- State Key Laboratory of Grassland Agro-Ecosystems, Key Laboratory of Grassland Livestock Industry Innovation, Ministry of Agriculture and Rural Affairs, College of Pastoral Agriculture Science and Technology, Lanzhou University, Lanzhou, China
Plant interactions are central in driving the composition and structure of plant populations and communities. Soil heterogeneity and species identity can modulate such interactions, yet require more studies. Thus, a manipulative experiment was done where three soil heterogeneity levels were developed by mixing local soil and sand in three different ratios (i.e., soil:sand ratio = 2:8, 5:5, and 8:2), and three typical species (i.e., Festuca elata, Bromus inermis, and Elymus breviaristatus) were used in different combinations. Soil heterogeneity was assumed to affect plant interactions, which were in turn modified by species. Plant height was applied as an indicator for plant interactions. Relative competition intensity (RCI) was used to quantify plant interactions, where RCI was applied as a ratio of monoculture and mixture performance. Results showed that soil heterogeneity and soil heterogeneity × species significantly affected the RCI in mixtures compared with plant individuals growing alone (i.e., RCI1). However, species as a single factor did not affect RCI1. Moreover, species and soil heterogeneity × species significantly affected the RCI in mixtures compared with two individuals growing together (i.e., RCI2), and the difference between RCI1 and RCI2 (i.e., RCIdiff). Soil heterogeneity significantly affected RCI2 of F. elata. This study suggests that soil heterogeneity could buffer the stability of plant populations by modifying plant interactions, which would subsequently drive plant establishment. To explore the underlying mechanisms of such patterns, further studies considering more species and plant traits are needed.
Introduction
Plant interactions play an important role in shaping the composition and structure of plant populations and communities (Baer et al., 2003; Rajaniemi et al., 2009; Wassmuth et al., 2009; Cahill and McNickle, 2011; Houseman, 2014; Zhang et al., 2020). Numerous studies have explored plant interactions (Robinson et al., 1999; O’Brien et al., 2007; Allesina and Levine, 2011; Roiloa et al., 2014; Ravenek et al., 2016; Fichtner et al., 2018; Garlick et al., 2021), yet, several factors impede researchers to exactly quantify such interactions. For example, it is difficult to connect the cause and effect of plant interactions in natural communities with multiple species growing together, where many other factors such as climate change, disturbance, and natural heterogeneity may affect the results (Ravenek et al., 2016; Liu et al., 2019). Previous controlled studies often explored plant interactions in pairs for simplification (Johansson and Keddy, 1991; Chesson, 2000; Day et al., 2003; Rajaniemi, 2011; Li et al., 2018). However, plant individuals interact not only in pairs but are also affected by higher-order patterns (i.e., the interactions between two species are likely to be modified by other species, Pierik et al., 2013; Grilli et al., 2017; Levine et al., 2017; Fichtner et al., 2018; Li et al., 2020, 2021; Chu et al., 2021). Thus, to quantify plant interactions more accurately, it is necessary to quantify the differences between higher-order interactions and pairwise interactions.
Natural soils are heterogeneous, and this soil heterogeneity has two components, namely, qualitative heterogeneity and configurational heterogeneity (Kelly and Canham, 1992; Maestre and Cortina, 2002; Wijesinghe et al., 2005; Maestre and Reynolds, 2007). The former refers to the variations of texture, nutrients, pH, etc., between patches in the soils, while the latter reflects the size of these patches (Dufour et al., 2006). Soil heterogeneity in this study refers to the qualitative heterogeneity. Qualitative heterogeneity affects plant interactions by altering the availability of soil resources such as water and nutrients (Fransen et al., 2001; Schenk, 2006; Zhang et al., 2020).
Plants growing in a population or a community interact with each other (Chesson, 2000; Levine et al., 2017; Garlick et al., 2021). However, how to quantify such interactions is a hot topic in ecology. Many parameters can be applied to quantify plants interactions (Weigelt and Jolliffe, 2003), where relative competition intensity (RCI) is a widely used one. Previous studies found that plant interactions vary with species identity (Catorci et al., 2011; Raath-Krüger et al., 2019) and their growing stages (Yang and Rudolf, 2010; Li et al., 2019). Plant interaction at the early growing stage is crucial for plant establishment in plant populations and communities (Lortie and Turkington, 2008; Hart et al., 2018), and warrants more research. Plant height is a good indicator of plant interactions since it plays a vital role in determining light interception (Xiao et al., 2007), and thus, it is widely applied to quantify plant interactions non-destructively (Cui et al., 2004; Chen and Nelson, 2006; Xiao et al., 2007).
To explore the effects of soil heterogeneity and species identity on plant interactions, a controlled experiment was conducted, where three levels of soil heterogeneity were developed by mixing local soil and sand in three different ratios (i.e., soil:sand ratio = 2:8, 5:5, and 8:2), and three typical forage grasses were used (Festuca elata, Bromus inermis, and Elymus breviaristatus). RCI was applied to quantify plant interactions, where RCI was calculated as a direct ratio of monoculture and mixture performance, and positive (or negative) values simply indicated that a plant individual experiences stronger (or weaker) competition in mixtures compared with growing alone (Weigelt and Jolliffe, 2003). Specifically, we put forward three hypotheses: (i) RCI is expected to increase with increasing soil heterogeneity, where higher soil heterogeneity could reduce plant interactions due to an increase in the available resources at higher soil heterogeneity levels (Levine et al., 2017; Liu et al., 2017b); (ii) Such patterns may be modified by plant species identity since responses to soil heterogeneity can be modified via strategies or traits such as plasticity (Hodge, 2004; Craine and Dybzinski, 2013); (iii) Soil heterogeneity and species are assumed to jointly affect RCI since plant responses to soil resources depend on their neighbor identity and the distribution pattern of soil resources (Mommer et al., 2012; Garlick et al., 2021).
