- School of BioSciences, University of Melbourne, Melbourne, VIC, Australia
Bioinspiration and biomimetics is a rapidly growing field where insights from biology are used to solve current design challenges. Nature provides an abundance of inspiration to draw upon, yet biological information is under-exploited due to a concerning lack of engagement from biologists. To assess the extent of this problem, we surveyed the current state of the field using the Web of Science database and found that only 41% of publications on bioinspired or biomimetic research included an author affiliated with a biology-related department or organisation. In addition, most publications focus exclusively on a limited range of popular model species. Considering these findings, we highlight key reasons why greater engagement from biologists will enable new and significant insights from natural selection and the diversity of life. Likewise, biologists are missing unique opportunities to study biological phenomena from the perspective of other disciplines, particularly engineering. We discuss the importance of striving toward a bioinformed approach, as current limitations in the field can only be overcome with a greater understanding of the ecological and evolutionary contexts behind each bioinspired/biomimetic solution.
Introduction
The natural world has inspired creative minds throughout time, from Da Vinci’s flying machines to Gaudi’s Sagrada Familia, but only in the mid-20th century did this design philosophy become popular within the academy. Indeed, the term biomimetics was first coined in 1957 by Otto Herbert Schmitt and refers to the transfer of knowledge or principles from biological systems to engineering or design (ISO/TC266, 2015). Biomimetics has since further diversified into related terms such as “bioinspiration”, a creative approach where design concepts are inspired from biology (ISO/TC266, 2015). These terms are sometimes used synonymously and other times as similar but separate approaches, and their broadly ambiguous use has resulted in many cases where the bioinspiration or knowledge transfer is trivial. For example, the behaviour of an animal (e.g., the flapping of wings or proboscis extension in butterflies) can be replicated using soft robotics without reference to the underlying biological mechanisms (Lin and Liu, 2019; Yu et al., 2021).
A superficial approach is not necessarily unwelcome, as some applications may depend on the broader biological concepts only (Cutkosky, 2015; Whitesides, 2015). However, we contend that there are substantial opportunity costs in having only a shallow understanding of the biological system, and that effective inspiration or mimicry requires being truly “bioinformed”. In other words, a bioinformed approach reflects an understanding of the mechanisms and processes underlying the biological system that is inspiring the innovative design. For example, early attempts at human flight were certainly inspired by nature but poorly informed by the actual biomechanics of bird flight – with sometimes fatal consequences. In contrast, a classic success story of a bioinformed design is the bullet-shaped nose of the Shinkansen train, which resembles the beak of the kingfisher. Although the inspiration may not be obvious at first glance, it is far from superficial – the shape of the kingfisher’s beak allows it to forage for aquatic prey by diving into water at great speed, moving rapidly from mediums of low (air) to high (water) density without pushing the beak into the back of its head, and with barely a splash. Mimicking this structure allows a Shinkansen train to similarly “pierce” the compression waves that build up in front of the train as it enters a tunnel. The choice of bird species as a source of inspiration or mimicry is critical – the beak of crows would not work, and even the beak of certain kingfishers, such as kookaburras, may not work as these birds are rarely faced with the same problem as the Shinkansen train. On the other hand, further improvements to the Shinkansen design might come from investigations of sea birds, such as terns, which similarly forage by diving at speed into the sea. Understanding the selection pressures favouring the biological structure or processes is key, and biologists are well placed to provide these insights to facilitate bioinformed innovation.
In this perspective we make a case for greater engagement from biologists in projects that draw on biological systems as inspiration for resolving technological and design problems. We see a parallel between the design paradox – success through failure (Petroski, 2006), and the process of natural selection, in which optimum designs are achieved as natural selection ruthlessly weeds out design failures, thereby resulting in incremental improvements. Indeed, natural selection has, over a 3.8-billion-year time span, refined biological forms, functions and processes according to extraordinarily different biological and physical environments. We argue that by combining an understanding of the biological system with design and engineering ingenuity, we can use nature to solve many problems – from the nanoscale to the global. We first consider the current state of the field by asking three questions: (i) what is the growth in bioinspired research; (ii) what is the level of engagement from biologists to this research; (iii) to what extent is research in this area utilising biological diversity? Finally, we highlight three major areas in which biologists can participate and facilitate the advancement of bioinspired or biomimetic research.
What is the Growth and Where are the Biologists?
There has been a remarkable growth in interest in bioinspiration and biomimetics, but to what extent are biologists engaged with this research? We address this question by using publications as a measure of research engagement. We searched the Web of Science (WoS) database (Clarivate, 2021) for peer-reviewed articles and reviews from 1990 to 2020 that mention bioinspiration (bioinsp*) or biomimicry (biomim*) as key terms. We then searched the author addresses of these articles and calculated the proportion of research involving an author affiliated with a bio-related department (author address with the search string “bio” e.g., Biology, Biological Sciences, BioSciences, or Biochemistry; or string of “Ecol or Environ or Evol or Zool or Botan” e.g., Ecology, Evolution, Environmental Sciences, Zoology, and Botany).
Our findings confirmed that research in bioinspiration and biomimetics has grown exponentially over the past 30 years (Supplementary Figure 1), with an accumulation of 35265 papers since 1990. Indeed, we also found a similar growth rate in articles and reviews published in materials science, to which bioinspiration has contributed. However, very few biologists appear to have participated in the bioinspired research output – our survey revealed that less than half of papers included an author from a biology related department or organisation. Specifically, only 41% of papers included an author affiliated with a biology related department. These findings are relatively consistent with Snell-Rood (2016) who found that less than 8% of biologists were involved in biomimetics research, albeit using a stricter search criterion on a subset of 300 papers. Altogether, these findings are surprising given the assumed inter- and multi-disciplinary nature of bioinspired research and suggests that either biologists are not engaging in this research and/or that biological inspiration comes from relatively few model species.
What is the Taxonomic Diversity in Bioinspiration/Biomimetics Research?
