- 1Independent Researcher, North Freedom, WI, United States
- 2Department of Biology, Coastal Carolina University, Conway, SC, United States
Turtle biologists have long been interested in the biotic and abiotic factors that influence the detection of freshwater turtle nests by mammalian predators. Increased knowledge of nest predation dynamics may help develop conservation strategies to increase turtle nesting success by altering or reducing the signal strength of predominant nest location cues. However, despite this long-standing interest, the related research has produced inconsistent and sometimes conflicting results across studies. Here we review much of the existing literature on freshwater turtle nest predation by mammalian predators and attempt to synthesize some general, underlying themes. Available data suggest that raccoons (Procyon lotor) primarily use olfactory cues associated with nest cavity construction to locate turtle nests. However, some other predators, including red foxes (Vulpes vulpes) and other canids, may commonly use a wider array of cues, including scents from nesting turtles and their eggs as well as visual cues, while foraging. The literature also suggests that the length of the period turtle nests remain vulnerable to nest predation is dependent on the predator community, with raccoons exhibiting relatively short timelines relative to some other predators, including canids. This review has revealed a strong North American bias in published work, highlighting the need for additional studies of turtle nest predation dynamics in other areas of the world where chelonians are often imperiled elements of the biota.
Introduction
For oviparous reptiles, the embryonic stage is one of the most vulnerable phases of the life cycle (Fitch and Fitch, 1967), and high levels of egg predation characterize many chelonian populations (Iverson, 1991). For the majority of turtle species, females provide little or no maternal care, thus successful incubation requires that females select nest sites with appropriate thermal and hydric conditions for incubation (e.g., Schwarzkopf and Brooks, 1987; Wilson, 1998; Morjan, 2003; Pruett et al., 2019) and that nests evade detection by egg predators (Spencer, 2002; Spencer and Thompson, 2003, 2005). Turtle eggs comprise an energy-rich food source (Booth, 2003) and vertebrate egg predators are a predominant source of egg mortality in freshwater turtle species worldwide. Because many turtle species have relatively specific habitat requirements (e.g., Burger and Montevecchi, 1975; Bodie et al., 1996; Wilson, 1998; Paterson et al., 2013) and often exhibit high levels of nest site fidelity (Freedberg et al., 2005), populations are increasingly vulnerable to elevated nest predation rates as habitat loss and degradation (e.g., vegetational succession) reduces the size of nesting habitat and nest predators such as raccoons (Procyon lotor) concentrate their foraging efforts in the remaining areas (e.g., Temple, 1987; Jackson and Walker, 1997; Marchand and Litvaitis, 2004).
Turtle nest predation and the factors that influence it have long been of interest to both amateur natural historians and academics alike. Sensory cues used by predators to identify location of turtle nests are of considerable importance to researchers interested in turtle ecology and conservation, as reduction of the signal strength of these cues may aid management efforts to increase hatchling recruitment. Beginning with possibly the first reference to raccoon depredation of turtle nests/eggs (Kennicott, 1858) and the first experimental efforts to understand the underlying nest location dynamics (Moll and Legler, 1971; Wilhoft et al., 1979), numerous studies spanning nearly 70 years have speculated on, or experimentally evaluated, the sensory cues used by mammalian predators to locate turtle nests. In spite of these efforts, little consensus has emerged as to the primary nest location cues used by mammals, and the related literature is inconsistent and sometimes conflicting; especially perhaps, for raccoons, the most commonly studied turtle nest predator in the world.
The purpose of this review is to provide the first comprehensive synthesis of our present understanding of the sensory cues used by mammalian predators to identify and locate nests of freshwater turtles and to suggest productive areas for future research. In our review, we place an emphasis on predation by raccoons, and often compare findings on other predators to them, because of the disproportionately large number of studies where they were documented to be the predominant nest predator in this cue-focused review (Table 1). Nonetheless, we have included results from studies of other mammalian species where available.
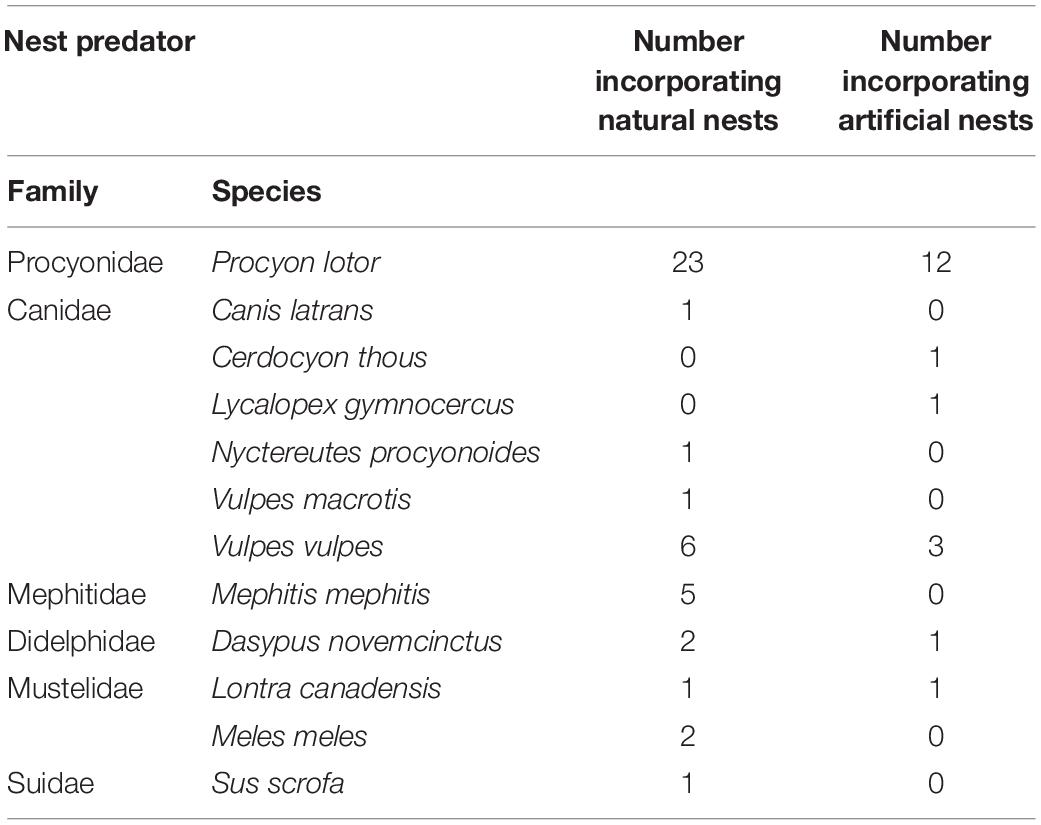
Table 1. Number of papers in this review reporting predominant mammalian predators of natural or artificial nests (n = 46 citations).
Methods
Literature Review and Analysis
During the course of this review, we surveyed over 90 papers focusing on or incidentally reporting nest predation metrics for freshwater turtles. We found primary literature by on-line searches using Google, Google Scholar, and the academic research databases of the University of Wisconsin Library System (>1100 e-collection content selections from Primo Central Index [PCI] from Ex Libris [ProQuest] including Web of Science and Scopus) using the keywords: “turtle:nest:predation”. We also subsequently reviewed the literature-cited sections of all these papers for additional sources relevant to freshwater turtle nest predation. Materials were mostly peer-reviewed, published works, although some unpublished MSc and Ph.D. theses were also included in this review.
For simplicity, we grouped the proposed freshwater turtle nest location cues used by mammalian predators in the reviewed literature into recurring principal types (visual, tactile, olfactory). Within each category we highlight certain studies when, in our view, the presented data are unique or particularly definitive in support of study conclusions or, contrarily, when alternate interpretations appear available for reported results. The provided tables offer an overview of either the claims made or inferences which could be drawn (as in the text, sometimes not stated by the authors) from all reviewed materials. Papers that suggest that certain nest location cues may be operative, but only cite previous publications without providing new data in support, are either not noted or are distinguished as such. Finally, we also made a distinction between nest location cues present soon after nest construction and those present during the hatchling emergence period, and report on these as appropriate. While we recognize that predators likely do not rely exclusively on a single cue, and may use multiple cues to locate turtle nests depending on environmental conditions, our intent is to provide a synthesis of the evidence for the primary sensory cues used by mammalian predators as documented in the literature.
Results
Categories of Proposed Cues
Three broad categories of cues are prominent among those proposed to explain how raccoons and other mammalian predators locate newly created, freshwater turtle nests: (1) olfactory, (2) visual, and (3) tactile. Investigations within each of these categories have attempted to determine the cues of primary importance to nest-foraging predators (Table 2), with varying levels of empirical support—from observation-based speculation to supporting data. Consequently, in some cases, there is disagreement among researchers as to which cues have most explanatory value in observed nest predation rates (Tables 2, 3).
