- 1Chromatin and Epigenetics Lab, Department of Biotechnology, University of Kashmir, Srinagar, India
- 2Department of Botany, University of Kashmir, Srinagar, India
- 3Centre for Interdisciplinary Research and Innovations, University of Kashmir, Srinagar, India
While differences in the methylation patterns of ABC transporters under different environmental conditions and their role in plant growth, development, and response to biotic and abiotic stresses are well documented, less is known about the variation in the methylation patterns of ABC transporters in plant species in the native and non-native ranges. In this study, we present the results of differences in methylation of ABC transporters of Conyza canadensis L. in its native (North America) and non-native (Kashmir Himalaya) ranges. Our data show that ABC transporter genes have reduced DNA methylation in Kashmir Himalaya than in North America. Furthermore, in the non-native range of Kashmir Himalaya, we found that ABC transporter genes have enriched RNA Pol-II binding and reduced nucleosome occupancy, both hallmarks of transcriptional activity. Taken together, our study showed differential DNA methylation in the ABC transporter genes in the native range of North America and non-native range of Kashmir Himalaya in Conyza canadensis and that the reduced DNA methylation and increased RNA Pol-II binding is one of the possible mechanisms through which this species in the non-native range of Kashmir Himalaya may show greater gene expression of ABC transporter genes. This increased ABC transporter gene expression may help the plant to grow in different environmental conditions in the non-native range. Furthermore, this study could pave way for more studies to better explain the enigmatic plant invasions of C. canadensis in the non-native range of Kashmir Himalaya.
Introduction
DNA methylation, one of the most common epigenetic modifications, contributes to the overall growth, development, and tolerance to both biotic and abiotic factors (Alonso et al., 2018; Agarwal et al., 2020; Eriksson et al., 2020; Gallego-Bartolom, 2020; Liu and He, 2020; Kumar and Mohapatra, 2021). DNA methylation and other epigenetic modifications are now known to adjust phenotypes instantaneously and/or generate new phenotypes in a reversibly heritable manner (Flatscher et al., 2012; Miryeganeh and Saze, 2019). These heritable epigenetic variations could directly or indirectly influence the course of evolution in plants, as they can affect the processes of adaptation (Rapp and Wendel, 2005; Jablonka and Raz, 2009).
Epigenetic processes, including DNA methylation, have emerged as important mechanisms contributing to adaptation and spread of alien plants in new habitats in the non-native ranges (Perez et al., 2015), despite several bottlenecks, such as low propagule pressure, low genetic variability, climate incompatibility, inadequate or inappropriate resources, or small population size (Banerjee et al., 2019). Conyza canadensis, commonly called as horseweed, has successfully adapted to several non-native habitats across the world and is considered a highly invasive problematic annual weed species of many agronomic crops in about 70 countries (Travlos and Chachalis, 2010; Moretti et al., 2017). This species has a very prolific growth and is reported to produce up to 200,000 seeds, which are easily dispersed by wind to infest new territories (Dauer et al., 2007). To control this weed species, commercial herbicides are in use for a long time but Conyza species have developed resistance against these herbicides, particularly glyphosate (Moretti et al., 2017). Vacuolar sequestration of herbicides has been identified as the predominant resistance mechanism that neutralizes its activity mediated through tonoplast localized ABC transporters (Peng et al., 2010; Douglas and Gaines, 2014; Ge et al., 2014). ABC transporter genes such as M10 and M11 are highly expressed in herbicide-resistant Conyza species (Peng et al., 2010; Nol et al., 2012; Moretti et al., 2017). Owing to environmental influence, there is a decreased expression of ABC transporters such as M10 and M11 under conditions of low temperature resulting in a lack of glyphosate resistance (Tani et al., 2016). The possible mechanisms of ABC transporter gene expression in invasive populations could be DNA methylation-induced alterations in chromatin structure, which, in turn, may be responsible for higher adaptability and invasiveness. Keeping in view the differential methylation patterns across various habitats and the potential role played by epigenetic diversity in influencing the invasive traits of a plant (Zhang et al., 2016; Banerjee et al., 2019), we hypothesized differential epigenetic signatures of C. canadensis in its native range of North America and non-native range of Kashmir Himalaya. The study, the first of its kind on C. canadensis, is likely to open new vistas of investigating the invasiveness of alien species in the non-native ranges, which is a prerequisite for their better management.
Materials and Methods
Sampling of Leaf Material
Leaf samples at the flowering stage were collected from both the native range of North America and the non-native range of Kashmir Himalaya from 5 different sites. Each sample was collected along with three biological replicates and three technical replicates. The sites, namely, Kangan, Gulmarg, Budgam, KUBG1 (Kashmir University botanical garden), and KUBG2, were selected for the collection of non-native leaf samples of Kashmir Himalaya, while Turah, Clinton, Clarke, Butte, and Lolo were selected for the native leaf samples of North America.
Chromatin Extraction Followed by Methylated DNA Immunoprecipitation
Chromatin was extracted from the leaf samples of both the native range of North America and the non-native range of Kashmir Himalaya of C. canadensis. After quantification, approximately 6 μg of DNA was taken for sonication (20% amplitude, pulse: 30 s, and time: 3 min). Samples were normalized and incubated at 4°C using an antimethyl cytosine antibody overnight with end-to-end rotation. For pull-down, equilibrated Dynabeads were added to each sample. Then samples were treated with proteinase K and glycogen, incubated at 55°C, 1,200 rpm overnight (Fabio et al., 2009). DNA was extracted using PCI (phenol:chloroform:isoamyl) and resuspended in 1 × TE buffer, followed by quantitative PCR (qPCR).