The number of formally described species is currently 2.12 million (IUCN, 2021), which is widely acknowledged to be a gross underestimate of actual global biodiversity. The number of extant eukaryotic species is estimated at 5 ± 3 million (Costello et al., 2013), as well as an estimated 1 trillion microbial species (Locey and Lennon, 2016). This represents an extraordinary diversity of solutions to environmental challenges; but is research in bioinspiration taking advantage of this diversity? We addressed this question from the perspective of both research species and exemplar design challenges.
Research Species
We arbitrarily chose two groups of animals that commonly contribute to bioinspired research in materials: butterflies, whose wing characteristics have informed the development of technologies such as electronic displays and solar cells; and spiders, whose silk characteristics have wide applications as a sturdy yet light-weight biomaterial. We searched the WoS database for peer-reviewed research articles using bioinspiration, biomimetics, and butterflies or spiders as key search terms and screened each article for relevant taxonomic information. Specifically, we identified the species that each study was primarily based upon, as well as studies involving multiple focal species. Using a systematic search protocol involving the filtering of irrelevant papers (e.g., articles with no mention of species) and the retrieval of relevant papers from reference lists (e.g., articles using specific terms such as the names of common genera e.g., Morpho for butterflies, Trichonephila or Nephila for spiders), we arrived at 173 research articles for butterflies and 218 for spiders (Supplementary Information).
A similar pattern can be observed in both butterfly and spider inspired research papers: the representation of focal species in this literature is unevenly distributed and focussed on a fraction of the described biodiversity (Figure 1). For example, while the 173 articles that drew inspiration from butterflies included species from 35 genera (Supplementary Table 1), 64% of these species belonged to the family Nymphalidae, and 44% focussed on a single genus, namely Morpho. Clearly, we have explored only the tip of the existing butterfly biodiversity, given the 18,000 formally described species across 125 families (Heppner, 2008; Espeland et al., 2018). A similar pattern emerges for spiders, where 33% of 218 articles focussed on the genus Trichonephila (golden orb-weaving spiders previously a subgenus of Nephila) from a total of 29 represented genera (Supplementary Table 2). In addition, 78% of the articles that drew inspiration from spiders belonged to the superfamily Araneoidea, of which 94% belonged to the family Araneidae (orb-weaving spiders). Nevertheless, the pattern for spiders is perhaps even more alarming than that of butterflies, since spiders are arguably a broader taxonomic group (Araneae), with approximately 49,500 described species from 4033 genera and 113 families (Selden, 2017; World Spider Catalog, 2021). Further, only 12% of butterfly- and 14% of spider-inspired research drew inspiration from multiple focal species.
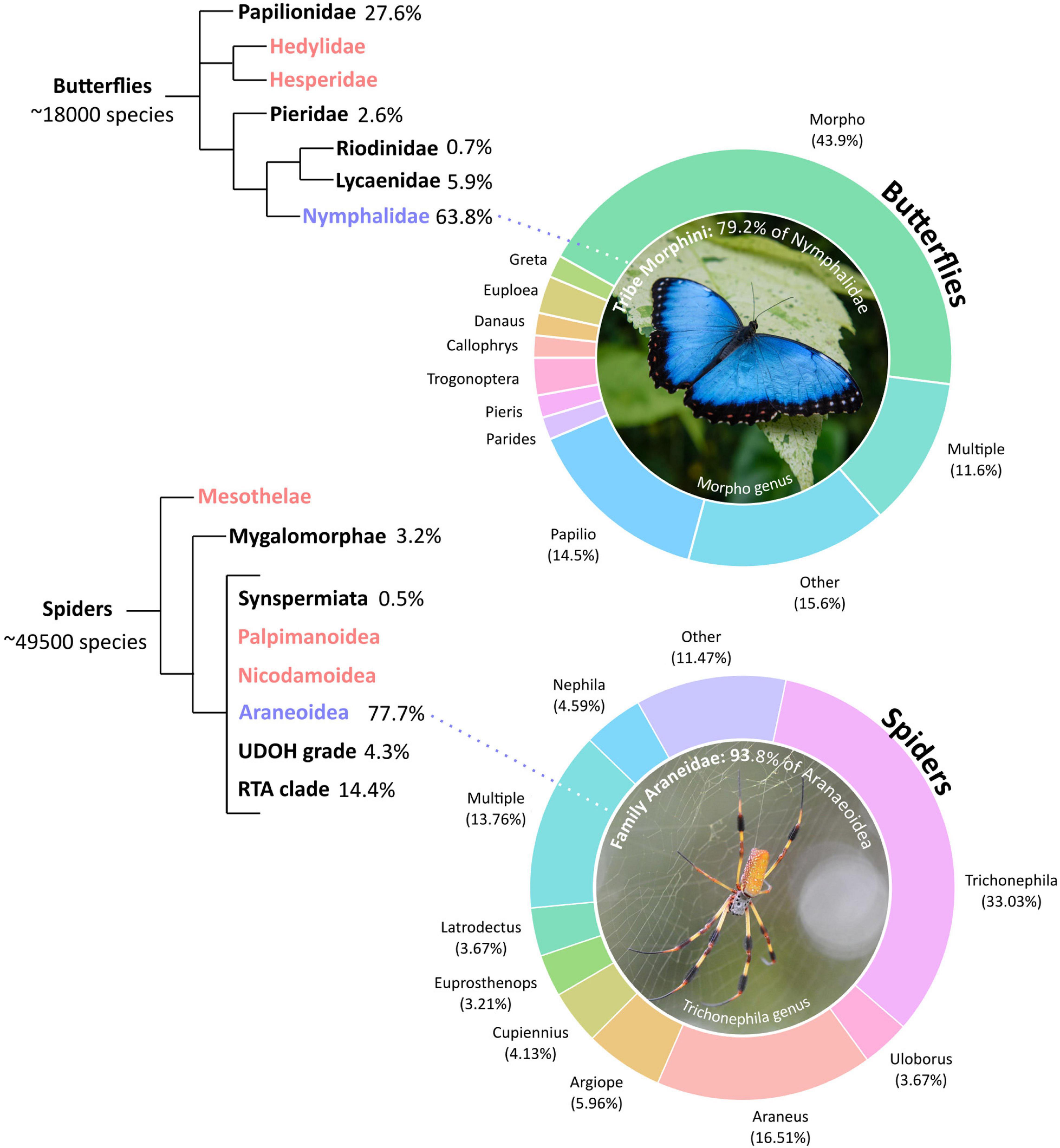
Figure 1. Taxonomic distribution of butterfly and spider bioinspiration/biomimetic research. Donut charts represent the distribution of genera for each taxonomic group, based on 173 papers on butterflies and 218 papers on spiders. Percentages are the percentage of the identified papers focusing on each taxon. “Multiple” indicates research including multiple focal species, while “Other” indicates the sum of genera with less than 3% representation. Tree diagrams show the representation of major phylogenetic groups within butterflies (Rhopalocera) and spiders (Araneae). Red indicates groups with no representation, and blue represents the group with the most representation. Most species within both animal groups belong to a select few genera, and are largely represented by two phylogenetic groups only. Original photos by Michal Mrozek (butterfly) and Tom Earnhardt (spider).