Materials and Methods
Experimental Design
This experiment was conducted at Linze Grassland Agriculture Trial Station of Lanzhou University (m.a.s.l. 1,400 m, 100°3′25″E, 39°14′30″N), in Gansu Province, China. This station is located in the middle of the Hexi Corridor (Hou and Shen, 1999). It is characterized by a temperate continental arid monsoon climate. The mean annual temperature and mean annual precipitation are 9.3°C and 112.9 mm, respectively. Temperatures range between extremes of 3°C and −28°C, and more than 60% of the rainfall occurs in summer and autumn, while the mean annual evaporation is 2,338 mm. The natural soils are saline due to the large difference between rainfall and evaporation (Zhu et al., 1997).
To explore the effects of soil heterogeneity (i.e., soil–sand ratio in this case) and species identity on plant interactions (i.e., quantified by RCI), a controlled experiment was conducted (Figure 1). Three levels of soil–sand ratio (i.e., soil:sand = 2:8, 5:5, and 8:2) were applied (details of these soil–sand ratios can be found in Table 1), where the sand was bought from a local commercial company, while the soil was collected from the local crop field. Three typical forage grasses were used (i.e., F. elata, B. inermis, and E. breviaristatus). These species were selected as they are dominant in the grasslands of China, and they differ both in their adaptation to saline soils and in their phenology, which should yield some growth divergence in our experimental treatments. Seeds of these species were bought from a commercial company (BEST, Beijing, China). On May 31, 2021, they were separately sowed into three identical trays with the same soils collected from the nearby field. Pots of 17.5 cm height, with a 16 cm top diameter and 13 cm bottom diameter were filled with one of the three types of soil–sand ratios. Seedlings of similar size were transplanted into the pots 1 week after sowing using three patterns (i.e., one, two, or three individuals). There were five replications of each treatment. Six holes with a 10-mm diameter were drilled in the bottom of each pot to ensure adequate drainage of water. Pots were watered evenly using a hose (0.4 L per pot per day), avoiding water runoff at the soil surface.
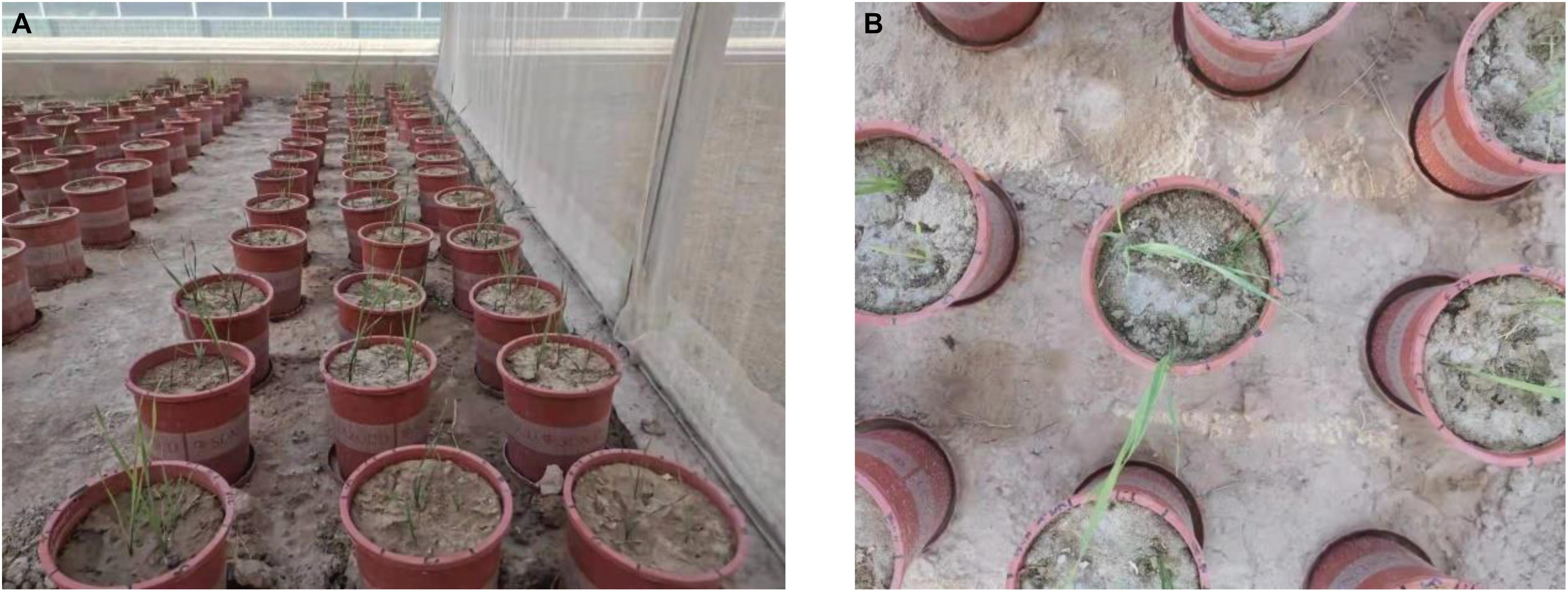
Figure 1. Set-up of experiment (A). All pots were randomly distributed in three separate rooms, which have the same condition. Top view of a pot in this experiment (B).
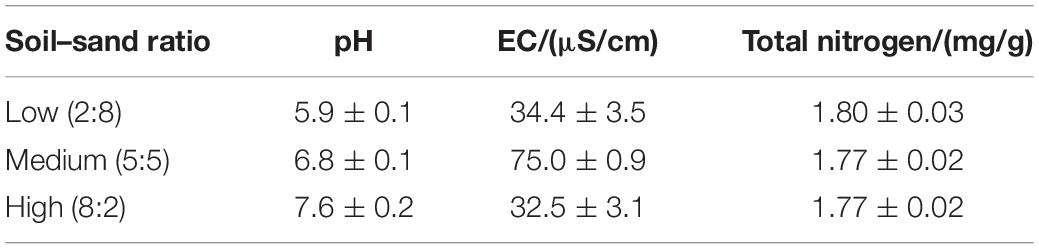
Table 1. Mean ± SE of pH, electrical conductivity (EC), and total nitrogen of the three soil–sand ratios tested at the beginning of the experiment.