Chromatin Immunoprecipitation Assay
Approximately 2 g leaf samples of C. canadensis from both the native range of North America and the non-native range of Kashmir Himalaya were taken and submerged in 37% formaldehyde and 2.5 M glycine for crosslinking of tissue using a vacuum infiltrator (Saleh et al., 2008). The tissue was then washed two times with double distilled water and then resuspended in extraction buffer 1 (0.4 M sucrose, 10 mM Tris-HCl, 5 mM βmerc, 0.1 mM PMSF, PIC) and filtered through Mira cloth (Merck Millipore, Burlington, MA, United States). After centrifugation for 20 min at 5,000 rpm, the pellet was resuspended in 1 ml of extraction buffer 2 (0.25 M sucrose, 10 mM Tris-HCl, 10 mM MgCl2, 1% Triton x-100, 5 mM βmerc, 0.1 mM PMSF, and PIC) followed by 300 μl of extraction buffer 3 (1.7 M sucrose, 10 mM Tris-HCl, 0.15% Triton x-100, 2 mM MgCl2, 5 mM βmerc, 0.1 mM PMSF, and PIC). Isolated chromatin was resuspended in nuclei lysis buffer (50 mM Tris-HCl, 10 mM EDTA, 1% SDS, PMSF, and PIC) followed by sonication (30 s ON, 30 s OFF, 4 cycles, amplitude 20%) for chromatin fragments approximately to the size of 250–500 bp. After centrifugation for 30 min, the supernatant of samples was normalized and the chromatin solution was subjected to O/N incubation at 4°C on a rotor with antibody (anti-POL II/anti-H3K14ac). Equilibrated Dynabeads (protein A and G) were added to each sample and incubated at 4°C for 3 h. Samples were washed with high salt buffer, low salt buffer, and LiCl buffer. The samples were eluted in TE/SDS at 65°C for 15 min. Samples were kept for reverse crosslinking at 65°C. DNA extraction was carried out using PCI and the pellet obtained was resuspended in TE and followed by qPCR.
MNase Titration Assay
Briefly, 2 g leaf samples of C. canadensis from both the native (North America) and non-native (Kashmir Himalaya) ranges were collected and ground using liquid nitrogen. Nuclei extraction buffer (0.25 M sucrose, 60 mM KCl, 0.8% Triton x-100, 15 mM MgCl2, 1 mM CaCl2, 15 mM HEPES, 0.1 mM PMSF) was added to leaf samples for nucleus isolation. The isolated nucleus was then resuspended into MNase digestion buffer and incubated with different units of MNase (1U, 2U, 3.5U, and 5U) at 30°C for 10 min (Pajoro et al., 2018). The reaction was then stopped using a stop buffer (250 mM EDTA and 10% SDS). All the samples were then subjected to 3 μl RNase treatment and 1/2 h incubation. This was followed by proteinase K treatment followed by 1 h incubation. DNA was then extracted, purified, and resuspended in TE buffer. The pattern of digested chromatin was confirmed on a 2% agarose gel, while its quantity and purity analyzed using NanoDropTM (Thermo Fisher Scientific, Waltham, MA, United States). The digested chromatin was quantified using ImageJ software (NIH Java-based).
Results
ABC Transporter Genes Show Reduced DNA Methylation in the Non-native Range of Kashmir Himalaya
Using methylated DNA immunoprecipitation (MeDIP) with a 5-methylcytidine antibody followed by quantitative real-time (qRT-PCR), we compared the DNA methylation level at the ABC transporter genes of C. canadensis in the native range of North America and non-native range of Kashmir Himalaya. MeDIP is an immunocapturing approach for unbiased detection of methylated DNA, wherein genomic DNA is sheared by sonication followed by immunoprecipitation using an antimethyl cytosine antibody (Fabio et al., 2009). The C. canadensis within the non-native range of Kashmir Himalaya showed significantly reduced DNA methylation at ABC transporter genes as compared to the native range of North America (p = 0.0072). The qRT-PCR results showed an IP/input value between 2.16 and 6.88 in samples from the native range of North America, while samples from the non-native range of Kashmir Himalaya showed significantly reduced IP/input values between 0.11 and 0.75 (Figure 1). Quite interestingly, the DNA methylation patterns of ABC transporter genes also varied across different populations within the native range of North America and the non-native range of Kashmir Himalaya. These results confirm that DNA methylation at ABC transporter genes could be one of the major factors regulating the expression of ABC transporter genes in the native range of North America and the non-native range of Kashmir Himalaya and that the reduced DNA methylation in the non-native range of Kashmir Himalaya may lead to increased ABC gene expression and hence more adaptability.
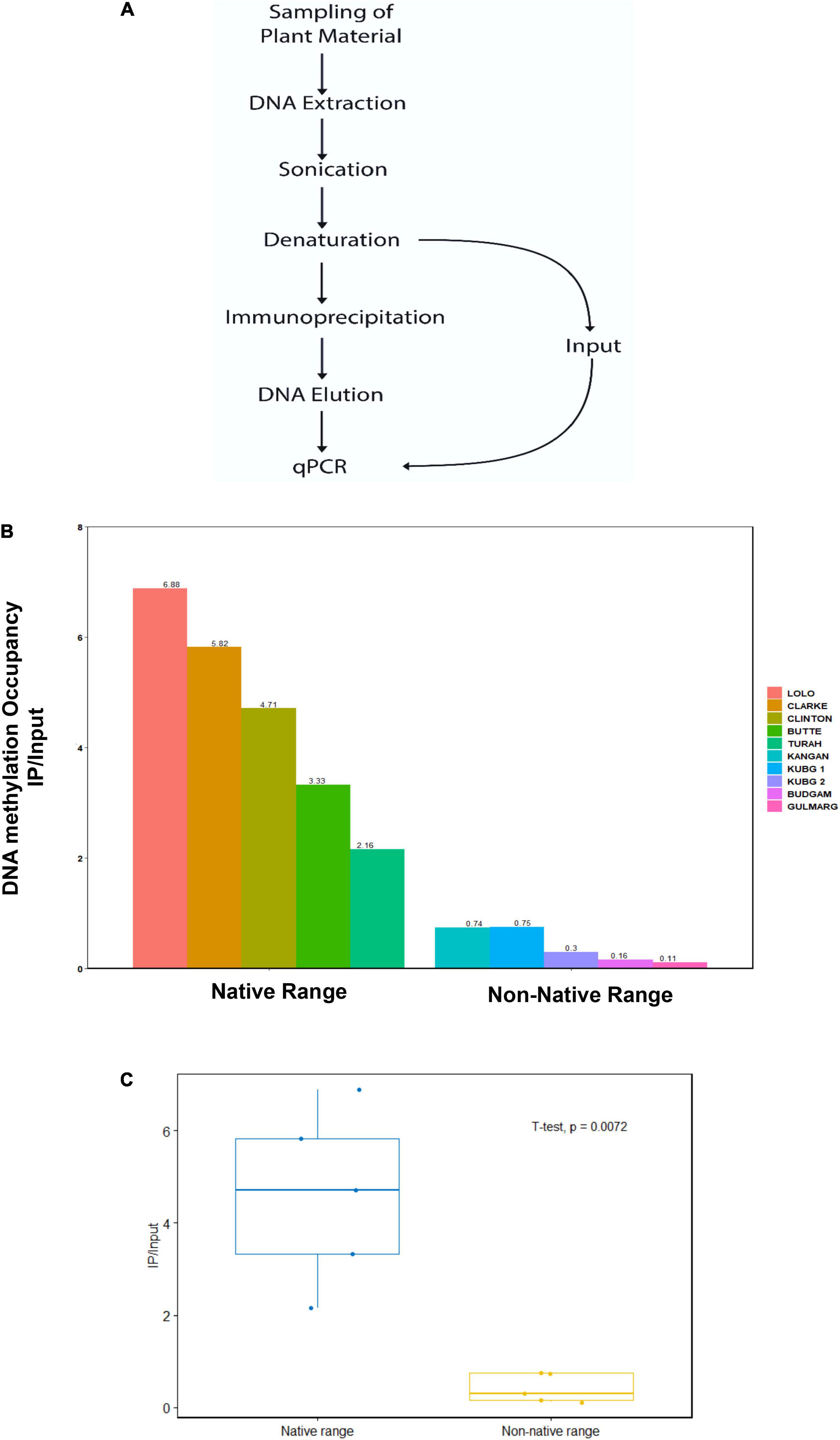
Figure 1. DNA methylation levels at ABC transporter gene in the native (North America) and non-native (Kashmir Himalaya) range plants. (A) The plant material was collected at different geographical locations as mentioned in “Materials and Methods” section. The DNA was isolated, sonicated, and subjected to immunoprecipitation using an antibody against 5-methyl cytosine and subjected to PCR with specific primers. (B) The IP/input ratio for 5-methyl cytosine in DNA of native and non-native range plants across different sites at ABC transporter gene. (C) The IP/input values at ABC transporter gene in native and non-native range obtained using R software, n = 5.
