- Wildlife Institute of India, Dehradun, India
The woolly wolf Canis lupus chanco is increasingly being accepted as a unique taxon that needs immediate protection and management; however, information on its ecology remains limited across its range. We used camera trapping data set of 4 years (2015–2019) to investigate seasonal activity patterns and space use and assessed woolly wolf food habits in the Gangotri National Park, western Himalaya, India. We used generalized linear mixed models to assess the distribution of the wolf about prey, seasonal livestock grazing, human presence, habitat, and seasons. We observed a positive association with elevation and a negative response to an increase in ruggedness. The capture of wolves increased in winters, indicating a possible effect of snow on the ranging pattern. Spatial avoidance to anthropogenic pressure was not evident in our study; however, temporal avoidance was observed. The activity pattern of the wolf varied among seasons. Wolves were mostly active in the morning and late evening hours in summer and showed a diurnal activity pattern in winter. A less diverse diet was observed where the mean percentage frequency of occurrence and relative biomass was highest for bharal, followed by livestock. Himalayan marmot Marmota himalayana, birds, and rodents also form minor constituents to the diet. Synthesizing all three factors (space, diet, and activity), it may be stated that the wolf presence in the region is influenced by both wild prey availability and seasonality. Therefore, conservation of woolly wolves would require securing a vast landscape with optimal wild prey.
Introduction
The status of large carnivores is declining across the globe (Ripple et al., 2014), with a negative impact on the structure and functioning of ecosystems (Estes et al., 2011). They influence ecosystems with “top-down” control, which helps to balance the effects of environmental or “bottom-up” factors, such as primary productivity or climate (Ripple et al., 2016). Due to high metabolic demands, these carnivores often require large prey and expansive habitats (Ripple et al., 2014). However, such requirements often conflict with humans and livestock, making them the most controversial and challenging species to conserve (Noss et al., 1996; Chapron et al., 2014; Lute et al., 2018). Wolves are typical examples of carnivores that have suffered and been extirpated from much of their range (Reynolds and Tapper, 1996; Laliberte and Ripple, 2004; Musiani et al., 2005; Newsome et al., 2016). As a result, in many places, wolves have become mostly restricted to remote areas with low human presence (Paquet and Carbyn, 2003; Chapron et al., 2014; López-Bao et al., 2015).
The woolly wolf of the Himalaya has been proposed to be listed as a subspecies of gray wolf (Joshi et al., 2020), which needs research and conservation attention (Aggarwal et al., 2003; Lyngdoh, 2020). The woolly wolf is an apex flagship predator of the high elevation ecosystem of the Himalayan region, whose role is intricately linked with the ecology of the region (Sharma et al., 2007). However, the status of the woolly wolf in the region is decreasing due to persecution (Namgail et al., 2007; Kaczensky et al., 2008) and declines in prey species (Werhahn et al., 2019). Although the concept of woolly wolves as a unique taxon is gaining support through scientific evidence (Sharma et al., 2004; Aggarwal et al., 2007; Werhahn et al., 2019), its ecology remains poorly explored (Werhahn et al., 2019). One of the earliest attempts to understand its ecology in the Ladakh and Spiti regions recorded extremely low abundance in these areas (Fox and Chundawat, 1995). Subsequently, research in other areas resulted in new distribution records, including Uttarakhand and Sikkim states in India and Nepal (Jackson et al., 1996; Bhattacharya and Sathyakumar, 2010; Chanchani et al., 2011; Habib et al., 2013; Maheshwari et al., 2013). Despite an early start to understand its ecology, most studies so far have used indirect accounts (except Lyngdoh, 2020) such as interviews, signs and, scats to understand habitat requirements (Subba, 2012; Chetri et al., 2017; Werhahn, 2020), and conflict issues (Subba, 2012; Habib et al., 2013; Suryawanshi et al., 2013; Chetri et al., 2020; Werhahn, 2020).
Woolly wolves inhabit cold, arid high- elevation areas of the western Himalaya in northern India to the eastern Himalaya in Nepal and the Tibetan Plateau in China. Most protected areas in the region are small, and wildlife populations mostly occur across the landscape often intermixed with human use (Mishra et al., 2010). Livestock grazing is the most predominant land use type in the region and is practiced pervasively across the landscape, including protected areas (Rawat, 2007). The high livestock density and associated declines in wild prey apparently have a cascading effect of intensifying the conflict between humans and large carnivores over livestock depredation (Mishra et al., 2010; Suryawanshi et al., 2013). Other than anthropogenic influences, topography and seasonal habitat conditions are also expected to influence the habitat use of wolves (Lyngdoh, 2020). The high elevation habitat exposes species to constraints imposed by extremely low temperatures such as long, variable snow and ice loading periods and limited food availability (Sandercock et al., 2005). Some preys and co-occurring species of the woolly wolf respond to these changes by migrating [e.g., chiru (Schaller, 1998) or entering hibernation (e.g., marmot and Himalayan brown bear)]. During winter, the presence of herders and livestock is mostly absent or reduced in the high-elevation region of the Himalaya (Rawat, 2007). The presence of snow also influences animal behavior (Moriarty et al., 2015), physiology (Shipley et al., 2019), and movement (Martin et al., 2020). In the case of the woolly wolf, relationships between space use and other aspects of landscape pattern, such as how they respond to changes in habitat conditions caused by the seasonal presence of snow and grazing practices, have not been well studied.
We examined the space use and diet of the Woolly wolves in Gangotri National Park, Uttarakhand state in the western Himalayan region, to evaluate the relative influence of abiotic (topography and season) and biotic (prey and anthropogenic pressure) factors on their spatial ecology. To understand the spatial use, we collected occurrence data of wolves using camera traps along the elevation gradient of 3,000 to 5,000 m in two seasons (summer and winter) over multiple sessions (November 2015 –March 2019). Based on previous studies, we hypothesized that topography (rugged or steep slopes) would influence the space use of the wolves (Jumabay-Uulu et al., 2014; Lyngdoh, 2020). We expected to see high seasonality in wolf space use due to the absence of humans and livestock and the presence of snow cover in the winter. Such seasonal disturbance and changes in temperature are also expected to influence the activity patterns of the species (Nielsen, 1984; Patterson et al., 1999). Previous studies on wolves have suggested the influence of humans (Vilà et al., 1995; Ciucci et al., 1997; Kusak et al., 2005) and day heat (Theuerkauf, 2009) on activity patterns. Thus, to understand the influence of seasonal changes and anthropogenic pressures on the activity pattern of the woolly wolf in Gangotri National Park, we investigated seasonal activity patterns and their overlap with anthropogenic disturbances (records of people, livestock, and domestic dogs). Furthermore, we described the food habits of the woolly wolf in the study area to understand their dietary requirements. The study contributes vital information on less studied woolly wolf distribution and resource use for designing effective land sharing and conservation planning in the Gangotri landscape.
Materials and Methods
Study Area
The Gangotri National Park (2,390 km2) located in Uttarkashi district of the Uttarakhand State, India, covers a continuous zone of the greater and trans-Himalayan region of the western Himalaya. The study was carried out in a park in the trans-Himalayan valley (Nelang) located between 31°00′ 44.1″–31°27′06.26″ latitudes and 78°53′39″–79°15y″ E longitude (Figure 1). Spread over about 1,360 km2, it forms the catchment area of the Jadh Ganga river and its tributaries. This area represents the southeastern extension range of Zanskar and is a collision zone between the Asian and Indian plates lying immediately north of the Main Central Thrust (Chandola, 2008). The Nelang valley exhibits geobotanical affinity to the trans-Himalaya (Kumar et al., 2017). Nearly 25% of the Nelang valley is (southwestern part) is extremely rugged and steep in the form of a deep canyon formed by the Jadh Ganga river. The northern parts are characterized by gentle slopes and plateau. The lower part of the valley (3,000–3,500 m) supports a temperate forest comprising mainly of blue pine Pinus wallichiana. Mid elevation (3,500–3,800 m) sees a gradual change from blue pine to open juniper and alpine scrub comprising species such as Artemisia spp., Juniperus spp., Cotoneaster spp., and Lonicera hypoleuca. Beyond 4,000 m, the valleys and undulating slopes exhibit steppe vegetation characteristics dominated by L. spinosa and Caragana versicolor. Dataloggers (HOBO U23 Pro V2, accuracy level ± 0.2°C) deployed in the Nelang valley (∼4,000 m) have documented (2018) a mean annual maximum of 11.6°C and a minimum of 4.6°C temperature, and the temperature ranges between a maximum of 28.9°C to a minimum of − 26.5°C. The maximum snowfall occurs from December to March. From 2000 to 2008, the average winter snowfall was ∼546 mm (2000–2008, Bhambri et al., 2011). Snow leopard (Panthera uncia) and woolly wolf are the large predators found in the area. Potential wild prey species include Himalayan blue sheep or bharal (Pseudois nayaur), Himalayan marmot (Marmota himalayana), and Tibetan woolly hare (Lepus oiostolus). Galliformes species reported in the Nelang valley include Himalayan snowcock (Tetraogallus himalayensis), chukar partridge (Alectoris chukar), Tibetan snowcock (Tetraogallus tibetanus), and Tibetan sandgrouse (Syrrhaptes tibetanus). Tibetan argali (Ovis ammon) is rare in the region (Chandola, 2008; Pal et al., 2018). Human presence in the area includes seasonal livestock grazing (∼30,000), border personnel, and seasonal laborers for the construction and maintenance activities related to infrastructure development (Chandola, 2008). A previous analysis of seasonal anthropogenic pressure in the study area showed a low presence of humans and associated activities in winters compared with summer (Pal et al., 2020).
