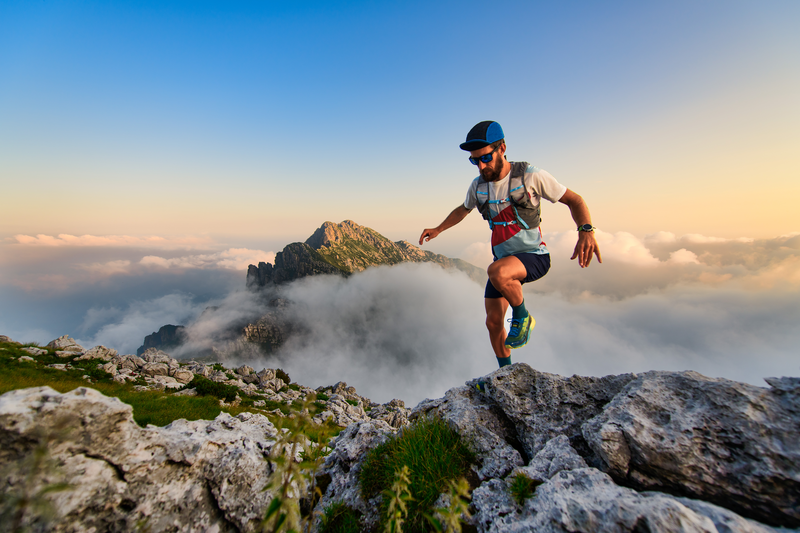
95% of researchers rate our articles as excellent or good
Learn more about the work of our research integrity team to safeguard the quality of each article we publish.
Find out more
ORIGINAL RESEARCH article
Front. Ecol. Evol. , 16 December 2021
Sec. Population, Community, and Ecosystem Dynamics
Volume 9 - 2021 | https://doi.org/10.3389/fevo.2021.774744
This article is part of the Research Topic Drivers of Small-Mammal Community Structure in Tropical Savannas View all 14 articles
Years of fire suppression, decreases in herbivores, and global climate change have led to shifts in savannas worldwide. Natural open vegetation such as grasslands and shrublands is increasing in wood density, but the effects for small mammals are not well understood. While most of the mammal studies from the Brazilian Cerrado are concentrated in the core area of this large Neotropical savanna, its southern portions are suffering from biome shifting through woody encroachment. Herein, we surveyed a small mammal community from the southeastern boundary of Cerrado (Santa Bárbara Ecological Station) and evaluated the micro and macro environmental variables shaping community structure in order to investigate how the woody encroachment in the last 15 years may have influenced this assemblage. We recorded 17 species of marsupials and rodents along five distinct habitats in a gradient from grasslands to woodlands. Although richness was not affected by microhabitat variables, total and relative abundance varied according to habitat type and in relation to herbaceous, shrub, and tree density. Rodents such as Calomys tener and Clyomys laticeps were positively affected by increasing herb cover, Cerradomys scotti and Oligoryzomys nigripes by shrub cover, while the marsupial Didelphis albiventris had higher association with increasing tree cover. We detected an increase of 27.4% in vegetation density (EVI) between 2003 and 2018 in our study site, and this woody encroachment negatively affected the abundance of some small mammals. The open-area specialists Cryptonanus chacoensis and C. scotti had a decrease in abundance, while D. albiventris and O. nigripes were favored by woody encroachment. Our data suggest that woody encroachment is shifting community composition: small mammals often associated with grasslands and open savannas are likely to be negatively affected by woody encroachment; while species that rely on tree-covered habitats are likely to benefit from an increasing woody landscape. Therefore, forest-dwellers are gradually replacing open-vegetation inhabitants. Active management of open formations (e.g., with prescribed burning) may be needed to maintain Cerrado biodiversity, especially considering the open-area endemics.
Savannas worldwide have faced an increase in woody plant cover in the last century (Stevens et al., 2016a; Archer et al., 2017; García Criado et al., 2020). Changes in savannas natural landscapes due to tree encroachment have been described in Africa (e.g., Mitchard and Flintrop, 2013; Blaser et al., 2014; Stevens et al., 2016b), Australia (e.g., Fensham et al., 2005; Price and Morgan, 2008), North America (e.g., Archer, 1994; Van Auken, 2009; Ratajczak et al., 2012), and South America (e.g., Honda and Durigan, 2016; Passos et al., 2018; Rosan et al., 2019). The fast increase in woody biomass, stem density, woody cover, and/or woody density in an ecosystem defines woody encroachment, which leads to the conversion of natural open habitats into woodlands (Stevens et al., 2016a; Rosan et al., 2019; Eldridge and Ding, 2021). This increase in forest formations across savanna landscapes often leads to a decrease in herbaceous cover and changes in associated biodiversity, primarily at the expense of savanna specialists (Van Auken, 2009; Abreu et al., 2017; Archer et al., 2017). The main causes of woody encroachment vary regionally as different and complex mechanisms act across savanna ecoregions, differing in ecological, climatic, evolutionary, and social aspects (Archer, 1994; Archer et al., 2017). Changes in natural fire regime, herbivory, climate (temperature and precipitation), land use, and higher atmospheric CO2 are suggested as the main causes for this phenomenon and are often associated with human disturbance in these ecosystems (Bond and Midgley, 2000; Stevens et al., 2016a; García Criado et al., 2020).
South American savannas showed the highest mean of woody cover increase (7.4% per decade) across tropical savannas (Stevens et al., 2016a). Moreover, 19% of the remaining Brazilian Cerrado areas are under woody encroachment, probably induced by fire suppression and agricultural land abandonment, intensified by the increase in atmospheric CO2 (Moreira, 2000; Roitman et al., 2008; Passos et al., 2018; Rosan et al., 2019). Fire is a determinant driver for savanna dynamics, which has a historical evolution modeled by fire and herbivory processes, along with other fire-prone ecosystems (Bond and Keeley, 2005; Parr et al., 2014; Fidelis, 2020). The Cerrado high biodiversity is a result of these processes, with a rich mosaic of habitats (from open grasslands to woodland savannas) and endemic species adapted and often dependent on frequent fire events to maintain their populations (Simon et al., 2009; Fidelis and Blanco, 2014; Pilon et al., 2018, 2020). Brazilian Cerrado already lost about half of its natural vegetation due to extensive agriculture, especially soybean monoculture, and human occupation, leading to a high level of fragmentation (Motta et al., 2002; Sano et al., 2010; Alencar et al., 2020). Changes in the natural balance of the local fire regime (such as frequency and intensity) can lead to declines in local populations or even local extinction, particularly for savanna specialists (Bowman et al., 2020). For instance, the Santa Bárbara Ecological Station (SBES), located in southeastern Brazil, has been under almost complete fire suppression for about 30 years, and recently (since 2015) fire management was introduced (Durigan et al., 2020). Over three decades (1985–2015), this remnant of Cerrado experienced woody encroachment and decreases in plant (especially herbs and shrubs) and ant species typical of open habitats (Abreu et al., 2017, 2021).
Most studies on the consequences of woody encroachment are focused on plants and biogeochemical cycles (e.g., Moreira, 2000; Huxman et al., 2005; Price and Morgan, 2008; Van Auken, 2009; Ratajczak et al., 2012; Blaser et al., 2014; Honda and Durigan, 2016; García Criado et al., 2020) with few dedicated to the responses of animal communities (e.g., Eldridge et al., 2011; Abreu et al., 2017; Stanton et al., 2017; Andersen and Steidl, 2019). Stanton et al. (2017) highlight a minor research effort about shrub encroachment impacts on vertebrates in all continents, with a higher number of studies on bird communities (almost twice those on mammals and herpetofauna, individually). For mammals, studies show a decrease in diversity and abundance with shrub encroachment in Africa (Stanton et al., 2017). Thus, it is urgent to study the possible impacts of woody encroachment on mammals from Cerrado, the richest savanna for mammal species, most of which composed of small mammals (Paglia et al., 2012; Mendonça et al., 2018). About 20% of the Cerrado non-flying small mammals (rodents and marsupials) are endemic and present high habitat selectivity and low dispersal capacity, which makes them highly vulnerable to changes in their habitat remnants (Pardini et al., 2010; Carmignotto et al., 2012; Gutiérrez and Marinho-Filho, 2017; Carmignotto, 2019).
Habitat structure modifications unleashed by the increase in tree density include a decrease in the area covered by the herbaceous layer due to competition for resources such as soil moisture, nutrients, and light, and intolerance to high canopy shading (Archer, 1994; Van Auken, 2009; Parr et al., 2014). This modified ecosystem will probably impact animal communities of savanna specialists, for instance, through changes in macro and microhabitat variables, such as the amount of sunlight that reaches the soil, microclimate, water, food, and refuge availability, and vegetation structure (Abreu et al., 2017; Loggins et al., 2019). As small mammals exhibit high habitat selectivity and have a heterogeneous distribution associated with the mosaic of habitats in the Cerrado, these changes in habitat structure can lead to a shift in the composition of local small mammal communities (Carmignotto et al., 2012, 2014). Although the microhabitat perspective has been explored by small mammal ecologists since the 60s (e.g., Morris, 1987; Stancampiano and Schnell, 2004; Melo et al., 2013; Corrêa et al., 2017), the definition and clarity of which variables and scale define it vary among studies [see review by Jorgensen (2004)]. There are few studies evaluating microhabitat effects on the density of Cerrado small mammals (e.g., Vieira, 2003; Rocha et al., 2011), a topic that is broadly explored for the neighbor Atlantic Forest (e.g., Püttker et al., 2008; Melo et al., 2013). In this study, we evaluated habitat selectivity from both the macro and microhabitat perspectives, aiming to contribute to the understanding of which factors, at different scales, shape a small mammal community at the southeastern Cerrado boundary. We also aimed to evaluate how the woody encroachment in the last 15 years may have influenced community structure. We expect a notable change in the small mammal community of Cerrado sites under woody encroachment, with forest-dwellers gradually replacing open-vegetation inhabitants, partially due to differential habitat and microhabitat preferences.
