- 1Division of Science, Yale-NUS College, Singapore, Singapore
- 2Institute for Biodiversity and Environmental Research, Universiti Brunei Darussalam, Gadong, Brunei
- 3Institute of Biological Sciences, Faculty of Science, University of Malaya, Kuala Lumpur, Malaysia
Although morphological adaptations leading to crypsis or mimicry have been studied extensively, their interaction with particular behaviors to avoid detection or recognition is understudied. Yet animal behaviors interact with morphology to reduce detection risk, and the level of protection conferred likely changes according to the surrounding environment. Apart from providing a locational cue for predators, prey motion can also serve as concealing behavior in a dynamic environment to prevent detection by potential predators or prey. Phasmids are conventionally known to rely on their adaptive resemblance to plant parts for protection, and this resemblance may vary across life stages and species. However, little is known about how their behaviors interact with their appearance and their environment. We investigated two species of phasmids with varying morphology and color patterns at different ontogenetic stages and examined their behavioral responses to a wind stimulus as a proxy for a dynamic environment. While adult behaviors were mostly species-specific, behavioral responses of nymphs varied with appearance and environmental condition. Display of different behaviors classified as revealing was positively correlated, while the display of concealing behaviors, except for swaying, was mostly negatively correlated with other behaviors. Exhibition of specific behaviors varied with appearance and environmental condition, suggesting that these behavioral responses could help reduce detection or recognition cues. We discuss the differences in behavioral responses in the context of how the behaviors could reveal or conceal the phasmids from potential predators. Our results provide a novel investigation into adaptive resemblance strategies of phasmids through the interaction of behavior and morphology, and highlight the importance of considering the effects of dynamic environments on sending and receiving cues.
Introduction
Predator–prey relationships are fundamental in shaping animal morphology and animal behavior. In the constant evolutionary arms race (Dawkins and Krebs, 1979), predators need to be capable of detecting and catching prey, while potential prey need to avoid detection or escape capture through morphological or behavioral adaptations (Ruxton et al., 2018). The chances of survival for prey can increase through minimizing cues that predators could exploit. Adaptive resemblance allows the prey to dupe a potential predator by pretending to be an inanimate object (Starrett, 1993). Concealing behaviors, such as specific postures or resting habits, can interact with morphological adaptations and decrease the risk of detection or recognition, thus improving protection from predators (Stevens and Ruxton, 2019). However, the undetectability of an inanimate object can also be broken by motion (Regan and Beverley, 1984; Ioannou and Krause, 2009; Hall et al., 2013; Cuthill, 2019). Revealing behaviors, such as locomotion, can provide information about the presence and location of an animal and function as a cue for predators (Ioannou and Krause, 2009). Although avoiding motion is clearly an effective way of reducing the risk of detection, other strategies have evolved to minimize cues that reveal the presence, location, or identity of an animal (Tan and Elgar, 2021). Motion can even decrease the probability of detection or recognition through motion camouflage or motion masquerade (Stevens and Merilaita, 2009; Hall et al., 2017; Cuthill, 2019).
Depending on the prevailing environmental conditions, animals can show different behavioral responses to improve their protection. Cryptically colored animals often modify their flight responses depending on their level of exposure (Cuadrado et al., 2001; Maritz, 2012). Behavioral modifications of resemblance strategies are especially important if the environment of the animal is not static but changes dynamically, e.g., through moving backgrounds or changes in illumination (Cuthill et al., 2019). Wind can be the underlying cause of a dynamic environment, e.g., by agitating leaves or creating water caustics (Cuthill et al., 2019). If the anti-predator behavior of an animal relies on blending in with its environment, it is necessary to take this dynamically changing background into account. For instance, several species of phasmids are known to sway in response to a wind stimulus (e.g., Rupprecht, 1971; Bian et al., 2016), which could potentially enhance the resemblance of phasmids to plants when seen against a backdrop of moving vegetation, as the swaying behavior resembles the movement patterns of the plants (Bian et al., 2016). Since wind can dynamically alter the background of an animal, its presence can be used as a proxy for a dynamic environment. If an animal can perceive changes in air currents and adjust its behavior as a response, it allows for maintaining the benefits of adaptive resemblance without the need to integrate detailed information about concrete background changes.
Animals can possess life-stage specific behaviors and color patterns to avoid detection by predators. Ontogenetic changes in color patterns can reduce detection or increase the warning signal as the animal increases in size (Grant, 2007; Tan et al., 2016), while behavioral responses can vary depending on individual size (Cuadrado et al., 2001). Ontogenetic changes in foraging behavior and habitat used are widespread across animal taxa and have profound impacts on individual survival and ecological interactions (Werner and Gilliam, 1984; Hughes et al., 1992; Lind and Welsh, 1994; Hochuli, 2001; Arthur et al., 2008; Nakazawa, 2015; Ohba and Tatsuta, 2016). However, organisms at the same life stage living in a similar habitat are expected to face similar challenges (Fairclough, 2016; Tan et al., 2017).
A textbook example of adaptive resemblance, phasmids are predominantly nocturnally foraging herbivores that are known for their remarkable resemblance to sticks or leaves. These plant-resembling phenotypes have existed for at least 47 million years (Wedmann et al., 2007), attesting to their evolutionary success. Phasmid coloration varies from cryptic green and brown colors (e.g., Lonchodes brevipes, Figures 1A–C) to more “conspicuous” red, blue, and yellow colors (e.g., Calvisia flavopennis, Figures 1D,F). As hemimetabolous insects, phasmids go through several nymphal stages before emerging as adults, which often differ to varying degrees in their appearance from the adult forms (e.g., L. brevipes and C. flavopennis, Figure 1). With different phenotypes and ecological requirements at different life stages, we can expect the behaviors across these life stages to differ as well. Several predator avoidance behaviors in phasmids have been described, yet their adaptive advantages have not been thoroughly studied. Upon disturbance, some phasmid species may escape by dropping from the substrates to the ground or jump off the substrate (Steiniger, 1933; Robinson, 1968a; Zeng et al., 2020), while other species of phasmids may remain immobile (Strong, 1975). In the case of detection by a predator, some phasmid species show a secondary line of defense behaviors, such as abdomen rearing, stridulation, deimatic displays, or spraying of defensive secretions (Eisner, 1965; Bedford and Chinnick, 1966; Löser and Schulten, 1981; Dräger, 2011; Hennemann et al., 2016).
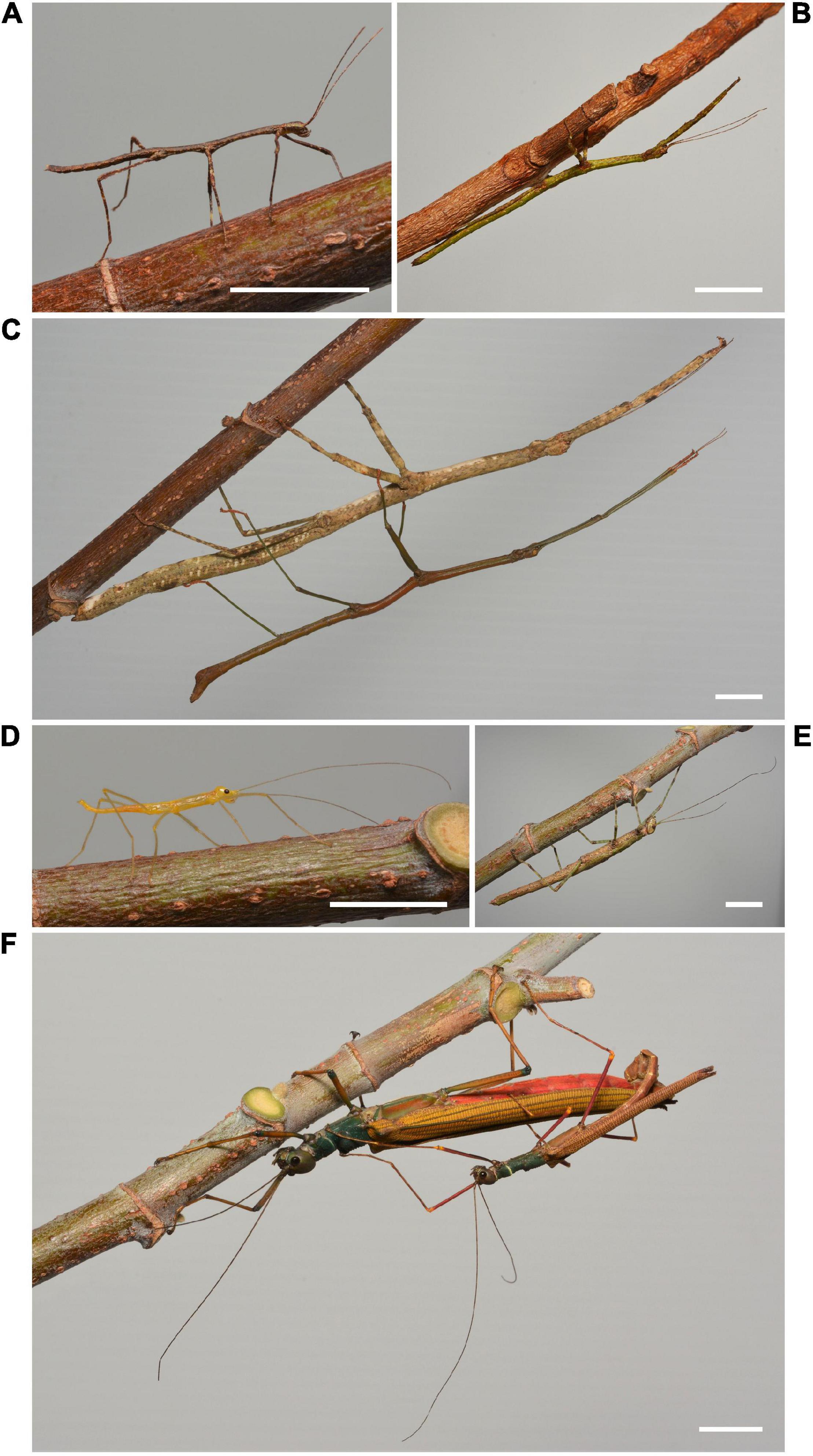
Figure 1. A typical stick-like phasmid, Lonchodes brevipes, and a brightly colored phasmid, Calvisia flavopennis, at different life stages: (A) first-instar nymph of L. brevipes; (B) late-instar nymph of L. brevipes; (C) L. brevipes adults, with the male hanging from the female; (D) first-instar nymph of C. flavopennis; (E) late-instar nymph of C. flavopennis; (F). C. flavopennis adults, with the smaller male copulating with the female. The white bar represents 10 mm in each panel.