ABC Transporter Genes Show Enriched Pol II Occupancy in the Non-native Range of Kashmir Himalaya
Using chromatin immunoprecipitation with RNA Pol II antibody followed by qRT-PCR, we looked at RNA polymerase-II occupancy at ABC transporter genes in the native (North America) and non-native (Kashmir Himalaya) leaf samples. Pol II ChIP detects RNA polymerase II within the coding region of genes using polymerase antibodies analyzed using PCR (Sandoval et al., 2004). As expected, we observed increased RNA Pol II occupancy at ABC transporter genes in the non-native range of Kashmir Himalaya as compared to the native range of North America (Figure 2). The qRT-PCR results showed Pol II occupancy value between 0.128 and 0.296 in leaf samples of the native range of North America, while the leaf samples from the non-native range of Kashmir Himalaya showed Pol II occupancy value between 0.8 and 1.206. Furthermore, like DNA methylation, RNA Pol-II binding at ABC transporter genes also showed a variation across the different sites both within the native range of North America and the non-native range of Kashmir Himalaya. Reduced DNA methylation and subsequent increased RNA Pol-II binding may bring about the transcriptional activation of ABC transporter genes in the non-native range of Kashmir Himalaya and hence their increased expression.
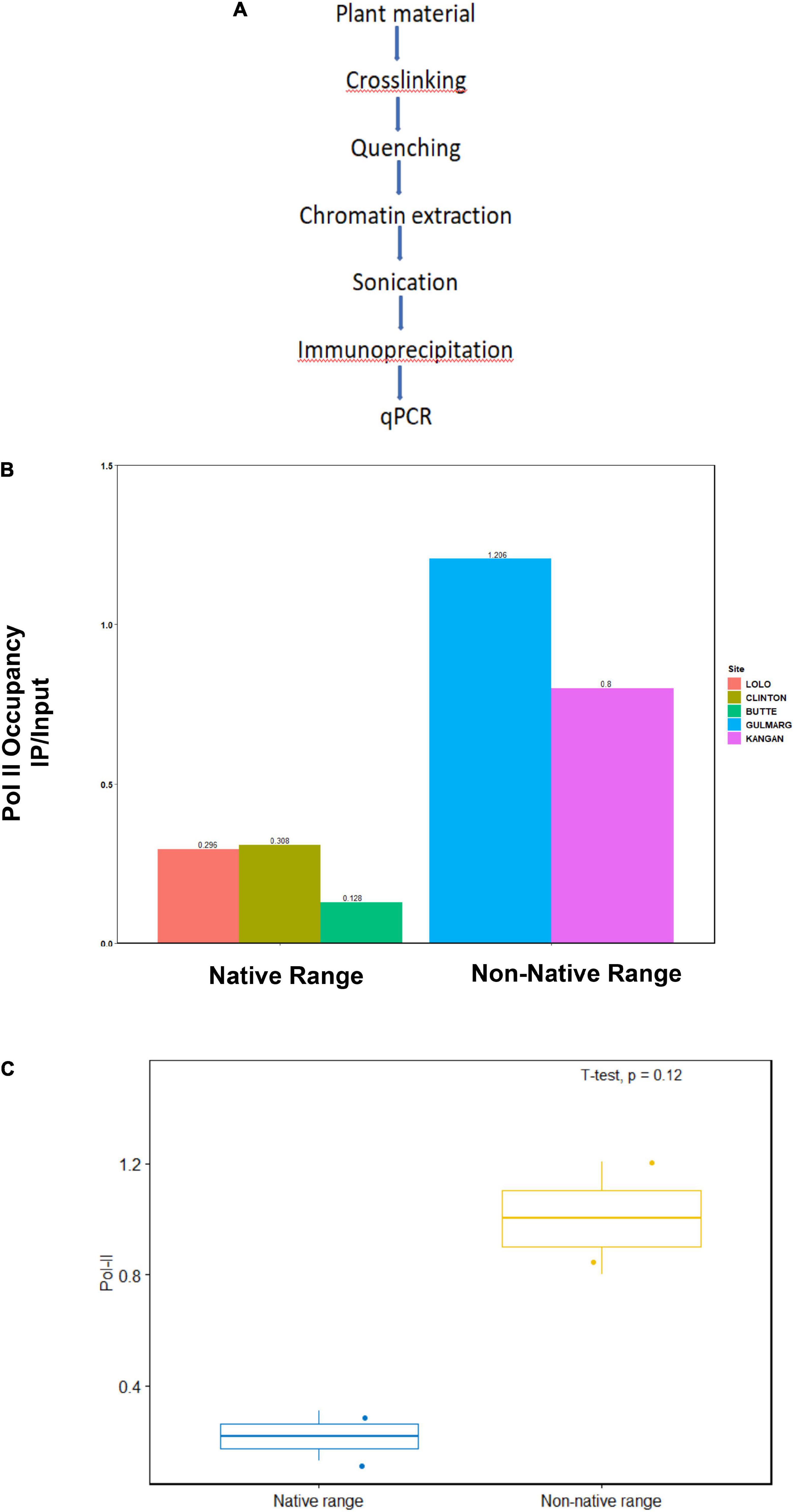
Figure 2. Pol-II occupancy at ABC transporter genes in the native (North America) and non-native (Kashmir Himalaya) range plants. (A) The plant material was collected at different geographical locations as mentioned in “Materials and Methods” section. The DNA-protein interaction was cross-linked with a suitable cross-linking agent. Subsequently, the DNA was isolated, sonicated, and then subjected to immunoprecipitation using specific antibodies. (B) The bar graphs represent the RNA Pol-II occupancy at ABC transporter genes in native and non-native range plants. (C) RNA Pol-II occupancy at ABC transporter gene in the native and non-native range obtained using R software, n = 3.
