- School of Biological Sciences, University of Auckland, Auckland, New Zealand
Birds are our best models to understand vocal learning – a vocal production ability guided by auditory feedback, which includes human language. Among all vocal learners, songbirds have the most diverse life histories, and some aspects of their vocal learning ability are well-known, such as the neural substrates and vocal control centers, through vocal development studies. Currently, species are classified as either vocal learners or non-learners, and a key difference between the two is the development period, extended in learners, but short in non-learners. But this clear dichotomy has been challenged by the vocal learning continuum hypothesis. One way to address this challenge is to examine both learners and canonical non-learners and determine whether their vocal development is dichotomous or falls along a continuum. However, when we examined the existing empirical data we found that surprisingly few species have their vocal development periods documented. Furthermore, we identified multiple biases within previous vocal development studies in birds, including an extremely narrow focus on (1) a few model species, (2) oscines, (3) males, and (4) songs. Consequently, these biases may have led to an incomplete and possibly erroneous conclusions regarding the nature of the relationships between vocal development patterns and vocal learning ability. Diversifying vocal development studies to include a broader range of taxa is urgently needed to advance the field of vocal learning and examine how vocal development patterns might inform our understanding of vocal learning.
Introduction
The ability to alter or incorporate vocalizations based on auditory experience is termed vocal learning (Petkov and Jarvis, 2012). Although vocal learning is thought to be a rare trait in the animal kingdom, it is found in three clades of birds, the hummingbirds, parrots and songbirds, but is considered most pervasive amongst songbirds (Goller and Shizuka, 2018; Tyack, 2019; Aamodt et al., 2020). Songbirds are the most diverse and specious order of birds and include three sub-orders, the oscines (suborder: Passeri,∼5,000 species), suboscines (suborder: Tyranni,∼1,000 species), and the New Zealand wrens (suborder: Acanthisitti, ∼2 species) (Oliveros et al., 2019). The ability to incorporate novel sounds into the repertoire is thought to be an essential driver of the oscine rapid diversification in the Miocene (23.5 million years ago) compared to its non-learning sister suborders (Seddon, 2005; Mason et al., 2017; Oliveros et al., 2019; Kuhl et al., 2020). The critical difference between these groups is that oscines require hearing conspecifics songs in order to sing species typical songs, while suboscines can develop typical songs without hearing conspecific vocalizations during development (Kroodsma and Konishi, 1991; Kiefer et al., 2006; Mooney, 2009). Vocal learning in New Zealand wrens is unknown but presumed to be like suboscines (Raikow and Bledsoe, 2000), and will be considered with suboscines for the remainder of the text. Distinct differences are also observed in syrinx morphology and brain structures (Amador et al., 2008; Düring and Elemans, 2016; Garcia et al., 2017). It has also been stated that the two groups differ in terms of vocal development periods, but without a systematic examination of this distinction (Doupe, 1997; Ashmore et al., 2005; London, 2019; Adam and Elemans, 2020).
The Life History and Vocal Development in Songbirds
Songbirds are the most diverse group of vocal learners in nature, with 63 documented families of vocal learning species (Kuhl et al., 2020) and 43 families of vocal mimicking species (Goller and Shizuka, 2018). Songbirds also have diverse life-history strategies, having filled a wide array of ecological and acoustic niches during evolution (Xiao et al., 2017), which in turn drives vocal evolution (Seddon, 2005). These diverse life-histories are reflected in their vocal development strategies (Nottebohm, 1970; Matsunaga and Okanoya, 2009). For example, migratory species often experience seasonal changes in testosterone levels that affect the length of the critical learning window (Goldman and Nottebohm, 1983; Whaling et al., 1995; Alliende et al., 2010). Species with longer lifespans, changing social environments, or delayed sexual maturity may have an extended critical vocal learning period (Kroodsma et al., 2013; Wirthlin et al., 2018). Polygamous breeders constantly compete with peers for new partners and may be under more substantially stronger sexual selection pressure than monogamous breeders. Thus, polygamous breeders may more commonly retain the ability to learn throughout adulthood (Creanza et al., 2016; Robinson et al., 2019). Therefore, songbirds are an outstanding group for comparative analyses between species using life-history traits and vocal development patterns to understand vocal learning (Matsunaga and Okanoya, 2009).
Using Songbird Vocal Development to Understand the Vocal Learning Continuum
Oscine songbirds have a sensitive period during vocal development to acquire songs by listening to tutors. Vocal development in oscines follow three stages, (1) subsong, akin to babbling in children, (2) plastic song, a period of vocal exploration involving overproduction of sounds, and (3) crystallization, an ultimate stage where the adult song is achieved. In contrast, the current wisdom around non-learners, such as suboscine songbirds and other non-oscines, is that their vocal development does not rely on auditory feedback and thus is shorter, limited only by the maturation of the vocal organ (but see Liu et al., 2013; Maxwell et al., 2021).
The duration of the sensitive period and each vocal development phase, and the extent to which they overlap, vary between species. In our opinion, this variation in vocal development periods is an excellent tool for contrasting between the traditional ideas around vocal learning – that vocal learning is a binary trait, present in just a few groups and absent in all others (Jarvis, 2006) – and the recently proposed vocal learning continuum hypothesis, which has the potential to be paradigm-shifting (Arriaga et al., 2012; Petkov and Jarvis, 2012; Arriaga and Jarvis, 2013; Jarvis, 2019; Wirthlin et al., 2019; Martins and Boeckx, 2020). This emerging hypothesis argues that vocal learning exists along a gradient instead of a dichotomy and may be more widespread in the animal world than previously thought. The continuum hypothesis argues that vocal learning follows stepwise transitions from having (1) innate vocalizations, (2) limited learning (i.e., strong learning template of conspecifics), and (3) learning limited to the juvenile period, to finally (3) open-ended learning (i.e., adult plasticity and vocal mimicking of its own and other species) (Petkov and Jarvis, 2012). Recent advancements on this continuum hypothesis suggests that vocal learning has multiple dimensions and modules with gray areas in classification, which may benefit from a functional and mechanistic approach in understanding vocal learning (Wirthlin et al., 2019; Vernes et al., 2021; Wright and Derryberry, 2021). Behavioral trait sharing between oscine and suboscine species has been supported in a few studies; some suboscines show long development periods and some form of vocal change influenced by auditory feedback (Lovell and Lein, 2004; Leger, 2005; Saranathan et al., 2007; Kroodsma et al., 2013; Liu et al., 2013; Capelli et al., 2020).
