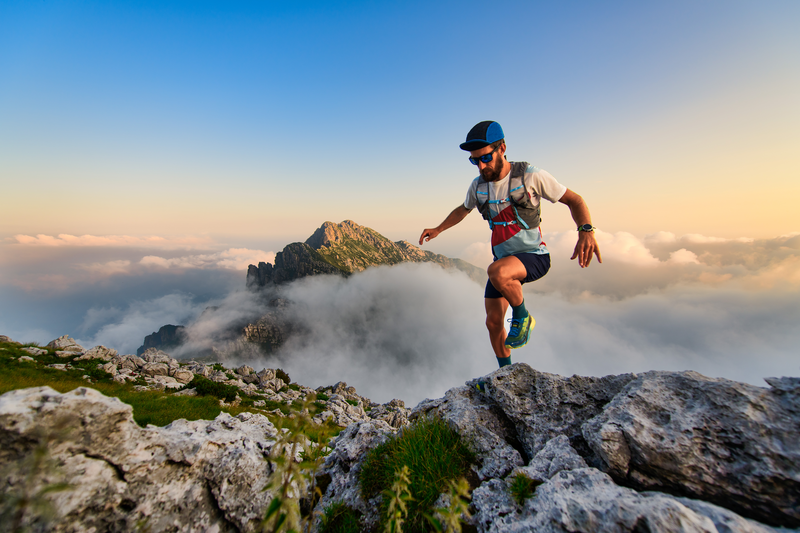
94% of researchers rate our articles as excellent or good
Learn more about the work of our research integrity team to safeguard the quality of each article we publish.
Find out more
ORIGINAL RESEARCH article
Front. Ecol. Evol. , 04 January 2022
Sec. Population, Community, and Ecosystem Dynamics
Volume 9 - 2021 | https://doi.org/10.3389/fevo.2021.761293
This article is part of the Research Topic Animal Seed Dispersal: An Ecosystem Service in Crisis View all 10 articles
The importance of vertebrate animals as seed dispersers (zoochory) has received increasing attention from researchers over the past 20 years, yet one category in particular, diploendozoochory, remains understudied. As the term implies, this is a two-phase seed dispersal system whereby a secondary seed predator (carnivorous vertebrate) consumes a primary seed predator or granivore (rodent and bird) with undamaged seeds in their digestive tract (mouth, cheek pouch, crop, stomach, or other organ), which are subsequently eliminated with feces. Surprisingly, although snakes are among the most abundant predators of granivorous vertebrates, they are the least studied group insofar as our knowledge of seed rescue and secondary dispersal in a diploendozoochorous system. Here, using live snake subjects of the Sonoran Desert (one viperid and two colubrid species) and seeds of the Foothill Palo Verde (Parkinsonia microphylla), a dominant tree of the same region, we experimentally tested germination frequency and rate, and seedling viability. Specifically, to mimic rodents with seed-laden cheek pouches, we tested whether wild-collected P. microphylla seeds placed in the abdomen of thawed laboratory mice and ingested by the snakes would retain their germination viability. Second, we examined whether seeds exposed to gut transit germinated at a greater frequency and rate than the controls. While we found strong statistical support for our first hypothesis, both aspects of the second one were not significant. Accordingly, we provide an explanation for these results based on specific life-history traits (dormant and non-dormant seeds) of P. microphylla. Our study provides support for the role of snakes as important agents of seed rescue and dispersal in nature, their potential as ecosystem engineers, and crucial evidence for the investment of field-based studies on diploendozoochorous systems in deserts and other ecosystems.
The significance of diverse seed dispersal systems to biotic communities, especially concerning the important role of vertebrate animals as dispersers (zoochory), has received increasing attention from researchers over the past several decades (Correa et al., 2007; Traveset et al., 2007, 2008, 2014; Schupp et al., 2010; Hämäläinen et al., 2017; Coughlan et al., 2019; Beckman et al., 2021). In the dispersal system termed endozoochory, seeds are directly consumed (e.g., by bears, primates, rodents, birds, or turtles) and later voided via regurgitation or defecation (Traveset et al., 2001, 2007, 2008; Steyaert et al., 2019). Endozoochory is prevalent in many vertebrate lineages, including freshwater fishes (Galetti, 2007), anuran amphibians (Silva and de Britto-Pereira, 2006; Hocking and Babbitt, 2014), a variety of nonavian reptiles (Blake et al., 2012; Reiserer et al., 2018; Valido and Olesen, 2019; Falcón et al., 2020), birds (Nathan et al., 2008; Heleno et al., 2011; Padilla et al., 2012; Baños-Villalba et al., 2017; Bartel et al., 2018; Blanco et al., 2018; Coughlan et al., 2019; Bravo et al., 2021), and both placental and marsupial mammals (Traveset et al., 2014; Jaganathan et al., 2016; Hämäläinen et al., 2017).
Diploendozoochory, possibly first documented by Darwin (1859), differs from endozoochory in that it is a two-phase seed dispersal system with several key players. In this system, a secondary (P2) predator (e.g., carnivorous vertebrate) consumes a primary (P1) seed predator (granivore) such as a rodent or bird with seeds in its digestive tract (e.g., mouth, cheek pouch, crop, and stomach) which are subsequently eliminated in the feces of P2 (Hämäläinen et al., 2017). In diploendozoochorous systems, when a secondary predator (P2) such as a rattlesnake subjugates and consumes a primary seed predator (P1) that has intact seeds in its cheek pouches (e.g., in our system, a heteromyid and geomyid rodent), the seeds are transported to the digestive tract of P2 and ultimately excreted. Consequently, some or all excreted seeds from P2 potentially survive this journey and germinate; thus, they can be viewed as “rescued” from P1 (post-dispersal seed predation, see Gong et al., 2015). Unlike endozoochory, our present knowledge of seed germination and the fate of dispersed seeds by P2 in diploendozoochorous systems is limited (Vander Wall and Longland, 2004; Hämäläinen et al., 2017; van Leeuwen et al., 2017). Nonetheless, with increased knowledge of the trophic behavior and ecology of carnivorous vertebrates that feed on granivores, the role of seed rescue and secondary dispersal appears to be non-trivial (Vander Wall and Longland, 2004; Hämäläinen et al., 2017; van Leeuwen et al., 2017; Pérez-Méndez and Rodríguez, 2018; Reiserer et al., 2018). Importantly, diploendozoochory can influence plant fitness in a number of ways including (a) seed transport, (b) altering the viability of transported seeds, and (c) changing the quantity of seeds that are dispersed (Hämäläinen et al., 2017; Saldaña-Vázquez et al., 2019; Rubalcava-Castillo et al., 2020). Accordingly, numerous avenues of inquiry remain to be investigated in diploendozoochorous systems which include diversity of species as agents of seed rescue and dispersal (Hämäläinen et al., 2017; Reiserer et al., 2018).
Among the terrestrial vertebrates, snakes are the least studied group with respect to seed rescue and secondary dispersal (Engel, 1997; Reiserer et al., 2018). This deficiency is somewhat perplexing and lacks a clear explanation given that they can be among the most abundant predators (high population densities and biomass per hectare) of seed-eating mammals (e.g., rodents) and birds in temperate and tropical regions (Klauber, 1972; Greene, 1997; Bonnet et al., 2002; King et al., 2018; Reiserer et al., 2018; Martins and Lillywhite, 2019; Henderson et al., 2021). And recent work on rattlesnakes indicates their potential importance as agents in diploendozoochorous systems (Reiserer et al., 2018). Specifically, in a museum study of 50 preserved rattlesnake specimens, nearly 1000 seeds were found to be indirectly ingested by way of consuming rodents possessing seed pouches, particularly heteromyids and geomyids. Careful examinations of entire digestive tracts revealed that not only were rodent-derived seeds abundant, but that numerous seeds germinated in the snakes’ colons (Reiserer et al., 2018).
