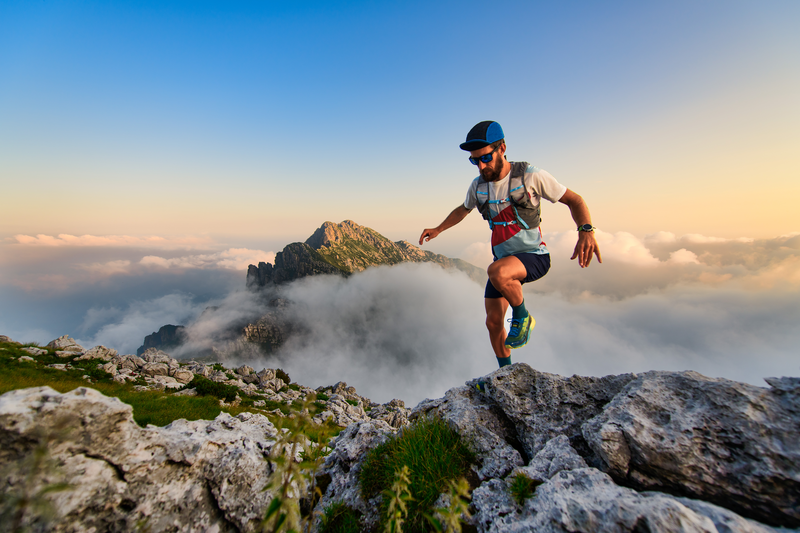
94% of researchers rate our articles as excellent or good
Learn more about the work of our research integrity team to safeguard the quality of each article we publish.
Find out more
SYSTEMATIC REVIEW article
Front. Ecol. Evol. , 11 January 2022
Sec. Conservation and Restoration Ecology
Volume 9 - 2021 | https://doi.org/10.3389/fevo.2021.805707
This article is part of the Research Topic Ecological Impacts of Transportation Networks at Large Extents View all 7 articles
The global use of off-road vehicles (ORVs) in natural environments has accelerated rapidly over the last few decades, resulting in significant social and environmental consequences. As the demand, use, and promotion of light-duty ORVs like all-terrain vehicles (ATVs), motorcycles, four-wheel drive trucks and sport utility vehicles (SUVs) increases in remote wilderness, the landscape is becoming fragmented into disorganized and destructive networks of trails and roads. Substantial ecological impacts to a wide range of ecosystem structures and functions will likely result from ORV activity. Applying a global systematic review, we examine 105 publications about plant, soil, and wildlife responses to ORV traffic in different habitats to help guide the direction of future research, monitoring programs, and mitigation efforts. Most studies investigated impacts to animals, followed by soils, then vegetative responses. Soil studies primarily focused on physical impacts to the soil (i.e., compaction, erosion, rut depth), but some studies suggest that soil chemical and biological properties may also be impacted by ORV traffic. The literature on plant responses to ORV activities primarily explored vegetation loss, although impacts on the plant community were also investigated. Animal studies investigated impacts of ORV use on invertebrates, mammals, birds, and to a lesser extent reptiles/amphibians, including population-level, community-level, and behavioral responses. Overall, research on environmental impacts of ORV traffic is biased to coastal and desert ecosystems in the northern hemisphere (primarily in the US), often does not address mechanisms that may produce ecological impacts (e.g., intensity of vehicular disturbance and ecosystem- or species-specific sensitivity to ORV activities), and frequently focused on short-term responses. More research is needed to understand the mechanisms that cause the different responses of soil, plant, and animals to ORVs over the long-term in a broad range of ecosystems to support real-time management and conservation efforts.
Global use of off-road vehicles (ORVs) in natural environments has accelerated rapidly over the past few decades (Cordell et al., 2005; Navas Romero et al., 2019; Enríquez-de-Salamanca, 2021), and over this time, it has become increasingly evident that ORV use can result in significant social and environmental consequences (Monz et al., 2010; Crisfield et al., 2012; Besserer and Caron, 2013). As the promotion, demand, and access to light-duty ORVs, such as all-terrain vehicles (ATVs), motorcycles, four-wheel drive trucks and sport utility vehicles (SUVs), continues to increase for both personal and commercial practices (Cole, 2019; Navas Romero et al., 2019), more and more informal transportation networks are being created in increasingly remote wilderness, fragmenting the landscape into disorganized and destructive networks of trails and roads (Trombulak and Frissell, 2000; Shanley and Pyare, 2011).
Concerns over the growth in ORV activity include substantial ecological impacts on a wide range of ecosystem structures and functions, including potential undesirable consequences to soils, flora, and fauna (Navas Romero et al., 2019; Cheung et al., 2021). ORV traffic can alter habitat structure and function through alterations to physiochemical properties of soil, loss of vegetative cover, and changes in plant community structure and function (Slaughter et al., 1990; Navas Romero et al., 2019; Sumanapala and Wolf, 2019), which often results in undesirable consequences for the fauna that inhabit them (Schlacher and Thompson, 2008; Cole, 2019). For instance, soils can be physically damaged through increased compaction, which may alter the success of certain plant species, altering species diversity (Brown and Schoknecht, 2001; Assaeed et al., 2019). While vegetation loss is common with ORV activity (Al-Awadhi, 2013; Cheung et al., 2021), community level responses have also been found, including alterations in species composition and conditions that may favor the introduction and spread of invasive plants (Milchunas et al., 2000; Assaeed et al., 2019; Navas Romero et al., 2019). Further, while wildlife can be directly affected by ORV use (e.g., collision with a vehicle), we focused primarily on the impacts on wildlife that result from changes to soil and vegetation, such as habitat loss, alterations in breeding behaviors and migration routes, and consequences of habitat fragmentation. Research has demonstrated that many factors influence the severity of disturbance caused by ORVs, including the type of vehicle used, frequency and intensity of use, operator techniques (e.g., acceleration, braking, and velocity), and site-specific parameters (e.g., soil type, soil ice or moisture content, and topographical features) (Ahlstrand and Racine, 1993; Kutiel et al., 2000; Li and Sandu, 2007). But, it remains unclear how these factors may influence the flora and fauna in different habitats. Thus, using a global systematic review, we investigate the current state of the literature on plant, soil, and wildlife responses to ORV traffic to help guide the direction of future research, monitoring programs, and mitigation efforts.
Given the idiosyncratic nature of use-impact relationships of various habitats, we examine the potential sensitivity of habitats to ORV use, particularly regarding soil quality, and plant community dynamics, as well as habitat resistance, the capacity of the system to withstand change following a disturbance, and resilience, the degree to which the system recovers to its initial composition. This research aims to understand our current understanding of the durability (i.e., resilience and resistance) of the natural environment and the types and levels of ORV-use that can be tolerated without causing undesirable change. Understanding the resilience and resistance of various habitats to disturbance caused by ORV activity will be critical for their long-term sustainable management and will provide researchers, stakeholders and managers critical information for future modeling, and projections that will aid in conserving these lands and the wildlife that inhabit them.
We conducted a systematic review using the Preferred Reporting Items for Systematic Reviews and Meta-Analyses in ecology and evolution (PRISMA-EcoEvo) guidelines (Moher et al., 2009; O'Dea et al., 2021). We searched the Web of Science database in May–July 2021 using a combination of terms (Table 1) to quantify the current body of literature exploring the impacts of light-duty, wheeled ORV activities on the environment. Further, the search terms used only identified one paper that explored the environmental impacts snowmobiles on alpine plant communities (Greller et al., 1974), thus, we excluded tracked vehicles like snowmobiles from this literature review. Citations from these studies were also used to locate relevant articles.
Articles were reviewed and were included or excluded based on pre-determined inclusion/exclusion criteria (Table 2) using the title and/or abstract. Retained articles were examined in more detail, and the following information was recorded: (1) the location of the study (country, latitude, and longitude); (2) the test subject (plant, soil, and/or animal) (3) the method of data collection/synthesis (field experiment or observational study), and (4) the habitat studied (tundra/polar desert, coastal, desert, forest, grassland/shrubland, bog, or riparian). When not directly presented in the text, study coordinates were estimated based on the description presented in the article (e.g., a city or a national/local park name). Habitat was determined based on information provided by author(s). Several publications reported on the environmental effects of ORVs in different habitats and/or on multiple test subjects or species. Thus, we counted these as separate reports for the general systematic review.
Reports exploring soil parameters were grouped into the type of soil data collected: physical, chemical, hydrological, or biological. Vegetation responses were grouped according to the type of vegetation data that was collected: biomass, community composition, cover/abundance, height, injury, population, or seed bank/transport. For reports presenting results on animal test subjects, we recorded whether the animal was an invertebrate or vertebrate, the general animal subject (mammal, bird, arthropod, or reptile/amphibian). Each of these animal subjects were grouped according to the type of data collected: behavioral, physiological, population, or community.
After applying the inclusion/exclusion criteria, a total of 105 publications were selected for our systematic review, which corresponds to 17.5% of the total (n = 597). Supplementary Figure 1 shows the flowchart for identification, screening, and eligibility of the selected articles (n = 105). Articles were published between 1974 and 2021 with most publications occurring after 2005 (Supplementary Figure 2). Publications (n = 105) were found from 16 different countries (including Antarctica) with the majority being conducted in the United States (n = 68, 64.8%) followed by Australia (n = 13, 12.4%), Canada (n = 4, 3.8%), South Africa (n = 4, 3.8%), Mongolia (n = 3, 2.9%), Kuwait (n = 2, 1.9%), and Saudi Arabia (n = 2, 1.9%; Figure 1). The remaining nine countries had one published article (n = 1, < 1%; Supplementary Figure 3).
Figure 1. Map of reports of the impacts of light-duty off-road vehicle (ORV) on soils, plants, and animals according to country. Each red dot represents the location of each study (n = 105). Countries are color-coded according to the percentage of reports each country contributed to the published studies, where darker shades of blue indicate a larger percentage.
After accounting for publications that studied multiple habitats and/or subjects (i.e., soil, plant, or animal), there were 131 reports that explored impacts of ORV activity. Reports (n = 131) were conducted in seven habitats: coastal (n = 39, 29.8%), desert (n = 33, 25.2%), grassland/shrubland (n = 24, 18.3%), forest (n = 21, 16%), tundra/polar desert (n = 6, 4.6%), wetland (n = 5, 3.8%), and riparian habitats (n = 3, 2.3%; Supplementary Figure 4; Figure 2). Most of the reports (n = 131) explored impacts on animals (n = 55, 42%) followed by soils (n = 39; 29.8%) then vegetation (n = 37, 28.2%; Figure 3). Most animal studies occurred primarily in coastal, desert, or forest habitats, and soil and vegetation studies were primarily conducted in coastal, desert, or grassland/shrubland habitats.
Figure 2. Examples different habitats and locations impacted by off-road vehicle (ORV) traffic. (A) ORV vehicle tracks on a beach in New Jersey, USA (Kelly, 2014); (B) Scale of impacted dunes from high- to low impact (left to right) in New Brunswick, Canada (Hogan and Brown, 2021); (C) Pictorial demonstration of different microhabitats created by ORV tracks in Saudi Arabia (Assaeed et al., 2019); (D) ORV trail in Douglas-fir forest in the interior of British Columbia, Canada (Photo by: Laura Ploughe) (E) ORV trail in a chapparal in California, USA (Cole et al., 2019).
