- 1Instituto de Investigaciones en Ecosistemas y Sustentabilidad, Universidad Nacional Autónoma de México, Morelia, Mexico
- 2Posgrado en Ciencias Biológicas, Universidad Nacional Autónoma de México, Ciudad de México, Mexico
Migratory birds can be familiar winter visitors of Neotropical cities. However, of the regional migrant species bird pool, only a few species are abundant in urban areas. Their presence inside cities has been positively related to green urban areas with high tree cover. However, urban elements like artificial lights can also attract them to cities. Habitat quality that enables energetic refueling for migrant birds is crucial in all their annual migratory stages. While some Nearctic cities offer a high-quality refueling habitat for migrant birds, we lack this information for Neotropical cities where migratory birds winter. In this study, we evaluate whether the urban green areas of a Neotropical city act as high-quality habitats for two abundant species of migratory warblers: Setophaga coronata and Leiothlypis ruficapilla. We assessed this by capturing birds inside three urban green areas and comparing their abundances, population structure, and individual quality (scaled-mass index—SMI) with individuals spending winter in natural vegetation habitats outside the city. We found that both species do not show differences in SMI between urban and non-urban sites. We also found that Setophaga coronata had a higher capture rate in urban than non-urban sites, while Leiothlypis ruficapilla had similar capture rates in both habitats. Our findings indicate that the urban green areas of cities can act as suitable quality habitat during winter for two abundant migrant birds. Our results indicate that cities can have a higher conservation potential for a declining and sensitive group such as the Nearctic-Neotropical migratory birds than previously thought. We also point out the need to acknowledge that migratory species can be urban dwellers.
Introduction
Urban areas have become the most important habitat for humans, harboring more than half of our worldwide population (Grimm et al., 2008; Elmqvist et al., 2019). Urbanization transforms local habitats by replacing natural features with human-made structures that maintain large resident human populations and their emergent activities (Grimm et al., 2008; Forman, 2014). They concentrate economic activities, pollution emissions, and a large diversity of resources with a local, regional or global origin (Forman, 2014; McPhearson et al., 2016). Cities modify biogeochemical and social processes and weather and biodiversity patterns at local and regional scales (McKinney, 2002; Grimm et al., 2008).
While the concept of urban ecosystem is not new (Stearns and Montag, 1974), cities tend to be perceived by their human inhabitants not as ecologically functional systems but as sites with high levels of environmental degradation caused by the human disturbance of natural processes (Grimm et al., 2017; Groffman et al., 2017). This negative perception of urban systems is reinforced by the fact that some animal species from the regional pool cannot maintain viable populations inside urban areas (Fischer et al., 2015; Aronson et al., 2016). Still, due to their high environmental heterogeneity and emergent properties, cities can offer crucial resources like food (Tryjanowski et al., 2015; Seress et al., 2020), water (Barbosa et al., 2020), and shelter (Davis et al., 2011), and limit predation to vertebrate species (Zuñiga-Palacios et al., 2021). They also offer more stable climatic conditions year-round than those present in the habitats that surround them (Leveau, 2018; Stewart and Waitayachart, 2020). Thus, they can act as critical habitats for many regional species (Aronson et al., 2014).
The importance that urban ecosystems have for different species of the regional fauna is species-specific. For some species, they can represent an ecological trap (Zuñiga-Palacios et al., 2021). At the same time, they can act as a suitable environment for others (Møller, 2009; Spotswood et al., 2021). The interaction between the functional characteristics of wildlife species and the local context of the urban habitat determines which species are filtered, given their phylogenetic and functional traits, and how their populations respond to urbanization (Aronson et al., 2016; Evans et al., 2018). Changes in wildlife behavior to cope with human presence can also be crucial at the species and the individual level (Sol et al., 2018). Furthermore, when the ecological systems surrounding a city become degraded, local fauna can perceive urban habitats as suitable places to survive (Davis et al., 2011; MacGregor-Fors et al., 2020; Zuñiga-Palacios et al., 2021). Consequently, the role of cities as ecological traps or as suitable habitats for regional fauna requires a species-specific assessment, focusing on the individual quality of organisms and their population attributes (Spotswood et al., 2021; Zuñiga-Palacios et al., 2021).
Nearctic-Neotropical migrant birds (hereafter referred to as migrant birds; Hayes, 1995) can be abundant winter visitors of Neotropical urban areas. However, only a few species tend to be present inside cities, occurring mainly in urban green areas with abundant tree features (MacGregor-Fors et al., 2010; Carbó-Ramírez and Zuria, 2011; Amaya-Espinel and Hostetler, 2019). As a result, urbanization has been considered harmful for this group of birds. Recent studies have shown that this view is not entirely accurate, with migratory birds being able to use urban habitats as stopover sites to refuel their fat reserves similarly to how they do it in non-urban habitats (Seewagen and Slayton, 2008; Seewagen et al., 2011). Due to their capacity to use an extensive array of habitats during their annual cycle and their flight and dispersal capacities, migrant birds can occupy and use cities more extensively than resident species (Zuckerberg et al., 2016; La Sorte et al., 2020).
In this study, we focus on urban green areas’ role in supporting the migrant bird populations of two species of warblers during their overwintering stage: Setophaga coronata and Leiothlypis ruficapilla. Migratory birds like these species need to rebuild their body condition after their long-distance fall migration (Sherry and Holmes, 1996; Holmes, 2007). This requires good quality habitats that allow them to survive their overwintering period and prepare for their northbound spring migration (Schuster et al., 2019; Albert et al., 2020). As a result, these species are ideal models for evaluating the quality of green urban habitats compared to those present around cities. To evaluate the urban habitat quality, we principally focused on the bird’s body condition because the first response of wildlife to disturbance occurs at the physiological level modifying their individual quality (Chávez-Zichinelli et al., 2013; Zuñiga-Palacios et al., 2021).
Materials and Methods
Study Site
We studied migratory warblers in urban green areas (UGAs) of the city of Morelia, inside the urban matrix, and in native vegetation areas with low human disturbance located in the vicinity of the city (non-urban sites from hereafter; Figure 1). The city of Morelia is the capital of the state of Michoacán and presents a population of 850,000 inhabitants (Instituto Nacional de Estadística, Geografía e Informática [INEGI], 2020). It is located in central-western Mexico (19° 42′08″N 101° 11′34″ W; Figure 1), within the biogeographic province known as the Trans-Mexican Volcanic Belt (Morrone, 2019). This biogeographic zone is considered a critical region for conserving overwintering migratory birds, as it has the highest winter densities of these birds in North America (Bayly et al., 2018). It is also a critical stopover region used by migrant birds during both their fall and spring migrations (La Sorte et al., 2016; Bayly et al., 2018).
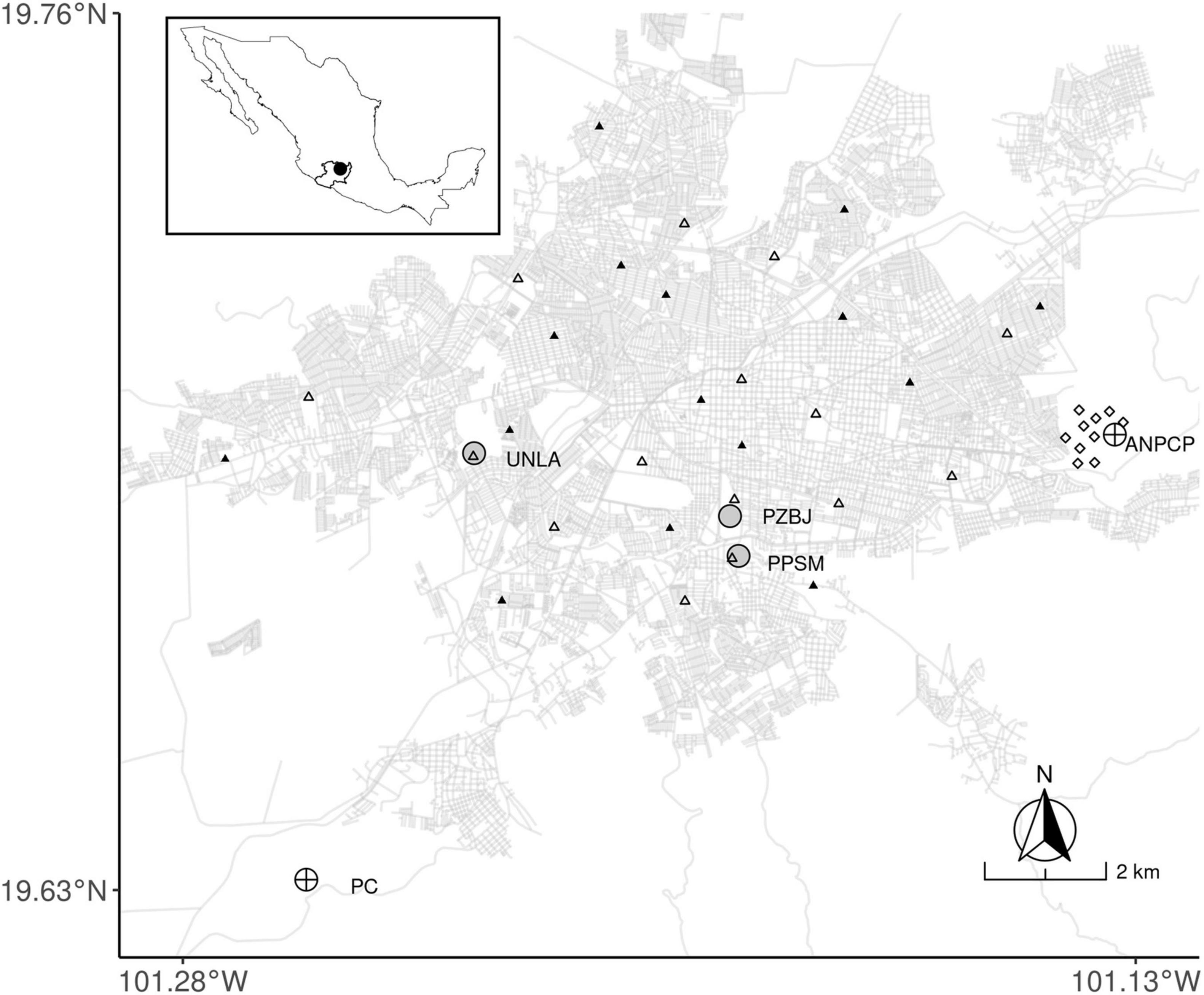
Figure 1. Map of the study area with all the capturing (circles) and bird censuses (triangles and rhombuses) sites. The urban area of the city of Morelia is represented by its streets and roads. Two of our urban green areas (PZBJ and PPSM) are situated closer to the center of the city, while the third one (UNLA) was closer to the city edge (gray circles). The non-urban vegetated site ANPCP is located just at the periphery of Morelia, while the PC site is ∼16 km from the city (crossed circles). Urban sites for bird censuses are shown as black triangles (>50% impervious surfaces), while the urban green sites are shown as white triangles (50% green cover). The non-urban bird censuses were performed in the ANPCP and are shown as rhombuses.