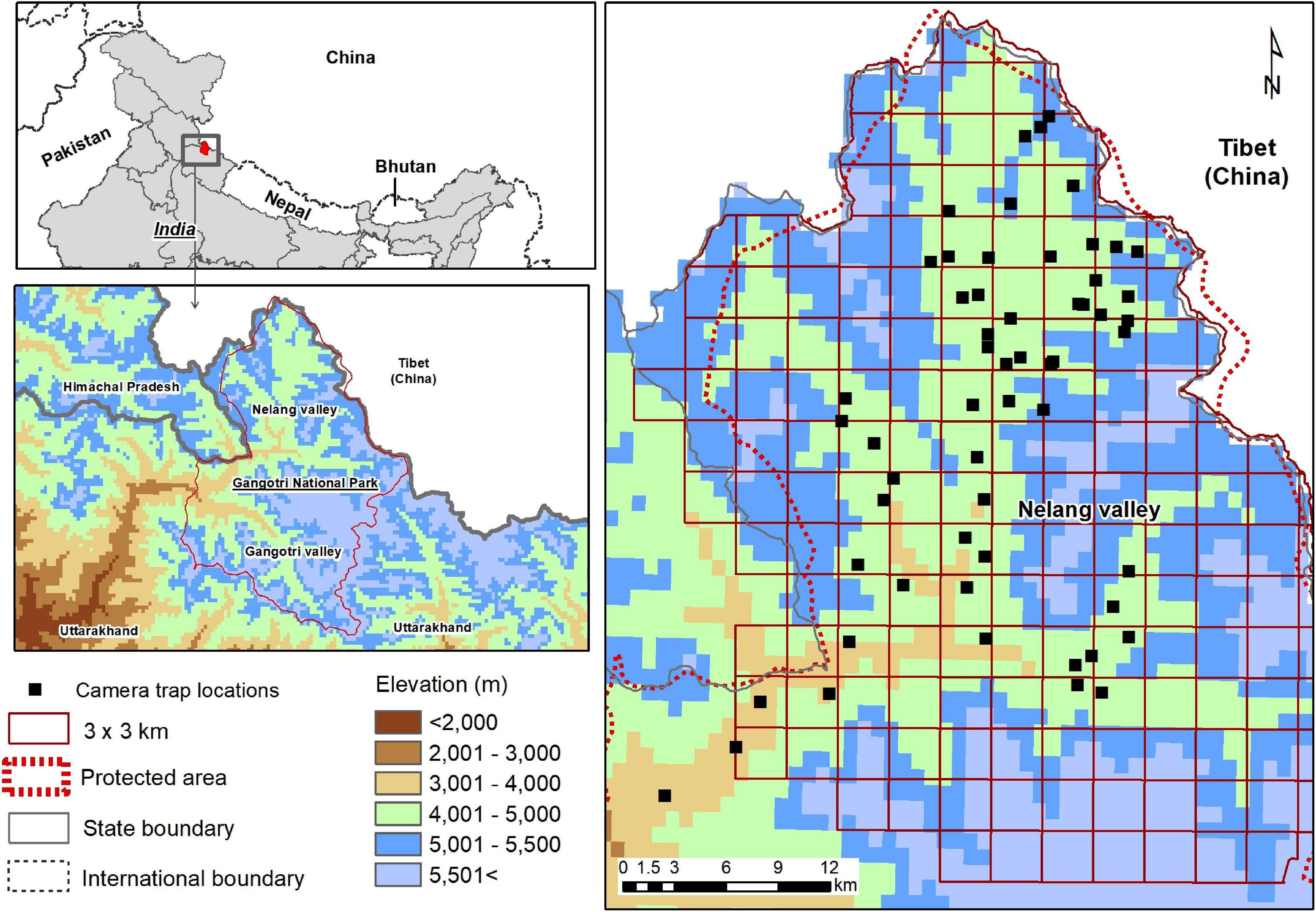
Figure 1. Locations of camera traps in the Nelang valley of the Gangotri National Park, Uttarakhand, India.
Method
Space Use
Camera trapping was carried out from October 2015 to March 2019, focusing on two seasons: summer (May to September) and winter (November to March) (Table 1). The duration for the session was selected such that the conditions in terms of anthropogenic disturbance and season remained the same. Human presence (grazing, construction, and paramilitary) increases in summer from May to September. Conversely, livestock grazing is absent, and the area has snow cover from November to March. Cuddeback C1 Cuddeback, De Pere, WI, USA camera traps were deployed to document the seasonal presence of large mammals along an elevation gradient of 3,000–5,000 m. In the initial 2 years (October 2015 to April 2017), camera traps were deployed using 4 km × 4 km grids. From May 2017 to March 2019, the sampling intensity was increased using 3 km × 3 km grids. The mean spacing between camera traps across the session was 2.38 km (SD: 1.77). At each site, camera traps were deployed (affixed to a pile of stones) in locations likely to be used by mammals based on signs such as scats and pugmark/pellets, at a height of c. 30–45 cm above the ground. Each camera site could be visited only once per season due to logistic constraints.
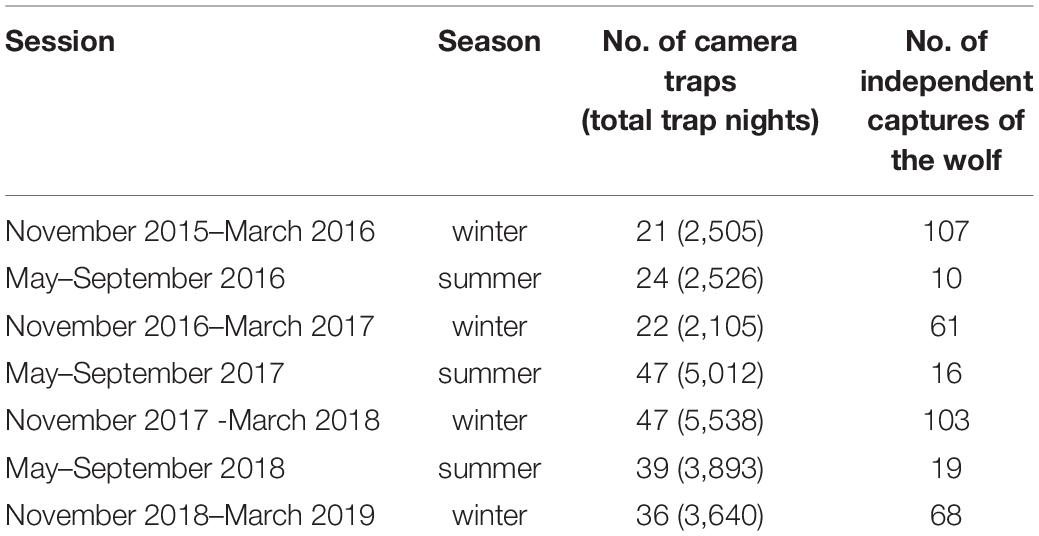
Table 1. Summary of seven camera trapping sessions (2015–2019), conducted in the Nelang valley of the Gangotri National Park, Uttarakhand, India: the year of the survey, season, number of camera stations with a number of trap nights, number of the photographs of the independent wolves (multiple captures of the same individual within 30 min at a camera site were excluded).
We examined the effect of habitat and human disturbance using generalized linear mixed models, using the glmmTMB package1 (Magnusson et al., 2017) in R 3.6.2 (R Core Team, 2019). Some of the grid cells had more than one camera location. Therefore, we tested for spatial autocorrelation among sampled locations, using the weighted correlation coefficient of Moran (Moran’s I) in ArcGIS 10.4 (Esri, Redlands, United States). We used camera trap data from repeated samplings at the same sites (summer and winter) and incorporated sites as a random effect variable. We used captures of woolly wolves as the response variable and the number of trap days (log-transformed) as an offset to account for variation in the trapping effort between sites (Table 2). Capture rates of prey species, humans, and free-ranging dogs were calculated as independent capture events for these variables at each camera location divided by the number of trap nights that the cameras at that location were operational, multiplied by 100 (Bashir et al., 2014). The potential prey species were grouped into three categories: (i) bharal (the only large wild prey in the area), (ii) livestock, and (iii) marmot, the woolly hare is rare in the area; hence, they were grouped as small prey species (Table 2). Habitat features, potential prey species, and humans and dogs were used as fixed predictor variables (Table 2). Continuous variables were z-standardized before the analysis. We tested for the presence of overdispersion in the dataset and selected the negative binomial distribution (i.e., Poisson and negative binomial). We also evaluated the data for zero inflation. We used Akaike’s information criterion (AICc) adjusted for the sample size to rank models and considered models with ΔAICc values < 2 to have equivalent support to the top-ranked models (Burnham and Anderson, 2002). We applied model averaging and calculated a model-averaged parameter estimate and conditional standard error for each parameter. To examine any multicollinearity between predictor variables, we performed a Pearson correlation test. Correlated variables (Pearson correlation coefficient > 0.7) were not used in the same model.
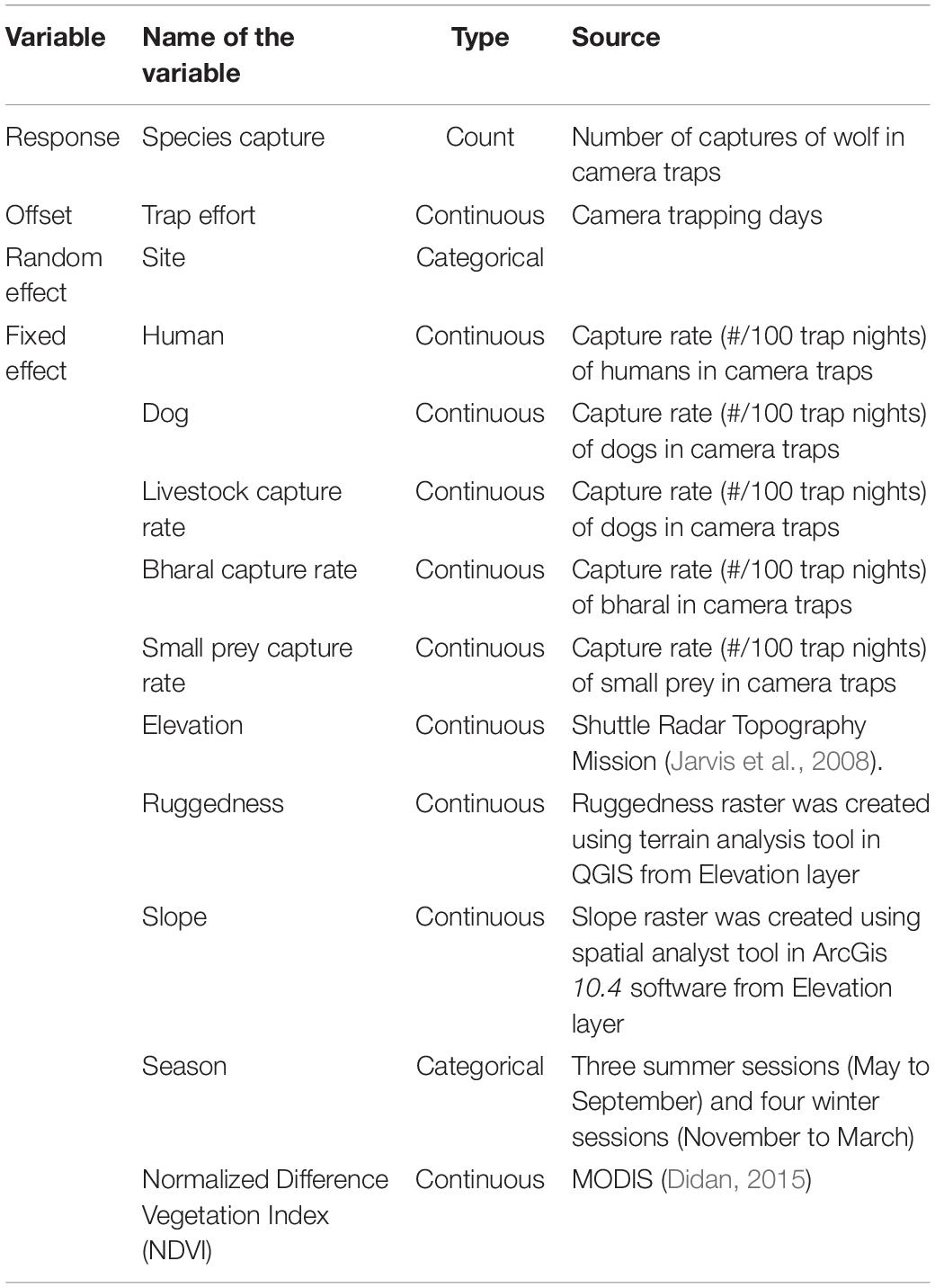
Table 2. Description of the variables used in the generalized linear mixed models used to understand the response of the woolly wolf to habitat and anthropogenic factors in the Nelang valley of the Gangotri National Park, Uttarakhand, India.