In North America, geomyid, heteromyid and some sciurid rodents have specialized cheek pouches for transporting seeds from plant source to cache sites, where they are often eliminated from the pool of plant propagules by consumption (Price et al., 2000; Kaufman et al., 2004; Hope and Parmenter, 2007). However, in some cases, seeds stored in these caches will germinate after a rainy season. Distances for seed dispersal in scatter-hoarding mammals (Morris, 1962) vary greatly and depend on species, size of seeds, nutrition value, season, and a host of other factors. For example, kangaroo rats, species with cheek pouches (heteromyids), typically predate, and cache seeds close to their home burrow systems (Jones, 1989; Daly et al., 1992; see Price et al., 2000; Lichti et al., 2017; Wang and Cortlett, 2017). Lifetime dispersal distances in Merriam’s kangaroo rat (Dipodomys merriami), a common rodent in the Sonoran Desert, range from 0 to 265 m in males, and from 0 to 158 m in females (Jones, 1989).
Seed-laden rodents are commonly consumed by snakes as they forage, but unlike raptors, coyotes, bobcats, and other endothermic predators which eat rodents and are known (or implicated) to be secondary seed dispersers (Sarasola et al., 2016), the role of snakes in seed dispersal in nature remains unexplored (Reiserer et al., 2018). Nevertheless, desert-dwelling rattlesnakes and other vipers can be abundant (e.g., western diamond-backed rattlesnake, Crotalus atrox, more than 50 adults per km2), and individuals are capable of consuming 12–20 rodent meals and potentially hundreds of seeds (Vander Wall et al., 1998) during an active season lasting 25–30 weeks. Moreover, individuals occupy large home ranges in which they sometimes travel more than 2 km within several days (Beaupre, 2016; Schuett et al., 2016; DeSantis et al., 2020). Consequently, vast numbers of seeds may potentially achieve exceptionally long secondary dispersal distances, perhaps several times greater than those distributed by the rodents themselves (Nathan et al., 2008; Wang and Cortlett, 2017). Despite the importance of the findings by Reiserer and colleagues (Reiserer et al., 2018) and the potential of snakes as seed dispersers outlined above, the most crucial ecological and evolutionary corollaries of this system hinge on the survival of voided (excreted) seeds and viability of seedlings (Hämäläinen et al., 2017).
Here, using live snake subjects, we experimentally tested germination properties in seeds of the Foothill Palo Verde (Parkinsonia microphylla), an ecologically important and dominant tree of the Sonoran Desert (Bowers, 1994, 2004). We first tested whether wild-collected seeds of P. microphylla placed in the abdomen of thawed laboratory mice and ingested by viperid and colubrid snakes would germinate and produce viable seedlings. Specifically, in our primary study, we tested the Western-Diamond-backed Rattlesnake, Crotalus atrox (Figure 1), a pitviper (viperid) species, based on results of a previous study (Reiserer et al., 2018). In a secondary study, we tested two species of sympatric colubrid snakes in which seed rescue and consumption are unknown but predicted to be present based on their diets, the Desert Kingsnake, Lampropeltis splendida, and the Sonoran Gopher Snake, Pituophis catenifer affinis (Figure 2). Second, in the primary study only, we tested whether the seeds exposed to gut transit germinated at a greater rate and frequency than those of the controls, viz. the accelerated germination hypothesis (Castilla, 2000; Traveset et al., 2001; Hämäläinen et al., 2017; Hanish et al., 2020; Salazar-Rivera et al., 2020; Yang et al., 2021).
Figure 1. Western Diamond-backed Rattlesnake (Crotalus atrox). New Mexico SR 9, near Animas, Hidalgo County, New Mexico. Photograph courtesy of William Wells.
Figure 2. (A) Sonoran Gophersnake (Pituophis catenifer). Vulture Mine Road, Maricopa County, Arizona. (B) Desert Kingsnake (Lampropeltis splendida). Animas Road, Hidalgo County, New Mexico. Photographs courtesy of William Wells.
The Foothill Palo Verde (Parkinsonia microphylla), the State Tree of Arizona1, was selected for this study for several reasons. First, detailed information on its biology and life-history is widely available, including details on germination under natural and artificial conditions (Benson and Darrow, 1981; McAuliffe, 1986, 1990; Bowers, 1994, 2004; Bowers and Turner, 2002; Medeiros and Drezner, 2012). Second, the seeds are reasonably large (several mm across) and easily manipulated for the experiments we executed. Third, wild heteromyid and geomyid rodents (seed predators) are known to harvest the seeds of P. microphylla, temporarily storing them undamaged in their external cheek pouches and subsequently cache them below the soil surface, such as in burrows (McAuliffe, 1990; G. Schuett, pers. observ.). Furthermore, both of these rodent groups are common prey species of snakes from the Sonoran Desert, including the rattlesnake species (Crotalus atrox) and colubrid species (Lampropeltis splendida and Pituophis catenifer affinis) used in this study (Schuett et al., 2016; Reiserer et al., 2018; see Supplementary Figure 1). Last, Foothill Palo Verde is one of the most widely distributed and abundant trees of the Sonoran Desert (Arizona and northern Mexico) and its seeds are easily harvested (Bowers, 2004).
Though there are many approaches to reveal the impacts of digestion on seed germination and seedling viability (e.g., Guillen et al., 2009; Benítez-Malvido et al., 2014; Gonzalez-Di Pierro et al., 2021), few studies have assessed these metrics in diploendozoochorous systems, and none have examined them in snake species. We, therefore, developed a novel approach in which seeds of P. microphylla for this study were collected locally (Phoenix, Maricopa County, Arizona) from mature pods of a single tree in late June 2019. In most seeds, both germination frequency and rate are dependent on numerous factors (Mitchell et al., 2017), including genetic diversity within (Hantsch et al., 2013) and among populations (Ginwal and Gera, 2000; Donohue et al., 2005). Accordingly, to explicitly decouple these aspects of germination from potential genetic effects, we chose to select seeds from a single source tree. The study was divided into two treatments conducted at two different locations. The primary treatment group was conducted at the Chiricahua Desert Museum (Rodeo, Hidalgo County, New Mexico) and involved using the Western Diamond-backed Rattlesnake, Crotalus atrox, Trials involving the secondary group were conducted at the Desert Botanical Garden. The Phoenix Zoo and involved using the Desert Kingsnake (Lampropeltis splendida) and Sonoran Gopher Snake (Pituophis catenifer affinis).