We selected sites for capturing birds and for conducting bird censuses. Our field work took place during November and December 2020. Our study sites for capturing birds were based on their environmental characteristics such as abundant tree coverage, spatial location, the security level they presented for our team members to work there, and the presence of access limitations that control the number of visitors. We compared UGAs and non-urban sites with natural vegetation. Our selected sites acted as replicates of this two conditions. UGAs were represented by three replicates: (1) Santa María water treatment plant (Planta Potabilizadora Santa María: PPSM; 19° 40′46.0 “N, 101° 11′34.6″W), 2) Morelia’s Benito Juárez Zoo (Parque Zoológico Benito Juárez: PZBJ; 19° 41′08.3 “N, 101° 11′37.0″ W), and 3) the vegetated grounds of a university (Universidad Latina de América: UNLA; 19° 41′42.1 “N, 101° 14′04.3″ W). Non-urban vegetated sites were represented by two replicates: (1) Cerro Punhuato State Protected Natural Area (Area Natural Protegida Cerro Punhuato: ANPCP; 19° 41′53.0 “N, 101° 08′09.4″ W); and (2) a patch of pine-oak vegetation located within the facilities of the Cointzio reservoir, administered by the National Water Commission (Presa Cointzio: PC; 19° 37′52.1 “N, 101° 15′37.3″ W). The spatial location of all sampling sites is shown in Figure 1. We were unable to include a third replica due to access limitations to other suitable sampling areas. The characteristics of all our bird capturing sites are the following:
Urban green areas:
• The PPSM site has an area of 4 ha. Vegetation covers 55% of the area (grasses 15%, and trees 40%). The rest of the area is covered by parking lots, concrete esplanades, roads, and buildings (25%), and water treatment infrastructure (20%). The most common tree species include both native (Fraxinus uhdei, Salix bonplandiana) and exotic species (Eriobotrya japonica, Ficus benjamina, Eucalyptus camaldulensis, and Casuarina equisetifolia). Shrub cover under the trees is low (10%) and includes several species. The area has permanent access to water resources in the form of water treatment ponds and channels. The human activities carried out in this area include maintaining gardens (grass areas) and the water treatment infrastructure. Residential areas surround the site with a population density of 4.13 people/km2 (Instituto Nacional de Estadística, Geografía e Informática [INEGI], 2020).
• The PZBJ site has 24 ha, with vegetation covering 60% of the area (pastures and open gardens 30%, and trees 30%). Shrub cover under trees and in open areas is low and mostly limited to ornamental species used as path walls. The urban infrastructure consists of roads and buildings, covering 30% of the area. The remaining 10% of the area is cover by an artificial lake. Trees are sparse and large, including native (Fraxinus uhdei) and exotic species (Eucalyptus camaldulensis, Casuarina equisetifolia, Spathodea campanulata, Ficus benjamina, and Phoenix canariensis). Animals are exhibited in fenced enclosures, and their food is available to birds and other wildlife. The artificial lake, a small stream, and animal water troughs are abundant and permanent water sources. During our study, the zoo was closed due to the COVID-19 pandemic, and human activities were limited to essential maintenance by zoo staff. Nonetheless, this was the sampled UGA with the highest human activity. The zoo’s surrounding urban area includes two main avenues, two educational institutions, a public park, and residential and commercial areas with a population density of 6.58 people/km2 (Instituto Nacional de Estadística, Geografía e Informática [INEGI], 2020).
• The vegetated grounds of the UNLA have a size of 20 ha. From this area, 20% was occupied by open areas with grass used for sports activities, 10% by a pond, and the rest (70%) is an area with tree cover that is susceptible to flooding and part of the year acts as a wetland. The dominant tree species are the native species Salix humboldtiana, S. bonplandiana, Fraxinus uhdei, and Taxodium mucronatum. Also, there are some scattered individuals of non-native trees (Eucalyptus camaldulensis and Casuarina equisetifolia). Shrub cover is low, with scattered individuals. Water resources are available all year long and include the pond, some water channels, and the wetland. The urban surroundings of the UNLA include the university buildings and parking lots, vegetated lots, and residential and commercial areas with a population density of 6.73 people/km2 (Instituto Nacional de Estadística, Geografía e Informática [INEGI], 2020). Human activities were restricted due to the COVID-19 pandemic and limited to maintenance activities by the university staff. Previously to the pandemic, various sports and cultural activities were carried out in this area regularly.
Non-urban sites:
• The ANPCP is located on the periphery of the city of Morelia and covers an area of 117 ha. Restoration activities have been carried out in this protected area for more than 20 years. The area presents patches of Quercus forest, gallery forest, subtropical scrub, and exotic vegetation. Dominant Quercus species are Q. obtusata, Q. castanea, Q. deserticola, and Q. magnolifolia. Other dominant tree species are Bursera cuneata, B. bipinnata, Ipomea murocoides, Acacia farnesiana, A. pennatula, Condalia velutina, and Opuntia spp. Tree species such as Fraxinus uhdei and non-native species such as Eucalyptus camaldulensis and Casuarina equisetifolia occurred scattered through the area and on its urban periphery. Shrub cover is diverse and abundant below the tree canopy. The site has two main buildings that serve as a managing station and a telecommunications station and cover less than 1% of the area. Some unpaved roads extended throughout the area, covering less than 5% of its surface. Human activity on the site is low and limited to individuals or small groups of people who visit the area to perform recreational or educational activities. The protected natural area is surrounded by patches of native vegetation (25%), non-intensive rainfed crops (25%), and the rest (50%) presents residential areas with a population density of 5.21 hab/km2 (Instituto Nacional de Estadística, Geografía e Informática [INEGI], 2020) and a 4-lane highway.
• The PC site has an extension of 16 ha. It is located 4.5 km from the edge of the city of Morelia. The site is fenced and has restricted access. Nearly all the area is covered by remnant pine-oak vegetation. However, it has an open area with pastures (10%), a permanent water stream (5%), and a few buildings and a paved road covering less than 5% of the area. The vegetation structure of the shrub and tree layers is complex and diverse. Species of Pinus and Quercus characterize the arboreal vegetation. There are also individuals of Fraxinus uhdei, Salix sp., Bursera cuneata, B. bipinnata, Ipomea murocoides, Acacia farnesiana, A. pennatula, Condalia velutina, Opuntia spp., and a few individuals of the invasive tree species Eucalyptus camaldulensis. There is very little human activity in the area. The area is surrounded by native forest, cultivated areas, a few houses, and a reservoir.
We determined the density of our selected migrant bird species (see in the section above) using 10 min unlimited radius point counts with distance estimations (Ralph et al., 1996). All bird records were performed by the same observer (A. C-M). We only included in our analyses the birds we detected inside a 50 m radius. A minimum distance of 250 m separated all point counts. We deployed 30 random points within the city of Morelia: 15 points in urban green areas (>50% green cover) and 15 urban points (>50% impervious surface cover). The point count in urban green areas included one point count in each of the three UGAs where we capture birds. Due to the size of the UGAs where we captured birds, we were forced to sample several additional locations to have independence among our point counts. We also deployed ten points within the ANPCP non-urban site. Distances of bird records were obtained with a range-finder (Nikon Rangefinder Forestry Pro 550). We used these records to calculate the density of S. coronata and L. ruficapilla in the three habitat types. The location of our bird censuses sites is shown in Figure 1.
We described the habitat components of both our bird capture and bird censuses sites. We characterized both the green and gray habitat elements using 25 m radius sampling points located around each of the ten mist nets we used to capture birds and around all point count sites. The green elements quantified in both habitats were the number of arboreal and shrub morphospecies as their species richness, respectively, the densities of trees and shrubs, tree height (m), tree diameter at chest height (DBH; cm), and the minimum and maximum shrub height (m). The gray elements quantified in both habitats were the number of buildings, their maximum height, and the number of artificial elements that were not buildings. We measured the proportion of cover represented by the tree canopy, the shrub layer, herbaceous layer, bare soil, and buildings for each habitat sampling point. Additionally, we quantify the minimum and maximum background noise levels (dB) using a sound level meter (EXTECH EN300). We obtained all measured heights and distances with a range-finder.
To determine how similar our sampling sites were (for UGAs and non-urban sites), we compared the habitat elements of all sampling sites using a principal component analysis (PCA), followed by an oblimin rotation. Additionally, we included the ten most urbanized bird censuses point count sites (≥50% of urban impervious and building coverage) and ten natural vegetation sites located away from the city (∼35 km; Querendaro county) as a reference to compare our sites. A throughout explanation of our habitat characterization and their contrast using the oblimin rotated PCA is provided in Supplementary Material.
Focal Species
We focused on two selected migratory warblers: Setophaga coronata and Leiothlypis ruficapilla. Both species are abundant in urban and vegetated non-urban areas during winter in the city of Morelia (MacGregor-Fors et al., 2010; personal observations). Setophaga coronata is a migratory warbler that breeds in mature coniferous and mixed coniferous-deciduous forest habitats of temperate zones north of the continent, wintering in a large diversity of Neotropical habitats (oak-pine forests, forest edges, and open areas including anthropized habitats; Howell and Webb, 1995; Liu and Swanson, 2015). It forages for insects both in trees and open grass areas (Greenberg, 1979) and is considered the most opportunistic species within the Setophaga group due to its plasticity in foraging strategies (Greenberg, 1979). We evaluated the Setophaga coronata auduboni subspecies, because this is the only subspecies present in western Mexico. Leiothlypis ruficapilla is a species that breeds in second-growth, open deciduous, or mixed-species forests of temperate zones north of the continent and winters primarily in low deciduous open forest and urban residential areas (Howell and Webb, 1995). It forages for insects mainly in the shrub layer (Howell and Webb, 1995). Moreover, both birds respond to different habitat characteristics through changes in their abundances or body weight during migration (Rodewald and Brittingham, 2007) and in their overwintering territories (Greenberg et al., 1997; Murphy et al., 2001).