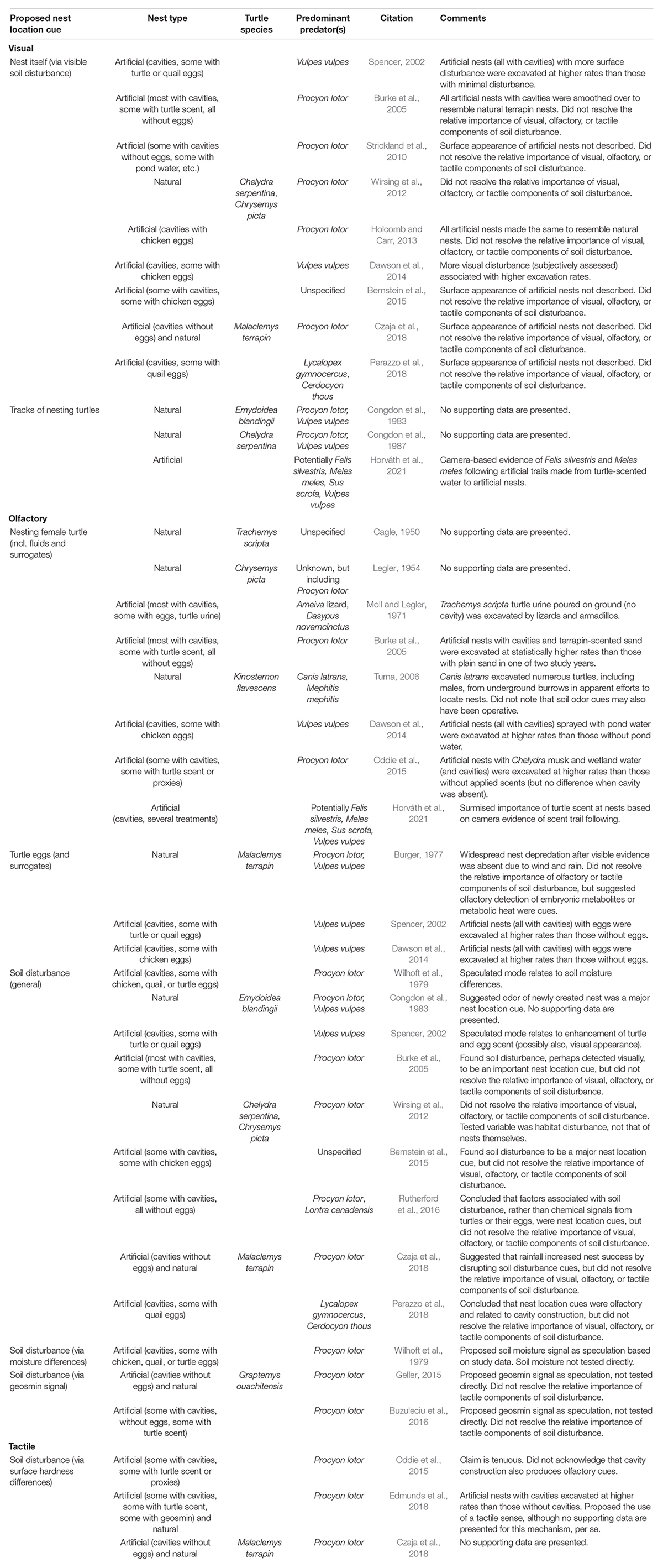
Table 2. Papers proposing cues used by mammalian predators to locate freshwater turtle nests before the emergence period.
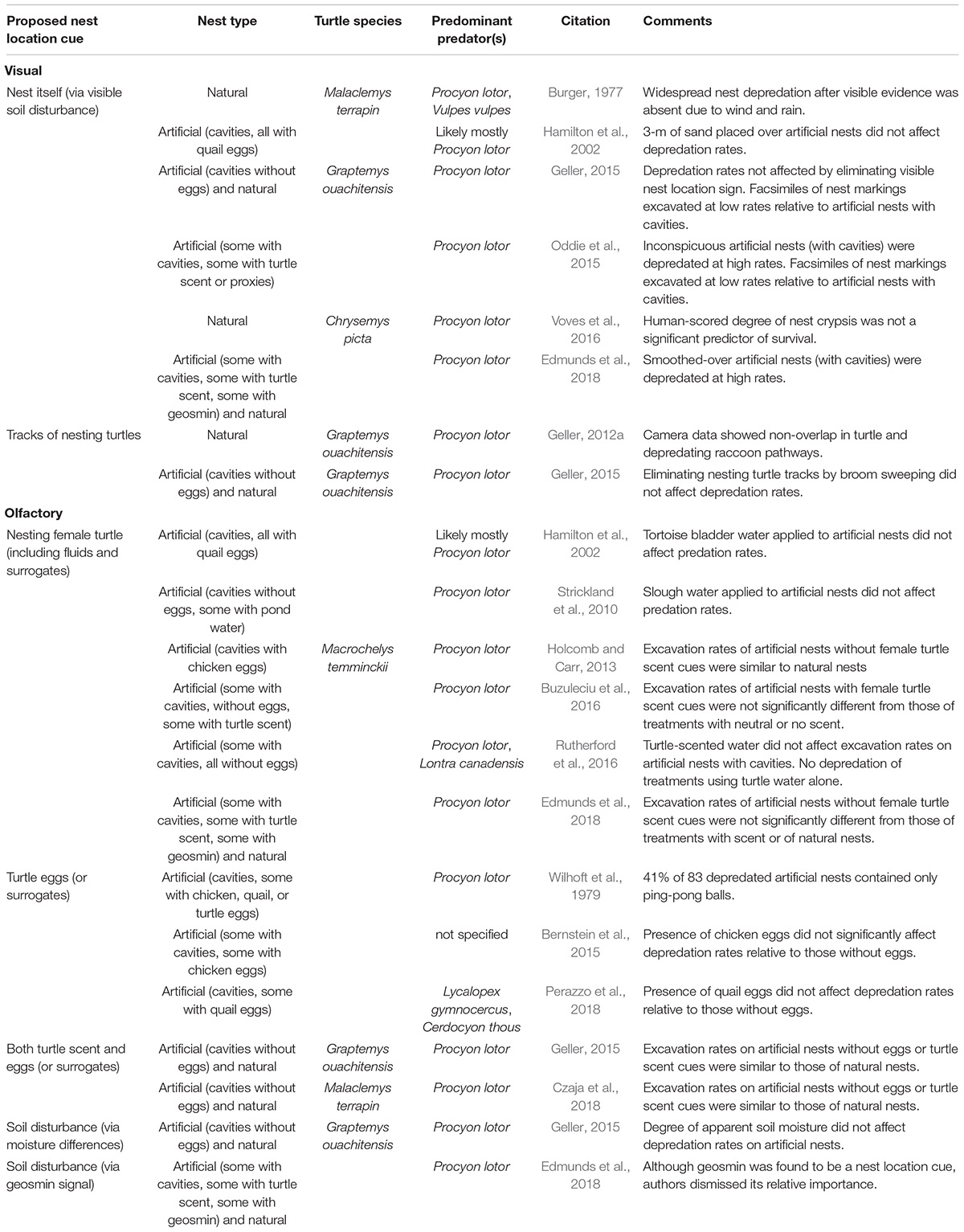
Table 3. Papers discounting particular cues used by predators to locate freshwater turtle nests before the emergence period.
Visual Cues
Assessment of Nest-Related Visual Cues
Relatively few studies investigating the sensory cues used by mammalian predators to locate freshwater turtle nests have provided definitive evidence of reliance on visual cues, although most studies to-date have focused largely on just raccoons and study designs sometimes limit interpretations (Table 2). For example, while experimental studies using artificial nests have suggested that nest-surface markings may be important cues for foraging raccoons, uniformity in surface markings among artificial nest treatments (e.g., all smoothed over or all made to resemble natural nest appearance) reduce the ability to test effects of different visual signal strengths or isolate them from other potentially co-occurring cues inherent in artificially constructed nest cavities: a limitation which is only sometimes implied/acknowledged (Burke et al., 2005; Bernstein et al., 2015; Czaja et al., 2018; Perazzo et al., 2018).
Burger (1977) was apparently the first to suggest that visual cues were not necessary for successful nest location by raccoons and red foxes (Vulpes vulpes), based on widespread predation of diamondback terrapin (Malaclemys terrapin) nests after visual cues had been eliminated on the sand-substrate nesting site by wind and rain. Results of several subsequent studies align with these results, including the experimental evidence provided by Hamilton et al. (2002) who found that 3-m wide areas of sand smoothed over artificial cavities containing quail eggs did not lower excavation rates by raccoons and other predators relative to unobscured nests (see also Edmunds et al., 2018; Table 3). Geller (2015) demonstrated that elimination of surface markings at both natural and artificial nests by broom sweeping did not reduce raccoon depredation rates between experimental and control nests. Additionally, artificial representations of the visual markings made by Ouachita map turtles (Graptemys ouachitensis) and snapping turtles (Chelydra serpentina) during nesting, but without underlying cavities, were excavated at low rates relative to artificial nests with cavities (≤26 vs. 94%, Geller, 2015; ca. 10 vs. ca. 50%, Oddie et al., 2015, from their Figure 3A; respectively). Finally, Voves et al. (2016) found that human evaluation of the degree of visual conspicuousness of natural painted turtle (Chrysemys picta) nests was not a consistent predictor of predation likelihood by raccoons.
In contrast, artificial nest cavities simulating surface disturbance by nesting female Australian Murray River turtles (Emydura macquarii) were excavated at greater rates by introduced red foxes than control nests with minimal surface disturbance, independent of whether artificial nests contained turtle eggs (80% depredation on disturbed treatment vs. 60% control), Coturnix quail eggs (70% disturbed vs. 57% undisturbed), or were without eggs (Spencer, 2002; his Figure 3). Similarly, Dawson et al. (2014) found that red foxes excavated more artificial cavities simulating oblong turtles Chelodina colliei nests initially scored as more obvious than those that were more cryptic.
Collectively, we found no papers that suggest visual cues at nests may be important to foraging raccoons provide unambiguous data in support (see Table 2), while several other papers present data to the contrary (Table 3). However, foxes may use visual cues associated with surface disturbance, likely in combination with other signals, to locate turtle nests.
Assessment of Visual/Olfactory Cues From Tracks of Nesting Turtles
Congdon et al. (1983) were the first to suggest that predators may use tracks of nesting females (which contain both visual and olfactory elements) to locate turtle nests. However, their statement was likely based on observation or anecdotal reports. The first experimental test of this hypothesis found that eliminating the tracks of nesting females by daily broom sweeping did not reduce depredation rates by raccoons on either natural or artificial nests (Geller, 2015). Camera data has also demonstrated that the foraging paths of nest depredating raccoons do not align with the paths of nesting Graptemys (Geller, 2012a,2015). Moreover, inducing raccoons to follow scent trails in experimental settings has proved difficult (S. Temple, pers. comm.). While these limited data suggest tracks may not be necessary, per se, for raccoons to find turtle nests, it is possible that nesting tracks are followed when more evident, such as in moist soils following rainfall or may be otherwise context dependent at the nest site or geographic location level.
For non-raccoons, however, Horváth et al. (2021), in Slovakia, EU, reported camera-based evidence of trail-following by both European wildcats (Felis silvestris) and European badgers (Meles meles); both of which followed artificially provided scent trails made of turtle-scented water to artificial European pond turtle (Emys orbicularis) nests. To our knowledge, this is the first well-documented report of trail following by a predator of freshwater turtle nests to appear in the literature.