The primary study (Chiricahua Desert Museum) used two Western Diamond-backed Rattlesnake, Crotalus atrox (Figure 1), collected as juveniles in Cochise County, Arizona as neonates (summer 2017), and reared individually to adulthood (1 m in total length at time of testing, 2019–2020). We followed standard institutional protocols for housing and husbandry for these two venomous snake species (e.g., Warwick et al., 1995; Smith, 2005). Five trials per snake (10 trials in total for both snakes) were carried out on the same day from December 2019 to April 2020. In each trial the two snake subjects were fed thawed (frozen) laboratory-reared mice (40 g) obtained from a common source that were impregnated with seeds of P. microphylla. Specifically, seeds for the treatment and control groups were randomly selected from a common source (∼1000 seeds). For each of the treatment trials, a total of 10 seeds (5–7 mm length) were used; five seeds were placed in the abdominal cavity in each of two adult commercial white laboratory mice (40 g each) that had been thawed. Owing to restrictions and difficulties of using wild heteromyid or geomyid rodents to feed snakes at our respective institutions (e.g., potential of disease transmission; availability of appropriately sized rodents), we lacked a rodent model with cheek pouches as vehicle for intact seeds. To mitigate this problem, we used a simple method to act as surrogate to the rodent cheek pouch: a small (1 cm) incision was made at the mid-venter of each thawed lab-reared mouse; five randomly selected seeds were inserted by hand and pushed gently just beneath the skin in the abdominal cavity; the incision was then lightly pinched to prevent seeds from being expelled during ingestion by the snakes. Both snake subjects were then promptly hand-fed two seed-impregnated mice. Each snake was observed during feeding until both mice were consumed. Hence, by coupling intact seeds with the body of the rodent, we experimentally mimicked the way seeds are handled by mice in nature. Since our main goal was to test for germination viability (performance) of snake-ingested foothill palo verde seeds (P. microphylla), the rodent was the natural vehicle for the seeds. Thus, whether test seeds were located in cheek pouches or just beneath the abdominal skin (abdominal cavity) of the ingested rodent, the fate of the seeds remains the same in being readily exposed to the digestive processes of the test snakes. No meal was refused by the snakes during the 5-month period of testing (December 18, 2019 to April 31, 2020).
Typically, the snakes had their first defecation within 5–7 days after ingesting the two mice implanted with seeds; a second defecation occurred up to 14 days after the treatment meal. Snakes were checked 3–4 times daily to obtain seeds as quickly as possible after defecation. Wastes were carefully removed from the cages and placed into a fine metal wire strainer. Tap water was gently run to help separate the seeds from the wastes (feces and urates). All harvested seeds were placed onto damp paper toweling before being incubated for germination tests. Five control trials, each with a total of 10 seeds (total of 50 seeds), were set up the same day (within 60 min) as the snakes were fed their treatment meal. None of the control seeds were scarified or altered in any way.
The P. microphylla seeds harvested from the feces of C. atrox were placed on damp paper toweling inside a plastic germination box. Each box was 28 L × 18 W × 13 H cm and had three small (7 mm) holes drilled on each side for air circulation. Furthermore, the seeds were covered with a single layer of wet (damp) white paper toweling (no dyes). The paper toweling and seeds were never allowed to become dry. The germination boxes were placed on a commercial rack equipped with 5 cm wide heat tape at one end. Each rack could hold 10 boxes. One end of each germination box was exposed to heat tape (28–30°C), which was set by an electric timer for 12D:12N. The seeds were never exposed directly to the heat tape. Air temperature inside the box during the day (12 h) was 26–30°C. During night, when the heat tape was off, air and substrate temperature was 21–24°C. Seeds used for the control trials were set up in different boxes, but were identical in all other respects to the treatment group.
The treatment and control groups of the primary study were checked 3–4 times daily for evidence of germination. Once seeds became imbibed (e.g., larger and paler in color than their original state), germination was imminent (hours). A seed was scored as germinated when a radicle was visible. Although the overall study was conducted from December 18, 2019 to August 31, 2020, a total of 257 days, each trial was allowed to run for approximately 4 months (128 days) for balance. Owing to diversified germination behavior (dormant and non-dormant seeds) in this species (Bowers and Turner, 2002; Bowers, 2004), this amount of time allotted for germination was deemed to be sufficient. For the treatment group, germination (days) was calculated as the period from the day of feeding until the appearance of the radicle.
To determine whether the germinated seeds of P. microphylla in the primary study would show seedling viability, multiple germinated seeds from each of the five treatment (snake-1: n = 19; snake-2: n = 12) and control trials (n = 14) were sown in small pots with commercial soil and allowed to grow to approximately 13–15 cm in height. The remaining germinated seeds were preserved in 95% EtOH. Germinated seeds in the secondary study were not tested for seedling viability.
To test hypothesis 1, we used a binomial linear regression with germination (0 = no germination and 1 = germination) as the response variable, and treatment (snake digested and control), trial (first or second), snake (1 or 2), and defecation as explanatory variables. To test hypothesis 2, we used the non-parametric Mann-Whitney U test, as data were non-normally distributed, with time to germination (in days) as the response variable and treatment (as above) as the explanatory variable. The first analysis was conducted in R (R Core Team, 2021) in R Studio (R Studio Team, 2019), and the second using the open-source project JASP (Love et al., 2019)2.
Our secondary study using P. microphylla seeds was executed using two species of nonvenomous colubrid (colubrine) snakes, the Sonoran Gophersnake, Pituophis catenifer, and the Desert Kingsnake, Lampropeltis splendida (Figure 2). Both species are inhabitants of the Sonoran Desert and sympatric with the Western Diamond-backed Rattlesnake, Crotalus atrox (Figure 1). To our knowledge, there are no publications on seed rescue and germination in colubrid snakes. Importantly, as adults, both colubrid species consume similar mammalian prey (e.g., heteromyid and geomyid rodents) as the Western Diamond-backed Rattlesnake, C. atrox (Holycross and Mitchell, 2021).
Trials consisted of using two species of the two colubrid snakes (as above) and the same set of seeds collected for the primary study. Snakes were maintained at The Phoenix Zoo (PZ) as part of their public outreach collections. Owing to close proximity (1.6 km) seed germination was overseen at the Desert Botanical Garden (DBG). Three trials were run: the first was from August 23 to September 03, 2019 (11 days); the second was from October 1–28, 2019 (27 days); and the third was from March 1 to June 4, 2020 (95 days) Feeding of snakes at PZ was conducted as in the primary study, but only five seeds per feeding were used instead of 10. Snakes were checked once daily for voided excreta (feces and urates) and seeds. Excreta were placed in small plastic tubes (Supplementary Figure 2) and transferred to the DBG (1.6 km) for germination trials. In cases where the germination tests could not be started immediately, the samples were refrigerated at 1°C until use with 24–48 h. In trials 1 and 2, the seeds were not removed from the excreta before initiating germination tests. This was done to determine if any compounds in the excreta itself inhibit or promote germination and subsequent growth. The seeds used in the third trial were gently removed from excreta and briefly washed with tap water, similar to the trials in the primary study.
All seeds (in excreta or washed) were placed on Whatman® qualitative filter paper, Grade 1, 90 mm (Sigma-Aldrich, Inc., St. Louis, MO, United States) inside a plastic petri dish and moistened with distilled water. The petri dishes were then placed into a Percival 36-L germination chamber, model GR36L (Percival Scientific, Inc., Perry, IA, United States) with a schedule of 12 h day/12 h night, 25°C day /15°C night, and 75 micromole light intensity.
In the primary study, each trial in the treatment and control groups was 128 days. Excreted seeds of P. microphylla successfully germinated (Figure 3). Specifically, in the treatment group (five trials for each of the two rattlesnake subjects), 94 of 100 seeds (94%) were recovered from the feces; 78 (83%) of those successfully germinated (Table 1). Mean gut-passage time of seeds was 6 to 14 days (9.1 ± 2.5 days), and in eight instances germinated seeds were recovered in snake feces, implicating germination occurred during gut transit (sensu Reiserer et al., 2018). All seeds in preparation of germination (i.e., appearance of the radicle) became imbibed, swelling to twice their original size (11–13 mm length) and were noticeably paler in coloration (Figure 4). Of the 16 treatment seeds that did not germinate, 2 developed mold, yet the remaining 14 were normal in appearance and thus likely were viable (Bowers, 2004). In the control group (five trials), 36 of 50 seeds (72%) successfully germinated; 2 of the 14 ungerminated seeds developed mold yet the remaining 12 appeared to be viable. No significant differences were found in germination frequency with respect to any of the explanatory variables (i.e., treatment, trial, snake, and/or defecation) (Table 2).