Activity Pattern
Based on the time stamp on the camera trap images, we assessed the activity pattern of wolves, humans, dogs, and livestock in the summer and winter seasons. Additionally, we assessed the interspecific temporal interaction by estimating the temporal overlap between species. Camera trap captures of species at a site were considered as independent records at 30 min. All the independent captures of the species in different years were pooled according to the winter and summer seasons to establish the overall activity pattern for the species. The time of captures of species was first converted into radians to account for the circular distribution of the time of the day (Meredith and Ridout, 2014). Subsequently, a non-parametric kernel density function was used to estimate the daily activity patterns of the species. To assess the activity overlaps, we calculated the coefficients of overlap (Δ) in a pair-wise manner for each season. The Δ ranges from 0 (no overlap) to 1 (complete overlap) and indicates the extent of overlap between two kernel density estimates (i.e., daily activity patterns of the two species compared) (Ridout and Linkie, 2009). We used the overlap coefficient Δ1 as recommended by Ridout and Linkie (2009) in cases of low sample sizes. Temporal analysis was performed using the overlap package (Meredith and Ridout, 2014) in program R 3.6.2 (R Core Team, 2019).
Food Habits
For food habits, scats encountered during camera surveys, once every season from June 2015 to July 2019, were opportunistically collected for investigating food habits. Scat samples were identified in the field according to size and appearance. These were later verified using mtDNA analysis, as field misclassification can be high (Jumabay-Uulu et al., 2014; Weiskopf et al., 2016). Scats were oven-dried at 40°c until the moisture was not observed in the middle of the sample (Piggott and Taylor, 2003). After drying the samples, only the upper mucosal layer of scat was scraped, and < 0.2 g was taken for genetic analysis. The remaining part was kept for food habit analysis at room temperature with silica (silica:scat = 4:1) in plastic bags. DNA was extracted using a QIAamp DNA stool kit (QIAGEN, Germany), following the Qiagen manual with slight modifications. Negative control was also run with the samples to check exogenous DNA contamination.
For the amplification of mtDNA carnivore-specific primers 146 bp long cytochrome b gene, F 5′-TATTCTTTATCTGCCT ATACATRCACG-3′; R 5′-AAACTGCAGCCCCTCAGAATGAT ATTTGTCCTCA-3′ (Farrell et al., 2000); 144 bp long ATP6-DF3; 5′-AACGAAAATCTATTCGCCTCT-3′; DR1, 5′-CCAGTATT TGTTTTGATGTTAGTTG-3′ (Chaves et al., 2012); and 12S rRNA, namely, F 5′-AAAAAGCTTCAAACTGGGATTAGATA CCCCACTAT-3′ and R 5′-TGACTGCAGAGGGTGACGGGC GGTGTGT-3′ (Kocher et al., 1989) were used. These primers are known to maximize efficiency even in degraded samples. DNA was amplified in 10 μl reaction mixture containing 10 × PCR buffer that includes 20 mM MgCl2, 20 mg/ml BSA, 2 mM (each) dNTPs mix, 0.05 μmol primer, 5 U/μl Taq DNA polymerase (Thermo Fisher Scientific, Lithuania), and distilled water. PCR conditions were followed by initial denaturation at 95°C for 3 min, 40 cycles of denaturation at 95°C for 30 s, annealing at 52°C (cytb), 55°C (ATP6) and 60°C (12s rRNA) for 45 s, 72°C for 55, and final extension for 10 min at 72°C. Sequencing of amplified PCR products was first performed using exonuclease-I and shrimp alkaline phosphatase (Thermo-Scientific Inc.) for the removal of free dNTPs and primer residues. The forward primer of each fragment was used for the BigDye version 3.1 kit cycle sequencing. Later, the fragments were washed using sodium acetate to precipitate the DNA, followed by chilled alcohol. Then, the clean desired DNA fragments were sequenced using Applied BioSystem Bio-Analyzer 3500 XL. Subsequently, the obtained sequence was examined by searching BLAST (NCBI, MD, United States) to identify the closest homologous similarity of the unknown sequence. DNA samples that showed the highest similarity with reference sequences (NCBI) were considered distinguishable in terms of species identification.
The scats were washed in running water through a fine sieve of 125 μm, ensuring that no digested material could pass through the sieve. Indigestible items (hair, feathers, bones, claws, teeth, chitin remnants of insects, seeds, grasses, and other plant materials) and human-derived materials (cloth, paper, plastic, and rubber) were collected for further identification. After drying the derived hair and feather samples in an oven at 40°C for 48 h, the hair was washed and water mounted to observe under a microscope (40× or 100×). Samples of 20 hairs from each scat were randomly drawn for analysis (Jethva and Jhala, 2003). Reference hair samples for wild prey species were collected from carcasses encountered during the fieldwork. The species for which reference samples could not be collected in the field were identified using the database of Wildlife Institute of India (Bahuguna et al., 2010) and published reference keys (Oli, 1993). Features such as color, length, thickness, characteristic medullar configurations, cortex-to-medulla ratio, and cuticle pattern (Reynolds and Aebischer, 1991; Mukherjee et al., 1994) were used for the identification of prey. Some of the rodent species (except Himalayan marmot) could not be identified as species and were placed in a broad category as “small prey.” The utilization rate of different food items was calculated using the frequency of occurrence (FO) per item as F = n/N, where n is the number of scats with a particular species and N is the total number of scats analyzed (Karanth and Sunquist, 1995; Bashir et al., 2014). We subjected the results of the occurrence analysis to resampling using the bootstrap method. Subsamples equaling the original sample size of scats were iterated 10,000 times to generate means and bias-corrected 95% confidence intervals for percentage frequency of prey items in scats (Mukherjee et al., 2004). Utilization indices, in particular FO, tend to underestimate the share of big prey compared with the small ones and can be misleading when prey greatly differ in size (Klare et al., 2011). Therefore, the frequency of occurrence of prey species in the scats was converted to relative biomass (Karanth and Sunquist, 1995; Bashir et al., 2014) as this provides the best approximation of the actual food habits (Klare et al., 2011). For the biomass model calculation, we used the relative frequency as the equivalent of relative volume and used the latter in the biomass calculation model developed for the gray wolf based on feeding trials (Floyd et al., 1978; Weaver, 1993). To estimate food ingested per prey species, we used the biomass calculation suggested by Weaver (1993). BioWeaver: Y = 0.439 + 0.008X, where Y is the biomass of prey consumed to produce a scat, and X is the average body weight of each prey species.
Results
The camera trapping effort of 25,219 trap nights resulted in 384 incidences of wolf captures. The capture rate (number of captures/100 trap days; 95% confidence interval) of wolves in summer and winter was 0.174 (0–0.52) and 2.55 (1.45–3.65), respectively. Capture rates of snow leopard and potential prey species are mentioned in Table 3. The presence of humans and dogs was higher in summer than in winter (Table 3). Livestock is present only in the summer season (Table 3).
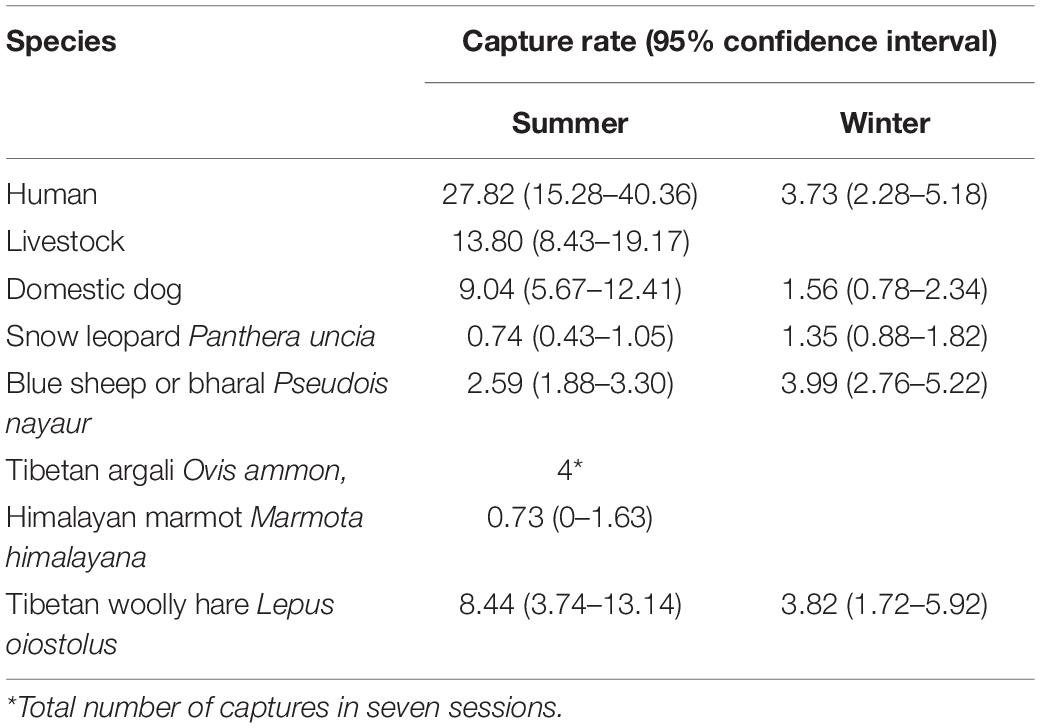
Table 3. Seasonal capture rate (#/100 trap nights) of humans, livestock, dogs, snow leopard, and potential prey species of the woolly wolf in the Nelang valley of the Gangotri National Park, Uttarakhand, India.