Design Challenges
We arbitrarily chose two design challenges, which have attracted a great deal of bioinspired attention – drag reduction and surface adhesion. Engineers have often looked to nature for ways to improve the hydrodynamic performance of ships and aircrafts; similarly, animals have evolved countless solutions for interfacing with complex natural surfaces, inspiring the development of adhesive tapes and glues. Here, we used the same systematic protocol for research species but instead searched for peer-reviewed research articles with either drag reduction or surface adhesion as key terms. We arrived at 156 papers for drag reduction and 272 papers for surface adhesion (Supplementary Figure 2).
Again, we see a remarkably similar pattern – solutions to both design challenges are primarily drawn from a few model species (Figure 2 and Supplementary Tables 3, 4). In particular, the Tokay gecko (Gecko gecko) is dominant in the surface adhesion literature despite being an unusually large gecko species. We also found that 3% of surface adhesion papers and 10% of drag reduction papers involved species from multiple taxonomic classes, showing that researchers do not usually consider more than one species. This problem of representation was further exacerbated by the lax reporting of taxonomy, especially in the context of popular models. For example, studies involving gecko-based adhesion often simply use the generalised term “gecko” without specifying further details.
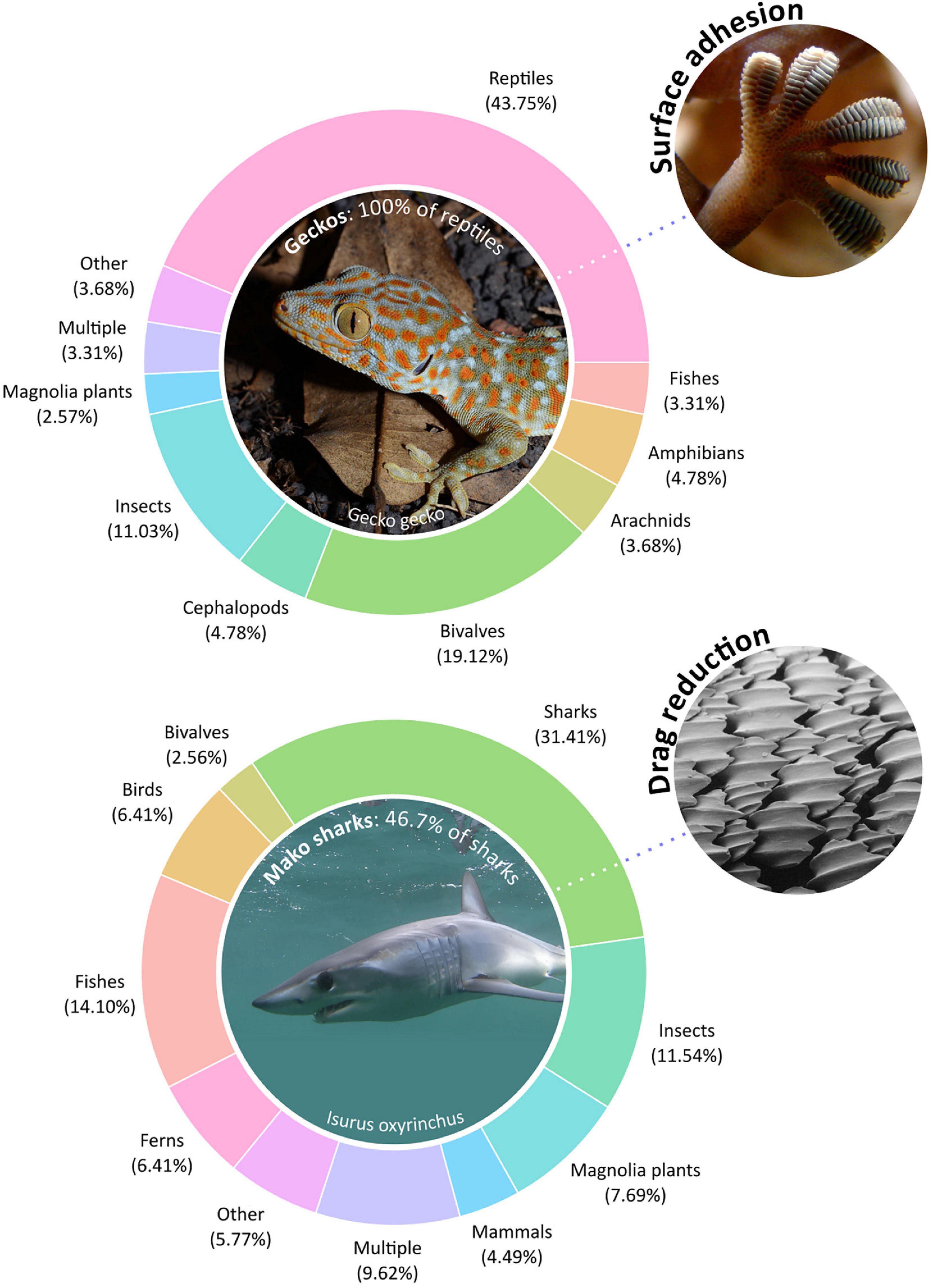
Figure 2. Taxonomic distribution of surface adhesion and drag reduction research. We identified 156 papers for drag reduction and 272 papers for surface adhesion. Percentages are the percentage of the identified papers focusing on each taxon. “Multiple” indicates research including multiple focal species, while “Other” indicates the sum of genera with less than 4% representation. Most of the research is concentrated in a select few model species despite the biological diversity from which insights can be potentially drawn. Only few studies involve multiple species and instead focus on a single model. Original photos by Patrick Randall (gecko), Bjørn Tørrissen (gecko foot), Elaine Brewer (shark), and Pascal Deynat (shark skin).