Habitat Quality for Migrant Birds
We evaluated how important UGAs are for wintering migrant warblers directly by evaluating the body condition of individuals of both species and indirectly by determining their abundances. We compared abundances because they tend to reflect habitat quality (Bock and Jones, 2004). We conducted both assessments relative to our sampled non-urban areas. To evaluate the individual body condition, we captured individuals using ten mist nets per site (12 × 2.5 m nets with a mesh size of 16 mm) for 4 weeks during the winter period. We capture birds from November 23 to December 19, 2020. During this period, migrant birds have established winter territories after their southbound migration, and their body condition is dependent on their wintering habitat (Sherry and Holmes, 1996; Bayly et al., 2018). Additionally, no migrant birds pass through the area during this period while migrating to other regions (La Sorte et al., 2016, 2017). We operated nets from sunrise to 11:30 a.m. with a team of 3–5 people, conducting net-rounds every 30 min (Ralph et al., 1996). All sites were sampled at least one time per week during the sampling period. Because our sampling effort differed slightly among sites, we calculated and standardized the catch-per-unit effort to the number of individual captured per 100 net h at each site (captures/100 net h). All captured migratory birds were identified and tagged with numbered aluminum rings from the BIRDS.MX system. We took the following data from each bird: age (only in Setophaga coronata: subadult and adult), sex (males or females), body mass (± 0.01 g: Ohaus digital Scout Pro electronic scale), wing chord length (± 0.5 mm: millimeter ruler with wing stop), tarsal length (± 0.01 mm: millimeter Vernier), flight-feather wear, number of ectoparasites in its wing feathers, and levels of fat reserves (following Kaiser, 1993).
We assessed the individual body condition for each migrant bird species by calculating a modified scaled-mass index (SMI; Peig and Green, 2009), following Brodeur et al. (2020). The modified SMI was calculated by substituting the scaling factor obtained by modeling a standardized major axis regression (SMA) to a scaling factor obtained by modeling a non-linear power function regression (Brodeur et al., 2020). The SMI adjusts the mass of all individuals of each species, given a scaling exponent from the relationship of mass and a body size measurement (Peig and Green, 2009). As recommended by Peig and Green (2009), we choose wing chord length as body size measurement as it was the best-correlated measurement of body size with the mass on a log-log scale for both species (S. coronata: r = 0.51, P ≤ 0.001 and L. ruficapilla: r = 0.4, P ≤ 0.01). The SMI must be independent of body size measurement (Brodeur et al., 2020). Our unmodified calculated SMIs for both species were negatively correlated with our selected body size measurement (S. coronata: r = −0.49, P ≤ 0.001 and L. ruficapilla: r = −0.56, P ≤ 0.001). However, our modified SMIs were uncorrelated to their respective body size measurements (S. coronata: r = 0, P = 1 and L. ruficapilla: r = 0, P = 1). Thus, we used the modified SMI (hereafter referred to as just SMI) as body condition index. To calculate the scaling factor, we removed three individuals of each migrant bird species due to mass measurement errors during processing. We also tested if individuals of each species grouped by sex or habitat had different slopes but they did not differ. We obtained the SMI scaling factor through the power function of mass and body length for both migrant bird species (S. coronata: y = −0.66 × ^0.729 and L. ruficapilla: y = 0.51 ×^0.396). Finally, we assessed the SMI association to energy stores in each migrant bird species, through their Pearson correlations with our fat category index with a confidence interval of 95%.
Data Analysis
The density of each species recorded by our survey point counts was contrasted between habitat types (urban, UGAs, and non-urban sites). We assessed this contrast by fitting a negative binomial and a Poisson generalized linear model (GLM) for S. coronata and L. ruficapilla, respectively, with habitat type as a fixed factor. Posteriorly, for each model, we computed the estimated marginal means and performed a pair-wise contrast of our factor categories considering a 95% confidence level.
We compared the individual captures/100 net h, the individual SMI, the flight-feather wear, and the ectoparasite load of each migrant warbler species between our two habitat conditions (UGAs and non-urban) and between sexes. We fitted GLMs to evaluate individual captures/100 net h, linear mixed models (LMM) to evaluate the individual SMI, and generalized linear mixed models (GLMM) to evaluate flight-feather wear and ectoparasite load. All models had habitat type and sex as a fixed factor, and mixed models had site as a random factor to allow for similarity of measurements from the same sites. For each of the four measurements of each species assessed, we fitted a pool of five models: a multiplicative, an additive, a single term structure of factors (one for sex and habitat, separately), and also a null model. Models evaluation was conducted through AICc. We selected models with the lowest AICc (Δ AIC < 2). We used Poisson and negative binomial models to assess the individual captures/100 net h, the flight-feather wear, and the ectoparasite load, given that the data was from counts and was non-normally distributed. We choose negative binomial models over Poisson models if overdispersion was detected in the latter. To perform our SMI analysis, we first checked the data for normality for each bird species through a Shapiro-Wilk test (S. coronata: p > 0.05 and L. ruficapilla: p > 0.05). We checked all selected models through Q-Q plots. Our analysis that assessed the individual captures/100 net h, the individual SMI, the flight-feather wear, and the ectoparasite load in both our selected bird species did not include data from recaptures. All analyses were performed through R language (R Core Team, 2021).
Results
Habitat Differences Among Sites
The classification of our sampling sites by their habitat characteristics (PCA oblimin rotated analysis) showed a clear separation of urban areas, UGAs, and non-urban areas by the values of dimension 1 and dimension 2 (71.4% of variance explained; Figure 2). Based on the oblimin rotated loadings (Figure 2), the urban site negative values from dimension 1 and the positive values from dimension 2 were principally driven by the number of constructions, a dominant proportion of construction land cover, and the maximum background noise levels (max. dB). The Dimension 2 of our analysis separated UGAs from non-urban areas. UGAs showed higher minimum background noise levels (min. dB), a higher proportion of bare ground land cover, and a greater density of artificial elements (elements/area) relative to non-urban sites. Non-urban sites had a more significant proportion of land covered by herbs and greater values of shrub species richness, abundances, and land cover proportion (Figure 2). From all UGAs, UNLA showed habitat characteristics closest to non-urban sites, with the least amount of constructions and artificial elements. However, it did not have an abundant shrub layer. The loading values of the oblimin rotated PCA dimension 1 and dimension 2 are shown in Supplementary Material.
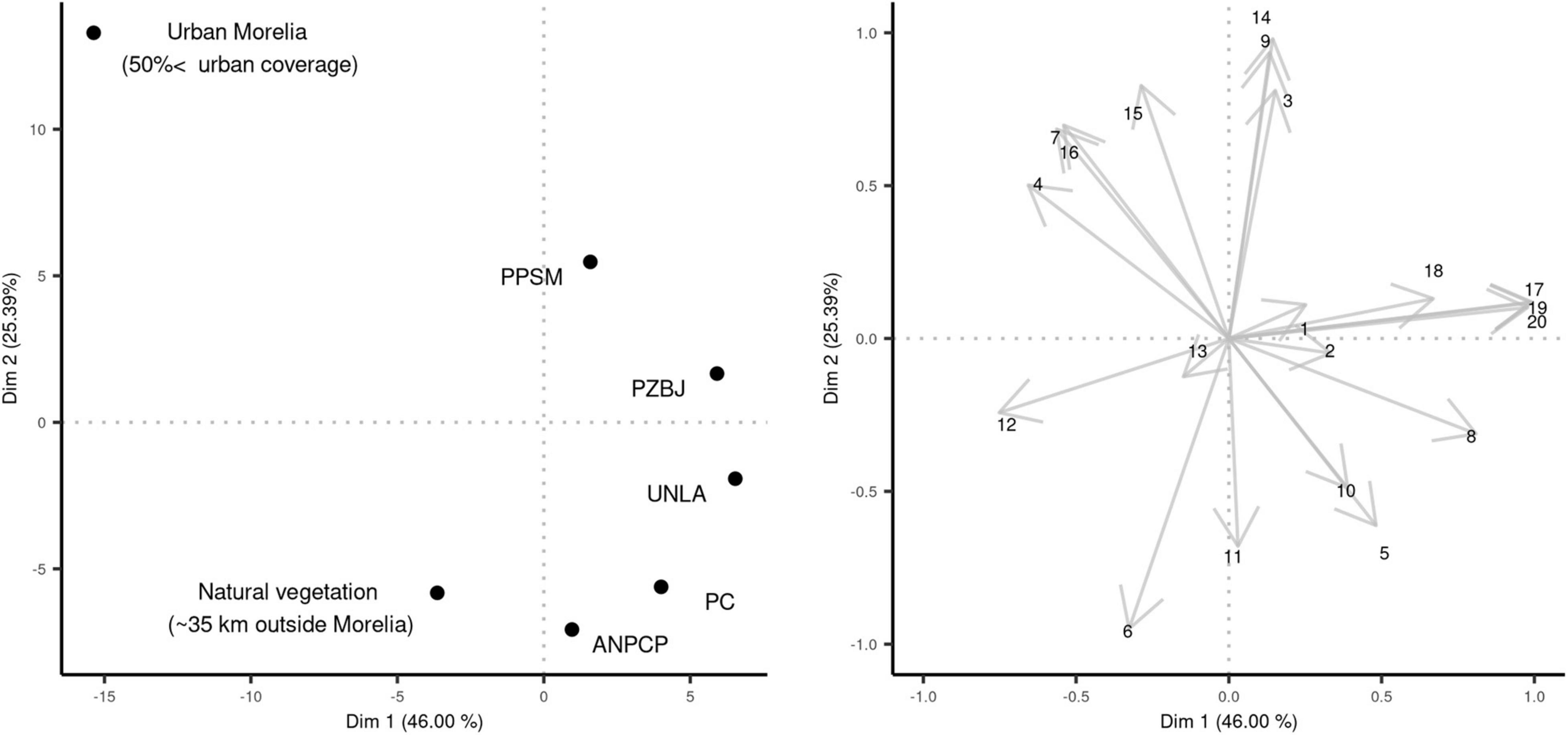
Figure 2. Classification of our study sites by their habitat characteristics assessed by the dimension 1 and dimension 2 (46 and 25.4% of variance explained, respectively) of the oblimin rotated PCA. The (left panel) shows a biplot of the rotated scores for the sites. The (right panel) shows a biplot of the habitat characteristics measured in each site as the oblimin rotated PCA loadings: Tree sp. richness (1), Tree abundances (2), Minimum background noise (dB; 3), Maximum background noise (dB; 4), Herbs land cover proportion (5), Shrubs land cover proportion (6), Construction land cover proportion (7), Tree land cover proportion (8), Bare ground land cover proportion (9), Shrub sp. richness (10), Shrub abundances (11), Minimum shrub height (m; 12), Maximum shrub height (m; 13), Number of artificial elements (14), Maximum construction height (m; 15), Density of constructions (16), Mean tree height (m; 17), Standard deviation of tree height (cm; 18), Mean tree diameter at breast height (19), and Standard deviation of tree diameter at breast height (20).