The plant height of each individual in each pot was measured 1 month after transplanting (details of plant height growing in different patterns can be found in Appendix Figures 1–3). RCI was calculated to quantify the plant interactions in mixtures with two and three individuals growing together.
Data Analysis and Statistics
No competition exists in pots with one individual, while only direct interaction occurred in pots with two individuals. However, both direct and indirect interactions appeared in pots with three individuals. To separate and quantify the indirect interaction in pots with three individuals, two ways could be applied to calculate plant interactions in this mixture. One is by comparing three individuals growing together with one individual, and the other is by comparing three individuals growing together with two individuals growing together (Figure 2).
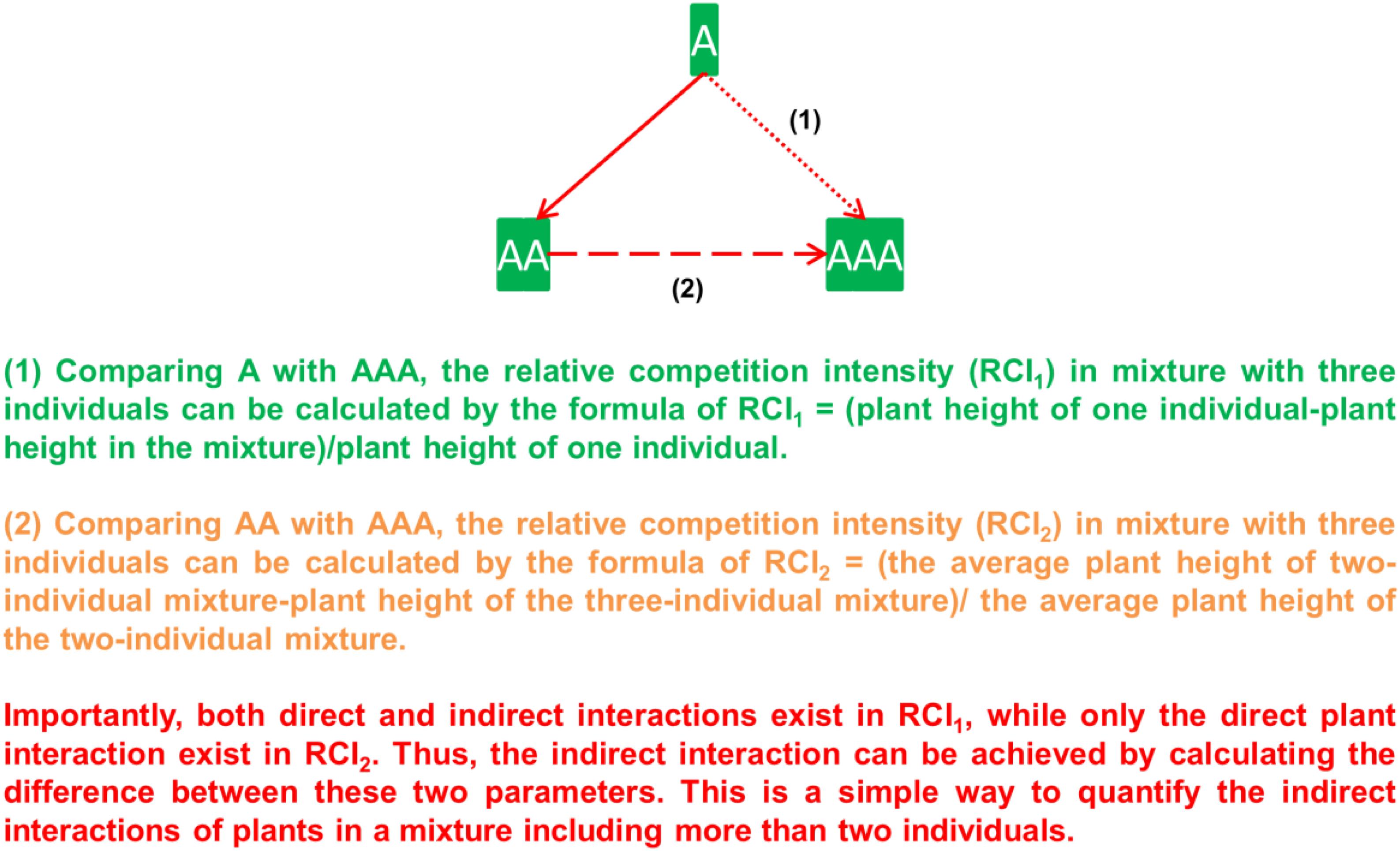
Figure 2. Diagram of the calculation of relative competition intensity (RCI) in two different ways, where Festuca elata (labeled as A) is used as an example, and it grows either along (i.e., A), or with a neighbor (i.e., AA) or with two neighbors (i.e., AAA). (1) Comparing A with AAA, RCI with neighbors can be calculated as RCI1, which includes both direct and indirect interactions. (2) Comparing AA with AAA, RCI with two neighbors can be calculated as RCI2, which includes only direct interaction. As a result, the indirect interaction can be calculated by the difference between RCI1 and RCI.
(1) By comparing with plant individuals growing alone, plant interactions in pots with three individuals can be calculated as RCI1 = (plant height in pots with one individual – plant height in pots with three individuals)/plant height in pots with one individual. The same calculation is used to quantify plant interactions in pots with two individuals.