Olfactory Cues
Research on which olfactory cues predators use to identify turtle nests appears early in the literature and continues to be an area of great interest for turtle biologists. In one form or another, olfactory cues have generated most research interest to date (referenced in almost all of the studies in Tables 2, 3). Within the context of nest predation, predators can potentially detect nests directly, using scents produced by nesting turtles, turtle eggs, or disturbed soil profiles produced during nest construction, or indirectly by following scent trails (see above) produced by nesting females during travel to and from nesting sites.
Assessment of Olfactory Cues From Nesting Turtles
In some of the earliest studies addressing the question of turtle nest predation, authors suggested that scents directly associated with oviposition could be used by predators to identify nest location. For example, Cagle (1950) suggested, based on anecdotal reports from professional egg collectors, that scent from gravid female turtle urine or oviductal fluids released during oviposition may function as a nest location cue for predators. Similarly, Legler (1954) speculated that “odoriferous fluid voided by the nesting female” may provide an olfactory cue for predators, although he did not provide data in support. In a subsequent study, however, Moll and Legler (1971) found that Ameiva lizards and armadillos (Dasypus novemcinctus) preferentially excavated artificial nests treated with female turtle urine compared to controls. Although that study was based on a small sample size (n = 1 treatment replicate), to our knowledge, this is the first experimental study to conclude that some vertebrate predators locate nests using scent cues directly produced by gravid female turtles during oviposition.
Subsequent experimental studies have continued to investigate the relative importance of olfactory cues directly associated with gravid female turtles during nesting. In these studies, authors typically applied water from nearby wetlands or from containers housing captive turtles to artificial nests and measured predation rates (Tables 2, 3). Most of this research has not provided evidence that scent from nesting female turtles increases the probability of nest predation by raccoons (Table 3). However, Burke et al. (2005) found that raccoons excavated a greater proportion of smoothed-over artificial nests with cavities refilled with Malaclemys terrapin-scented sand than when only unscented sand was used (means ca. 93%, n = 56 vs. 50%, n = 56, respectively; estimated from their Figure 1; difference statistically significant in one of two study years). In a follow-up study (Edmunds et al., 2018), 47% (n = 17) of artificial treatments consisting of 100 mL of terrapin-scented sand deposited on the surface were excavated by raccoons, suggesting the use turtle scent as a cue (not emphasized by authors). However, potentially informative controls of same-source sand without turtle scent were absent, and the excavation rate of artificial nests with cavities with terrapin scent (82.7%, n = 75) was similar to that of cavities without turtle scent (75%, n = 116) (2-tailed Fisher’s exact test p-value = 0.2831; analysis ours). Similarly, Oddie et al. (2015) found that raccoon excavation rates on their visually inconspicuous artificial nests with cavities supplemented with Chelydra musk (ca. 40%) or pond water (ca. 55%) were greater than those without these applied scent cues (ca. 20%; from their Figure 3A). However, locations where these same scents were applied to “artificial nests” that consisted only of visual surface markings were excavated at lower rates (<5% each) than when a cavity was present (overall mean ca. 47%), indicating that some factor associated with nest cavity presence was more influential in nest predation risk than odors directly associated with nesting turtles. Essentially the same conclusion was reached by Oddie et al. (2015), who suggested a synergistic effect of multiple cues in both nest detection and nest predation. However, they considered that cavity-related cue to be tactile, in contrast to some other explanations (such as soil odor, see below).
Related studies on non-raccoon predators are more limited, however Dawson et al. (2014) reported that artificial turtle nests with cavities sprayed with turtle pond water were excavated at higher rates by red foxes than those without pond water, both when they contained chicken eggs (53 vs. 48%) and when chicken eggs were absent (43 vs. 38%). Tuma (2006) noted that coyotes excavated adult yellow mud turtles (Kinosternon flavescens), including males, from their underground burrows while searching for nests. He attributed this to coyotes being attracted to the smell of the turtles, rather than to the smell of the eggs, although other cues, such as odors from disturbed soils, may also have been involved. And recently, Horváth et al. (2021) documented scent trail following by two European mesopredators and surmised that odors from nesting Emys orbicularis were likely the primary nest location cues.
Overall, although not without exception, most research on raccoons indicates little use of residual turtle scent at nests as nest location cues, while limited evidence suggests that canids and other nest predator taxa may use turtle scent cues to greater extents. More studies on the nest-foraging behaviors of a wider array of mammalian nest predators will be necessary to reveal potential interspecific differences in the reliance on scents associated with gravid female turtles.
Assessment of Olfactory Cues From Turtle Eggs
Artificial nest studies comparing depredation rates of nests with turtle eggs or surrogates to those without eggs provide experimental tests of the importance of egg presence as a factor in nest predation dynamics. In an early study of this type, Wilhoft et al. (1979) found that raccoons actually excavated a greater percentage of the artificial nests depredated in their study (n = 83) when they contained only ping-pong balls (41%) than when they contained either turtle eggs (25%) or bird eggs (34%). Similarly, neither the presence nor absence of eggs appeared to influence artificial nest excavation rates in results obtained by Bernstein et al. (2015) in North America (predator species not reported), or by Perazzo et al. (2018) for two native fox species in southern Brazil.
A few raccoon-based studies (Geller, 2015; Oddie et al., 2015; Czaja et al., 2018) have compared concurrent nest depredation rates between natural nests and artificial nests with cavities lacking both turtle scent cues and eggs (i.e., just refilled cavities). With the exception of Oddie et al. (2015), which found predation rates on natural nests (57% within 4 days of oviposition) to be higher than those of artificial nests without applied scent cues, eggs, or visual disturbance (20%; from their Figure 3A), these studies found that raccoon depredation rates of artificial nests lacking these olfactory signals were similar to those of natural nests, where these potential cues were present.
However, these results contrast with those obtained both by Spencer (2002) and Dawson et al. (2014) for red fox depredation of simulated nests in Australia. Spencer (2002) found higher excavation rates of artificial nests with either turtle or quail eggs than those without eggs, both when surface disturbance was reported as more evident (ca. 80% for turtle eggs and 70% for quail eggs vs. 60 and 45%, respectively) and when efforts were made to minimize disturbance (ca. 60% for turtle eggs and 57% for quail eggs vs. 20 and 15%, respectively; from his Figure 3). Dawson et al. (2014) also reported that artificial nests with chicken eggs were excavated by foxes at higher rates than those without eggs both when sprayed with pond water (53 vs. 43%, respectively) and when not sprayed (48 vs. 38%, respectively).
Overall, there is limited definitive evidence to date suggesting that olfactory cues from nesting turtles or turtle eggs are important cues to raccoons foraging for newly constructed freshwater turtle nests. However, as noted regarding visual cues, olfactory cues from eggs or nesting turtles may be used to a greater extent by nest-foraging red foxes and certain other nest predators (Tables 2, 3).
Tactile and Olfactory Cues From Soil Disturbance
Many researchers have suggested that some factor(s) associated with soils disinterred during the course of nest construction may function as location cues to freshwater turtle nest predators, with at least 13 of 26 studies reviewed in Tables 2, 3 either suggesting this possibility or providing data demonstrating increased nest predation associated with soil disturbance.
Assessment of Olfactory Cues From Soil Disturbance Versus Tactile Cues
Efforts to resolve which factors associated with soil disturbance function as nest location cues to predators have been complicated by the confounding presence of co-occurring visual, olfactory, and tactile signals inherent in nest cavity construction, resulting in differences in proposed nest detection mechanisms across studies (Table 2). For example, in the earliest experimental demonstration of the role of disturbed soil as a scent cue, Wilhoft et al. (1979) speculated that olfactory differences caused by the variation in soil moisture between disturbed and intact soils were responsible for increased raccoon predation of artificial nests. Alternatively, Spencer (2002) suggested that disturbed soils, in addition to increasing the potential visual evidence, may enhance the odors of nesting females or their eggs. Several studies have reported on predator excavation of artificial nests constructed by removing soil and replacing it into the nest cavity without surrogate eggs or the application of additional olfactory cues (Tables 2, 3). The elimination of experimental additions of olfactory or visual cues from artificial nests presumably limits predators to two options for locating nests: either olfactory cues originating directly from soil disturbance, or tactile cues resulting from differences in soil density between the nest and the surrounding area.
Geller (2015) and Buzuleciu et al. (2016) attempted to decouple the co-occurring sensory cues present at newly constructed turtle nests, each concluding that the odor of disturbed soils is the primary cue used by raccoons when foraging for turtle nests. In Geller (2015), high predation rates on broom-swept, natural G. ouachitensis nests and artificial nest cavities without eggs, showed that neither visual evidence of nesting turtles, nor olfactory cues from nesting turtles or their eggs were necessary for raccoons to locate nests. Although surface hardness differences between natural and artificial nests and the surrounding substrates were not tested, camera data consistently documented raccoons foraging for turtle eggs with noses close to the ground as they searched turtle nesting areas, strongly suggesting a reliance on olfactory, rather than tactile nest cues (Geller, 2015; Figure 1). Based on these observations, scents derived directly from soils disinterred during nest construction were considered primary nest location cues.