Our study was conducted in the Santa Bárbara Ecological Station (hereafter SBES; 22°46′–22°51′S/49°10′–49°16′ W, 600–680 m above sea level, Águas de Santa Bárbara municipality, São Paulo, Brazil), one of the few protected areas that preserves open savannas in the southern Brazilian Cerrado (Durigan et al., 2020). SBES is characterized by a mosaic of savannas and Atlantic Forest patches (Melo and Durigan, 2011), comprising 2,715 ha. Currently, SBES vegetation is mostly represented by native Cerrado formations, from grasslands (“campo sujo” and “campo cerrado”) and savannas (“cerrado sensu stricto”) to woodlands (“cerradão”) (Oliveira-Filho and Ratter, 2002; Araujo et al., 2010; Melo and Durigan, 2011). The SBES was under a fire suppression policy for 30 years (1985–2015; Abreu et al., 2017; Durigan et al., 2020). The grasslands that we studied here are among the few ones that had some accidental fire events more recently. The 2003 “campo limpo” (“campo sujo” in the 2017–2018 survey) patch had at least four fire events before 2008, when the last one was registered. One “campo cerrado” patch had only one fire event recorded in the last 30 years, in 2001, and the other patch in 2011, and both have been under fire suppression since then. The other plots that were sampled, burned for the last time before 1985 (Melo and Durigan, 2011; Conciani et al., 2021). Although these sparse fire events contributed to the grasslands maintenance in the area, SBES have been suffering under woody encroachment across this period (Melo and Durigan, 2011; Abreu et al., 2017, 2021). The study site also holds stands of exotic timber plantations, including Eucalyptus sp. and Pinus sp. Dry/cold (April to September) and wet/warm (October to March) seasons are strongly marked. The climate is classified as Köppen Cwa-type, with annual rainfall between 1010 and 2051 mm and an average of 1454 mm (Alvares et al., 2013; CIIAGRO, 2016). The mean temperature of the coldest months is 17°C and for the hottest months, 24°C, with a maximum of 35.2°C and a minimum of 3.4°C. These data correspond to the period from 1995–2014 and come from the weather station in the municipality of Manduri, São Paulo, Brazil, 20 km from our study area (CIIAGRO, 2016). SBES soils are characterized as deep oxisols with low nutrient and high sand content, high saturation of aluminum, and low capacity of holding water (Melo and Durigan, 2011).
To characterize the habitat and microhabitat preferences in order to investigate the role of woody encroachment in the small mammal community (rodents and marsupials), we used data from two temporally spaced surveys: (1) the 2003 survey carried out during January and February 2003, in four Cerrado habitats of SBES including open grasslands (“campo limpo” and “campo sujo”), savanna (“cerrado sensu stricto”), and woodland (“cerradão”), comprising a 10-day field-trip and a capture effort of 1,365 live trap nights and 1,680 pitfall trap nights [more details about the sampling design of the 2003 survey can be found in Carmignotto (2005)]; and (2) the 2017–2018 survey, from August 2017 to July 2018, with 12 monthly 10-day field trips, also in four Cerrado habitats, from grassland to woodland (“campo sujo,” “campo cerrado,” “cerrado sensu stricto,” and “cerradão”), comprising 2,880 live trap nights and 2,880 pitfall trap nights in each habitat surveyed (three sampling points by habitat), totaling 11,520 live trap nights and 11,520 pitfall trap nights across the 12 sampling points. Each sampling point had eight live traps: four Sherman traps (25 cm × 8 cm × 9 cm, Sherman™) and four-wire mesh traps (two of 30 cm × 16 cm × 18 cm and two of 32 cm × 20 cm × 20 cm, Metal Miranda), alternated and 15 m apart. Traps were distributed in two arrays (A and B, each with two Shermans and two-wire mesh traps) 60 m apart. At the beginning of each field trip, the live traps were set on the ground and baited with a mix of peanut butter, cornmeal, and canned sardines. This bait was fixed on pieces of sweet potatoes in the wire mesh traps. Each sampling point was also composed of two lines of pitfall traps, 60 m apart, each with four 100-L buckets, 10 m apart, connected by a 60–70 cm high and ∼10 cm buried plastic drift fence. The pitfall trap arrays were 60 m apart from the live trap lines, and the buckets were opened and closed at the beginning and ending of each field trip.
The individuals captured were identified at species level (Voss and Jansa, 2009, and Fegies et al., 2021, for marsupials; and Patton et al., 2015, for rodents), weighted, and sexed. Other information about age (juvenile, subadult, and adult), behavior, and reproduction was taken when possible. We collected tissue samples from the ear of all individuals for taxonomic identification through molecular analysis. This small cut also served as recapture recognition for individuals weighing less than 30 g (although not allowing recognition at the individual level). We used numbered ear tags (ZT 900 by Zootech) for individual identification of animals weighing over 30 g. Some individuals were collected for morphological taxonomic identity and subjected to taxidermy or fixed with 10% formaldehyde solution and preserved in 70% alcohol (SISBIO 50658-3 collection permit). These vouchers will be deposited in the mammal collection of the Museu de Zoologia da Universidade de São Paulo (MZUSP), São Paulo, Brazil. All procedures of capture and collection were made following the ASM guidelines for the use of wild mammals in research (Sikes et al., 2016) and were approved by the Animal Ethical Committee (#CEUA-IB-USP 241/2016).
In order to assess the habitat use by the SBES small mammals, we used the number of individuals captured in the 2017–2018 survey along the four habitat types sampled. These habitats were chosen in the field and represent a gradient of natural increasing woody cover, typical of Cerrado mosaics. The “campo sujo” and “campo cerrado” are both open formations with a predominance of herbaceous layer, but “campo sujo” is a grassland characterized by scattered shrubs and small trees, and “campo cerrado” by sparse trees and higher shrub cover, but still with a large proportion of herbs; “cerrado sensu stricto” is also known as “typical cerrado,” and is a savanna formation dominated by shrubs and trees up to 3–8 m tall often covering 30% of the crown canopy, but still with a high herb cover; and “cerradão” is a forest formation, a woodland savanna with often 50–90% of canopy coverage composed of higher trees 8–12 m tall (see Oliveira-Filho and Ratter, 2002). The field classification was later confirmed by the enhanced vegetation index (EVI) obtained at each habitat surveyed.
To evaluate the potential role of microhabitat features in structuring small mammal communities, we measured seven variables at each live trap station during the 2017–2018 survey: (1) percentage of canopy cover; (2) number of trees with diameter at breast height (DBH) > 10 cm; (3) percentage of herbaceous soil cover; (4) percentage of soil covered by the invasive grass, Brachiaria sp.; (5) number of stems of shrubs that branch up to 1 m in height; and understory density, through (6) the number of touches up to 0.5 m height, and (7) between 0.5 and 1 m in height on a stick held vertically in different directions (S, N, L, and O). Variables 1–3 were visually estimated within a radius of 5 m from each live trap, and variables 4–7 were measured within a radius of 2 m from the live traps. These variables were selected based on their use in previous microhabitat small mammal studies (e. g., Freitas et al., 2002; Vieira et al., 2005; Rocha et al., 2011), and due to their close relationship with vegetation cover (herb, shrub, and trees) and canopy openness, differentiating well the distinct Cerrado habitats analyzed. We did not measure the microhabitat variables for pitfall stations since the area near each trap was cleared for the installation of pitfall traps. Therefore, the capture data used for microhabitat analysis were restricted to live trap stations. To increase independence of records, we did not consider recaptures of the same individual in the same live trap and month. Recaptures in different field trips and live trap lines were kept, as they could indicate the suitability of microhabitats for that species.
We used the enhanced vegetation index (EVI) to quantify the vegetation change over the 15 years between both surveys (2003 and 2017--2018) across the sampled habitats. We calculated the mean EVI values for each sampling point based on surface reflectance images from Landsat 7 for 2003, and from Landsat 8 for 2018, obtained from the United States Geological Survey satellite products (USGS1). Furthermore, we used the available images from the survey periods, or as near as possible to the date, that presented conditions with minimum cloud cover. The satellite image manipulation and EVI values calculation were performed in the software QGis version 3.4.14 (QGIS, 2021). Abreu et al. (2017) showed that the EVI index is highly correlated with tree basal area, and Chaves et al. (2013) also argue that the EVI index is more sensitive to canopy changes, mainly in places with a higher concentration of biomass when compared with the NDVI index. Thereby, we consider the EVI index a suitable proxy to quantify woody encroachment in our study site.
We used different datasets for each analysis performed here, according to its scale and goals. The datasets are derived from the total data we collected in both surveys (2003 and 2017–2018) and are described in Table 1 and at each analysis item below.
Statistical analyzes were performed in R (version 4.1.0; R Core Team, 2021). The model selection for all linear models described below was made through hypothesis testing approach (ɑ = 0.05), comparing nested models through ANOVA function using the variance partition from the F-statistic for linear models and the residual deviance and chi-square tests for generalized linear models (Zuur et al., 2009). The diagnoses of the fitted models were made with the “DHARMa” package, we tested the model fitness for over and underdispersion, uniformity, outliers (along with Cook’s distance plot), and zero-inflation (Harting, 2021, Supplementary Table 1). The prediction graphics of the models were made with the package “ggiraphExtra,” function ggPredict (Lüdecke, 2018).
To characterize the community structure and compare patterns of small mammals between the two distinct surveys, we considered all data (Dataset 1, Table 1) from pitfalls and live traps (except for recaptures, which were removed) from both the 2003 and 2017–2018 surveys. Community structure was characterized by its alpha taxonomic diversity with the CHAO1 estimator, which allows estimating the absolute number of species in a community based on the number of rare species in the sample (Whittaker, 1972; Dias, 2004). We also estimated species richness through rarefaction/extrapolation species curves (Colwell et al., 2012; Chao et al., 2014). To evaluate the adequacy of sampling effort, the abundance data were used to calculate the coverage estimator which represents the proportion of the total number of individuals in an assemblage belonging to a species represented in the sample, estimated in percentage, as a measure of completeness. These analyses were made using the “iNEXT” R package (Hsieh et al., 2016). Confidence intervals (95%) were used as thresholds in comparisons (Chao and Chiu, 2016). Abundance was defined as the total number of individuals captured per species, and to determine the dominance and identify rare species in the communities, we used the Abundance-based Coverage Estimator (ACE) with the ChaoSpecies function from the “SpadeR” package (Moreno, 2001; Chao and Chiu, 2016).