(2) By comparing with two individuals growing together, plant interactions in pots with three individuals can be calculated: RCI2 = (the average plant height in pots with two individuals – plant height in pots with three individuals) the average plant height in pots with two individuals.
RCI1 in pots with three individuals includes both direct and indirect interactions. However, RCI2 in pots with three individuals can be viewed as only including direct interactions. This is similar to comparing two individuals growing together with one individual growing alone. The only difference is that the two individuals were grouped in formula (2). As a result, the indirect interaction in pots with three individuals (labeled as RCIdiff) can be calculated by the following formula:
To explore the effects of soil–sand ratio (i.e., low, medium, and high), species (i.e., F. elata, B. inermis, and E. breviaristatus), and the number of individuals (i.e., two or three individuals in a pot) and their interactions on the RCI1, ANOVA were conducted. To investigate the effects of soil–sand ratio, species, number of individuals, and their interactions on RCI2 and RCIdiff, ANOVAs were performed. Results show that species identity was a significant factor. Thus, RCI2 and RCIdiff of each species were analyzed separately, with soil–sand ratio and their interaction as fixed factors. Note that plant individuals in a mixture with two or three individuals growing together were treated as an extra factor as these individuals may perform differently. Post hoc analyses (pairwise comparisons with Bonferroni corrections) were carried out in these analyses when differences among the variables were significant. Log transformations were performed when necessary. All statistics were carried out with SPSS 21.0.
Note that biomass was not measured at this stage since doing so would impact plant growth at the following stages. However, measuring plant height was non-destructive, making it a reasonable parameter for exploring plant interactions at the early growing stage of plants.
Results
Soil–sand ratio and species × soil–sand ratio significantly affected RCI1 (Table 2 and Figure 3). For mixtures with two individuals, RCI1 of F. elata and B. inermis was highest at the medium soil–sand ratio, while RCI1 of E. breviaristatus was highest at high soil–sand ratio (Figure 3A). For mixtures with three individuals, similar patterns were found (Figure 3B). However, species as a single factor did not affect RCI1 (Table 2).
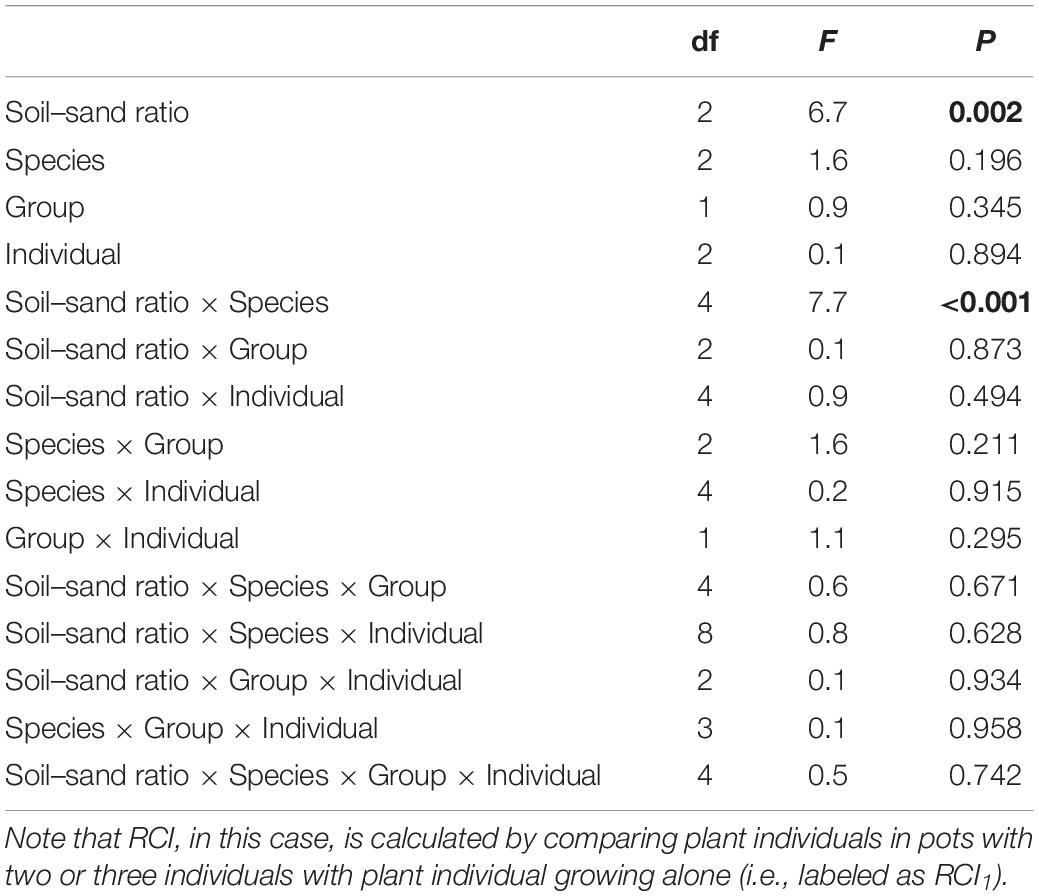
Table 2. Effects of soil–sand ratio (i.e., low, medium, or high), species (i.e., Festuca elata, Bromus inermis, and Elymus breviaristatus), group (two or three individuals in a pot), and individual and their interactions on the relative competition intensity (RCI) in ANOVA, where F-values, degree of freedom (df), and P-value are given, and significant results (P < 0.05) are labeled in bold.