For the spatial analysis, the correlation test using the Pearson’s test showed a significant correlation between the livestock and dogs (r = 0.7) and between ruggedness and slope (r = 0.95); therefore, these variables were not used together in the models. No spatial autocorrelation was detected among the sites (all Z-scores were between −1.96 and 1.96) except for the winter 2015 and 2016 sessions (Z-score > 1.96) (Table 4). This was caused by fewer cameras and few captures of wolves restricted to the northern part of the study area. Given the limited dataset, we did not remove these sessions from the analysis, as spatial autocorrelation only in two seasons is not likely to influence the overall analysis. We tested 27 biologically meaningful models (Table 5, Supplementary Table 1). The most supported model (ΔAICc values < 2) showed that the presence of woolly wolves is influenced by season, topographical variables, and prey (Figure 2). The probability of occurrence of woolly wolves was positively associated with the winter season (β = 1.96, 95% CI: 1.33–2.59) and higher elevations (β = 1.1, C.I: 0.3–1.90) but negatively associated with steep slopes (β = − 0.69, C.I: − 1.34 to − 0.04) (Figure 2). Even though bharal and NDVI were part of the top models (ΔAICc values < 2), the slope estimates of these variable overlapped with zero and their impact on the space use of wolves remain unclear (Table 6). The temporal activity pattern of wolves differed in summer and winter. In summer, wolves showed two distinct peaks at ∼6:00 a.m. and 8 p.m. In winter, wolves lacked a clear preference pattern but were mostly active during the day between 6 a.m. and 6 p.m. Temporal overlap analysis showed a considerable overlap (Δ1 ≥ 50) with humans, dogs, and livestock activity in the area (Figure 3).
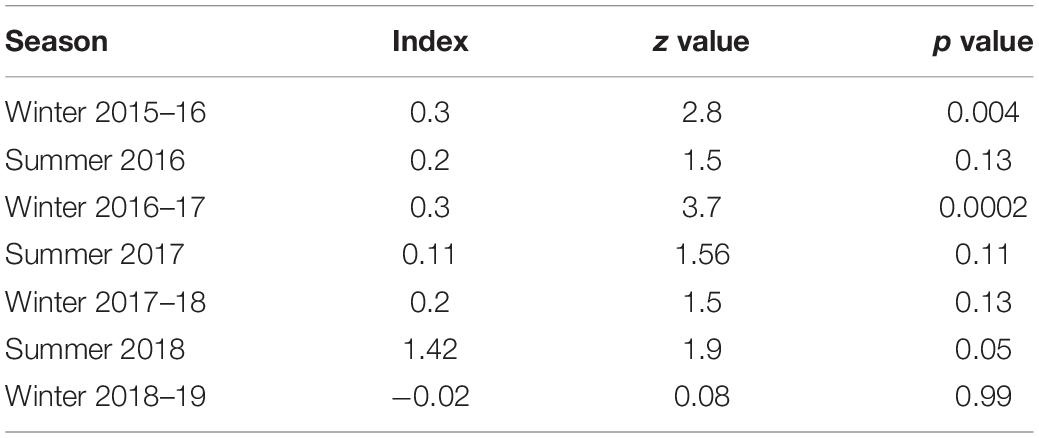
Table 4. Spatial autocorrelation (Moran’s I) test was used to examine whether camera-trap sites were independent across seven sampling sessions, 2015–2019, in the Nelang valley of the Gangotri National Park, Uttarakhand, India.
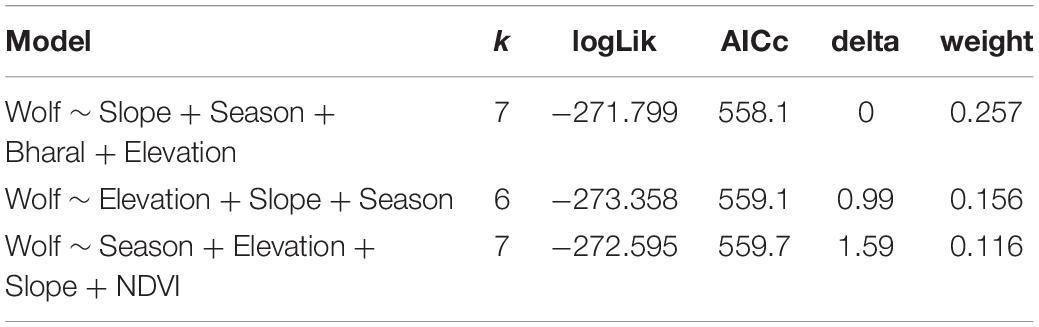
Table 5. Top three candidate models for examining the relationship between the relative abundance of the woolly wolf, habitat, season, and seasonal anthropogenic pressures in the Nelang valley of the Gangotri National Park, Uttarakhand, India, with Akaike information criterion of each model adjusted for small sample size (AICc), number of parameters (k), the difference in AICc from the best-performing model (ΔAICc), and Akaike weight.
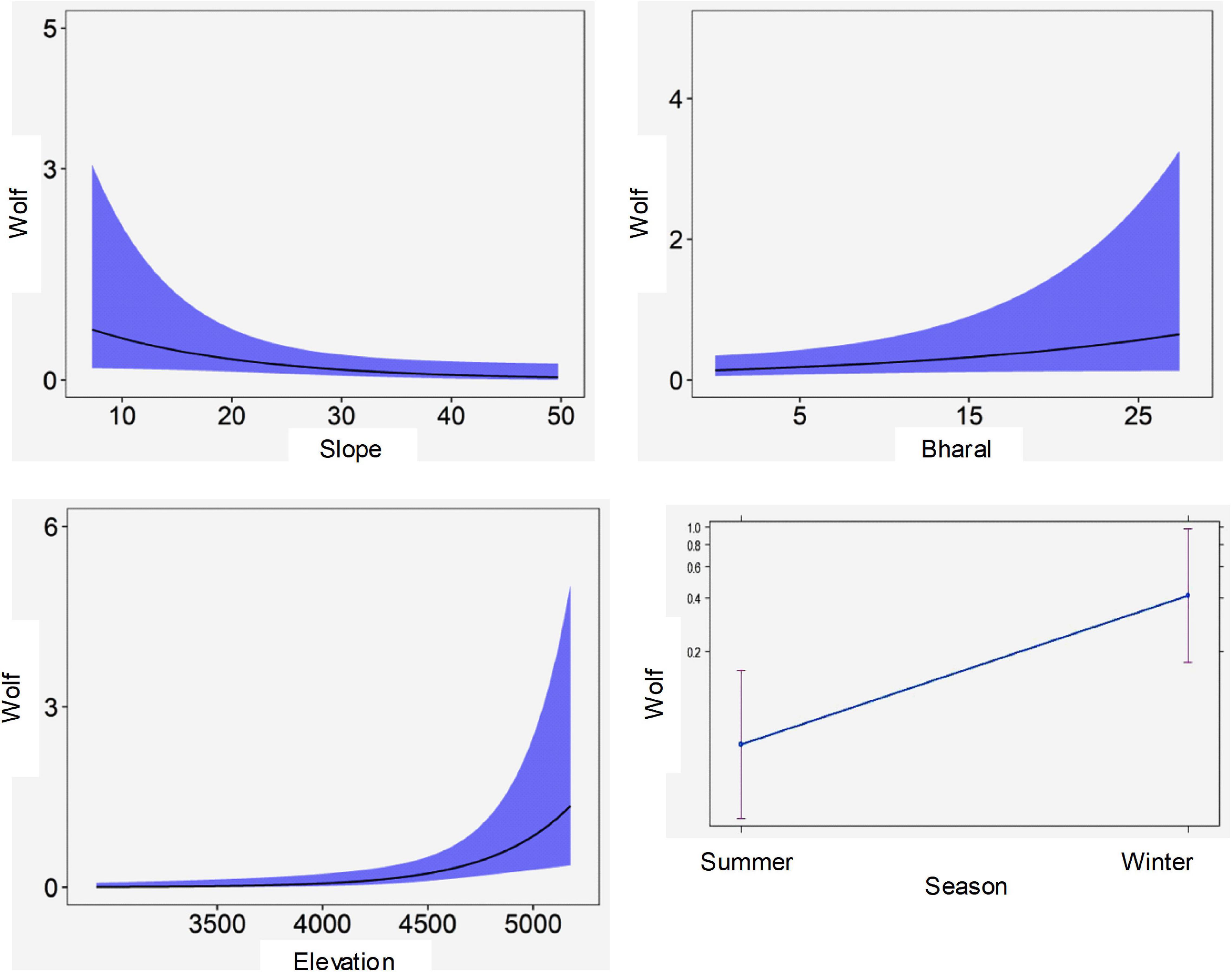
Figure 2. Predicted relationship between the woolly wolf capture rate and habitat variables: elevation, slope and season, and capture rate of prey species: bharal as determined by the generalized linear mixed models in the Nelang valley of the Gangotri National Park, Uttarakhand, India. The y-axis of each graph depicts the photo-capture rate and the x-axis depicts the variable value (elevation in m, slope in degree, bharal capture rate/100 days).
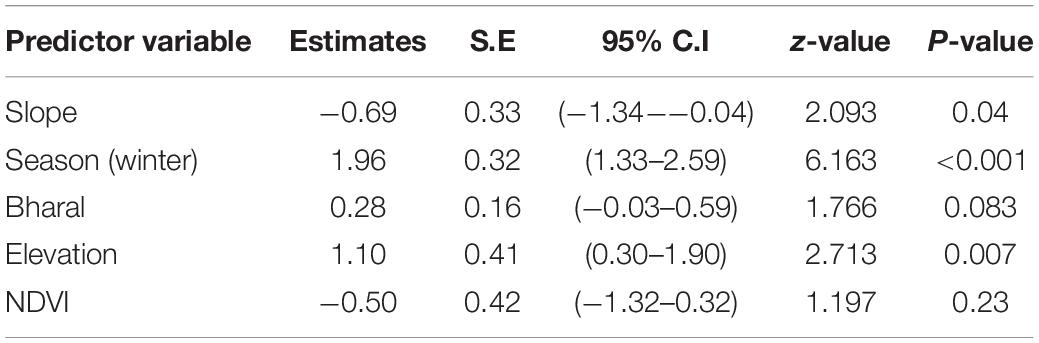
Table 6. Summary of fixed effect estimates of the average model for supported models (ΔAICc values < 2; wolf ∼ slope + season + bharal + elevation, wolf ∼ elevation + slope + season, wolf ∼ season + elevation + slope + NDVI) of the woolly wolf in the Nelang valley of the Gangotri National Park, Uttarakhand, India. The p-values < 0.05 indicate that the estimate is significant.