Figure 3. Summary of germination frequency of P. microphylla seeds in the primary study involving the rattlesnakes (Crotalus atrox). Five trials were conducted and each one lasted 128 days. Germination (days) was calculated as the period from the day of feeding until the appearance of the radicle. The histogram was generated using the Histogram function in Excel version 16.48 Data Analysis Tool.
Table 1. Seed counts of P. microphylla in trial conditions of the primary study involving the Western Diamond-backed Rattlesnakes (C. atrox).
Figure 4. Seeds of P. microphylla post-gut transit and recovered from feces of a rattlesnake (C. atrox) in the primary study. The seed on the left has undergone imbibition (imbibed and swelled) and germinated (presence of the radicle) while the other seed shows no outward change in appearance from its original state despite having been exposed to the digestive tract.
Over the full duration of the experiment (128 days), mean germination time (GT) for treatment seeds was 31.9 days (SD: ± 27.2 days; min-max: 6–112 days) and 40.56 days (SD: ± 36.3 days; min-max: 2–123 days) for control seeds. No significant difference in GT was found between the treatment and control groups (Mann-Whitney U test: U = 1465.5, p = 0.626, ns) (Figure 5). All germinated seeds (n = 45) that were planted (treatment: n = 31; control: n = 14) from the primary treatment group survived and were grown to a height of 13–15 cm (Figure 6).
Figure 5. Results of the primary study involving Western Diamond-backed Rattlesnakes (C. atrox). Box plots of mean germination times (GT - days) of P. microphylla seeds for control (c) and treatment (t) groups for 128 days. Horizontal line in boxes = median. Mann-Whitney U test: U = 1465.5, P = 0.626, ns.
Figure 6. Examples of P. microphylla seedlings derived from the treatment group (snake gut transit) in the primary study involving Western Diamond-backed Rattlesnakes (C. atrox).
The three trials yielded 22, 23, and 17 excreted seeds, respectively, from both snake species, and tests for germination ran for 11, 27, and 95 days, respectively. Five untreated seeds were used as controls for each of the three trials. A total of 16 seeds germinated after passing through the digestive tracts of the Sonoran Gophersnake (4 of 17 = 17.4%) and Desert Kingsnake (12 of 29 = 41.4%). Comparing trials 1 and 2, in which the feces containing the seeds were placed in the petri dishes, vs. trial 3, where seeds were removed from the fecal bolus and washed with tap water, revealed a large difference in germination frequency. Germination for the combined trials 1 and 2 were 15.6% (7/45) vs. 53% (9/17). Mold was an issue in many cases in the combined trials (Supplementary Figure 3), but far less so in trial 3 where the seeds were gently washed in tap water. Mold was rarely observed in the primary trials (see main text). The combined results of the three secondary trials revealed that the germination rate of the treatment seeds (16/62 = 25.8%) was not significantly different (7/25 = 28%) (Z-test, two-tailed: Z = −0.210, p > 0.05, ns) from the control seeds.
The most important outcome in all diploendozoochorous systems, from ecological and evolutionary perspectives, is that seeds survive all steps of the process—harvested or swallowed by a seed predator, swallowed whole or stored undamaged (e.g., cheek pouches and crop), swallowed undamaged by a secondary predator, and retention of germination viability after elimination from the gut of the secondary predator (Traveset et al., 2008, 2019; Hämäläinen et al., 2017). In a study involving preserved museum specimens of rattlesnakes (Reiserer et al., 2018), seeds found in the guts of snakes were derived from heteromyid and geomyid rodents, which were likely harvested and stored unharmed in their seed pouches. Uniquely, rattlesnakes and most other snake species subjugate and consume these rodents whole and do not chew (crush); consequently, most seeds transmitted through the GI tracts of snakes from these sources do not appear to be mechanically damaged. Furthermore, owing to long retention times in the digestive tracts of rattlesnakes, some seeds are capable of germination during gut transit (Reiserer et al., 2018; see Cabral et al., 2019; Carbajal-Márquez et al., 2020).
In support of our main hypothesis, we provide the first experimental evidence, to our knowledge, that seeds of the Foothill Palo Verde (P. microphylla) retain germination performance (e.g., viability) when indirectly consumed by snakes—one species of rattlesnake and two species of colubrids— and subsequently recovered from their excreta (Figures 1, 2 and Table 1). Importantly, seeds found to be imbibed and germinated in excreta (feces/urates) produced healthy seedlings (Figure 6). In the primary study, all germinated seeds subsequently tested for seedling viability grew normally and developed into healthy plants.
Under the conditions of the primary study, both aspects of our second hypothesis (accelerated germination) were not statistically supported. The mature seeds of P. microphylla (located in pods) are dry and have physical dormancy (“hardseededness”) resulting from densely packed layers of palisade cells impregnated with water-repellent substances which constrains imbibition of water (Baskin et al., 2000; Baskin and Baskin, 2014), a necessary step for germination. Thus, based on studies of other organisms (reviewed in Hämäläinen et al., 2017), we anticipated that gut-passage in the snakes would aid in chemical and mechanical scarification and accelerate germination frequency and rate. In other studies involving reptiles, the effects of gut passage on germination rate and frequency are mixed; in some cases both germination rate and frequency are accelerated (e.g., Yang et al., 2021), yet in others neither of these effects are observed (e.g., Castilla, 2000). Clearly, for reptiles, studies of diploendozoochory are in their infancy and it would be premature to make hard conclusions at this time based on the little available information (Hämäläinen et al., 2017; Reiserer et al., 2018). Nonetheless, the importance of positive effects of gut passage, such as described in other studies (Hämäläinen et al., 2017), cannot be overlooked and should encourage further research in this understudied area of ecology.
We provide several possible explanations for our results with respect to the second hypothesis tested in the primary study. Foothill Palo Verde (P. microphylla) is a species in which the seed crop is comprised of both non-dormant and dormant seeds and thus diversified germination behavior is exhibited (Bowers and Turner, 2002; Bowers, 2004). Consequently, a variable proportion (e.g., 20 to 34%) of them can germinate shortly after dispersal from their pods and, importantly, without scarification (Bowers, 2004). The dormant seeds can persist into a second season (or even longer) and require exposure to environmental scarification (high heat, rain, wind, and abrasion) for germination to occur (Bowers and Turner, 2002; Bowers, 2004). Second, in germination studies of P. microphylla, we suspect capturing subtle differences in germination rate and frequency will likely require a much larger sample size of seeds to achieve statistical significance for a potentially small effect. Perhaps a 10-fold increase of seeds (e.g., 1000) would be required to overcome the abovementioned problems associated with dormant and non-dormant seed types (see Figure 5). Finally, because wet heat appears to be an important release of dormancy in a closely related species of tree from Mexico (Parkinsonia aculeate), and thus likely others (Van Klinken et al., 2006), subjecting P. microphylla seeds to warmer conditions (e.g., 38–45°C) during germination tests in future studies is warranted.