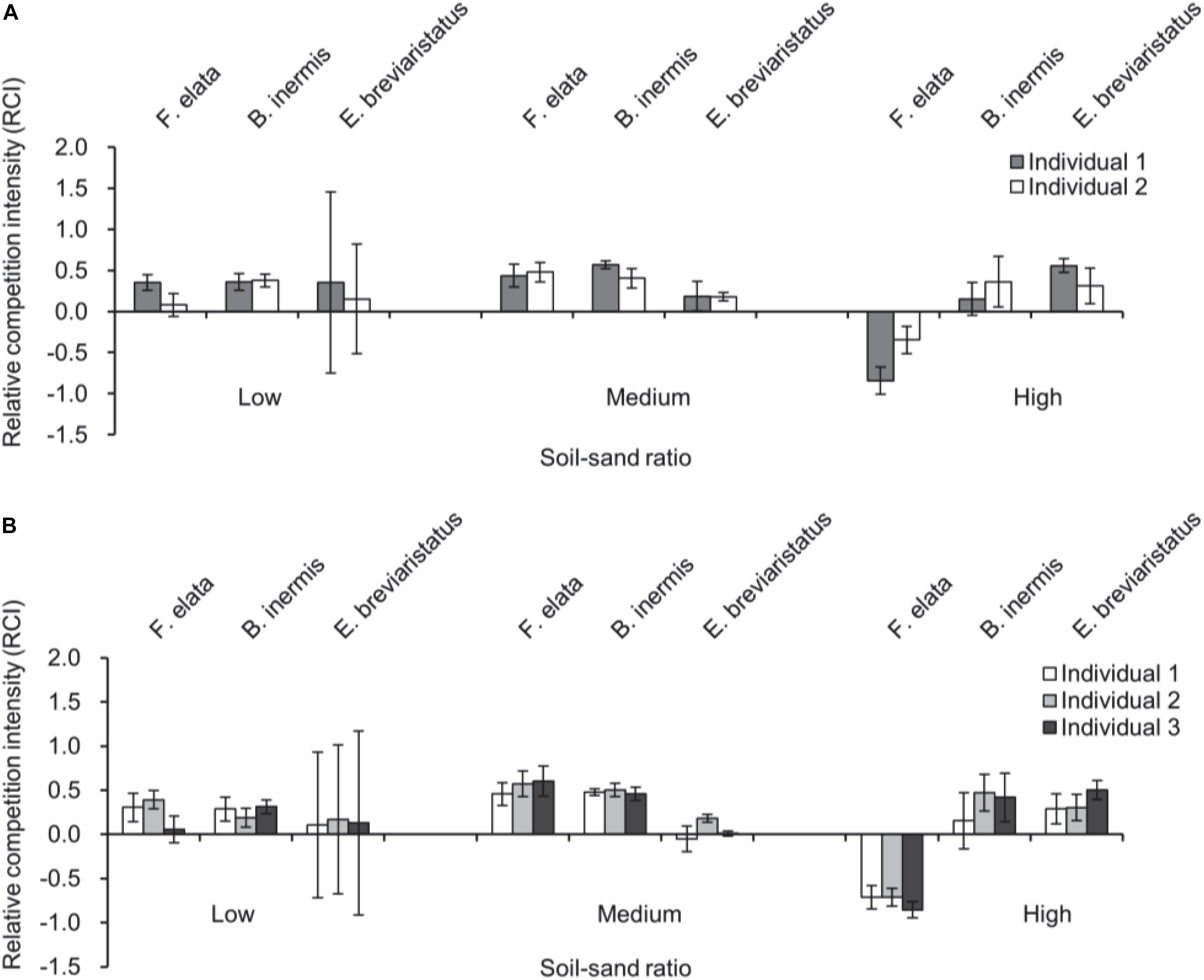
Figure 3. Relative competition intensity of plant individuals in pots with two individuals (A) and three individuals of the three target plant species (i.e., Festuca elata, Bromus inermis, and Elymus breviaristatus) along with soil–sand ratio (i.e., low, medium, and high). Note that RCI, in this case, is calculated by comparing plant individuals in pots with two individuals (A) or three individuals (B) with plant individuals growing along (i.e., RCI1).
Species, soil–sand ratio × species significantly affected RCI2 (Table 3 and Figure 4A). Specifically, RCI2 of F. elata was higher in medium soil–sand ratio, while RCI2 of B. inermis and E. breviaristatus increased with increasing soil–sand ratios (Figure 4A). However, soil–sand ratio as a single factor did not affect RCI2 (Table 3). The separated analyses demonstrated that soil–sand ratios significantly affected RCI2 of F. elata (Table 4). Specifically, RCI2 of F. elata was higher at the medium soil–sand ratio, while RCI2 of B. inermis and E. breviaristatus increased with increasing soil–sand ratios (Figure 4B).
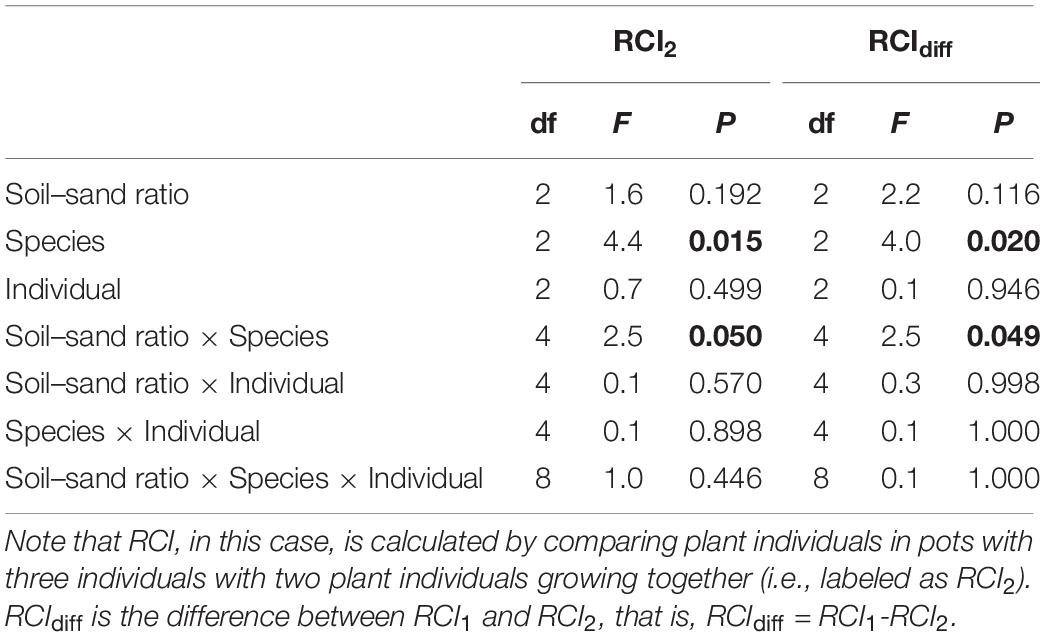
Table 3. Effects of soil–sand ratio (i.e., low, medium, or high), species (i.e., Festuca elata, Bromus inermis, and Elymus breviaristatus), and individual and their interactions on the relative competition intensity (RCI) in ANOVA, where F-values, degree of freedom (df), and P-value are given, and significant results (P < 0.05) are labeled in bold.