In order to investigate the habitat use by the SBES small mammals, we performed a Non-Metric Multidimensional Scaling (NMDS) ordination with the “vegan” R package (Oksanen et al., 2020), function metaMDS, based on the number of individuals captured in the four habitats surveyed (“campo sujo,” “campo cerrado,” “cerrado sensu stricto,” and “cerradão”). For the NMDS, we used Dataset 2 (Table 1), which consisted of the total number of individuals captured in pitfalls and live traps (except for recaptures, which were removed) in the 2017–2018 survey. In order not to obscure the patterns found, we considered only species with n > 5 individuals. We also performed the Shepard plot (or stress plot), in order to observe the goodness of fit of the data into the NMDS analysis, using the stressplot R function.
The microhabitat analyzes were performed only for the 2017–2018 survey, considering only the live trap data (Dataset 3, Table 1), as explained above. First, we reduced the dimensionality of the seven microhabitat variables through a principal components analysis (PCA) with a correlation matrix using the “stats” package and retained the principal components with eigenvalues > 1. Then we fitted generalized linear mixed models (GLMM) with the package “lme4” (glmer function, family = poisson, link = log; Bates et al., 2021) for the abundance (number of captures per species) and richness of the small mammals as a function of the two principal components extracted from the PCA. The random effect considered for these GLMM was the 12 sampling points (three for each habitat), since for the microhabitat analyzes we treated each trap station as our sampling unit and considered it as non-independent within the 12 sampling points analyzed. For species with n > 10 individuals, we also built GLMM for the number of captures of each species in relation to the principal components selected.
In order to investigate the effect of woody encroachment across time on small mammals, we used the Dataset 4 (Table 1), which included the total number of individuals captured in both pitfall and live traps (except the recaptures), of the 2003 survey (3,045 trap-nights). For the 2017–2018 survey, we restricted our data to those obtained in January-February (3,840 trap-nights), in order to standardize the capture effort between the two surveys analyzed. The same approach was employed for the analysis of satellite images, as explained in the data sampling item above.
To quantify the increase in tree cover between the two surveys, we fitted linear models (“stats” R package, lm function) using the mean EVI values for each sampling point as a function of the sampling period (2003 × 2018) and habitat (“campo limpo,” “campo sujo,” “campo cerrado,” “cerrado sensu stricto,” and “cerradão”). To test the woody encroachment impact on the small mammal community, we fitted generalized linear models (GLM) for total richness and abundance of small mammals, and per order (rodents and marsupials), as a function of the EVI mean values for each sampling unit for the 2003 and 2018 surveys. A dissimilarity analysis based on the number of individuals captured between the two surveys was made by estimating beta diversity using the function beta from the “BAT” package (Carvalho et al., 2012; Cardoso et al., 2015) in order to detect changes in species composition across time between the two assemblages analyzed.
We captured 1,112 individuals of 17 native species of marsupials and rodents, in the gradient from grasslands to woodland savanna, considering both surveys (2003 and 2017–2018). Rodents and marsupials represented 67 and 33% of the total richness, respectively, (Table 2).
Table 2. Relative abundance (%) of small mammals in the Santa Bárbara Ecological Station, São Paulo, Brazil, in the 2003 and 2017–2018 surveys.
The 2003 survey recorded 10 species of small mammals (60% of total SBES richness), of which 70% were rodents and 30% marsupials, with a total of 124 individuals captured. In the 2017–2018 survey, 988 individuals were captured belonging to 17 species (100% of SBES total richness), of which 65% were rodents and 35% marsupials. Comparing both surveys, for 2003 we did not record the rodents Hylaeamys megacephalus, Nectomys cf. squamipes, Oxymycterus delator, and Rhipidomys cf. macrurus, and the marsupials Didelphis albiventris, Gracilinanus agilis, and Gracilinanus microtarsus in the habitats analyzed.
Figure 1. Rarefaction/Extrapolation curves for (A) the 2003 survey generated with 1,000 bootstraps and 100 individuals as endpoint and for (B) the 2017–2018 survey generated with 1,000 bootstraps and 420 individuals as endpoint. The shadow surface represents the 95% confidence interval for each curve. The habitats are: CL: “campo limpo”; CS: “campo sujo”; CC: “campo cerrado”; CE: “cerrado sensu stricto”; and CD: “cerradão.”
Figure 2. Relative abundances and number of individuals (in parentheses) of small mammals from SBES captured in (A) 2003 survey (3,045 trap-nights) and (B) 2017–2018 survey (23,040 trap-nights).
Figure 3. Non-metric multidimensional scaling (NMDS) for small mammal abundances during the 2017–2018 survey. Small mammal species are: Ak_mo: Akodon cf. montensis; Ca_te: Calomys tener; Ce_sc: Cerradomys scotti; Cl_la: Clyomys laticeps, Cr_ch: Cryptonanus chacoensis; Cr_af: Cryptonanus aff. chacoensis; Di_al: Didelphis albiventris; Gr_ag: Gracilinanus agilis; Gr_mi: Gracilinanus microtarsus; Hy_me: Hylaeamys megacephalus; Mo_ku: Monodelphis kunsi; Ne_la: Necromys lasiurus; Ol_ma: Oligoryzomys mattogrossae; Ol_ni: Oligoryzomys nigripes; Rh_ma: Rhipidomys cf. macrurus. The habitats are: CS: “campo sujo”; CC: “campo cerrado”; CE: “cerrado sensu stricto”; and CD: “cerradão.”
The species richness observed for the complete 2003 survey and for “campo sujo” and “cerradão” habitats were relatively close to the richness estimated by the Chao1 estimator (Table 3), indicating that the sampling effort was appropriate to survey this community, as was shown also by the coverage estimate (98, 97, and 83%, respectively). On the other hand, for the “campo limpo” and “cerrado sensu stricto,” the observed richness was only half of the estimated richness, which is clear through the low coverage value for “cerrado sensu stricto” (55%), but not for “campo limpo” (92%), indicating that a more extensive sampling in the “cerrado sensu stricto” would be necessary (Table 3). Despite the extremely low species richness value obtained for “cerradão,” the rarefaction-extrapolation curves for the 2003 assemblage indicate no differences in the species richness among the habitats sampled, with values estimated for extrapolated data, corroborating the results of the Chao1 richness estimator (Figure 1A).
Table 3. Observed and estimated species richness, with standard errors and sample coverage, of small mammals for the 2003 and 2017–2018 surveys in distinct habitats surveyed at SBES.
For the 2017–2018 survey, all the observed and estimated species richness were also similar, with high coverage values (99% for total data and habitats), evidencing that the sampling effort was sufficient to estimate the community richness (Table 3). This result corroborates those obtained by the rarefaction/extrapolation curves, which also indicates that only the “campo cerrado” differed in relation to the other habitats surveyed, showing comparatively lower richness (Figure 1B).
The species with the highest abundance for the 2003 survey was Necromys lasiurus, representing 71% of the assemblage (Figure 2A), followed by other rodents such as Cerradomys scotti (6.5%) and Oligoryzomys mattogrossae (4.8%). Furthermore, the less abundant species were the rodents Akodon cf. montensis and Calomys tener, with only one individual captured each, representing, together, 1.6% of total abundance (Figure 2A). This pattern became clear in the Abundance-based Coverage Estimator (ACE) analysis, which considered only N. lasiurus as an abundant species, and the nine remaining species as rare (k < 10). For the 2017–2018 survey, the species of Oligoryzomys were the most abundant, with O. nigripes comprising 25.4% of the assemblage and O. mattogrossae 18.6%. Other abundant species in this assemblage were the rodents Calomys tener (14.8%) and Cerradomys scotti (11.2%). The rarest species were the rodents Nectomys cf. squamipes (one individual) and Oxymycterus delator (two individuals), representing, together, less than 0.4% of total abundance (Figure 2B). For 2017–2018, the ACE analysis indicates 12 abundant species (k > 10), and five rare species.
For patterns of habitat use, the non-metric multidimensional scaling for the 2017–2018 survey (Figure 3; stress = 0.09, Supplementary Figure 1) showed distinct species groups, one composed by Calomys tener, Cerradomys scotti, Oligoryzomys mattogrossae, and Cryptonanus chacoensis that seemed more related to “campo cerrado,” a grassland formation; and another formed by D. albiventris, Gracilinanus agilis, and Hylaeamys megacephalus that were located closer to “cerradão,” a forested habitat. Also related with “cerradão” are Gracilinanus microtarsus and Rhipidomys cf. macrurus, but more dispersed than the previous species. Akodon cf. montensis and Oligoryzomys nigripes were located in between “cerrado sensu stricto” (savanna) and “cerradão” (forest). The species Cryptonanus aff. chacoensis and Clyomys laticeps were a bit more dispersed, but relatively close to the “campo sujo,” the most open habitat at SBES. Monodelphis kunsi was located in a transition between “cerradão,” “cerrado sensu stricto,” and “campo sujo,” and Necromys lasiurus between “cerrado sensu stricto,” “campo cerrado,” and “campo sujo.”
The seven microhabitat variables were reduced to two principal components with eigenvalues > 1 (3.12 and 1.58, respectively, Supplementary Table 2) that represented about two-thirds of the total variance in our dataset (67.2%, Supplementary Table 2 and Supplementary Figure 2). The first principal component (hereafter PC1 variable) represented 44.6% of the total variance and is an indicator of high herb cover and low tree cover. The second principal component retained (hereafter PC2 variable) is an indicator of high shrub cover and low herb cover and represented 22.6% of the total variance (Supplementary Table 3). The gradient in relation to vegetation cover and canopy openness is visible in the PCA graph and is evidently related to the PC1 and PC2 variables (Supplementary Figure 2). There was no difference in richness considering the PC1 and PC2 variables (null model: p = 0.50), i.e., richness did not differ across the habitat gradient. Despite that, total abundance was positively affected by shrub cover and negatively by herb cover (PC2), with an average increase of 18% in abundance per unit of PC2 (p < 0.001).