Similar to the results of the primary study involving Western Diamond-backed Rattlesnakes (C. atrox) mice impregnated with seeds of P. microphylla were consumed by the Sonoran Gophersnake (P. catenifer) and Desert Kingsnake (L. splendida). Some of those seeds that transited the digestive tracts retained viability and germinated (see Supplementary Figure 3). Nonetheless, when compared to the primary study, germination frequency was significantly greater in the primary study (z-test, two-tailed: z = 7.140, p < 0.001). This result was likely due to greater trial duration in the primary study (trials were run for 128 days) and germination conditions were likely superior (e.g., less moisture, warmer, and better air circulation) for P. microphylla. Mold was commonly associated with seeds kept in their feces in trials 1 and 2 of the secondary study. However, we suspect that the highly moist conditions and lack of air flow in petri dishes contributed to this issue. In the Sonoran Desert, similar condition would rarely be encountered. Clearly, in the secondary study, better results were obtained when seeds were removed from the feces and urates. Germination conditions that more closely mimic natural situations (e.g., drier and warmer) are warranted in future studies, which we envision to include performing tests under a range of natural conditions (e.g., outdoor plots).
We provide herein support for the role of viperid and colubrid snakes as important agents of seed rescue and dispersal in nature, highlight their importance as potential ecosystem engineers, and offer sound justification for future field-based studies (Reiserer et al., 2018). Clearly, more research is needed, especially studies that are field-based, to provide context and to better understand the ecological and evolutionary ramifications of this fascinating, yet mostly understudied, diploendozoochorous system (Franklin et al., 2016). Although our current focus has been on organisms of North American deserts, clearly other regions (e.g., tropics) should be explored (Reiserer et al., 2018; Dugger et al., 2019). Furthermore, other plant and snake species would be desirable to study purely for inclusion of greater biodiversity and exploration of potential variation (Hämäläinen et al., 2017).
Topics closely related to seed dispersal and their fates will need to be investigated in field-based studies of diploendozoochory in snakes. For example, despite an abundance of research on defecation sites of lizards, the closest extant relatives of snakes, similar detailed studies are nonexistent, to the best of our knowledge, for wild snakes. Unquestionably, the fate of eliminated seeds in instances of diploendozoochory by snakes and other vertebrates may be further influenced, both positively and negatively, by abiotic (e.g., rain and temperature) and biotic factors (Vander Wall and Longland, 2004). Invertebrates, for instance, such as ants (Pascov et al., 2015; Luna et al., 2018; Anjos et al., 2020) and dung beetles (D’hondt et al., 2007; Midgley et al., 2015), are common vectors in several ecosystems that disperse and alter ground-based seeds both mechanically and chemically (Franklin et al., 2016). Given that only anecdotal data are available, the ecology of defecation sites in snakes and the fate of seeds present in their feces remain open research questions. Indeed, as stated by Anni Hämäläinen and her colleagues (Hämäläinen et al., 2017, p. 13), “It is currently unknown how important the phenomenon (of diploendozoochory) is ecologically, but given its potentially vast prevalence and the possible implications, it is possible that ignoring it could impair the interpretation of broad ecological patterns or hinder conservation efforts.” Importantly, it is incumbent upon us to comment that many of the vipers and other snake species that are candidate ecosystem engineers are themselves endangered for various reasons, including from direct persecution by humans (Maritz et al., 2016; Birskis-Barros et al., 2019; Fathinia et al., 2020). It is hoped that highlighting their potential new role as agents of seed rescue and secondary dispersal for deserts and other ecosystems will encourage both academic and public involvement (e.g., citizen scientists) in generating interest and legislature for their protection and long-term conservation.
The datasets presented in this study can be found in online repositories. The names of the repository/repositories and accession number(s) can be found below: Dryad Digital Repository: https://doi.org/10.5061/dryad.65gr2.
Ethical review and approval was not required for the animal study because our study involved collecting seeds from the fecal wastes of museum and zoo snakes. No animals were harmed in this study. Written informed consent was obtained from the owners for the participation of their animals in this study.
GS and RR conceived the study. GS, AS, WH, SB, JH, RZ, and CF collected the data. RR, GS, AS, and MD analyzed data. GS, RR, AS, WH, MD, and HG wrote the manuscript. All authors contributed to the article and approved the submitted version.
GS was supported by the Chiricahua Desert Museum and Georgia State University. AS, SB, and WH were supported by the Desert Botanical Garden. Support for JH, RZ, and CF was by The Phoenix Zoo. HG has been most recently supported by the Lichen Fund and US National Science Foundation (OPUS 1354156).
The authors declare that the research was conducted in the absence of any commercial or financial relationships that could be construed as a potential conflict of interest.
All claims expressed in this article are solely those of the authors and do not necessarily represent those of their affiliated organizations, or those of the publisher, the editors and the reviewers. Any product that may be evaluated in this article, or claim that may be made by its manufacturer, is not guaranteed or endorsed by the publisher.
We are grateful to our respective institutions (Chiricahua Desert Museum, Georgia State University, Desert Botanical Garden, and The Phoenix Zoo) for access to animals, plants, laboratory space, and other courtesies. Brandon La Forrest provided the photo of the snake scat sample (Supplementary Figure 1). Two reviewers provided helpful suggestions that improved an earlier version of the manuscript.
The Supplementary Material for this article can be found online at: https://www.frontiersin.org/articles/10.3389/fevo.2021.761293/full#supplementary-material
Anjos, D. V., Leal, L. C., and Del-Carlo, K. (2020). Ants as diaspore removers of non-myrmecochorous plants: a meta-analysis. Oikos 129, 775–786. doi: 10.1111/oik.06940
Baños-Villalba, A., Blanco, G., Diaz-Luque, J. A., Denes, F. V., Hiraldo, F., and Tella, J. L. (2017). Seed dispersal by macaws shapes the landscape of an Amazonian ecosystem. Sci. Rep. 7:7373. doi: 10.1038/s41598-017-07697-5
Bartel, R. D., Sheppard, J. L., Lovas-Kiss, A., and Green, A. J. (2018). Endozoochory by mallards in New Zealand: what seeds are dispersed and how far? PeerJ 6:e4811. doi: 10.7717/peerj.4811
Baskin, J. M., Baskin, C. C., and Li, X. (2000). Taxonomy, anatomy and evolution of physical dormancy in seeds. Plant Species Biol. 15, 139–152. doi: 10.1046/j.1442-1984.2000.00034.x
Baskin, C. C., and Baskin, J. M. (2014). Seeds: Ecology, Biogeography, and Evolution of Dormancy and Germination, 2nd Edn. San Diego, CA: Academic Press.
Beaupre, S. J. (2016). “Novel tracking and remote monitoring technologies: applications to studying wild rattlesnakes,” in Rattlesnakes of Arizona, Vol. 2, eds G. W. Schuett, M. J. Feldner, C. F. Smith, and R. S. Reiserer (Rodeo, NM: ECO Publishing), 345–363.
Beckman, N. G., Aslan, C. E., Rogers, H. S., Kogan, O., Bronstein, J. L., Bullock, J. M., et al. (2021). Advancing an interdisciplinary framework to study seed dispersal ecology. AoB Plants 12:lz048. doi: 10.1093/aobpla/plz048
Benson, L., and Darrow, R. A. (1981). Trees and Shrubs of the Southwestern Deserts. Tucson, AZ: University of Arizona Press.