Figure 3. Number of reports (n = 142) that accessed the impacts of off-road vehicle (ORV) use across three test subject types (animal, soil, or vegetation). Results are categorized by ecosystem type.
Soil reports (n = 40) primarily used an observational approach (n = 29; 72.5%). There was also one study that used a combination of observational and modeling approaches (2.5%) and ten studies that used an experimental approach (25%; Supplementary Figure 5). Observational studies compared impact areas, where ORV use is permitted, and control areas, where ORV use is restricted. Most of these studies did not specify the level of ORV activity (n = 22). There were four studies that described heavy use of ORV with >100 vehicles per day, and two studies that had fewer than 50 vehicles per day. One study explored the impacts of single use tracks. Treatments for experimental studies (n = 10) involved driving four-wheel drive vehicles (n = 8), ATVs (n = 2), and/or motorcycles (n = 4) a specified number of times over the experimental area.
After classifying soils according to the type of data collected (i.e., biological, chemical, erosion, hydrology, or physical), we found that several papers reported on multiple aspects of the soil (e.g., both physical and chemical). Therefore, in Figure 4, reports that collected more than one type of data were considered to be individual soil descriptors, making n = 52. Most studies explored physical changes to the soil (n = 39, 75%) and were conducted in desert (n = 13, 33.3%), grassland/shrubland (n = 9, 23.1%), forest (n = 8, 20.5%), and coastal habitats (n = 6, 15.4%; Figure 4). Physical effects of soils were the only aspect of soils that was studied in coastal, riparian, and wetland habitats. In desert habitats, studies also explored chemical (n = 2), biological (n = 1), and hydrological (n = 1) changes to the soil. Studies exploring chemical changes to soils following ORV activity were found in grassland/shrubland (n = 4) and forest habitats (n = 1), and hydrological studies in grassland/shrubland (n = 3) and riparian habitats (n = 1).
Figure 4. Number of soil reports (n = 52) that accessed the impacts of off-road vehicle (ORV) use across the ecosystem studied. Results are categorized by the type of ecological data collected (biological, chemical, erosion, hydrology, and physical).
Vegetation studies (n = 38) primarily used observational methods (n = 27, 71.1%), although experimental methods (n = 11; 28.9%) were also used (Supplementary Figure 6). Observational studies (n = 27, 71.1% comparing impact and control areas generally did not report the specific levels of ORV activity but were assumed to be moderate or heavy use (n = 22). Two studies reported high levels of ORV activity (>100 vehicles per day). Two studies explored low levels of ORV use with a maximum of 5 cars per day, and another study explored single passage tracks. Experimental treatments (n = 11) involved driving four-wheel drive vehicles (n = 8), ATVs (n = 2), and/or motorcycles (n = 2) over an area a specified number of times. There was also one study that explored seed transport on different types of vehicle tires: ATVs, four-wheel drive, and tracked vehicles.
Several vegetation studies collected more than one type of data (i.e., biomass, community composition, cover/abundance, height, injury, population, or seed bank/transport). Thus, vegetation reports that collected more than one type of data were considered to be individual reports, making n = 56 for this portion. Vegetation reports (n = 56) were collected in six habitats, including grassland/shrubland (n = 17, 30.4%), desert (n = 16, 28.6%), coastal (n = 14, 25%), forest (n = 4, 7.1%), tundra/polar desert (n = 3, 5.4%), and wetland habitats (n = 2, 3.6%; Figure 5).
Figure 5. Number of vegetation reports (n = 38) that accessed the impacts of off-road vehicle (ORV) use across the ecosystem studied. Results are categorized by the type of ecological data collected (biomass, community, cover/abundance, height, injury, population, and seed bank/transport).
Of the 17 vegetation studies in grassland/shrubland habitat, most reported on community composition (n = 7, 41.2%), followed by cover/abundance (n = 5, 29.4%), height (n = 2, 11.8%), injury (n = 2, 11.2%), biomass (n = 1, 5.9%), and seed bank/transport (n = 1, 5.9%; Figure 5). In desert habitats (n = 16), vegetation studies measured total cover/abundance (n = 8, 50%) followed by community composition (n = 4, 25%). Measurements for height, injury, and seed bank/transport were found in one time each. There was also one study conducted in a desert habitat that explored population-level changes to a threatened species, Astragalus magdalenae var. peirsonii (Pierson's milk vetch). Studies in coastal habitats (n = 14) primarily reported on cover/abundance (n = 6, 42.9%) followed by community composition (n = 4; 28.6%), vegetation height (n = 2; 14.3%, biomass (n = 1; 7.1%), and population changes of a rare plant, Ammophila breviligulata (n = 1; 7.1%). In forest habitats (n = 4), studies measured seed bank/transport (n = 2), community composition (n = 1), and shrub injury (n = 1). The three studies found in tundra/polar desert habitats (n = 4; Figure 5) measured community composition (n = 2) and injury to shrubs, grasses, and sedges (n = 1). In wetland habitats (n = 2), community composition and the population a rare plant (Drosera filiformis) were studied.
Finally, most of the reports on vegetation focused on the relatively immediate impacts of ORV use on vegetation and only four studies were found to explore the recovery following the use of ORVs (7.1%). Two vegetation recovery studies were found in grassland habitats. One of these studies explored sites that suspended ORV use 4 years prior to data collected. The other study explored two sites and estimated the time since ORV use as between 3 and 5 years at one site and 15–20 years of the second site. Recovery of a tundra habitat was explored 20 years after road abandonment. The last study used an experimental approach on a coastal and explored recovery 1 year after experimental treatments.
From the 53 reports on the impacts of ORV on animals, there were 21 reports that explored the effects on invertebrates. The majority of these studies used an observational approach (n = 16; 76.2%; Supplementary Figure 7). Most observational studies compared areas designated for ORV to areas restricting ORV activity (n = 14) ORV activity in these studies tended to be high with several hundred vehicles reported per day (n = 9); although, one study reported low ORV activity with 7–40 vehicles reported per day. The remaining studies did not report ORV use levels or activity (n = 4) or explored effects of different ORV restrictions (e.g., 24 h or nighttime restrictions, n = 1) and organism transport (n = 1). Five invertebrate studies used an experimental approach (23.8%) manipulating the number of vehicles passes to explore impacts on invertebrates. One experimental study explored the attachment probability and survival distance of aquatic invertebrates based on density of individuals. Invertebrate studies (n = 21) were primarily conducted in coastal habitats (n = 16; 76.2%; Supplementary Figure 8). There were also three invertebrate studies in desert habitats (14.3%), and one invertebrate study in riparian and wetland habitats (4.76%, respectively).
Of the 21 reports on invertebrates, there were several that studied more than one type of organism. Therefore, Figure 6 displays these incidences as separate reports (n = 27). Eight of these studies investigated the impacts of ORV traffic on Ocypodinae spp., including behavioral studies (n = 4), exploring burrowing patterns and home ranges, and population studies (n = 4), examining abundance and mortality (Figure 6). There were eight other studies that explored populations of benthic macroinvertebrates (n = 7), including Bullia rhodostoma (n = 1), Donax deltoides (n = 2), Donax sordidus (n = 1), Donax variabilis (n = 1), Emerita talpoida (n = 1), Gastrosaccus psammodytes (n = 1), Scopimera inflata (n = 1), and Tylos capensis (n = 1). There was one population study in a desert habitat exploring effects of ORV use on Cicindela albis-sima. Of the seven reports exploring effects on invertebrate communities, five explored benthic macroinvertebrates and two explored desert invertebrates, Arthropoda (n = 1) and Coleoptera (n = 1). There were also three organism transport reports, including the transport of aquatic invertebrates in a wetland habitat, and a study exploring the transport of Physella acuta and Procambarus clarkia in a riparian habitat.
Figure 6. Number of reports (n = 27) that accessed the impacts of off-road vehicle (ORV) use on invertebrates across the type of ecological data collected (behavior, community-level, population-level, or organism transport). Results are categorized by the organism or group of organisms that were studied.
Thirty-three reports studying the effects of ORV on vertebrates were found. Studies generally used an observational approach (n = 23; 69.7%), but experimental (n = 9; 27.3%) as well as a combination of observation and experiment (n = 1; 3%) were also used (Supplementary Figure 9). Of the 33 vertebrate reports found, there were a few studies that explored more than one type of vertebrate responses (e.g., both population-level abundance and physiological responses) and/or more than one type of vertebrate (e.g., mammals and reptiles). Thus, for the ease of visualizing these results, these incidences were counted as separate reports for the remainder of this section (n = 36).
Studies exploring impacts of ORV activity on birds (n = 16, 44.4%) were found in four habitat types: coastal (n = 8, 50%), forest (n = 3, 18.8%), grassland/shrubland (n = 4, 25%), and wetland habitats (n = 1, 6.2%; Figure 7). Most of these studies used an observational approach (n = 11, 68.8%) and assessed bird responses in existing ORV areas using various monitoring approaches (e.g., video or observer). The remaining studies used ATVs, motorcycles, and four-wheel drive vehicles to experimentally mimic ORV activities (n = 5).
Figure 7. Number of reports that accessed the impacts of off-road vehicle (ORV) use on vertebrates (n = 36) across the type of vertebrate studied (bird, mammal, or reptiles/amphibians). Results are categorized by habitat type.
Seven studies (43.8%) explored behavioral responses of birds to ORV activity (Figure 8), specifically responses of Aquila chrysaetos (n = 3), Haematopus palliates (n = 2), coastal birds (n = 1), and wading birds (n = 1). Population-level studies (n = 3, 12.5%) examined reproductive success of Strix occidentalis caurina and chick survival of Charadrius melodus. Community-level responses to ORV activity were explored for woodland birds, coastal birds, and breeding songbirds in a grassland habitat (n = 3, 18.8%). Physiological responses of birds to ORV activity (n = 3; 18.8%) were studied in Haematopus palliates (n = 2) and Strix occidentalis caurina (n = 1).
Figure 8. Number of reports that accessed the impacts of off-road vehicle (ORV) use on birds (n = 16) across the type of data collected (behavior, population, anatomy/physiology, and community) Results are categorized by species or group of birds that were studied.
Within the vertebrate reports (n = 36), twelve studied the responses of mammals to ORV activity (33.3%: Figure 7). Mammalian studies used both observational (n = 7, 58 %) and experimental methods (n = 5, 42%), where responses were measured in areas with existing ORV activity or in areas where ORV activity was experimentally created with ATVs (n = 3) or four-wheel drive vehicles (n = 2), respectively. Half of these studies (n = 6) were conducted in forest habitats, and the remaining were in desert (n = 3, 25%), tundra/polar desert (n = 2, 16.7%), and grassland/shrubland habitats (n = 1, 8.3%) (Figure 7). Most of the mammal studies explored behavioral responses (n = 10, 83.3%) of the following species: Alces alces (n = 2), Cervus canadensis (n = 1), Cervus elaphus (n = 2), Leptonychotes weddellii (n = 1), Martes americana (n = 1), Oreamnos americanus (n = 1), Puma concolor coryi (n = 1), Vulpes macrotis (n = 1, Figure 9). The remaining mammalian studies explored community-level responses in desert habitats (n = 2; 16.7); one studied all mammals (e.g., rats, bats, etc.) and the other focused on rodents.