To examine the interactions between behavior, life stage, and phenotype, we chose two species of phasmids that live in forest habitats in Southeast Asia. The first species, Gray’s Malayan stick insect (L. brevipes), is a cryptically colored phasmid with a typical stick-like morphology (Seow-Choen, 2017). The second species, C. flavopennis, is a brightly colored phasmid that is fully winged at adulthood and capable of flight (Seow-Choen, 2016). Both species look distinctly different at the various life stages of first-instar nymph (Figures 1A,D), late-instar nymph (Figures 1B,E), and as adult males and females (Figures 1C,F). We examined how the behaviors of these phasmids differ according to their appearance, as determined by species and life stage, using the presence or absence of a wind stimulus as a proxy for a dynamic environment. We predict that phasmids of different appearances exhibit corresponding differences in their behavior and selectively modify their behavioral responses in the presence of a wind stimulus. We envisage that phasmids show specific behaviors that provide them with protection from predators, according to their appearance. Specifically, we expect phasmids with cryptic coloration to adopt behaviors that enhance their adaptive resemblance to inanimate objects and thus reduce their likelihood of detection or recognition, and more conspicuously colored phasmids to adopt behaviors that lower their risk of detection or capture, such as hiding or startle displays.
Materials and Methods
Experimental Subjects
To ensure that adaptations to domestication have not been introduced, none of the experimental subjects were from hobbyist cultures. We used laboratory-reared offspring from field-caught phasmids in this study. We collected L. brevipes on Pulau Ubin, Singapore (1.409°N, 103.955°E), and C. flavopennis near the Kuala Belalong Field Studies Centre, Temburong District, Brunei Darussalam (4.547°N, 115.158°E). We carried out collections between 19:00 h and midnight while walking through the forests. Individuals of L. brevipes were collected from shrubs and trees between 0.5 and 2.5 m above the ground, while C. flavopennis was found above 3.5 m height. Phasmids were housed in a temperature-controlled laboratory at 24°C with a 12 h:12 h light:dark cycle. All individuals were provided food plants ad libitum and monitored to be feeding and behaving normally before the behavioral trials. Individuals with missing or misshapen legs or wings were not used in our experiments, as these may impede their natural responses. No individual was subjected to more than one trial at each life stage. We performed 20 successful trials for each combination of species and life stage—first instar, late instar, adult male, adult female—to a total of 80 trials with L. brevipes and 80 trials with C. flavopennis. “Late instar” denotes nymphs of both species in the later stages of their development, which look dissimilar from both first instar nymphs and adults. In particular, we did not use the final instar of C. flavopennis as they started to develop the adult color patterns. Correspondingly, we did not use the final instar of L. brevipes.
Experimental Setup
Trials were performed in the same laboratory at 24°C during daylight hours under constant artificial light conditions (170–190 lx), in an enclosure (60 cm × 60 cm × 60 cm) with mesh and plastic sides to contain the phasmids during the trials. To simulate a branch for phasmids to rest on, a wooden dowel (300 mm length and 85 mm diameter) was elevated horizontally 145 mm from the bottom of the enclosure (Supplementary Figure 1). We used a portable fan, directed at the phasmid, to simulate a wind stimulus. The fan was moved along a fixed track parallel to the position of the phasmid on the dowel to ensure that the distance of the fan to the phasmid, and hence the wind speed, was constant during and across experiments. Before each trial, the wind velocity was measured to ensure that it was 2.0 ± 0.2 m/s from the track to the dowel. The trials were video-recorded for subsequent behavioral scoring.
Acclimatization
Before each observation, phasmids were introduced to the dowel and allowed to acclimatize for 120 s. Acclimatization was defined as successful when the phasmid adopted a stationary behavior (cf. Table 1) on the dowel for at least the last 30 s of the acclimatization period, and the observation commenced by exposing the phasmid to the wind or control treatment. If the phasmid moved off from the dowel, it was replaced onto the dowel and the acclimatization period would be repeated once, following the procedure above. If the phasmid did not adopt a stationary behavior within 90 s after the start of the acclimatization, the trial was aborted for the day and the phasmid was returned to its housing, until a new trial on a subsequent day.
Treatment
Each trial consisted of two separate observations, one per treatment (control or wind). Each individual was subjected to both the control and wind treatment. The order of the observations was determined by the flip of a coin, with a 180 s time-out between the two observations. During the time-out, the phasmid was placed in an interim cage with fresh leaves.
For the wind treatment, the fan was switched on while it was covered with a cardboard box, to allow the fan to pick up its final wind speed of 2.0 ± 0.2 m/s (see Bian et al., 2016). The box was then removed to expose the phasmid to the wind stimulus, marking the beginning of the observation. After 5 s, the cover was replaced to end exposure of the phasmid to the wind stimulus. For the control treatment, the procedure was identical, except that the fan was not switched on.
Behavioral Scoring
Video recordings of the observations were played back, and all behaviors were scored using the software BORIS (Friard and Gamba, 2016). The scored behaviors and their definitions are listed in Table 1. We referred to the literature for previously described behaviors, and list and describe additional behaviors (please refer to Supplementary Materials for videos and images of additionally described behaviours). An observation started with the beginning of the treatment and ended when the phasmid was stationary for at least 5 s after the end of the treatment. An observation could end prematurely if the insect displayed any of the active escape behaviors (e.g., flying away, Table 1). Behaviors were scored as state events, and the duration of the behavior was recorded. Two stationary behaviors (cf. Table 1) were mutually exclusive, while non-stationary behaviors could co-occur with stationary behaviors as well as with non-stationary behaviors. Active escape behaviors that ended an observation were scored as point events, i.e., they were either observed or not, and no duration was recorded.
Statistical Analyses
Statistical analyses were performed using the R base package (R Core Team, 2019) and PAST 4.03 (Hammer et al., 2001). To adjust for the different total duration of each observation, we calculated, separately for each observation and behavior, the behavioral response as the proportion of time (p) that the insect displayed that behavior during an observation, by dividing the time an individual showed that specific behavior (b) by the total duration of the observation (o): p = b/o. We then performed a permutational multivariate analysis of variance (PERMANOVA) using the function adonis2 from the vegan package (Oksanen et al., 2019) to test the differences in these behavioral responses, with Bray–Curtis distances and 9,999 permutations. The full model included morphological type (specified as the combination of species and life stage), treatment (wind or control), and an interaction term between morphological type and treatment, with the behavioral responses as response variables. We tested for pairwise differences between groups using the function pairwise.adonis from the pairwiseAdonis package (Martinez Arbizu, 2020), with Bray–Curtis distances, 9,999 permutations, and Benjamini–Hochberg adjusted p-values.
Using the metaMDS function from the vegan package (Oksanen et al., 2019), we performed non-metric multidimensional scaling (NMDS) with Bray–Curtis distances, 999 random starts, and autotransform set to FALSE to analyze the differences in behavioral responses between individuals. We used the envfit function from the vegan package with 9,999 permutations to determine the behaviors (i.e., the intrinsic variables) that shape the distribution pattern of the ordination. We visualized the results in NMDS ordination plots and added arrows to visualize the direction of behavioral responses that significantly contributed to the distribution pattern.
Integrating data from all individuals, separately for the control and the wind treatment, we created correlation matrices for the significantly contributing behaviors using the function cor from the R base package with Spearman correlation coefficients and the packages reshape2 (Wickham, 2007) and ggplot2 (Wickham, 2016) to examine if any of these behaviors are correlated in the control or the wind treatment. We tested correlations for significance using the function rcorr from the package Hmisc (Harrell, 2021).
We performed Kruskal–Wallis and Dunn’s post hoc tests using PAST to determine differences in the proportion of total duration of these behaviors between the different groups (characterized by morphological type and treatment), as indicated by Bonferroni corrected p-values. We visualized the proportion of total duration of these behaviors in the different groups with radar plots using the package fmsb (Nakazawa, 2021).
Results
In our experiments, phasmids exhibited a range of different behaviors, in both the control and the wind treatment. We found differences in the behavioral responses between species as well as across life stages of the same species. Morphological type and treatment affected the proportion of time a specific behavior was displayed. Revealing behaviors were positively correlated while concealing behaviors, except for swaying, were mostly negatively correlated.
Comparison of Overall Behavioral Responses Across Groups
Phasmid behavior differs with appearance and environmental condition. We found differences in the overall behavioral responses between individuals depending on morphological type (PERMANOVA, F7,319 = 28.55, p = 0.0001) and treatment (PERMANOVA, F1,319 = 9.44, p = 0.0001), as well as an interaction term between morphological type and treatment (PERMANOVA, F7,312 = 1.77, p = 0.0134). Differences in the overall behavioral responses between groups are visualized in an NMDS plot in Figure 2. Of the 15 recorded state behaviors, eight behaviors contributed significantly to the NMDS data separation: explore, extend, flatten, hang, raise, stand, sway, and walk (all p ≤ 0.041; Table 2). Pairwise comparisons revealed differences in the overall behavioral responses between specific groups (Supplementary Table 1 and Supplementary Figure 2). In the control treatment, all morphological types differed in their overall behavioral responses from each other, except for two sets: (1) adult male C. flavopennis, adult female C. flavopennis, and first instars of C. flavopennis, and (2) adult male L. brevipes and adult female L. brevipes (Table 3A and Figure 3A). In the wind treatment, all morphological types show differences in their overall behavioral responses from each other, except for the following three sets: (1) all C. flavopennis, (2) adult male L. brevipes and adult female L. brevipes, and (3) first instar L. brevipes and late instar L. brevipes (Table 3B and Figure 3B). Comparing the overall behavioral responses of individuals of the same morphological type between the control and wind treatments, we found differences only for first instars of C. flavopennis (F = 3.82, p = 0.0188) and first instars of L. brevipes (F = 6.92, p = 0.0002; Table 3C).