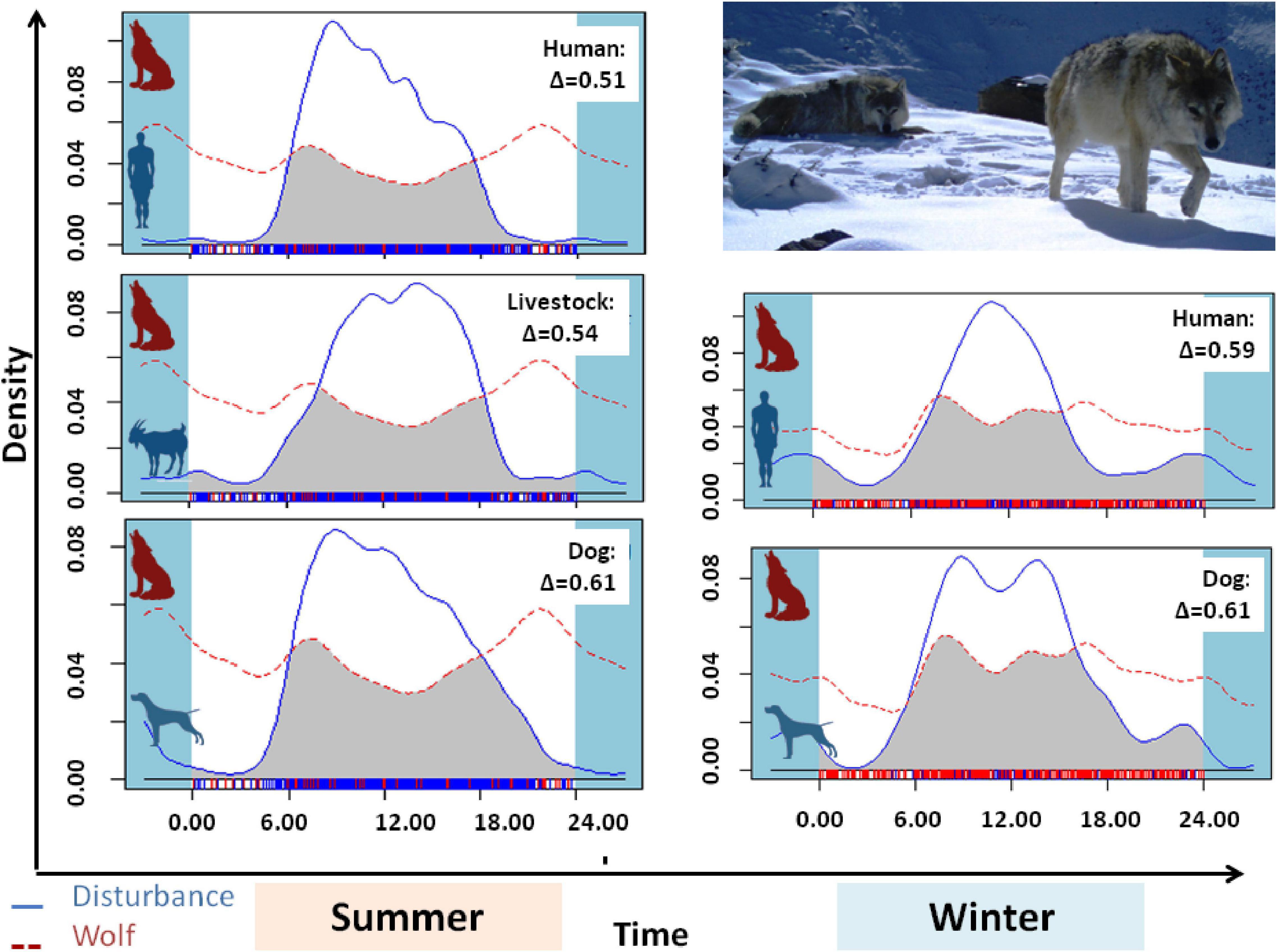
Figure 3. The activity overlap (gray area) and overlap coefficient (Δ) of anthropogenic disturbance (people, livestock, and domestic dogs) with woolly wolves in the summer and winter seasons in the Nelang valley of the Gangotri National Park, Uttarakhand, India. Original observations are displayed as a rug at the bottom of the plot.
For the food habit analysis, a total of 94 scats was collected from the Nelang valley. Genetic confirmation was achieved for 63 scats, out of which 32 were found to be of woolly wolves. Our field identification of wolf scats was to be 44.5% accurate and was confused with snow leopard scats (55.5%). The field identification was insufficient to confirm the scat identity of the woolly wolf, so we used only the genetically confirmed scats to study the food habit. Due to a limited number of scats, we could not study the seasonal food habit of the wolf, and all the scats were pooled to study the general information on the prey consumed. According to Trites and Joy (2005), a minimum of 59 scats is required to accurately describe the diet of species from a site. Our sample size of 32 scats is small to describe the dietary habits of wolves in the Nelang valley and results are to be considered as baseline data on the prey consumed by the woolly wolves in the Gangotri National Park. The undigested matter was present in decreasing order as follows: hair (100%, present in all scats), bone (81.3%), hoof (18.8%), claws (3.1%), feathers (9.8%), remains of grass (6.2%), and eggshells (6.2%). The mean percentage frequency of occurrence and relative biomass was highest for bharal followed by livestock, marmot M. himalayana, birds, and small prey (Table 7).
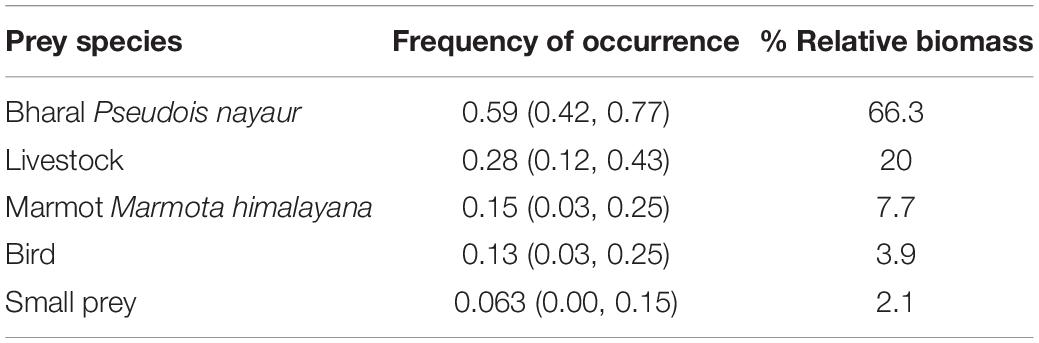
Table 7. Content of 32 woolly wolf scats in the Gangotri National Park, Uttarakhand, India, with the mean percentage frequency of prey items (with 95% confidence intervals, CI, from bootstrapping), estimated% relative biomass of the prey consumed.
Discussion
The primary space use of any animal is determined by obtaining enough food resources for survival and secure successful reproduction (White, 1978). Additionally, for carnivores, population density (Ballard et al., 1987), interterritorial competition (Hayes and Harestad, 2000), migration of prey (Mech and Boitani, 2003), landscape features correlated with hunting success (Rich et al., 2012), and anthropogenic influence (Rich et al., 2012) may play a crucial role in modulating space use in a landscape.
Our results suggest that woolly wolves prefer high elevation, less steep plateau habitats. Most of the wolf presence records in the Himalaya have appeared from the trans-Himalayan landscape (Sharma et al., 2004; Habib et al., 2013; Chetri et al., 2016) and rarely from the greater Himalayan regions (Bhattacharya and Sathyakumar, 2011). The positive association of wolves with grassland and plateau habitats that we observed has been found throughout the range of the woolly wolf (Subba, 2012; Habib et al., 2013; Rana, 2018). The effects of season, migratory prey, and anthropogenic factors on the size and configuration of home ranges have been extensively studied on other wolf species in Nearctic and Palearctic zones (Fuller, 1989; Theuerkauf et al., 2003a; Kaartinen et al., 2005; Mattisson et al., 2013). In these studies, the wolf space use was determined by both humans and prey density (Ballard et al., 1997). Wolves adapt large home ranges to accommodate seasonal variation in resource availability (Hinton et al., 2016) or to encompass retreat areas large and secluded enough from human activity (Mancinelli et al., 2018) and traveled less when prey was abundant (Jędrzejewski et al., 2002; Kusak et al., 2005). A recent study in the Himalayan ecosystem suggested seasonality in residency and recursive ranges (Lyngdoh, 2020). Based on collaring data from three wolves, the study showed a reduced home range for one wolf in winter, whereas the other two showed an increase in range (Lyngdoh, 2020). Home range and movement of wolves in summer and winter showed different core areas probably influenced by snow depth and prey availability (Lyngdoh, 2020).
We found an increase in wolf captures inside the park in winters, indicating a similar possible effect of snow on the ranging pattern. Snow increases the cost of movement (Parker et al., 1984; Crête and Larivière, 2003), and animals adapt to such conditions by changing their travel speed and travel path (Droghini and Boutin, 2018). Additionally, snow increases the success rate of ungulate kills by wolves (Nelson and Mech, 1986; Fuller, 1991; Huggard, 1993). In the Gangotri National Park, wolves may be using these areas intensively in winters to gain easy access to their prime prey, bharal. Carcasses of a large number of bharal kills were observed after winter in the field (R Pal per observation). During summers, the wolves may be using higher elevations and near international borders areas (adjoining the Tibetan Plateau) where anthropogenic pressure is very less. Other contributing factors for more captures in winters could be reduced human and dog presence in the area; however, these were not significant in our study.
Although our sample size was small, results show that in the Gangotri National Park wolves are dependent on large ungulate species, which concur with previous findings (Lyngdoh and Habib, 2019) from the Himalayan region (but see Chetri et al., 2017). Small preys such as Himalayan marmot M. himalayana, birds, and rodents also form minor constituents to the food habit. The density of bharal in the Nelang valley is 0.64 SE 0.2 individuals/km2 (Pal et al., 2021), and it is an important year-round source of food for wolves inside the park. The other important prey source is livestock, which is available only for 4 months (June-September). Around 30,000 livestock visit the Nelang valley (∼1,100 sq km) in summer (Chandola, 2008). Such a high density is likely to increase the competition for forage and space for bharal, pushing them to marginal habitats (Mishra et al., 2002; Bhattacharya et al., 2020; Koetke et al., 2020). Consequently, an overabundance of livestock may increase the chance of wolf predation on livestock, which is also observed in other Himalayan landscapes (Werhahn et al., 2019; Lyngdoh, 2020). Such livestock depredation could escalate into conflicts with humans (Chetri et al., 2020), which is the primary reason for wolf extirpation in many areas. We recommend assessing the response of herders to wolf predation on livestock in the region, the understanding of which is currently lacking but is vital for developing appropriate management strategies. Our diet analysis of wolves only represents baseline information on the diet of wolves in the study area due to the low sample size. Additionally, the high seasonal difference in diet (Chetri et al., 2017) is expected due to the absence of livestock in winters and hibernation of prey species such as marmot. More research is needed to understand the full spectrum of the diet of wolves and seasonal prey requirements.
In this study, woolly wolves were most active during the early morning and late evening during summer. A considerable amount of temporal overlap was found between humans and associated activities with wolves, largely contributed by activity overlap during the early morning hours (∼6 a.m.). Wolves showed a higher peak in late evening hours, which could be a strategy to access livestock and avoid human presence (Theuerkauf, 2009). Additionally, the relatively higher summer temperatures may also have favored reduced activity of wolves in the middle of the day (Theuerkauf, 2009). Conversely, in winters, wolves did not show a clear preference and were mostly diurnal (Figure 3). Such a pattern could be because daytime heat avoidance is not required in winter. A similar pattern was observed in subarctic sites in Alaska and Finland, where wolves were diurnal in winter (data only for Alaska) and nocturnal in summer (Theuerkauf, 2009). Another contributing factor could be a comparatively less human presence in winter. Although, compared with summer, wolves showed a higher overlap with humans in winters, but human presence is limited (livestock grazing and construction activities are absent) and is less likely to be influenced by their presence. Predators are also known to synchronize their activity pattern with prey species (Theuerkauf et al., 2003b). Interestingly, wolf activity in winters correlated closely with bharal, showing a diurnal activity pattern (Pal et al., 2021). The temporal activity of wolves overlapped highest with dogs in both seasons.