Figure 9. Number of reports that accessed the impacts of off-road vehicle (ORV) use on mammals (n = 12) across the type of data collected (behavior or community). Results are categorized by species or group of mammals that were studied.
Amphibian and reptile studies were conducted primarily in desert habitats (n = 5, 62.5%), and one time in each of the following habitats: coastal, forest, and riparian. Three of these studies (37.5%) explored community-level responses of lizards in desert habitats (n = 2) and amphibians and reptiles in a forest habitat (n = 1, Figure 7). Population studies (n = 2, 25%) explored how ORV activity impacted the abundance of Apalone spp. and Phrynosoma mcallii (Figure 10). Two behavioral studies (n = 25%) explored responses of Phrynosoma mcallii and Caretta to ORV activity (Figure 10). Finally, there was one study that examined anatomical responses of a lizard, Sceloporus occidentalis, as an indicator of stress (Tull and Brussard, 2007).
Figure 10. Number of reports that accessed the impacts of off-road vehicle (ORV) use on reptiles/amphibians (n = 12) across the type of data collected (community, behavior, population, anatomy/physiology). Results are categorized by species or group studied.
Most of the research on the environmental impacts of ORV activity was conducted in the northern hemisphere, biased to coastal and desert habitats, and often explored impacts to existing ORV tracks through observational studies. The level of impact varies between ORV areas, and there is currently not a consensus on traffic levels or the extent to which the landscape is disturbed by ORVs, as most studies report ORV use at regional- or local scales. In the US, where most of the research was conducted, ORVs are state regulated, making it difficult to determine the exact extent of ORV activities at a national scale. While the US is thought to have one of the highest rates of ORV activity, off-road driving is altering landscapes across Europe (Enríquez-de-Salamanca, 2021), Australia (Davies et al., 2016), Canada (Trip and Wiersma, 2015), as well as less-developed nations like Mongolia (Li et al., 2006), Argentina (Navas Romero et al., 2019), and Saudi Arabia (Assaeed et al., 2019).
Physical properties of soils were common measures to explore the impacts of ORVs on the terrain and were found to be relatively sensitive to ORV activities. In ORV areas, soils tend to have higher levels of compaction in the form of increased bulk density (Sparrow et al., 1978; Wilshire et al., 1978; Payne et al., 1983; Pérez, 1991; Al-Awadhi, 2013; Dewidar et al., 2016; Assaeed et al., 2019) and penetration resistance (Hosier and Eaton, 1980; Pérez, 1991; Brown and Schoknecht, 2001; Al-Awadhi, 2013; Davies et al., 2016; Knisley et al., 2018; Navas Romero et al., 2019). Compaction tended to increase with the number of vehicle passes and vehicle weight (Adams et al., 1982; Kutiel et al., 2000; Hirst et al., 2003; Webb et al., 2013), and often increased significantly after a single pass of an ORV, including motorcycles, ATVs, and four-wheel drive vehicles (Adams et al., 1982; Hirst et al., 2003; Webb et al., 2013). However, the sensitivity of different habitats to compaction may vary. In a mixed prairie habitat, Payne et al. (1983) found that bulk density did not increase when passes with a 4-wheeled vehicle were fewer than 32 times a month between May and September. The discrepancies may be a result of different aspects of the site (e.g., soil type, vegetation, soil moisture, terrain, etc.) or the type of ORV disturbance was applied (e.g., vehicle weight, time of disturbance, etc.).
In addition to soil compaction, soils experienced other physical changes, including altered particle size and the creation of ruts. Interestingly, in both a desert and grassland habitat, ORV activity altered the particle size of the soil, increasing the percentage of coarse particles compared to finer particles (Pérez, 1991; Brown and Schoknecht, 2001; Al-Awadhi, 2013). Several studies reported significant ruts being created in designated ORV areas in desert (Webb et al., 2013), coastal (Davies et al., 2016) and grassland habitats (Trip and Wiersma, 2015). The depth of rutting may be attributed to increasing vehicle passes (Webb et al., 2013) or habitat type (Trip and Wiersma, 2015), but factors such as seasonal changes in soil moisture have been largely understudied. For example, in a study comparing the impacts of ORV use on grassland, forest, and wetland habitats, rut depth was deeper on heath and bog trails compared to forested trails and on wet soils compared to dry (Trip and Wiersma, 2015).
Physical alterations to the soil structure can also result in changes to the hydrology and erosion at the site. Specifically, ORV areas were found to have lower infiltration rates and soil moisture in grassland/shrubland (Wilshire et al., 1978; Eckert et al., 1979; Payne et al., 1983) and desert habitats (Al-Awadhi, 2013). Further, thaw patterns following disturbance with ATVs with different weights and ground pressures were dependent on the seasonal timing and type of the disturbance (Racine and Ahlstrand, 1991). In early spring, lighter ATVs with higher ground pressure produced more subsurface thaw than heavier vehicles with lower ground pressure, but in the fall, heavier vehicles had a greater impact on thaw patterns (Racine and Ahlstrand, 1991). Sediment yield and erosion were found to be significant in coastal (Thompson and Schlacher, 2008; Houser et al., 2013; Davies et al., 2016), desert (Al-Awadhi, 2013; Belnap et al., 2014), grassland (Eckert et al., 1979; Nauman et al., 2018), forest (Sack and da Luz, 2003; Padgett et al., 2008; Marion et al., 2019; Ramos-Scharrón, 2021), and wetland habitats (Trip and Wiersma, 2015). The sensitivity to erosion may also be dependent on habitat type, as Trip and Wiersma (2015) found that bogs had low resistance to erosion compared to a dry, forested site, which tended to be resistant to on-trail erosion. Depending on the system, sediment yield may be more significant than hydrological changes. For example, in a stream crossing, sediment impacts were greater than runoff impacts; although, this was highly variable depending on the geomorphology of the stream (Marion et al., 2014).
While physical properties of the soil will likely be impacted to some extent, changes in soil chemistry following ORV activity were more indeterminate. For instance, following ORV activity, electrical conductivity (EC) was found to be higher in both desert and grassland soils (Assaeed et al., 2019; Navas Romero et al., 2019), and soil pH tended to be lower in grasslands (Pérez, 1991; Navas Romero et al., 2019), but the same parameters were not altered a desert habitat (Brown and Schoknecht, 2001; Assaeed et al., 2019). It has been suggested that these differences in soil EC and pH could been attributed to differences in soil texture induced by ORV activity (Pérez, 1991; Assaeed et al., 2019), although climate and the intensity and frequency of ORV activity will also likely play a role.
These changes in soil EC and pH can alter nutrient availability and cycling (Arnold et al., 2005; Singh et al., 2011). Soil nutrients were lower on vehicle tracks in desert (Brown and Schoknecht, 2001) and forest habitats (Webb et al., 1978). However, soil chemical properties were not always negative, as reductions in soil organic matter (SOM) were found in grassland soils (Wilshire et al., 1978; Pérez, 1991), whereas others found no difference in SOM, total N or P on desert soils (Brown and Schoknecht, 2001; Assaeed et al., 2019). Further research is needed to understand whether these chemical changes to the soil are more sensitive in certain ecosystems, climates or under different ORV use levels. For instance, Belnap and Eldridge (2001) found a reduction in nitrogenase activity after 4 passes of a four-wheel drive on desert soil, suggesting that low levels of ORV activity may have significant effects on soil nutrients while other parameters may be more resistant to change.
ORV activity was found to greatly reduce vegetative cover in all the habitats studied, including coastal (Hosier and Eaton, 1980; Anders and Leatherman, 1987; Schlacher et al., 2008b; Kelly, 2014), desert (Luckenbach and Bury, 1983; McGrann et al., 2005; Al-Awadhi, 2013; Dewidar et al., 2016; Knisley et al., 2018; Assaeed et al., 2019; Cheung et al., 2021), and grassland/shrubland habitats (Wilshire et al., 1978; Kinugasa et al., 2015). Vegetation loss tended to increase with increasing levels of ORV use (Rickard and Brown, 1974; Kutiel et al., 2000; Kelly, 2014), vehicle weight (Ahlstrand and Racine, 1993), and in the weeks and months following ORV disturbance (Kutiel et al., 2000). Vegetative cover is plays an important role as a shielding boundary between the soil and atmosphere and is not only important for regulating sediment availability for atmospheric dust emission, but in regulating surface albedo and surface temperature (Al-Awadhi, 2013; Al-Awadhi et al., 2014; Cheung et al., 2021). Increased soil exposure resulting from ORV traffic were found to increase daytime surface temperatures in a desert (Cheung et al., 2021) and in a grassland (Webb et al., 2013).
In addition to vegetation loss, ORV activity can significantly reduce vegetation height and increase injury to some plants. In a desert habit, a negative association was found between woody plant height and distance from the tracks in ORV impact areas (Assaeed et al., 2019). ORVs were found to reduce vegetation height, which was negatively associated with the number of passes (Rickard et al., 1994), vehicle weight (Ahlstrand and Racine, 1993), and time since the disturbance (Kutiel et al., 2000). In a study exploring vehicles with different weights, 1-Ton and 4-Ton four-wheel drive vehicles, sward height was reduced by all treatments, except a single pass with the 1-Ton vehicle (Hirst et al., 2003). Although several authors reported significant visual damage to shrubs (Vollmer et al., 1977; Sparrow et al., 1978; Ahlstrand and Racine, 1993), damage to shrub leaves from fugitive dust caused by ORV activity is unlikely to harm native vegetation in areas with frequent rainfall (Padgett et al., 2008).
Disturbance by ORVs can also lead to community-level changes and often results in decreases in species richness (Hosier and Eaton, 1980; Thompson and Schlacher, 2008; Andreyashkina, 2012; Navas Romero et al., 2019). However, the intensity of the plant community response may depend on site characteristics, such as physical damage to the soil caused by ORV use, microclimatic conditions (e.g., soil moisture or nutrient availability), the level of ORV use, and/or the type of vegetation. For instance, rut depth was associated with impacts to coastal dune vegetation, where total native species decreased on ATV trails and on the edge of trails, and non-native species slightly increased 15 m past the edge of trails (Hogan and Brown, 2021). Further, in a study conducted by Trip and Wiersma (2015), three ecosystem (boreal forest, heath, and bogs) were found to differ in resistance and resilience to both soil erosion as well as indirect off-trail vegetative responses. Here, dry forested areas were less resistant to ORV activity and had sharper edges (stronger species turnover gradients) than heath or bogs. Heath trails were resistant and had the softest edge (lowest amount of species turnover) compared to forests and bogs (Trip and Wiersma, 2015). Similarly, differences were found in the community responses between different types of grasslands, where native species were not affected in serpentine grasslands, but native species were reduced near ORV trails in non-serpentine grasslands (Gelbard and Harrison, 2003).