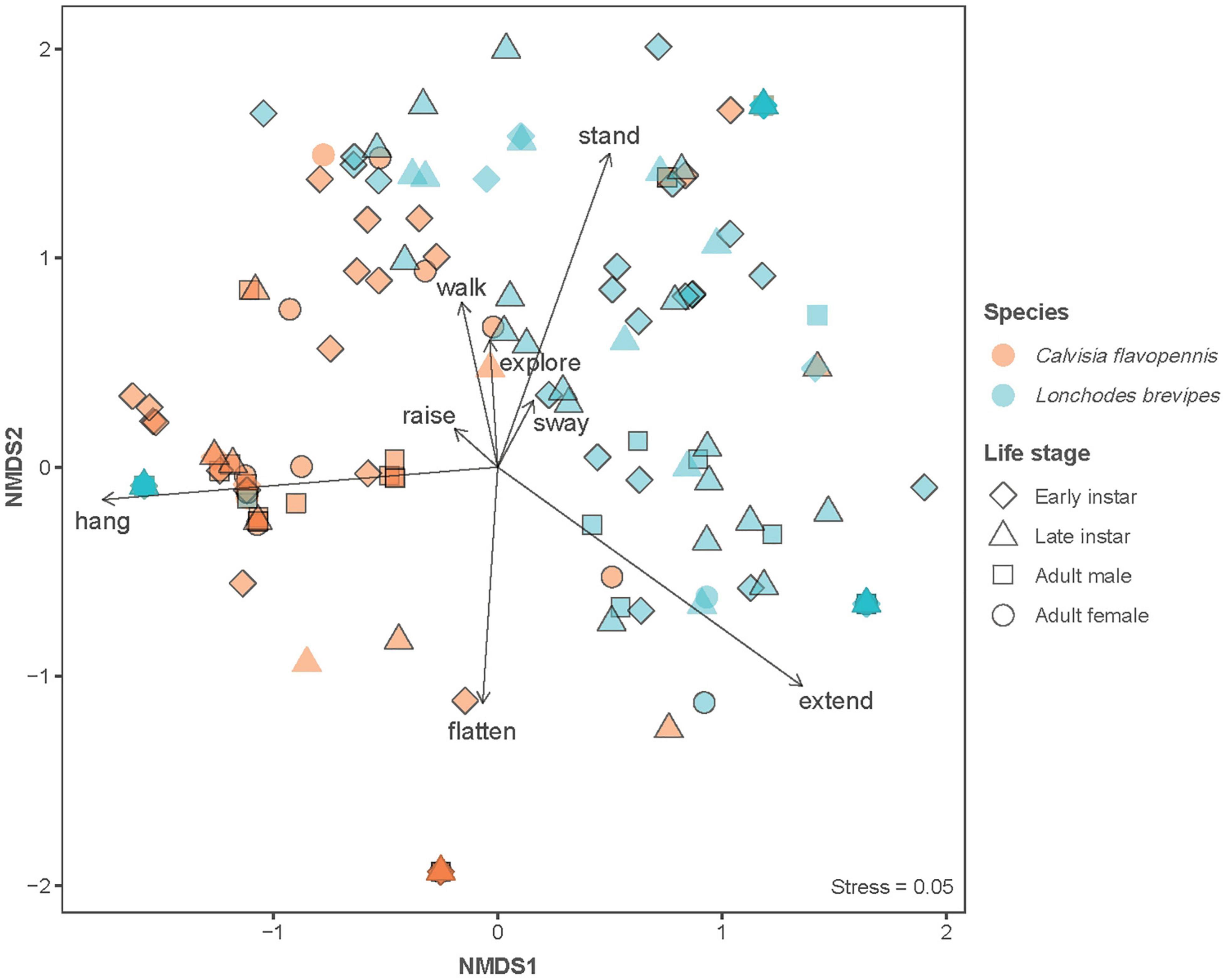
Figure 2. Non-metric multidimensional scaling (NMDS) plot to visualize the differences in behavioral responses. Symbol shape indicates life stage, while symbol color indicates species. Symbols without black outline indicate control observations, while symbols with black outline indicate observations with wind stimulus. Arrows indicate behaviors that significantly contributed to data separation, with arrow length indicating relative contribution. The stress value is reported as an indicator of disagreement in the reduced dimensions.
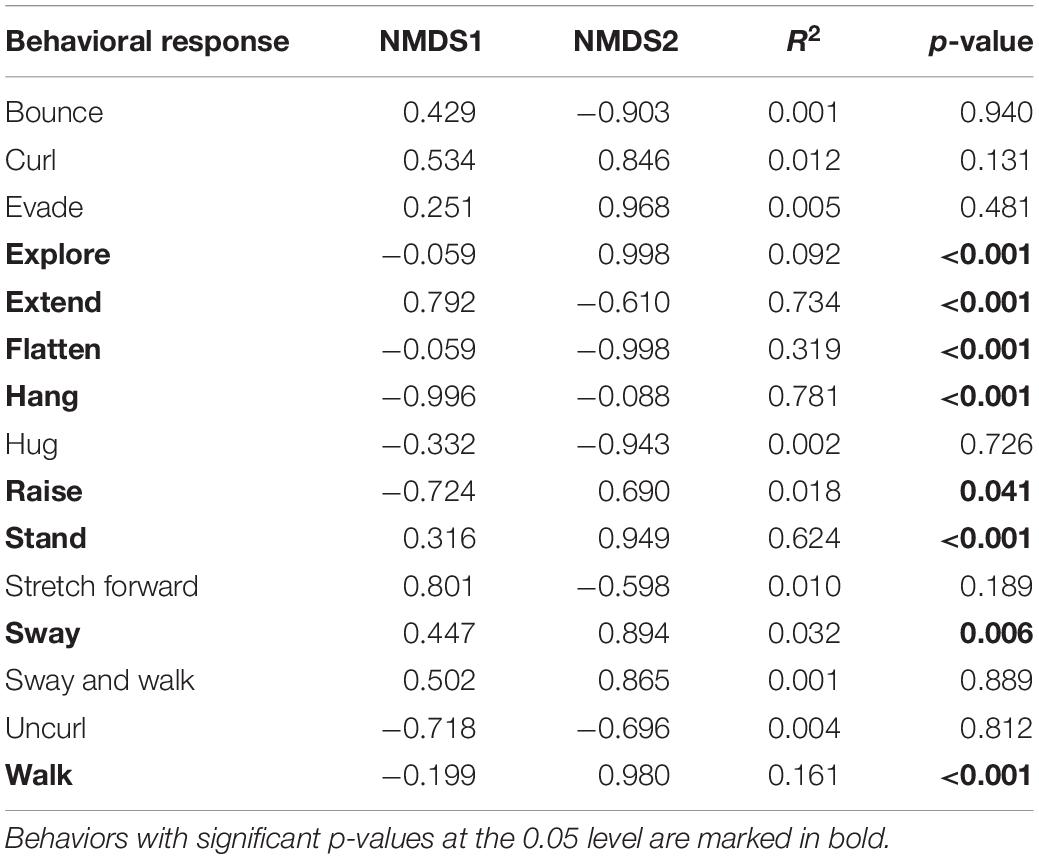
Table 2. The contribution of individual behaviors to the non-metric multidimensional scaling (NMDS) data separation.
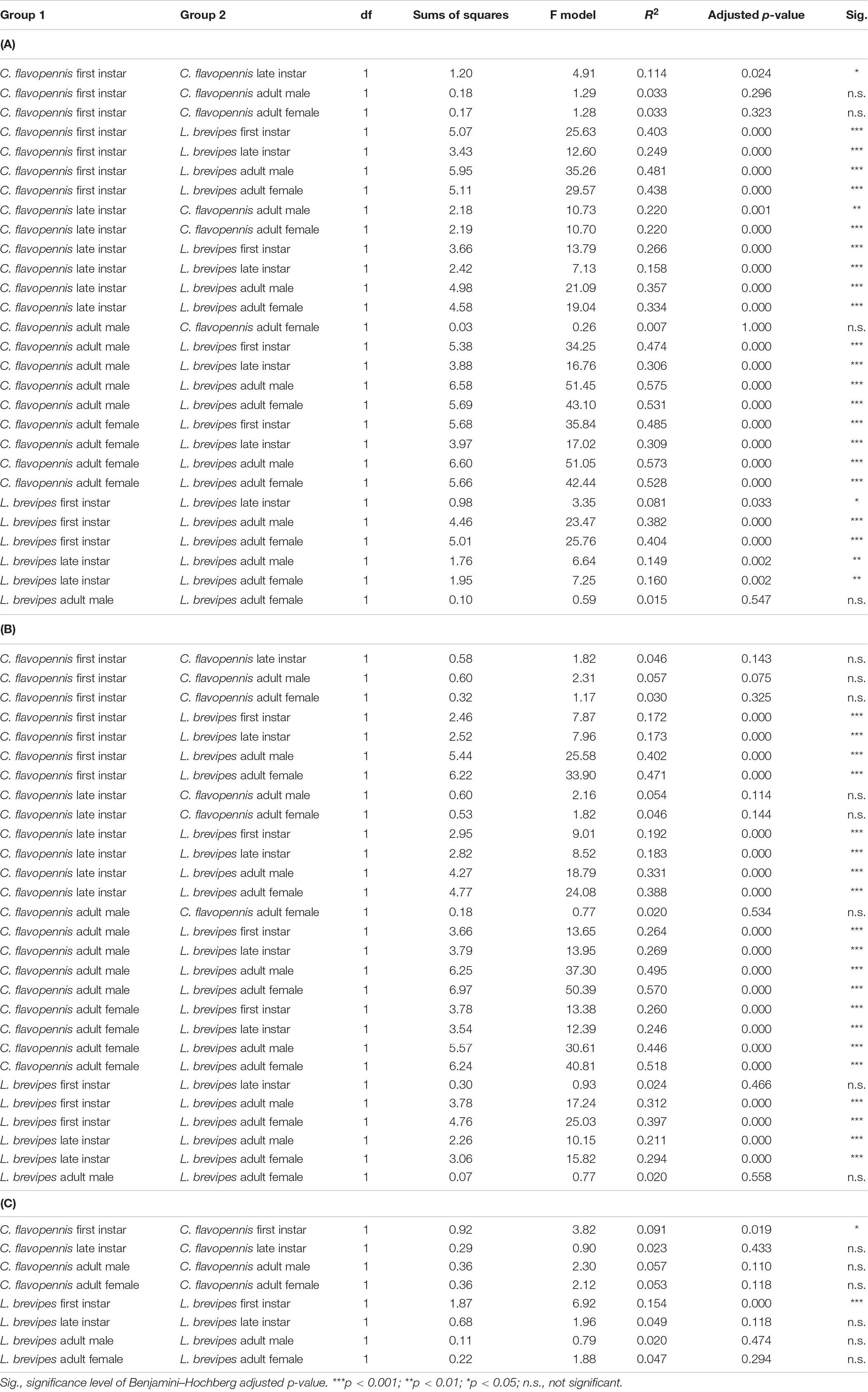
Table 3. Pairwise comparisons of the overall behavioral responses between (A) all morphological types in the control treatment and (B) all morphological types in the wind treatment, and (C) comparisons of the overall behavioral responses between the wind and control treatments for individuals of the same morphological type.