Because the traditional dichotomy between learners and non-learners predicts clear differences in the vocal development periods of the two groups, vocal development periods could provide critical data for evaluating these contrasting hypotheses. However, as detailed below, most vocal development research has focused, for good reasons, on robust species that are easy to rear in captivity. Most of this research has relied on methods requiring auditory and visual isolation from tutors. Procedures like these are not feasible for many species, such as those that have a specialized diet, do not survive well in captivity, or are threatened or endangered. Less invasive vocal development studies have investigated natural free-ranging populations by monitoring artificial nest boxes (Sawhney et al., 2006) or natural nests with automated bio-acoustic recorders (Loo et al., unpublished data) and conducting playback experiments (Mennill et al., 2018, 2019) during vocal development stages (Moran et al., unpublished data). Although these techniques have less experimental control than laboratory experiments, they still provide valuable insight into the variation in vocal development strategies while allowing research into broader array of species and optimizing animal welfare. If vocal development patterns and life history strategies are associated with learning abilities, we could use vocal development as a proxy for estimating learning ability in species that are not amenable to traditional laboratory learning research.
However, elucidating these relationships requires a wide array of species in broad taxonomic levels, including those that are canonically non-vocal learners. Here, we examine the existing literature to determine the breadth and depth of vocal development data, in an effort to determine whether we can explore the potential questions outlined above, and which taxa would be the most useful for expanding these studies. Because we find large gaps and biases in the data we do have, we also offer recommendations for alternative methods of gathering the needed data.
Gaps in Current Vocal Development Research
We conducted a systematic literature review of songbird vocal development periods (see Supplementary Table 1 for review methods). We included only manuscripts which documented the natural onset dates or periods of subsong, plastic song, crystallization, and/or sensitive learning. We excluded inconclusive studies and studies without documentation of vocal development dates in relation to age. We also excluded studies reporting vocal development dates that were shifted by experimentation. We found only 79 manuscripts (including an unpublished study on Acanthisitta chloris granti) documenting vocal development times in birds (Supplementary Data 1). Within these studies, only 42 species (including the additional two subspecies of white-crowned sparrows) had exact or estimated dates for any vocal development stage (Figure 1), with scattered information on subsong onset (n = 24), crystallization (n = 21), begging call onset (n = 12), sensitive period (n = 10), contact call onset (n = 9), and plastic song onset (n = 8), where, n is the number of species. In addition, we found that the available studies showed a distinct bias toward a handful of species. More than one-third (35.44%) of the 79 studies focus on only two species, zebra finches (Taeniopygia guttata, 25.31%) and white-crowned sparrows (Zonotrichia leucophrys, 10.13%; including the two subspecies, Z. l. oriantha and Z. l. nuttalli). Furthermore, species documented were mainly found in North America (n = 20), Central America (n = 15), and Europe (n = 9) while other regions covered eight species or fewer. Two studies out of 42 studied female vocal development while the rest of the studies are either on males or of unspecified sex. We also found that the vocal development times of the studied species are highly variable and do not show any clear patterns, and certainly do not match the two well-studied model species (Figure 2). This variation is apparent when comparing each species’ vocal development strategies and life history with available vocal development data (Figure 2 and Supplementary Data 1).
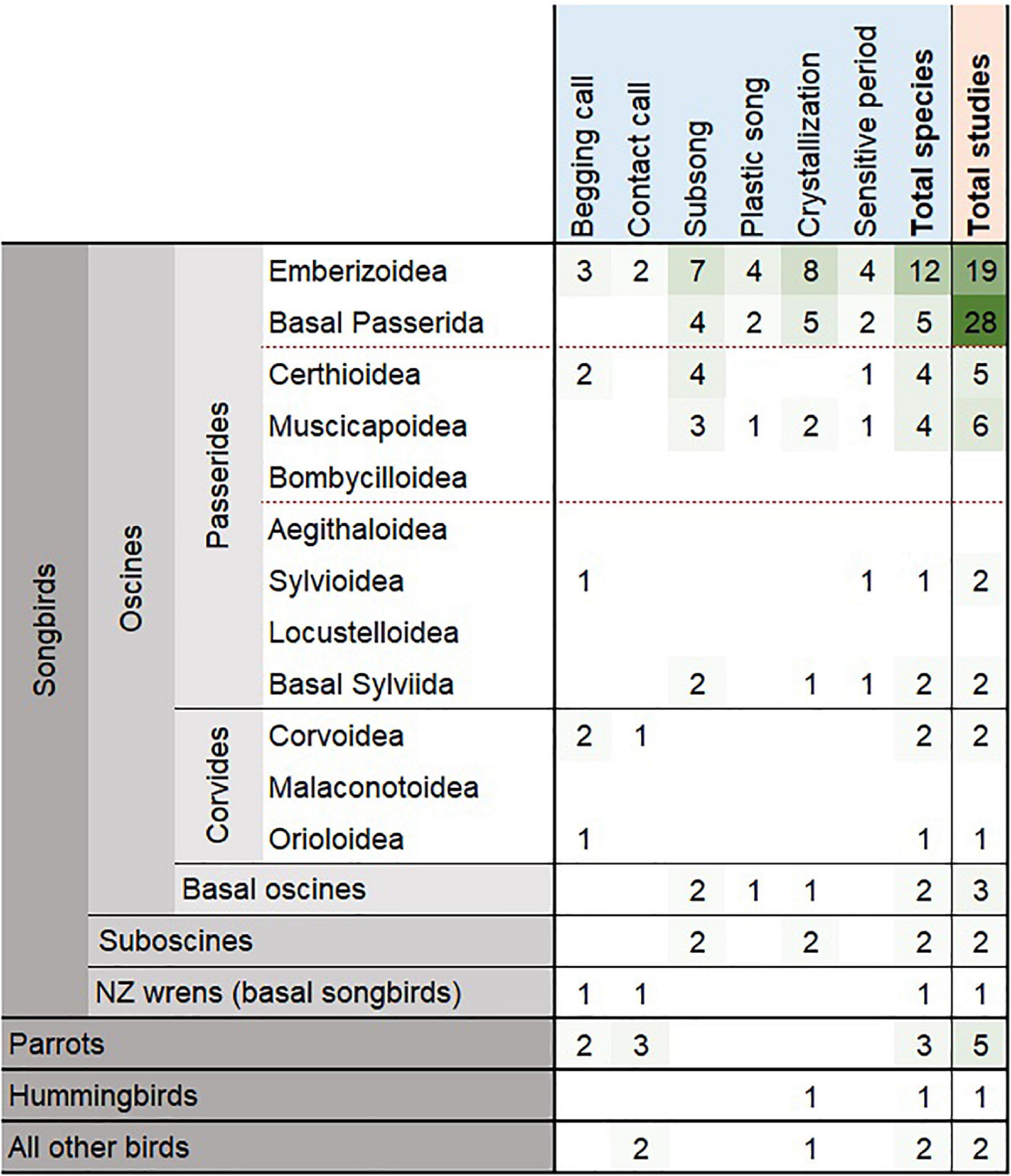
Figure 1. The number of species (light blue) and number of studies (pale orange) with reported vocal development dates of birds (see Supplementary Data 1 for citations). Bird group classifications follow Oliveros et al. (2019), and are represented as dark gray for orders, medium gray for suborders, and light gray for infraorders. The oscines are represented by basal oscines, Corvides and Passerides infraorders, and red dashed lines separate parvorder groups of superfamily lineages. Parrots, hummingbirds, and all other birds are represented only in order level or higher for phylogenetic comparison to songbirds. Intensity in green shows number of species or studies in each bird group.