For the relative abundance (for species with n > 10 captures), the rodents Calomys tener (p < 0.001) and Clyomys laticeps (p = 0.01) were positively associated with herb cover. The estimated average increase in the relative abundance of Calomys tener in areas with high herb cover was 80.7% per PC1 unit (p < 0.001). On the other hand, Clyomys laticeps showed an average decrease of 74% per unit of PC2 (p = 0.004), which indicates a high positive relation with herb cover and a negative relation with shrub cover. Cerradomys scotti (p = 0.015) and Oligoryzomys nigripes (p = 0.02) were the species positively associated with shrub cover, with an average increase of 45 and 51.8% (p = 0.02) in their relative abundance per unit of PC2, respectively. Oligoryzomys mattogrossae was associated with both herb and shrub habitats (p < 0.001), with an estimated average increase of 53% (p = 0.04) in herbaceous areas and 58% in shrub units (p < 0.001). The marsupial D. albiventris was positively related to tree and shrub cover (p < 0.001), with an average increase of 67.3% in its abundance in forest areas and 18% for shrublands. The marsupial Gracilinanus agilis and the rodent Hylaeamys megacephalus were not influenced by PC1 and PC2 in their relative abundances (p = 0.14 and 0.58, respectively).
Our linear model showed an increase of 0.1 on the EVI mean between the 2003 and 2018 survey (R2 adj. = 0.26, F1,14 = 6.38, p = 0.02). This result indicates an increase in vegetation density of 27.4% in 15 years, affecting primarily the open areas (Figures 4, 5A). The linear model for EVI regarding the habitat types demonstrated a clear difference in its EVI mean values (R2 adj. = 0.62, F4,11 = 7.1, p = 0.004, Figure 5B), with the open habitats (“campo limpo,” “campo sujo,” and “campo cerrado”) not different considering their EVI mean values, despite following a gradual increase on averages (0.30, 0.36, and 0.41, respectively). However, for the intermediate habitat, “cerrado sensu stricto,” we estimate an increase of 46.6% on the EVI mean value compared to the more open habitat (“campo limpo”), with an EVI mean value of 0.44. For the forest formation, “cerradão,” the model estimated a difference in the EVI mean value of 70% higher in relation to the “campo limpo” area, reaching the highest EVI mean value, 0.51 (Figure 5B).
Figure 4. Variation in the enhanced vegetation index (EVI) between the sampling periods of (A) 2003 and (B) 2018 for the study area, Santa Barbara Ecological Station (SBES), São Paulo, Brazil. Dots indicate the location of sampling units.
Figure 5. Means of enhanced vegetation index (EVI) and standard deviation estimated by linear models for (A) sampling periods and (B) Cerrado habitats. The habitats are: CL: “campo limpo”; CS: “campo sujo”; CC: “campo cerrado”; CE: “cerrado sensu stricto”; and CD: “cerradão.”
The richness of small mammals was not affected by the increase in wood density (p = 0.39). We also tested the richness per mammal order, and obtained the same results, with no difference in richness as a function of EVI mean (Rodents: p = 0.27; Marsupials: p = 0.90). The total abundance of small mammals, on the other hand, was negatively affected by an increase in wood density (p = 0.02, Figure 6A), and for the rodents alone, a similar result was obtained (p = 0.01, Figure 6B). However, the total abundance of marsupials was not affected by changes in mean EVI (p = 0.61). We also fitted models excluding captures of Necromys lasiurus (due to its dominance in the 2003 survey), and the null models were selected for total and rodent abundances (Total community: p = 0.17; Rodents: p = 0.06).
Figure 6. Predicted effect of mean EVI on the (A) total abundance of small mammals, (B) abundance of rodents, and abundance of different species: (C) Cryptonanus chacoensis, (D) Didelphis albiventris, (E) Cerradomys scotti, (F) Oligoryzomys nigripes, and (G) Necromys lasiurus estimated by generalized linear models for the sampling units in SBES. The shadows represent the 95% confidence intervals. All photos by Bruno Ferreto Fiorillo.
Among the species with at least 10 individuals captured, the rodents Calomys tener and Oligoryzomys mattogrossae seemed to be unaffected by the increase in EVI mean values (p = 0.44 and 0.20, respectively). The marsupial Cryptonanus chacoensis and the rodents Cerradomys scotti and Necromys lasiurus were negatively affected by the local woody encroachment, with a decrease in their abundances related to higher mean EVI (p = 0.001, <0.001, and 0.01, respectively, Figures 6C,E,G). The marsupial D. albiventris and the rodent Oligoryzomys nigripes were positively affected by increases in EVI mean (p = 0.001 and p < 0.001, respectively, Figures 6D,F).
The Beta diversity analysis showed high dissimilarity between the 2003 and 2018 surveys (βtotal = 0.84), which is explained by the replacement of species (βrepl = 0.84 and βrich = 0). The composition in the 2018 survey presented an increase of four species compared to the 2003 survey, D. albiventris, Gracilinanus agilis, Hylaeamys megacephalus, and Rhipidomys cf. macrurus, most of them associated with the “cerradão,” a forest formation (Figure 3).
We found that SBES small mammal community is structured by the main microhabitat characteristics associated with the different habitats, including herb and canopy cover, and shrub and tree density. This community is responding to the advancing woody encroachment in the area through time. Our models indicated that such changes are predictable, with species typical of open-vegetation habitats (such as Cryptonanus chacoensis and Cerradomys scotti) showing declines while forest-dwellers (such as D. albiventris and Oligoryzomys nigripes) are increasing in abundance. Therefore, woody encroachment is changing this community structure in predictive ways and creating “winners and losers,” which turn on an alert about the future of open-area specialists in face of the Cerrado encroachment.
A high diversity of small mammals was found in our study area. We recorded at SBES 15% of the 113 native small mammal species from Cerrado (Mendonça et al., 2018; Fegies et al., 2021). The total richness observed (17 species) is high, given that only about 8% of the Cerrado small mammal communities are composed of more than 10 species (Mendonça et al., 2018), evidencing the completeness of the surveys in SBES. Rodents were the richest order, a recurrent pattern (Quintela et al., 2020; Abreu et al., 2021), with five sigmodontine rodents representing 74% of the total abundance in the SBES assemblage (Oligoryzomys nigripes, O. mattogrossae, Calomys tener, Cerradomys scotti, and Necromys lasiurus). The general abundance pattern recorded for SBES is also in accordance with previous Cerrado studies (see Mendonça et al., 2018 review), with Necromys lasiurus being the dominant species in the 2003 survey, and O. nigripes in the 2017–2018 survey.
Although based on different sampling designs and efforts, the two temporally spaced surveys showed high values of sampling coverage, both for the total assemblages, and for each similar habitat surveyed, allowing general comparisons between the community structure patterns found. While the 2003 survey was characterized by lower species richness, the habitats with higher observed and estimated richness were the grasslands (“campo limpo” and “campo sujo”) and the savanna (“cerrado sensu stricto”), with the forest “cerradão” being the poorest habitat. On the other hand, the higher species richness found in the 2017–2018 survey was evenly distributed among grasslands (“campo sujo”), savannas (“cerrado sensu stricto”) and “cerradão,” but with “campo cerrado,” a grassland habitat, showing lower species richness. Moreover, the seven additional species recorded in the 2017–2018 survey are mostly associated with denser covered habitats, such as savannas and forests, as is the case of D. albiventris, Gracilinanus agilis, Hylaeamys megacephalus, and Rhipidomys cf. macrurus (e.g., Santos-Filho et al., 2012; Carmignotto et al., 2014; Carmignotto, 2019; this study). Indeed, two species typical of the Atlantic Forest (G. microtarsus and Nectomys cf. squamipes), which also occur in southern and eastern Cerrado, especially using gallery forests, seasonal forests, and “cerradão” patches (Costa, 2003; Carmignotto et al., 2012), were only represented in the 2017–2018 survey.
Regarding species abundances, we observed that in the 2003 survey, the most abundant species were also represented by grassland and savanna species. Although Necromys lasiurus is considered a habitat generalist (Vieira et al., 2005; Ribeiro et al., 2019), in Cerrado, it prefers open habitats, represented by grasslands and savannas (Becker et al., 2007; Carmignotto, 2019). A similar pattern was found for C. scotti and O. mattogrossae (Henriques et al., 1997; Vieira et al., 2005; Weksler and Bonvicino, 2015). In the 2017–2018 survey, in turn, the most abundant species, the rodent O. nigripes, is more associated with savanna and forests (Weksler and Bonvicino, 2005, 2015). Moreover, some species that were more abundant in the 2003 survey, became rare in 2017–2018, such as the grassland specialist Cryptonanus aff. chacoensis (Fegies et al., 2021). Vegetation shifts may trigger shifts in small mammal abundance (Loggins et al., 2019), but other factors, such as resource availability, reproductive activity, and presence of competitors (Verberk, 2011), may also play a role. Since several of our comparisons were made between 2003 and 2017–2018 surveys controlling for the period of sampling (January–February), we believe we were able to reduce the influence of some of these confounding factors in our results.
Cerrado small mammals present high habitat association, contributing with the well-known pattern of open (grasslands and savannas) versus forest specialists found across this ecoregion (e.g., Alho, 2005; Carmignotto et al., 2012). The SBES assemblage also fits into this pattern, showing open and forest specialists as well as generalists (occupying both open and forest habitats). Considering habitat use, the 2003 survey was mostly represented by open-habitat species, with few generalists. In the 2017–2018 survey, we observed an increase in generalists and forest specialists. Despite the maintenance of grassland specialists between surveys, two species (Cryptonanus aff. chacoensis and Clyomys laticeps) were restricted to the most open habitat currently present at SBES (“campo sujo”), indicating a strong association with open grasslands (Carmignotto et al., 2014; Bezerra and Bonvicino, 2015; Bezerra et al., 2016; Fegies et al., 2021).