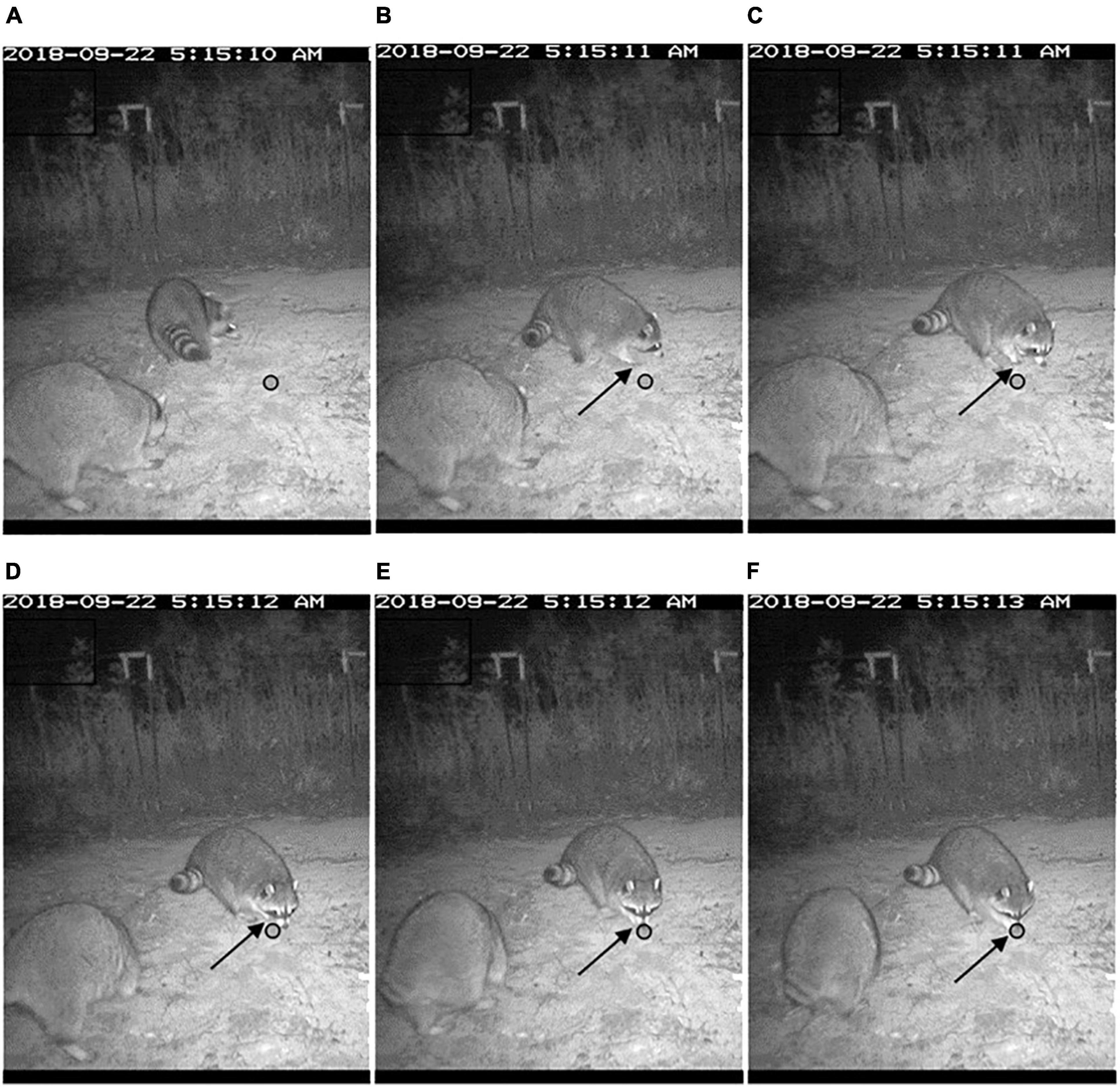
Figure 1. Typical raccoon foraging sequence in finding natural turtle nests and artificial nest cavities: (A,B) approach with nose within 2 cm of surface, (C,D) nose and head move over detected cavity, (D–F) forefoot moves forward into position under nose, (F) excavation begins. Forefoot position indicated by arrows; cavity location indicated by dark circle.
Similarly, Buzuleciu et al. (2016) demonstrated that artificial nests with cavities were associated with three to four times higher raccoon predation than those consisting only of surface-applied scent (gravid female M. terrapin scent, neutral scent, and no-scent control). This study also tested the hypothesis that scent of recently disinterred soil was the primary olfactory cue used by raccoons to locate terrapin nests by comparing the predation rate of unprotected, recently constructed artificial nests to that of artificial nests “aged” for 48 h within raccoon exclusion cages. They found that depredation rates of newly created artificial nests (all with 10–12 cm deep, refilled cavities) were about five times higher than that for 48-h-old nests (84 vs. 16%, respectively). Caging artificial nests for 48 h presumably allowed volatile organic compounds to dissipate, resulting in reduced olfactory cues for the raccoons. Given that artificial nests were caged for a relatively brief duration (48 h) and no rainfall occurred during the experimental period, it is unlikely that soil compaction in the caged treatments prevented raccoons from using tactile searching to locate the opening of the artificial nest chamber.
Both Geller (2015) and Buzuleciu et al. (2016) independently proposed that geosmin, an odiferous metabolite from the soil microbe Actinomycetes aerosolized from disturbed soil profiles and recognized by humans as the smell of disturbed soil (Lindbo et al., 2012), may be one of the underlying olfactory cues produced during nest cavity construction. Based on this proposition, the odor of geosmin alone, or in combination with other related microbial hydrocarbons released by soil disturbance during nest cavity construction, serves as a point-source signal identifying locations of turtle nests to raccoons and possibly other nest predators.
Edmunds et al. (2018), in the first direct test of geosmin as a nest location cue, found that locations where small amounts of geosmin were shallowly injected under soil substrates were, indeed, excavated by raccoons. They further noted that greater administered volumes of geosmin at the same concentration (0.5 mg geosmin/1 mL methanol) resulted in higher excavation rates (25% excavation rate at 0.1 mL, n = 20 vs. 37% at 0.2 mL, n = 19). However, because excavation rates of geosmin treatments were lower than those of both natural nests (67%; n = 42) and standard artificial nest cavities without eggs (overall 82.7%, n = 75, from Treatment 1 in their Table 1), Edmunds et al. (2018) instead concluded that raccoons primarily use tactile cues associated with differences in soil density between nests and surrounding substrates to identify nest locations, although they did not experimentally test anything related to substrate density. Moreover, they did not explore how additional increases in amounts or concentrations of geosmin in their experimental trials may have influenced nest predation rates or discus how the volatilization timelines of the small amounts of injected geosmin in their study may differ from that within the volumes of soil disturbed during natural nest construction.
The only study purporting to provide data supporting the use of surface hardness as a tactile cue for foraging raccoons is Oddie et al. (2015; Table 2). In that study, artificial nests with manufactured cavities (presenting a less-compacted soil surface relative to the surrounding substrate) experienced a higher depredation frequency compared to artificial nests without cavities, independent of whether visual or additional olfactory cues were present. While Oddie et al. (2015) concluded that raccoons probably use more than one sensory cue to locate nests, they proposed that tactile cues resulting from cavity presence were used to make a final determination as to whether to excavate a nest. However, because all treatment combinations used to test a “tactile” (cavity present) predator response (i.e., applied turtle scent, pond water scent, no-scent, or visual treatment) shared hand-excavated and refilled cavities, their results are confounded by scent cues originating from soil disturbance and therefore make the role of tactile cues difficult, if not impossible, to resolve.
How Long Do Nest Location Cues Last?
Assessment of Longevity of Nest Location Cues
With few exceptions, studies indicate that newly constructed turtle nests experience most predation within a few days of construction (Table 4). Nonetheless, a few studies (e.g., Wilhoft et al., 1979) have suggested that the risks of predation on freshwater turtle nests can extend much later into reproductive periods (Table 5). However, one caveat to interpreting the scent cue longevity observations in Wilhoft et al. (1979) is that their raccoon-depredated artificial nests were made in late July, weeks after natural Chelydra serpentina nesting activity had ended. Thus, their results only show that raccoons were still foraging at their study sites and would depredate newly constructed artificial nests during these later dates, not that the aging, natural turtle nests made during the just-completed nesting season were still vulnerable to predators. As a result, some authors have used this paper as support for claims of late-season nest cue retention and vulnerability to depredation, without recognizing this important distinction.
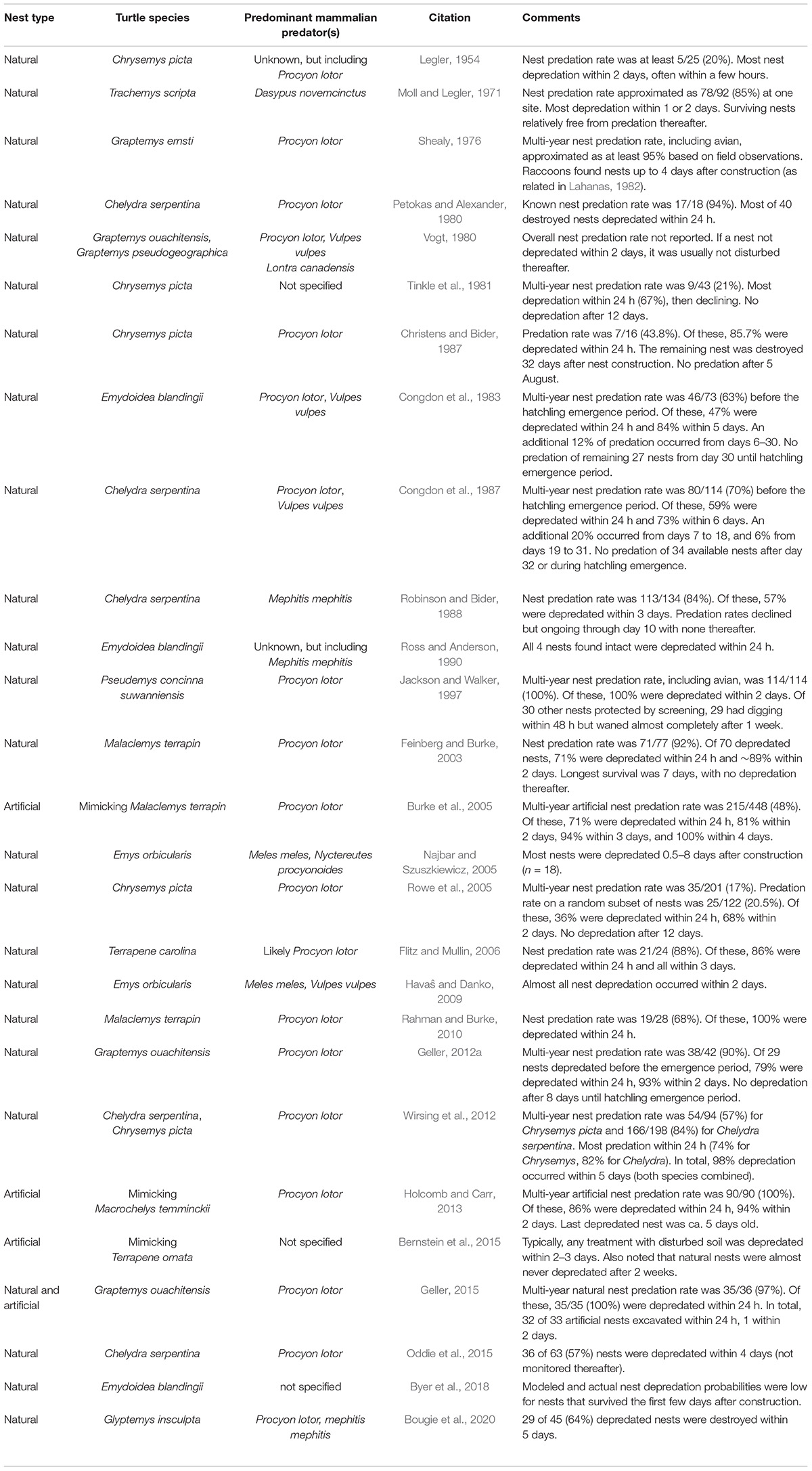
Table 4. Papers proposing signal strength of cues used by mammalian predators to locate freshwater turtle nests decreases soon after nest construction.