Points Count Records and Bird Captures
Our bird census for S. coronata recorded 94 individuals in urban green sites, 55 individuals in non-urban sites, and three in urban sites. For L. ruficapilla we recorded twelve individuals in UGAs, 13 individuals in non-urban sites, and none in urban sites. The point count density of both migrant species and their contrast among habitats showed that urban sites had fewer individuals of S. coronata than the non-urban and urban green habitat types (Table 1 and Figure 3). Densities for S. coronata were similar between UGAs and non-urban sites (Table 1 and Figure 3). For L. ruficapilla, our analyses show no differences in the densities among habitat types (Table 1 and Figure 3).
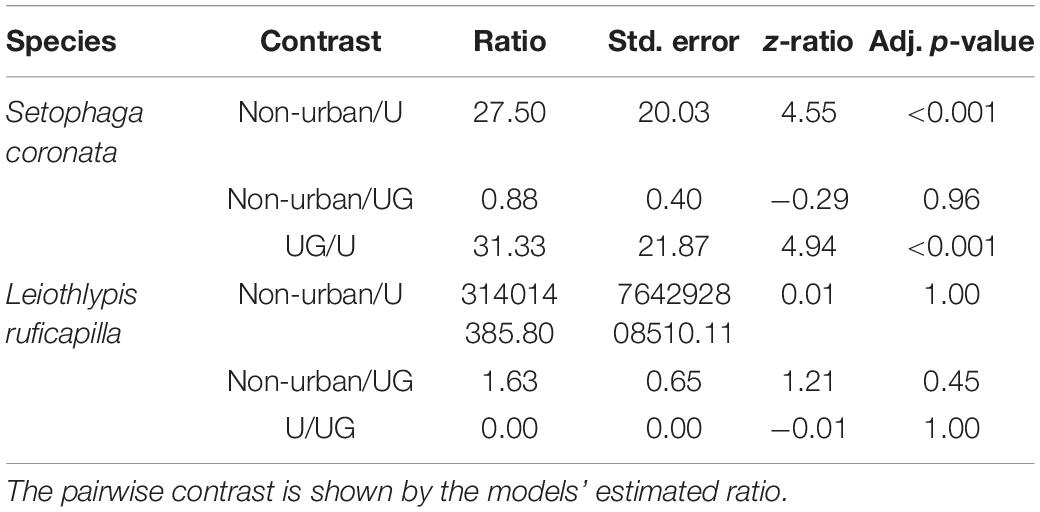
Table 1. Differences of bird densities by habitat type (U, Urban and UG, urban green) of both overwintering warbler species, assessed by a pairwise contrast of the negative binomial (S. coronata) and the Poisson (L. ruficapilla) GLMs estimated marginal means.
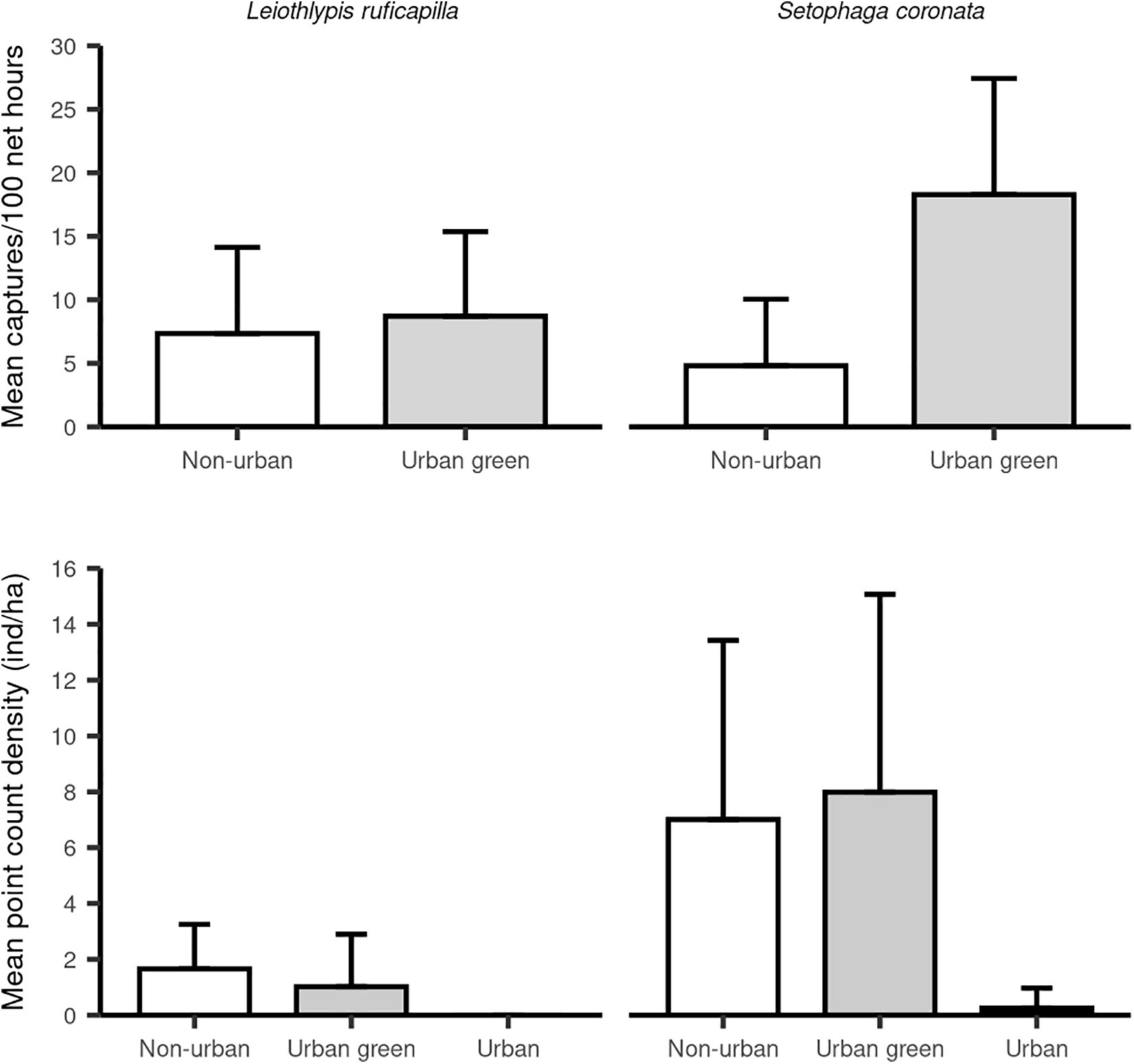
Figure 3. Capture rates (captures/100 net h) and densities (ind/ha) of Setophaga coronata (right panels) and Leiothlypis ruficapilla (left panels). Upper panels represent mean captures/100 net h in urban green areas (three sites) and non-urban areas (two sites). Lower panels show mean densities (ind./ha) in urban green areas (>50% green cover), urban sites (>50% impervious surfaces) and non-urban areas. Individuals/100 net h for both species did not differ between habitats. However, S. coronata presented slightly higher capture rates in urban-green areas. Point counts in urban sites did not detected individuals of L. ruficapilla and only a few individuals of S. coronata. Both species had similar abundances between urban green and non-urban areas.
In our banding sites, we captured a total of 95 individuals of S. coronata and 72 individuals of L. ruficapilla. For S. coronata we captured 48 females and 47 males, while for L. ruficapilla we captured 25 females, 39 males, and eight individuals whose sex was not identified. By habitat, we capture 82 individuals of S. coronata in UGAs, and 13 individuals in non-urban areas. In comparison, we captured 38 individuals of L. ruficapilla in UGAs and 34 individuals in non-urban areas. We recaptured two individuals of S. coronata and four individuals for L. ruficapilla. After their original capture day, two S. coronata were recaptured in UGAs 7 and 14 days later. We did not recapture any individual of S. coronata in a non-urban area. For L. ruficapilla, we recaptured two individuals 14 days after their original capture in UGAs. For non-urban areas, one individual of this species was recaptured 7 days after its capture and another 14 days later. Mean individual captures/100 net h for both species by habitat type and sex are presented in Table 2. Capture rates of S. coronata differ by habitat type, being slightly higher in UGAs relative to non-urban areas (Tables 3, 4 and Figure 3). Capture rates of L. ruficapilla did not differ between habitats, but they did by sex (Table 3 and Figure 3), with males presenting higher capture rates than females (Table 4 and Figure 3).
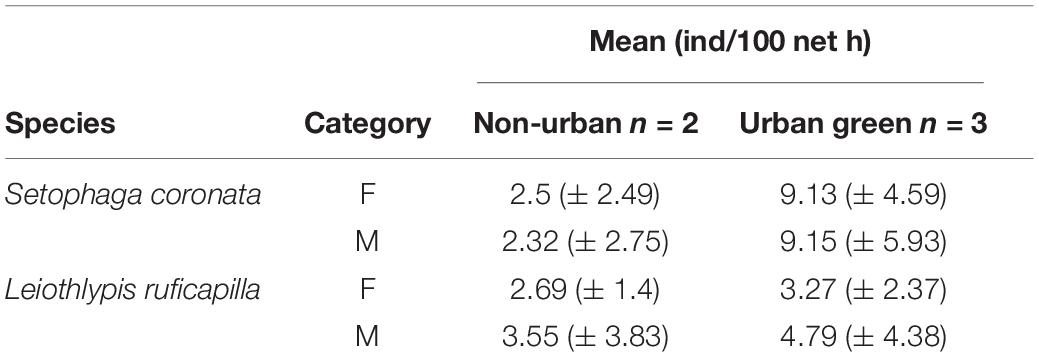
Table 2. Captures rates (individuals/100 net h) of both focal migrant bird species, by sex (F, Females and M, Males) and by habitat type.