The microhabitat preference of species corroborates the results found at a larger, habitat scale in our study. For instance, Calomys tener and Clyomys laticeps show a higher association with high herbaceous cover (Carmignotto and Aires, 2011; Rocha et al., 2011; Bezerra et al., 2016). For Clyomys laticeps, however, our models indicate a negative relationship with high shrub cover, highlighting the dependence of this species on open grasslands (Vieira, 2003; Bezerra and Bonvicino, 2015). Other rodents (Cerradomys scotti, Oligoryzomys nigripes, and O. mattogrossae) were positively affected by shrub density. Cerradomys scotti and O. mattogrossae are open-habitat species, exhibiting a preference for grassland areas with higher shrub cover (Vieira et al., 2005). Oligoryzomys nigripes, however, is known to be associated with forest habitats, such as gallery forest in the Cerrado (Weksler and Bonvicino, 2005). Our findings are in accordance with these results since most captures were recorded in the “cerradão.” Püttker et al. (2008) also reported a higher association of this species with areas with low canopy and dense understory in the Atlantic Forest. D. albiventris, on the other hand, seems to benefit from increasing canopy cover and tree density, agreeing with previous studies where its presence was related to fallen logs and shrub (Piper sp.) density (Melo et al., 2013). Gracilinanus agilis and Hylaeamys megacephalus showed no clear response to the microhabitat variables tested, and their presence should be due to other factors not considered in our study, such as resource abundance or disturbances (Verberk, 2011; Loggins et al., 2019). These results highlight the importance of evaluating habitat use at different scales to better understand the potential reasons behind differential occurrence of small mammal species across the Cerrado, and to reinforce why some species would be favored or disfavored in a woody encroachment scenario.
We observed an increase in vegetation density in SBES throughout the 15 years between the two surveys analyzed (from 2003 to 2018), corroborating that woody encroachment may be a common phenomenon in the Cerrado (e.g., Moreira, 2000; Roitman et al., 2008; Pinheiro and Durigan, 2009; Abreu et al., 2017, 2021). The 27% increase in the mean EVI affected primarily the grasslands. Indeed, the “campo sujo” surveyed in 2018 were located at the same areas previously classified as “campo limpo” in the 2003 survey, clearly showing a shift in vegetation with the increase in density of shrubs and small trees (see also Melo and Durigan, 2011). Overall, the small mammal species richness was not affected by the woody encroachment observed in the study area, but the total abundance was negatively related to the increase in mean EVI, as observed for other plant and invertebrate groups studied at SBES (Abreu et al., 2017). Indeed, our results show a clear negative effect of woody encroachment on the density of rodents, but not for marsupials. This is expected since most marsupials are associated with savannas or forest habitats due to their scansorial and arboreal habits (Astúa, 2015). Rodents have shown a two-way relationship with vegetation thickening in other open regions. On one hand, they can contribute to seed removal and consequently decrease woody density at habitats in the initial phase of encroachment, as recorded for open habitats of Africa, Argentina, and Australia (Busch et al., 2012; Bergstrom, 2013; Teman et al., 2021). On the other hand, they can be negatively affected by the encroachment, which impacts their ecological features, such as reducing predator detection, habitat use, and local persistence (Loggins et al., 2019), similar to what our results have indicated here. Our results for total and rodent abundance may have been influenced by the high number of Necromys lasiurus individuals in the 2003 survey. When we excluded this species from the analyses, no influence of woody encroachment for small mammals and rodent abundance was detected. Thus, these results should be carefully interpreted due to this outlier influence and low sample size, and additional sampling should be made to confirm our interpretations.
Regarding the seven most abundant species, two open-habitat specialists (C. tener and O. mattogrossae) were not affected by woody encroachment. These species can occupy a wide range of open habitats, from grasslands to savannas in the Cerrado (Carmignotto et al., 2014; Salazar-Bravo, 2015; Weksler et al., 2017; Bezerra et al., 2020). This pattern indicates plasticity in their habitat use. On the other hand, the open-habitat specialists Cryptonanus chacoensis, Cerradomys scotti, and Necromys lasiurus were negatively affected by woody encroachment, indicating a dependence on grasslands formations at SBES. The negative impacts of woody encroachment on species of the genus Cryptonanus are expected, since its diversification is highly associated with the open habitats of the Cerrado (Fegies et al., 2021). In fact, SBES shelters a sympatric species, Cryptonanus aff. chacoensis, considered rare and endemic of Brazilian Cerrado, which may face even more severe impacts by the woody encroachment (Fegies et al., 2021). Cerradomys scotti showed a slight preference for shrubby habitats in our microhabitat analyzes, which is in accordance with the negative response to the tree encroachment found here, a pattern previously observed by Vieira et al. (2005), where this species was related to grass height but not to arboreal cover. In fact, there seems to be a tenuous line for species that may benefit from shrub cover due to protection against predation, and also may be negatively affected by a decrease in food resources, since several species use herbaceous sources for feeding (Vieira et al., 2005; Ribeiro et al., 2019), which, in turn, decrease with shrub encroachment, as shown for small mammals in African shrub-invaded grasslands (Loggins et al., 2019).
The rodent Necromys lasiurus also showed a decrease in abundance between 2003 and 2017–2018 potentially due to woody encroachment, since it is associated with open and grassy areas (Vieira et al., 2005; Becker et al., 2007; Rocha et al., 2011). This species was the most abundant in open areas in 2003 but among the rarest ones in 2018, extending its distribution to savanna habitats, its preferred habitat in other studies (Henriques and Alho, 1991), indicating its habitat plasticity within open formations. Our results show that Cryptonanus chacoensis and Cerradomys scotti may not persist in areas with woody encroachment if grasslands disappear. Meanwhile, forest-specialists and opportunistic species seem to benefit from woody encroachment at the SBES. D. albiventris, although considered a habitat generalist (Cáceres et al., 2012), was not captured in the 2003 survey, but in 2018 became the most abundant marsupial in the assemblage. This didelphid was also the only species that was positively associated with microhabitats with higher tree density, which corroborates its fostering by woody encroachment. The rodent Oligoryzomys nigripes was also favored by the encroachment, and its higher association with forest habitats may allow its spread in habitats with higher woody density (Weksler and Bonvicino, 2015). Mammals across savannas worldwide are differently influenced by vegetation encroachment (Stevens et al., 2016a), but they seem to be more sensitive than other vertebrates, such as birds, due to their specialized habitat preferences and foraging strategies. Turnover in species composition following woody encroachment is also recorded in African savannas, with browser mammals replacing grazers (Smit and Prins, 2015), pointing to woody encroachment as a general concern across savannas.
Our models indicate shifts in species abundance across time, with such changes being predictable to some extent. Species typical of grasslands show declines, while forest-dwellers are increasing in abundance. In the case of forest-savanna ecotone regions, climate, and land-use change, especially fire and deforestation, are leading to an invasion of savanna species into disturbed forests, shifting the forest fauna assemblages toward a “savannization” (Sales et al., 2020). Similarly, the woody encroachment is changing southern Cerrado assemblages toward a “forestization,” with the invasion of species from adjacent forest biomes and the loss of savanna specialists (Abreu et al., 2017, 2021). Our findings highlight the importance of the maintenance of the mosaic of open formations in Cerrado remnants in order to shelter a high diversity of small mammal grassland specialists. Species that showed a clear decline and high association with open vegetation structure in fact can become locally extinct, consequently altering species range, since these areas are mainly located at Cerrado boundaries. This may be particularly true for Cerrado endemics and regionally vulnerable species such as Clyomys laticeps and Cerradomys scotti (Percequillo et al., 2008; São Paulo, 2018), besides a rare and still undescribed species (Cryptonanus aff. chacoensis; Fegies et al., 2021). In fact, the SBES was created with the goal to protect the open formations of the southern Cerrado, but 30 years of fire suppression are probably the main cause of the local woody encroachment and the resulting changes in biodiversity (Abreu et al., 2017, 2021). Fire management is considered a key tool to maintain open savannas and its associated diversity, and the current fire experiments at SBES so far demonstrate no loss in small mammal diversity with prescribed fire (Durigan and Ratter, 2016; Durigan et al., 2020). Without active management of the landscape to keep open habitats, the long-term maintenance of the open-habitat specialists (with special attention to the rarest ones) will give place to an increasing replacement by forest specialists and habitat generalists in the SBES small mammal community and in other remnants in the southern portion of the Cerrado. Woody encroachment needs to be treated as a global scale problem to natural open ecosystems (Stevens et al., 2016a), and its impacts on biodiversity at local scales should continue to be investigated in order to guarantee the conservation of savanna and grassland biodiversity.
The original contributions presented in the study are included in the article/Supplementary Material; further inquiries can be directed to the corresponding author/s.
The animal study was reviewed and approved by Comissão de Ética no Uso de Animais (CEUA), Instituto de Biociências da Universidade de São Paulo (#CEUA-IB-USP 241/2016).
APC, AVC, and MM conceived this study and designed the methodology. APC, PL, GF, and LF collected the data. LF performed the statistical analysis and led the manuscript writing. APC, AVC, LF, and MM interpreted results and contributed to the writing and reviews. All authors gave final approval for submission.
AVC was funded by Neotropical Grassland Conservancy, MM and APC were funded by FAPESP grants #2015/21259-8, #2018/14091-1, and #2020/12658-4, APC was also funded by FAPESP grants #98/05075-7 and #00/06642-4, and LF was funded by CAPES – Finance Code 001.
The authors declare that the research was conducted in the absence of any commercial or financial relationships that could be construed as a potential conflict of interest.
All claims expressed in this article are solely those of the authors and do not necessarily represent those of their affiliated organizations, or those of the publisher, the editors and the reviewers. Any product that may be evaluated in this article, or claim that may be made by its manufacturer, is not guaranteed or endorsed by the publisher.
We are grateful to the Santa Barbara Ecological Station management team, especially Marcos Pestana, and Instituto Florestal for all the support during the field campaigns. We are also thankful to Bárbara Armando Godinho, Bruna de França Gomes, Bruno Ferreto Fiorillo, Carolina Farhat, Carolina Henkes Inamassu, Evelin de Campos da Costa, Giordano Novak Rossi, Guilherme Zamarian Rezende, Jairo Roldan, Jorge Henry Maciel, Julia dos Santos Gutierres, Karine Yumi Tominaga, and Mirela Alcolea for the assistance in data collection; Bruno Ferreto Fiorillo for kindly providing the small mammal photos; João Paulo dos Santos Vieira-Alencar and Carolina Freitas Schlosser for help with the EVI values extraction; and Yohann Freddi for the assistance with the software programs. We also thank Giselda Durigan and Alexandre Reis Percequillo for valuable suggestions for this study. The illustrations from Figure 5 were modified from vectors designed by Freepik. The collection permit was issued by Instituto Chico Mendes de Conservação da Biodiversidade (ICMBio) #50658-1.