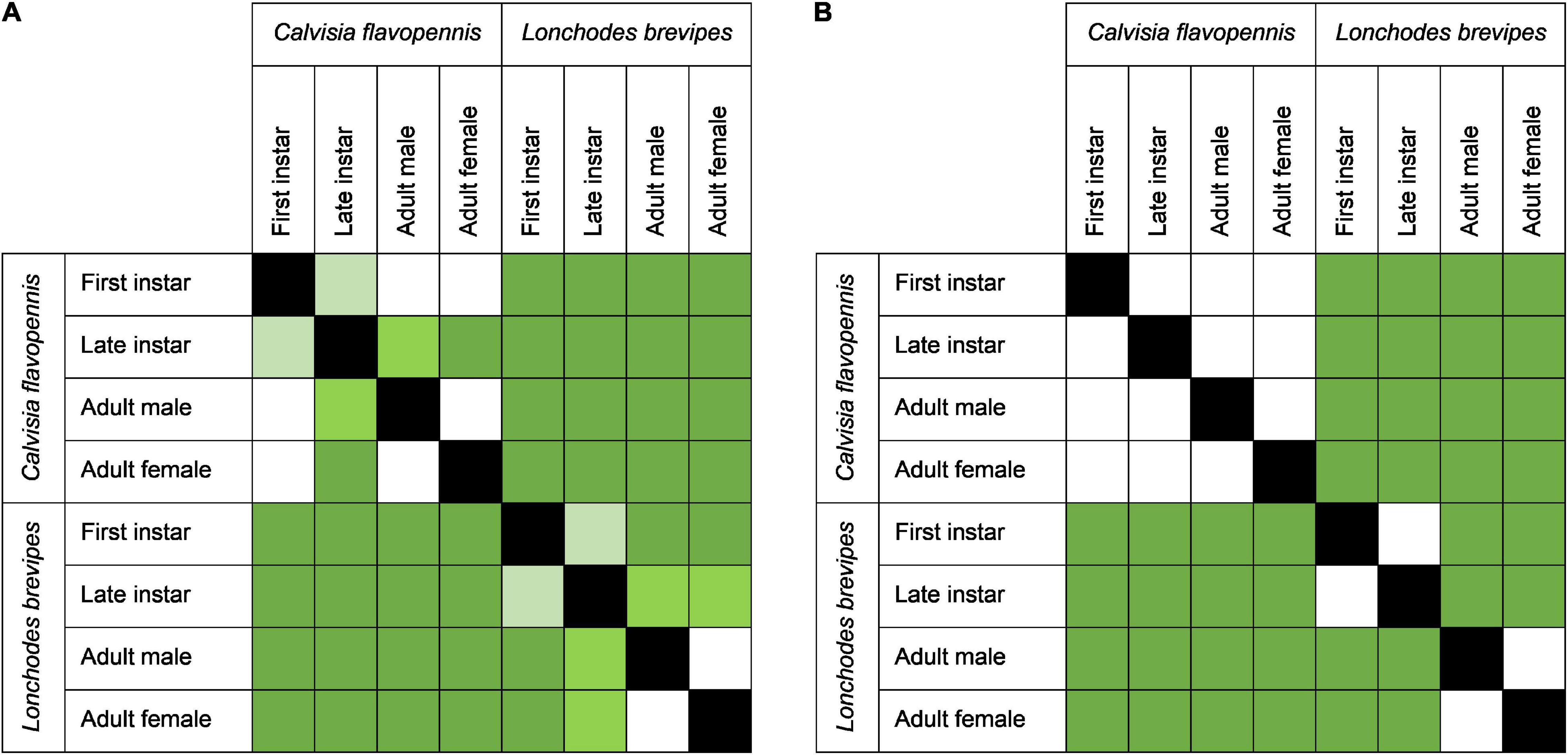
Figure 3. Heat maps of the pairwise comparisons of overall behavioral responses across species and life stages in (A) the control treatment and (B) the wind treatment. The intensity of green in the squares indicates the significance levels of Benjamini–Hochberg adjusted p-values of comparisons between morphological types, dark green, p < 0.001; medium green, p < 0.01; light green, p < 0.05, while white squares represent comparisons that were not statistically significant. Black boxes indicate no comparison (same morphological type).
Comparison of Specific Behaviors Across Groups
For each of the eight behaviors that contributed significantly to the NMDS data separation, we found differences in the proportion of total duration of the behavior between the sixteen combinations of two species, four life stages, and two treatments (Kruskal–Wallis tests, all p < 0.001, Table 4 and Figure 4). We highlight notable patterns of differences between combinations of morphological type and treatment for these behaviors, as indicated by Dunn’s post hoc tests (all p-values are Bonferroni corrected; for full results, please refer to Supplementary Table 2). We group the behaviors according to their potential to conceal or reveal the phasmids.
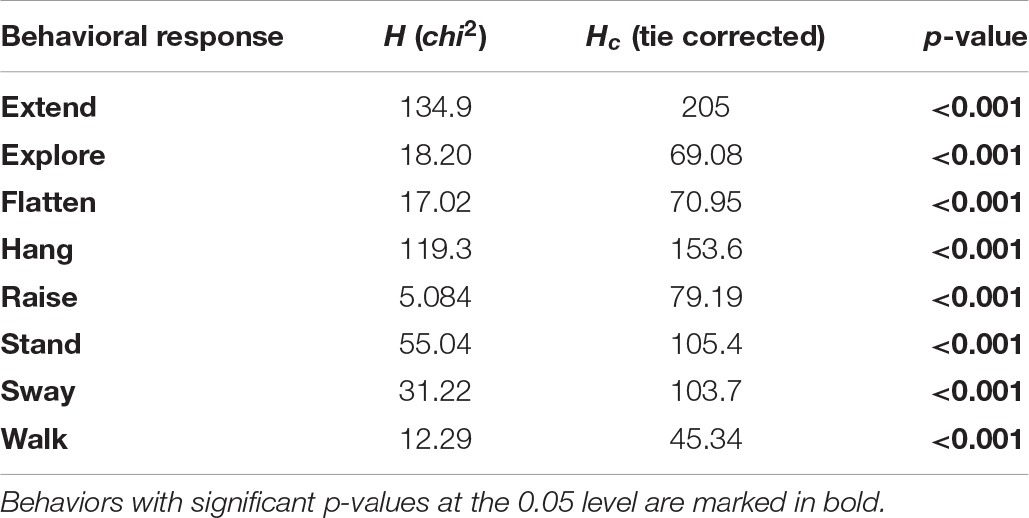
Table 4. Differences in individual behaviors between experimental groups, for behaviors that significantly contributed to the distribution pattern during NMDS.
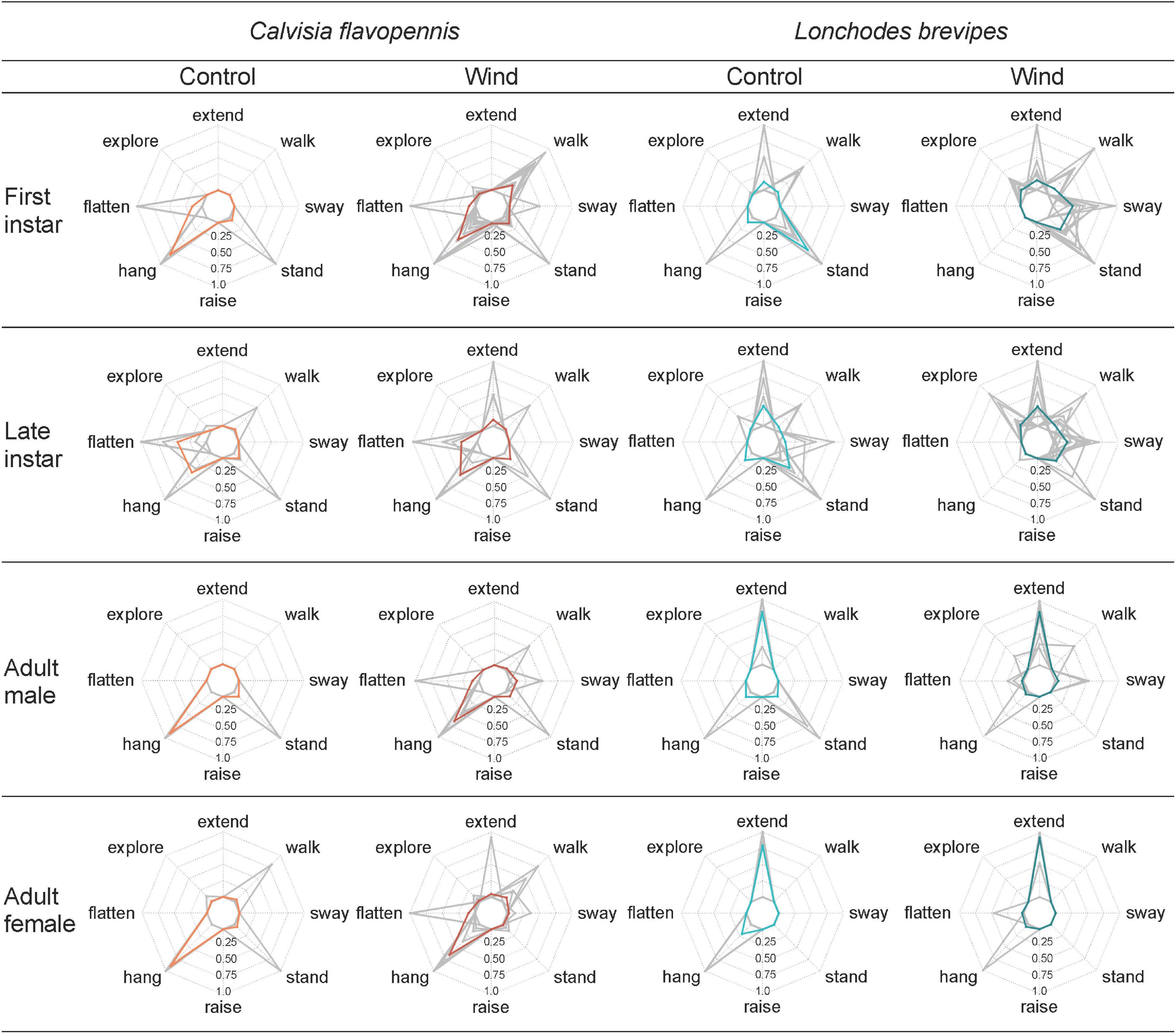
Figure 4. Radar plots display the proportion of time that individuals of different groups (characterized by morphological type and treatment) spent on behaviors that significantly contributed to the distribution pattern during non-metric multidimensional scaling (NMDS). Mean values for each combination of life stage (rows) and treatment (columns) are represented in red for C. flavopennis and in blue for L. brevipes. Gray lines correspond to individual observations.
Concealing Behaviors
Displays of concealing behaviors varied depending on species, life stage, and treatment. Adult L. brevipes spent a higher proportion of time with their anterior limbs extended than any C. flavopennis, regardless of treatment (all p < 0.001). Within L. brevipes, adults in the wind treatment spent a higher proportion of time with their anterior limbs extended than both first and late instars in either treatment (all p < 0.016). Adults in the control treatment spent a higher proportion of time with their anterior limbs extended than first instars in either treatment (all p < 0.002), and adult males in the control treatment spent a higher proportion of time with their anterior limbs extended than late instars in the control treatment (p = 0.024). Late instar C. flavopennis in the control treatment spent a higher proportion of time flattened than all other morphological types in either treatment (all p < 0.001), except for late instar C. flavopennis in the wind treatment (p = 1). First instar C. flavopennis, adult male C. flavopennis, and adult female C. flavopennis in the control treatment spent a higher proportion of time hanging than all life stages of L. brevipes in either treatment (all p < 0.008). In the wind treatment, these three morphological types spent a higher proportion of time hanging than all life stages of L. brevipes in the wind treatment (all p < 0.036). First and late instar L. brevipes in the wind treatment spent a higher proportion of time swaying than all other morphological types in either treatment (all p < 0.017), except for adult female C. flavopennis in the wind treatment (all p > 0.21). We recorded a raised abdomen only occasionally (N = 7 observations) and only in the wind treatment. Except for one first instar L. brevipes individual, only first instar C. flavopennis showed this behavior; the latter spent a higher proportion of time raising their abdomens than all other morphological types in either treatment (all p < 0.001).