The camera capture rates and the less diverse diet of wolves indicate the scarcity of other prey sources in the area. Studies from the Himalayan region have shown wolf preference for open rolling over cliff-dwelling ungulates (Chetri et al., 2017; Werhahn et al., 2019). However, potential prey species preferring rolling terrain, argali (Sathyakumar and Bhatnagar, 2002; Odonjavkhlan et al., 2021), are extremely rare in the region. Argali was photo captured only on four occasions in winters (Pal et al., 2018). Excessive grazing during summer and feral dogs are the main threats to the ungulates in the landscape (Chandola, 2008; Pal et al., 2020). Additionally, the area is going through changes due to border security infrastructural expansion (Chandola, 2008; Pal et al., 2020). Even though grazing is practiced only during summer, this is also the prime growing season for plants. Prior consumption of resources by livestock degrades the pastures and negatively impacts the ungulates (Bhattacharya and Sathyakumar, 2011; Koetke et al., 2020). Such a gradual and often unnoticed degradation of grasslands and subalpine scrublands could be the reason for the decline in ungulate diversity in the region (Bauer, 1990; Rawat, 2007; Bhattacharya and Sathyakumar, 2011).
Realizing the need for conservation and lack of ecological information, we examined the spatial ecology of the woolly wolf in the context of seasonality in habitat conditions and anthropogenic pressures. The woolly wolf favored less steep high elevation pastures. Tragically, these habitats are also the most convenient livestock grazing ground for pastoralists (Chetri et al., 2017; Bhattacharya et al., 2020); hence, wolves are often at the forefront to face negative consequences of grazing and human presence. The seasonal overabundance of livestock will inevitably cause predation of wolves on livestock, as indicated by food habit analysis in our study. The presence of dogs inside the park poses additional risks such as hybridization and disease transmission to the woolly wolves (Hennelly et al., 2015), and hence need management action. This study also highlighted the seasonality in the intensity of space use in the study area. In winter, increased captures could be due to intensive use of the study area due to restrictions on movements or easy access to prey due to heavy snow cover. Spatial avoidance to anthropogenic pressure was not evident in our study; however, temporal adaptation was observed. Temporal avoidance may also be due to avoiding comparatively higher temperatures during the middle of the day in summers. On synthesizing the use of space, food habits, and activity, it may be stated that both wild prey availability and seasonality influence the presence of wolves in the region. Consequently, the conservation of woolly wolves would require a vast landscape with optimal prey. The area has the potential to increase the status of prey (e.g., argali). Developing livestock grazing-free pockets is an effective solution for practicing grazing sustainably in alpine habitats and revives the prey base (Mishra et al., 2016). A similar approach can be adopted in the Gangotri National Park, accompanied by awareness programs, compensation programs, and support of the local communities (Mishra et al., 2017). Wolves were mostly captured near the northern boundary of the park, which forms an international boundary with China (Tibet) Supplementary Figure 1. Hence, any management or conservation initiative will require transboundary cooperation. Further conclusive understanding of wolves ranging behavior and variability of habitat selection will require monitoring movement using radio collar studies.
Data Availability Statement
The original contributions presented in the study are included in the article/Supplementary Material, further inquiries can be directed to the corresponding author/s.
Ethics Statement
Ethical review and approval was not required for the animal study because our study was non-invasive and so did not require clearance from the Institute Animal Ethics Committee.
Author Contributions
SS and RP: conception of study. RP: data collection. AP and SG: genetic analysis. RP and AP: analysis and writing. SS and SG: revisions. All authors contributed to the article and approved the submitted version.
Funding
This work was supported by the Department of Science and Technology (DST) [/SPLICE/CCP/NMSHE/TF-2/WII/2014(G) dated 26.08.2014].
Conflict of Interest
The authors declare that the research was conducted in the absence of any commercial or financial relationships that could be construed as a potential conflict of interest.
Publisher’s Note
All claims expressed in this article are solely those of the authors and do not necessarily represent those of their affiliated organizations, or those of the publisher, the editors and the reviewers. Any product that may be evaluated in this article, or claim that may be made by its manufacturer, is not guaranteed or endorsed by the publisher.
Acknowledgments
This work was part of a project initiated under the National Mission for Sustaining the Himalayan Ecosystem (NMSHE) Programme funded by the Department of Science and Technology (DST), Government of India. We would like to thank the Director and Dean, Wildlife Institute of India, for their guidance and support. We would like to thank D. V. S. Khati, Principal Chief Conservator of Forests and Chief Wildlife Warden, Uttarakhand, for granting us the research permission and Shrawan Kumar, N. B. Sharma, Deputy Director of the Gangotri National Park for providing necessary support and cooperation. We would like to thank Shagun Thakur, Shashank Arya, Naitik Patel, Manish Bhardwaj, Raagini Muddaiah, Vinod Rana, Uttam Rana, and Ram Naresh for their support in the fieldwork. We would also like to thank Anukul Nath and Raagini Muddaiah for proofreading the manuscript.
Supplementary Material
The Supplementary Material for this article can be found online at: https://www.frontiersin.org/articles/10.3389/fevo.2021.782339/full#supplementary-material
Footnotes
References
Aggarwal, R. K., Kivisild, T., Ramadevi, J., and Singh, L. (2007). Mitochondrial DNA coding region sequences support the phylogenetic distinction of two Indian wolf species. J. Zool. Syst. Evol. Res. 45, 163–172.
Aggarwal, R. K., Ramadevi, J., and Singh, L. (2003). Ancient origin and evolution of the Indian wolf: evidence from mitochondrial DNA typing of wolves from Trans-Himalayan region and Pennisular India. Genome Biol. 4, 1–30.
Bahuguna, A., Sahajpal, V., Goyal, S. P., Mukherjee, S. K., and Thakur, V. (2010). Species Identification from Guard Hair of Selected Indian mammals. Dehradun: Wildlife Institute of India.
Ballard, W. B., Ayres, L. A., Krausman, P. R., Reed, D. J., and Fancy, S. G. (1997). Ecology of wolves in relation to a migratory caribou herd in northwest Alaska. Wildl. Monogr. 135, 1–47.
Ballard, W. B., Whitman, J. S., and Gardner, C. L. (1987). Ecology of an exploited wolf population in south-central Alaska. Wildl. Monogr. 98, 1–54.
Bashir, T., Bhattacharya, T., Poudyal, K., Roy, M., and Sathyakumar, S. (2014). Precarious status of the Endangered dhole Cuon alpinus in the high elevation Eastern Himalayan habitats of Khangchendzonga Biosphere Reserve, Sikkim, India. Oryx 48, 125–132. doi: 10.1017/s003060531200049x
Bauer, J. J. (1990). The analysis of plant-herbivore interactions between ungulates and vegetation on alpine grasslands in the Himalayan region of Nepal. Vegetatio 90, 15–34. doi: 10.1007/bf00045586
Bhambri, R., Bolch, T., Chaujar, R. K., and Kulshreshtha, S. C. (2011). Glacier changes in the Garhwal Himalaya, India, from 1968 to 2006 based on remote sensing. J. Glaciol. 57, 543–556. doi: 10.3189/002214311796905604
Bhattacharya, A., Chatterjee, N., Rawat, G. S., and Habib, B. (2020). Blue sheep resource selection in alpine grasslands of a western himalayan landscape–a point process approach. Zool. Stud. 59:e11. doi: 10.6620/ZS.2020.59-11
Bhattacharya, T., and Sathyakumar, S. (2010). Sighting of Tibetan wolf Canis lupus chanko in the greater Himalayan range of Nanda Devi biosphere reserve, Uttarakhand, India: a new record. J. Threat. Taxa 12, 1345–1348. doi: 10.11609/jott.o2423.1345-8
Bhattacharya, T., and Sathyakumar, S. (2011). Natural resource use by humans and response of wild ungulates. Mt. Res. Dev. 31, 209–219. doi: 10.1659/mrd-journal-d-10-00069.1
Burnham, K. P., and Anderson, D. R. (2002). Model Selection and Inference: A Practical Information / Theoretic Approach, 2nd Edn. Berlin: Springer.
Chanchani, P., Rawat, G. S., and Goyal, S. P. (2011). Ecology and Conservation of Ungulates in Tso Lhamo, North Sikkim. Biodiversity of Sikkim-Exploring and Conserving a Global Hotspot. Gangtok: Information and Public Relations Department, 351–362.
Chandola, S. (2008). Vegetation Inventory of Cold Desert Habitat of Nilang Area of Jadh Ganga Catchment (Uttarkashi) in Garhwal Himalaya. Degree of Doctor of Philosophy in Botany. Srinagar: H.N.B Garhwal University.
Chapron, G., Kaczensky, P., Linnell, J. D., Von Arx, M., Huber, D., Andrén, H., et al. (2014). Recovery of large carnivores in Europe’s modern human-dominated landscapes. Science 346, 1517–1519. doi: 10.1126/science.1257553
Chaves, P. B., Graeff, V. G., Lion, M. B., Oliveira, L. R., and Eizirik, E. (2012). DNA barcoding meets molecular scatology: short mtDNA sequences for standardized species assignment of carnivore noninvasive samples. Mol. Ecol. Resour. 12, 18–35. doi: 10.1111/j.1755-0998.2011.03056.x
Chetri, M., Jhala, Y. V., Jnawali, S. R., Subedi, N., Dhakal, M., and Yumnam, B. (2016). Ancient himalayan wolf (Canis lupus chanco) lineage in upper mustang of the annapurna conservation area Nepal. ZooKeys 21, 143–156. doi: 10.3897/zookeys.582.5966
Chetri, M., Odden, M., Devineau, O., McCarthy, T., and Wegge, P. (2020). Multiple factors influence local perceptions of snow leopards and Himalayan wolves in the central Himalayas Nepal. PeerJ 8:e10108. doi: 10.7717/peerj.10108
Chetri, M., Odden, M., and Wegge, P. (2017). Snow leopard and Himalayan wolf: food habits and prey selection in the Central Himalayas Nepal. PLoS One 12:e0170549. doi: 10.1371/journal.pone.0170549
Ciucci, P., Boitani, L., Francisci, F., and Andreoli, G. (1997). Home range, activity and movements of a wolf pack in central Italy. J. Zool. 243, 803–819. doi: 10.1111/j.1469-7998.1997.tb01977.x
Crête, M., and Larivière, S. (2003). Estimating the costs of locomotion in snow for coyotes. Can. J. Zool. 81, 1808–1814. doi: 10.1139/z03-182
Didan, K. (2015). MOD13Q1 MODIS/Terra Vegetation Indices 16-Day L3 Global 250m SIN Grid V006. NASA EOSDIS Land Processes DAAC. Available online at: https://doi.org/10.5067/MODIS/MOD13Q1.006 [Accessed January 23, 2021].