The Supplementary Material for this article can be found online at: https://www.frontiersin.org/articles/10.3389/fevo.2021.774744/full#supplementary-material
Abreu, R. C. R., Durigan, G., Melo, A. C. G., Pilon, N. A. L., and Hoffmann, W. A. (2021). Facilitation by isolated trees triggers woody encroachment and a biome shift at the savanna–forest transition. J. Appl. Ecol. 58, 2650–2660. doi: 10.1111/1365-2664.13994
Abreu, R. C. R., Hoffmann, W. A., Vasconcelos, H. L., Pilon, N. A. L., Rossatto, D. R., and Durigan, G. (2017). The biodiversity cost of carbon sequestration in tropical savanna. Sci. Adv. 3:e1701284. doi: 10.1126/sciadv.1701284
Alencar, A., Shimbo, J. Z., Lenti, F., Marques, C. B., Zimbres, B., Rosa, M., et al. (2020). Mapping three decades of changes in the brazilian savanna native vegetation using landsat data processed in the google earth engine platform. Remote Sens. 12:924. doi: 10.3390/rs12060924
Alho, C. J. R. (2005). Intergradation of habitats of non-volant small mammals in the patchy Cerrado landscape. Arq. Mus. Nac 63, 41–48.
Alvares, C. A., Stape, J. L., Sentelhas, P. C., Gonçalves, J. L. M., and Sparovek, G. (2013). Köppen’s climate classification map for Brazil. Meteor. Zeitsc. 22, 711–728. doi: 10.1127/0941-2948/2013/0507
Andersen, E. M., and Steidl, R. J. (2019). Woody plant encroachment restructures bird communities in semiarid grasslands. Biol. Conserv. 240:108276. doi: 10.1016/j.biocon.2019.108276
Araujo, C. O., Côrrea Filho, D. T., and Sawaya, R. J. (2010). Snake assemblage of estação ecológica de santa bárbara, sp: a cerrado remnant in Southeastern Brazil. Bio. Neotrop. 10, 235–245. doi: 10.1590/S1676-06032010000200026
Archer, S. R. (1994). “Woody plant encroachment into southwestern grasslands and savannas: rates, patterns and proximate causes,” in Ecological Implications of Livestock Herbivory in the West, eds M. Vavra, W. A. Laycock, and R. D. Pieper (Denver, CO: Society for Range Management).
Archer, S. R., Andersen, E. M., Predick, K. I., Schwinning, S., Steidl, R. J., and Woods, S. R. (2017). “Woody plant encroachment: causes and consequences,” in Rangeland Systems - Processes, Management and Challenges, ed. D. D. Briske (New York, NY: Springer Series on Environmental Management), 25–84.
Astúa, D. (2015). Morphometrics of the largest new world marsupials, opossums of the genus Didelphis (Didelphimorphia, Didelphidae). Oecol. Aust. 19, 117–142. doi: 10.4257/oeco.2015.1901.08
Bates, D., Maechler, M., Bolker, B., Walker, S., Christensen, R. H. B., Singmann, H., et al. (2021). Package ‘lme4’. linear mixed-effects models using ‘Eigen’ and S4. J. Stat. Softw. 67, 1–97.
Becker, R. G., Paise, G., Baumgarten, L. C., and Vieira, E. M. (2007). Estrutura de comunidades de pequenos mamíferos e densidade de Necromys lasiurus (Rodentia, Sigmodontinae) em áreas abertas de Cerrado no Brasil central. Mast. Neot. 14, 157–168.
Bergstrom, B. J. (2013). Would East African savanna rodents inhibit woody encroachment? Evidence from stable isotopes and microhistological analysis of feces. J. Mamm. 94, 436–447. doi: 10.1644/12-MAMM-A-146.1
Bezerra, A. M. R., and Bonvicino, C. R. (2015). “Genus clyomys thomas, 1916,” in Mammals of South America, Volume 2 Rodents, eds J. L. Patton, U. F. J. Pardiñas, and G. D’Elía (Chicago, IL: The University of Chicago Press), 417–437.
Bezerra, A. M. R., Castiglia, R., Pereira, L. G., Moreira, J. C., and Bonvicino, C. R. (2020). Molecular systematics of the genus Necromys (Rodentia: Cricetidae: Sigmodontinae) reveals two cryptic and syntopic species in western Cerrado of Brazil. Zool. Anz. 285, 147–158. doi: 10.1016/j.jcz.2020.02.007
Bezerra, A. M. R., Oliveira, J. A., and Bonvicino, C. R. (2016). Clyomys laticeps (Rodentia: Echimyidae). Mamm. Spec. 48, 83–90. doi: 10.1093/mspecies/sew009
Blaser, W. J., Shanungu, G. K., Edwards, P. J., and Venterink, H. O. (2014). Woody encroachment reduces nutrient limitation and promotes soil carbon sequestration. Ecol. Evol. 4, 1423–1438. doi: 10.1002/ece3.1024
Bond, W. J., and Keeley, J. E. (2005). Fire as a global ‘herbivore’: the ecology and evolution of flammable ecosystems. Trends Ecol. Evol. 20, 387–394. doi: 10.1016/j.tree.2005.04.025
Bond, W. J., and Midgley, G. F. (2000). A proposed CO2-controlled mechanism of woody plant invasion in grasslands and savannas. Glob. Change Biol. 6, 865–869. doi: 10.1046/j.1365-2486.2000.00365.x
Bowman, D. M. J. S., Kolden, C. A., Abatzoglou, J. T., Johnston, F. H., Werf, G. R., and Flannigan, M. (2020). Vegetation fires in the Anthropocene. Nat. Rev. Earth Environ. 1, 500–515. doi: 10.1038/s43017-020-0085-3
Busch, M., Knight, C., Mazía, C. N., Hodara, K., Muschetto, E., and Chaneton, E. (2012). Rodent seed predation on tree invader species in grassland habitats of the inland Pampa. Ecol. Res. 27, 369–376. doi: 10.1007/s11284-011-0909-1
Cáceres, N. C., Prevedello, J. A., and Loretto, D. (2012). “Uso do espaço por marsupiais: fatores influentes, comportamento e heterogeneidade espacial,” in Os marsupiais do Brasil: biologia, ecologia e evolução, ed. N. C. Cáceres (Campo Grande, BR: UFMS), 327–346.
Cardoso, P., Rigal, F., and Carvalho, J. C. (2015). BAT – biodiversity assessment tools, an R package for the measurement and estimation of alpha and beta taxon, phylogenetic and functional diversity. Met. Ecol. Evol. 6, 232–236. doi: 10.1111/2041-210X.12310
Carmignotto, A. P. (2005). Pequenos Mamíferos Terrestres do Bioma Cerrado: Padrões Faunísticos Locais e Regionais. Ph.D. thesis. São Paulo, BR: Universidade de São Paulo.
Carmignotto, A. P. (2019). Effects of damming on a small mammal assemblage in Central Brazilian Cerrado. Bol. Soc. Bras. Mastozool. 85, 63–73.
Carmignotto, A. P., and Aires, C. C. (2011). Non-volant mammals (Mammalia) from estação ecológica serra geral do tocantins. Bio. Neotrop. 11, 307–322. doi: 10.1590/S1676-06032011000100029
Carmignotto, A. P., Bezerra, A. M. R., and Rodrigues, F. H. G. (2014). Nonvolant small mammals from a southwestern area of Brazilian Cerrado: diversity, habitat use, seasonality, and biogeography. Therya 5, 535–558. doi: 10.12933/therya-14-197
Carmignotto, A. P., Vivo, M., and Langguth, A. (2012). “Mammals of the cerrado and caatinga: distribution patterns of the tropical open biomes of Central South America,” in Bones, Clones, and Biomes: History and Geography of Recent Neotropical Mammals, eds B. D. Patterson and L. P. Costa (Chicago, IL: University of Chicago Press).
Carvalho, J. C., Cardoso, P., and Gomes, P. (2012). Determining the relative roles of species replacement and species richness differences in generating beta-diversity patterns. Glob. Ecol. Biogeogr. 21, 760–771. doi: 10.1111/j.1466-8238.2011.00694.x
Chao, A., Chiu, C. H. (2016). “Species richness: estimation and comparison,” in Wiley StatsRef: Statistics Reference Online, eds Balakrishnan, N., Colton, T., Everitt, B., Piegorsch, W., Ruggeri, F., and Teugels, J. (Hoboken, NJ: JohnWiley & Sons, Ltd), 1–26.
Chao, A., Gotelli, N. J., Hsieh, T. C., Sander, E. L., Ma, K. H., Colwell, R. K., et al. (2014). Rarefaction and extrapolation with Hill numbers: a framework for sampling and estimation in species diversity studies. Ecol. Monogr. 84, 45–67. doi: 10.1890/13-0133.1
Chaves, M. E. D., Lázaro, A. S., Nassur, O. A. C., and Conceição, F. G. (2013). “Utilização dos índices de vegetação EVI e NDVI como ferramenta de análise da dinâmica da vegetação no Parque Nacional da Serra da Canastra – MG,” in Proceedings of the XVI Simpósio Brasileiro de Sensoriamento Remoto - SBSR, (Brasil: INPE).
CIIAGRO (2016). Centro Integrado de Informações Agrometeorológicas. Available online at: http://www.ciiagro.sp.gov.br/ciiagroonline/ (accessed August 11, 2021).
