird communities across grazing systems in the Nebraska Sandhills. J. Wildl. Manage. 84, 802–812. doi: 10.1002/jwmg.21825
Sliwinski, M., Powell, L., and Schacht, W. (2019). Grazing systems do not affect bird habitat on a sandhills landscape. Rangel. Ecol. Manag. 72, 136–144. doi: 10.1016/j.rama.2018.07.006
Souther, S., Loeser, M., Crews, T. E., and Sisk, T. (2020). Drought exacerbates negative consequences of high-intensity cattle grazing in a semiarid grassland. Ecol. Appl. 30:e02048. doi: 10.1002/eap.2048
Spratt, E., Jordan, J., Winsten, J., Huff, P., van Schaik, C., Jewett, J. G., et al. (2021). Accelerating regenerative grazing to tackle farm, environmental, and societal challenges in the upper Midwest. J. Soil Water Conserv. 76, 15A–23A. doi: 10.2489/jswc.2021.1209A
Stinner, D. H., Stinner, B. R., and Martsolf, E. (1997). Biodiversity as an organizing principle in agroecosystem management: case studies of holistic resource management practitioners in the USA. Agric. Ecosyst. Environ. 62, 199–213. doi: 10.1016/S0167-8809(96)01135-8
Sun, D., and Liddle, M. J. (1993). Plant morphological characteristics and resistance to simulated trampling. Environ. Manage. 17, 511–521. doi: 10.1007/BF02394666
Teague, R., and Barnes, M. (2017). Grazing management that regenerates ecosystem function and grazingland livelihoods. Afr. J. Range Forage Sci. 34, 77–86. doi: 10.2989/10220119.2017.1334706
Teague, R., and Kreuter, U. (2020). Managing grazing to restore soil health, ecosystem function, and ecosystem services. Front. Sustainable Food Syst. 4:534187. doi: 10.3389/fsufs.2020.534187
Teague, R., Provenza, F., Kreuter, U., Steffens, T., and Barnes, M. (2013). Multi-paddock grazing on rangelands: why the perceptual dichotomy between research results and rancher experience? J. Environ. Manage. 128, 699–717.
Teague, W. R., Dowhower, S. L., Baker, S. A., Haile, N., DeLaune, P. B., and Conover, D. M. (2011). Grazing management impacts on vegetation, soil biota and soil chemical, physical and hydrological properties in tall grass prairie. Agric. Ecosyst. Environ. 141, 310–322. doi: 10.1016/j.agee.2011.03.009
Teutscherová, N., Vázquez, E., Sotelo, M., Villegas, D., Velásquez, N., Baquero, D., et al. (2021). Intensive short-duration rotational grazing is associated with improved soil quality within one year after establishment in Colombia. Appl. Soil Ecol. 159:103835. doi: 10.1016/j.apsoil.2020.103835
Tom, N., Raman, A., Hodgkins, D. S., and Nicol, H. (2006). Populations of soil organisms under continuous set stocked and high intensity-short duration rotational grazing practices in the central tablelands of New South Wales (Australia). N. Z. J. Agric. Res. 49, 261–272. doi: 10.1080/00288233.2006.9513717
Toombs, T. P., Derner, J. D., Augustine, D. J., Krueger, B., and Gallagher, S. (2010). Managing for biodiversity and livestock. Rangelands 32, 10–15. doi: 10.2307/40802633
Trimble, S. W., and Mendel, A. C. (1995). The cow as a geomorphic agenta critical review. Geomorphology 13, 233–253. doi: 10.1016/0169-555X(95)00028-4
Van Noordwijk, C. G. E., Flierman, D. E., Remke, E., WallisDeVries, M. F., and Berg, M. P. (2012). Impact of grazing management on hibernating caterpillars of the butterfly melitaea cinxia in calcareous grasslands. J. Insect Conserv. 16, 909–920. doi: 10.1007/s10841-012-9478-z
Venter, Z. S., Hawkins, H. J., and Cramer, M. D. (2019). Cattle don’t care: animal behaviour is similar regardless of grazing management in grasslands. Agric. Ecosyst. Environ. 272, 175–187. doi: 10.1016/j.agee.2018.11.023
Venter, Z. S., Hawkins, H. J., and Cramer, M. D. (2021). Does defoliation frequency and severity influence plant productivity? The role of grazing management and soil nutrients. Afr. J. Range Forage Sci. 38, 141–156. doi: 10.2989/10220119.2020.1766565
Wagner, P. M., Abagandura, G. O., Mamo, M., Weissling, T., Wingeyer, A., and Bradshaw, J. D. (2020). Abundance and diversity of dung beetles (coleoptera: scarabaeoidea) as affected by grazing management in the nebraska sandhills ecosystem. Environ. Entomol. 50, 1–10. doi: 10.1093/ee/nvaa130
Wang, C., and Tang, Y. (2019). A global meta-analyses of the response of multi-taxa diversity to grazing intensity in grasslands. Environ. Res. Lett. 14:114003. doi: 10.1088/1748-9326/ab4932
Wang, F., Apfelbaum, S. I., Thompson, R. L., Teague, R., and Byck, P. (2021). Effects of adaptive multiple paddock and continuous grazing on fine-scale spatial patterns of vegetation species and biomass in commercial ranches. Landscape Ecol. 36, 2725–2741. doi: 10.1007/s10980-021-01273-z
Weigel, J. R., McPherson, G. R., and Britton, C. M. (1989). Effects of short-duration on winter annuals in the texas rolling plains. Rangel. Ecol. Manag. 42, 372–375. doi: 10.2307/3899541
White, C. (2020). Why regenerative agriculture? Am. J. Econ. Socio. 79, 799–812. doi: 10.1111/ajes.12334
Keywords: adaptive multi-paddock grazing, holistic planned grazing, livestock density, species richness, wildlife
Citation: Morris CD (2021) How Biodiversity-Friendly Is Regenerative Grazing? Front. Ecol. Evol. 9:816374. doi: 10.3389/fevo.2021.816374
Received: 16 November 2021; Accepted: 06 December 2021;
Published: 23 December 2021.
Edited by:
Steve Monfort, Smithsonian Conservation Biology Institute (SI), United StatesReviewed by:
Jennifer Lesley Silcock, The University of Queensland, AustraliaCopyright © 2021 Morris. This is an open-access article distributed under the terms of the Creative Commons Attribution License (CC BY). The use, distribution or reproduction in other forums is permitted, provided the original author(s) and the copyright owner(s) are credited and that the original publication in this journal is cited, in accordance with accepted academic practice. No use, distribution or reproduction is permitted which does not comply with these terms.
*Correspondence: Craig D. Morris, bW9ycmlzQHVrem4uYWMuemE=
Disclaimer: All claims expressed in this article are solely those of the authors and do not necessarily represent those of their affiliated organizations, or those of the publisher, the editors and the reviewers. Any product that may be evaluated in this article or claim that may be made by its manufacturer is not guaranteed or endorsed by the publisher.
Research integrity at Frontiers
Learn more about the work of our research integrity team to safeguard the quality of each article we publish.