Droghini, A., and Boutin, S. (2018). Snow conditions influence grey wolf (Canis lupus) travel paths: the effect of human-created linear features. Can. J. Zool. 96, 39–47. doi: 10.1139/cjz-2017-0041
Estes, J. A., Terborgh, J., Brashares, J. S., Power, M. E., Berger, J., Bond, W. J., et al. (2011). Trophic downgrading of planet. Earth. Sci. 333, 301–306. doi: 10.1126/science.1205106
Farrell, L. E., Roman, J., and Sunquist, M. E. (2000). Dietary separation of sympatric carnivores identified by molecular analysis of scats. Mol. Ecol. 9, 1583–1590. doi: 10.1046/j.1365-294x.2000.01037.x
Floyd, T. J., Mech, L. D., and Jordan, P. A. (1978). Relating wolf scat content to prey consumed. J. Wildl. Manage. 43, 528–532. doi: 10.2307/3800814
Fox, J. L., and Chundawat, R. S. (1995). Wolves in the Transhimalayan region of India: the continued survival of a low-density population. Ecol. Conserv. Wolves Chang. World 35, 95–103.
Fuller, T. K. (1989). Population dynamics of wolves in north-central Minnesota. Wildl. Monogr. 80, 3–41.
Fuller, T. K. (1991). Effect of snow depth on wolf activity and prey selection in north central Minnesota. Can. J. Zool. 69, 283–287.
Habib, B., Shrotriya, S., and Jhala, Y. V. (2013). Ecology and Conservation of Himalayan Wolf. Technical Report No TR–2013/01. Dehradun: Wildlife Institute of India, 46.
Hayes, R. A., and Harestad, A. S. (2000). Demography of a recovering wolf population in the Yukon. Can. J. Zool. 78, 36–48. doi: 10.1139/z99-186
Hennelly, L., Habib, B., and Lyngdoh, S. (2015). Himalayan wolf and feral dog displaying mating behaviour in Spiti Valley, India, and potential conservation threats from sympatric feral dogs. Canid Biol. Conserv. 18, 27–30.
Hinton, J. W., Proctor, C., Kelly, M. J., van Manen, F. T., Vaughan, M. R., and Chamberlain, M. J. (2016). Space use and habitat selection by resident and transient red wolves (Canis rufus). PLoS One 11:e0167603. doi: 10.1371/journal.pone.0167603
Huggard, D. J. (1993). Effect of snow depth on predation and scavenging by gray wolves. J. Wildl. Manage. 57, 382–388. doi: 10.2307/3809437
Jackson, R. M., Ahlborn, G. G., Gurung, M., and Ale, S. (1996). “Reducing livestock depredation losses in the Nepalese Himalaya,” in Proceedings of the Vertebrate Pest Conference, Vol. 17, eds R. M. Timm and A. C. Crabb (Davis, CA: University of California).
Jarvis, A., Guevara, E., Reuter, H. I., and Nelson, A. D. (2008). Hole-Filled SRTM for the Globe, Version 4. CGIAR-CSI SRTM 90m Database, CGIAR Consortium for Spatial Information.
Jędrzejewski, W., Schmidt, K., Theuerkauf, J., Jędrzejewska, B., Selva, N., Zub, K., et al. (2002). Kill rates and predation by wolves on ungulate populations in Białowieża Primeval Forest (Poland). Ecology 83, 1341–1356.
Jethva, B. D., and Jhala, Y. V. (2003). Sample size considerations for food habits studies of wolves from scats. Mammalia 67, 589–592.
Joshi, B., Lyngdoh, S., Singh, S. K., Sharma, R., Kumar, V., Tiwari, V. P., et al. (2020). Revisiting the Woolly wolf (Canis lupus chanco) phylogeny in Himalaya: addressing taxonomy, spatial extent and distribution of an ancient lineage in Asia. PLoS One 15:e0231621. doi: 10.1371/journal.pone.0231621
Jumabay-Uulu, K., Wegge, P., Mishra, C., and Koustubh, S. (2014). Large carnivores and low diversity of optimal prey: a comparison of the diets of snow leopards Panthera uncia and wolves Canis lupus in Sarychat-Ertash Reserve in Kyrgyzstan. Oryx 48, 529–535. doi: 10.1017/s0030605313000306
Kaartinen, S., Kojola, I., and Colpaert, A. (2005). Finnish wolves avoid roads and settlements. Ann. Zool. Fennici 42, 523–532.
Kaczensky, P., Enkhsaikhan, N., Ganbaatar, O., and Walzer, C. (2008). The great gobi B strictly protected area in mongolia-refuge or sink for wolves Canis lupus in the Gobi. Wildl. Biol. 14, 444–456. doi: 10.2981/0909-6396-14.4.444
Karanth, K. U., and Sunquist, M. E. (1995). Prey selection by tiger, leopard and dhole in tropical forests. J. Anim. Ecol. 64, 439–450. doi: 10.2307/5647
Klare, U., Kamler, J. F., and Macdonald, D. W. (2011). A comparison and critique of different scat-analysis methods for determining carnivore diet. Mamm. Rev. 41, 294–312. doi: 10.1111/j.1365-2907.2011.00183.x
Kocher, T. D., Thomas, W. K., Meyer, A., Edwards, S. V., Pääbo, S., Villablanca, F. X., et al. (1989). Dynamics of mitochondrial DNA evolution in animals: amplification and sequencing with conserved primers. Proc. Natl. Acad. Sci. U. S. A. 86, 6196–6200. doi: 10.1073/pnas.86.16.6196
Koetke, L. J., Bhattacharya, T., and Sathyakumar, S. (2020). Diet overlap between livestock and wild herbivores in the Greater Himalaya. Mt. Res. Dev. 40, R21–R27.
Kumar, A., Adhikari, B. S., and Rawat, G. S. (2017). Biogeographic delineation of the Indian Trans-Himalaya: need for revision. Curr. Sci. 113, 1032–1033.
Kusak, J., Skrbinšek, A. M., and Huber, D. (2005). Home ranges, movements, and activity of wolves (Canis lupus) in the dalmatian part of Dinarids. Croatia. Eur. J. Wildl. Res. 51, 254–262. doi: 10.1007/s10344-005-0111-2
Laliberte, A. S., and Ripple, W. J. (2004). Range contractions of North American carnivores and ungulates. BioScience 54, 123–138. doi: 10.1641/0006-3568(2004)054[0123:rconac]2.0.co;2
López-Bao, J. V., Blanco, J. C., Rodríguez, A., Godinho, R., Sazatornil, V., Alvares, F., et al. (2015). Toothless wildlife protection laws. Biodivers. Conserv. 24, 2105–2108. doi: 10.1007/s10531-015-0914-8
Lute, M. L., Carter, N. H., López-Bao, J. V., and Linnell, J. D. (2018). Conservation professionals agree on challenges to coexisting with large carnivores but not on solutions. Biol. Conserv. 218, 223–232. doi: 10.1016/j.biocon.2017.12.035
Lyngdoh, S. (2020). Ecology and Conservation of Himalayan Wolf. PhD thesis. Rajkot: Saurashtra University.
Lyngdoh, S., and Habib, B. (2019). Predation by Himalayan wolves: understanding conflict, culture and co-existence amongst Indo-Tibetan community and large carnivores in High Himalaya. BioRxiv. doi: 10.1101/2019.12.16.877936
Magnusson, A., Skaug, H., Nielsen, A., Berg, C., Kristensen, K., Maechler, M., et al. (2017). Package ‘glmmTMB’. R Package Version 0.2. 0.
Maheshwari, A., Sharma, D., and Sathyakumar, S. (2013). Snow leopard (Panthera uncia) surveys in the Western Himalayas, India. J. Ecol. Nat. Environ. 5, 303–309. doi: 10.5897/jene2013.0382
Mancinelli, S., Boitani, L., and Ciucci, P. (2018). Determinants of home range size and space use patterns in a protected wolf (Canis lupus) population in the central Apennines, Italy. Can. J. Zool. 96, 828–838.
Martin, M. E., Moriarty, K. M., and Pauli, J. N. (2020). Forest structure and snow depth alter the movement patterns and subsequent expenditures of a forest carnivore, the Pacific marten. Oikos 129, 356–366.
Mattisson, J., Sand, H., Wabakken, P., Gervasi, V., Liberg, O., Linnell, J. D., et al. (2013). Home range size variation in a recovering wolf population: evaluating the effect of environmental, demographic, and social factors. Oecologia 173, 813–825. doi: 10.1007/s00442-013-2668-x
Mech, L. D., and Boitani, L. (2003). “Chapter 5: wolf-prey relations,” in Wolves: Behaviour, Ecology and Conservation, eds L. D. Mech and L. Boitani (Chicago, IL: The University of Chicago Press), 131–160.
Meredith, M., and Ridout, M. (2014). overlap: Estimates of Coefficient of Overlapping for Animal Activity Patterns. R Package Version 0.2.3. Available online at: http://CRAN.R-project.org/package=overlap. [Accessed September 21, 2014]
Mishra, C., Bagchi, S., Namgail, T., and Bhatnagar, Y. V. (2010). “Multiple use of Trans-Himalayan rangelands: reconciling human livelihoods with wildlife conservation,” in Wild Rangelands: Conserving Wildlife While Maintaining Livestock in Semi-Arid Ecosystems, eds J. T. du Toit, R. Kock, and J. C. Deutsch (Hoboken, NJ: Wiley), 291–311. doi: 10.1002/9781444317091.ch11
Mishra, C., Redpath, S. R., and Suryawanshi, K. R. (2016). “Livestock predation by snow leopards: conflicts and the search for solutions,” in Snow Leopards, eds T. McCarthy and D. Mallon (Cambridge, MA: Academic Press), 59–67. doi: 10.1016/b978-0-12-802213-9.00005-5
Mishra, C., Van Wieren, S. E., Heitkönig, I. M., and Prins, H. H. (2002). A theoretical analysis of competitive exclusion in a Trans-Himalayan large-herbivore assemblage. Anim. Conserv. 5, 251–258. doi: 10.1017/s1367943002002305
Mishra, C., Young, J. C., Fiechter, M., Rutherford, B., and Redpath, S. M. (2017). Building partnerships with communities for biodiversity conservation: lessons from Asian mountains. J. Appl. Ecol. 54, 1583–1591. doi: 10.1111/1365-2664.12918
Moriarty, K. M., Epps, C. W., Betts, M. G., Hance, D. J., Bailey, J. D., and Zielinski, W. J. (2015). Experimental evidence that simplified forest structure interacts with snow cover to influence functional connectivity for Pacific martens. Landsc. Ecol. 30, 1865–1877.
Mukherjee, S., Goyal, S. P., and Chellam, R. (1994). Standardisation of Scat Analysis Techniques for Leopard (Panthera pardus), Gir National Park Western India. Mammalia, 58, 139–143.