Embracing Biodiversity
The taxonomically narrow approach to research species selection is problematic because significant insights can be overlooked by focusing on only a single genus or family. For example, it was recently discovered that the nanostructures of butterflies facilitates heat dissipation, allowing their thin wings to remain cool despite their large size (Tsai et al., 2020). This insight was made possible by using a multi-species comparative approach to understand processes common to all butterflies, rather than focussing on a single species. Tsai et al. (2020) found key commonalities among the 50 species studied: all had structures that enhanced radiative cooling of the thermally sensitive living parts of the wings (scent patches, scent pads, and wing veins). Differences between species were just as informative: different species used varying combinations of adaptations including cuticle thickness, specialised scale nanostructures, contrasting dorsal and ventral wing coloration, and finely-tuned behavioural reactions to enable temperature to be exquisitely tailored to environmental conditions. Due to the specific combination of traits, butterflies in tropical and temperate environments can bask longer to warm up their bodies while reducing the risk of overheating the wings: whereas butterflies at high latitudes or altitudes can warm up their wings efficiently. This example highlights that comparison of multiple species can reveal how different traits can vary and be combined to suit a wide range of environmental conditions.
Studying multiple species can also highlight the variation in the uses or properties of an adaptation. For example, all spiders produce at least one of seven types of silk that are used in conjunction with different activities, including attracting conspecific mates, forming a cocoon that protects vulnerable eggs, dispersing very considerable distances through ballooning, or capturing prey (Blamires et al., 2017). Yet our knowledge of the properties of spider silk is mostly confined to silks used for foraging, and in particular the dragline silk produced by orb-weaving spiders, the latter informed by a handful of species. Of course, there are excellent reasons for focussing on this type of silk, since it combines two seemingly incompatible properties – strength and elasticity, necessary to absorb the energy of insects arrested at speed. Nevertheless, orb-weaving spiders (Araneidae) are a large and diverse taxonomic group comprising over 3000 species in 177 genera. This taxonomic breadth incorporates remarkable diversity in their foraging ecology, which likely influences the properties of the dragline silk used to support the foraging webs: spiders in the genus Plebs and Leucage build small, delicate and easily broken webs, while the dragline silk that supports the orb-webs of Darwin’s bark spider Caerostris darwini extends tens of meters across rivers (Agnarsson et al., 2010) and is twice as strong as that produced by other orb-weaving spiders (Garb et al., 2019). While differences in dragline silk mechanical properties are also reported among similarly-sized, closely related species (Kerr et al., 2018), the properties of other types of spider silk have been largely ignored. For example, the potential diversity of the tubuliform and aciniform silk used to construct egg cases is poorly understood, but the remarkably rigid, free-standing foraging web of the thomisid spider Saccodomus formivorus also appears to comprise these silks, which could inspire rigid thread designs for tissue engineering (Haynl et al., 2020).
This approach of embracing biodiversity can also be expanded to organisms that live in unusual or hostile habitats. These environments are, by definition, difficult to access and study, and so organisms within them are likely to reveal novel solutions to specific environmental challenges. Notably, this perspective has led to the emergence of “extreme biomimetics”, defined by its focus on bioinspired solutions informed from biologically extreme conditions that are well outside the human comfort zone (Ehrlich, 2017; Petrenko et al., 2019). For example, the skeletal structure of fauna endemic to hydrothermal vents have inspired the study of biopolymers that are thermally stable and high-pressure tolerant under such conditions (Unterlass, 2017). Recent advances in this field have focused on the development of inorganic-organic hybrid materials using chitin or spongin scaffolds exposed to hydrothermal treatments (Ehrlich et al., 2013; Petrenko et al., 2019). This biomaterial fabrication strategy was only made possible by investigating chitin, collagen, and spongin skeletal structures across taxa, eventually settling on sponges (Porifera) as an ideal and renewable source of thermal and pressure tolerant biopolymers (Ehrlich, 2017). Hydrothermal vents are only one example of an extreme environment, and there is obvious value in drawing inspiration from organisms that live in habitats that reflect the extremities of other dimensions, such as oxygen concentration, humidity or cold temperatures. For example, the rapidly growing developments in bioinspired anti-freeze proteins from polar fish and freeze-tolerant insects have promising future biomedical applications (Xiang et al., 2020).
Clearly, there is untapped potential in shifting attention outside of obvious model species, especially as they may not always represent the optimal solution in every context. For example, shark skin is famous for its drag reducing properties, but is by no means the most effective material; other solutions include dolphin skin, penguin-inspired micro-bubbles, and lotus leaf surface structures (Yu et al., 2020). Notably, these species belong to very different phylogenetic groups and their adaptations reflect solutions from different evolutionary perspectives. Similarly, additional insights into surface adhesion may come from insect solutions (Zhou et al., 2014). In short, an optimal bioinspired/biomimetic design can be achieved by understanding, comparing, and even combining insights from nature’s diversity of solutions.