Colwell, R. K., Chao, A., Gotelli, N. J., Lin, S., Mao, C. X., Chazdon, R. L., et al. (2012). Models and estimators linking individual-based and sample-based rarefaction, extrapolation and comparison of assemblages. J. Plant Ecol. 5, 3–21. doi: 10.1093/jpe/rtr044
Conciani, D. E., Santos, L., Silva, T. S. F., Durigan, G., and Alvarado, S. T. (2021). Human–climate interactions shape fire regimes in the Cerrado for São Paulo State, Brazil. J. Nat. Conserv. 61:126006. doi: 10.1016/j.jnc.2021.126006
Corrêa, M. R. J., Ballagamba, Y. M., Magalhães, A. P., Martins, J. P. V., Antônio, J. R. C., Kozovitz, A. R., et al. (2017). Microhabitat structure and food availability modelling a small mammal assemblage in restored riparian forest remnants. Mammalia 82:4. doi: 10.1515/mammalia-2017-0026
Costa, L. P. (2003). The historical bridge between the Amazon and the Atlantic Forest of Brazil: a study of molecular phylogeography with small mammals. J. Biogeogr. 30, 71–86. doi: 10.1046/j.1365-2699.2003.00792.x
Dias, S. C. (2004). Planejando estudos de diversidade e riqueza: uma abordagem para estudantes de graduação. Acta Sci. Biol. Sci. 26, 373–379. doi: 10.4025/actascibiolsci.v26i4.1511
Durigan, G., Pilon, N. A. L., Abreu, R. C. R., Hoffmann, W. A., Martins, M., Fiorillo, B. F., et al. (2020). No net loss of species diversity after prescribed fires in the Brazilian Savanna. Front. Glob. Change 3:13. doi: 10.3389/ffgc.2020.00013
Durigan, G., and Ratter, J. A. (2016). The need for a consistent fire policy for Cerrado conservation. J. Appl. Ecol. 53, 11–15. doi: 10.1111/1365-2664.12559
Eldridge, D. J., Bowker, M. A., Maestre, F. T., Roger, E., Reynolds, J. F., and Whitford, W. G. (2011). Impacts of shrub encroachment on ecosystem structure and functioning: towards a global synthesis. Ecol. Lett. 14, 709–722. doi: 10.1111/j.1461-0248.2011.01630.x
Eldridge, D. J., and Ding, J. (2021). Remove or retain: ecosystem effects of woody encroachment and removal are linked to plant structural and functional traits. New Phytol. 229, 2637–2646. doi: 10.1111/NPH.17045
Fegies, A. C., Carmignotto, A. P., Perez, M. F., Guilardi, M. D., and Lessinger, A. C. (2021). Molecular phylogeny of Cryptonanus (Didelphidae: Thylamyini): evidence for a recent and complex diversification in South American open biomes. Mol. Phyl. Evol. 162:107213. doi: 10.1016/j.ympev.2021.107213
Fensham, R. J., Fairfax, R. J., and Archer, R. (2005). Rainfall, land use and woody vegetation cover change in semi-arid Australian savanna. J. Ecol. 93, 596–606. doi: 10.1111/j.1365-2745.2005.00998.x
Fidelis, A. (2020). Is fire always the “bad guy”? Flora 268:151611. doi: 10.1016/j.flora.2020.151611
Fidelis, A., and Blanco, C. (2014). Does fire induce flowering in Brazilian subtropical grasslands? Appl. Veget. Sci. 17, 690–699. doi: 10.1111/avsc.12098
Freitas, S. R., Cerqueira, R., and Vieira, M. V. (2002). A device and standard variables to describe microhabitat structure of small mammals based on plant cover. Braz. J. Biol. 62, 795–800. doi: 10.1590/S1519-69842002000500008
García Criado, M., Myers-Smith, I. H., Bjorkman, A. D., Lehmann, C. E. R., and Stevens, N. (2020). Woody plant encroachment intensifies under climate change across tundra and savanna biomes. Glob. Ecol. Biogeogr. 29, 925–943. doi: 10.1111/geb.13072
Gutiérrez, E. E., and Marinho-Filho, J. (2017). The mammalian faunas endemic to the Cerrado and the Caatinga. ZooKeys 644, 105–157. doi: 10.3897/zookeys.644.10827
Harting, F. (2021). DHARMa: Residual Diagnostics for Hierarchical (Multi-Level / Mixed) Regression Models. R Topics Documented. R Package Version 0.4.1. Available online at: https://CRAN.R-project.org/package=DHARMa
Henriques, R. P. B., and Alho, C. J. R. (1991). Microhabitat selection by two rodent species in the Cerrado of Central Brazil. Mammalia 55, 49–56. doi: 10.1515/mamm.1991.55.1.49
Henriques, R. P. B., Bizerril, M. X. A., and Kohlsdorf, T. (1997). “Abundância, riqueza e seleção de habitat de pequenos mamíferos dos cerrados do Brasil Central,” in Contribuição ao Conhecimento Ecológico do Cerrado, eds L. L. Leite and C. H. Saito (Brasília: Universidade de Brasília), 127–130.
Honda, E. A., and Durigan, G. (2016). Woody encroachment and its consequences on hydrological processes in the savannah. Phil. Trans. R. Soc. B. 371:20150313. doi: 10.1098/rstb.2015.0313
Hsieh, T. C., Ma, K. H., and Chao, A. (2016). iNEXT: an R package for rarefaction and extrapolation of species diversity (Hill numbers). Met. Ecol. Evol. 7, 1451–1456. doi: 10.1111/2041-210X.12613
Huxman, T. E., Wilcox, B. P., Breshears, D. D., Scott, R. L., Snyder, K. A., Small, E. E., et al. (2005). Ecohydrological implications of woody plant encroachment. Ecology 86, 308–319. doi: 10.1890/03-0583
Jorgensen, E. E. (2004). Small mammal use of microhabitat reviewed. J. Mamm. 85, 531–539. doi: 10.1644/BER-019
Loggins, A. A., Monadjem, A., Kruger, L. M., Reichert, B. E., and McCleery, R. A. (2019). Vegetation structure shapes small mammal communities in African savannas. J. Mamm. 100, 1243–1252. doi: 10.1093/jmammal/gyz100
Lüdecke, D. (2018). ggeffects: Tidy data frames of marginal effects from regression models. J. Open Sour. Softw. 3:772. doi: 10.21105/joss.00772
Melo, A. C. G., and Durigan, G. (2011). Plano de Manejo da Estação Ecológica de Santa Bárbara. São Paulo: Instituto Florestal - Secretaria do Meio Ambiente.
Melo, G. L., Miotto, B., Peres, B., and Cáceres, N. C. (2013). Microhabitat of small mammals at ground and understorey levels in a deciduous, southern Atlantic Forest. Acad. Bras. Cienc. 85:2. doi: 10.1590/S0001-37652013000200017
Mendonça, A. F., Percequillo, A. R., Camargo, N. F., Ribeiro, J. F., Palma, A. R. T., Oliveira, L. C., et al. (2018). Cerrado small mammals: a dataset of abundance and distribution of marsupials, lagomorphs, and rodents in a neotropical savanna. Ecology 99:1900. doi: 10.1002/ecy.02367
Mitchard, E. T. A., and Flintrop, C. M. (2013). Woody encroachment and forest degradation in sub-Saharan Africa’s woodlands and savannas 1982–2006. Phil. Trans. R. Soc. B. 368:20120406. doi: 10.1098/rstb.2012.0406
Moreira, A. G. (2000). Effects of fire protection on savanna structure in Central Brazil. J. Biogeogr. 27, 1021–1029.
Moreno, C. E. (2001). Métodos Para Medir la Biodiversidad: Manuales y Tesis SEA. Zaragosa: Programa Iberoamericano de Ciencia y Tecnología para el Desarrollo.
Motta, P. E. F., Curi, N., and Franzmeier, D. P. (2002). “Relation of soils and geomorphic surfaces in the Brazilian Cerrado,” in The Cerrados of Brazil: Ecology and Natural History of a Neotropical Savanna, eds P. S. Oliveira and R. J. Marquis (Chicago,IL: Columbia University Press), 13–32.
Oksanen, J., Blanchet, F. G., Friendly, M., Kindt, R., Legendre, P., McGlinn, D., et al. (2020). Package ‘vegan’ Community Ecology Package. R Package Version 2.5-7. Available online at: https://CRAN.R-project.org/package=vegan
Oliveira-Filho, A. T., and Ratter, J. A. (2002). “Vegetation physiognomies and woody flora of the cerrado biome,” in The Cerrados of Brazil: Ecology and Natural History of a Neotropical Savanna, eds P. S. Oliveira and R. J. Marquis (Chicago, IL: Columbia University Press), 91–120.
Paglia, A. P., Fonseca, G. A. B., Rylands, A. B., Herrmann, G., Aguiar, L. M. S., Chiarello, A. G., et al. (2012). “Annotated checklist of brazilian mammals,” in Paper Presentated at Conservation Biology, 2nd Edn, (Arlington, TX: Conservation International).
Pardini, R., Bueno, A. A., Gardner, T. A., Prado, P. I., and Metzger, J. P. (2010). Beyond the fragmentation threshold hypothesis: regime shifts in biodiversity across fragmented landscapes. PLoS One 5:e13666. doi: 10.1371/journal.pone.0013666
Parr, C. L., Lehmann, C. E. R., Bond, W. J., Hoffmann, W. A., and Andersen, A. N. (2014). Tropical grassy biomes: misunderstood, neglected, and under threat. Trends Ecol. Evol. 29, 205–213. doi: 10.1016/j.tree.2014.02.004
Passos, F. B., Marimon, B. S., Phillips, O. L., Morandi, P. S., Neves, E. C., Elias, F., et al. (2018). Savanna turning into forest: concerted vegetation change at the ecotone between the Amazon and “Cerrado” biomes, Brazil. J. Bot. 41, 611–619. doi: 10.1007/s40415-018-0470-z
Patton, J. L., Pardiñas, U. F. J., and D’Elía, G. (2015). Mammals of South America, Volume 2 Rodents. Chicago, IL: The University of Chicago Press.
Percequillo, A. R., Hingst-Zaher, E., and Bonvicino, C. R. (2008). Systematic Review of genus cerradomys Weksler, Percequillo and Voss, 2006 (Rodentia: Cricetidae: Sigmodontinae: Oryzomyini), with description of two new species from eastern Brazil. Am. Mus. Nat. Hist. 3622, 1–46.