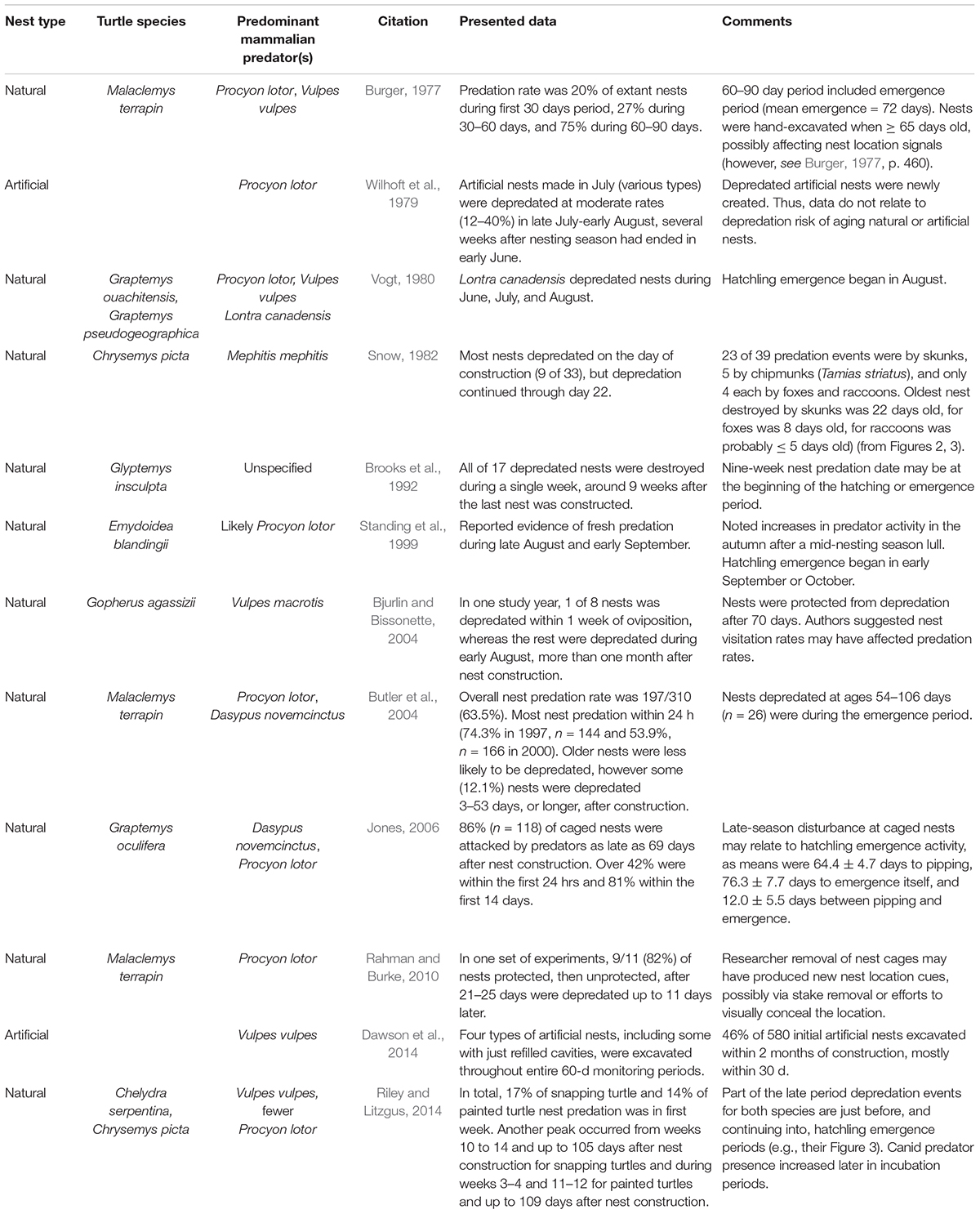
Table 5. Papers suggesting the signal strength of cues used by mammalian predators to locate freshwater turtle remains long after nest construction.
Further examination of papers demonstrating long-term nest predation risk reveals two main commonalities which may explain divergence from studies that report shorter nest vulnerability timelines: the inclusion of data from hatchling emergence periods in predation risk assessments or having nesting areas with more diverse predator communities, rather than just raccoons (Table 5). Both of these factors have introduced complexity, as well as some confusion, into the literature on turtle nest predation dynamics, and are discussed below.
Evidence for Renewed Nest Location Cues During Hatchling Emergence Periods
Beginning with Burger (1977), many authors have suggested that new nest location cues arise at the onset of, or during, the hatching period as hatchlings fracture eggshells and absorb residual yolk sacs, yet remain in or above the nest chamber prior to emergence. In studies surveying entire reproductive periods, these renewed cues result in a secondary nest predation peak and extend the reported age of depredated nests relative to studies with shorter timelines. Pre-emergence cues of nest location may include olfactory signals from embryonic fluids and disturbed soils created by subterranean hatchling movements, or hatchling vocalizations (Ferrara et al., 2012; Riley and Litzgus, 2014; Geller and Casper, 2019). Upon surface emergence, new nest location cues potentially include odors arising from nest cavities via exit holes (Congdon et al., 1983; Christens and Bider, 1987), and visual cues provided by hatchling tracks (Congdon et al., 1983; S. D. Gillingwater, pers. comm., in Riley and Litzgus, 2014). However, the importance of potentially confounding, olfactory cues co-occurring with visible tracks has not been investigated, nor have any published studies attempted to resolve the relative importance of these possible cues on predation frequency during the hatchling emergence period.
Assessment of Differences Among Predator Species in Nest Detection Timelines
A less often recognized source of variance in reported nest survival timelines concerns the composition of the involved predator community. To date, Galois (1996) represents the only experimental work that has attempted to directly assess the differential sensory capabilities of common turtle nest predators, finding that olfactory cues likely play a predominant role in turtle nest detection by both striped skunks (Mephitis mephitis) and raccoons, based on food-conditioning and discrimination learning trials. Galois (1996) further determined that striped skunks are likely more narrowly reliant on olfaction than raccoons, in accord with previous research demonstrating the importance of olfaction to foraging skunks (Langley, 1979; Nams, 1991). Raccoons, though also characterized by a well-developed olfactory sense, were found to use both tactile and visual cues to a greater degree than skunks.
Some inferences regarding the role of differential olfactory sense development on nest depredation dynamics in field settings can perhaps be gained by comparing depredation timelines in studies of both natural and artificial turtle nests. For example, in Snow (1982), often cited in support of long nest vulnerability timelines, striped skunks were strongly predominant predators, with little on-site presence by raccoons. Although that study did not examine nor discuss the potential implications of differential sensory capabilities among predator species, it is possible to deduce from Figures 2, 3 in that study that the oldest nests destroyed by skunks and foxes were 22 days old and 8 days old, respectively, while the oldest nest depredated by raccoons was likely ≤ 5 days old. Similarly, Congdon et al. (1987), proposing a differential, olfactory basis, found that red foxes destroyed older Chelydra serpentina nests than did raccoons (mean = 9.4 days, n = 32 vs. mean = 1.9 days, n = 13, respectively). Dawson et al. (2014) also documented long predation timelines by red foxes, with ongoing excavation of artificial nests up to 60 d old occurring throughout monitoring periods. The mid-season nest predation exhibited by skunks and canids likely reflects reliance on turtle egg scent cues themselves, as these odors are presumably the only ones remaining after potential visual, tactile, and other olfactory cues have faded.
In a recent review of nest predation timelines, Riley and Litzgus (2014) presented age-related nest predation data both from their own as well as previously published research. They concluded that nest location cues persist during the several-week period following nest construction and suggested that additional nest predation peaks after week one for the turtle nests in their study were associated with increased numbers of canids at these later dates. They also noted that interspecific differences in cues used by predators, in addition to predator densities and individual behavioral differences, may be partially responsible for reported variation in nest predation timelines. However, while Riley and Litzgus (2014) thus noted the potential for a predator species effect on nest depredation timelines, they did not discuss how differential sensory capabilities among predator species, per se, may have impacted their findings or largely explain the results of other research [e.g., the long vs. short predation risk timelines of Snow (1982) and Burke et al. (2005), respectively; see their Table 1]. In fact, red foxes, able to detect and depredate relatively old nests (see above), were strongly predominant in their study and may have been responsible for the somewhat atypical nest predation patterns they observed.
Available evidence thus indicates that the age-related risk of predation is, at least in part, dependent on the species composition of the local predator community due to differential sensory abilities among species.