Alterations to plant communities because of ORV use can impact the success of plant species differently, and it will be important to consider species responses when managing ORV areas. For example, ORV can reduce plant species that provide important ecosystem services like Ammophila breviligulata (American dune grass), a key dune-stabilizing species (Brodhead and Godfrey, 1977; Hogan and Brown, 2021). It has also been reported that certain plant functional types may be more susceptible to ORV use and can be reduced with very few passes by an ORV, such as lichens (Belnap, 2002), annual plants (Vollmer et al., 1977; Adams et al., 1982), and other herbaceous and perennial plants (Luckenbach and Bury, 1983; Groom et al., 2007). Unfortunately, ORV areas can also decrease the success of threatened or rare plants, such as the rare desert plant, Astragalus magdalenae var. peirsonii (Peirson's milk-vetch) (Groom et al., 2007). While ORV activity can be damaging to vegetation, there are also some instances where ORV activity increases the success of certain species, namely exotic or unwanted weeds (Kinugasa et al., 2015; Assaeed et al., 2019; Navas Romero et al., 2019), but also native species that can take advantage of the microenvironment created by ORV tracks (Brown and Schoknecht, 2001; Taylor and Raney, 2013). For instance, an endangered bog species, Drosera filiformis (thread-leaved sundew), was found to be significantly higher in water-filled ATV tracks than the drier, undisturbed Sphagnum around them (Taylor and Raney, 2013). Also, in a degraded desert ecosystem, species density and richness were much greater in slight depressions caused by a single passage of a vehicle (Brown and Schoknecht, 2001). While it has been suggested that ORV may influence plant communities through the transport of seeds attached to vehicle tires, the rate at which vehicles accrue seeds can vary greatly (Lonsdale and Lane, 1994; Rew et al., 2018). Factors such as driving surface, surface conditions, season of use, and type of vehicle impacted the rate of seed accrual on ORVs (Rew et al., 2018).
In grassland habitats, cover and aboveground biomass were found to recover 4 years following ORV road abandonment (Kinugasa et al., 2015; Navas Romero et al., 2019), and 1 year after applying low levels of ORV disturbance (2 or 8 passes a month) (Payne et al., 1983). In a coastal ecosystem, aboveground vegetation was completed destroyed after 270 or 650 passes by a four-wheel drive vehicle, but there was an adequate amount of belowground biomass remaining allowing for a small amount of regrowth after cessation of the treatments (Brodhead and Godfrey, 1977). While vegetative regrowth can show relatively quick recovery, impacts to the vegetative community may be more long-term. For instance, in tundra ecosystems, floristic and phytocenotic diversity were significantly lower 20 years after ORV use was restricted (Andreyashkina, 2012), and grasses and sedges were generally first to revegetate areas impacted by ORV activity (Sparrow et al., 1978). In grasslands, species that can germinate, root, and survive on compacted surfaces are the first to establish, and overtime, improvements to surface microenvironmental conditions can led to the colonization of other plants, increasing species richness and diversity (Li et al., 2006). However, there are instances where abandoned roads affect the surrounding vegetation through the colonization and expansion of clonal, often exotic, species (Kinugasa et al., 2015). Further, the seed banks on these abandoned roads can be limited (Kinugasa and Oda, 2014).
Although ORV activity was generally found to have significant impacts on invertebrate populations, communities, behaviors, and transport, the vast majority of studies explored impacts to benthic macroinvertebrates. ORV areas were found to have lower species richness, diversity, and abundance of benthic macroinvertebrate communities compared to areas where ORV use was restricted (Schlacher and Thompson, 2007; Schlacher et al., 2008a; Walker and Schlacher, 2011; Davies et al., 2016; Bom and Colling, 2020). While most of these ORV areas had high traffic volumes (hundreds of vehicles per day), one study reported similar shifts in community composition and structure with low levels of ORV traffic per day (7–40 vehicles/day) (Davies et al., 2016). These negative impacts on benthic macroinvertebrates are likely species dependent, as some species have been reported to be highly tolerable to ORV activity, including Bullia rhodostoma (bivalve), Donax variabilis (bivalve), Donax sordidus (bivalve), Emerita talpoida (mole crabs), and Tylos capensis (isopod). Whereas other species, Donax deltoides (bivalve), Gastrosaccus psammodytes (mysid), and Ocypodinae spp (ghost crabs), were highly susceptible to injury or death as a result of ORV use (Wolcott and Wolcott, 1984; Schlacher et al., 2007).
Ghost crabs (Ocypodinae spp) are sensitive to human stressors, making them useful ecological indicators to access the ecological condition of beaches and dunes in ORV areas(Moss and McPhee, 2006; Schlacher and Lucrezi, 2010). Ghost crabs were not only found to have higher mortality and injury rates as a consequence of ORV activity (Wolcott and Wolcott, 1984; Schlacher et al., 2007) but were also found to have altered behavior patterns and movements (Moss and McPhee, 2006; Schlacher et al., 2007; Lucrezi and Schlacher, 2010; Lucrezi et al., 2014). Ghost crab mortality decreased with depth, and individuals were protected by burrows as shallow as 5 cm in high traffic areas (Wolcott and Wolcott, 1984; Schlacher et al., 2013). Since ghost crabs are nocturnal, night-time traffic restrictions have been suggested as a potential management strategy (Hobbs et al., 2008). However, a study exploring different types of ORV restrictions, including banning nighttime time, found that ghost crab density only increased when coastal driving was restricted to 24 h (Hobbs et al., 2008).
While it is generally accepted that ORV have a largely negative impact on benthic macroinvertebrate community structure and function, there were only three studies that explored impacts on other terrestrial invertebrates; all of which were conducted on desert sand dunes. These studies revealed that arthropod abundance decreased in high traffic ORV areas (Luckenbach and Bury, 1983) with a notably negative effect on Coleoptera (Luckenbach and Bury, 1983; Van Dam and Van Dam, 2008; Knisley et al., 2018). Declines in Coleoptera have been largely attributed to removal of available plant food and habitat, although ORVs likely adversely affect life histories. For example, the tiger beetle (Cicindela albis-sima) relies on compaction and moisture to provide cohesive sand required for larvae to maintain burrows during development (Knisley et al., 2018). Compacted sand, soil moisture, and vegetation that support larval prey are largely absent in heavy ORV-use areas, reducing tiger beetle larvae abundance (Knisley et al., 2018). Ultimately, research into the effects of ORVs on terrestrial invertebrates is limited, and more research is needed to better understand how these organisms respond to ORV use.
Finally, in addition to affecting invertebrate communities and species directly, ORVs have been attributed to the transport of aquatic invertebrates. Invasive species including the red swamp crayfish (Procambarus clarkia) and bladder snail (Physella acuta) can be transported via mud adhering to ORVs and can survival long distances, 83.2 and 92.2 km, respectively (Banha et al., 2014). Unintentional transport of aquatic invertebrates adhering to vehicles used for ecological field work may result in frequent dispersal of aquatic invertebrates, including an invasive species (Artemia franciscana), on a local scale (Waterkeyn et al., 2010).
ORV use resulted in numerous responses of various bird populations and communities; however, these studies were limited to responses of four species and primarily focused on coastal birds. ORV activity was found to reduce total shorebird abundance (Tarr et al., 2010) but only reduced the abundance of 2 of 18 species of breeding songbirds (Barton and Holmes, 2007). Further, a study at a recreational area in California found non-negative effects on community-level bird abundance and no discernable effect on diversity in both the winter and spring (Cole et al., 2019), suggesting that effects of ORV use on bird communities may vary depending upon the habitat, driver behavior (e.g., speed, direction, etc.), or species-specific responses. For instance, species of wading birds (e.g., egrets/herons) (Stolen, 2003) and golden eagles (Aquila chrysaetos) (Spaul and Heath, 2017) were more likely to be disturbed when vehicles slowed or stopped adjacent to them than when vehicles continued driving by. Slower vehicle speeds (<30 km h−1) and a buffer distance of at least 25 m improved bird escape response of shore birds (Schlacher et al., 2013).
From the select number of species observed, ORV activity can affect both behavior and abundance of some birds. ORV activity reduced the amount of time American oystercatchers (H. palliates) were found to be on their nest during ORV events (McGowan and Simons, 2006; Borneman et al., 2016; Felton et al., 2018) and resulted in greater nest desertion and abandonment in breeding songbirds (Barton and Holmes, 2007). Territories with ORV use were less occupied by golden eagles (A. chrysaetos) than territories without ORV use (Steenhof et al., 2014; Spaul and Heath, 2016).
In addition to altering behavior and abundance of birds, ORV were found to reduce the reproductive success of several species, including American oystercatchers (H. palliates) (Borneman et al., 2016), golden eagles (A chrysaetos) (Steenhof et al., 2014), piping povers (Charadrius melodus) (DeRose-Wilson et al., 2018), and the northern spotted owl (Strix occidentalis caurina) (Hayward et al., 2011). Avian stress was assessed using various physiological responses of S. occidentalis caurina and H. palliates, where S. occidentalis caurina were found to have better nutrition near roads, based on fecal indicators (Hayward et al., 2011) but may (Felton et al., 2018) or may not (Borneman et al., 2014) produce changes in the heart rate in H. palliates.
Most of the studies on mammalian responses to ORV activity explored species-level behavioral responses, and only two studies explored community-level responses, which found conflicting results. Here, Luckenbach and Bury (1983) found that mammalian abundance declined in an ORV area reporting 5,000 dune buggies on an average weekend. Conversely, after experimentally driving over an area a specified number of times for a year and a half, more young rodents were trapped on experimental plots, which was attributed to regrowth from damaged shrubs (Vollmer et al., 1977). There are several potential reasons for these differences, but it is likely that there were differences in data collection methods and variation in the level of disturbance caused by ORVs.
Similarly, while most studies exploring species-specific responses to ORV activity found significant behavioral responses, certain species like the American Marten (Martes americana) may be resistant to ORV disturbance (Zielinski et al., 2008). Conversely, mammals, including elk (Cervus spp.) (Preisler et al., 2006; Naylor et al., 2009; Wisdom et al., 2018), moose (Alces alces) (Shanley and Pyare, 2011; Brown et al., 2018), mountain goats (Oreanmnos americanus) (St-Louis et al., 2013), Florida panthers (Puma concolor coryi) (Janis and Clark, 2002), and kit foxes (Vulpes macrotis) (Jones et al., 2017), were found to alter their movement and habitat selection as a result of ORV activity. During ORV activities, several species were found to avoid areas when ORVs were in use (Janis and Clark, 2002; Brown et al., 2018; Wisdom et al., 2018) and responded to ORVs at relatively long distances (1,000 m) (Preisler et al., 2006; Shanley and Pyare, 2011) with relatively low levels of vehicular activity (Shanley and Pyare, 2011). Species like elk (C. elaphus) may be particularly sensitive to off-road vehicle use, as feeding times were found to decrease and travel time increased during ATV exposure compared to other recreational activities (biking, hiking, and horseback riding) (Naylor et al., 2009). Mountain goats behavior levels were influenced by the direction and speed of ATVs, but not by other environmental or group related factors (e.g., time of year, group size/type) (St-Louis et al., 2013).
While mammals often demonstrate behavioral responses to ORVs, there is a need for a clearer understanding of the impacts of ORVs on mammal communities and populations with a specific focus on understanding how the level or timing of ORV use affect these responses and identifying sensitive species and/or habitats to ORV activities. Further, research is needed on the demographic and physiological responses of mammals to ORV activity.