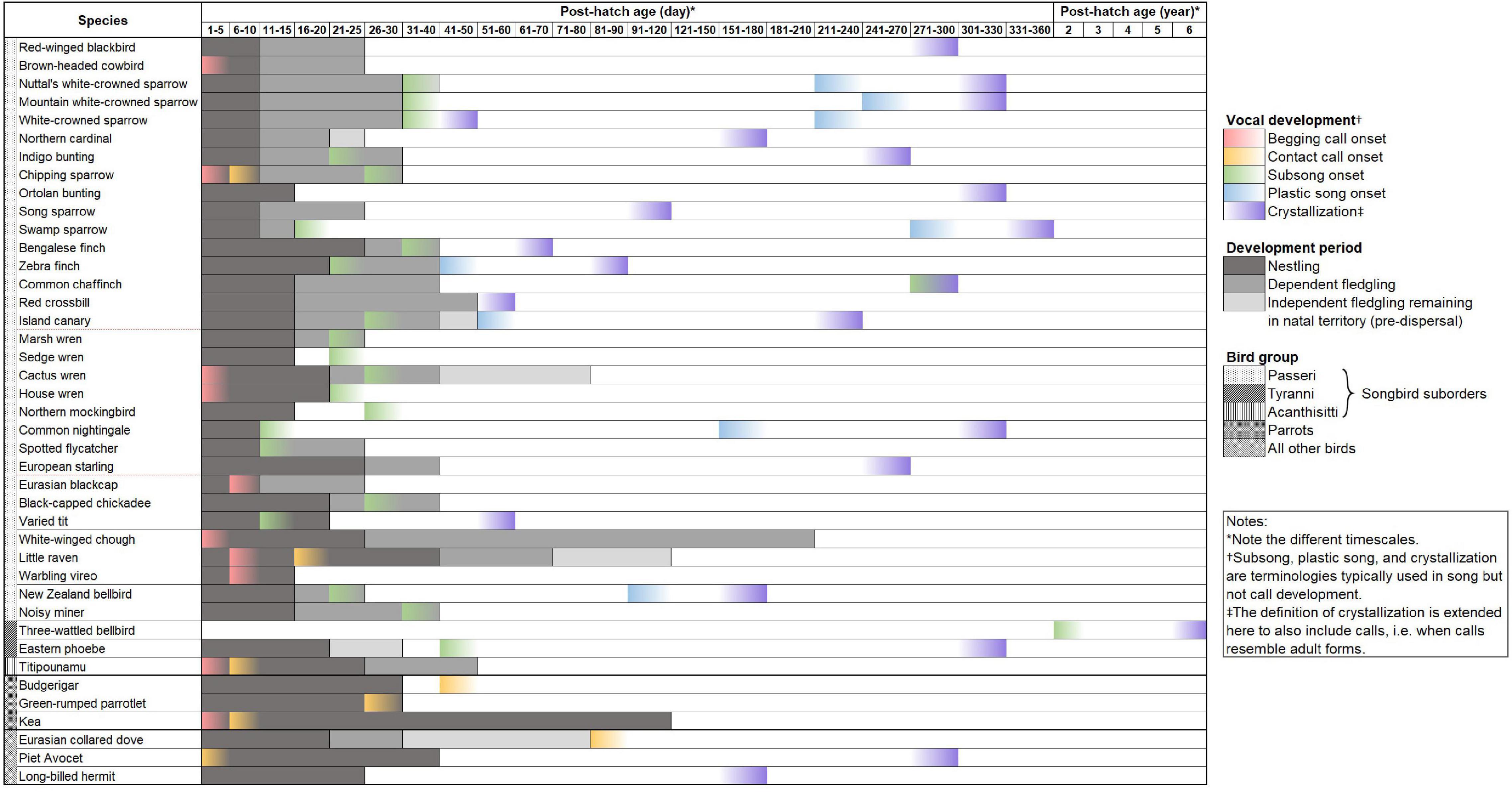
Figure 2. Bird species [ordered from derived clades at the top to older clades at the bottom following Oliveros et al. (2019)] with published vocal development data, showing the onset of vocal development stages and the first instance of crystallization, which excludes subsequent adult learning and crystallization, e.g., in seasonal birds such as Island canaries (see Supplementary Data 1 for citations). This data shows that closely related songbird species can have different vocal development patterns, and that there is a lack of representation of suboscines. Parrots are added for comparison with songbirds, as parrots are vocal learners that mainly have calls. Some non-oscine non-learners such as Eurasian collared dove and pied avocets are also included for comparisons between learners and non-learners. The other song learning group, the hummingbirds, is only represented by one species, the long-billed hermit, but is added for completeness of data. On the species column, classifications follow Oliveros et al. (2019); thick horizontal solid lines separate bird orders, thin horizontal solid lines separate different suborders within songbirds and parrots, and red dashed lines separate parvorders in songbirds. Future research contributing to this dataset by adding underrepresented species will be critical to quantify the variation on vocal development periods and potentially producing a method to systematically sort species along the vocal learning continuum.