Discussion
In this review we have attempted to synthesize much of the literature regarding the cues used by mammals in the predation of turtle nests derived from observational and experimental research produced over the past several decades. The bulk of the empirical data from predation studies on both natural and artificial nests suggests that raccoons, the predator most often the subject of such studies, predominantly rely on scent cues of disturbed soils to locate recently constructed freshwater turtle nests. Predation rates of natural nests remain high even after all potential visual cues (i.e., sign of nests and nesting turtle tracks) have been experimentally eliminated, and artificial nests with manufactured cavities are consistently found and excavated by raccoons at higher rates relative to those without cavities, regardless of whether or not they contain turtle scent, egg scent, or visual surface markings. In this regard, suggestions that female turtles make purposeful attempts to visually conceal nest locations by carefully finessing the nesting substrate (sensu Strickland et al., 2010) are, thus, called into question as far as raccoon predation is concerned. However, reducing the visual cues to nest presence is likely important in reducing predation risk by avian predators, whose foraging for turtle nests appears to be primarily visually based (e.g., Burger, 1977; Jackson and Walker, 1997; Butler et al., 2004; see also Voves et al., 2016). In contrast, foxes and other canids, striped skunks, and other predators may not only have better-developed olfactory senses than raccoons, but may also more commonly use a greater number of nest location signals in addition to those from recently disturbed soils, including visual surface disturbances and odors of nesting turtles and their eggs, to locate nests.
Raccoons are particularly effective nest predators because they have the capacity to respond to seasonal changes in food supply (Daglish and Anderson, 1979) and concentrate foraging effort at turtle nesting areas when these new food resources become available (Petokas and Alexander, 1980; Geller, 2012a). Raccoons are naturally inquisitive, and it is likely that they investigate a variety of scents suggestive of food. Typically, when turtle nests are located in soil or sand substrates, both egg and soil odors co-occur in newly constructed nests. Although it is possible that odors from disturbed soils may initially present a stronger olfactory signal than that from buried eggs, raccoons may still be able to resolve the egg-related odors within the olfactory mix, at least when nests are only a few days old. Nonetheless, raccoons appear to use the scent cue produced by disinterred soil as a “default” cue to indicate the presence of turtle eggs or perhaps other food items. Cueing in on olfactory signals of soil disruption would be an efficient foraging strategy because many potential prey items below the substrate may produce these same environmental signs, therefore reducing or eliminating the need for prey-specific scent recognition or detection.
In contrast, reliance on tactile cues to initially locate freshwater turtle nests would seem unlikely from an efficiency perspective, as it is reasonable to assume that raccoons, like other predators, use a foraging strategy that optimizes energetic gain while minimizing expenditure of energy and time (Emlen, 1966). Given the lack of unambiguous data in support of tactile searching by nest-foraging raccoons, and the likely inefficiency of physically detecting point sources of varying surface hardness over large nesting areas compared to “distance” senses of olfaction or vision (Galois, 1996), available evidence suggests that tactile searching is, at best, a complimentary, rather than primary means of locating turtle nests.
Survival of turtle nests is not only a function of nest cue signal strength, but also of predator species, the timing of predator presence after nest construction, and predator proximity to nests. Relatively short nest survival intervals, typically within 2 or 3 days, appear nearly universal where raccoons are the predominant predator (Table 4), while at least some nests that initially survive short-term predation are depredated at later dates when the mammalian predator community is more diverse and includes, for example, canids (Table 5). Canids, have long been known to possess advanced olfactory senses (Green et al., 2012; Lea and Osthaus, 2018). Although comparative behavioral studies between canids and other carnivores are scarce, at least some canids have large olfactory turbinal surface areas relative to most other carnivores that have been examined (reviewed in Lea and Osthaus, 2018) and red foxes have exceptionally large olfactory turbinal surface areas for their size (Green et al., 2012). We thus suggest that generalized claims as to nest predation risk extending well into nesting seasons be interpreted cautiously, as these timelines appear dependent, at least in part, on the composition of the local predator community. In areas where raccoon predation is dominant, nests may be most at risk shortly after oviposition and then again during hatching and emergence. In contrast, in environments where canids, for example, are dominant, the predation window may extend from oviposition through hatching due to their exceptional olfactory sensitivities.
While this review has allowed us to make generalized statements regarding the primary cues used by predators, the potential for local or context-dependent variation in nest predation dynamics must also be recognized. For example, some individual raccoons or populations may habitually follow the tracks of nesting female turtles to find nests, particularly when tracks are most evident in sparsely vegetated, moist substrates (e.g., see pg. 26 in Buhlmann et al., 2008). Similarly, the occasional excavation of artificially made markings that mimic those of natural nests (Geller, 2015; Oddie et al., 2015), suggests some use of visual cues in locating nests, as does the direct sighting of in-process, nesting turtles themselves (e.g., Jackson and Walker, 1997). Nesting sites also vary in surface soil hardness (e.g., sandy vs. organic soils), influencing the potential for tactile nest detection among sites (Oddie et al., 2015). Thus, while the well-established importance of olfaction to raccoon foraging (Bowman and Harris, 1980; Ruzicka and Conover, 2011) aligns with the primary findings of this review, some variation in cues and sensory modes used to locate nests is to be expected given possible differences in the relative strengths of nest location signals among settings and the wide array of well-developed senses available to these and other turtle nest predators.
Based on the results of this review, we have identified areas of nest predation dynamics in need of further study. We support Edmunds et al. (2018) in calling for more research on the role of geosmin—and possibly other volatile soil hydrocarbons—in turtle nest detection, particularly as it may be a common factor underlying mammalian detection of natural nest cavities globally. More generally, this review has revealed a significant need for expanded research on turtle predation dynamics in areas outside of North America (85.7% of the 49 citations in Tables 2–5 originate from the United States and Canada). In addition to expanding our knowledge of similarities and differences among mammalian taxa regarding sensory cues used to identify turtle nests, a broader overview of species-specific mammalian nest predation dynamics may help inform local conservation strategies as to which predator species are in greatest need of management and when nest protection measures are most effectively applied. In these efforts, we anticipate that the increasing use of trail cameras will likely lead to larger and better-resolved datasets regarding turtle nest survival timelines and nest predators in both natural and experimental settings. Multi-year studies on both specific turtle populations and across geographies will be particularly useful in understanding how nest predation dynamics may change over space and time (Edmunds et al., 2018) as a result of individual predator variation or culturally influenced learning (e.g., for raccoons, Gehrt, 2004).
Regardless of the potential for targeted management to reduce turtle nest predation by disrupting nest location cues (e.g., by shallow-tilling substrates to obscure the olfactory signals of newly constructed nests), maintaining or optimizing nesting area integrity in terms of vegetative cover, soil condition, and other ecological characteristics over long periods of time remains paramount. In addition to predator exclusion (Buhlmann and Osborn, 2011; Geller, 2012b; Quinn et al., 2015), efforts to increase turtle nesting success where raccoons are the predominant nest predator may benefit from enlarging and maintaining the open habitats often used by nesting turtles, thereby minimizing chance encounters with the point sources of disturbed soils identified as predominant nest location cue for raccoons (see Temple, 1987; Jackson and Walker, 1997; Marchand and Litvaitis, 2004; Vilardell et al., 2008). However, even with such efforts, success may be limited in areas where raccoons have substantially elevated populations due to anthropogenic influence or where foxes are important nest predators, as they, and possibly some other nest predators, may also use scent trails and other cues to individually track and locate nests over greater distances in a more directed fashion.
One of the few ways turtles may be able to reduce the olfactory and visual cues that necessarily arise during nest cavity construction is to nest shortly before significant rainfall. However, to date, there is little evidence that turtles show this propensity (e.g., see Czaja et al., 2018). Total annual precipitation and single event precipitation amounts are expected to increase worldwide under present and projected climate change scenarios, although these effects will not be evenly distributed (Wuebbles et al., 2017). In areas likely to experience increased precipitation frequency and amounts (e.g., northeastern United States) nest success may increase due to greater proportions of nests being constructed before rainfall by chance alone, while the opposite may be true in areas likely to experience historically lower levels of precipitation (e.g., Mediterranean Basin) (see also Czaja et al., 2018). However, as changing rainfall patterns also impact air and substrate temperatures, nest site flooding potentials, and the amount and composition of vegetational cover on nesting areas—all of which have implications for nesting habitat suitability and embryo/hatchling survival—the ultimate impact of anthropogenic precipitation change on worldwide turtle populations is unclear and will likely be context dependent.
Author Contributions
GG conducted the literature review and wrote the first draft including 4 of the 5 tables and the figure. SP edited and contributed to the first and subsequent drafts, provided additional citations, and contributed to 1 of 5 tables. Both authors contributed to the article and approved the submitted version.
Conflict of Interest
The authors declare that the research was conducted in the absence of any commercial or financial relationships that could be construed as a potential conflict of interest.
Publisher’s Note
All claims expressed in this article are solely those of the authors and do not necessarily represent those of their affiliated organizations, or those of the publisher, the editors and the reviewers. Any product that may be evaluated in this article, or claim that may be made by its manufacturer, is not guaranteed or endorsed by the publisher.
Acknowledgments
We would like to thank A. Pidgeon for assistance with literature searches and Coastal Carolina University’s Gupta College of Science and Department of Biology for financial and logistical support. We are also grateful for the efforts of several reviewers in improving early manuscript drafts.
References
Bernstein, N. P., McCollum, S. A., and Black, R. W. (2015). How do predators locate nests of Ornate Box Turtles (Terrapene ornata)? A field experiment. Herpetol. Conserv. Biol. 10, 44–53.