All of the studies explored impacts or ORV activities to reptiles, except Hunkapiller et al., 2009, where ATV traffic in a forest was found to have no effect on abundance, richness, evenness, or diversity amphibians/reptiles. Although, traffic levels for this study were relatively low with only 1 or 2 users per day (Hunkapiller et al., 2009). Lizards in desert ecosystems were found to be differentially resistant to ORV activities. While there is some evidence that lizard populations can be adversely affected by ORV activity (Busack and Bury, 1974; Luckenbach and Bury, 1983; Tull and Brussard, 2007), there was evidence that certain species of lizards are resistant to ORV activity (Vollmer et al., 1977; Grant and Doherty, 2009). For instance, in an experimental study, the flat-tailed horned lizard (Phrynosoma mcallii) suffered little, if any, direct mortality from ORV traffic during hibernation even those hibernating at shallow depths (Grant and Doherty, 2009). However, ORV was found to impact the behavior of P. mcallii, altering habitat select to one different from its previously defined habitat (Beauchamp et al., 1998). Further, there is also evidence that ORV activity can increase wildlife stress (Tull and Brussard, 2007). In this case, Tull and Brussard (2007) used fluctuating asymmetry of the bilateral head-scale patterns of phrynosomatid lizards like Sceloporus occidentalis, an indicator of stress. Finally, increased vehicular activity in riparian habitats was found to increase softshell turtle (Apalone spp.) nest mortality, where the most vulnerable nests were shallower, driven over more slowly, and turned upon (Godwin et al., 2021). Further, ruts from ORVs were found to interfere with the ability of hatchling Loggerhead turtles to reach the ocean (Hosier et al., 1981).
In our review, we relied on the authors descriptions of off-road vehicle use, but it was often unclear as to the extent of ORV trails or levels of use. Thus, it will be important for future research to provide clear descriptions of the types of activities occurring (e.g., recreational, research, hunting, etc.), the level of ORV activity (e.g., types of vehicles, number of vehicles, timing of use, days in use, etc.), and the extent of ORV trails when possible. Further, this review was limited to wheeled ORVs, as the search terms only identified one paper using snowmobiles, despite several studies exploring the impacts of snowmobiles (e.g., Crête and Larivière, 2003; Mahoney et al., 2016; Mullet and Morton, 2021). This suggests that authors often do not apply the terms off-road vehicle or off-highway vehicle to tracked vehicles like snowmobiles. Since this systematic review was limited to published literature, there may be gray literature that may provide more insight on this information as well as more site-specific responses and concerns.
While off-road driving has been a popular activity in the United States for a long time and has reportedly increased at a global level (Schlacher and Thompson, 2008; Al-Awadhi, 2013; Kinugasa et al., 2015; Dewidar et al., 2016; Enríquez-de-Salamanca, 2021), it was difficult to determine the global extent off-road vehicle use. In a report from the US Forest Service assessing the status and trends of outdoor recreation across the United States, nature-based recreation grew by 7.1 % and the number of activity days grew by 40% in the first decade of the 21st century (Cordell, 2012). Of the activities explored, driving motorized off-highway was the fastest growing by a wide margin (Cordell, 2012), but current US and global motorized off-road driving trends are vague. New technologies may aid in the management and monitoring of regulated and unauthorized ORV trails on various spatial and temporal scales. For instance, Navas Romero et al. (2019) identified a network of unauthorized trails created by users (i.e., not professionally designed) in Argentina using popular, volunteered geographic information platforms like wikilock (www.wikiloc.com) and runstatic (www.runtastic.com/es). Remote sensing using high spatial resolution images, such as those obtained by satellites GeoEye-1, IKONOS-2, Terra, and Aqua, and associated products and instrumentation [e.g., Worldview and Moderate Resolution Imaging Spectroradiometer (MODIS)] have also been used to monitor the spatial-temporal effects of ORV activities (Dewidar et al., 2016; Al-Dousari et al., 2019; Cheung et al., 2021). These types of technology, crowd-sourced data, and government registration of ORVs may aid in assessing the extent of ORV trails around the world and will be pertinent for future ORV trail design. Unfortunately, while there are some trail management publications that provide insight into designing, constructing, and maintaining natural-surfaced trails for activities like hiking, horseback riding, and ATV use, there is a lack of literature that provides scientific basis for this guidance (Marion and Wimpey, 2017).
Management of ORV activities is multifaceted, requiring consideration of the needs and aspirations of multiple users with often conflicting interests, including social, economic, and environmental factors (Schlacher and Thompson, 2007; Enríquez-de-Salamanca, 2021). While entirely excluding vehicular traffic on the landscape is unrealistic, it will be important to maximize the social and economic benefits of outdoor recreation and ecotourism while minimizing the potentially adverse ecological impacts of ORV traffic (Westcott and Andrew, 2015). Current research on environmental impacts of ORV traffic is biased to coastal and desert ecosystems in the northern hemisphere, often does not address the mechanisms that may produce ecological impacts (e.g., intensity of vehicular disturbance or ecosystem- or species-specific sensitivity to ORV activities), and frequently focuses on short-term responses.
Future research is needed to explore the resistance (short-term responses) and resilience (long-term responses) of a broad range of ecosystems to gain better insight into the establishment and management of ORV areas. Generally, controlled research is needed to understand the effectiveness of suggested management plans and to explore potential mechanisms causing the apparent environmental impacts by ORVs. For instance, in coastal regions, management suggestions have focused on the idea that the more compact areas of the lower and middle beach are less sensitive to ORVs (Schlacher and Thompson, 2007), resulting in suggestions like limiting beach traffic to daytime hours (Moss and McPhee, 2006) and restricting driving during high tides to protect the upper shore (Cordell et al., 2005). However, Schlacher and Thompson (2007) found that these restrictions resulted in greater exposure of intertidal fauna to vehicles. Thus, management plans should consider ecosystem-level consequences before establishing ORV areas.
Management actions to maintain sustainable use of ORVs for activities like recreation, harvesting, hunting, or search and rescue will require directing ORV activity to designated areas and limiting access and proliferation of informal tracks into undisturbed areas (Payne et al., 1983; Westcott and Andrew, 2015; Davies et al., 2016; Jones et al., 2017; Navas Romero et al., 2019). Expanding ORV road networks will likely result in effective habitat loss or habitat compression for species like kit foxes or elk (Jones et al., 2017; Wisdom et al., 2018). Thus, appropriate measures should be taken to prevent the construction of new roads in undisturbed or ecologically important habitats (Gelbard and Belnap, 2003; Jones et al., 2017). Practical solutions include education and strict enforcement or rules and regulations (e.g., establish physical barriers, require ORV registration, etc.) (Watson et al., 1996; Jones et al., 2017). Managers will need to determine whether areas are suitable for ORV activity by considering the flora and fauna that inhabit the area and restricting or regulating ORV traffic to professionally designated tracks.
Regulations that have been suggested for minimize environmental impacts include reducing or restricting ORV use during certain times of the day or season (Watson et al., 1996; Barton and Holmes, 2007; Hobbs et al., 2008; DeRose-Wilson et al., 2018), setting limits on traffic volumes (Racine and Ahlstrand, 1991; Schlacher et al., 2008a) and the types of vehicles used (Ahlstrand and Racine, 1993), and informing driver behavior (Watson et al., 1996). However, more information on the relationship between traffic intensity, timing, and impact size are needed to better inform threshold levels of traffic and to understand the underlying mechanisms for ecological impacts in various habitats. For instance, several have attributed the severity of impacts to the high ground contact pressure from ORVs (Monz et al., 2010; Navas Romero et al., 2019); however, in a shrub-tussock tundra, differential responses to ground pressure were found depending of the season of use (Racine and Ahlstrand, 1991). The authors suggest that activity should be confined to heavier vehicles with low ground pressure early in the thaw season and lighter vehicles late in the thaw season (Racine and Ahlstrand, 1991). Regulations may also include ways to alter driver behavior by not only displaying notices and signs but making users aware of the reasons for these regulations (Watson et al., 1996). For example, if mangers established “no stopping” zones, it should be explained that birds like golden eagles (Aquila chrysaetos) are more disturbed when passengers exit their vehicles than when they continue driving (Stolen, 2003; Spaul and Heath, 2017).
Advances in technology will be important understanding the extent of ORV impacts on the environment and may be used to develop methods for reducing environmental impacts. Remote sensing and GIS technology will be important tools for monitoring the extent of ORV networks, the intensity and frequency of use, and evaluating driver behavior (e.g., Mohtashami et al., 2012; Tømmervik et al., 2012). While vehicle technologies have focused on improved vehicle performance like vehicle–soil dynamics (e.g., Li and Sandu, 2007; Lee and Gard, 2014) or terrain navigation (e.g., Armbrust et al., 2010; Hsu, 2016; Xiao et al., 2021), more research is needed into developing technologies that minimize environmental impacts on the terrain, such as controlling the ground (contact) pressure exerted by the vehicle on the terrain by optimizing tread design, tire size, and tire inflation rates.
While the extent of ORV use remains unclear, research has shown that ORVs are altering habitats and impacting the flora and fauna that inhabit them. However, the sensitivity of various ecosystems to the short-term and long-term effects of ORV use remains uncertain. Different aspects of the site (e.g., soil type, vegetation, soil moisture, terrain, prior disturbance, etc.) and/or the type of ORV disturbance applied (e.g., vehicle weight/type, frequency of use, time of disturbance, etc.) likely play important roles in the apparent impacts of ORV use. Further, animal responses to ORV use are likely species-specific and influenced by driver behavior (e.g., speed, direction, frequency and intensity of use, etc.) and the time of the disturbance (e.g., breeding season, stage of life cycle, nighttime/daytime). There is a need for a clearer understanding of how ORVs impact demographic and physiological responses of animals. More research is needed to understand the mechanisms that cause the different responses of soil, plant, and animals to ORVs in a broad range of ecosystems to support real-time management and conservation efforts.
Publicly available datasets were analyzed in this study. This data can be found here: https://figshare.com/articles/dataset/ORV_systematic_review/16899610.
LP developed the methodology, conducted the systematic review, and wrote the manuscript. LF provided feedback on the methodology and drafts of the manuscript. All authors contributed to the article and approved the submitted version.
Funding was provided through MITACS Accelerate Industrial Postdoc fellowship (Application ref. IT23499) in partnership with KIQ X Industries Inc. and Thompson Rivers University.
The authors declare that this study received funding from KIQ X Industries Inc. The funder was not involved in the study design, collection, analysis, interpretation of data, the writing of this article or the decision to submit it for publication.
The authors declare that the research was conducted in the absence of any commercial or financial relationships that could be construed as a potential conflict of interest.
All claims expressed in this article are solely those of the authors and do not necessarily represent those of their affiliated organizations, or those of the publisher, the editors and the reviewers. Any product that may be evaluated in this article, or claim that may be made by its manufacturer, is not guaranteed or endorsed by the publisher.