Pitfalls of Universality
We identified four primary forms of biases in existing vocal development studies caused by the skewed representation of species: (1) a handful of model species are unlikely to be representative of the variation of vocal development patterns and life history strategies across all vocal learners; (2) the vocal development of oscines is far better documented than that of suboscines and non-oscines; (3) vocal learning research has mainly focused on Northern hemisphere species in which only males sing, but in most species, both sexes produce song; and (4) vocal development research has focused on songs while neglecting calls. Below we discuss the consequences of those biases and why they are problematic for our understanding of vocal development in relation to vocal learning.
Focus on Two Model Species
The tight focus on two species, zebra finches and white-crowned sparrows, for understanding vocal learning and development in songbirds has contributed to an incredibly detailed understanding of the neuro-mechanics of vocal learning (Nottebohm, 1992; Brainard and Doupe, 2002; Fee et al., 2004; Mooney, 2009). These two species are then used as models for inferring the vocal learning abilities of all other species (Petkov and Jarvis, 2012). For example, zebra finches crystallize their adult song within the first 3 months of life (Nottebohm, 1992), but most other studied species crystallize in the following spring. Similarly, white-crowned sparrows begin their subsong after leaving the natal territory, but most other birds begin their subsong when they are still dependent fledglings (Nelson et al., 1995; Whaling et al., 1995; Park et al., 2005). Both zebra finches and white-crowned sparrows crystallize their song before their first breeding season and are unable to add or improvise vocalizations in adulthood, unlike open-ended vocal learners (see Araya-Salas and Wright, 2013; Labra and Lampe, 2018). Furthermore, even from the limited data available, it is clear that even among closely related species (such as among white-crowned sparrow subspecies and between song sparrows and swamp sparrows, see Figure 2), there are differences in vocal development stages, such as length and onset, which may be related to life history strategy, such as migratory status, age of sexual maturity, mating strategy, and lifespan (see Supplementary Data 1). These factors may provide critical insights into the evolution of vocal learning and development. In addition, both model species are recently evolved species and are unlikely to be good representatives of the variation in vocal development for all songbirds. Further, zebra finches are nomadic and opportunistic breeders, while white-crowned sparrows are migratory, with very short breeding seasons, neither of which represent the majority of bird species.
Ignoring Suboscines
Of the 79 studies on vocal development found in our literature search, there was only two on suboscines (three-wattled bellbird, Procnias tricarunculatus and eastern phoebe, Sayornis Phoebe), and only six are on non-oscine species. This suggests that the distinct difference in the vocal development patterns of vocal learners and non-learners, as commonly referred to in the literature, is not based on solid empirical data patterns. Further, our data suggest that even among the species that have been studied, there is no apparent difference in the development patterns of oscine and suboscines (Saranathan et al., 2007). For example, Liu et al. (2013) found that the song crystallization of eastern phoebes is approximately 305 days post hatch, which is as long as (e.g., sparrows and common nightingale) or longer (e.g., finches and European starling) than some oscines (see Figure 2).
A hallmark of vocal learning in oscine songbirds is that they produce atypical songs when raised in isolation, without a tutor (Eda-Fujiwara et al., 1995; Livingston and Mooney, 2001). However, in one population of tutor-less zebra finches, song features converged and resembled those of normally reared birds within a few generations (Fehér et al., 2009; Love et al., 2019). In addition, recent work has shown that rudimentary vocal learning may be present in some suboscines, which are assumed to be non-vocal learners. Evidence suggests that behaviors associated with vocal learning can also be found in suboscine songbirds. For example, both three-wattled bellbirds and bare-throated bellbirds (Procnias nudicollis) exhibit traits thought to only be found in vocal learners, such as heterospecific mimicry during vocal development, abnormal isolate songs, a protracted vocal development period of 6 years, and adult plasticity (Kroodsma et al., 2013). This ability may be an essential factor driving suboscine evolution (Freeman et al., 2017).
A key prediction of the vocal learning continuum hypothesis is a gradation in vocal learning ability (Petkov and Jarvis, 2012; Martins and Boeckx, 2020). Ignoring suboscines and other non-vocal learning species means we are unable to detect nascent or rudimentary vocal learning; we are not even looking for it. Thus, it is imperative to determine whether oscines and suboscines have different learning mechanisms to classify these species along the continuum. Again, this effort would require detailed studies of vocal development in underrepresented taxa.
Ignoring Females
In most model song species, only males sing. Moreover, only two studies on vocal development reports patterns for females, the New Zealand bellbirds (Anthornis melanura) (Roper et al., 2018), and the northern cardinals (Richmondena cardinalis) (Dittus and Lemon, 1969). However, it is now clear that female song is widespread in songbirds, the ancestral state, and is the norm in the tropics and southern hemispheres (Odom et al., 2014; Odom and Benedict, 2018). Studies show that female song serves a critical function as a competitive trait among females to compete for reproductive resources (Langmore, 1998; Cain and Langmore, 2015; Brunton et al., 2016). Further, even for well-studied species in which females were not reported to sing, new research has shown that females simply sing less frequently, for shorter periods, or for different purposes (Reichard et al., 2018; Wilkins et al., 2020; Sierro et al., 2021), thus reducing the detectability of female song, especially in monomorphic species. However, almost nothing is known about female song learning and their development periods, whom they learn from, how they learn, or whether their development patterns are similar to males (Riebel et al., 2005; Evans and Kleindorfer, 2016; Roper et al., 2018). Choe and Jarvis (2021) recently found that males and females use similar mechanisms for vocal learning circuits, and sex chromosomes and sex steroid hormones may explain the loss of vocal learning in females of some species. Thus, a clear understanding of female vocal development patterns and how they relate to life-history traits is vital to understand why females in some species and regions have lost their song, whether males and females use different mechanisms, and whether a switch from social selection pressures that affect males and females similarly, to sex-specific sexual selection pressures, leads to new patterns (Rivera-Cáceres and Templeton, 2019).