Mukherjee, S., Goyal, S. P., Johnsingh, A. J. T., and Pitman, M. L. (2004). The importance of rodents in the diet of jungle cat (Felis chaus), caracal (Caracal caracal) and golden jackal (Canis aureus) in Sariska Tiger Reserve, Rajasthan, India. J. Zool. 262, 405–411.
Musiani, M., Muhly, T., Gates, C. C., Callaghan, C., Smith, M. E., and Tosoni, E. (2005). Seasonality and reoccurrence of depredation and wolf control in western North America. Wildl. Soc. Bull. 33, 876–887. doi: 10.2193/0091-7648(2005)33[876:saroda]2.0.co;2
Namgail, T., Fox, J. L., and Bhatnagar, Y. V. (2007). Carnivore-caused livestock mortality in Trans-Himalaya. Environ. Manage. 39, 490–496. doi: 10.1007/s00267-005-0178-2
Nelson, M. E., and Mech, L. D. (1986). Relationship between snow depth and gray wolf predation on white-tailed deer. J. Wildl. Manage. 50, 471–474. doi: 10.2307/3801108
Newsome, T. M., Boitani, L., Chapron, G., Ciucci, P., Dickman, C. R., and Dellinger, J. A. (2016). Food habits of the world’s grey wolves. Mamm. Rev. 46, 255–269. doi: 10.1038/s41598-019-51884-5
Nielsen, E. T. (1984). Relation of behavioural activity rhythms to the changes of day and night. A revision of views. Behaviour 89, 147–173. doi: 10.1186/s12913-016-1423-5
Noss, R. F., Quigley, H. B., Hornocker, M. G., Merrill, T., and Paquet, P. C. (1996). Conservation biology and carnivore conservation in the Rocky Mountains. Conserv. Biol. 10, 949–963. doi: 10.1046/j.1523-1739.1996.10040949.x
Odonjavkhlan, C., Alexsander, J. S., Mishra, C., Samelius, G., Sharma, K., Lkhagvajav, P., et al. (2021). Factors affecting the spatial distribution and co-occurrence of two sympatric mountain ungulates in southern Mongolia. J. Zool. 314, 266–274.
Oli, M. K. (1993). A key for the identification of the hair of mammals of a snow leopard (Panthera uncia) habitat in Nepal. J. Zool. 231, 71–93.
Pal, R., Bhattacharya, T., Qureshi, Q., Buckland, S. T., and Sathyakumar, S. (2021). Using distance sampling with camera traps to estimate the density of group-living and solitary mountain ungulates. Oryx 55, 668–676.
Pal, R., Thakur, S., Arya, S., Bhattacharya, T., and Sathyakumar, S. (2020). Mammals of the Bhagirathi basin, Western Himalaya: understanding distribution along spatial gradients of habitats and disturbances. Oryx 55, 1–11.
Pal, R. B., Bhattacharya, T., and Sathyakumar, S. (2018). First Confirmation on the Occurrence of Threatened Tibetan Argali in Gangotri National Park, Uttarakhand, India. Caprinae Newsletter 1: 13–15.
Paquet, P. C., and Carbyn, L. N. (2003). “Gray wolf,” in Wild Mammals of North America: Biology, Management, and Conservation, eds G. A. Feldhamer, B. C. Thompson, and J. A. Chapman (Baltimore MD: Johns Hopkins University Press), 482–510.
Parker, K. L., Robbins, C. T., and Hanley, T. A. (1984). Energy expenditures for locomotion by mule deer and elk. J. Wildl. Manage. 482, 474–488.
Patterson, B. R., Bondrup-Nielsen, S., and Messier, F. (1999). Activity patterns and daily movements of the eastern coyote, Canis latrans, in Nova Scotia. Can. Field Nat. 113, 251–257.
Piggott, M. P., and Taylor, A. C. (2003). Remote collection of animal DNA and its applications in conservation management and understanding the population biology of rare and cryptic species. Wildl. Res. 30, 1–13. doi: 10.1071/wr02077
R Core Team (2019). R: A Language and Environment for Statistical Computing. Vienna: R Foundation for Statistical Computing. Available online at: https://www.R-project.org/
Rana, D. B. (2018). Distribution Occupancy, Potential Habitat and Conservation of Recolonized Himalayan Wolf in Upper Mustang, Annapurna Conservation Area, Nepal. Doctoral dissertation. Kirtipur: Tribhuvan University.
Rawat, G. S. (2007). Pastoral practices, wild mammals and conservation status of alpine meadows in Western Himalaya. J. Bombay Nat. Hist. Soc. 104:5.
Reynolds, J. C., and Aebischer, N. J. (1991). Comparison and quantification of carnivore diet by faecal analysis: a critique, with recommendations, based on a study of the fox Vulpes vulpes. Mamm. Rev. 21, 97–122.
Reynolds, J. C., and Tapper, S. C. (1996). Control of mammalian predators in game management and conservation. Mamm. Rev. 26, 127–155. doi: 10.1111/j.1365-2907.1996.tb00150.x
Rich, L. N., Mitchell, M. S., Gude, J. A., and Sime, C. A. (2012). Anthropogenic mortality, intraspecific competition, and prey availability influence territory sizes of wolves in Montana. J. Mammal. 93, 722–731. doi: 10.1644/11-mamm-a-079.2
Ridout, M. S., and Linkie, M. (2009). Estimating overlap of daily activity patterns from camera trap data. J. Agric. Biol. Environ. Stat. 14, 322–337. doi: 10.3390/ani10050865
Ripple, W. J., Estes, J. A., Beschta, R. L., Wilmers, C. C., Ritchie, E. G., Hebblewhite, M., et al. (2014). Status and ecological effects of the world’s largest carnivores. Science 343:1241484. doi: 10.1126/science.1241484
Ripple, W. J., Estes, J. A., Schmitz, O. J., Constant, V., Kaylor, M. J., Lenz, A., et al. (2016). What is a trophic cascade? Trends Ecol. Evol. 31, 842–849.
Sandercock, B. K., Martin, K., and Hannon, S. J. (2005). Demographic consequences of age-structure in extreme environments: population models for arctic and alpine ptarmigan. Oecologia 146, 13–24. doi: 10.1007/s00442-005-0174-5
Sathyakumar, S., and Bhatnagar, Y. V. (2002). Mountain Ungulates ENVIS Bulletin: Wildlife and Protected Areas. Dehradun: Wildlife Institute of India.
Sharma, D. K., Maldonado, J. E., Jhala, Y. V., and Fleischer, R. C. (2004). Ancient wolf lineages in India. Proc. Biol. Sci. 271, S1–S4. doi: 10.1098/rsbl.2003.0071
Sharma, S., Dutta, T., and Bhatnager, Y. V. (2007). Snow Leopard in Himalaya: Resource Partitioning and Coexistence with Tibetan Wolf. Felid Biology and Conservation. The Wildlife Conservation Research Unit. Oxford: Oxford University, 87.
Shipley, A. A., Sheriff, M. J., Pauli, J. N., and Zuckerberg, B. (2019). Snow roosting reduces temperature-associated stress in a wintering bird. Oecologia 190, 309–321. doi: 10.1007/s00442-019-04389-x
Subba, S. A. (2012). Assessing the Genetic Status, Distribution, Prey Selection and Conservation Issues of Himalayan wolf (Canis himalayensis). Trans-Himalayan Dolpa, Nepal. MSc. Lund: Lund University.
Suryawanshi, K. R., Bhatnagar, Y. V., Redpath, S., and Mishra, C. (2013). People, predators and perceptions: patterns of livestock depredation by snow leopards and wolves. J. Appl. Ecol. 50, 550–560. doi: 10.1111/1365-2664.12061
Theuerkauf, J. (2009). What drives wolves: fear or hunger? Humans, diet, climate and wolf activity patterns. Ethology 115, 649–657. doi: 10.1111/j.1439-0310.2009.01653.x
Theuerkauf, J., Jędrzejewski, W., Schmidt, K., and Gula, R. (2003a). Spatiotemporal segregation of wolves from humans in the Białowieża Forest (Poland). J. Wildl. Manage. 67, 706–716.
Theuerkauf, J., Jędrzejewski, W., Schmidt, K., Okarma, H., Ruczyński, I., Śniezko, S., et al. (2003b). Daily patterns and duration of wolf activity in the Białowieza Forest, Poland. J. Mammal. 84, 243–253. doi: 10.1644/1545-1542(2003)084<0243:dpadow>2.0.co;2
Trites, A. W., and Joy, R. (2005). Dietary analysis from fecal samples: how many scats are enough? J. Mammal. 86, 704–712.
Vilà, C., Urios, V., and Castroviejo, J. (1995). “Observations on the daily activity patterns in the Iberian wolf,” in Ecology and Conservation of Wolves in a Changing World, eds L. N. Carbyn, S. H. Fritts, and D. R. Seip (Edmonton, AB: Canadian Circumpolar Institute), 335–340. Occasional Publication No. 35.
Weaver, J. L. (1993). Refining the equation for interpreting prey occurrence in gray wolf scats. J. Wildl. Manage. 57, 534–538. doi: 10.2307/3809278
Weiskopf, S. R., Kachel, S. M., and McCarthy, K. P. (2016). What are snow leopards really eating? Identifying bias in food-habit studies. Wildl. Soc. Bull. 40, 233–240. doi: 10.1002/wsb.640
Werhahn, G. (2020). Phylogeny and Ecology of the Himalayan wolf. Doctoral dissertation. Oxford: University of Oxford.
Werhahn, G., Kusi, N., Li, X., Chen, C., Zhi, L., Martín, R. L., et al. (2019). Himalayan wolf foraging ecology and the importance of wild prey. Glob. Ecol. Conserv. 20:e00780.
Keywords: camera trap, food habit, grazing pressure, generalized linear mixed models, genotyping
Citation: Pal R, Panwar A, Goyal SP and Sathyakumar S (2022) Space Use by Woolly Wolf Canis lupus chanco in Gangotri National Park, Western Himalaya, India. Front. Ecol. Evol. 9:782339. doi: 10.3389/fevo.2021.782339
Received: 24 September 2021; Accepted: 13 December 2021;
Published: 14 January 2022.
Edited by:
Attila D. Sándor, University of Agricultural Sciences and Veterinary Medicine of Cluj-Napoca, RomaniaReviewed by:
Robert W. Myslajek, University of Warsaw, PolandJohn Bruggink, Northern Michigan University, United States
Copyright © 2022 Pal, Panwar, Goyal and Sathyakumar. This is an open-access article distributed under the terms of the Creative Commons Attribution License (CC BY). The use, distribution or reproduction in other forums is permitted, provided the original author(s) and the copyright owner(s) are credited and that the original publication in this journal is cited, in accordance with accepted academic practice. No use, distribution or reproduction is permitted which does not comply with these terms.
*Correspondence: Sambandam Sathyakumar, c3NrQHdpaS5nb3YuaW4=