Key Reasons for Engagement From Biologists
Bioinspired design and engineering have had some impressive success stories, but our analysis suggests that we are barely scratching the surface of biological insights. Below, we highlight three key reasons that greater engagement from biologists is crucial for effective bioinspired or biomimetic design:
Understanding Evolution
The near infinite functional traits found in nature have been forged by natural selection; this evolutionary design process has quietly progressed for many millions of years. Therefore, by studying living organisms that have survived nature’s optimisation algorithm (Maynard Smith, 1978), we can reap the rewards while skipping our own iterative and time-consuming design processes. This is one of the main appeals of bioinspiration/biomimetics, and biologists are well placed to fully appreciate these systems. However, effective bioinspiration/biomimetics is more complicated than simply selecting a species and replicating the desired adaptation; it can be difficult to identify the most functionally relevant traits to abstract into a design. One solution is to study the evolution of the trait in question using comparative methods, observing whether it appears in related species exhibiting the desired function, and whether it was lost in those without (Adriaens, 2019). Such a comparative approach enables insights into how traits are associated with specific environmental conditions. Again, such an approach requires an appreciation for evolutionary history to take advantage of insights from natural selection (Wolff et al., 2017).
In addition, non-biologists are often unaware of the limitations of natural selection as a design inspiration. While evolution has analogies to the design process, it is not exactly the same and suffers from different constraints. Engineers are often interested in designs that can optimally achieve a single function, however, biological traits are the product of balancing multiple functions and do not represent the objectively optimal solution for one specific function. For example, butterfly wings have inspired the production of structurally coloured materials (Schroeder et al., 2018), yet light manipulation is not their only function. The wings must also be hydrophobic and self-cleaning (Fang et al., 2015), thermally efficient for the animal’s environment (Tsai et al., 2020), and flexible yet durable for flight (Johansson and Henningsson, 2021). Natural selection has favoured a solution that suits all these biological requirements, resulting in a wing structure that is a functional compromise for the animal’s specific environmental conditions. Different species face different environmental challenges and will have varying adaptations to reflect these conditions. This is very relevant to bioinspired/biomimetic design challenges as most synthetic structures or materials also have multiple functional requirements. Identifying biological analogues to these requirements will therefore result in solutions that are better optimised for multiple conditions.
An awareness of phylogenetic constraints is also crucial. For example, while bats have evolved the capacity to fly, it is suggested that their aerodynamic efficiency is inferior to birds due to the phylogenetic constraints associated with their wing design (Muijres et al., 2012). Flexible membranes and elongated digits evolved in bats not because it was necessarily the optimal solution to flight, but because of its evolutionary context (Amador et al., 2019). Engineers also have access to structures and processes that are not readily available to natural selection. For example, the wheel is an energetically efficient invention to facilitate movement, but continuous rotation is rarely possible for animals constrained by musculoskeletal systems (Fish and Beneski, 2014). Biologists are well equipped to navigate these limitations and have the knowledge to properly abstract biological principles whilst avoiding their constraints.
Drawing From Diversity
Natural selection provides a diversity of solutions to life’s design challenges; different species can represent distinct or sometimes convergent solutions to similar problems. A focus on single study species provides only one answer to a design challenge, yet the diversity of life shows that there are multiple solutions which can be more or less optimal depending on environmental conditions. Therefore, a focus on the feature rather than species can be more rewarding and provides insights only possible when drawing from the full diversity of life. For example, adaptations for surface adhesion are widespread in the animal kingdom. An awareness of attachment strategies in animals of different sizes can provide insight into issues of scaling with size (Labonte and Federle, 2015), as well as possible solutions to the problem. Interactions between multiple solutions can also be observed when focusing on the feature rather than species. For instance, some animals have both adhesive pads and claws which can potentially be synergistic, or in other cases, redundant (Song et al., 2016; Naylor and Higham, 2019). Understanding the evolutionary contexts behind such interactions can inform the performance and necessity of multiple features in bioinspired/biomimetic designs. Another recent example is the insights drawn from the diversity of insect cuticles. Most insect exoskeletons feature gradual changes in stiffness across the cuticle and analysing this property has directly informed the development of functionally graded materials (Jafarpour et al., 2020). The uncommon, common feature of these examples are that they all draw insights from the diversity of life, rather than focussing on individual species.
In attempts to better appreciate biological diversity for use in art and design, tools such as AskNature have been developed to translate biological information to non-biologists (Deldin and Schuknecht, 2014). These tools serve as rich databases of biological strategies organised by function and can be extremely useful in the initial search for relevant solutions. However, the accessibility of these tools can ironically lead to the perpetuation of popular model species. Such databases do not represent the actual diversity of potential solutions in nature and are by no means an effective replacement for biologists who have expert knowledge on underappreciated systems or strategies (Graeff et al., 2019; Willocx et al., 2020). Therefore, the involvement of biologists continues to be crucial for diverse biological insights in bioinspired and biomimetic designs.
Embracing Bioinformed Design
A crucial component of biomimetic design is the abstraction process, as it is often unnecessary to entirely replicate a biological feature (Cutkosky, 2015; Whitesides, 2015). Nevertheless, a nuanced understanding of the biological system is required to abstract a feature effectively. For example, the micro-structure of shark skin needs to be understood in detail before it can be properly simplified into a synthetic riblet (Domel et al., 2018). Incorrectly translating such structures into the final design can result in a potential loss in performance. In addition, a holistic understanding of the system will prevent researchers from becoming too focused on a specific feature in isolation. For example, the Geckskin adhesive technology was developed by abandoning the conventional focus on gecko setae and instead developing a product inspired by the entire gecko foot (Patek, 2014). Here, the research team realised that the setae alone did not explain adhesive performance, but that effective attachment was the outcome of a system of synergistic features at different scales (Imburgia et al., 2019): specifically, the stiff tendons attached to their toepads also played a significant role in effective attachment (King et al., 2014).