Benítez-Malvido, J., González-Di Pierro, A. M., Lombera, R., Guillén, S., and Estrada, A. (2014). Seed source, seed traits, and frugivore habits: implications for dispersal quality of two sympatric primates. Am. J. Bot. 101, 970–978. doi: 10.3732/ajb.1400147
Birskis-Barros, I., Alencar, L. R. V., Prado, P. I., Bohm, M., and Martins, M. (2019). Ecological and conservation correlates of rarity in New World pitvipers. Diversity 11, 147–162. doi: 10.3390/d11090147
Blake, S., Wikelski, M., Cabrera, F., Guezou, M., Silva, M., Sadeghayobi, E., et al. (2012). Seed dispersal by Galápagos tortoises. J. Biogeogr. 39, 1961–1972. doi: 10.1111/j.1365-2699.2011.02672.x
Blanco, G., Hiraldo, F., and Tella, J. L. (2018). Ecological functions of parrots: an integrative perspective from plant life cycle to ecosystem functioning. Emu 118, 36–49. doi: 10.1080/01584197.2017.1387031
Bonnet, X., Pearson, D., Ladyman, M., Lourdais, O., and Bradshaw, D. (2002). “Heaven” for serpents? A mark-recapture study of tiger snakes (Notechis scutatus) on Carnac Island, Western Australia. Austral. Ecol. 27, 442–450. doi: 10.1046/j.1442-9993.2002.01198.x
Bowers, J. E. (1994). Natural conditions for seedling emergence of three woody species in the northern Sonoran Desert. Madroño 41, 73–84.
Bowers, J. E. (2004). Diversified germination behavior of Parkinsonia microphylla (Foothill Paloverde, Fabaceae). Madroño 51, 287–292.
Bowers, J. E., and Turner, R. M. (2002). The influence of climatic variability on local population dynamics of Cercidium microphyllum (Foothill Paloverde). Oecologia 130, 105–113. doi: 10.1007/s004420100779
Bravo, C., Chamorro, D., Hiraldo, F., Speziale, K., Lambertuccci, S. A., Tella, J. L., et al. (2021). Physiological dormancy broken by endozoochory: Austral parakeets (Enicognathus ferrugineus) as legitimate dispersers of calafate (Berberis microphylla) in the Patagonian Andes. J. Plant Ecol. 13, 538–544. doi: 10.1093/jpe/rtaa041
Cabral, S., Frietas, I. S., Morlanes, V., Katzenberger, M., and Calabuig, C. (2019). Potential seed dispersers: a new facet of the ecological role of Boa constrictor constrictor Linnaeus 1758. Biota Neotropica 19:e20180626. doi: 10.1590/1676-0611-bn-2018-0626
Carbajal-Márquez, R. A., Cedeno-Vazquez, J. R., Gonzalez-Solis, D., and Martins, M. (2020). Diet and feeding ecology of Crotalus tzabcan (Serpentes: Viperidae). S. Amer. J. Herpetol. 15, 9–19. doi: 10.2994/SAJH-D-17-00081.1
Castilla, A. M. (2000). Does passage time through the lizard Podarcis lilfordi’s guts affect germination performance in the plant Withania frutescens? Acta Oecol. 21, 119–124. doi: 10.1016/S1146-609X(00)00120-X
Correa, S. B., Winemiller, K. O., LoPez-Fernandez, J., and Galetti, M. (2007). Evolutionary perspectives on seed consumption and dispersal by fishes. Bioscience 57, 748–756. doi: 10.1641/B570907
Coughlan, N. E., Dickey, J. W. E., Cuthbert, R. N., Kelly, T. C., Jansen, M. A. K., and Dick, J. T. A. (2019). Driver’s Seat: understanding divergent zoochorous dispersal of propagules. Front. Ecol. Evol. 7:70. doi: 10.3389/fevo.2019.00070
Daly, M., Jacobs, L. F., Wilson, M. I., and Behernds, P. R. (1992). Scatter hoarding by kangaroo rats (Dopodomys merriami) and pilferages from their caches. Behavioral Ecology 3, 102–111. doi: 10.1093/beheco/3.2.102
Darwin, C. R. (1859). On the Origin of Species By Means of Natural Selection, Or The Preservation of Favoured Races in the Struggle For Life, 1st Edn. London: John Murray. doi: 10.5962/bhl.title.162283
DeSantis, D. L., Mata-Silva, V., Johnson, J. D., and Wagler, A. E. (2020). Integrative framework for long-term activity monitoring of small and secretive animals: validation with a cryptic pitviper. Front. Ecol. Evol. 8:169. doi: 10.3389/fevo.2020.00169
D’hondt, B., Bossuyt, B., Hoffman, M., and Bonte, D. (2007). Dung beetles as secondary seed dispersers in a temperate grassland. Basic Appl. Ecol. 9, 542–549. doi: 10.1016/j.baae.2007.11.002
Donohue, K., Dorn, L., Griffith, C. C., Kim, E. S., Aguilera, A., Polisety, C. R., et al. (2005). Environmental and genetic influences on the germination of Arabidopsis thaliana in the field. Evolution 59, 740–757. doi: 10.1554/04-419
Dugger, P. J., Blendinger, P. G. M., Bohning-Gaese, K., Chama, L., Correia, M., Dehling, D. M., et al. (2019). Seed-dispersal networks are more specialized in the Neotropics than in the Afrotropics. Global Ecol. Biogeogr. 28, 248–261. doi: 10.1111/geb.12833
Falcón, W., Moll, D., and Hansen, D. M. (2020). Frugivory and seed dispersal by chelonians: a review and synthesis. Biol. Rev. 95, 142–166. doi: 10.1111/brv.12558
Fathinia, B., Rodder, D., Rastegar-Pouyani, N., Rastegar-Pouani, E., Hosseinzadeh, M. S., and Kazemi, S. M. (2020). The past, current and future habitat range of the spider-tailed viper, Pseudocerastes urarachnoides (Serpentes: Viperidae) in western Iran and eastern Iraq as revealed by habitat modelling. Zool. Middle East 66, 197–205. doi: 10.1080/09397140.2020.1757910
Franklin, K. A., Sommers, P. N., Aslan, C. E., Lopez, B. R., Bronsetin, J. L., Bustamente, E., et al. (2016). Plant biotic interactions in the Sonoran Desert: Current knowledge and future research perspectives. Int. J. Plant Sci. 177, 217–234. doi: 10.1086/684261
Galetti, M. (2007). Evolutionary perspectives on seed consumption and dispersal by fishes. Bioscience 57, 748–756. doi: 10.1641/B570907
Ginwal, H. S., and Gera, M. (2000). Genetic variation in seed germination and growth performance of 12 Acacia nilotica provenances in India. J. Trop. For. Sci. 12, 286–297.