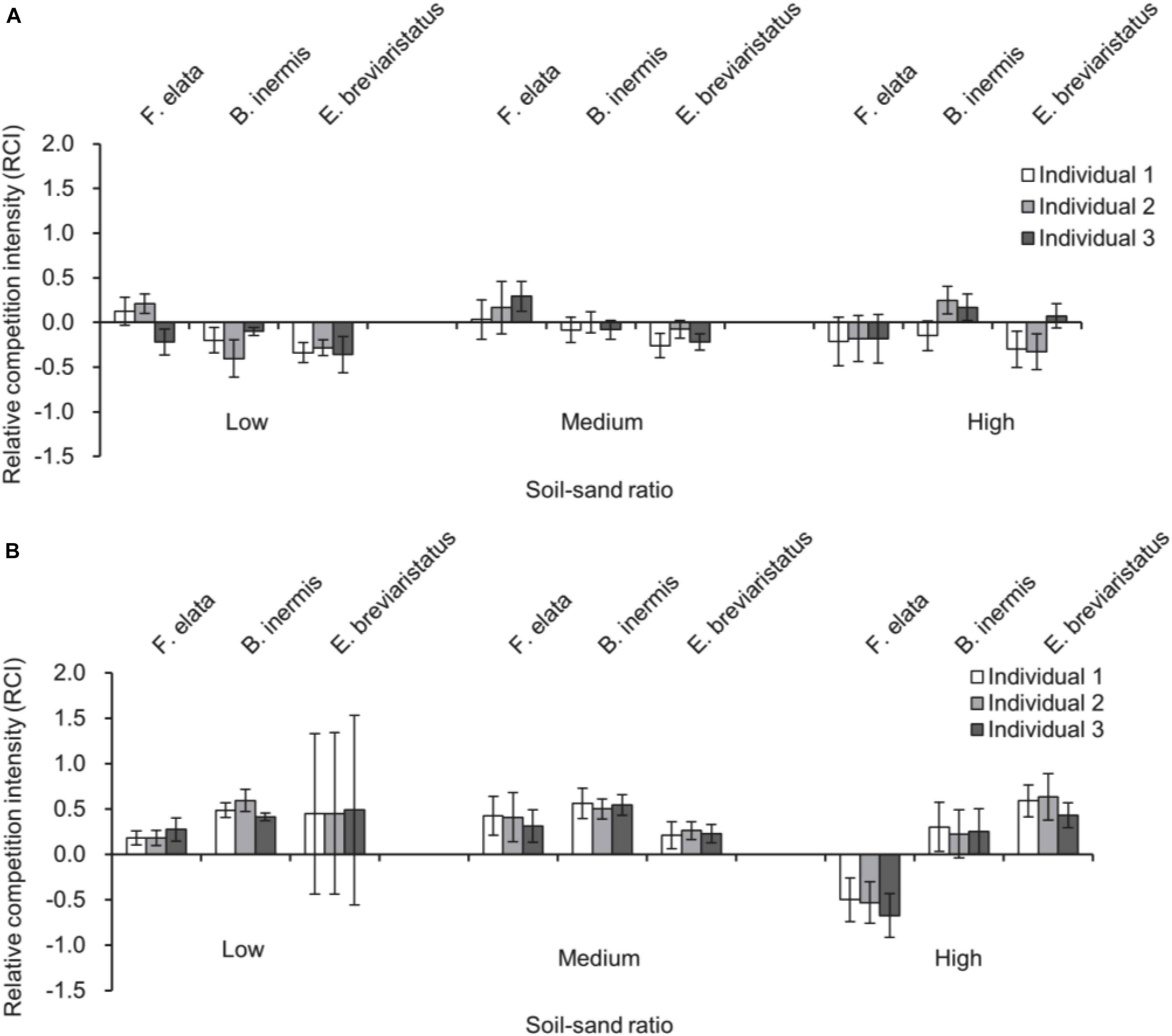
Figure 4. Relative competition intensity of plant individuals in pots with three individuals (A) and the differences between this RCI and the one in pots with three individuals in (B) of the three target plant species (i.e., Festuca elata, Bromus inermis, and Elymus breviaristatus) along with soil–sand ratio (i.e., low, medium, and high). Note that RCI, in this case, is calculated by comparing plants in pots with three individuals with plants in pots with two individuals (i.e., RCI2, A), and the difference between them is shown in (B), which is labeled as RCIdiff.