The Supplementary Material for this article can be found online at: https://www.frontiersin.org/articles/10.3389/fevo.2021.805707/full#supplementary-material
Adams, J. A., Endo, A. S., Stolzy, L. H., Rowlands, P. G., and Johnson, H. B. (1982). Controlled experiments on soil compaction produced by off-road vehicles in the Mojave Desert, California. J. Appl. Ecol. 19:167. doi: 10.2307/2403001
Ahlstrand, G. M., and Racine, C. H. (1993). Response of an Alaska, USA, shrub-tussock community to selected all- terrain vehicle use. Arct. Alp. Res. 25, 142–149. doi: 10.2307/1551551
Al-Awadhi, J. M. (2013). A Case assessment of the mechanisms involved in human-induced land degradation in Northeastern Kuwait. Land Degrad. Dev. 24, 2–11. doi: 10.1002/ldr.1090
Al-Awadhi, J. M., Al-Dousari, A. M., and Khalaf, F. I. (2014). Influence of land degradation on the local rate of dust fallout in Kuwait. Atmos. Clim. Sci. 04, 437–446. doi: 10.4236/acs.2014.43042
Al-Dousari, A. M., Alsaleh, A., Ahmed, M., Misak, R., Al-Dousari, N., Al-Shatti, F., et al. (2019). Off-road vehicle tracks and grazing points in relation to soil compaction and land degradation. Earth Syst. Environ. 3, 471–482. doi: 10.1007/s41748-019-00115-y
Anders, F. J., and Leatherman, S. P. (1987). Effects of off-road vehicles on coastal foredunes at Fire Island, New York, USA. Environ. Manage. 11, 45–52. doi: 10.1007/BF01867178
Andreyashkina, N. I. (2012). Composition of plant communities in natural and technogenically disturbed ecotopes on watersheds of the Yamal Peninsula: Floristic diversity. Russ. J. Ecol. 43, 19–23. doi: 10.1134/S1067413611060038
Armbrust, C., Braun, T., Föhst, T., Proetzsch, M., Renner, A., Schäfer, B. H., et al. (2010). Ravon: the robust autonomous vehicle for off-road navigation. Using Robot Hazard Environ. Landmine Detect Demining Other Appl. 2011, 353–396. doi: 10.1533/9780857090201.3.353
Arnold, S., Doran, J., Schepers, D., and Wienhold, B. (2005). Portable probes to measure electrical conductivity and soil quality in the field. Commun. Soil Sci. Plant Anal. 36, 2271–2287. doi: 10.1080/00103620500196689
Assaeed, A. M., Al-Rowaily, S. L., El-Bana, M. I., Abood, A. A., Dar, B. A., and Hegazy, A. K. (2019). Impact of off-road vehicles on soil and vegetation in a desert rangeland in Saudi Arabia. Saudi J. Biol. Sci. 26, 1187–1193. doi: 10.1016/j.sjbs.2018.05.001
Banha, F., Marques, M., and Anastácio, P. M. (2014). Dispersal of two freshwater invasive macroinvertebrates, Procambarus clarkii and Physella acuta, by off-road vehicles. Aquat. Conserv. Mar. Freshw. Ecosyst. 24, 582–591. doi: 10.1002/aqc.2453
Barton, D. C., and Holmes, A. L. (2007). Off-highway vehicle trail impacts on breeding songbirds in Northeastern California. J. Wildl. Manage. 71, 1617–1620. doi: 10.2193/2006-026
Beauchamp, B., Wone, B., Bros, S., and Kutilek, M. (1998). Habitat use of the flat-tailed horned lizard (Phrynosoma mcallii) in a disturbed environment. J. Herpetol. 32, 210–216. doi: 10.2307/1565299
Belnap, J. (2002). Impacts of off-road vehicles on nitrogen cycles in biological soil crusts: resistance in different U.S. deserts. J. Arid. Environ. 52, 155–165. doi: 10.1006/jare.2002.0991
Belnap, J., and Eldridge, D. (2001). Disturbance and recovery of biological soil crusts. In: Belnap, J, and Lange, O, editors. Biological Soil Crusts: Structure, Function, and Management. Berlin Heidelberg, New York, NY: Springer.
Belnap, J., Walker, B. J., Munson, S. M., and Gill, R. A. (2014). Controls on sediment production in two U.S. deserts. Aeolian. Res. 14, 15–24. doi: 10.1016/j.aeolia.2014.03.007
Besserer, F. A., and Caron, N. R. (2013). Patterns of outdoor recreational injury in northern British Columbia. Wilderness Environ. Med. 24, 397–401. doi: 10.1016/j.wem.2013.04.004
Bom, F. C., and Colling, L. A. (2020). Impact of vehicles on benthic macrofauna on a subtropical sand beach. Mar. Ecol. 41, 1–9. doi: 10.1111/maec.12595
Borneman, T. E., Rose, E. T., and Simons, T. R. (2014). Minimal changes in heart rate of incubating American Oystercatchers (Haematopus palliatus) in response to human activity. Condor 116, 493–503. doi: 10.1650/CONDOR-14-48.1
Borneman, T. E., Rose, E. T., and Simons, T. R. (2016). Off-road vehicles affect nesting behaviour and reproductive success of American Oystercatchers Haematopus palliatus. Ibis 158, 261–278. doi: 10.1111/ibi.12358
Brodhead, J. M., and Godfrey, P. J. (1977). Off road vehicle impact in Cape Cod national seashore: disruption and recovery of dune vegetation. Int. J. Biometeorol. 21, 299–306. doi: 10.1007/BF01552884
Brown, C. L., Kielland, K., Brinkman, T. J., Gilbert, S. L., and Euskirchen, E. S. (2018). Resource selection and movement of male moose in response to varying levels of off-road vehicle access. Ecosphere 9:e02405. doi: 10.1002/ecs2.2405
Brown, G., and Schoknecht, N. (2001). Off-road vehicles and vegetation patterning in a degraded desert ecosystem in Kuwait. J. Arid Environ. 49, 413–427. doi: 10.1006/jare.2000.0772
Busack, S. D., and Bury, R. B. (1974). Some effects of off-road vehicles and sheep grazing on lizard populations in the mojave desert. Biol. Conserv. 6, 179–183. doi: 10.1016/0006-3207(74)90064-0
Cheung, S. Y., Walker, I. J., Myint, S. W., and Dorn, R. I. (2021). Assessing land degradation induced by recreational activities in the Algodones Dunes, California using MODIS satellite imagery. J. Arid Environ. 185:104334. doi: 10.1016/j.jaridenv.2020.104334
Cole, D. N. (2019). The Relationship between Amount of Visitor Use and Social Impacts. (US Department of the Interior, National Park Service).
Cole, J. S., Michel, N. L., Siegel, R. B., and Somilleda, N. (2019). Effects of off-highway vehicles on avian abundance and diversity in a designated vehicular recreation area. Avian Conserv. Ecol. 14:9. doi: 10.5751/ACE-01422-140209
Cordell, H. K., Betz, C. J., Green, G., and Owens, M. (2005). Off-Highway Vehicle Recreation in the United States, Regions and States: A National Report from the National Survey on Recreation and the Environment (NSRE). Availabkle online at: http://www.fs.fed.us/recreation/programs/ohv/OHV_final_report.pdf (accessed October 18, 2021).
Cordell, K. H. (2012). Outdoor Recreation Trends and Futures: A technical document supporting the Forest Service 2010 RPA Assessment. Asheville, NC: U.S. Dept. of Agriculture.
Crête, M., and Larivière, S. (2003). Estimating the costs of locomotion in snow for coyotes. Can. J. Zool. 81, 1808–1814. doi: 10.1139/z03-182
Crisfield, V., MacDonald, S., and Gould, A. (2012). Effects of recreational traffic on alpine plant communities in the northern Canadian Rockies. Arctic Antarct. Alp. Res. 44, 277–287. doi: 10.1657/1938-4246-44.3.277
Davies, R., Speldewinde, P. C., and Stewart, B. A. (2016). Low level off-road vehicle (ORV) traffic negatively impacts macroinvertebrate assemblages at sandy beaches in south-Western Australia. Sci. Rep. 6, 1–8. doi: 10.1038/srep24899
DeRose-Wilson, A. L., Hunt, K. L., Monk, J. D., Catlin, D. H., Karpanty, S. M., and Fraser, J. D. (2018). Piping plover chick survival negatively correlated with beach recreation. J. Wildl. Manage. 82, 1608–1616. doi: 10.1002/jwmg.21552
Dewidar, K., Thomas, J., and Bayoumi, S. (2016). Detecting the environmental impact of off-road vehicles on Rawdat Al Shams in central Saudi Arabia by remote sensing. Environ. Monit. Assess. 188:396. doi: 10.1007/s10661-016-5400-6
Eckert, R. E., Wood, M. K., Blackburn, W. H., and Peterson, F. F. (1979). Impacts of off-road vehicles on infiltration and sediment production of two desert soils. J. Range Manage. 32, 394–397. doi: 10.2307/3898025
Enríquez-de-Salamanca, Á (2021). The impact of quad tourism: a preventive action for the Mediterranean. Environ. Dev. Sustain. 23, 9454–9476. doi: 10.1007/s10668-020-01036-x
Felton, S. K., Pollock, K. H., and Simons, T. R. (2018). Response of beach-nesting American Oystercatchers to off-road vehicles: an experimental approach reveals physiological nuances and decreased nest attendance. Condor 120, 47–62. doi: 10.1650/CONDOR-17-84.1
Gelbard, J. L., and Belnap, J. (2003). Roads as conduits for exotic plant invasions in a semiarid landscape. Conserv. Biol. 17, 420–432. doi: 10.1046/j.1523-1739.2003.01408.x
Gelbard, J. L., and Harrison, S. (2003). Roadless habitats as refuges for native grasslands: interactions with soil, aspect, and grazing. Ecol. Appl. 13, 404–415. doi: 10.1890/1051-0761(2003)013[0404:RHARFN]2.0.CO;2
Godwin, C. D., Doody, J. S., and Crother, B. I. (2021). The impact of ATVs on survival of softshell turtle (Apalone spp.) nests. J. Herpetol. 55, 201–207. doi: 10.1670/20-023
Grant, T. J., and Doherty, P. F. (2009). Potential mortality effects of off-highway vehicles on the flat-tailed horned lizard (Phrynosoma mcallii): a manipulative experiment. Environ. Manage. 43, 508–513. doi: 10.1007/s00267-008-9217-0
Greller, A. M., Goldstein, M., and Marcus, L. (1974). Snowmobile impact on three alpine tundra plant communities. Environ. Conserv. 1, 101–110. doi: 10.1017/S0376892900004227
Groom, J. D., McKinney, L. B., Ball, L. C., and Winchell, C. S. (2007). Quantifying off-highway vehicle impacts on density and survival of a threatened dune-endemic plant. Biol. Conserv. 135, 119–134. doi: 10.1016/j.biocon.2006.10.005
Hayward, L. S., Bowles, A. E., Ha, J. C., and Wasser, S. K. (2011). Impacts of acute and long-term vehicle exposure on physiology and reproductive success of the northern spotted owl. Ecosphere 2:art65. doi: 10.1890/ES10-00199.1
Hirst, R. A., Pywell, R. F., Marrs, R. H., and Putwain, P. D. (2003). The resistance of a chalk grassland to disturbance. J. Appl. Ecol. 40, 368–379. doi: 10.1046/j.1365-2664.2003.00800.x
Hobbs, C. H., Landry, C. B., and Perry, J. E. (2008). Assessing anthropogenic and natural impacts on ghost crabs (Ocypode quadrata) at Cape Hatteras National Seashore, North Carolina. J. Coast Res. 24, 1450–1458. doi: 10.2112/07-0856.1
Hogan, J. L., and Brown, C. D. (2021). Spatial extent and severity of all-terrain vehicles use on coastal sand dune vegetation. Appl. Veg. Sci. 24, 1–13. doi: 10.1111/avsc.12549
Hosier, P. E., and Eaton, T. E. (1980). The impact of vehicles on dune and grassland vegetation on a South-Eastern North Carolina Barrier Beach. J. Appl. Ecol. 17:173. doi: 10.2307/2402972
Hosier, P. E., Kochhar, M., and Thayer, V. (1981). Off-road vehicle and pedestrian track effects on the sea-approach of hatchling loggerhead turtles. Environ. Conserv. 8, 158–161. doi: 10.1017/S0376892900027284
Houser, C., Labude, B., Haider, L., and Weymer, B. (2013). Impacts of driving on the beach: case studies from Assateague Island and Padre Island National Seashores. Ocean Coast. Manag. 71, 33–45. doi: 10.1016/j.ocecoaman.2012.09.012
Hsu, C. (2016). Development of a Framework for predicting incident probabilities of all terrain vehicle use across a semi-arid landscape. Appl. Spat. Anal. Policy 9, 55–75. doi: 10.1007/s12061-014-9129-8
Hunkapiller, T., Ford, N., and Herriman, K. (2009). The effects of all-terrain vehicles on the herpetofauna of an east Texas floodplain. Texas J. Sci. 61, 3–14.