Ignoring Call Development
Vocal learning research in songbirds has almost exclusively focused on song learning, likely due to the assumption that calls are not learned (Beme, 2006; Balsby et al., 2012). This is a surprising assumption given that parrots, the most accomplished vocal learners outside of humans, do not sing but communicate mostly through calls (Balsby et al., 2012), apart from budgerigars (Melopsittacus undulatus) which is a parrotlet that produces so-called “warble songs” (Brockway, 1964a, b). However, this assumption is also rapidly collapsing as increasing data finds evidence of call learning in songbirds. Simpson and Vicario (1990) found that the same brain regions for learning songs are used for learning calls in male zebra finches. Furthermore, the food begging call of brood parasites matches that of its host species (Anderson et al., 2010; Ranjard et al., 2010; Liu et al., 2016). Another study on chipping sparrows (Spizella passerina), where only males sing and learn, showed that male food begging calls become more variable than females after fledging (Liu et al., 2009). In addition, call developmental patterns, such as voice breaking in cranes following delayed vocal development patterns akin to vocal learners, are overlooked (Klenova et al., 2010, 2014, 2020). Even vocalizations long thought to be innate, with no sexual selection pressure acting on them, such as distress calls, appear to change more rapidly in vocal learners than non-learners (Moran et al., unpublished data). In our opinion, studying species that use calls in multiple behavioral contexts will be helpful to understand why there is a spectrum in the vocal learning ability, whether it is governed by the need for incorporating complex functional or social information.
Call for Action to Advance the Field of Vocal Learning
The evolutionary pathway of vocal learning may have originated in non-mutually exclusive behavioral modules, such as vocal versatility, vocal production variability, and vocal coordination (Wirthlin et al., 2019). Categorizing species into these modules will be a crucial starting point in separating the different mechanisms underlying the vocal learning continuum hypothesis. The current body of knowledge is incapable of representing the complexity and variation in avian vocal development because of significant data gaps. To a large extent, model species have been explicitly selected because they are robust to captive environments. Though model species have made crucial advances on vocal learning, future research needs to focus on under-studied species (e.g., basal songbirds and suboscines) and other types of vocalizations (e.g., calls and female song) to capture the diversity of vocal development patterns in birds. We suggest that addressing the biases and gaps in vocal development research can put the vocal learning continuum hypothesis into context and thus for the field of vocal learning to advance. With rapidly evolving technology, such as passive acoustic recorders paired with motion- and heat-sensing cameras, backpack microphones, and microphone arrays, field studies are more amiable for threatened, and endangered species or species that do not survive in captivity. These methods will allow more ways to measure critical vocal learning periods in wild bird populations indirectly. If vocal development patterns become known across a broader range of life-history strategies and species, we may uncover the developmental pre-requisites of a vocal learner and further use these concepts to answer questions about why vocal learning evolved in the animal kingdom.
Data Availability Statement
The raw data supporting the conclusions of this article will be made available by the authors, without undue reservation.
Author Contributions
YL and KC contributed equally to the idea and opinion development. YL conducted the literature review and wrote the manuscript. KC wrote the “Ignoring Females” section and reviewed and edited the manuscript. Both authors contributed to the article and approved the submitted version.
Conflict of Interest
The authors declare that the research was conducted in the absence of any commercial or financial relationships that could be construed as a potential conflict of interest.
Publisher’s Note
All claims expressed in this article are solely those of the authors and do not necessarily represent those of their affiliated organizations, or those of the publisher, the editors and the reviewers. Any product that may be evaluated in this article, or claim that may be made by its manufacturer, is not guaranteed or endorsed by the publisher.
Acknowledgments
We thank the University of Auckland for funding this publication. We acknowledge the contribution of colleagues, Margaret Stanley, Sarah Withers, Ines Moran, Laura Duntsch, and Melissa Kam Yit Yee, who provided valuable feedback to an earlier version of this manuscript. We also thank the reviewers for their comments that improved the quality of this manuscript.
Supplementary Material
The Supplementary Material for this article can be found online at: https://www.frontiersin.org/articles/10.3389/fevo.2021.757972/full#supplementary-material
References
Aamodt, C. M., Farias-Virgens, M., and White, S. A. (2020). Birdsong as a window into language origins and evolutionary neuroscience. Philos. Trans. R. Soc. B Biol. Sci. 375:20190060. doi: 10.1098/rstb.2019.0060
Adam, I., and Elemans, C. P. H. (2020). Increasing muscle speed drives changes in the neuromuscular transform of motor vommands during postnatal development in songbirds. J. Neurosci. 40, 6722–6731. doi: 10.1523/JNEUROSCI.0111-20.2020
Alliende, J. A., Méndez, J. M., Goller, F., and Mindlin, G. B. (2010). Hormonal acceleration of song development illuminates motor control mechanism in Canaries. Dev. Neurobiol. 70, 943–960. doi: 10.1002/dneu.20835
Amador, A., Goller, F., and Mindlin, G. B. (2008). Frequency modulation during song in a suboscine does not require vocal muscles. J. Neurophysiol. 99, 2383–2389. doi: 10.1152/jn.01002.2007
Anderson, M. G., Brunton, D. H., and Hauber, M. E. (2010). Reliable information content and ontogenetic shift in begging calls of Grey Warbler nestlings. Ethology 116, 357–365. doi: 10.1111/j.1439-0310.2010.01750.x
Araya-Salas, M., and Wright, T. (2013). Open-ended song learning in a Hummingbird. Biol. Lett. 9:20130625. doi: 10.1098/rsbl.2013.0625
Arriaga, G., and Jarvis, E. D. (2013). Mouse vocal communication system: are ultrasounds learned or innate? Brain Lang. 124, 96–116. doi: 10.1016/j.bandl.2012.10.002
Arriaga, G., Zhou, E. P., and Jarvis, E. D. (2012). Of mice, birds, and men: the mouse ultrasonic song system has some features similar to humans and song-learning birds. PLoS One 7:e46610. doi: 10.1371/journal.pone.0046610
Ashmore, R. C., Wild, J. M., and Schmidt, M. F. (2005). Brainstem and forebrain contributions to the generation of learned motor behaviors for song. J. Neurosci. 25, 8543–8554. doi: 10.1523/JNEUROSCI.1668-05.2005
Balsby, T. J. S., Momberg, J. V., and Dabelsteen, T. (2012). Vocal imitation in parrots allows addressing of specific individuals in a dynamic communication network. PLoS One 7:e49747. doi: 10.1371/journal.pone.0049747
Beme, I. R. (2006). Formation of passerine (Passeriformes) vocalization in ontogeny: the current state of the problem. Zhurnal Obshchei Biologii 67, 268–279.