ABC Transporter Genes Show Loss of Nucleosomes in the Non-native Range of Kashmir Himalaya
In this study, we observed reduced acetylation levels of H3K14ac in the non-native range of Kashmir Himalaya as compared with the native range of North America, which could be due to changes in nucleosome occupancy (Figure 3). Chromatin immunoprecipitation analysis of histone H3 showed reduced nucleosome occupancy under non-native conditions. To get further insights into the changes in chromatin structure, we carried out micrococcal nuclease (MNase titrations) of chromatin from the native range of North America and the non-native range of Kashmir Himalaya. The MNase assay involves the digestion of chromatin using an enzyme MNase and understands the nucleosomal pattern by quantifying the protection of DNA from enzymatic digestion (Pass et al., 2017). Using the different concentrations of enzymes in an MNase assay, the non-native samples of Kashmir Himalaya showed more mononucleosomes as compared with the native ones of North America, indicating more open chromatin in the non-native C. canadensis within Kashmir Himalaya (Figure 4). This result suggests that the ABC locus in C. canadensis undergoes chromatin changes to provide phenotypic plasticity to the plant in the non-native range of Kashmir Himalaya. Collectively, reduced DNA methylation associated with increased Pol-II binding at the ABC transporter genes and open chromatin in C. canadensis within the non-native range of Kashmir Himalaya increases the transcriptional output that may increase the adaptability of these plants.
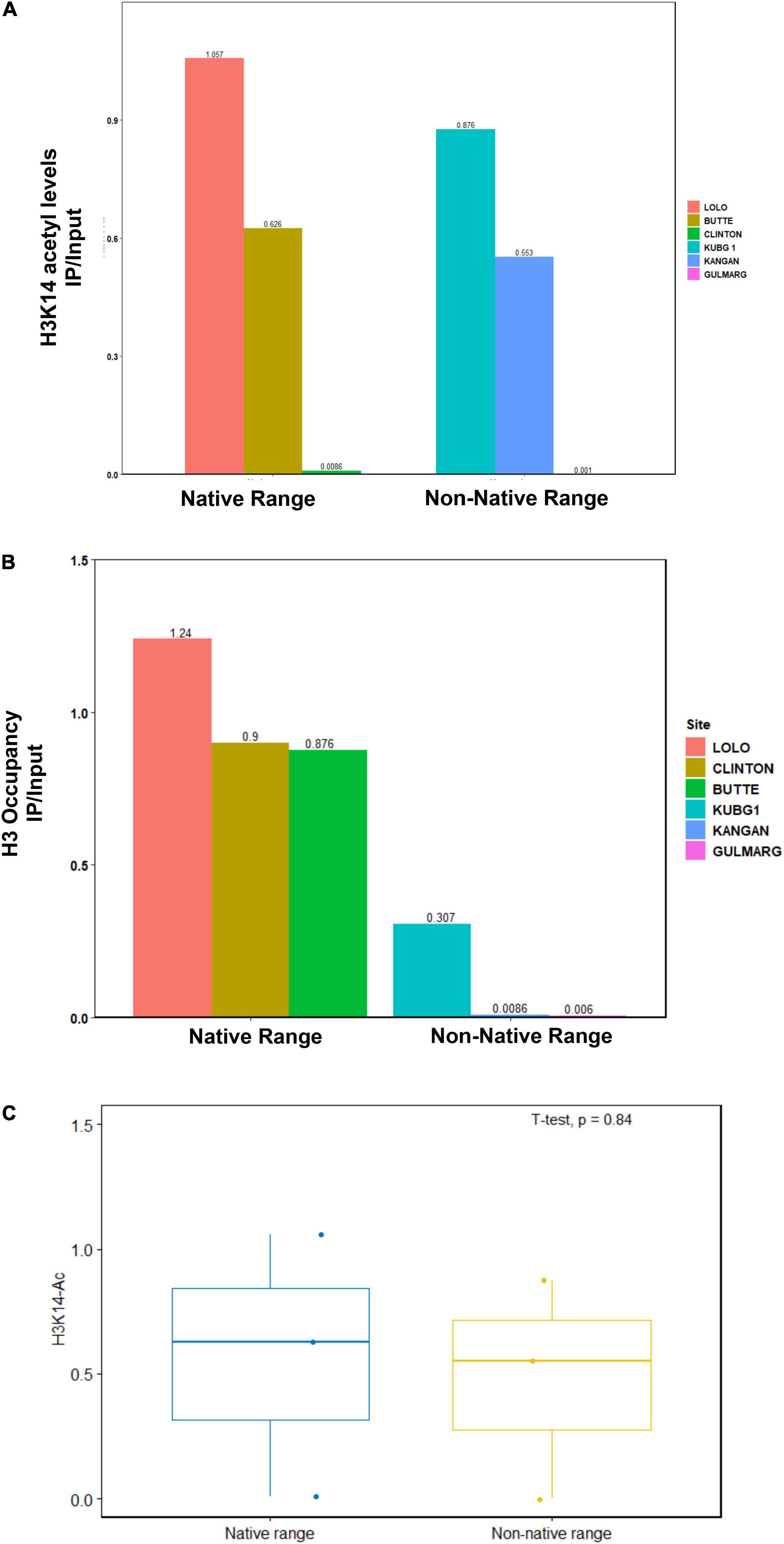
Figure 3. H3 and H3K14 acetyl occupancy in the native (North America) and non-native (Kashmir Himalaya) range plants. The plant material was collected at different geographical locations as mentioned in “Materials and Methods” section. The DNA-protein interaction was cross-linked with a suitable cross-linking agent. Subsequently, the DNA was isolated, sonicated, and then subjected to immunoprecipitation using specific antibodies. (A) The bar graphs represent the IP/Input ratio of H3K14ac occupancy at ABC transporter genes in the native and non-native range plants. (B) The bar graphs represent the IP/Input ratio of H3 occupancy at ABC transporter genes in the native and non-native range plants. (C) H3K14 acetyl occupancy at ABC transporter gene in the native and non-native range obtained using R software, n = 3.