It is important to note that gecko-inspired tapes have yet to match the performance of living geckos. Indeed, current gaps in the understanding of biological models remains a major obstacle in biomimetic design. For example, gecko-inspired adhesives have been designed to attach to smooth dry surfaces but are largely ineffective on non-ideal surfaces (Niewiarowski et al., 2016). To further approach the performance of the biological system, it is essential to study questions regarding the ecology of the animal: how are gecko toe pads tuned to specific environmental contexts? How do geckos move across irregular or wet surfaces? How do toe pads vary across species with different environmental challenges? There is considerable morphological variation in geckos, which is linked to habitat (Kulyomina et al., 2019; Norris et al., 2021). These insights from basic biological research are crucial to address current performance gaps and can only be achieved through the involvement of biologists (Higham et al., 2019).
Finally, we emphasise that a bioinspired/biomimetic approach to design is not a one directional relationship. Biologists also stand to gain valuable insights from the process: for example, engineers can provide a different approach toward problem-solving – whilst biologists may only arrive at a conceptual understanding of a trait, in many cases engineers can physically construct and test a structure or property. This direct approach is possible as engineers often have access to resources, technical expertise, and specialist equipment required for the testing and validation of functional hypotheses. Therefore, there is enormous potential for biologists to take advantage of the expertise and resources available to engineers to further explore biological questions in a physical and tangible context (Roberts et al., 2014). For example, biologists often have difficulty studying the functional morphology of extinct species due to the limitations of fossil evidence. Biomimetic tools have allowed for the structures of species such a fossil earwig (Saito et al., 2020) or remora (Gamel et al., 2019) to be replicated to study their evolution and function. In return, these findings provide insights for biomimetic designs, such as the improvement of underwater attachment (remora disc) or folding patterns (earwig wing). A move toward “bioinformation” is both synergistic and mutualistic, allowing biologists and engineers to produce insightful science and useful designs.
Concluding Remarks
The current state of bioinspired/biomimetic design suffers from a surprising paradox: effective bioinspiration or biomimetics requires intimate knowledge of biology, yet there is a lack of engagement from biologists in such projects. Here, we show that the output of bioinspired research seems to be characterised by limited collaboration with biologists and a very extensive focus on a few representative species. This is problematic as significant insights from evolution and ecology are overlooked, and likewise, biologists are also missing unique opportunities to approach hypotheses from an engineering perspective. We therefore advocate for greater engagement between biologists and engineers – biologists benefit from a greater awareness of design challenges and methodological approaches in engineering, and engineers benefit from a greater awareness of the diversity of strategies forged by natural selection. There is no shortcut or secret method for success in interdisciplinary collaboration; both parties must actively seek opportunities to share and discuss ideas or challenges. Despite the well-known barriers to interdisciplinary engagement (Mazzocchi, 2019), we believe that such collaborations will not only promote the value of basic science but also generate rewarding opportunities for biologists to view their study system from a new perspective. There is evidently remarkable value and demand for biological expertise, and input from biologists will be key in overcoming current limitations of bioinspired/biomimetic technologies.
Data Availability Statement
The raw data supporting the conclusions of this article will be made available by the authors, without undue reservation.
Author Contributions
LN conducted the systematic assessment of the bioinspiration/biomimetic literature and analysed the findings. LN and ME drafted the manuscript. All authors contributed and edited the final manuscript and conceived of the work.
Conflict of Interest
The authors declare that the research was conducted in the absence of any commercial or financial relationships that could be construed as a potential conflict of interest.
Publisher’s Note
All claims expressed in this article are solely those of the authors and do not necessarily represent those of their affiliated organizations, or those of the publisher, the editors and the reviewers. Any product that may be evaluated in this article, or claim that may be made by its manufacturer, is not guaranteed or endorsed by the publisher.
Supplementary Material
The Supplementary Material for this article can be found online at: https://www.frontiersin.org/articles/10.3389/fevo.2021.790270/full#supplementary-material
References
Adriaens, D. (2019). “Evomimetics: the biomimetic design thinking 2.0,” in Proceedings of SPIE 10965: SPIE Smart Structures + Nondestructive Evaluation, eds R. K. Martín-Palma, M. Knez, and A. Lakhtakia (Denver, CO: SPIE).
Agnarsson, I., Kuntner, M., and Blackledge, T. A. (2010). Bioprospecting finds the toughest biological material: extraordinary silk from a giant riverine orb spider. PLoS One 5:e11234. doi: 10.1371/journal.pone.0011234
Amador, L. I., Almeida, F. C., and Giannini, N. P. (2019). Evolution of traditional aerodynamic variables in bats (Mammalia: Chiroptera) within a comprehensive phylogenetic framework. J. Mamm. Evol. 27, 549–561. doi: 10.1007/s10914-019-09475-8
Blamires, S. J., Blackledge, T. A., and Tso, I.-M. (2017). Physicochemical property variation in spider silk: ecology, evolution, and synthetic production. Annu. Rev. Entomol. 62, 443–460. doi: 10.1146/annurev-ento-031616-035615
Clarivate (2021). Web of Science [Online]. Available online at: www.webofscience.com (accessed June 23, 2021)
Costello, M. J., May, R. M., and Stork, N. E. (2013). Can we name earth’s species before they go extinct? Science 339, 413–416. doi: 10.1126/science.1230318
Cutkosky, M. R. (2015). Climbing with adhesion: from bioinspiration to biounderstanding. Interface Focus 5:20150015. doi: 10.1098/rsfs.2015.0015
Deldin, J.-M., and Schuknecht, M. (2014). “The asknature database: enabling solutions in biomimetic design,” in Biologically Inspired Design, eds A. Goel, D. McAdams, and R. Stone (London: Springer), 17–27.