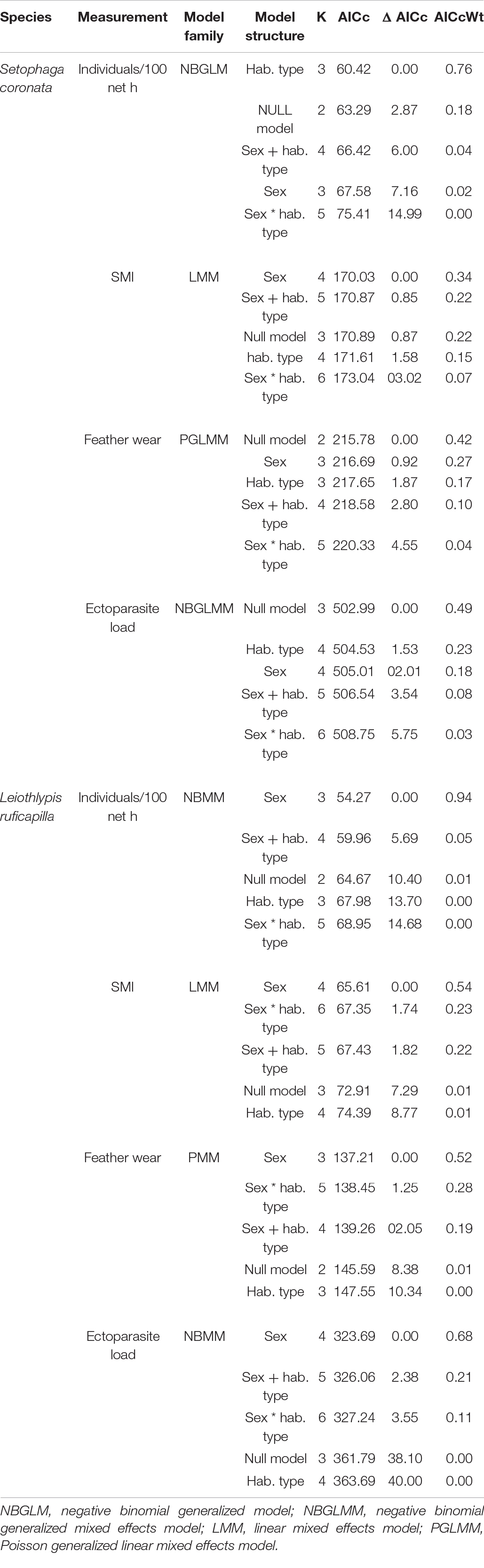
Table 3. S. coronata and L. ruficapilla GLM, LMM, and GLMMs’ pools that evaluate the role of habitat type and sex on their capture rates (individuals/100 net h), body condition (SMI), flight-feather wear and ectoparasite load through the Akaike criteria.
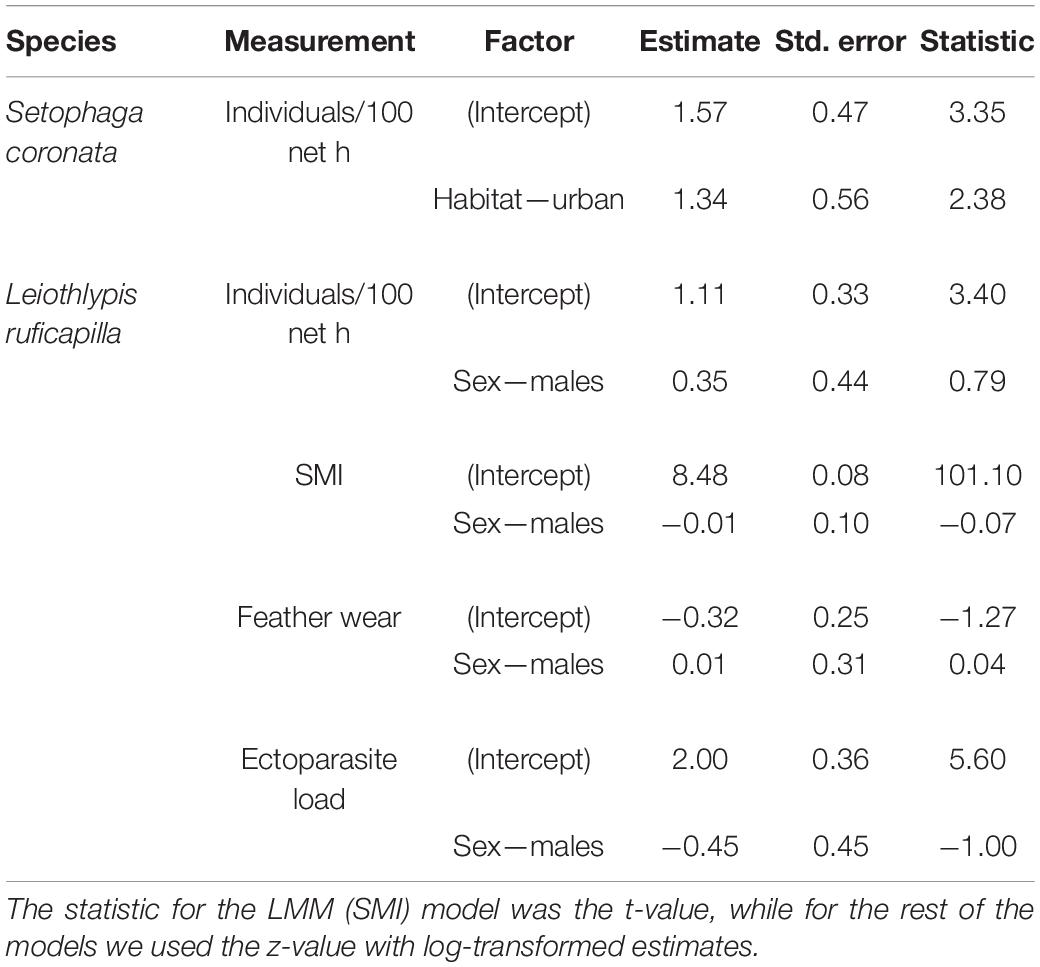
Table 4. Models with the lowest AICc selected from de model pool with an effect given by our fixed factors: habitat type (Urban green and non-urban areas) and sex.
Evaluation of Individuals
Both species’ body condition (SMI) did not differ between UGAs and non-urban areas (Table 3 and Figure 4). S. coronata did not present differences by habitat type or sex in SMI, flight-feather wear, and ectoparasite load. For this species, the null model was indistinguishable from other models in their respective pool (Table 3). In L. ruficapilla our analyses indicate differences by sex in SMI, flight-feather wear, and ectoparasite load (Table 3). Furthermore, the pool of models of the SMI and the flight-feather wear in L. ruficapilla showed modest support for differences given by the interaction of habitat type and sex (Δ AICc < 2; Table 3 and Figure 4), but showed an AICc weight lower than 50% (Table 3). Our selected models show that L. ruficapilla males had slightly lower SMI values, more flight-feather wear, and lower ectoparasite loads, regardless of habitat type (Table 4). SMI and values of fat categories were positively correlated in S. coronata (r = 0.21, df = 90, p ≤ 0.05; Figure 5). However, while they were not correlated in L. ruficapilla, they showed a positive trend (r = 0.21, df = 64, p = 0.08; Figure 5).
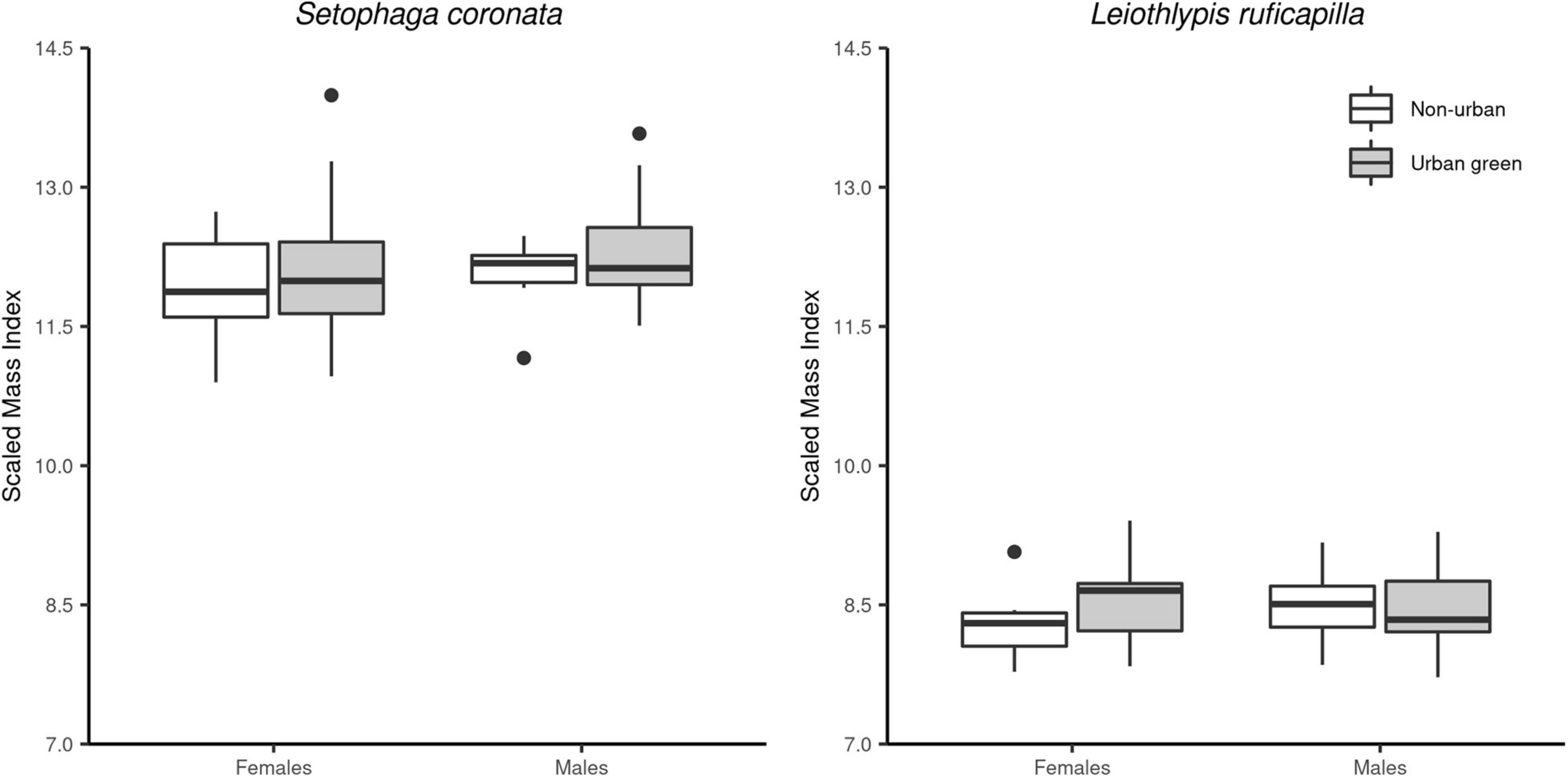
Figure 4. Calculated standardized-mass index (SMI) for S. coronata (left panel) and L. ruficapilla (right panel) as a measurement of body condition. We show SMI values for both migrant bird species by sex in both habitat conditions. The SMI did not differ between sexes and habitats for both species.