Pilon, N. A. L., Cava, M. G. B., Hoffmann, W. A., Abreu, R. C. R., Fidelis, A., and Durigan, G. (2020). The diversity of post-fire regeneration strategies in the cerrado ground layer. J. Ecol. 109, 154–166. doi: 10.1111/1365-2745.13456
Pilon, N. A. L., Hoffmann, W. A., Abreu, R. C. R., and Durigan, G. (2018). Quantifying the short-term flowering after fire in some plant communities of a cerrado grassland. Plant Ecol. Div. 11, 259–266. doi: 10.1080/17550874.2018.1517396
Pinheiro, E. S., and Durigan, G. (2009). Dinâmica espaço-temporal (1962-2006) das fitofisionomias em unidade de conservação do Cerrado no sudeste do Brasil. Ver. Bras. Bot. 32, 441–454. doi: 10.1590/S0100-84042009000300005
Price, J. N., and Morgan, J. W. (2008). Woody plant encroachment reduces species richness of herb-rich woodlands in southern Australia. Aust. Ecol. 33, 278–289. doi: 10.1111/j.1442-9993.2007.01815.x
Püttker, T., Pardini, R., Meyer-Lucht, Y., and Sommer, S. (2008). Responses of five small mammal species to micro-scale variations in vegetation structure in secondary Atlantic Forest remnants, Brazil. BMC Ecol. 8:9. doi: 10.1186/1472-6785-8-9
QGIS (2021). QGIS Geographic Information System. Available online at: http://www.qgis.org (accessed September 12, 2021).
Quintela, F. M., Da Rosa, C. A., and Feijó, A. (2020). Updated and annotated checklist of recent mammals from Brazil. Acad. Bras. Cienc. 92, (Suppl.2):e20191004. doi: 10.1590/0001-3765202020191004
R Core Team (2021). R: A Language and Environment For Statistical Computing. R Foundation for Statistical Computing. Vienna: R Core Team.
Ratajczak, Z., Nippert, J. B., and Collins, S. L. (2012). Woody encroachment decreases diversity across North American grasslands and savannas. Ecology 93, 697–703. doi: 10.2307/23213717
Ribeiro, J. F., Guaraldo, A., Nardoto, G. B., Santoro, G., and Vieira, E. M. (2019). Habitat type and seasonality influence the isotopic trophic niche of small mammals in a neotropical savanna. Hystrix It. J. Mamm. 30, 30–38. doi: 10.4404/hystrix-00150-2018
Rocha, C. R., Ribeiro, R., Takahashi, F. S. C., and Marinho-Filho, J. (2011). Microhabitat use by rodent species in a central Brazilian cerrado. Mamm. Biol. 76, 651–653. doi: 10.1016/j.mambio.2011.06.006
Roitman, I., Felfili, J. M., and Rezende, A. V. (2008). Tree dynamics of a fire-protected cerrado sensu stricto surrounded by forest plantations, over a 13-year period (1991–2004) in Bahia, Brazil. Plant Ecol. 197, 255–267. doi: 10.1007/s11258-007-9375-9
Rosan, T. M., Aragão, L. E. O. C., Oliveiras, I., Phillips, O. L., Malhi, Y., Gloor, E., et al. (2019). Extensive 21st-century woody encroachment in South America’s Savanna. Geophys. Res. Lett. 46, 6594–6603.
Salazar-Bravo, J. (2015). “Genus calomys waterhouse, 1837,” in Mammals of South America, Volume 2 Rodents, eds J. L. Patton, U. F. J. Pardiñas, and G. D’Elía (Chicago, IL: The University of Chicago Press), 417–437.
Sales, L. P., Galetti, M., and Pires, M. M. (2020). Climate and land-use change will lead to a faunal “savannization” on tropical rainforests. Glob. Change Biol. 26, 7036–7044. doi: 10.1111/gcb.15374
Sano, E. E., Rosa, R., Brito, J. L. S., and Ferreira, L. G. (2010). Land cover mapping of the tropical savanna region in Brazil. Environ. Monit. Assess. 166, 113–124. doi: 10.1007/s10661-009-0988-4
Santos-Filho, M., Frieiro-Costa, F., Ignácio, ÁR. A., and Silva, M. N. F. (2012). Use of habitats by non-volant small mammals in Cerrado in Central Brazil. Braz. J. Biol. 72, 893–902. doi: 10.1590/S1519-69842012000500016
São Paulo (2018). Decreto n° 63.853, de 27 de Novembro de 2018. São Paulo: Assembleia Legislativa do Estado de São Paulo.
Sikes, R. S. The Animal Care., and Use Committee of the American Society of Mammalogists. (2016). 2016 guidelines of the american society of mammalogists for the use of wild mammals in research and education. J. Mamm. 97, 663–688. doi: 10.1093/jmammal/gyw078
Simon, M. F., Grether, R., Queiroz, L. P., Skema, C., Pennington, R. T., and Hughes, C. E. (2009). Recent assembly of the Cerrado, a neotropical plant diversity hotspot, by in situ evolution of adaptations to fire. Proc. Natl. Acad. Sci. U.S.A. 106, 20359–20364. doi: 10.1073/pnas.0903410106
Smit, I. P. J., and Prins, H. H. T. (2015). Predicting the effects of woody encroachment on mammal communities, grazing biomass and fire frequency in African Savannas. PLoS One 10:e0137857. doi: 10.1371/journal.pone.0137857
Stancampiano, A. J., and Schnell, G. D. (2004). Microhabitat affinities of small mammals in southwestern Oklahoma. J. Mamm. 85, 948–958. doi: 10.1644/BFW-005
Stanton, R. A., Boone, W. W., Soto-Shoender, J., Fletcher, R. J., Blaum, N., and McCleery, R. A. (2017). Shrub encroachment and vertebrate diversity: a global meta-analysis. Glob. Ecol. Biogeogr. 27, 368–379. doi: 10.1111/geb.12675
Stevens, N., Lehmann, C. E. R., Murphy, B. P., and Durigan, G. (2016a). Savanna woody encroachment is widespread across three continents. Glob. Change Biol. 23, 235–244. doi: 10.1111/gcb.13409
Stevens, N., Erasmus, B. F. N., Archibald, S., and Bond, W. J. (2016b). Woody encroachment over 70 years in South African savannahs: overgrazing, global change or extinction aftershock? Phil. Trans. R. Soc. B. 371:20150437. doi: 10.1098/rstb.2015.0437
Teman, S. J., Stevens, N., Monadjem, A., Fletcher, R. J. Jr., Austin, J. D., and McCleery, R. (2021). Savanna rodents’ selective removal of an encroaching plant’s seeds increased with grass biomass. Front. Ecol. Evol. 9:676572. doi: 10.3389/fevo.2021.676572
Van Auken, O. W. (2009). Causes and consequences of woody plant encroachment into western North American grasslands. J. Environ. Manag. 90, 2931–2942. doi: 10.1016/j.jenvman.2009.04.023
Verberk, W. C. E. P. (2011). Explaining general patterns in species abundance and distributions. Nat. Educ. Knowl. 3:38.
Vieira, E. M., Iob, G., Briani, D. C., and Palma, A. R. T. (2005). Microhabitat selection and daily movements of two rodents (Necromys lasiurus and Oryzomys scotti) in Brazilian Cerrado, as revealed by a spool-and-line device. Mamm. Biol. 70, 359–365. doi: 10.1016/j.mambio.2005.08.002
Vieira, M. V. (2003). Seasonal niche dynamics in coexisting rodents of the Brazilian Cerrado. Stud. Neotrop. Fauna Environ. 38, 7–15. doi: 10.1076/snfe.38.1.7.14034
Voss, R. S., and Jansa, S. A. (2009). Phylogenetic relationships and classification of didelphid marsupials, an extant radiation of new world metatherian mammals. Bull. Am. Mus. Nat. His. 322, 1–177. doi: 10.1206/322.1
Weksler, M., and Bonvicino, C. R. (2005). Taxonomy of pigmy rice rats genus Oligoryzomys bangs, 1900 (Rodentia, Sigmodontinae) of the Brazilian cerrado, with the description of two new species. Arq. Mus. Nac 63, 113–130.
Weksler, M., and Bonvicino, C. R. (2015). “Genus oligoryzomys bangs, 1900,” in Mammals of South America, Volume 2 Rodents, eds J. L. Patton, U. F. J. Pardiñas, and G. D’Elía (Chicago, IL: The University of Chicago Press), 417–437. doi: 10.7208/chicago/9780226169606.001.0001
Weksler, M., Lemos, E. M. S., D’Andrea, S., and Bonvicino, C. R. (2017). The taxonomic status of Oligoryzomys mattogrossae (Allen 1916) (Rodentia: Cricetidae: Sigmodontinae), reservoir of Anajatuba Hantavirus. Amer. Mus. Nov. 3880, 1–32. doi: 10.1206/3880.1
Whittaker, R. H. (1972). Evolution and measurement of species diversity. Taxon 21, 213–251. doi: 10.2307/1218190
Keywords: Cerrado (Brazilian savanna), Didelphimorphia, EVI, microhabitat selectivity, habitat use, Rodentia, fire suppression, grasslands
Citation: Furtado LO, Felicio GR, Lemos PR, Christianini AV, Martins M and Carmignotto AP (2021) Winners and Losers: How Woody Encroachment Is Changing the Small Mammal Community Structure in a Neotropical Savanna. Front. Ecol. Evol. 9:774744. doi: 10.3389/fevo.2021.774744
Received: 12 September 2021; Accepted: 18 November 2021;
Published: 16 December 2021.
Edited by:
Emerson M. Vieira, Universidade de Brasília, BrazilReviewed by:
Ian Radford, Department of Biodiversity, Conservation and Attractions (DBCA), AustraliaCopyright © 2021 Furtado, Felicio, Lemos, Christianini, Martins and Carmignotto. This is an open-access article distributed under the terms of the Creative Commons Attribution License (CC BY). The use, distribution or reproduction in other forums is permitted, provided the original author(s) and the copyright owner(s) are credited and that the original publication in this journal is cited, in accordance with accepted academic practice. No use, distribution or reproduction is permitted which does not comply with these terms.
*Correspondence: Luciana O. Furtado, bHVjaWFuYWZ1cnRhZG9AdXNwLmJy, ZnVydGFkby5sb2ZAZ21haWwuY29t
Disclaimer: All claims expressed in this article are solely those of the authors and do not necessarily represent those of their affiliated organizations, or those of the publisher, the editors and the reviewers. Any product that may be evaluated in this article or claim that may be made by its manufacturer is not guaranteed or endorsed by the publisher.
Research integrity at Frontiers
Learn more about the work of our research integrity team to safeguard the quality of each article we publish.