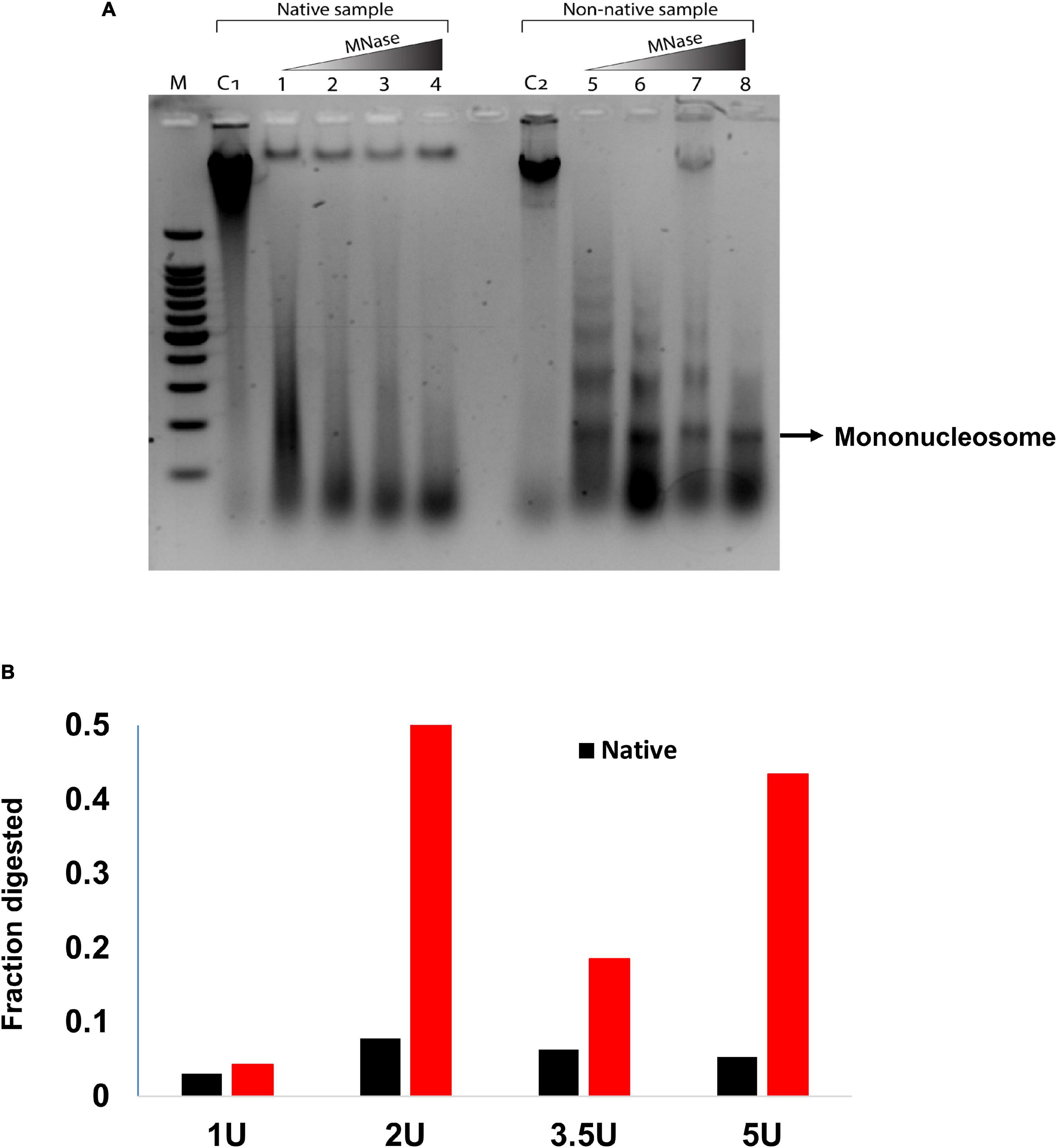
Figure 4. MNase digestion assay in the native (North America) and non-native (Kashmir Himalaya) range plants. (A) The chromatin was isolated from plant samples of both native and non-native range Conyza canadensis and subjected to MNase digestion with different enzyme concentrations as mentioned in “Materials and Methods” section. (B) The bar graph represents the percentage of mononucleosomal fractions digested in the native and non-native range plants.