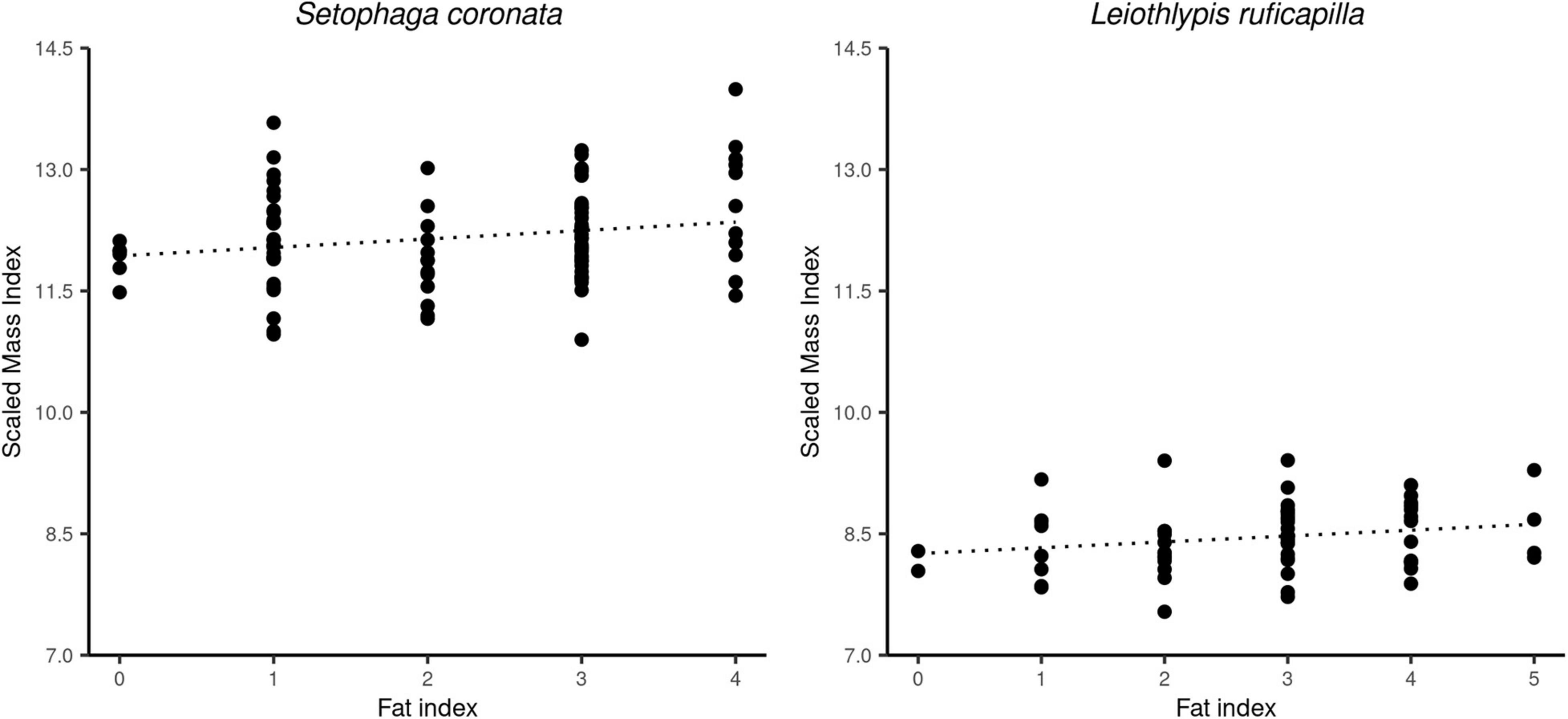
Figure 5. Correlations between the calculated standardized mass index (SMI) and the measured fat index for individuals of S. coronata (left panel) and L. ruficapilla (right panel). S. coronata showed a positive correlation, while L. ruficapilla did not. However, the latter presented a positive trend.
Discussion
The body condition, abundances, the proportion of sexes, and wintering residency of both of our studied species indicate that cities, through their green areas, can maintain viable winter populations of some species of migratory birds. In this section, we first compare the habitat characteristics of both UGAs and non-urban native vegetation areas. Second, we discuss how human management generates specific habitat elements in UGAs that favor migratory birds. Third, we compare the individual body condition of migratory birds in UGAs and non-urban native vegetation areas. Finally, we propose that migratory birds can be considered urban dwellers, and included in urban planning strategies.
Our UGAs and non-urban areas showed fundamental differences in their vegetation structure and the characteristics of their surrounding habitats. Both habitat categories were grouped apart from our urban sites reference (>50% impervious surfaces) due to their differences in habitat structure, principally by a greater urban intensity given by more construction features and high noise levels (Figure 2). The habitat differences between our UGAs and non-urban areas were principally driven by their vegetation complexity. Non-urban areas were characterized by presenting a greater grass and shrub land coverage proportion and higher values of shrub species richness and abundances. Additionally, they presented a lower number of artificial elements. This result is consistent with previous studies that show that UGAs generally exhibit less vegetation complexity in the shrub layer than forest habitats outside cities (Threlfall et al., 2016). The absence of a complex shrub layer in UGAs has been reported to reduce the habitat quality for resident birds (Savard et al., 2000). Interestingly, our results show that this is not the case for our studied migratory bird species.
Surprisingly, our studied UGAs presented a tree layer with greater complexity than the non-urban areas (Figure 2). This was related to the presence of broader and higher trees in UGAs. This structural difference in the tree layer was linked to dominant exotic trees that grow to larger sizes than most native species found outside the city (e.g., Eucalyptus camaldulensis, and Casuarina equisetifolia). Additionally, the management of the UGAs, which includes using large quantities of water for irrigation, and local changes in weather inside the city (e.g., reduced seasonality, urban heat island), allows urban trees to grow larger (Pretzsch et al., 2017). This more complex tree layer found in the UGAs seems to provide suitable habitat quality for insectivore migratory warblers, a group of birds associated to this habitat feature (Amaya-Espinel and Hostetler, 2019).
Previous studies showed that UGAs’ tree species richness did not affect migratory birds (MacGregor-Fors et al., 2010; Carbó-Ramírez and Zuria, 2011). This seems to explain why both the presence and abundance of exotic and native tree species inside the UGAs of Morelia did not affect migratory birds’ abundances and individual quality. Both S. coronata and L. ruficapilla showed similar body conditions in UGAs dominated by exotic trees (Eucalyptus camaldulensis, Casuarina equisetifolia, Spathodea campanulata, Ficus benjamina, and Phoenix canariensis; PZBJ) and in those that mainly presented native tree species (Salix humboldtiana, S. bonplandiana, Fraxinus uhdei, and Taxodium mucronatum; UNLA). However, while migrating through temperate urban areas, these birds have been associated with native trees (Wood and Esaian, 2020). This significant difference in their behavior during the migration and wintering periods indicates that the role of urban trees for migrant birds requires further investigation, considering aspects like tree identity, architecture, and biogeographic origin to understand their use by migratory bird species (Wood and Esaian, 2020).
The similarity of body condition of our study species between our UGAs and non-urban conditions indicates that UGAs can provide a habitat quality comparable to those of non-urban habitats. Our findings are similar to those from temperate cities, showing that migrant birds can replenish their fat reserves using UGAs as stopover sites (Seewagen, 2008; Seewagen and Slayton, 2008; Seewagen et al., 2011). These urban stopover sites allow migrant birds to generate similar amounts of fat than those individuals using areas of native vegetation with low human disturbance located away from cities (Liu and Swanson, 2014). The absence of differences in feather wear and ectoparasite load in both species also indicates that both UGAs and non-urban conditions have similar habitat quality (Delgado-V and French, 2012). Furthermore, the similar recorded densities and capture rates of both species in urban and non-urban sites also support this claim (Bock and Jones, 2004). We found a slightly higher capture rate for S. coronata in UGAs relative to non-urban areas, which may represent an increment in capture rates due to compact bird densities in a limited space. However, it is important to notice that the absence of records or the low densities of both migrant species in our urban matrix sites indicate that they avoid urban habitats with high built cover and low abundance of trees as have been reported before (Zúñiga-Vega et al., 2019).
Overwintering migrant birds populations tend to present segregation by sex and age categories when they occupy areas with heterogeneous habitat quality (Sherry and Holmes, 1996; Albert et al., 2020). Adult males control better quality habitats, and females and juvenile males use lower quality habitats (Sherry and Holmes, 1996). We did not detect any differences by sex within habitat types, which indicates that UGAs offer resources that allow populations un-skewed by sex or with similar differences as in non-urban habitats (Sherry and Holmes, 1996; Liu and Swanson, 2014). Our result for L. ruficapilla suggests this is the case between their male and female population in the region, regardless of habitat type. Our selected SMI model for L. ruficapilla shows relevant statistical differences given by sex, but the effect might be biologically irrelevant [∼0.1% difference in L. ruficapilla mean mass (g); Table 4]. However, higher capture rates and more flight-feather wear in males may indicate accentuated territorial behavior (Murphy et al., 2001). Given that they are insect forage gleaners, more flight-feather wear in L. ruficapilla males can also reflect the use of denser vegetation. Furthermore, their lower ectoparasite load support that they occupy better quality habitats (Delgado-V and French, 2012). These differences by sex can also be attributed to the earlier arrival of males relative to females in overwintering, but this topic requires further research (Francis and Cooke, 1986).
The correlation between the body condition (SMI) and the fat categories in S. coronata, and the positive trend between these variables shown by L. ruficapilla indicate that the SMI reflects differences in the abundance of their energetic reserves (Peig and Green, 2009; Labocha and Hayes, 2012). Most of our captured birds for both focal species presented low-fat values (1–4 on a scale of 8; Kaiser, 1993; Figure 5). While this may be perceived as birds inhabiting low-quality habitats, we have a different interpretation. Our capture period encompassed the moment of the annual cycle of migrant warblers where they just established winter territories and are finishing their recovery from the fall migration (La Sorte et al., 2016, 2017; Albert et al., 2020). As a result, most migrant birds will show low or intermediate fat values because they have not reached the period of winter where they become hyperphagic in preparation for the spring northbound migration (Sherry and Holmes, 1996; Albert et al., 2020).
While our recapture data for both species in UGAs and non-urban areas was low, it was not different from those previously reported in different regions of the Neotropics (Ruiz-Gutiérrez et al., 2012; Monroy-Ojeda et al., 2013; Valdez-Juárez et al., 2018). Low recaptures rates in mist-nets are common for migratory warblers during the winter, even at sites where these birds present strong territoriality and philopatry (Monroy-Ojeda et al., 2013). Our data is very similar to that reported by Monroy-Ojeda et al. (2013) for an UGA in central Mexico. While running a winter banding station in the botanical garden of the city of Oaxaca for 10 years, these authors reported that recaptures from the same season for warblers (repeats that indicate winter residency) average 6.7% of all banded individuals. They also found that recaptures from different years, that indicate winter fidelity for a site, are higher than recaptures from the same season, suggesting that it is hard to recapture birds during the same wintering period. Our recapture data for only one season, along with the information reported by Monroy-Ojeda et al. (2013) and visual recaptures of some of our banded individuals by birdwatchers at our study sites, suggest that both species were maintaining winter residency both in urban green and non-urban areas at our study site. However, this topic requires further study.