Revealing Behaviors
Revealing behaviors were observed across the two species and varied depending on species, life stage, and treatment. Late instar L. brevipes in the wind treatment spent a higher proportion of time exploring than all other morphological types in either treatment (all p < 0.024), except for first instar L. brevipes, first instar C. flavopennis, and adult female C. flavopennis in the wind treatment (all p > 0.57). First instar L. brevipes under wind conditions spent a higher proportion of time exploring than adult male C. flavopennis and adult female L. brevipes in either treatment, than late instar C. flavopennis in the wind treatment, as well as than first instar C. flavopennis and adult male L. brevipes in the control treatment (all p < 0.013). First instar L. brevipes in the control treatment spent a higher proportion of time standing than all other morphological types in either treatment (all p < 0.004), except for first instar L. brevipes in the wind treatment and late instar L. brevipes in either treatment (all p > 0.37). First instar L. brevipes in the wind treatment and late instar L. brevipes in both treatments spent a higher proportion of time standing than adult female L. brevipes in either treatment and adult male L. brevipes in the wind treatment (all p < 0.032). First instar C. flavopennis in the wind treatment spent a higher proportion of time walking than adult female L. brevipes in either treatment, as well as than adult male L. brevipes and first instar and adult male C. flavopennis in the control treatment (all p < 0.021).
Correlation of Behaviors in Control and Wind Treatments
We found correlations between several behaviors in both the control treatment (Table 5A and Figure 5A) and the wind treatment (Table 5B and Figure 5B), integrating data from all individuals in a treatment. Except for swaying, we found positive correlations only between revealing behaviors, while we found negative correlations between concealing behaviors and both other concealing behaviors and revealing behaviors alike. In the control treatment, hang was negatively correlated with explore, extend, flatten, stand, and walk (all p < 0.043). Extend was negatively correlated with flatten and stand (all p < 0.024). Walk was positively correlated with explore and stand (all p < 0.002), and explore was positively correlated with stand (p = 0.019). In the wind treatment, hang was negatively correlated with explore, extend, flatten, stand, and sway (all p < 0.024). Extend was negatively correlated with explore, stand, walk, and sway (all p < 0.024). Flatten was negatively correlated with stand and sway (all p < 0.041). Explore was positively correlated with stand, sway, and walk (all p < 0.001). Stand was positively correlated with sway and walk (all p < 0.011), and raise was positively correlated with hang (p = 0.019).
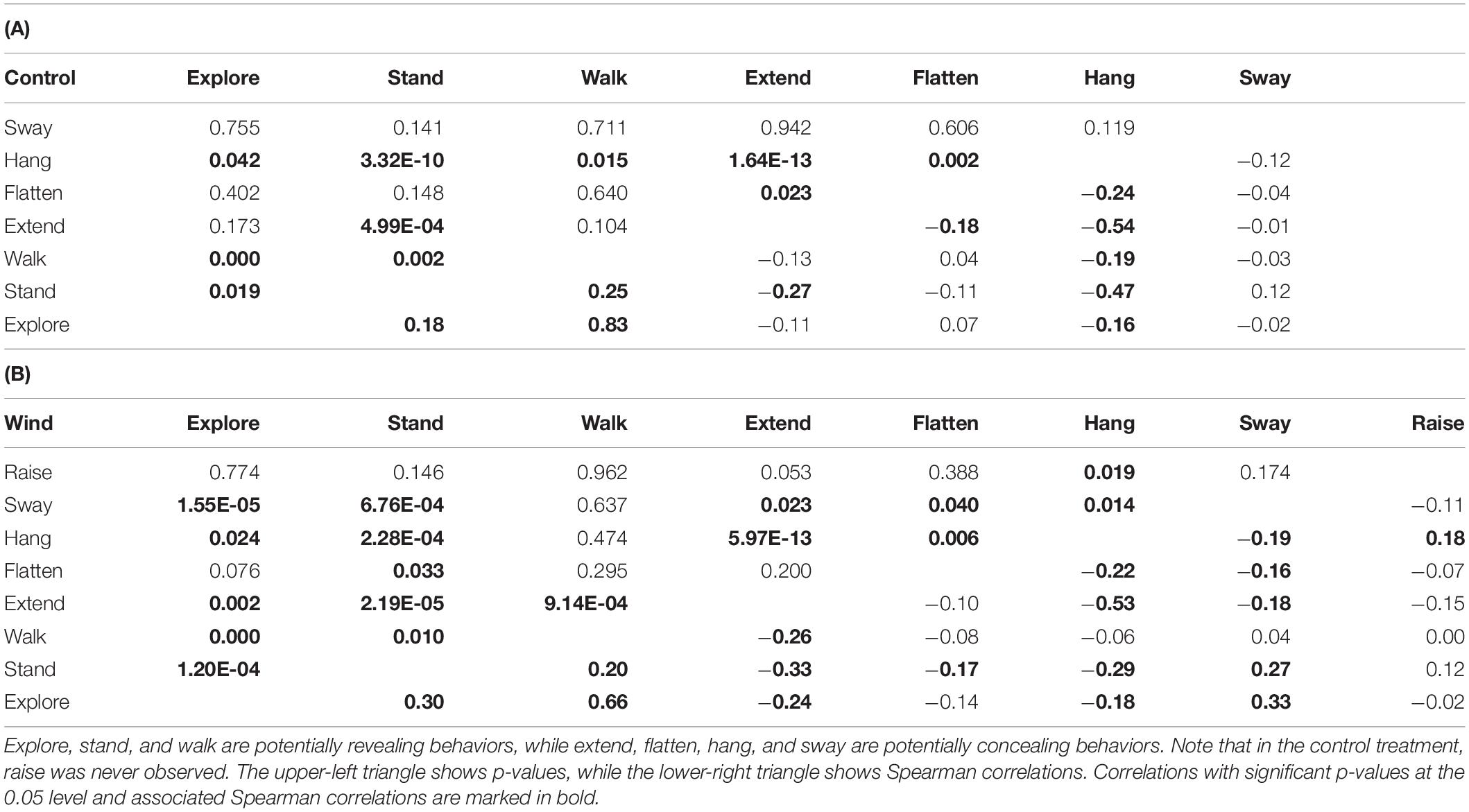
Table 5. Pairwise correlations between the proportions of time spent on behaviors that significantly contributed to the distribution pattern during NMDS, for (A) the control treatment and (B) the wind treatment, integrated for all individuals in a treatment.
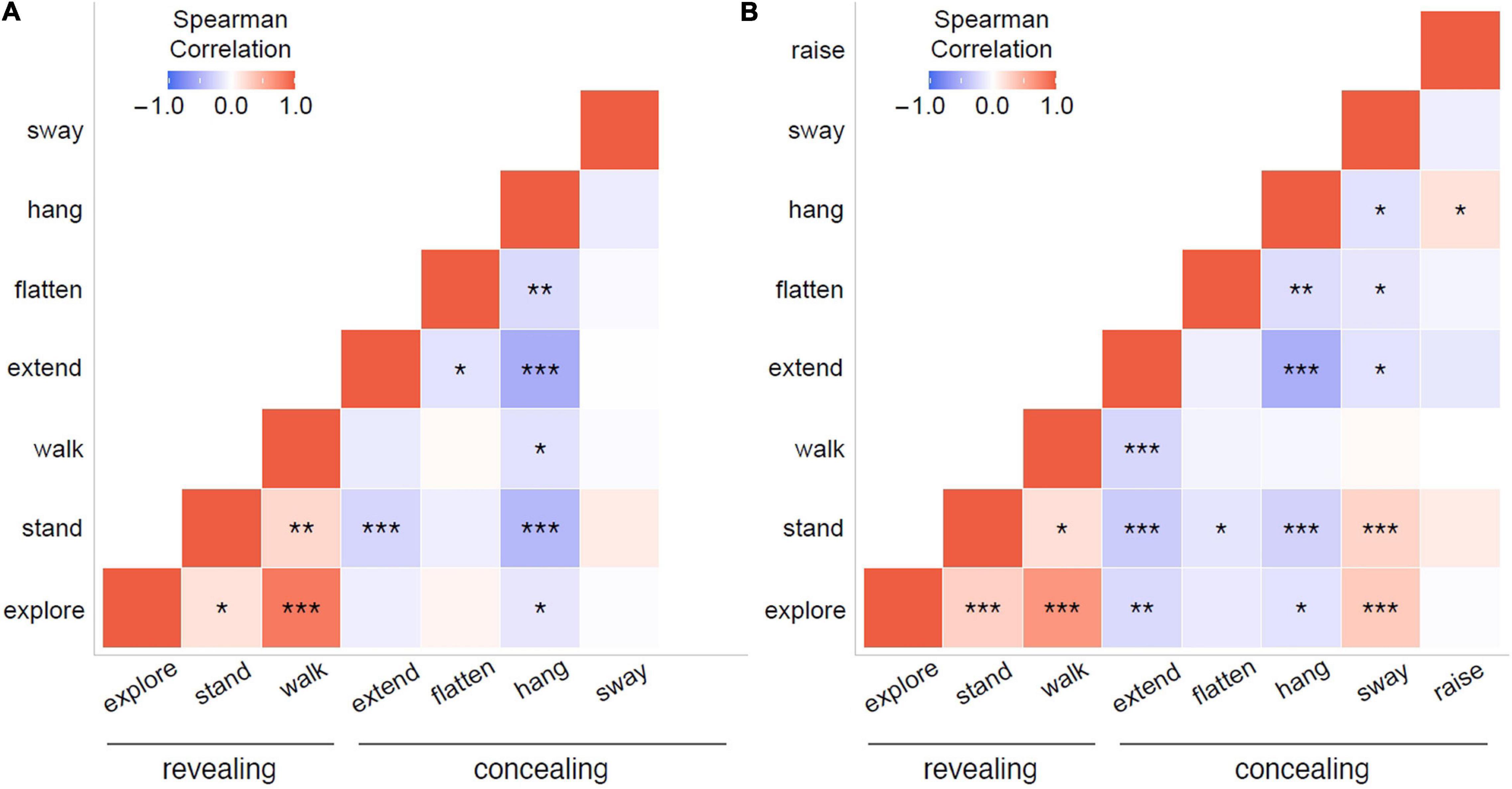
Figure 5. Heat maps with pairwise correlations between the proportions of time spent on behaviors that significantly contributed to the distribution pattern during NMDS, for (A) the control treatment and (B) the wind treatment, integrated for all individuals in a treatment. Explore, stand, and walk are potentially revealing behaviors, while extend, flatten, hang, and sway are potentially concealing behaviors. Note that in the control treatment, raise was never observed. Colors and shading of the squares indicate the direction and strength of Spearman correlations, respectively. Asterisks indicate significance levels: ***p < 0.001; **p < 0.01; *p < 0.05; fields without asterisks are not statistically significant.