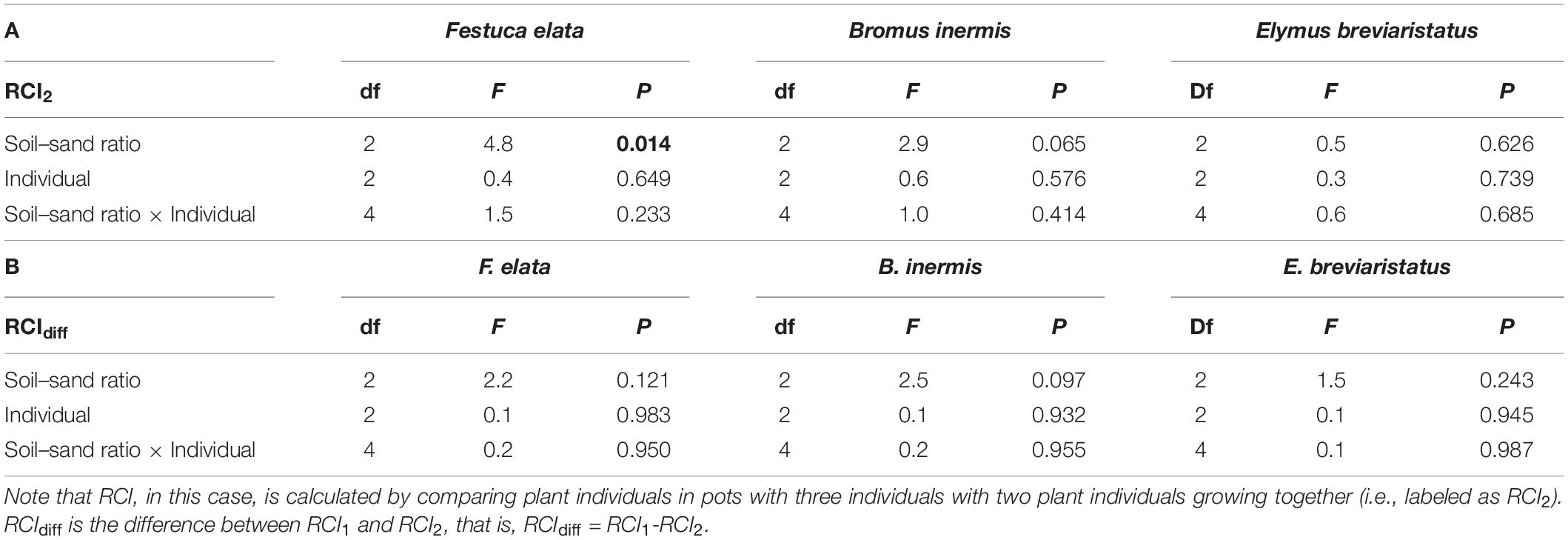
Table 4. Effects of soil–sand ratio (i.e., low, medium, or high), species (i.e., Festuca elata, Bromus inermis, and Elymus breviaristatus), and individual and their interactions on the relative competition intensity (RCI) of each species (Festuca elata, Bromus inermis, and Elymus breviaristatus) in ANOVA, where F-values, degree of freedom (df), and P-value are given, and significant results (P < 0.05) are labeled in bold.
Furthermore, species, soil–sand ratio × species significantly affected RCIdiff (Table 3 and Figure 4B). However, soil–sand ratio as a single factor did not affect the RCIdiff (Table 3).
Discussion
We found that plant interactions were affected by soil–sand ratio, which differed among plant species. Specifically, RCI (RCI1, RCI2, or RCIdiff) of F. elata and B. inermis was the highest in the medium soil–sand ratio, while RCI1 of E. breviaristatus was the highest in the high soil–sand ratio.
Our first hypothesis, stating that RCI would increase with increasing soil heterogeneity (i.e., soil–sand ratio), was supported. We found that soil–sand ratio significantly affected RCI1 (Figure 3 and Table 2), which is likely caused by the impacts of soil heterogeneity on the root foraging and distribution of plants (Levine et al., 2017; Liu et al., 2017b). However, root performance was not measured at this stage as harvesting roots affects plant growth at the following stages. This finding (i.e., RCI increased with increasing soil heterogeneity) is consistent with previous studies, such as that of Yu et al. (2019) who found that soil heterogeneity increased plant competition intensity within a population at a relatively low plant density. Such positive effects of soil–sand ratio on RCI could be caused by the indirect effect of soil–sand ratio on mycorrhizal fungi, where the effects of mycorrhizal fungi on plant performance increased with increasing soil–sand content (Zaller et al., 2011). Yet, other studies reported that plant competition was affected by resource heterogeneity only when plant individuals were not genetically identical (Day et al., 2003; Zhou et al., 2012). Furthermore, the positive effect may disappear at the later growing stage of plants when the soil–sand ratio was modified by these plants during the growing stages (Roiloa and Retuerto, 2006; Dong et al., 2015). Moreover, such an effect may disappear in a population or community since some individuals in this population or community could detect the neighbors and avoid direct competition (Novoplansky, 2009; Lepik et al., 2021), and some other individuals may perform in the opposite direction. The result depends on the combination of these two effects. Thus, we assumed that both positive and negative effects on plant competition are present simultaneously along the gradient of soil–sand ratio. Generally, plants adopt a diversity of responses to environmental variation (Lawson et al., 2015). However, the potential mechanisms of soil heterogeneity on plant interactions merit further investigation.
Our second hypothesis assumed that the effects of soil–sand ratio on RCI could be modified by plant species identity. In line with our expectation, species significantly affected RCI2 and RCIdiff (Table 3). This is consistent with previous studies that grasses-mixture had higher shoot biomass than root biomass (Bessler et al., 2009). Specifically, we found that in mixtures with two individuals, the medium soil–sand ratio supported higher RCI2 of F. elata than the other two ratios. This may be related to differences in the electrical conductivity (EC), where the medium soil–sand ratio had a higher EC value than the other two ratios (Table 1). However, the high soil–sand ratio supported a higher RCI2 of B. inermis and E. breviaristatus than the other two ratios (Figure 3A). This may be related to pH, where the high ratio had the highest pH value (Table 1). Yet, the underlying mechanisms of these two different patterns merit further study.