Brainard, M. S., and Doupe, A. J. (2002). What songbirds teach us about learning. Nature 417, 351–358. doi: 10.1038/417351a
Brockway, B. F. (1964a). Ethological studies of the budgerigar (Melopsittacus undulatus): non-reproductive behavior. Behaviour 22, 193–222.
Brockway, B. F. (1964b). Ethological studies of the budgerigar: reproductive behavior. Behaviour 23, 294–324.
Brunton, D. H., Roper, M. M., and Harmer, A. M. T. (2016). Female song rate and structure predict reproductive success in a socially monogamous bird. Front. Ecol. Evol. 4:13. doi: 10.3389/fevo.2016.00013
Cain, K. E., and Langmore, N. E. (2015). Female and male song rates across breeding stage: testing for sexual and nonsexual functions of female song. Anim. Behav. 109, 65–71. doi: 10.1016/j.anbehav.2015.07.034
Capelli, D., Batalha-Filho, H., and Japyassú, H. F. (2020). Song variation in the Caatinga suboscine Silvery-cheeked Antshrike (Sakesphorus cristatus) suggests latitude and São Francisco River as drivers of geographic variation. J. Ornithol. 161, 873–884. doi: 10.1007/s10336-020-01779-4
Choe, H. N., and Jarvis, E. D. (2021). The role of sex chromosomes and sex hormones in vocal learning systems. Horm. Behav. 132:104978.
Creanza, N., Fogarty, L., and Feldman, M. W. (2016). Cultural niche construction of repertoire size and learning strategies in songbirds. Evol. Ecol. 30, 285–305. doi: 10.1007/s10682-015-9796-1
Dittus, W. P. J., and Lemon, R. E. (1969). Effects of song tutoring and acoustic isolation on the song repertoires of Cardinals. Anim. Behav. 17(Pt 3), 523–533. doi: 10.1016/0003-3472(69)90157-2
Doupe, A. J. (1997). Song- and order-selective neurons in the songbird anterior forebrain and their emergence during vocal development. J. Neurosci. 17, 1147–1167. doi: 10.1523/jneurosci.17-03-01147.1997
Düring, D. N., and Elemans, C. P. H. (2016). “Embodied motor control of avian vocal production,” in Vertebrate Sound Production and Acoustic Communication, Vol. 53, eds R. Suthers, W. Fitch, R. Fay, and A. Popper (Cham: Springer). doi: 10.1007/978-3-319-27721-9_5
Eda-Fujiwara, H., Watanabe, A., and Okumura, H. (1995). Effects of deafening on the temporal pattern of vocalizations in the Budgerigar Melopsittacus undulatus. J. Ethol. 13, 145–152. doi: 10.1007/BF02350105
Evans, C., and Kleindorfer, S. (2016). Superb fairy-wren (Malurus cyaneus) sons and daughters acquire song elements of mothers and social fathers. Front. Ecol. Evol. 4:9. doi: 10.3389/fevo.2016.00009
Fee, M., Kozhevnikov, A., and Hahnloser, R. (2004). Neural mechanisms of vocal sequence: generation in the songbird. Ann. N. Y. Acad. Sci. 1016, 153–170. doi: 10.1196/annals.1298.022
Fehér, O., Wang, H., Saar, S., Mitra, P. P., and Tchernichovski, O. (2009). De novo establishment of wild-type song culture in the Zebra Finch. Nature 459, 564–568. doi: 10.1038/nature07994
Freeman, B. G., Montgomery, G. A., and Schluter, D. (2017). Evolution and plasticity: divergence of song discrimination is faster in birds with innate song than in song learners in Neotropical passerine birds. Evolution 71, 2230–2242. doi: 10.1111/evo.13311
Garcia, S. M., Kopuchian, C., Mindlin, G. B., Fuxjager, M. J., Tubaro, P. L., and Goller, F. (2017). Evolution of vocal diversity through morphological adaptation without vocal learning or complex neural control. Curr. Biol. 27, 2677–2683.
Goldman, S. A., and Nottebohm, F. (1983). Neuronal production, migration, and differentiation in a vocal control nucleus of the adult female Canary brain. Proc. Natl. Acad. Sci. U.S.A. 80, 2390–2394. doi: 10.1073/pnas.80.8.2390
Goller, M., and Shizuka, D. (2018). Evolutionary origins of vocal mimicry in songbirds. Evol. Lett. 2, 417–426. doi: 10.1002/evl3.62
Jarvis, E. D. (2006). Selection for and against vocal learning in birds and mammals. Ornithol. Sci. 5, 5–14. doi: 10.2326/osj.5.5
Jarvis, E. D. (2019). Evolution of vocal learning and spoken language. Science 366, 50–54. doi: 10.1126/science.aax0287
Kiefer, S., Spiess, A., Kipper, S., Mundry, R., Sommer, C., Hultsch, H., et al. (2006). First-year common nightingales (Luscinia megarhynchos) have smaller song-type repertoire sizes than older males. Ethology 112, 1217–1224. doi: 10.1111/j.1439-0310.2006.01283.x
Klenova, A. V., Goncharova, M. V., Bragina, E. V., and Kashentseva, T. A. (2014). Vocal development and voice breaking in Demoiselle Cranes (Anthropoides virgo). Bioacoustics 23, 247–265. doi: 10.1080/09524622.2014.900648
Klenova, A. V., Goncharova, M. V., Kashentseva, T. A., and Naidenko, S. V. (2020). Voice breaking and its relation to body mass and testosterone level in the Siberian Crane (Leucogeranus leucogeranus). J. Ornithol. 161, 859–871. doi: 10.1007/s10336-020-01773-w
Klenova, A. V., Volodin, I. A., Volodina, E. V., and Postelnykh, K. A. (2010). Voice breaking in adolescent red-crowned cranes (Grus japonensis). Behaviour 147, 505–524. doi: 10.1163/000579509X12591315521811
Kroodsma, D. E., Hamilton, D., Sánchez, J. E., Byers, B. E., Fandiño-Mariño, H., Stemple, D. W., et al. (2013). Behavioral evidence for song learning in the suboscine Bellbirds (Procnias spp.; Cotingidae). Wilson J. Ornithol. 125, 1–14. doi: 10.1017/CBO9781107415324.004
Kroodsma, D. E., and Konishi, M. (1991). A suboscine bird (Eastern Phoebe, Sayornis phoebe) develops normal song without auditory feedback. Anim. Behav. 42, 477–487. doi: 10.1016/S0003-3472(05)80047-8
Kuhl, H., Frankl-Vilches, C., Bakker, A., Mayr, G., Nikolaus, G., Boerno, S. T., et al. (2020). An unbiased molecular approach using 3’UTRs resolves the avian family-level tree of life. Mol. Biol. Evol. 38, 108–127. doi: 10.1093/molbev/msaa191
Labra, A., and Lampe, H. M. (2018). The songs of male Pied Flycatchers: exploring the legacy of the fathers. PeerJ 6:e5397. doi: 10.7717/peerj.5397
Langmore, N. E. (1998). Functions of duet and solo songs of female birds. Trends Ecol. Evol. 13, 136–140.