Discussion
Our study provides a first insight into the variability of behavioral responses of different phasmid species and life stages in response to a variable environmental factor. The results for both the control and the wind treatment indicate that the behaviors of phasmids varied through ontogeny and between species. The proportion of time spent on specific behaviors differed between groups of different morphological types and treatments. Within the same morphological type, only first instars showed significant changes in their overall behavior as a response to different environmental conditions. Through the interaction of behavior and morphology, phasmids may be able to adjust their adaptive resemblance strategies to specific situations. We discuss the differences in behavioral responses in the context of how the behaviors could reveal the phasmids or conceal them from potential predators. Investigating the behavioral components of predator avoidance strategies is a further step toward a more complete understanding of the complex interactions between morphology, behavior, sensory ecology, and environmental factors. Ultimately, this work will allow us to understand the selective pressures and evolutionary dynamics that shape evolutionary strategies of phasmids.
Overall Behavioral Differences
Adaptive strategies to evade predation can be shaped by extrinsic factors, such as the predator community, and intrinsic factors, such as current life history requirements (Mappes et al., 2014; Valkonen et al., 2014). Individuals at the same life stage leading a similar lifestyle in a similar habitat might be expected to face similar challenges and thus exhibit similar behaviors and color patterns, even across species (Tan et al., 2017). Life stage influences phasmid behavior, particularly in the absence of wind, yet species is a better predictor of phasmid behavior across environmental conditions. The two species in this study differ in their overall behavior, regardless of life stage and treatment. Additionally, ontogenetic changes could be accompanied by changes in behavior. For example, the shield bug Graphosoma lineatum changes from a cryptic appearance pre-hibernation to an aposematic appearance post-hibernation, with aposematic forms showing higher mobility than cryptic forms (Johansen et al., 2011). The overall behavioral responses of C. flavopennis to wind do not differ across life stages, whereas L. brevipes in the wind treatment show differences in the overall behavior between adults and nymphs, but not between adult sexes or nymphs of different ages. Intraspecific behavioral differences depending on the life stage are also observed in other phasmid species, with smaller and younger individuals of Timema cristinae stick insects more likely to exhibit thanatosis (Farkas, 2016). While our study directly compares the behavior of different life stages of two phasmid species, identification of broad evolutionary trends would require comparative analyses to adjust for phylogenetic relationships (Harvey and Pagel, 1991).
Group-Specific Behaviors
By disguising their shape, prey can become unrecognizable to predators (Merilaita et al., 2017). Behaviors such as extend and flatten have the potential to conceal the presence of an individual and hence reduce the likelihood of detection or recognition by potential predators. The extension of anterior limbs aligned with the antennae masks the typical morphology of an insect, as phasmids lose the distinctive shape of six legs and antennae (Robinson, 1968b). Unlike C. flavopennis, L. brevipes individuals have legs that fit closely to the head when extended with the antennae, and all life stages of the cryptically colored species L. brevipes resemble twigs and branches of plants. Extension of anterior limbs was more common in the adults of L. brevipes. Possibly due to their larger size, and thus detectability, it might be more important for larger insects to adopt this behavior, which alters their appearance.
Plant resemblance could also play a role during the flattening behavior. By flattening themselves against the substrate, phasmids could lose the distinct morphology of an insect, and thus reduce detection. Flattening can additionally enhance protection by eliminating shadow casting, as seen in frogs (Ferreira et al., 2019). Combined with cryptic coloration, a phasmid flattened against its substrate would be concealed from predators. In fact, late instar C. flavopennis, which have a mottled brown, bark-like appearance (Figure 1E), behaved distinctly different from the other life stages: in the control treatment, they spent a higher proportion of time flattened than all other morphological types. As the wooden dowel used in the experimental setup resembles a plant branch, flattening against a branch could be a behavior that reduces the detection of the insect. The combination of flattened resting positions with a dorsoventrally flattened morphology has been demonstrated to reduce detection by predators in the spider mite Aponychus corpuzae (Chittenden and Saito, 2006).
Prey can reduce their exposure to predators by selecting specific microhabitats. For instance, prey location and foliage structure influence prey capture rates, with lower leaf surfaces experiencing less predation overall (Whelan, 2001; Johnson et al., 2007). By hanging under the substrate, the phasmid could be concealed from predators above. Contrary to our expectations, hanging was more often observed in brightly colored individuals of C. flavopennis than in L. brevipes. First instar C. flavopennis, adult male C. flavopennis, and adult female C. flavopennis spent a higher proportion of time hanging than all life stages of L. brevipes in the same treatment. Instead of performing evasive behaviors, hanging under a substrate could be an adaptation by the brightly colored life stages of C. flavopennis to reduce the risk of predation. Although the main predators of C. flavopennis are currently unknown, a field study of insectivorous bird species in a similar tropical forest in Malaysia found that these insectivores rarely foraged from the underside of branches (Mansor and Mohd Sah, 2012).
While movement may alert a predator to the presence of prey, it does not necessarily compromise camouflage, as there are several ways in which prey may specifically incorporate motion into their arsenal of defense strategies to compromise the cues used by their natural enemies (reviewed by Stevens and Ruxton, 2019; Caro and Koneru, 2021). In some cases, specific motion can enhance the effectiveness of camouflage (Fleishman, 1985; Huffard et al., 2005; Cuthill et al., 2019). Under dynamic background conditions, such as those caused by wind, swaying could improve concealment by reducing the signal-to-noise ratio (Cuthill et al., 2019). Swaying has previously been reported as a behavior that enhances camouflage in other cryptically colored animals, such as snakes, mantids, and spiders (e.g., Fleishman, 1985; Jackson, 1985; Watanabe and Yano, 2009, 2012, 2013). In the presence of wind, L. brevipes first and late instars generally spent more time swaying than the other morphological types. Swaying could enhance the resemblance of phasmids to an uninteresting object through motion masquerade (Hall et al., 2017; Cuthill, 2019). As prey animals will risk predation to continue with other profitable activities if the risk is low (Ydenberg and Dill, 1986), swaying could reduce the detection risk of phasmids during activities such as foraging, which is crucial for developing nymphs. Swaying in the phasmid Extatosoma tiaratum was quantitatively similar to that of moving plants, consistent with motion masquerade where animals may use motion as a concealing behavior against a moving background (Bian et al., 2016).
Unlike concealing behaviors, behaviors such as standing, exploring, and walking could reveal the identity and/or location of an individual. When insects are standing with their body elevated from the substrate, they exhibit a distinct form, with their body, six legs, and antennae visible. The cryptically colored first instar L. brevipes, particularly in the control treatment, spent more time standing than C. flavopennis and adult L. brevipes. It is possible that the combination of cryptic coloration and smaller size makes them less detectable and, hence, standing disrupts their cryptic appearance less. Explore, where the insect probes a gap with its antennae and first pair of legs (Blaesing and Cruse, 2004), reveals the presence of the insect and is similarly risky. In the field, one of the authors (ET) observed a phasmid being preyed on during exploration. Wind is a cue to inform jumping spiders whether it is appropriate to jump (Aguilar-Arguello et al., 2021) and might similarly serve as a cue for exploration in phasmids. Walking, which is the forward movement along the substrate, is risky as it can break the crypsis of the insect. In the presence of predators, the caridean shrimp Tozeuma carolinense decreases the time spent walking on their seagrass blade substrate and shows an increase in clinging behavior that resembles flattening in phasmids (Main, 1987). In the wind treatment, exploration behavior was increased in both L. brevipes instars, while first instar C. flavopennis spent a higher proportion of time walking than other groups. The risk of detection during these behaviors could be lower for nymphs due to their smaller size.
Interaction of Concealing and Revealing Behaviors
Once phasmids adopt a concealing behavior, a change of behavior could break their concealment. However, if phasmids already adopted a revealing behavior, there could be fewer costs to switching to a different revealing behavior. In fact, they could then benefit from a combination of different behaviors – for example, a combination of exploring and walking could lead them to a better-suited spot for resting or feeding. The phasmid Haplopus scabricollis was observed to intersperse walking with exploration behaviors often during locomotion (Stockard, 1908). We found that sway was the only concealing behavior that correlated positively with any revealing behaviors (stand and explore, in the wind treatment). Swaying at intervals could thus provide phasmids some form of concealment between revealing behaviors through motion masquerade in a dynamic environment.
Conclusion
Future studies to test the predictions about the role of particular behaviors as antipredator strategies will need to determine how likely predators are to detect phasmids. Using prey models that systematically vary in size, coloration, posture, and location (e.g., Cuthill and Székely, 2009; Webster et al., 2009; Tan et al., 2016, 2020), it will be possible to elucidate the importance of these characteristics for concealment and predator avoidance in phasmids. Field observations can provide information about the range of natural predators of phasmids and indicate the preferred foraging microhabitats of the predators. Anecdotal reports describe spiders, mantids, and birds (Paine, 1968; Robinson and Robinson, 1973; Bragg, 1992; Suetsugu et al., 2018) as natural predators of phasmids in the wild. In captivity, phasmids are readily accepted by lizards and amphibians (Bragg, 1992), suggesting that these animals could be potential predators of phasmids in the natural environment. Specific knowledge about phasmid predators will also provide further insight into the role of coloration, as color vision differs between different predator groups (Jacobs, 1993; Briscoe and Chittka, 2001; Hart, 2001; Théry and Gomez, 2010; Fabricant and Herberstein, 2015). Species-specific attributes, such as the presence or absence of wings, and the resulting different niches could provide additional explanations for differences in the behaviors between species. Behavioral experiments would be required to investigate the effect and function of active behaviors. For example, to investigate whether swaying behavior in the species tested in this study is a form of motion masquerade as suggested for E. tiaratum (Bian et al., 2016), it is necessary to examine the effect of swaying on potential predators and to quantify the swaying behavior in response to wind. Future studies, such as a comparative analysis of morphology and behaviors across phasmid species that considers the phylogenetic relationships, would allow us to elucidate the evolution of predator avoidance strategies. By using an integrated approach that combines behavioral experiments with biophysical measurements and field observations, it will be possible to gain further insights into processes of adaptive resemblance.
Data Availability Statement
The original contributions presented in the study are included in the article/Supplementary Material, further inquiries can be directed to the corresponding author.