Bjurlin, C. D., and Bissonette, J. A. (2004). Survival during early life stages of the Desert Tortoise (Gopherus agassizii) in the south-central Mojave Desert. J. Herpetol. 38, 527–535. doi: 10.1670/94-02a
Bodie, J. R., Smith, K. R., and Burke, V. J. (1996). A comparison of diel nest temperature and nest site selection for two sympatric species of freshwater turtles. Am. Midl. Nat. 136, 181–186. doi: 10.2307/2426643
Booth, D. T. (2003). Composition and energy density of eggs from two species of freshwater turtle with twofold ranges in egg size. Comp. Biochem. Physiol.Part A Mol. Integr. Physiol. 134, 129–137. doi: 10.1016/s1095-6433(02)00216-7
Bougie, T. A., Byer, N. W., Lapin, C. N., Peery, M. Z., Woodford, J. E., and Pauli, J. N. (2020). Wood turtle (Glyptemys insculpta) nest protection reduces depredation and increases success, but annual variation influences its effectiveness. Can. J. Zool. 98, 715–724. doi: 10.1139/cjz-2020-0064
Bowman, G. B., and Harris, L. D. (1980). Effect of spatial heterogeneity on ground-nest predation. J. Wildl. Manag. 44, 806–813. doi: 10.2307/3808308
Brooks, R. J., Shilton, C. M., Brown, G. P., and Quinn, N. W. S. (1992). Body size, age distribution, and reproduction in a northern population of wood turtles (Clemmys insculpta). Can. J. Zool. 70, 462–469. doi: 10.1139/z92-070
Buhlmann, K. A., and Osborn, C. P. (2011). Use of an artificial nesting mound by wood turtles (Glyptemys insculpta): a tool for turtle conservation. Northeast. Nat. 18, 315–334. doi: 10.1656/045.018.0305
Buhlmann, K., Tuberville, T., and Gibbons, J. W. (2008). Turtles Of The Southeast. Athens: University of Georgia Press, 252.
Burger, J. (1977). Determinants of hatching success in diamond-back terrapin, Malaclemys terrapin. Am. Midl. Nat. 97, 444–464. doi: 10.2307/2425108
Burger, J., and Montevecchi, W. A. (1975). Nest site selection in the terrapin Malaclemys terrapin. Copeia 1975, 113–119. doi: 10.2307/1442413
Burke, R. L., Schneider, C. M., and Dolinger, M. T. (2005). Cues used by raccoons to find turtle nests: effects of flags, human scent, and Diamond-backed Terrapin sign. J. Herpetol. 39, 312–315.
Butler, J. A., Broadhurst, C., Green, M., and Mullin, Z. (2004). Nesting, nest predation and hatchling emergence of the Carolina Diamondback Terrapin, Malaclemys terrapin centrata, in northeastern Florida. Am. Midl. Nat. 152, 145–155. doi: 10.1674/0003-0031(2004)152[0145:nnpahe]2.0.co;2
Buzuleciu, S. A., Crane, D. P., and Parker, S. L. (2016). Scent of disinterred soil as an olfactory cue used by raccoons to locate nests of Diamond-backed Terrapins (Malaclemys terrapin). Herpetol. Conserv. Biol. 11, 539–551.
Byer, N. W., Reid, B. N., Seigel, R. A., and Peery, M. Z. (2018). Applying lessons from avian ecology to herpetological research: techniques for analyzing nest survival due to predation. Herpetol. Conserv. Biol. 13, 517–532.
Cagle, F. R. (1950). The life history of the slider turtle, Pseudemys scripta troostii (Holbrook). Ecol. Monogr. 20:1.
Christens, E., and Bider, J. R. (1987). Nesting activity and hatching success of the painted turtle (Chrysemys picta marginata) in southwestern Quebec. Herpetologica 43, 55–65.
Congdon, J. D., Breitenbach, G. L., van Loben Sels, R. C., and Tinkle, D. W. (1987). Reproduction and nesting ecology of snapping turtles (Chelydra serpentina) in southeastern Michigan. Herpetologica 43, 39–54.
Congdon, J. D., Tinkle, D. W., Breitenbach, G. L., and van Loben Sels, R. C. (1983). Nesting ecology and hatching success in the turtle Emydoidea blandingi. Herpetologica 39, 417–429.
Czaja, R. A., Kanonik, A., and Burke, R. L. (2018). The effect of rainfall on predation of Diamond-backed Terrapin (Malaclemys terrapin) nests. J. Herpetol. 52, 402–405. doi: 10.1670/17-167
Daglish, J., and Anderson, S. (1979). A field experiment on learning by raccoons. J. Mammal. 60, 620–622. doi: 10.2307/1380105
Dawson, S. J., Adams, P. J., Huston, R. M., and Fleming, P. A. (2014). Environmental factors influence nest excavation by foxes. J. Zool. 294, 104–113. doi: 10.1111/jzo.12158
Edmunds, S. E., Kasparov, C. N., Yoon, J. B., Kanonik, A. K., and Burke, R. L. (2018). Twelve years later: reassessing visual and olfactory cues raccoons use to find diamondback terrapin nests. J. Herpetol. 52, 307–312. doi: 10.1670/17-029
Emlen, J. M. (1966). The role of time and energy in food preference. Am. Nat. 100, 611–617. doi: 10.1086/282455
Feinberg, J. A., and Burke, R. L. (2003). Nesting ecology and predation of diamondback terrapins, Malaclemys terrapin, at Gateway National Recreation Area, New York. J. Herpetol. 37, 517–526. doi: 10.1670/207-02a
Ferrara, C. R., Vogt, R. C., and Sousa-Lima, R. S. (2012). Turtle vocalizations as the first evidence of posthatching parental care in chelonians. J. Comp. Psychol. 127, 24–32. doi: 10.1037/a0029656
Fitch, H. S., and Fitch, A. V. (1967). Preliminary experiments on physical tolerances of eggs of lizards and snakes. Ecology 48, 160–165. doi: 10.2307/1933430
Flitz, B. A., and Mullin, S. J. (2006). Nest site selection in the Eastern Box Turtle, Terrapene carolina carolina, in Illinois. Chelonian Conserv. Biol. 5, 309–312. doi: 10.2744/1071-8443(2006)5[309:nsiteb]2.0.co;2
Freedberg, S., Ewert, M. A., Ridenhour, B. J., Neiman, M., and Nelson, C. E. (2005). Nesting fidelity and molecular evidence for natal homing in the freshwater turtle, Graptemys kohnii. Proc. R. Soc. Lond. B Biol. Sci. 272, 1345–1350. doi: 10.1098/rspb.2005.3080
Galois, P. (1996). Turtle nest sensory perception by raccoon (Procyon lotor) and striped skunk (Mephitis mephitis): an approach through discrimination learning of potential nest cues. PhD Dissertation. Montreal: McGill University.
Gehrt, S. D. (2004). “Ecology and management of striped skunks, raccoons, and coyotes in urban landscapes,” in Predators and People: From Conflict to Conservation, eds N. Fascione, A. Delach, and M. Smith (Washington, DC: Island Press), 81–104.
Geller, G. A. (2012a). Notes on the nest predation dynamics of Graptemys at two Wisconsin sites using trail camera monitoring. Chelonian Conserv. Biol. 11, 197–205. doi: 10.2744/ccb-0992.1
Geller, G. A. (2012b). Reducing predation of freshwater turtle nests with a simple electric fence. Herpetol. Rev. 43, 398–403.
Geller, G. A. (2015). A test of substrate sweeping as a strategy to reduce raccoon predation of freshwater turtle nests, with insights from supplemental artificial nests. Chelonian Conserv. Biol. 14, 64–72. doi: 10.2744/ccab-14-01-64-72.1
Geller, G. A., and Casper, G. S. (2019). Late-term embryos and hatchlings of Ouachita Map Turtles (Graptemys ouachitensis) make sounds within the nest. Herpetol. Rev. 50, 449–452.
Green, P. A., Van Valkenburgh, B., Pang, B., Bird, D., Rowe, T., and Curtis, A. (2012). Respiratory and olfactory turbinal size in canid and arctoid carnivorans. J. Anat. 221, 609–612. doi: 10.1111/j.1469-7580.2012.01570.x
Hamilton, A. M., Freedman, A. H., and Franz, R. (2002). Effects of deer feeders, habitat, and sensory cues on predation rate of artificial turtle nests. Am. Midl. Nat. 147, 123–134. doi: 10.1674/0003-0031(2002)147[0123:eodfha]2.0.co;2
Havaŝ, P., and Danko, S. (2009). “The European pond turtle in slovakia,” in European Pond Turtles, Emys orbicularis, ed. M. Rogner (Frankfurt: Edition Chimaira), 199–210.
Holcomb, S. R., and Carr, J. L. (2013). Mammalian depredation of artificial Alligator Snapping Turtle (Macrochelys temminckii) nests in North Louisiana. 2013. Southeast. Nat. 12, 478–491. doi: 10.1656/058.012.0303
Horváth, E., Kaňuch, P., and Uhrin, P. (2021). Predation on nests of the european pond turtle (Emys orbicularis): remarks from failed field experiments. Herpetol. Notes 14, 1067–1072.
Iverson, J. B. (1991). Patterns of survivorship in turtles (Order Testudines). Can. J. Zool. 69, 385–391. doi: 10.1139/z91-060
Jackson, D. R., and Walker, R. N. (1997). Reproduction in the Suwannee cooter, Pseudemys concinna suwanniensis. Bull. Fla. Mus. Nat. Hist. 41, 69–167.
Jones, R. L. (2006). Reproduction and nesting of the endangered ringed map turtle, Graptemys ocuilifera, in Mississippi. Chelonian Conserv. Biol. 5, 195–209. doi: 10.2744/1071-8443(2006)5[195:ranote]2.0.co;2
Kennicott, R. (1858). “Quadrupeds of Illinois injurious and beneficial to the farmers,” in Ex. Doc. 105, 35th Congress, 2nd Session, Rept. Commissioner Patents 1858, Agriculture, Vol. 1859, (Washington, DC), 241–256.
Lahanas, P. N. (1982). Aspects of The Life History Of The Southern Black-Knobbed Sawback, Graptemys nigrinoda delticola Folkerts and Mount. Unpublished M.S. thesis. Auburn, Ala: Auburn University.
Langley, W. M. (1979). Preference of the striped skunk and opossum for auditory over visual prey stimuli. Carnivore 2, 31–34.
Lea, S. E. G., and Osthaus, B. (2018). In what sense are dogs special? Canine cognition in comparative context. Learn. Behav. 46, 355–363.
Legler, J. M. (1954). Nesting habits of the Western Painted Turtle, Chrysemys picta bellii (Gray). Herpetologica 10, 137–144.
Lindbo, D. L., Kozlowski, D. A., and Robinson, C. (eds) (2012). Know Soil, Know Life. Madison, WI: Soil Science Society of America, 206.