Leger, D. W. (2005). First documentation of combinatorial song syntax in a suboscine passerine species. Condor 107:765. doi: 10.1650/7851.1
Liu, W. C., Rivers, J. W., and White, D. J. (2016). Vocal matching and intensity of begging calls are associated with a forebrain song circuit in a generalist brood parasite. Dev. Neurobiol. 76, 615–625. doi: 10.1002/dneu.22348
Liu, W. C., Wada, K., Jarvis, E., and Nottebohm, F. (2013). Rudimentary substrates for vocal learning in a suboscine. Nat. Commun. 4:2082. doi: 10.1038/ncomms3082
Liu, W. C., Wada, K., and Nottebohm, F. (2009). Variable food begging calls are harbingers of vocal learning. PLoS One 4:e5929. doi: 10.1371/journal.pone.0005929
Livingston, F. S., and Mooney, R. (2001). Androgens and isolation from adult tutors differentially affect the development of songbird neurons critical to vocal plasticity. J. Neurophysiol. 85, 34–42. doi: 10.1152/jn.2001.85.1.34
London, S. E. (2019). Developmental song learning as a model to understand neural mechanisms that limit and promote the ability to learn. Behav. Processes 163, 13–23. doi: 10.1016/j.beproc.2017.11.008
Love, J., Hoepfner, A., and Goller, F. (2019). Song feature specific analysis of isolate song reveals interspecific variation in learned components. Dev. Neurobiol. 79, 350–369. doi: 10.1002/dneu.22682
Lovell, S. F., and Lein, M. R. (2004). Song variation in a population of Alder Flycatchers. J. Field Ornithol. 75, 146–151.
Martins, P. T., and Boeckx, C. (2020). Vocal learning: beyond the continuum. PLoS Biol. 18: e3000672. doi: 10.1371/JOURNAL.PBIO.3000672
Mason, N. A., Burns, K. J., Tobias, J. A., Claramunt, S., Seddon, N., and Derryberry, E. P. (2017). Song evolution, speciation, and vocal learning in passerine birds. Evolution 71, 786–796. doi: 10.1111/evo.13159
Matsunaga, E., and Okanoya, K. (2009). Evolution and diversity in avian vocal system: an Evo-Devo model from the morphological and behavioral perspectives. Dev. Growth Differ. 51, 355–367. doi: 10.1111/j.1440-169X.2009.01091.x
Maxwell, A., Adam, I., Larsen, P. S., Sørensen, P. G., and Elemans, C. P. H. (2021). Syringeal vocal folds do not have a voice in Zebra Finch vocal development. Sci. Rep. 11:6469. doi: 10.1038/s41598-021-85929-5
Mennill, D. J., Doucet, S. M., Newman, A. E. M., Williams, H., Moran, I. G., Thomas, I. P., et al. (2018). Wild birds learn songs from experimental vocal tutors. Curr. Biol. 28, 3273–3278.e4. doi: 10.1016/j.cub.2018.08.011
Mennill, D. J., Doucet, S. M., Newman, A. E. M., Williams, H., Moran, I. G., Thomas, I. P., et al. (2019). Eavesdropping on adult vocal interactions does not enhance juvenile song learning: an experiment with wild songbirds. Anim. Behav. 155, 67–75. doi: 10.1016/j.anbehav.2019.05.019
Mooney, R. (2009). Birdsong: the neurobiology of avian vocal learning. Encycl. Neurosci. 2009, 247–251. doi: 10.1016/B978-008045046-9.01942-2
Nelson, D. A., Marler, P., and Palleroni, A. (1995). A comparative approach to vocal learning: intraspecific variation in the learning process. Anim. Behav. 50, 83–97. doi: 10.1006/anbe.1995.0223
Nottebohm, F. (1970). Ontogeny of bird song. Science 167, 950–956. doi: 10.1126/science.167.3920.950
Nottebohm, F. (1992). The search for neural mechanisms that define the sensitive period for song learning in birds. Neth. J. Zool. 43, 193–234. doi: 10.1163/156854293X00296
Odom, K. J., and Benedict, L. (2018). A call to document female bird songs: applications for diverse fields. Auk 135, 314–325. doi: 10.1642/AUK-17-183.1
Odom, K. J., Hall, M. L., Riebel, K., Omland, K. E., and Langmore, N. E. (2014). Female song is widespread and ancestral in songbirds. Nat. Commun. 5:3379. doi: 10.1038/ncomms4379
Oliveros, C. H., Field, D. J., Ksepka, D. T., Keith Barker, F., Aleixo, A., Andersen, M. J., et al. (2019). Earth history and the passerine superradiation. Proc. Natl. Acad. Sci. U.S.A. 116, 7916–7925. doi: 10.1073/pnas.1813206116
Park, K. H. J., Meitzen, J., Moore, I. T., Brenowitz, E. A., and Perkel, D. J. (2005). Seasonal-like plasticity of spontaneous firing rate in a songbird pre-motor nucleus. J. Neurobiol. 64, 181–191. doi: 10.1002/neu.20145
Petkov, C. I., and Jarvis, E. D. (2012). Birds, primates, and spoken language origins: behavioral phenotypes and neurobiological substrates. Front. Evol. Neurosci. 4:12. doi: 10.3389/fnevo.2012.00012
Raikow, R. J., and Bledsoe, A. H. (2000). Phylogeny and evolution of the passerine birds. BioScience 50, 487–499.