Ethics Statement
The research reported in this article, which involved insects only, was exempt from ethics approval procedures by the National University of Singapore. We collected L. brevipes in Singapore (permit number NP/RP18-062) and C. flavopennis in Brunei Darussalam (export permit number BioRIC/HoB/TAD/51-131).
Author Contributions
ET and SP conceptualized the project. ET, SP, and YN-R acquired funding. RW coordinated the access to Bruneian phasmids. ET, SP, MS, and KL collected the specimens. KL, HB, MS, and YP performed data collection. ET and SP performed data analyses and wrote the first draft of the manuscript. All authors contributed to manuscript revision, read, and approved the final manuscript.
Funding
This project was funded in part by the grant of a National Geographic Explorer (NGS-KOR-301R-18) to ET, SP, and YN-R; a Yale-NUS College Summer Research Programme grant to HB; and the Ministry of Education, Singapore, and Yale-NUS College Start-up Grant to ET.
Conflict of Interest
The authors declare that the research was conducted in the absence of any commercial or financial relationships that could be construed as a potential conflict of interest.
Publisher’s Note
All claims expressed in this article are solely those of the authors and do not necessarily represent those of their affiliated organizations, or those of the publisher, the editors and the reviewers. Any product that may be evaluated in this article, or claim that may be made by its manufacturer, is not guaranteed or endorsed by the publisher.
Acknowledgments
The authors thank Francis Seow-Choen for his help in identifying the species, Mishak Shunari and John Lee for their help with fieldwork, as well as Salleh Abdullah and the staff at Kuala Belalong Field Studies Center for the field support. The authors are grateful to Bruno Kneubühler for his advice with phasmid husbandry, to Andreia Ko and Anuji Diyana for their help with recording videos of phasmids, and to Mark Elgar for valuable comments on an earlier draft of this manuscript.
Supplementary Material
The Supplementary Material for this article can be found online at: https://www.frontiersin.org/articles/10.3389/fevo.2021.767940/full#supplementary-material
References
Aguilar-Arguello, S., Taylor, A. H., and Nelson, X. J. (2021). Jumping spiders attend to information from multiple modalities when preparing to jump. Anim. Behav. 171, 99–109. doi: 10.1016/j.anbehav.2020.11.013
Arthur, K. E., Boyle, M. C., and Limpus, C. J. (2008). Ontogenetic changes in diet and habitat use in green sea turtle (Chelonia mydas) life history. Mar. Ecol. Prog. Ser. 362, 303–311. doi: 10.3354/meps07440
Bedford, G. O., and Chinnick, L. J. (1966). Conspicuous displays in two species of Australian stick insects. Anim. Behav. 14, 518–521. doi: 10.1016/S0003-3472(66)80053-2
Bian, X., Elgar, M. A., and Peters, R. A. (2016). The swaying behavior of Extatosoma tiaratum: motion camouflage in a stick insect? Behav. Ecol. 27, 83–92. doi: 10.1093/beheco/arv125
Blaesing, B., and Cruse, H. (2004). Stick insect locomotion in a complex environment: climbing over large gaps. J. Exp. Biol. 207, 1273–1286. doi: 10.1242/jeb.00888
Bragg, P. E. (1992). Phasmids and cockroaches as prey of spiders and mantids. Bull. Amat. Entomol. Soc. 51, 19–20.
Briscoe, A. D., and Chittka, L. (2001). The Evolution of color vision in insects. Annu. Rev. Entomol. 46, 471–510. doi: 10.1146/annurev.ento.46.1.471
Caro, T., and Koneru, M. (2021). Towards an ecology of protective coloration. Biol. Rev. 96, 611–614. doi: 10.1111/brv.12670
Chittenden, A. R., and Saito, Y. (2006). Tactile crypsis against non-visual predators in the spider mite, Aponychus corpuzae Rimando (Acari: Tetranychidae). J. Insect. Behav. 19, 419–428. doi: 10.1007/s10905-006-9019-2
Cuadrado, M., Martín, J., and López, P. (2001). Camouflage and escape decisions in the common chameleon Chamaeleo chamaeleon. Biol. J. Linn. Soc. 72, 547–554. doi: 10.1111/j.1095-8312.2001.tb01337.x
Cuthill, I. C., and Székely, A. (2009). Coincident disruptive coloration. Philos. Trans. R. Soc. B Biol. Sci. 364, 489–496. doi: 10.1098/rstb.2008.0266
Cuthill, I. C., Matchette, S. R., and Scott-Samuel, N. E. (2019). Camouflage in a dynamic world. Curr. Opin. Behav. Sci. 30, 109–115. doi: 10.1016/j.cobeha.2019.07.007
Dawkins, R., and Krebs, J. R. (1979). Arms races between and within species. Proc. R. Soc. London. Ser. B. Biol. Sci. 205, 489–511. doi: 10.1098/rspb.1979.0081
Dräger, H. (2011). Die gespenstschrecken der familie heteropterygidae KIRBY, 1896 (Phasmatodea) - ein Überblick über bisher gehaltene Arten. Teil 1: die Unterfamilie Heteropteryginae KIRBY, 1896. ZAG Phoenix 2, 38–61.
Eisner, T. (1965). Defensive spray of a phasmid insect. Science 148, 966–968. doi: 10.1126/science.148.3672.966
Fabricant, S. A., and Herberstein, M. E. (2015). Hidden in plain orange: aposematic coloration is cryptic to a colorblind insect predator. Behav. Ecol. 26, 38–44. doi: 10.1093/beheco/aru157
Fairclough, D. V. (2016). Similar cryptic behaviour during the early juvenile phase of two unrelated reef fishes: Epinephelides armatus and Bodianus frenchii. Mar. Freshw. Behav. Physiol. 49, 109–117. doi: 10.1080/10236244.2015.1125122
Farkas, T. E. (2016). Body size, not maladaptive gene flow, explains death-feigning behaviour in Timema cristinae stick insects. Evol. Ecol. 30, 623–634. doi: 10.1007/s10682-016-9832-9
Ferreira, R. B., Lourenço-de-Moraes, R., Zocca, C., Duca, C., Beard, K. H., and Brodie, E. D. (2019). Antipredator mechanisms of post-metamorphic anurans: a global database and classification system. Behav. Ecol. Sociobiol. 73:69. doi: 10.1007/s00265-019-2680-1
Fleishman, L. J. (1985). Cryptic movement in the vine snake oxybelis aeneus. Copeia 1985, 242–245. doi: 10.2307/1444822
Friard, O., and Gamba, M. (2016). BORIS: a free, versatile open-source event-logging software for video/audio coding and live observations. Methods Ecol. Evol. 7, 1325–1330. doi: 10.1111/2041-210X.12584
Grant, J. B. (2007). Ontogenetic colour change and the evolution of aposematism: a case study in panic moth caterpillars. J. Anim. Ecol. 76, 439–447. doi: 10.2307/4539147
Hall, J. R., Baddeley, R., Scott-Samuel, N. E., Shohet, A. J., and Cuthill, I. C. (2017). Camouflaging moving objects: crypsis and masquerade. Behav. Ecol. 28, 1248–1255. doi: 10.1093/beheco/arx085
Hall, J. R., Cuthill, I. C., Baddeley, R., Shohet, A. J., and Scott-Samuel, N. E. (2013). Camouflage, detection and identification of moving targets. Proc. R. Soc. B Biol. Sci. 280:20130064. doi: 10.1098/rspb.2013.0064
Hammer, Ø, Harper, D. A. T., and Ryan, P. D. (2001). PAST: paleontological Statistics software package for education and data analysis. Palaeontol. Electron. 4:9.
Harrell, F. E. (2021). Hmisc: Harrell Miscellaneous. Available online at: https://cran.r-project.org/package=Hmisc (accessed May 27, 2021).
Hart, N. S. (2001). The visual ecology of avian photoreceptors. Prog. Retin. Eye Res. 20, 675–703. doi: 10.1016/S1350-9462(01)00009-X
Harvey, P. H., and Pagel, M. (1991). The Comparative Method In Evolutionary Biology. Oxford: Oxford University Press.
Hennemann, F. H., Conle, O. V., Brock, P. D., and Seow-Choen, F. (2016). Revision of the Oriental subfamily Heteropteryginae Kirby, 1896, with a re-arrangement of the family Heteropterygidae and the descriptions of five new species of Haaniella Kirby, 1904. (Phasmatodea: Areolatae: Heteropterygidae). Zootaxa 4159, 1–219. doi: 10.11646/zootaxa.4159.1.1
Hochuli, D. F. (2001). Insect herbivory and ontogeny: how do growth and development influence feeding behaviour, morphology and host use? Austral. Ecol. 26, 563–570. doi: 10.1046/j.1442-9993.2001.01135.x
Huffard, C. L., Boneka, F., and Full, R. J. (2005). Underwater bipedal locomotion by octopuses in disguise. Science 307:1927. doi: 10.1126/science.1109616
Hughes, R. N., Burrows, M. T., and Rogers, S. E. B. (1992). Ontogenetic changes in foraging behaviour of the dogwhelk Nucella lapillus (L.). J. Exp. Mar. Bio. Ecol. 155, 199–212. doi: 10.1016/0022-0981(92)90063-G
Ioannou, C. C., and Krause, J. (2009). Interactions between background matching and motion during visual detection can explain why cryptic animals keep still. Biol. Lett. 5, 191–193. doi: 10.1098/rsbl.2008.0758
Jackson, R. R. (1985). A web-building jumping spider. Sci. Am. 253, 102–115. doi: 10.1038/SCIENTIFICAMERICAN0985-102
Jacobs, G. H. (1993). The distribution and nature of colour vision among the mammals. Biol. Rev. 68, 413–471. doi: 10.1111/j.1469-185X.1993.tb00738.x
Johansen, A. I., Tullberg, B. S., and Gambrale-Stille, G. (2011). Motion level in Graphosoma lineatum coincides with ontogenetic change in defensive colouration. Entomol. Exp. Appl. 141, 163–167. doi: 10.1111/j.1570-7458.2011.01182.x
Johnson, M.-L., Armitage, S., Scholz, B. C. G., Merritt, D. J., Cribb, B. W., and Zalucki, M. P. (2007). Predator presence moves Helicoverpa armigera larvae to distraction. J. Insect Behav. 20, 1–18. doi: 10.1007/s10905-006-9048-x
Lind, A. J., and Welsh, H. H. (1994). Ontogenetic changes in foraging behaviour and habitat use by the Oregon garter snake, Thamnophis atratus hydrophilus. Anim. Behav. 48, 1261–1273. doi: 10.1006/anbe.1994.1362
Löser, S., and Schulten, D. (1981). Fortpflanzung und verhalten der malayischen riesengespenstschrecke Heteroptetyx dilatata Park (Phasmatodea. Phylliidae). Löbbecke Museum Aquarium Düsseld 24, 23–27.