Marchand, M. N., and Litvaitis, J. A. (2004). Effects of landscape composition, habitat features, and nest distribution on predation rates of simulated turtle nests. Biol. Conserv. 117, 243–251. doi: 10.1016/j.biocon.2003.07.003
Moll, E. O., and Legler, J. M. (1971). “The life history of a neotropical slider turtle, Pseudemys scripta, in Panama,” in Bulletin Los Angeles County Museum Natural History Society. (Los Angeles, LA: County Museum Natural History Society).
Morjan, C. L. (2003). Variation in nesting patterns affecting nest temperatures in two populations of painted turtles (Chrysemys picta) with temperature-dependent sex determination. Behav. Ecol. Sociobiol. 53, 254–261. doi: 10.1007/s00265-002-0570-3
Najbar, B., and Szuszkiewicz, E. (2005). Reproductive ecology of the European pond turtle Emys orbicularis (Linnaeus, 1758) (Testudines: Emydidae) in western Poland. Acta Zool. Crac. 48, 11–19. doi: 10.3409/173491505783995752
Nams, V. O. (1991). Olfactory search images in striped skunks. Behaviour 119, 267–284. doi: 10.1007/s004420050179
Oddie, M. A. Y., Coombes, S. M., and Davy, C. M. (2015). Investigation of cues used by predators to detect Snapping Turtle (Chelydra serpentina) nests. Can. J. Zool. 93, 299–305. doi: 10.1139/cjz-2014-0264
Paterson, J. E., Steinberg, B. D., and Litzgus, J. D. (2013). Not just any old pile of dirt: evaluating the use of artificial nesting mounds as conservation tools for freshwater turtles. Oryx 47, 607–615. doi: 10.1017/s0030605312000877
Perazzo, G. X., Garcez, D. K., Trindade, C. R., Pereira, K. M., and Tozetti, A. M. (2018). Is the presence of eggs a relevant cue for predators of freshwater chelonian nests? Neotr. Biol. Conserv. 13, 350–355.
Petokas, P. J., and Alexander, M. M. (1980). The nesting of Chelydra serpentina in northern New York. J. Herpetol. 14, 239–244. doi: 10.2307/1563545
Pruett, J. E., Addis, E. A., and Warner, D. A. (2019). The influence of maternal nesting behaviour on offspring survival: evidence from correlational and cross-fostering studies. Anim. Behav. 153, 15–24. doi: 10.1016/j.anbehav.2019.04.010
Quinn, D. P., Kaylor, S. M., Norton, T. M., and Buhlmann, K. A. (2015). Nesting mounds with protective boxes and an electric wire as tools to mitigate Diamond-backed Terrapin (Malaclemys terrapin) nest predation. Herpetol. Conserv. Biol. 10, 969–977.
Rahman, S., and Burke, R. L. (2010). “Evaluating Nest Protectors for Turtle Conservation,” in Final Reports of the Tibor T. Polgar Fellowship Program, 2009, Section VI Edn, eds D. J. Yozzo, S. H. Fernald, and H. Andreyko (New York, NY: Hudson River Foundation), 1–23.
Riley, J. L., and Litzgus, J. D. (2014). Cues used by predators to detect freshwater turtle nests may persist late into incubation. Can. Field Nat. 128, 179–188. doi: 10.22621/cfn.v128i2.1583
Robinson, C., and Bider, J. R. (1988). Nesting synchrony - A strategy to decrease predation of snapping turtle (Chelydra serpentina) nests. J. Herpetol. 22, 470–473. doi: 10.2307/1564342
Ross, D. A., and Anderson, R. K. (1990). Habitat use, movements, and nesting of Emydoidea blandingi in central Wisconsin. J. Herpetol. 24, 6–12. doi: 10.2307/1564283
Rowe, J. W., Coval, K. A., and Dugan, M. R. (2005). Nest placement, nest-site fidelity and nesting movements in midland painted turtles (Chrysemys picta marginata) on Beaver Island, Michigan. Am. Midl. Nat. 154, 383–397. doi: 10.1674/0003-0031(2005)154[0383:npnfan]2.0.co;2
Rutherford, J. L., Casper, G. S., and Graves, B. (2016). Factors affecting predation on Wood Turtle (Glyptemys insculpta) nests in the Upper Peninsula of Michigan. Chelonian Conserv. Biol. 15, 173–186.
Ruzicka, R. E., and Conover, M. R. (2011). Influence of wind and humidity on foraging behavior of olfactory mesopredators. Can. Field Nat. 125, 132–139. doi: 10.22621/cfn.v125i2.1196
Schwarzkopf, L., and Brooks, R. J. (1987). Nest-site selection and offspring sex ratio in painted turtles, Chrysemys picta. Copeia 1987, 53–61. doi: 10.2307/1446037
Shealy, R. M. (1976). The natural history of the Alabama map turtle, Graptemys pulchra Baur, in Alabama. Bull. Flor. Stat. Mus. Biol. Sci. 21, 47–111.
Snow, J. E. (1982). Predation on painted turtle nests: nest survival as a function of age. Can. J. Zool. 60, 3290–3292. doi: 10.1016/s0531-5565(03)00106-2
Spencer, R.-J. (2002). Experimentally testing nest site selection: fitness trade-offs and predation risk in turtles. Ecology 83, 2136–2144. doi: 10.1890/0012-9658(2002)083[2136:etnssf]2.0.co;2
Spencer, R.-J., and Thompson, M. B. (2003). The significance of predation in nest site selection of turtles: an experimental consideration of macro- and microhabitat preferences. Oikos 102, 592–600. doi: 10.1034/j.1600-0706.2003.12436.x
Spencer, R.-J., and Thompson, M. B. (2005). Experimental analysis of the impact of foxes on freshwater turtle populations. Conserv. Biol. 19, 845–854. doi: 10.1111/j.1523-1739.2005.00487.x
Standing, K. L., Herman, T. B., and Morrison, I. P. (1999). Nesting ecology of Blanding’s turtle (Emydoidea blandingii) in Nova Scotia, the northeastern limit of the species’ range. Can. J. Zool. 77, 1609–1614. doi: 10.1139/cjz-77-10-1609
Strickland, J., Colbert, P., and Janzen, F. J. (2010). Experimental analysis of effects of markers and habitat structure on predation of turtle nests. J. Herpetol. 44, 467–470. doi: 10.1670/08-323.1
Temple, S. A. (1987). Predation on turtle nests increases near ecological edges. Copeia 1987, 250–252. doi: 10.2307/1446069
Tinkle, D. W., Congdon, J. D., and Rosen, P. C. (1981). Nesting frequency and success: implications for the demography of painted turtles. Ecology 62, 1426–1432. doi: 10.2307/1941498
Tuma, M. W. (2006). Range, habitat use, and seasonal activity of the yellow mud turtle (Kinosternon flavescens) in northwestern Illinois: implications for site-specific conservation and management. Chelonian Conserv. Biol. 5, 108–120. doi: 10.2744/1071-8443(2006)5[108:rhuasa]2.0.co;2
Vilardell, A., Capalleras, X., Budó, J., Molist, F., and Pons, P. (2008). Test of the efficacy of two chemical repellants in the control of Hermann’s Tortoise nest predation. Eur. J. Wildl. Res. 54, 745–748. doi: 10.1007/s10344-008-0176-9
Vogt, R. C. (1980). Natural history of the map turtles Graptemys pseudogeographica and Graptemys ouachitensis in Wisconsin. Tulane Stud. Zool. Bot. 22, 17–48.
Voves, K. C., Mitchell, T. S., and Janzen, F. J. (2016). Does natural nest camouflage reduce turtle nest predation? Am. Midl. Nat. 176, 166–172. doi: 10.1674/0003-0031-176.1.166
Wilhoft, D. C., Del Baglivo, M. G., and Del Baglivo, M. D. (1979). Observations on mammalian predation of snapping turtle nests (Reptilia. Testudines, Chelydridae). J. Herpetol. 13, 435–438. doi: 10.2307/1563478
Wilson, D. (1998). Nest-site selection: microhabitat variation and its effects on the survival of turtle embryos. Ecology 79, 1884–1892. doi: 10.1890/0012-9658(1998)079[1884:nssmva]2.0.co;2
Wirsing, A. J., Phillips, J. R., Obbard, M. E., and Murray, D. L. (2012). Incidental nest predation in freshwater turtles: inter- and intraspecific differences in vulnerability are explained by relative crypsis. Oecologia 168, 977–988. doi: 10.1007/s00442-011-2158-y
Wuebbles, D. J., Easterling, D. R., Hayhoe, K., Knutson, T., Kopp, R. E., Kossin, J. P., et al. (2017). “Our globally changing climate,” in Climate Science Special Report: Fourth National Climate Assessment, Vol. 1, eds D. J. Wuebbles, D. W. Fahey, K. A. Hibbard, D. J. Dokken, B. C. Stewart, and T. K. Maycock (Washington, D.C: U.S. Global Change Research Program), 35–72. doi: 10.7930/J08S4N35
Keywords: Reptilia, Testudines, freshwater turtles, Procyon lotor, raccoon, sensory cues, nest predation
Citation: Geller GA and Parker SL (2022) What Are the Primary Cues Used by Mammalian Predators to Locate Freshwater Turtle Nests? A Critical Review of the Evidence. Front. Ecol. Evol. 9:784786. doi: 10.3389/fevo.2021.784786
Received: 28 September 2021; Accepted: 19 November 2021;
Published: 20 January 2022.
Edited by:
Jeanine M. Refsnider, University of Toledo, United StatesReviewed by:
Tom Langen, Clarkson University, United StatesEdward Narayan, The University of Queensland, Australia
Jenna Pruett, Auburn University, United States
Copyright © 2022 Geller and Parker. This is an open-access article distributed under the terms of the Creative Commons Attribution License (CC BY). The use, distribution or reproduction in other forums is permitted, provided the original author(s) and the copyright owner(s) are credited and that the original publication in this journal is cited, in accordance with accepted academic practice. No use, distribution or reproduction is permitted which does not comply with these terms.
*Correspondence: Gregory A. Geller, ggeller54@gmail.com