Discussion
In this study, we observed a differential DNA methylation pattern at ABC transporter genes in the leaf samples of C. canadensis collected from its native range of North America and the non-native range of Kashmir Himalaya. The samples from the non-native range of Kashmir Himalaya showed a significantly lower DNA methylation level at ABC transporter genes as compared with the native samples of North America (Figure 1). Furthermore, DNA methylation patterns also showed variation across different populations within the native and non-native ranges. Studies have shown the involvement of differential methylation patterns in the widespread distribution of species in the non-native ranges (Ardura et al., 2018; Hawes et al., 2019). These studies revealed that epigenetic changes vary in heterogeneous habitats and these changes are heritable. Studies in invasive plant species have shown a positive correlation between differential methylation status and phenotypic plasticity (Zhang et al., 2016; Banerjee et al., 2019). This shows that epigenetic diversity may contribute to the phenotypic plasticity in invasive species independent of genetic diversity. As a consequence of DNA methylation, chromatin compresses into heterochromatin at the transcription start site and hence reduces the gene activity and its transcription (Buitrago et al., 2021). The reduced DNA methylation observed in C. canadensis in the non-native range of Kashmir Himalaya might explain the increased expression of ABC transporter genes. Various studies have shown a direct link between the environmental condition and induction levels of key genes including ABC transporters and that these ABC transporters could be playing a role in the establishment and spread of C. canadensis in Kashmir Himalaya (Tani et al., 2016). A link has been deciphered between DNA demethylation and invasion during the stage of expansion (Ardura et al., 2017). This may happen because of gene activation and reactivation of transposable elements redefining gene expression. As a result, demethylation may confer varying phenotypic plasticity and fitness. Plants have at least three different DNA methyltransferases, also called cytosine methyltransferases, which differ in structure and function and methylates DNA at cytosine (m5C) and adenine (m6A) (Finnegan and Kovac, 2000; Vanyushin, 2006). These various classes of DNMTS within the plant system, unlike the mammalian system, show differential methyltransferase activity and context-dependent effect on cytosine base (Finnegan and Kovac, 2000). Given that we observed the differential DNA methylation patterns in native (North America) and non-native (Kashmir Himalaya) C. canadensis, it will be an interesting area of research to profile the C. canadensis in other native and non-native ranges for DNA methylation and differential DNA methylation patterns. Since, we hypothesize that DNA associated Pol-II elongation may increase the RNA Pol-II binding at ABC transporter genes (Jonkers and Lis, 2015), we observed increased RNA Pol-II binding in non-native (Kashmir Himalaya) range C. canadensis as compared with native (North America) samples (Figure 2). This result is in line with the fact that reduced DNA methylation is mostly associated with increased RNA Pol-II binding and hence results in increased gene expression (Bender, 2004; Takeshima et al., 2009). Furthermore, we observed the loss of nucleosome at the ABC gene transporters in non-native samples of Kashmir Himalaya as indicated by comparative ChIP analysis of H3K14ac and H3 (Figure 3). This loss of nucleosomes is an indication of open chromatin in the non-native range of Kashmir Himalaya. Therefore, we also compared the chromatin dynamics in C. canadensis within its native (North America) and non-native range (Kashmir Himalaya) using MNase assay. In this study, nucleosomal occupancy and positioning have a critical impact on the expression and regulation of genes (Zhang et al., 2015). The MNase assay performed on the leaf samples of C. canadensis from native (North America) and non-native (Kashmir Himalaya) range revealed an interesting phenomenon of chromatin changes in non-native range plants of Kashmir Himalaya. The non-native plants of Kashmir Himalaya showed an increased mononucleosomal fraction as compared with native ones of North America (Figure 4). The increased mononucleosomal fraction is an indication of open and more accessible chromatin in non-native plants of Kashmir Himalaya that may modulate gene expression of these plants in the non-native range of Kashmir Himalaya (Kenchanmane Raju, 2020).
Taken collectively, our study has identified the differential DNA methylation patterns at ABC transporter genes in the native (North America) and non-native (Kashmir Himalaya) samples of C. canadensis. Non-native leaf samples of Kashmir Himalaya showed reduced DNA methylation at these genes, which was further associated with enhanced Pol-II binding. The combinatorial effect of reduced DNA methylation and increased RNA Pol-II binding along with open chromatin may enhance the transcriptional activity of ABC transporter genes and finally may increase the plant growth in different environmental conditions and may be one of the possible mechanisms through which plant becomes invasive in its non-native range of Kashmir Himalaya (Figure 5). Although our study deals with the chromatin dynamics at the ABC transporter genes, understanding the downstream events could be an exciting area of research.
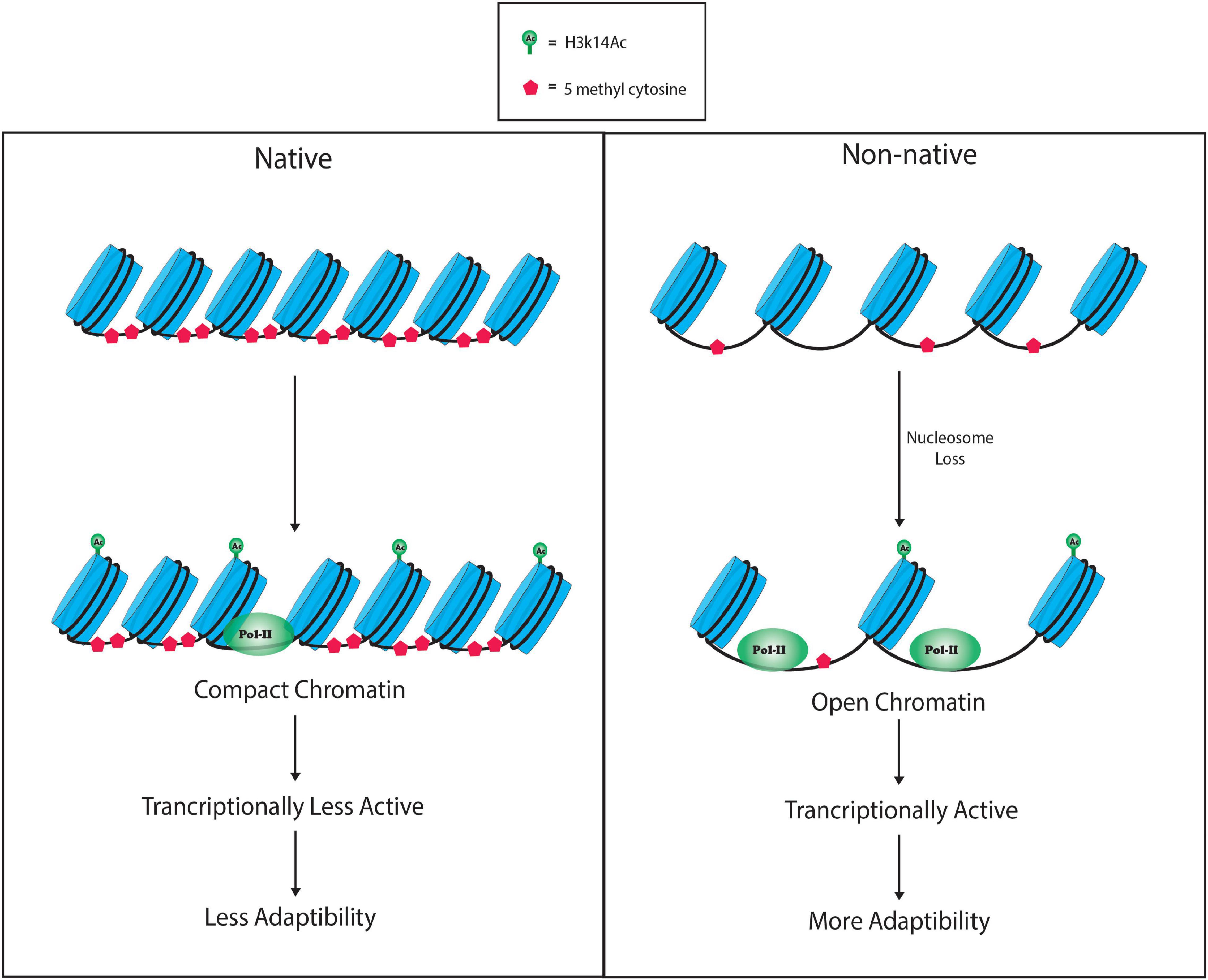
Figure 5. Model showing changes in chromatin organization in the native (North America) and non-native (Kashmir Himalaya) samples of C. canadensis. The model represents the compact and open chromatin at ABC transporter genes in the native and non-native range plants of C. canadensis. The non-native range plants showed open chromatin possibly due to decreased DNA methylation and increased H3K14ac occupancy at the ABC transporter gene and hence making the ABC transporter gene transcriptionally more active in the non-native ones as compared with native range plants of C. canadensis.