Main, K. L. (1987). Predator avoidance in seagrass meadows: prey behavior. Microhabitat selection, and cryptic coloration. Ecology 68, 170–180. doi: 10.2307/1938817
Mansor, M. S., and Mohd Sah, S. A. (2012). Foraging patterns reveal niche separation in tropical insectivorous birds. Acta Ornithol. 47, 27–36. doi: 10.3161/000164512X653890
Mappes, J., Kokko, H., Ojala, K., and Lindström, L. (2014). Seasonal changes in predator community switch the direction of selection for prey defences. Nat. Commun. 5:5016. doi: 10.1038/ncomms6016
Maritz, B. (2012). To run or hide?: escape behaviour in a cryptic African snake. Afr. Zool. 47, 270–274. doi: 10.1080/15627020.2012.11407555
Martinez Arbizu, P. (2020). Pairwiseadonis: Pairwise Multilevel Comparison Using Adonis. R package version 0.4. Available online at: https://github.com/pmartinezarbizu/pairwiseAdonis (accessed December 14, 2020).
Merilaita, S., Scott-Samuel, N. E., and Cuthill, I. C. (2017). How camouflage works. Philos. Trans. R. Soc. B Biol. Sci. 372:20160341. doi: 10.1098/rstb.2016.0341
Nakazawa, M. (2021). Fmsb: Functions For Medical Statistics Book With Some Demographic Data. Available online at: https://cran.r-project.org/package=fmsb (accessed May 27, 2021).
Nakazawa, T. (2015). Ontogenetic niche shifts matter in community ecology: a review and future perspectives. Popul. Ecol. 57, 347–354. doi: 10.1007/s10144-014-0448-z
Ohba, S.-Y., and Tatsuta, H. (2016). Young giant water bug nymphs prefer larger prey: changes in foraging behaviour with nymphal growth in Kirkaldyia deyrolli. Biol. J. Linn. Soc. 117, 601–606. doi: 10.1111/bij.12693
Oksanen, J., Blanchet, F. G., Friendly, M., Kindt, R., Legendre, P., McGlinn, D., et al. (2019). Vegan: Community Ecology Package. Available online at: https://cran.r-project.org/package=vegan (accessed July 15, 2020).
Paine, R. W. (1968). Investigations for the biological control in Fiji of the coconut stick-insect Graeffea crouanii (Le Guillou). Bull. Entomol. Res. 57, 567–604. doi: 10.1017/S0007485300052937
R Core Team (2019). R: A Language And Environment For Statistical Computing. R Foundation for Statistical Computing,. Vienna, Austria. Available online at: URL https://www.R-project.org/ (accessed July 15, 2020).
Regan, D., and Beverley, K. I. (1984). Figure–ground segregation by motion contrast and by luminance contrast. J. Opt. Soc. Am. A 1, 433–442. doi: 10.1364/JOSAA.1.000433
Robinson, M. H. (1968a). The defensive behaviour of the Javanese stick insect, Orxines macklotti De Haan, with a note on the startle display of Metriotes diocles Westw. (Phasmatodea, Phasmidae). Entomol. Mon. Mag. 104, 46–54.
Robinson, M. H. (1968b). The defensive behaviour of Pterinoxylus spinulosus Redtenbacher, a winged stick insect from Panama (Phasmatodea). Psyche 75, 195–207. doi: 10.1155/1968/19150
Robinson, M. H., and Robinson, B. C. (1973). Ecology and behavior of the giant wood spider Nephila maculata (Fabricius) in New Guinea. Smithson. Contrib. Zool. 149, 1–76. doi: 10.5479/SI.00810282.149
Rupprecht, R. (1971). Bewegungsmimikry bei Carausius morosus Br. (Phasmida). Experientia 27, 1437–1438. doi: 10.1007/BF02154275
Ruxton, G. D., Allen, W. L., Sherratt, T. N., and Speed, M. P. (2018). Avoiding Attack: The Evolutionary Ecology Of Crypsis, Warning Signals And Mimicry, 2nd Edn. Oxford: Oxford University Press.
Seow-Choen, F. (2016). A Taxonomic Guide To The Stick Insects Of Borneo. Kota Kinabalu: Natural History Publications (Borneo).
Seow-Choen, F. (2017). A Taxonomic Guide To The Stick Insects Of Singapore. Kota Kinabalu: Natural History Publications (Borneo).
Starrett, A. (1993). Adaptive resemblance: a unifying concept for mimicry and crypsis. Biol. J. Linn. Soc. 48, 299–317. doi: 10.1111/j.1095-8312.1993.tb02093.x
Steiniger, F. (1933). Die erscheinungen der katalepsie bei stabheuschrecken und wasserläufern. Z. Morph. Ökol. Tiere 26, 591–708.
Stevens, M., and Merilaita, S. (2009). Animal camouflage: current issues and new perspectives. Philos. Trans. R. Soc. B Biol. Sci. 364, 423–427. doi: 10.1098/rstb.2008.0217
Stevens, M., and Ruxton, G. D. (2019). The key role of behaviour in animal camouflage. Biol. Rev. 94, 116–134. doi: 10.1111/brv.12438
Stockard, C. R. (1908). Habits, reactions and mating instincts of the “Walking Stick,” Aplopus mayeri. Pap. Tortugas Lab. 2, 43–59.
Strong, L. (1975). Defence glands in the giant spiny phasmid Extatosoma tiaratum. J. Entomol. Ser. A Gen. Entomol. 50, 65–72. doi: 10.1111/j.1365-3032.1975.tb00093.x
Suetsugu, K., Funaki, S., Takahashi, A., Ito, K., and Yokoyama, T. (2018). Potential role of bird predation in the dispersal of otherwise flightless stick insects. Ecology 99, 1504–1506. doi: 10.1002/ecy.2230
Tan, E. J., and Elgar, M. A. (2021). Motion: enhancing signals and concealing cues. Biol. Open 10:bio058762. doi: 10.1242/bio.058762
Tan, E. J., Reid, C. A. M., and Elgar, M. A. (2016). Colour pattern variation affects predation in chrysomeline larvae. Anim. Behav. 118, 3–10. doi: 10.1016/j.anbehav.2016.05.019
Tan, E. J., Reid, C. A. M., and Elgar, M. A. (2017). Predators, parasites and heterospecific aggregations in chrysomeline larvae. Ethology 123, 293–306. doi: 10.1111/eth.12598
Tan, E. J., Wilts, B. D., Tan, B. T. K., and Monteiro, A. (2020). What’s in a band? The function of the color and banding pattern of the Banded Swallowtail. Ecol. Evol. 10, 2021–2029. doi: 10.1002/ece3.6034
Théry, M., and Gomez, D. (2010). ““Insect colours and visual appearance in the eyes of their predators,” in Advances in Insect Physiology: Insect Integument and Colour, eds J. Casas and S. J. Simpson (Cambridge, MA: Academic Press), 267–353. doi: 10.1016/S0065-2806(10)38001-5
Valkonen, J. K., Nokelainen, O., Jokimäki, M., Kuusinen, E., Paloranta, M., Peura, M., et al. (2014). From deception to frankness: benefits of ontogenetic shift in the anti-predator strategy of alder moth Acronicta alni larvae. Curr. Zool. 60, 114–122. doi: 10.1093/czoolo/60.1.114
Watanabe, H., and Yano, E. (2009). Behavioral response of mantid Hierodula patellifera to wind as an antipredator strategy. Ann. Entomol. Soc. Am. 102, 517–522. doi: 10.1603/008.102.0323
Watanabe, H., and Yano, E. (2012). Behavioral response of male mantid Tenodera aridifolia (Mantodea: Mantidae) to windy conditions as a female approach strategy. Entomol. Sci. 15, 384–391. doi: 10.1111/j.1479-8298.2012.00535.x
Watanabe, H., and Yano, E. (2013). Behavioral response of mantid Tenodera aridifolia (Mantodea: Mantidae) to windy conditions as a cryptic approach strategy for approaching prey. Entomol. Sci. 16, 40–46. doi: 10.1111/j.1479-8298.2012.00536.x
Webster, R. J., Callahan, A., Godin, J.-G. J., and Sherratt, T. N. (2009). Behaviourally mediated crypsis in two nocturnal moths with contrasting appearance. Philos. Trans. R. Soc. B Biol. Sci. 364, 503–510. doi: 10.1098/rstb.2008.0215
Wedmann, S., Bradler, S., and Rust, J. (2007). The first fossil leaf insect: 47 million years of specialized cryptic morphology and behavior. Proc. Natl. Acad. Sci. 104, 565–569. doi: 10.1073/pnas.0606937104
Werner, E. E., and Gilliam, J. F. (1984). The ontogenetic niche and species interactions in size-structured populations. Annu. Rev. Ecol. Syst. 15, 393–425. doi: 10.1146/annurev.es.15.110184.002141
Whelan, C. J. (2001). Foliage structure influences foraging of insectivorous forest birds: an experimental study. Ecology 82, 219–231. doi: 10.1890/0012-96582001 082[0219:FSIFOI]2.0.CO;2
Wickham, H. (2007). Reshaping data with the reshape package. J. Stat. Softw. 21, 1–20. doi: 10.18637/jss.v021.i12
Ydenberg, R. C., and Dill, L. M. (1986). “The economics of fleeing from predators,” in Advances in the Study of Behavior, eds J. S. Rosenblatt, C. Beer, M.-C. Busnel, and P. J. B. Slater (Cambridge, MA: Academic Press), 229–249. doi: 10.1016/S0065-3454(08)60192-8
Keywords: adaptive resemblance, Calvisia flavopennis, crypsis, Lonchodes brevipes, motion masquerade, Phasmatodea
Citation: Pohl S, Bungum HZ, Lee KEM, Sani MAB, Poh YH, Wahab RbHA, Norma-Rashid Y and Tan EJ (2022) Age and Appearance Shape Behavioral Responses of Phasmids in a Dynamic Environment. Front. Ecol. Evol. 9:767940. doi: 10.3389/fevo.2021.767940
Received: 31 August 2021; Accepted: 15 December 2021;
Published: 21 January 2022.
Edited by:
Ann Valerie Hedrick, University of California, Davis, United StatesReviewed by:
Richard Anthony Peters, La Trobe University, AustraliaMaría Del Carmen Viera, Universidad de la República, Uruguay
Yu Zeng, Chapman University, United States
Copyright © 2022 Pohl, Bungum, Lee, Sani, Poh, Wahab, Norma-Rashid and Tan. This is an open-access article distributed under the terms of the Creative Commons Attribution License (CC BY). The use, distribution or reproduction in other forums is permitted, provided the original author(s) and the copyright owner(s) are credited and that the original publication in this journal is cited, in accordance with accepted academic practice. No use, distribution or reproduction is permitted which does not comply with these terms.
*Correspondence: Eunice J. Tan, eunice.tan@yale-nus.edu.sg