Ranjard, L., Anderson, M. G., Rayner, M. J., Payne, R. B., McLean, I., Briskie, J. V., et al. (2010). Bioacoustic distances between the begging calls of brood parasites and their host species: a comparison of metrics and techniques. Behav. Ecol. Sociobiol. 64, 1915–1926. doi: 10.1007/s00265-010-1065-2
Reichard, D. G., Brothers, D. E., George, S. E., Atwell, J. W., and Ketterson, E. D. (2018). Female dark-eyed Juncos Junco hyemalis thurberi produce male-like song in a territorial context during the early breeding season. J. Avian Biol. 49, 1–6. doi: 10.1111/jav.01566
Riebel, K., Hall, M., and Langmore, N. (2005). Female songbirds still struggling to be heard. Trends Ecol. Evol. 20, 419–420. doi: 10.1016/j.tree.2005.04.013
Rivera-Cáceres, K. D., and Templeton, C. N. (2019). A duetting perspective on avian song learning. Behav. Processes 163, 71–80. doi: 10.1016/j.beproc.2017.12.007
Robinson, C. M., Snyder, K. T., and Creanza, N. (2019). Correlated evolution between repertoire size and song plasticity predicts that sexual selection on song promotes open-ended learning. Elife 8:e44454. doi: 10.7554/eLife.44454
Roper, M. M., Harmer, A. M. T., and Brunton, D. H. (2018). Developmental changes in song production in free-living male and female New Zealand Bellbirds. Anim. Behav. 140, 57–71. doi: 10.1016/j.anbehav.2018.04.003
Saranathan, V., Hamilton, D., Powell, G. V. N., Kroodsma, D. E., and Prum, R. O. (2007). Genetic evidence supports song learning in the three-wattled Bellbird Procnias tricarunculata (Cotingidae). Mol. Ecol. 16, 3689–3702. doi: 10.1111/j.1365-294X.2007.03415.x
Sawhney, M. H., Baker, M. C., and Bisbee, B. R. (2006). Development of vocalizations in nestling and fledgling House Wrens in natural populations. Bioacoustics 15, 271–287. doi: 10.1080/09524622.2006.9753554
Seddon, N. (2005). Ecological adaptation and species recognition drives vocal evolution in neotropical suboscine birds. Evolution 59, 200–215. doi: 10.1111/j.0014-3820.2005.tb00906.x
Sierro, J., de Kort, S. R., Riebel, K., and Hartley, I. R. (2021). Frequent female song in Blue Tits: behavioural context suggests a role in intrasexual competition. bioRxiv [Preprint]. doi: 10.1101/2021.07.01.450672
Simpson, H. B., and Vicario, D. S. (1990). Brain pathways for learned and unlearned vocalizations differ in zebra finches. J. Neurosci. 10, 1541–1556. doi: 10.1523/JNEUROSCI.10-05-01541.1990
Tyack, P. L. (2019). A taxonomy for vocal learning. Philos. Trans. R. Soc. B Biol. Sci. 375:20180406. doi: 10.1098/rstb.2018.0406
Vernes, S. C., Kriengwatana, B. P., Beeck, V. C., Fischer, J., Tyack, P. L., Ten Cate, C., et al. (2021). The multi-dimensional nature of vocal learning. Philos. Trans. R. Soc. B 376:20200236. doi: 10.1098/rstb.2020.0236
Whaling, C. S. S., Nelson, D. A., and Marler, P. (1995). Testosterone−induced shortening of the storage phase of song development in birds interferes with vocal learning. Dev. Psychobiol. 28, 367–376. doi: 10.1002/dev.420280703
Wilkins, M. R., Odom, K. J., Benedict, L., and Safran, R. J. (2020). Analysis of female song provides insight into the evolution of sex differences in a widely studied songbird. Anim. Behav. 168, 69-82. doi: 10.1101/2020.03.28.013433
Wirthlin, M., Chang, E. F., Knörnschild, M., Krubitzer, L. A., Mello, C. V., Miller, C. T., et al. (2019). A modular approach to vocal learning: disentangling the diversity of a complex behavioral trait. Neuron 104, 87–99. doi: 10.1016/j.neuron.2019.09.036
Wirthlin, M., Lima, N. C. B., Guedes, R. L. M., Soares, A. E. R., Almeida, L. G. P., Cavaleiro, N. P., et al. (2018). Parrot genomes and the evolution of heightened longevity and cognition. Curr. Biol. 28, 4001–4008.e7. doi: 10.1016/j.cub.2018.10.050
Wright, T. F., and Derryberry, E. P. (2021). Defining the multidimensional phenotype: new opportunities to integrate the behavioral ecology and behavioral neuroscience of vocal learning. Neurosci. Biobehav. Rev. 125, 328–338. doi: 10.1016/j.neubiorev.2021.02.022
Keywords: vocal learning, life history, songbirds, passeriformes, vocal development
Citation: Loo YY and Cain KE (2021) A Call to Expand Avian Vocal Development Research. Front. Ecol. Evol. 9:757972. doi: 10.3389/fevo.2021.757972
Received: 01 September 2021; Accepted: 11 October 2021;
Published: 01 November 2021.
Edited by:
Patricia Izar, University of São Paulo, BrazilReviewed by:
Nicolas Giret, UMR 9197 Institut des Neurosciences Paris Saclay (Neuro-PSI), FranceErich David Jarvis, Duke University, United States
Gabriel Mindlin, University of Buenos Aires, Argentina
Copyright © 2021 Loo and Cain. This is an open-access article distributed under the terms of the Creative Commons Attribution License (CC BY). The use, distribution or reproduction in other forums is permitted, provided the original author(s) and the copyright owner(s) are credited and that the original publication in this journal is cited, in accordance with accepted academic practice. No use, distribution or reproduction is permitted which does not comply with these terms.
*Correspondence: Yen Yi Loo, looyenyi@gmail.com