Gong, H., Tang, C., and Wang, B. (2015). Post-dispersal seed predation and its relations with seed traits: a thirty-species comparative study. Plant Species Biol. 30:193. doi: 10.1111/1442-1984.12051
Gonzalez-Di Pierro, A. M., Benitez-Malvido, J., and Lombera, R. (2021). Germination success of large-seeded plant species ingested by howler monkeys in tropical rain forest fragments. Am. J. Bot. 108, 1625–1634. doi: 10.1002/ajb2.1730
Greene, H. W. (1997). Snakes: The Evolution of Mystery in Nature. Berkeley, CA: University of California Press. doi: 10.1525/9780520935433
Guillen, S., Casas, A., Benıtez, J., and Martınez, Y. M. (2009). Seed germination of wild, managed in situ and cultivated populations of columnar cacti in the Tehuacan-Cuicatlan Valley. J. Arid Environ. 73, 407–413. doi: 10.1016/j.jaridenv.2008.12.018
Hämäläinen, A., Broadley, K., Droghini, A., Haines, J. A., Lamb, C. T., Boutin, S., et al. (2017). The ecological significance of secondary seed dispersal by carnivores. Ecosphere 8:e01685. doi: 10.1002/ecs2.1685
Hanish, C. J., Velez, S., Moore, J. A., and Anderson, C. D. (2020). Endozoochory of Chrysobalanus icaco (Cocoplum) by Gopherus polyphemus (Gopher Tortoise) facilitates rapid germination and colonization in a suburban nature preserve. AoB Plants 12, 1–12. doi: 10.1093/aobpla/plaa024
Hantsch, L., Bruelheide, H., and Erfmeier, A. (2013). High phenotypic variation of seed traits, germination characteristics, and genetic diversity of an invasive annual weed. Seed Sci. Res. 23, 27–40. doi: 10.1017/S096025851200030X
Heleno, R. H., Ross, G., Everard, A., Memmott, J., and Ramos, J. A. (2011). The role of avian ‘seed predators’ as seed dispersers. IBIS 153, 199–203. doi: 10.1111/j.1474-919X.2010.01088.x
Henderson, R. W., Hileman, E. T., Sajdak, R. A., Harrison, B. C., Powell, R., and Bradke, D. R. (2021). Effects of body size, diet, and transience on the demography of the arboreal boid snake Corallus grenadensis on Carriacou (Grenada Grenadines, West Indies). Pop. Ecol. 63, 177–188. doi: 10.1002/1438-390X.12079
Hocking, D. J., and Babbitt, K. J. (2014). Amphibian contributions to ecosystem services. Herpetol. Conserv. Biol. 9, 1–17. doi: 10.1371/journal.pone.0086854
Hope, A. G., and Parmenter, R. R. (2007). Food habits of rodents inhabiting arid and semi-arid ecosystems of central New Mexico. Special Publ. Mus. Southwest. Biol. 9, 1–75.
Jaganathan, G. K., Yule, K., and Liu, B. (2016). On the evolutionary and ecological value of breaking physical dormancy by endozoochory. Perspect. Plant Ecol. Evol. Syst. 22, 11–22. doi: 10.1016/j.ppees.2016.07.001
Jones, W. T. (1989). Dispersal distance and the range of nightly movements in Merriam’s kangaroo rats. J. Mammal. 70, 27–34. doi: 10.2307/1381666
Kaufman, K., Bowers, R., and Bowers, N. (2004). Mammals of North America. New York, NY: Houghton Mifflin Harcourt.
King, R. B., Stanford, K. M., and Jones, P. C. (2018). Sunning themselves in heaps, knots, and snarls: the extraordinary abundance and demography of island watersnakes. Ecol. Evol. 8, 7500–7521. doi: 10.1002/ece3.4191
Klauber, L. M. (1972). Rattlesnakes; Their Habits, Life histories, and Influence on Mankind, 2nd Edn, Vol. 2. Berkeley CA: University of California Press.
Lichti, N., Steele, M. A., and Swihart, R. K. (2017). Seed fate and decision-making processes in scatter hoarding rodents. Biol. Rev. 92, 474–504. doi: 10.1111/brv.12240
Love, J., Selker, R., Marsman, M., Jamil, T., Dropmann, D., Verhagen, J., et al. (2019). JASP: graphical statistical software for common statistical designs. J. Stat. Softw. 88, 1–17. doi: 10.18637/jss.v088.i02
Luna, P., Garcia-Chavez, J. H., and Dattilo, W. (2018). Complex foraging ecology of the red harvester ant and its effect on the soil seed bank. Acta Oecol. 86, 57–65. doi: 10.1016/j.actao.2017.12.003
Maritz, B., Penner, J., Martins, M., Crnobrnja-Isailovic, J., Spear, S., Alencar, L. R. V., et al. (2016). Identifying global priorities for the conservation of vipers. Biol. Conserv. 204, 94–102. doi: 10.1016/j.biocon.2016.05.004
Martins, M., and Lillywhite, H. B. (2019). “Ecology of snakes on islands,” in Islands and Snakes: Isolation and Adaptive Evolution, eds H. B. Lillywhite and M. M. Martins (New York, NY: Oxford University Press), 1–44.
McAuliffe, J. R. (1986). Herbivore-limited establishment of a Sonoran Desert tree Seed fate and decision-making processes in scatter hoarding rodents, Cercidium microphyllum. Ecology 67, 276–280. doi: 10.2307/1938533
McAuliffe, J. R. (1990). Paloverdes, pocket mice, and bruchid beetles: interrelationships of seeds, dispersers, and seed predators. Southwest. Nat. 35, 329–337. doi: 10.2307/3671949
Medeiros, A. S., and Drezner, T. D. (2012). Vegetation, climate, and soil relationships across the Sonoran Desert. Ecoscience 19, 148–160. doi: 10.2980/19-2-3485
Midgley, J. J., White, J. D. M., Johnson, S. D., and Bronner, G. N. (2015). Faecal mimicry by seeds ensures dispersal by dung beetles. Nat. Plants 1:15141. doi: 10.1038/nplants.2015.141
Mitchell, J., Johnston, I. G., and Bassel, G. W. (2017). Variability in seeds: biological, ecological, and agricultural implications. J. Exp. Bot. 68, 809–817.
Morris, D. (1962). The behaviour of the green acouchi (Myoprocta pratti) with special reference to scatter-hoarding. Proc. Zool. Soc. Lond. 139, 701–732. doi: 10.1111/j.1469-7998.1962.tb01601.x
Nathan, R., Schurr, F. M., Spiegel, O., Steinitz, O., and Trakhtenbrot, A. (2008). Mechanisms of long-distance seed dispersal. Trends Ecol. Evol. 23, 638–647. doi: 10.1016/j.tree.2008.08.003
Padilla, D. P., Gonzalez-Castro, A., and Nogales, M. (2012). Significance and extent of secondary seed dispersal by predatory birds on oceanic islands: the case of the Canary Archipelago. J. Ecol. 100, 416–427. doi: 10.1111/j.1365-2745.2011.01924.x
Pascov, C. M., Nevill, P. G., Elliott, C. P., Majer, J. D., Anthony, J. M., and Krauss, S. L. (2015). The critical role of ants in the extensive dispersal of Acacia seeds revealed by genetic parentage assignment. Oecologia 179, 1123–1134. doi: 10.1007/s00442-015-3400-9
Pérez-Méndez, N., and Rodríguez, A. (2018). “Raptors as seed dispersers,” in Birds of Prey: Biology and Conservation in the 21st century, ed. J. H. Sarasola (Gewerbestrasse: Springer International Publishing AG), 139–157. doi: 10.1007/978-3-319-73745-4_6
Price, M. V., Waser, N. M., and McDonald, S. (2000). Seed caching by heteromyid rodents from two communities: implications for coexistence. J. Mammal. 81, 97–106. doi: 10.1644/1545-1542(2000)081<0097:SCBHRF>2.0.CO;2
R Core Team (2021). R: A Language and Environment for Statistical Computing. Vienna: R Foundation for Statistical Computing.