To successfully survive their complex annual cycle, migratory birds require good habitat quality throughout all the geographic areas they use while breeding, migrating, and wintering (Schuster et al., 2019; Albert et al., 2020). Their probability of survival in one stage of their annual cycle can be overturned by cumulative carry-over effects from previous stages (Sherry and Holmes, 1996; Albert et al., 2020). Our data indicate that for S. coronata and L. ruficapilla, tropical cities can maintain overwintering bird populations with similar individual quality to those present in natural vegetation habitats located outside cities, acting as a habitat of sufficient quality to allow them to complete their complex annual cycle successfully.
Due to their use of urban areas during winter as non-breeders, these species would be categorized as urban utilizers under Fischer et al. (2015) classification. These authors apply this category to species that “occur in urban environments as non-breeders or as breeders that are present only because of dispersal from adjacent natural areas.” However, since our data suggest that migratory species can overwinter successfully inside urban areas, being independent of non-urban natural vegetation habitats, they should be considered winter urban dwellers (species whose persistence in an urbanized landscape is independent of natural areas). Using this category will acknowledge the importance of urban areas for some species of this important group of birds and help to create urban management strategies that include migrant birds and their conservation.
Data Availability Statement
The raw data supporting the conclusions of this article will be made available by the authors, without undue reservation.
Ethics Statement
Ethical review and approval was not required for the animal study because we complied with all legal requirements to capture birds. We also followed all the codes of conduct of the birds’ capture network to which we belong (BIRDS.MX). Our institution acknowledges our animal management practices during fieldwork. Additionally, we followed “The guidelines to the use of wild birds in research.” Ed. Fair, J. M. Third edition. 2010.
Author Contributions
All authors listed have made a substantial, direct, and intellectual contribution to the work, and approved it for publication.
Funding
This project was supported by the Universidad Nacional Autónoma de México (UNAM) through Programa de Apoyo a Proyectos para Innovar y Mejorar la Educación (PE-214519).
Conflict of Interest
The authors declare that the research was conducted in the absence of any commercial or financial relationships that could be construed as a potential conflict of interest.
Publisher’s Note
All claims expressed in this article are solely those of the authors and do not necessarily represent those of their affiliated organizations, or those of the publisher, the editors and the reviewers. Any product that may be evaluated in this article, or claim that may be made by its manufacturer, is not guaranteed or endorsed by the publisher.
Acknowledgments
We are grateful to Ian MacGregor-Fors and Luis Zambrano for their insightful and enriching criticism and ideas throughout the development of this study. We thank the reviewers for their thoughtful commentaries. We thank all volunteers who participated during fieldwork: Natalia Medrano-Minet, Salvador Pérez-Heredia, Luz Elena López-Ferreyra, Alejandra Sac Bel Monsiváis-Molina, Raiza González-Gómez, Juan Carlos Perez-Magaña, Liliana Trujillo-Pahua, José Luis Ramírez Espinosa, and Jorge Arturo Nuñez-Joaquin. We also want to thank all institutions that allowed us to capture birds in their premises: Universidad Latina de America (UNLA), Organismo Operador de Agua Potable Alcantarillado y Saneamiento de Morelia (Ooapas), Parque Zoológico Benito Juárez, Comisión Nacional del Agua (CONAGUA), and Secretaría de Medio Ambiente, Cambio Climático y Desarrollo (SEMACCDET). RP-M and AC-M thank CONACyT for a doctoral study scholarship. This manuscript constitutes a partial fulfillment of the requirements of the Graduate Program in Biological Sciences of the National Autonomous University of Mexico (PCBIOL-UNAM).
Supplementary Material
The Supplementary Material for this article can be found online at: https://www.frontiersin.org/articles/10.3389/fevo.2021.779507/full#supplementary-material
References
Albert, S., Wolfe, J. D., Kellerman, J., Sherry, T., Stutchbury, B. J. M., Bayly, N. J., et al. (2020). Habitat ecology of Nearctic–Neotropical migratory landbirds on the nonbreeding grounds. Condor 122:duaa055. doi: 10.1093/condor/duaa055
Amaya-Espinel, J. D., and Hostetler, M. E. (2019). The value of small forest fragments and urban tree canopy for Neotropical migrant birds during winter and migration seasons in Latin American countries: a systematic review. Landscape Urban Plann. 190:103592. doi: 10.1016/j.landurbplan.2019.103592
Aronson, M. F. J., La Sorte, F. A., Nilon, C. H., Katti, M., Goddard, M. A., Lepczyk, C. A., et al. (2014). A global analysis of the impacts of urbanization on bird and plant diversity reveals key anthropogenic drivers. Proc. R. Soc. B: Biol. Sci. 281:20133330. doi: 10.1098/rspb.2013.3330
Aronson, M. F. J., Nilon, C. H., Lepczyk, C. A., Parker, T. S., Warren, P. S., Cilliers, S. S., et al. (2016). Hierarchical filters determine community assembly of urban species pools. Ecology 97, 2952–2963. doi: 10.1002/ecy.1535
Barbosa, K. V. C., Rodewald, A. D., Ribeiro, M. C., and Jahn, A. E. (2020). Noise level and water distance drive resident and migratory bird species richness within a Neotropical megacity. Landscape Urban Plann. 197:103769. doi: 10.1016/j.landurbplan.2020.103769
Bayly, N. J., Rosenberg, K. V., Easton, W. E., Gómez, C., Carlisle, J., Ewert, D. N., et al. (2018). Major stopover regions and migratory bottlenecks for Nearctic-Neotropical landbirds within the Neotropics: a review. Bird Conserv. Int. 28, 1–26. doi: 10.1017/S0959270917000296
Bock, C. E., and Jones, Z. F. (2004). Avian habitat evaluation: should counting birds count? Front. Ecol. Environ. 2, 403–410. doi: 10.2307/3868428
Brodeur, J. C., Damonte, M. J., Vera Candioti, J., Poliserpi, M. B., D’Andrea, M. F., and Bahl, M. F. (2020). Frog body condition: basic assumptions, comparison of methods and characterization of natural variability with field data from Leptodactylus latrans. Ecol. Indicators 112:106098.
Carbó-Ramírez, P., and Zuria, I. (2011). The value of small urban greenspaces for birds in a Mexican city. Landscape Urban Plann. 100, 213–222.
Chávez-Zichinelli, C. A., Macgregor-Fors, I., Quesada, J., Talamás Rohana, P., Romano, M. C., Valdéz, R., et al. (2013). How stressed are birds in an urbanizing landscape? Relationships between the physiology of birds and three levels of habitat alteration:>qué tan estresadas están las aves en un paisaje urbanizado? Relaciones entre la fisiología de las aves y tres niveles de alteración de hábitat. Condor 115, 84–92. doi: 10.1525/cond.2013.110201
Davis, A., Taylor, C. E., and Major, R. E. (2011). Do fire and rainfall drive spatial and temporal population shifts in parrots? A case study using urban parrot populations. Landscape Urban Plann. 100, 295–301. doi: 10.1016/j.landurbplan.2010.12.017
Delgado-V, C. A., and French, K. (2012). Parasite–bird interactions in urban areas: current evidence and emerging questions. Landscape Urban Plann. 105, 5–14. doi: 10.1016/j.landurbplan.2011.12.019
Elmqvist, T., Andersson, E., Frantzeskaki, N., McPhearson, T., Olsson, P., Gaffney, O., et al. (2019). Sustainability and resilience for transformation in the urban century. Nat. Sustain 2, 267–273. doi: 10.1038/s41893-019-0250-1
Evans, B. S., Reitsma, R., Hurlbert, A. H., and Marra, P. P. (2018). Environmental filtering of avian communities along a rural-to-urban gradient in Greater Washington, D.C., USA. Ecosphere 9:e02402. doi: 10.1002/ecs2.2402
Fischer, J. D., Schneider, S. C., Ahlers, A. A., and Miller, J. R. (2015). Categorizing wildlife responses to urbanization and conservation implications of terminology: terminology and urban conservation. Conserv. Biol. 29, 1246–1248. doi: 10.1111/cobi.12451
Forman, R. (2014). “Foundations,” in Urban Ecology: Science of Cities, ed. Cambridge University Press (Cambridge: Cambridge University Press), 1–30.
Francis, C. M., and Cooke, F. (1986). Differential timing of spring migration in wood warblers (Parulinae). Auk 103, 548–556. doi: 10.1093/auk/103.3.548
Greenberg, R. (1979). Body size, breeding habitat, and winter exploitation systems in dendroica. Auk 96, 756–766.
Greenberg, R., Bichier, P., and Sterling, J. (1997). Acacia, cattle and migratory birds in southeastern Mexico. Biol. Conserv. 80, 235–247. doi: 10.1016/S0006-3207(96)00137-1
Grimm, N. B., Faeth, S. H., Golubiewski, N. E., Redman, C. L., Wu, J., Bai, X., et al. (2008). Global change and the ecology of cities. Science 319, 756–760. doi: 10.1126/science.1150195
Grimm, N. B., Pickett, S. T. A., Hale, R. L., and Cadenasso, M. L. (2017). Does the ecological concept of disturbance have utility in urban social–ecological–technological systems? Ecosyst. Health Sustainabil. 3:e01255. doi: 10.1002/ehs2.1255
Groffman, P. M., Cadenasso, M. L., Cavender-Bares, J., Childers, D. L., Grimm, N. B., Grove, J. M., et al. (2017). Moving towards a new urban systems science. Ecosystems 20, 38–43. doi: 10.1007/s10021-016-0053-4
Hayes, F. E. (1995). Definitions for migrant birds: what is a neotropical migrant? Auk 112, 521–523. doi: 10.2307/4088747
Holmes, R. T. (2007). Understanding population change in migratory songbirds: long-term and experimental studies of Neotropical migrants in breeding and wintering areas: population change in migratory songbirds. Ibis 149, 2–13. doi: 10.1111/j.1474-919X.2007.00685.x
Howell, S. N. G., and Webb, S. (1995). A Guide to the Birds of México and Northern Central America. Oxford: Oxford Universidad Press.
Instituto Nacional de Estadística, Geografía e Informática [INEGI] (2020). XIII Censo General de Población y Vivienda. México: Instituto Nacional de Estadística, Geografía e Informática.
Kaiser, A. (1993). A new multi-category classification of subcutaneous fat deposits of songbirds. J. Field Ornithol. 64, 246–255.