Domel, A. G., Domel, G., Weaver, J. C., Saadat, M., Bertoldi, K., and Lauder, G. V. (2018). Hydrodynamic properties of biomimetic shark skin: effect of denticle size and swimming speed. Bioinspir. Biomim. 13:056014. doi: 10.1088/1748-3190/aad418
Ehrlich, H., Simon, P., Motylenko, M., Wysokowski, M., Bazhenov, V. V., Galli, R., et al. (2013). Extreme biomimetics: formation of zirconium dioxide nanophase using chitinous scaffolds under hydrothermal conditions. J. Mater. Chem. B 1, 5092–5099. doi: 10.1039/C3TB20676A
Espeland, M., Breinholt, J., Willmott, K. R., Warren, A. D., Vila, R., Toussaint, E. F. A., et al. (2018). A comprehensive and dated phylogenomic analysis of butterflies. Curr. Biol. 28, 770–778.e5. doi: 10.1016/j.cub.2018.01.061
Fang, Y., Sun, G., Bi, Y., and Zhi, H. (2015). Multiple-dimensional micro/nano structural models for hydrophobicity of butterfly wing surfaces and coupling mechanism. Sci. Bull. 60, 256–263. doi: 10.1007/s11434-014-0653-3
Fish, F. E., and Beneski, J. T. (2014). “Evolution and bio-inspired design: natural limitations,” in Biologically Inspired Design, eds A. Goel, D. McAdams, and R. Stone (London: Springer), 287–312.
Gamel, K. M., Garner, A. M., and Flammang, B. E. (2019). Bioinspired remora adhesive disc offers insight into evolution. Bioinspir. Biomim. 14:056014. doi: 10.1088/1748-3190/ab3895
Garb, J. E., Haney, R. A., Schwager, E. E., Gregoriè, M., Kuntner, M., Agnarsson, I., et al. (2019). The transcriptome of Darwin’s bark spider silk glands predicts proteins contributing to dragline silk toughness. Commun. Biol. 2:275. doi: 10.1038/s42003-019-0496-1
Graeff, E., Maranzana, N., and Aoussat, A. (2019). Biomimetics, where are the biologists? J. Eng. Des. 30, 289–310. doi: 10.1080/09544828.2019.1642462
Haynl, C., Vongsvivut, J., Mayer, K. R. H., Bargel, H., Neubauer, V. J., Tobin, M. J., et al. (2020). Free-standing spider silk webs of the thomisid Saccodomus formivorus are made of composites comprising micro- and submicron fibers. Sci. Rep. 10:17624. doi: 10.1038/s41598-020-74469-z
Heppner, J. B. (2008). “Butterflies and moths (lepidoptera),” in Encyclopedia of Entomology, ed. J. L. Capinera (Dordrecht: Springer), 626–672.
Higham, T. E., Russell, A. P., Niewiarowski, P. H., Wright, A., and Speck, T. (2019). The ecomechanics of gecko adhesion: natural surface topography, evolution, and biomimetics. Integr. Comp. Biol. 59, 148–167. doi: 10.1093/icb/icz013
Imburgia, M. J., Kuo, C.-Y., Briggs, D. R., Irschick, D. J., and Crosby, A. J. (2019). Effects of digit orientation on gecko adhesive force capacity: synthetic and behavioral studies. Integr. Comp. Biol. 59, 182–192. doi: 10.1093/icb/icz024
ISO/TC266 (2015). ISO 18458:2015-Biomimetics — Terminology, Concepts and Methodology. Berlin: Beuth Verlag.
IUCN (2021). The IUCN Red List of Threatened Species [Online]. Available online at: https://www.iucnredlist.org/ (accessed September 21, 2021)
Jafarpour, M., Eshghi, S., Darvizeh, A., Gorb, S., and Rajabi, H. (2020). Functional significance of graded properties of insect cuticle supported by an evolutionary analysis. J. R. Soc. Interface 17:20200378. doi: 10.1098/rsif.2020.0378
Johansson, L. C., and Henningsson, P. (2021). Butterflies fly using efficient propulsive clap mechanism owing to flexible wings. J. R. Soc. Interface 18:20200854. doi: 10.1098/rsif.2020.0854
Kerr, G. G., Nahrung, H. F., Wiegand, A., Kristoffersen, J., Killen, P., Brown, C., et al. (2018). Mechanical properties of silk of the Australian golden orb weavers Nephila pilipes and Nephila plumipes. Biol. Open 7:bio029249. doi: 10.1242/bio.029249
King, D. R., Bartlett, M. D., Gilman, C. A., Irschick, D. J., and Crosby, A. J. (2014). Creating gecko-like adhesives for “real world” surfaces. Adv. Mater. 26, 4345–4351. doi: 10.1002/adma.201306259
Kulyomina, Y., Moen, D. S., and Irschick, D. J. (2019). The relationship between habitat use and body shape in geckos. J. Morphol. 280, 722–730. doi: 10.1002/jmor.20979
Labonte, D., and Federle, W. (2015). Scaling and biomechanics of surface attachment in climbing animals. Philos. Trans. R. Soc. Lond. B 370:20140027. doi: 10.1098/rstb.2014.0027
Lin, P. W., and Liu, C. H. (2019). Bio-inspired soft proboscis actuator driven by dielectric elastomer fluid transducers. Polymers (Basel) 11:142. doi: 10.3390/polym11010142
Locey, K. J., and Lennon, J. T. (2016). Scaling laws predict global microbial diversity. Proc. Natl. Acad. Sci. U. S. A. 113, 5970–5975. doi: 10.1073/pnas.1521291113
Maynard Smith, J. (1978). Optimization theory in evolution. Annu. Rev. Ecol. Syst. 9, 31–56. doi: 10.1146/annurev.es.09.110178.000335
Mazzocchi, F. (2019). Scientific research across and beyond disciplines: challenges and opportunities of interdisciplinarity. EMBO Rep. 20:e47682. doi: 10.15252/embr.201947682
Muijres, F. T., Johansson, L. C., Bowlin, M. S., Winter, Y., and Hedenstrom, A. (2012). Comparing aerodynamic efficiency in birds and bats suggests better flight performance in birds. PLoS One 7:e37335. doi: 10.1371/journal.pone.0037335
Naylor, E. R., and Higham, T. E. (2019). Attachment beyond the adhesive system: the contribution of claws to gecko clinging and locomotion. Integr. Comp. Biol. 59, 168–181. doi: 10.1093/icb/icz027
Niewiarowski, P. H., Stark, A. Y., and Dhinojwala, A. (2016). Sticking to the story: outstanding challenges in gecko-inspired adhesives. J. Exp. Biol. 219(Pt 7), 912–919. doi: 10.1242/jeb.080085
Norris, J., Tingley, R., Meiri, S., and Chapple, D. G. (2021). Environmental correlates of morphological diversity in Australian geckos. Glob. Ecol. Biogeogr. 30, 1086–1100. doi: 10.1111/geb.13284
Patek, S. N. (2014). Materials science. Biomimetics and evolution. Science 345, 1448–1449. doi: 10.1126/science.1256617
Petrenko, I., Summers, A. P., Simon, P., Żółtowska-Aksamitowska, S., Motylenko, M., Schimpf, C., et al. (2019). Extreme biomimetics: preservation of molecular detail in centimeter-scale samples of biological meshes laid down by sponges. Sci. Adv. 5:eaax2805. doi: 10.1126/sciadv.aax2805
Petroski, H. (2006). Success Through Failure: The Paradox of Design. Princeton, NJ: Princeton University Press.