Reiserer, R. S., Schuett, G. W., and Greene, H. W. (2018). Seed ingestion and germination in rattlesnakes: overlooked agents of rescue and secondary dispersal. Proc. R. Soc. B 285, 20172755. doi: 10.1098/rspb.2017.2755
Rubalcava-Castillo, F. A., Sosa-Ramirez, J., Luna-Ruiz, J. J., Valdivia-Flores, A. G., Diaz-Nunez, V., and Iniguez-Davalos, L. I. (2020). Endozoochorous dispersal of forest seeds by carnivorous mammals in Sierra Fría, Aguascalientes, Mexico. Ecol. Evol. 10, 2991–3003. doi: 10.1002/ece3.6113
Salazar-Rivera, G. I., Sanchez-Velasquez, L. R., and Inzunza, E. R. (2020). Passage through a bird’s gut confers a germination head start to the seeds of the Nightshade Witheringia stramoniifolia. Tropical Conserv. Sci. 13, 1–24. doi: 10.1177/1940082920931093
Saldaña-Vázquez, R. A., Castano, J. H., Baldwin, J., and Perez-Torres, J. (2019). Does seed ingestion by bats enhance germination? A new meta-analysis 15 years later. Mammal. Rev. 49, 201–209. doi: 10.1111/mam.12153
Sarasola, J. H., Zanon-Martinez, J. I, Costan, A. S., and Ripple, W. J. (2016). Hypercarnivorous apex predator could provide ecosystem services by dispersing seeds. Sci. Rep. 6, 19647. doi: 10.1038/srep19647
Schuett, G. W., Repp, R. A., Spencer, C. L., Beaman, K. R., and Painter, C. W. (2016). “Crotalus atrox (Western Diamond-backed Rattlesnake,” in Rattlesnakes of Arizona, Vol. 1, eds G. W. Schuett, M. J. Feldner, C. F. Smith, and R. S. Reiserer (Rodeo, NM: ECO Publishing), 333–394.
Schupp, E. W., Jordano, P., and Gomez, J. M. (2010). Seed dispersal effectiveness revisited: a conceptual review. New Phytol. 188, 333–353. doi: 10.1111/j.1469-8137.2010.03402.x
Silva, H. R., and de Britto-Pereira, M. C. (2006). How much fruit do fruit-eating frogs eat? An investigation on the diet of Xenohyla truncata (Lissamphibia: Anura: Hylidae). J. Zool. 270, 692–698. doi: 10.1111/j.1469-7998.2006.00192.x
Smith, B. W. (2005). Venomous Snakes in Captivity: Safety and Husbandry. Scotts Valley, CA: CreateSpace Independent Publishing Platform.
Steyaert, S. M. J. G., Hertel, A. G., and Swenson, J. E. (2019). Endozoochory by brown bears stimulates germination in bilberry. Wildlife Biol. 219, 1–5. doi: 10.2981/wlb.00573
Traveset, A., Escribano-Avila, G., Gómez, J. M., and Valido, A. (2019). Conflicting selection on Cneorum tricoccon (Rutaceae) seed size caused by native and alien seed dispersers. Evolution 73, 2204–2215. doi: 10.1111/evo.13852
Traveset, A., Heleno, R., and Nogales, M. (2014). “The ecology of seed dispersal,” in Seeds: The Ecology of Regeneration in Plant Communities, vol. 3.1, ed. R. S. Gallaguer (Oxfordshire: CAB International), 62–93. doi: 10.1079/9781780641836.0062
Traveset, A., Riera, N., and Mas, R. E. (2001). Passage through bird guts causes interspecific differences in seed germination characteristics. Funct. Ecol. 15, 669–675. doi: 10.1046/j.0269-8463.2001.00561.x
Traveset, A., Roberstson, A., and Rodriguez-Perez, J. (2007). “A review on the role of endozoochory on seed germination,” in Seed Dispersal: Theory and its Application in a Changing World, eds A. J. Dennis, E. W. Schupp, R. J. Green, and D. A. Westcott (Wallingford: CAB International), 78–103. doi: 10.1079/9781845931650.0078
Traveset, A., Rodriguez-erez, J., and Pias, B. (2008). Seed trait changes in dispersers’ guts and consequences for germination and seedling growth. Ecology 89, 95–106. doi: 10.1890/07-0094.1
Valido, A., and Olesen, J. M. (2019). Frugivory and seed dispersal by lizards: a global review. Front. Ecol. Evol. 7:49. doi: 10.3389/fevo.2019.00049
Van Klinken, R. D., Flacck, L. K., and Pettit, W. (2006). Wet-season dormancy release in seed banks of a tropical leguminous shrub is determined by wet heat. Ann. Bot. 98, 875–883. doi: 10.1093/aob/mcl171
van Leeuwen, C. H. A., Lovas-Kiss, A., Ovegard, M., and Green, A. J. (2017). Great cormorants reveal overlooked secondary dispersal of plants and invertebrates by piscivorous waterbirds. Biol. Lett. 13:20170406. doi: 10.1098/rsbl.2017.0406
Vander Wall, S. B., and Longland, W. S. (2004). Diplochory: are two seed dispersers better than one? Trends Ecol. Evol. 19, 155–161. doi: 10.1016/j.tree.2003.12.004
Vander Wall, S. B., Longland, W. S., Pyare, S., and Veech, J. A. (1998). Cheek pouch capacities and loading rates of heteromyid rodents. Oecologia 113, 21–28. doi: 10.1007/s004420050349
Wang, B., and Cortlett, R. T. (2017). Scatter-hoarding rodents select different caching habitats for seeds with different traits. Ecosphere 8:e01774. doi: 10.1002/ecs2.1774
Warwick, C., Frye, F. L., and Murphy, J. B. (1995). Health and Welfare of Captive Reptiles. London: Chapman and Hall. doi: 10.1007/978-94-011-1222-2
Keywords: Crotalus atrox, Foothill Palo Verde, diploendozoochory, Lampropeltis splendida, Pituophis catenifer, reptiles, seed rescue, seed dispersal
Citation: Schuett GW, Reiserer RS, Salywon AM, Blackwell S, Hodgson WC, Foster CD, Hall J, Zach R, Davis MA and Greene HW (2022) Secondary Seed Ingestion in Snakes: Germination Frequency and Rate, Seedling Viability, and Implications for Dispersal in Nature. Front. Ecol. Evol. 9:761293. doi: 10.3389/fevo.2021.761293
Received: 19 August 2021; Accepted: 24 November 2021;
Published: 04 January 2022.
Edited by:
Haldre S. Rogers, Iowa State University, United StatesReviewed by:
Julieta Benitez-Malvido, National Autonomous University of Mexico, MexicoCopyright © 2022 Schuett, Reiserer, Salywon, Blackwell, Hodgson, Foster, Hall, Zach, Davis and Greene. This is an open-access article distributed under the terms of the Creative Commons Attribution License (CC BY). The use, distribution or reproduction in other forums is permitted, provided the original author(s) and the copyright owner(s) are credited and that the original publication in this journal is cited, in accordance with accepted academic practice. No use, distribution or reproduction is permitted which does not comply with these terms.
*Correspondence: Gordon W. Schuett, Z3dzY2h1ZXR0QHlhaG9vLmNvbQ==; Mark A. Davis, ZGF2aXM2M0BpbGxpbm9pcy5lZHU=
Disclaimer: All claims expressed in this article are solely those of the authors and do not necessarily represent those of their affiliated organizations, or those of the publisher, the editors and the reviewers. Any product that may be evaluated in this article or claim that may be made by its manufacturer is not guaranteed or endorsed by the publisher.
Research integrity at Frontiers
Learn more about the work of our research integrity team to safeguard the quality of each article we publish.