Data Availability Statement
The original contributions presented in the study are included in the article/Supplementary Material, further inquiries can be directed to the corresponding author/s.
Author Contributions
AS carried out the experiments. AS, ZR, and MA designed the experiments, interpreted the results, and wrote the manuscript. All authors edited and approved it.
Funding
ZR acknowledged the financial support of the University Grants Commission under the CPEPA scheme. The work was supported by grants from the Department of Biotechnology, Government of India (BT/PR22348/BRB/10/1625/2017), DST-SERB (CRG/2020/003632), RUSA 2.0, and DST-PURSE (SR/PURSE/2020/31) to MA.
Conflict of Interest
The authors declare that the research was conducted in the absence of any commercial or financial relationships that could be construed as a potential conflict of interest.
Publisher’s Note
All claims expressed in this article are solely those of the authors and do not necessarily represent those of their affiliated organizations, or those of the publisher, the editors and the reviewers. Any product that may be evaluated in this article, or claim that may be made by its manufacturer, is not guaranteed or endorsed by the publisher.
Acknowledgments
We thank Ishfaq Ahamd Pandith for his help in the preparation of figures and tables. AS acknowledges CSIR India for Senior Research fellowship.
Supplementary Material
The Supplementary Material for this article can be found online at: https://www.frontiersin.org/articles/10.3389/fevo.2021.781498/full#supplementary-material
References
Agarwal, G., Kudapa, H., Ramalingam, A., Choudhary, D., Sinha, P., Garg, V., et al. (2020). Epigenetics and epigenomics: underlying mechanisms, relevance, and implications in crop improvement. Funct. Integr. Genomics 20, 739–761. doi: 10.1007/s10142-020-00756-7/Published
Alonso, C., Becker, C., and Ramos-Cruz, D. (2018). The role of plant epigenetics in biotic interactions. New Phytol. 221, 731–737. doi: 10.1111/nph.15408
Ardura, A., Clusa, L., Zaiko, A., Garcia-Vazquez, E., and Miralles, L. (2018). Stress related epigenetic changes may explain opportunistic success in biological invasions in Antipode mussels. Sci. Rep. 8:10793. doi: 10.1038/s41598-018-29181-4
Ardura, A., Zaiko, A., Morán, P., Planes, S., and Garcia-Vazquez, E. (2017). Epigenetic signatures of invasive status in populations of marine invertebrates. Sci. Rep. 7:42193. doi: 10.1038/srep42193
Banerjee, A. K., Guo, W., and Huang, Y. (2019). Genetic and epigenetic regulation of phenotypic variation in invasive plants–linking research trends towards a unified framework. Neo Biota 103, 77–103. doi: 10.3897/neobiota.49.33723
Bender, J. (2004). DNA methylation and epigenetics. Annu. Rev. Plant Biol. 55, 41–68. doi: 10.1146/ANNUREV.ARPLANT.55.031903.141641
Buitrago, D., Labrador, M., Arcon, J. P., Lema, R., Flores, O., Esteve-Codina, A., et al. (2021). Impact of DNA methylation on 3D genome structure. Nat. Commun. 12:3243. doi: 10.1038/s41467-021-23142-8
Dauer, J. T., Mortensen, D. A., and Vangessel, M. J. (2007). Temporal and spatial dynamics of long-distance Conyza canadensis seed dispersal. J. Appl. Ecol. 44, 105–114. doi: 10.1111/j.1365-2664.2006.01256.x
Douglas, R., and Gaines, T. A. (2014). Glyphosate resistance?: state of knowledge. Pest Manag. Sci. 70, 1367–1377. doi: 10.1002/ps.3743
Eriksson, M. C., Szukala, A., Tian, B., and Paun, O. (2020). Current research frontiers in plant epigenetics: an introduction to a virtual issue. New Phytol. 226, 285–288. doi: 10.1111/nph.16493
Fabio, M., Michael, W., Dirk, S., and Tim-Christoph, R. (2009). Methylated DNA immunoprecipitation (MeDIP). Methods Mol. Biol. 507, 55–64. doi: 10.1007/978-1-59745-522-0_5
Finnegan, E. J., and Kovac, K. A. (2000). Plant DNA methyltransferases. Plant Mol. Biol. 43, 189–201.