La Sorte, F. A., Aronson, M. F. J., Lepczyk, C. A., and Horton, K. G. (2020). Area is the primary correlate of annual and seasonal patterns of avian species richness in urban green spaces. Landscape Urban Planning 203:103892. doi: 10.1016/j.landurbplan.2020.103892
La Sorte, F. A., Fink, D., Blancher, P. J., Rodewald, A. D., Ruiz-Gutierrez, V., Rosenberg, K. V., et al. (2017). Global change and the distributional dynamics of migratory bird populations wintering in Central America. Global Change Biol. 23, 5284–5296. doi: 10.1111/gcb.13794
La Sorte, F. A., Fink, D., Hochachka, W. M., and Kelling, S. (2016). Convergence of broad-scale migration strategies in terrestrial birds. Proc. R. Soc. B: Biol. Sci. 283:20152588. doi: 10.1098/rspb.2015.2588
Labocha, M. K., and Hayes, J. P. (2012). Morphometric indices of body condition in birds: a review. J. Ornithol. 153, 1–22. doi: 10.1007/s10336-011-0706-1
Leveau, L. M. (2018). Urbanization, environmental stabilization and temporal persistence of bird species: a view from Latin America. PeerJ 6:e6056. doi: 10.7717/peerj.6056
Liu, M., and Swanson, D. L. (2014). Physiological evidence that anthropogenic woodlots can substitute for native riparian woodlands as stopover habitat for migrant birds. Physiol. Biochem. Zool. 87, 183–195. doi: 10.1086/671746
Liu, M., and Swanson, D. L. (2015). Stopover duration, movement patterns and temporary home ranges of fall migrant yellow-rumped warblers Setophaga coronata in native and anthropogenic woodlands of the Northern Prairie region, USA. J. Avian Biol. 46, 452–461. doi: 10.1111/jav.00672
MacGregor-Fors, I., Escobar-Ibáñez, J. F., Schondube, J. E., Zuria, I., Ortega-Álvarez, R., López, J. R. S., et al. (2020). The urban contrast: a nationwide assessment of avian diversity in Mexican cities. Sci. Total Environ. 753:141915.
MacGregor-Fors, I., Morales-Pérez, L., and Schondube, J. E. (2010). Migrating to the City: responses of neotropical migrant bird communities to urbanization. Condor 112, 711–717. doi: 10.1525/cond.2010.100062
McPhearson, T., Pickett, S. T. A., Grimm, N. B., Niemelä, J., Alberti, M., Elmqvist, T., et al. (2016). Advancing urban ecology toward a science of cities. BioScience 66, 198–212. doi: 10.1093/biosci/biw002
Møller, A. P. (2009). Successful city dwellers: a comparative study of the ecological characteristics of urban birds in the Western Palearctic. Oecologia 159, 849–858.
Monroy-Ojeda, A., Grosselet, M., Ruiz, G., and Valle, E. D. (2013). Winter site fidelity and winter residency of six migratory neotropical species in Mexico. Wilson J. Ornithol. 125, 192–196. doi: 10.1676/12-072.1
Morrone, J. J. (2019). Regionalización biogeográfica y evolución biótica de México: encrucijada de la biodiversidad del Nuevo Mundo. Rev. Mex. Biodiv. 90, 1–68, doi: 10.22201/ib.20078706e.2019.90.2980
Murphy, M. T., Pierce, A., Shoen, J., Murphy, K. L., Campbell, J. A., and Hamilton, D. A. (2001). Population structure and habitat use by overwintering neotropical migrants on a remote oceanic island. Biol. Conserv. 102, 333–345.
Peig, J., and Green, A. J. (2009). New perspectives for estimating body condition from mass/length data: the scaled mass index as an alternative method. Oikos 118, 1883–1891. doi: 10.1111/j.1600-0706.2009.17643.x
Pretzsch, H., Biber, P., Uhl, E., Dahlhausen, J., Schütze, G., Perkins, D., et al. (2017). Climate change accelerates growth of urban trees in metropolises worldwide. Sci. Rep. 7:15403. doi: 10.1038/s41598-017-14831-w
R Core Team. (2021). R: A Language and Environment for Statistical Computing. Vienna: R Foundation for Statistical Computing.
Ralph, C. J., Geupel, G. R., Pyle, P., Martin, T. E., DeSante, D. F., and Milá, B. (1996). Manual de Métodos de Campo Para el Monitoreo de Aves Terrestres. Albany, CA: U.S. Department of Agriculture, Forest Service, Pacific Southwest Research Station.
Rodewald, P. G., and Brittingham, M. C. (2007). Stopover habitat use by spring migrant landbirds: the roles of habitat structure, leaf development, and food availability. Auk 124, 1063–1074. doi: 10.1093/auk/124.3.1063
Ruiz-Gutiérrez, V., Doherty, P. F. Jr., Eduardo Santana, C., Martínez, S. C., Schondube, J., Munguía, H. V., et al. (2012). Survival of resident neotropical birds: considerations for sampling and analysis based on 20 years of bird-banding efforts in Mexico. Auk 129, 500–509. doi: 10.1525/auk.2012.11171
Savard, J.-P. L., Clergeau, P., and Mennechez, G. (2000). Biodiversity concepts and urban ecosystems. Landscape Urban Plann. 48, 131–142. doi: 10.1016/S0169-2046(00)00037-2
Schuster, R., Wilson, S., Rodewald, A. D., Arcese, P., Fink, D., Auer, T., et al. (2019). Optimizing the conservation of migratory species over their full annual cycle. Nat. Commun. 10:1754. doi: 10.1038/s41467-019-09723-8
Seewagen, C. L. (2008). Lipid content of nearctic-neotropical migratory passerines killed during stopovers in a New York city park. Nena 15, 87–96.
Seewagen, C. L., Sheppard, C. D., Slayton, E. J., and Guglielmo, C. G. (2011). Plasma metabolites and mass changes of migratory landbirds indicate adequate stopover refueling in a heavily urbanized landscape. Condor 113, 284–297. doi: 10.1525/cond.2011.100136
Seewagen, C. L., and Slayton, E. J. (2008). Mass changes of migratory landbirds during stopovers in a New York city park. Wilson J. Ornithol. 120, 296–303. doi: 10.1676/07-087.1
Seress, G., Sándor, K., Evans, K. L., and Liker, A. (2020). Food availability limits avian reproduction in the city: an experimental study on great tits Parus major. J. Anim. Ecol. 89, 1570–1580. doi: 10.1111/1365-2656.13211
Sherry, T. W., and Holmes, R. T. (1996). Winter habitat quality, population limitation, and conservation of neotropical-nearctic migrant birds. Ecology 77, 36–48. doi: 10.2307/2265652
Sol, D., Maspons, J., Gonzalez-Voyer, A., Morales-Castilla, I., Garamszegi, L. Z., and Møller, A. P. (2018). Risk-taking behavior, urbanization and the pace of life in birds. Behav. Ecol. Sociobiol. 72:59. doi: 10.1007/s00265-018-2463-0
Spotswood, E. N., Beller, E. E., Grossinger, R., Grenier, J. L., Heller, N. E., and Aronson, M. F. J. (2021). The biological deserts fallacy: cities in their landscapes contribute more than we think to regional biodiversity. BioScience 71, 148–160.
Stearns, F., and Montag, T. (1974). The Urban Ecosystem: A Holistic Approach: The Institute of Ecology Report of the Urban Ecosystems Project. Dowden, Hutchinson & Ross. Available online at: https://books.google.com.mx/books?id=DItizQEACAAJ (accessed September 16, 2021).
Stewart, A. B., and Waitayachart, P. (2020). Year-round temporal stability of a tropical, urban plant-pollinator network. PLoS One 15:e0230490. doi: 10.1371/journal.pone.0230490
Threlfall, C. G., Ossola, A., Hahs, A. K., Williams, N. S. G., Wilson, L., and Livesley, S. J. (2016). Variation in vegetation structure and composition across urban green space types. Front. Ecol. Evol. 4:66. doi: 10.3389/fevo.2016.00066
Tryjanowski, P., Skórka, P., Sparks, T. H., Biaduñ, W., Brauze, T., Hetmañski, T., et al. (2015). Urban and rural habitats differ in number and type of bird feeders and in bird species consuming supplementary food. Environ. Sci. Pollut. Res. 22, 15097–15103. doi: 10.1007/s11356-015-4723-0
Valdez-Juárez, S. O., Drake, A., Kardynal, K. J., Hobson, K. A., Krebs, E. A., and Green, D. J. (2018). Use of natural and anthropogenic land cover by wintering Yellow Warblers: the influence of sex and breeding origin. Condor 120, 427–438. doi: 10.1650/CONDOR-17-180.1
Wood, E. M., and Esaian, S. (2020). The importance of street trees to urban avifauna. Ecol. Appl. 30:ea2149. doi: 10.1002/eap.2149
Zuckerberg, B., Fink, D., La Sorte, F. A., Hochachka, W. M., and Kelling, S. (2016). Novel seasonal land cover associations for eastern North American forest birds identified through dynamic species distribution modelling. Diversity Distribut. 22, 717–730. doi: 10.1111/ddi.12428
Zuñiga-Palacios, J., Zuria, I., Castellanos, I., Lara, C., and Sánchez-Rojas, G. (2021). What do we know (and need to know) about the role of urban habitats as ecological traps? Systematic review and meta-analysis. Sci. Total Environ. 780:146559. doi: 10.1016/j.scitotenv.2021.146559
Keywords: Nearctic-Neotropical migrant, mist netting, body condition, vertebrates, Mexico, avian, point-count, non-urban
Citation: Pacheco-Muñoz R, Ceja-Madrigal A, Pérez-Negrón E, Ortiz-Asbun AK and Schondube JE (2022) Wintering Town: Individual Quality of Migratory Warblers Using Green Urban Areas in a Neotropical City. Front. Ecol. Evol. 9:779507. doi: 10.3389/fevo.2021.779507
Received: 18 September 2021; Accepted: 20 December 2021;
Published: 17 January 2022.
Edited by:
Álvaro Garitano-Zavala, Universidad Mayor de San Andrés, BoliviaReviewed by:
Yolanda E. Morbey, Western University, CanadaPiotr Tryjanowski, Poznań University of Life Sciences, Poland
Copyright © 2022 Pacheco-Muñoz, Ceja-Madrigal, Pérez-Negrón, Ortiz-Asbun and Schondube. This is an open-access article distributed under the terms of the Creative Commons Attribution License (CC BY). The use, distribution or reproduction in other forums is permitted, provided the original author(s) and the copyright owner(s) are credited and that the original publication in this journal is cited, in accordance with accepted academic practice. No use, distribution or reproduction is permitted which does not comply with these terms.
*Correspondence: Jorge E. Schondube, Y2hvbkBjaWVjby51bmFtLm14