Janis, M. W., and Clark, J. D. (2002). Responses of Florida panthers to recreational deer and hog hunting. J. Wildl. Manage. 66, 839–848. doi: 10.2307/3803148
Jones, A. S., Anderson, J. J., Dickson, B. G., Boe, S., and Rubin, E. S. (2017). Off-highway vehicle road networks and kit fox space use. J. Wildl. Manage. 81, 230–237. doi: 10.1002/jwmg.21204
Kelly, J. F. (2014). Effects of human activities (raking, scraping, off-road vehicles) and natural resource protections on the spatial distribution of beach vegetation and related shoreline features in New Jersey. J. Coast Conserv. 18, 383–398. doi: 10.1007/s11852-014-0324-1
Kinugasa, T., and Oda, S. (2014). Effects of vehicle track formation on soil seed banks in grasslands with different vegetation in the Mongolian steppe. Ecol. Eng. 67, 112–118. doi: 10.1016/j.ecoleng.2014.03.078
Kinugasa, T., Suzuyama, Y., Tsuchihashi, N., and Nachinshonhor, G. U. (2015). Colonization and expansion of grassland species after abandonment of dirt roads in the Mongolian steppe. Landsc. Ecol. Eng. 11, 19–27. doi: 10.1007/s11355-013-0230-y
Knisley, C. B., Gowan, C., and Fenster, M. S. (2018). Effects of off-highway vehicles on sandy habitat critical to survival of a rare beetle. Insect Conserv. Divers. 11, 185–193. doi: 10.1111/icad.12244
Kutiel, P., Eden, E., and Zhevelev, Y. (2000). Effect of experimental trampling and off-road motorcycle traffic on soil and vegetation of stabilized coastal dunes, Israel. Environ. Conserv. 27, 14–23. doi: 10.1017/S0376892900000035
Lee, J. H., and Gard, K. (2014). Vehicle-soil interaction: Testing, modeling, calibration and validation. J. Terramechanics 52, 9–21. doi: 10.1016/j.jterra.2013.12.001
Li, L., and Sandu, C. (2007). On the impact of cargo weight, vehicle parameters, and terrain characteristics on the prediction of traction for off-road vehicles. J. Terramechanics 44, 221–238. doi: 10.1016/j.jterra.2007.04.002
Li, S. G., Tsujimura, M., Sugimoto, A., Davaa, G., and Sugita, M. (2006). Natural recovery of steppe vegetation on vehicle tracks in central Mongolia. J. Biosci. 31, 85–93. doi: 10.1007/BF02705239
Lonsdale, W. M., and Lane, A. M. (1994). Tourist vehicles as vectors of weed seeds in Kakadu National Park, Northern Australia. Biol. Conserv. 69, 277–283. doi: 10.1016/0006-3207(94)90427-8
Luckenbach, R. A., and Bury, R. B. (1983). Effects of off-road vehicles on the biota of the Algodones Dunes, Imperial County, California. J. Appl. Ecol. 20, 265–286. doi: 10.2307/2403392
Lucrezi, S., Saayman, M., and Vam der Merwe, P. (2014). Impact of off-road vehicles (ORVs) on ghost crabs of sandy beaches with traffic restrictions: a case study of Sodwana Bay, South Africa. Environ. Manage. 53, 520–533. doi: 10.1007/s00267-013-0223-5
Lucrezi, S., and Schlacher, T. A. (2010). Impacts of off-road vehicles (ORVs) on burrow architecture of ghost crabs (Genus Ocypode) on sandy beaches. Environ. Manage. 45, 1352–1362. doi: 10.1007/s00267-010-9491-5
Mahoney, S. P., Lewis, K. P., Mawhinney, K., Mccarthy, C., Taylor, S., Anions, D., et al. (2016). Effects of snowmachine disturbance on the energetics and habitat selection of caribou (Rangifer tarandus) in Gros Morne National Park, Newfoundland. Can. Wildl. Biol. Manag. 5, 46–59.
Marion, D. A., Phillips, J. D., Yocum, C., and Jahnz, J. (2019). Sediment availability and erosion rates on off-highway vehicle trails in the Ouachita Mountains, USA. J. Am. Water Resour. Assoc. 55, 1425–1442. doi: 10.1111/1752-1688.12793
Marion, D. A., Phillips, J. D., Yocum, C., and Mehlhope, S. H. (2014). Stream channel responses and soil loss at off-highway vehicle stream crossings in the Ouachita National Forest. Geomorphology 216, 40–52. doi: 10.1016/j.geomorph.2014.03.034
Marion, J. L., and Wimpey, J. (2017). Assessing the influence of sustainable trail design and maintenance on soil loss. J. Environ. Manage. 189, 46–57. doi: 10.1016/j.jenvman.2016.11.074
McGowan, C. P., and Simons, T. R. (2006). Effects of human recreation on the incubation behavior of American Oystercatchers. Wilson J. Ornithol. 118, 485–493. doi: 10.1676/05-084.1
McGrann, A., McGrann, M., and Dial, R. (2005). Off-highway vehicle impact on Astragalus magdalenae var. peirsonii, and other critical plant species of the Algodones Dunes, California. Calif. Fish Game 91, 61–82.
Milchunas, D. G., Schulz, K. A., and Shaw, R. B. (2000). Plant community structure in relation to long-term disturbance by mechanized military maneuvers in a semiarid region. Environ. Manage. 25, 525–539. doi: 10.1007/s002679910041
Moher, D., Liberati, A., Tetzlaff, J., and Altman, D. G. Prisma Group (2009). Preferred reporting items for systematic reviews and meta-analyses: the PRISMA statement. PLoS Med. 6:e1000097. doi: 10.1371/journal.pmed.1000097
Mohtashami, S., Bergkvist, I., Löfgren, B., and Berg, S. (2012). A GIS approach to analyzing off-road transportation: a case study in Sweden. Croat J For Eng 33, 275–284.
Monz, C. A., Cole, D. N., Leung, Y. F., and Marion, J. L. (2010). Sustaining visitor use in protected areas: future opportunities in recreation ecology research based on the USA experience. Environ. Manage. 45, 551–562. doi: 10.1007/s00267-009-9406-5
Moss, D., and McPhee, D. P. (2006). The impacts of recreational four-wheel driving on the abundance of the ghost crab (Ocypode cordimanus) on a subtropical sandy beach in SE Queensland. Coast Manag. 34, 133–140. doi: 10.1080/08920750500379383
Mullet, T. C., and Morton, J. M. (2021). Snowmobile effects on height and live stem abundance of wetland shrubs in south-central Alaska. J. Outdoor Recreat. Tour. 33:100347. doi: 10.1016/j.jort.2020.100347
Nauman, T. W., Duniway, M. C., Webb, N. P., and Belnap, J. (2018). Elevated aeolian sediment transport on the Colorado Plateau, USA: The role of grazing, vehicle disturbance, and increasing aridity. Earth Surf. Process Landforms 43, 2897–2914. doi: 10.1002/esp.4457
Navas Romero, A. L., Herrera Moratta, M. A., Dalmasso, A. D., and Barros, A. (2019). Quad bike impacts on vegetation and soil physicochemical properties in an arid ecosystem. Acta Oecologica 97, 14–22. doi: 10.1016/j.actao.2019.04.007
Naylor, L. M., Wisdom, M. J., and Anthony, R. G. (2009). Behavioral responses of North American Elk to recreational activity. J. Wildl. Manage. 73, 328–338. doi: 10.2193/2008-102
O'Dea, R. E., Lagisz, M., Jennions, M. D., Koricheva, J., Noble, D. W., Parker, T. H., et al. (2021). Preferred reporting items for systematic reviews and meta-analyses in ecology and evolutionary biology: a PRISMA extension. Biol. Rev. 96, 1695–1722. doi: 10.1111/brv.12721
Padgett, P. E., Meadows, D., Eubanks, E., and Ryan, W. E. (2008). Monitoring fugitive dust emissions from off-highway vehicles traveling on unpaved roads and trails using passive samplers. Environ. Monit. Assess. 144, 93–103. doi: 10.1007/s10661-007-9948-z
Payne, G. F., Foster, J. W., and Leininger, W. C. (1983). Vehicle impacts on northern great plains range vegetation. J. Range Manag. 36, 327. doi: 10.2307/3898480
Pérez, F. L. (1991). Particle sorting due to off-road vehicle traffic in a high andean paramo. Catena 18, 239–254. doi: 10.1016/0341-8162(91)90024-R
Preisler, H. K., Ager, A. A., and Wisdom, M. J. (2006). Statistical methods for analysing responses of wildlife to human disturbance. J. Appl. Ecol. 43, 164–172. doi: 10.1111/j.1365-2664.2005.01123.x
Racine, C. H., and Ahlstrand, G. M. (1991). Thaw response of tussock-shrub tundra to experimental all-terrain vehicle disturbances in south-central Alaska. Arctic 44, 31–37. doi: 10.14430/arctic1515
Ramos-Scharrón, C. E. (2021). Impacts of off-road vehicle tracks on runoff, erosion and sediment delivery – a combined field and modeling approach. Environ. Model. Softw. 136:104957. doi: 10.1016/j.envsoft.2020.104957
Rew, L. J., Brummer, T. J., Pollnac, F. W., Larson, C. D., Taylor, K. T., Taper, M. L., et al. (2018). Hitching a ride: seed accrual rates on different types of vehicles. J. Environ. Manage. 206, 547–555. doi: 10.1016/j.jenvman.2017.10.060
Rickard, C., McLachlan, A., and Kerley, G. I. H. (1994). The effects of vehicular and pedestrian traffic on dune vegetation in South Africa. Ocean Coast. Manag. 23, 225–247. doi: 10.1016/0964-5691(94)90021-3
Rickard, W. E., and Brown, J. (1974). Effects of vehicles on Arctic Tundra. Environ. Conserv. 1, 55–62. doi: 10.1017/S0376892900003921
Sack, D., and da Luz, S. (2003). Sediment flux and compaction trends on off-road vehicle (ORV) and other trails in an appalachian forest setting. Phys. Geogr. 24, 536–554. doi: 10.2747/0272-3646.24.6.536
Schlacher, T. A., and Lucrezi, S. (2010). Compression of home ranges in ghost crabs on sandy beaches impacted by vehicle traffic. Mar. Biol. 157, 2467–2474. doi: 10.1007/s00227-010-1511-8
Schlacher, T. A., Nielsen, T., and Weston, M. A. (2013). Human recreation alters behaviour profiles of non-breeding birds on open-coast sandy shores. Estuar. Coast. Shelf Sci. 118, 31–42. doi: 10.1016/j.ecss.2012.12.016
Schlacher, T. A., Richardson, D., and McLean, I. (2008a). Impacts of off-road vehicles (ORVs) on macrobenthic assemblages on sandy beaches. Environ. Manage. 41, 878–892. doi: 10.1007/s00267-008-9071-0
Schlacher, T. A., Thompson, L., and Price, S. (2007). Vehicles versus conservation of invertebrates on sandy beaches: mortalities inflicted by off-road vehicles on ghost crabs. Mar. Ecol. 28, 354–367. doi: 10.1111/j.1439-0485.2007.00156.x
Schlacher, T. A., and Thompson, L. M. C. (2007). Exposure of fauna to off-road vehicle (ORV) traffic on sandy beaches. Coast Manag. 35, 567–583. doi: 10.1080/08920750701593402
Schlacher, T. A., and Thompson, L. M. C. (2008). Physical impacts caused by off-road vehicles to sandy beaches: spatial quantification of car tracks on an Australian barrier island. J. Coast Res. 24, 234–242. doi: 10.2112/06-0691.1
Schlacher, T. A., Thompson, L. M. C., and Walker, S. J. (2008b). Mortalities caused by off-road vehicles (ORVs) to a key member of sandy beach assemblages, the surf clam Donax deltoides. Hydrobiologia 610, 345–350. doi: 10.1007/s10750-008-9426-9
Shanley, C. S., and Pyare, S. (2011). Evaluating the road-effect zone on wildlife distribution in a rural landscape. Ecosphere 2, 1–16. doi: 10.1890/ES10-00093.1
Singh, B. P., Cowie, A. L., and Chan, K. Y. (2011). Soil Health and Climate Change. Berlin, Heidelberg: Springer-Verlag.