Flatscher, R., Frajman, B., Schönswetter, P., and Paun, O. (2012). Environmental heterogeneity and phenotypic divergence: can heritable epigenetic variation aid speciation? Genet. Res. Int. 2012, 1–9. doi: 10.1155/2012/698421
Gallego-Bartolom, J. (2020). DNA methylation in plants: mechanisms and tools for targeted manipulation. New Phytol. 227, 38–44. doi: 10.1111/nph.16529
Ge, X., Avignon, D. A., Ackerman, J. J. H., and Sammons, R. D. (2014). In Vivo 31 P-nuclear magnetic resonance studies of glyphosate uptake, vacuolar sequestration, and tonoplast pump activity in glyphosate- resiatant Horseweed. Plant Physiol. 166, 1255–1268. doi: 10.1104/pp.114.247197
Hawes, N. A., Amadorou, A., Tremblay, L. A., Pochon, X., Dunphy, B., Fidler, A. E., et al. (2019). Epigenetic patterns associated with an ascidian invasion: a comparison of closely related clades in their native and introduced ranges. Sci. Rep. 9:14275. doi: 10.1038/s41598-019-49813-7
Jablonka, E., and Raz, G. (2009). Transgenerational epigenetic inheritance: prevalence, mechanisms, and implications for the study of heredity and evolution. Q. Rev. Biol. 84, 131–176. doi: 10.1086/598822
Jonkers, I., and Lis, J. T. (2015). Getting up to speed with transcription elongation by RNA polymerase II. Nat. Rev. Mol. Cell Biol. 16:167. doi: 10.1038/NRM3953
Kenchanmane Raju, S. K. (2020). Comparative profiling examines roles of DNA regulatory sequences and accessible chromatin during cold stress response in grasses. Plant Cell 32, 2451–2452. doi: 10.1105/TPC.20.00471
Kumar, S., and Mohapatra, T. (2021). Dynamics of DNA methylation and its functions in plant growth and development. Front. Plant Sci. 12:596236. doi: 10.3389/fpls.2021.596236
Liu, J., and He, Z. (2020). Small DNA methylation, big player in plant abiotic stress responses and memory. Front. Plant Sci. 11:595603. doi: 10.3389/fpls.2020.595603
Miryeganeh, M., and Saze, H. (2019). Epigenetic inheritance and plant evolution. Popul. Ecol. 62, 17–27. doi: 10.1002/1438-390X.12018
Moretti, M. L., Ala, R., Pearce, S., Morran, S., and Hanson, D. (2017). Transcription of putative tonoplast transporters in response to glyphosate and paraquat stress in Conyza bonariensis and Conyza canadensis and selection of reference genes for qRT-PCR. PLoS One 12:e0180794. doi: 10.1371/journal.pone.0180794
Nol, N., Tsikou, D., Eid, M., Livieratos, I. C., and Giannopolitis, C. N. (2012). Shikimate leaf disc assay for early detection of glyphosate resistance in Conyza canadensis and relative transcript levels of EPSPS and ABC transporter genes. Weed Res. 52, 233–241. doi: 10.1111/J.1365-3180.2012.00911.X
Pajoro, A., Muiño, J. M., Angenent, G. C., and Kaufmann, K. (2018). Profiling nucleosome occupancy by MNase-seq: experimental protocol and computational analysis. Methods Mol. Biol. 1675, 167–181. doi: 10.1007/978-1-4939-7318-7_11
Pass, D. A., Sornay, E., Marchbank, A., Crawford, M. R., Paszkiewicz, K., Kent, N. A., et al. (2017). Genome-wide chromatin mapping with size resolution reveals a dynamic sub-nucleosomal landscape in Arabidopsis. PLoS Genet. 13:e1006988. doi: 10.1371/JOURNAL.PGEN.1006988
Peng, Y., Abercrombie, L. G., Yuan, J. S., Riggins, C. W., Sammons, R. D., Tranel, J., et al. (2010). Characterization of the horseweed (Conyza canadensis) transcriptome using GS-FLX 454 pyrosequencing and its application for expression analysis of candidate non-target herbicide resistance genes. Pest Manag. Sci. 66, 1053–1062. doi: 10.1002/ps.2004
Perez, J. E., Alfonsi, C., Salazar, S., and Munoz, C. (2015). Ecological, genetic and epigenetic basis for bioinvasions. Articulo De Revision Agrobiologia 27, 167–177.
Rapp, R. A., and Wendel, J. F. (2005). Epigenetics and plant evolution. New Phytol. 168, 81–91. doi: 10.1111/j.1469-8137.2005.01491.x
Saleh, A., Alvarez-Venegas, R., and Avramova, Z. (2008). An efficient chromatin immunoprecipitation (ChIP) protocol for studying histone modifications in Arabidopsis plants. Nat. Protoc. 3, 1018–1025. doi: 10.1038/nprot.2008.66
Sandoval, J., Rodríguez, J. L., Tur, G., Serviddio, G., Pereda, J., Boukaba, A., et al. (2004). RNAPol-ChIP: a novel application of chromatin immunoprecipitation to the analysis of real-time gene transcription. Nuclear Acids Res. 32:e88. doi: 10.1093/nar/gnh091
Takeshima, H., Yamashita, S., Shimazu, T., Niwa, T., and Ushijima, T. (2009). The presence of RNA polymerase II, active or stalled, predicts epigenetic fate of promoter CpG islands. Genome Res. 19, 1974–1982. doi: 10.1101/GR.093310.109
Tani, E., Chachalis, D., Travlos, I. S., and Bilalis, D. (2016). Environmental conditions influence induction of key ABC-transporter genes affecting glyphosate resistance mechanism in Conyza canadensis. Int. J. Mol. Sci. 17:342. doi: 10.3390/ijms17040342
Travlos, I. S., and Chachalis, D. (2010). Glyphosate-resistant hairy fleabane (Conyza bonariensis) is reported in Greece. Weed Technol. 24, 569–573. doi: 10.1614/wt-d-09-00080.1
Vanyushin, B. F. (2006). DNA methylation in plants. Curr. Top. Microbiol. Immunol. 301, 67–122. doi: 10.1007/3-540-31390-7_4
Zhang, T., Zhang, W., and Jiang, J. (2015). Genome-wide nucleosome occupancy and positioning and their impact on gene expression and evolution in plants. Plant Physiol. 168, 1406–1416. doi: 10.1104/PP.15.00125
Keywords: ABC transporters, chromatin, epigenetics, DNA methylation, plant invasion, phenotypic plasticity, adaptation
Citation: Shah AT, Reshi ZA and Altaf M (2022) DNA Methylation of ABC Transporters Differs in Native and Non-native Populations of Conyza canadensis L. Front. Ecol. Evol. 9:781498. doi: 10.3389/fevo.2021.781498
Received: 22 September 2021; Accepted: 16 December 2021;
Published: 03 February 2022.
Edited by:
Tanvir Ul Hassan Dar, Baba Ghulam Shah Badshah University, IndiaReviewed by:
Arif Tasleem Jan, Baba Ghulam Shah Badshah University, IndiaMohd Dar, Indian Institute of Integrative Medicine (CSIR), India
Copyright © 2022 Shah, Reshi and Altaf. This is an open-access article distributed under the terms of the Creative Commons Attribution License (CC BY). The use, distribution or reproduction in other forums is permitted, provided the original author(s) and the copyright owner(s) are credited and that the original publication in this journal is cited, in accordance with accepted academic practice. No use, distribution or reproduction is permitted which does not comply with these terms.
*Correspondence: Mohammad Altaf, YWx0YWZiaGF0QHVvay5lZHUuaW4=