Roberts, S. F., Hirokawa, J., Rosenblum, H. G., Sakhtah, H., Gutierrez, A. A., Porter, M. E., et al. (2014). Testing biological hypotheses with embodied robots: adaptations, accidents, and by-products in the evolution of vertebrates. Front. Robot. AI 1:12. doi: 10.3389/frobt.2014.00012
Saito, K., Perez-de la Fuente, R., Arimoto, K., Seong, Y. A., Aonuma, H., Niiyama, R., et al. (2020). Earwig fan designing: biomimetic and evolutionary biology applications. Proc. Natl. Acad. Sci. U. S. A. 117, 17622–17626. doi: 10.1073/pnas.2005769117
Schroeder, T. B. H., Houghtaling, J., Wilts, B. D., and Mayer, M. (2018). It’s not a bug, it’s a feature: functional materials in insects. Adv. Mater. 30:1705322. doi: 10.1002/adma.201705322
Selden, P. A. (2017). “Arachnids,” in Reference Module in Life Sciences, ed. B. D. Roitberg (Amsterdam: Elsevier).
Snell-Rood, E. (2016). Interdisciplinarity: bring biologists into biomimetics. Nature 529, 277–278. doi: 10.1038/529277a
Song, Y., Dai, Z., Wang, Z., Ji, A., and Gorb, S. N. (2016). The synergy between the insect-inspired claws and adhesive pads increases the attachment ability on various rough surfaces. Sci. Rep. 6:26219. doi: 10.1038/srep26219
Tsai, C.-C., Childers, R. A., Nan Shi, N., Ren, C., Pelaez, J. N., Bernard, G. D., et al. (2020). Physical and behavioral adaptations to prevent overheating of the living wings of butterflies. Nat. Commun. 11:551. doi: 10.1038/s41467-020-14408-8
Unterlass, M. M. (2017). Geomimetics and extreme biomimetics inspired by hydrothermal systems—what can we learn from nature for materials synthesis? Biomimetics 2:8. doi: 10.3390/biomimetics2020008
Whitesides, G. M. (2015). Bioinspiration: something for everyone. Interface Focus 5:20150031. doi: 10.1098/rsfs.2015.0031
Willocx, M., Ayali, A., and Duflou, J. R. (2020). Where and how to find bio-inspiration? CIRP J. Manuf. Sci. Technol. 31, 61–67. doi: 10.1016/j.cirpj.2020.09.013
Wolff, J. O., Wells, D., Reid, C. R., and Blamires, S. J. (2017). Clarity of objectives and working principles enhances the success of biomimetic programs. Bioinspir. Biomim. 12:051001. doi: 10.1088/1748-3190/aa86ff
World Spider Catalog (2021). World Spider Catalog [Online]. Available online at: http://wsc.nmbe.ch (accessed September 16, 2021)
Xiang, H., Yang, X., Ke, L., and Hu, Y. (2020). The properties, biotechnologies, and applications of antifreeze proteins. Int. J. Biol. Macromol. 153, 661–675. doi: 10.1016/j.ijbiomac.2020.03.040
Yu, C., Liu, M., Zhang, C., Yan, H., Zhang, M., Wu, Q., et al. (2020). Bio-inspired drag reduction: from nature organisms to artificial functional surfaces. Giant 2:100017. doi: 10.1016/j.giant.2020.100017
Yu, X.-Q., Hu, X.-H., Zhu, L., Li, G., Guo, M., Li, Q., et al. (2021). Fabrication of magnetically driven photonic crystal fiber film via microfluidic blow-spinning towards dynamic biomimetic butterfly. Mater. Lett. 291:129450. doi: 10.1016/j.matlet.2021.129450
Keywords: bioinspiration, biomimetic, collaboration, ecology, evolution, adaptation, natural selection, interdisciplinary
Citation: Ng L, Elgar MA and Stuart-Fox D (2021) From Bioinspired to Bioinformed: Benefits of Greater Engagement From Biologists. Front. Ecol. Evol. 9:790270. doi: 10.3389/fevo.2021.790270
Received: 06 October 2021; Accepted: 19 November 2021;
Published: 09 December 2021.
Edited by:
Isabel Marques, University of Lisbon, PortugalReviewed by:
Hermann Ehrlich, Freiberg University of Mining and Technology, GermanySang-im Lee, Daegu Gyeongbuk Institute of Science and Technology (DGIST), South Korea
Copyright © 2021 Ng, Elgar and Stuart-Fox. This is an open-access article distributed under the terms of the Creative Commons Attribution License (CC BY). The use, distribution or reproduction in other forums is permitted, provided the original author(s) and the copyright owner(s) are credited and that the original publication in this journal is cited, in accordance with accepted academic practice. No use, distribution or reproduction is permitted which does not comply with these terms.
*Correspondence: Leslie Ng, dHN6bjFAc3R1ZGVudC51bmltZWxiLmVkdS5hdQ==
†These authors share senior authorship