Slaughter, C. W., Racine, C. H., Walker, D. A., Johnson, L. A., and Abele, G. (1990). Use of off-road vehicles and mitigation of effects in Alaska permafrost environments: a review. Environ. Manage. 14, 63–72. doi: 10.1007/BF02394020
Sparrow, S., Wooding, F., and Whiting, E. (1978). Effects of off-road vehicle traffic on soils and vegetation in the Denali Highway region of Alaska. J. Soil Water Conserv. 33, 20–27.
Spaul, R. J., and Heath, J. A. (2016). Nonmotorized recreation and motorized recreation in shrub-steppe habitats affects behavior and reproduction of golden eagles (Aquila chrysaetos). Ecol. Evol. 6, 8037–8049. doi: 10.1002/ece3.2540
Spaul, R. J., and Heath, J. A. (2017). Flushing Responses of Golden Eagles (Aquila chrysaetos) in Response to Recreation. Wilson J. Ornithol. 129, 834–845. doi: 10.1676/16-165.1
Steenhof, K., Brown, J. L., and Kochert, M. N. (2014). Temporal and spatial changes in golden eagle reproduction in relation to increased off highway vehicle activity. Wildl. Soc. Bull. 38, 682–688. doi: 10.1002/wsb.451
St-Louis, A., Hamel, S., Mainguy, J., and Côt,é, S. D. (2013). Factors influencing the reaction of mountain goats towards all-terrain vehicles. J. Wildl. Manage. 77, 599–605. doi: 10.1002/jwmg.488
Stolen, E. D. (2003). The effects of vehicle passage on foraging behavior of wading birds. Waterbirds 26, 429–436. doi: 10.1675/1524-4695(2003)026[0429:TEOVPO]2.0.CO;2
Sumanapala, D., and Wolf, I. D. (2019). Recreational ecology: a review of research and gap analysis. Environ. MDPI 6, 20–23. doi: 10.3390/environments6070081
Tarr, N. M., Simons, T. R., and Pollock, K. H. (2010). An Experimental assessment of vehicle disturbance effects on migratory shorebirds. J. Wildl. Manage. 74, 1776–1783. doi: 10.2193/2009-105
Taylor, B. R., and Raney, S. (2013). Correlation between ATV tracks and density of a rare plant (drosera filiformis) in a Nova Scotia bog. Rhodora 115, 158–169. doi: 10.3119/12-10
Thompson, L. M. C., and Schlacher, T. A. (2008). Physical damage to coastal dunes and ecological impacts caused by vehicle tracks associated with beach camping on sandy shores: a case study from Fraser Island, Australia. J. Coast Conserv. 12, 67–82. doi: 10.1007/s11852-008-0032-9
Tømmervik, H., Johansen, B., Høgda, K. A., and Strann, K. B. (2012). High-resolution satellite imagery for detection of tracks and vegetation damage caused by all-terrain vehicles (ATVs) in Northern Norway. Land Degrad. Dev. 23, 43–52. doi: 10.1002/ldr.1047
Trip, N. V. V., and Wiersma, Y. F. (2015). A comparison of all-terrain vehicle (ATV) trail impacts on boreal habitats across scales. Nat. Areas J. 35, 266–278. doi: 10.3375/043.035.0207
Trombulak, S. C., and Frissell, C. A. (2000). Review of ecological effects of roads on terrestrial and aquatic communities. Conserv. Biol. 14, 18–30. doi: 10.1046/j.1523-1739.2000.99084.x
Tull, J. C., and Brussard, P. F. (2007). Fluctuating asymmetry as an indicator of environmental stress from off-highway vehicles. J. Wildl. Manage. 71, 1944–1948. doi: 10.2193/2006-397
Van Dam, A. R., and Van Dam, M. H. (2008). Impact of off-road vehicle use on dune endemic coleoptera. Ann. Entomol. Soc. Am. 101, 411–417. doi: 10.1603/0013-8746(2008)101[411:IOOVUO]2.0.CO;2
Vollmer, A. T., Maza, B. G., Medica, P. A., Turner, F. B., and Bamberg, S. A. (1977). The impact of off-road vehicles on a desert ecosystem. Environ. Manage. 1, 115–129. doi: 10.1007/BF01866102
Walker, S. J., and Schlacher, T. A. (2011). Impact of a pulse human disturbance experiment on macrofaunal assemblages on an Australian sandy beach. J. Coast Res. 27, 184–192. doi: 10.2112/JCOASTRES-D-09-00138.1
Waterkeyn, A., Vanschoenwinkel, B., Elsen, S., Anton-Pardo, M., Grillas, P., and Brendonck, L. (2010). Unintentional dispersal of aquatic invertebrates via footwear and motor vehicles in a Mediterranean wetland area. Aquat. Conserv. Mar. Freshw. Ecosyst. 20, 580–587. doi: 10.1002/aqc.1122
Watson, J. J., Kerley, G. I. H., and McLachlan, A. (1996). Human activity and potential impacts on dune breeding birds in the Alexandria Coastal Dunefield. Landsc. Urban Plan. 34, 315–322. doi: 10.1016/0169-2046(95)00239-1
Webb, R. H., Esque, T. C., Nussear, K. E., and Sturm, M. (2013). Disruption rates for one vulnerable soil in Organ Pipe Cactus National Monument, Arizona, USA. J. Arid Environ. 95, 75–83. doi: 10.1016/j.jaridenv.2013.03.016
Webb, R. H., Ragland, H. C., Godwin, W. H., and Jenkins, O. (1978). Environmental effects of soil property changes with off-road vehicle use. Environ. Manage. 2, 219–233. doi: 10.1007/BF01866550
Westcott, F., and Andrew, M. E. (2015). Spatial and environmental patterns of off-road vehicle recreation in a semi-arid woodland. Appl. Geogr. 62, 97–106. doi: 10.1016/j.apgeog.2015.04.011
Wilshire, H. G., Nakata, J. K., Shipley, S., and Prestegaard, K. (1978). Impacts of vehicles on natural terrain at seven sites in the San Francisco Bay area. Environ. Geol. 2, 295–319. doi: 10.1007/BF02430676
Wisdom, M. J., Preisler, H. K., Naylor, L. M., Anthony, R. G., Johnson, B. K., and Rowland, M. M. (2018). Elk responses to trail-based recreation on public forests. For. Ecol. Manage. 411, 223–233. doi: 10.1016/j.foreco.2018.01.032
Wolcott, T. G., and Wolcott, D. L. (1984). Impact of off-road vehicles on macroinvertebrates of a mid-atlantic beach. Biol. Conserv. 29, 217–240. doi: 10.1016/0006-3207(84)90100-9
Xiao, X., Biswas, J., and Stone, P. (2021). Learning inverse kinodynamics for accurate high-speed off-road navigation on unstructured terrain. IEEE Robot Autom. Lett. 6, 6054–6060. doi: 10.1109/LRA.2021.3090023
Keywords: amphibians, birds, invertebrates, mammals, motorized recreation, off-highway vehicle (OHV), reptiles, plant diversity
Citation: Ploughe LW and Fraser LH (2022) Find New RoadsTM? A Systematic Review on the Impacts of Off-Road Vehicle Activity on Soil, Vegetation, and Wildlife. Front. Ecol. Evol. 9:805707. doi: 10.3389/fevo.2021.805707
Received: 30 October 2021; Accepted: 15 December 2021;
Published: 11 January 2022.
Edited by:
Sarah E. Perkins, Cardiff University, United KingdomReviewed by:
Tom Langen, Clarkson University, United StatesCopyright © 2022 Ploughe and Fraser. This is an open-access article distributed under the terms of the Creative Commons Attribution License (CC BY). The use, distribution or reproduction in other forums is permitted, provided the original author(s) and the copyright owner(s) are credited and that the original publication in this journal is cited, in accordance with accepted academic practice. No use, distribution or reproduction is permitted which does not comply with these terms.
*Correspondence: Laura W. Ploughe, cGxvdWdoZWxAZ21haWwuY29t
Disclaimer: All claims expressed in this article are solely those of the authors and do not necessarily represent those of their affiliated organizations, or those of the publisher, the editors and the reviewers. Any product that may be evaluated in this article or claim that may be made by its manufacturer is not guaranteed or endorsed by the publisher.
Research integrity at Frontiers
Learn more about the work of our research integrity team to safeguard the quality of each article we publish.