Plant responses depend on neighbor identity and resource distribution (Mommer et al., 2012; Garlick et al., 2021). Yet, it refers to the same species but different individuals in this case. Thus, we expected that soil heterogeneity and species would jointly affect plant interactions. This was supported since significant interactions between soil–sand ratio and species were found (Table 2), in line with previous studies. For instance, Nakamura et al. (2008) found that soil heterogeneity affected larger individuals but not smaller individuals, where smaller individuals were strongly impacted by their neighbors (Chen et al., 2020). Moreover, the effects of soil heterogeneity on plant height could also be caused by the different growth rates of these individual plants (Hutchings, 1997), where plants growing in patches with more resources tend to grow faster. Such results could be derived from the different germinations at the beginning of the experiment, where Liu and Hou (2021) found that the heterogeneous distribution of soil resources affected seed germinations, which might further affect their heights. Plant heights of the target species in this study may differ after germinations even plants with the similar size were transplanted in this study since the earlier germination seeds may have a high chance to access light, which would be much higher in the later growing stage. However, the lack of germination information of these species in this study impedes us to further test such a hypothesis.
Results of this study should be interpreted and extrapolated with caution due to the following reasons. (1) This was a short-term experiment with only three soil–sand ratios, which impedes us to make comprehensive conclusions. Thus, further research should include a series of soil–sand ratios in longer term experiments. (2) Previous studies found that natural conditions include diverse plant functional groups. However, only three grass varieties were applied in this study. Therefore, future studies should consider competitive exclusion or a wider range of species, including clonal and N-fixing species (Mayfield and Levine, 2010), where the former can improve their nutrient-use efficiency via clone integration (Ying et al., 2018), and the latter can modify soil conditions through increasing soil nitrogen by fixing nitrogen from the air (Carlsson and Huss-Danell, 2003; Bhandari et al., 2020). (3) Plant interactions are affected by many factors, which is why drivers (e.g., climate) should be considered (McKane et al., 2002; Harpole and Tilman, 2007). (4) Similarly, other physiological and morphological traits such as specific leaf area should be considered in calculating plant interactions (Janecek et al., 2004). (5) Plant interactions vary in time, so results in this study at the early growing stage may not be applicable for the following growth stages (Li et al., 2019).
This is, to our best knowledge, likely to be the first time to quantify the indirect interaction of plants in a controlled experiment, and such interaction is modified by the joint effect of soil heterogeneity and species identity. Natural soils include both qualitative heterogeneity and configurational heterogeneity. However, this study only considered qualitative heterogeneity. Future studies should take the configurational heterogeneity, especially in three dimensions, into account (Liu et al., 2017a,b, 2021). Moreover, only aboveground competition intensity was explored here, future studies should consider plant interactions belowground as plants having a size-based competitive advantage aboveground may not have the same competitive advantage belowground and vice versa (Casper and Jackson, 1997; Poorter and Nagel, 2000; Brown et al., 2019; Liu et al., 2021).
Data Availability Statement
The original contributions presented in the study are included in the article/supplementary material, further inquiries can be directed to the corresponding author.
Author Contributions
YL designed the study and wrote the first draft of the manuscript. GL, MW, and YL conducted the experiment, collected the data, and conducted the analyses. All authors contributed substantially to this study.
Funding
This study was supported by the National Key Research and Development Program of China (2019YFC0507704) and Key Research and Development Program of Ningxia. YL holds a start-up fund from Lanzhou University (508000-561119213).
Conflict of Interest
The authors declare that the research was conducted in the absence of any commercial or financial relationships that could be construed as a potential conflict of interest.
Publisher’s Note
All claims expressed in this article are solely those of the authors and do not necessarily represent those of their affiliated organizations, or those of the publisher, the editors and the reviewers. Any product that may be evaluated in this article, or claim that may be made by its manufacturer, is not guaranteed or endorsed by the publisher.
Acknowledgments
We acknowledge the assistance of Danni Liang, Shenghua Chang, Cheng Zhang, Wanhe Zhu, and Shengwei Xu during the experiments. We thank Hans De Boeck from the University of Antwerp for suggesting linguistic improvements.
References
Allesina, S., and Levine, J. M. (2011). A competitive network theory of species diversity. Proc. Natl. Acad. Sci. U.S.A. 108, 5638–5642. doi: 10.1073/pnas.1014428108
Baer, S. G., Blair, J. M., Collins, S. L., and Knapp, A. K. (2003). Soil resources regulate productivity and diversity in newly established tallgrass prairie. Ecology 84, 724–735. doi: 10.1890/0012-9658(2003)084[0724:srrpad]2.0.co;2
Bessler, H., Temperton, V. M., Roscher, C., Buchmann, N., Schmid, B., Schulze, E. D., et al. (2009). Aboveground overyielding in grassland mixture in associated with reduced biomass partitioning to belowground organs. Ecology 90, 1520–1530. doi: 10.1890/08-0867.1
Bhandari, K. B., West, C. P., and Acosta-Martinez, V. (2020). Assessing the role of interseeding alfalfa into grass on improving pasture soil health in semi-arid Texas High Plains. Appl. Soil Ecol. 147:103399. doi: 10.1016/j.apsoil.2019.103399
Brown, C., Oppon, K. J., and Cahill, J. F. Jr. (2019). Species-specific size vulnerabilities in a competitive arena: nutrient heterogeneity and soil fertility alter plant competitive size asymmetries. Funct. Ecol. 33, 1491–1503. doi: 10.1111/1365-2435.13340
Cahill, J. F., and McNickle, G. G. (2011). The behavioral ecology of nutrient foraging by plants. Annu. Rev. Ecol. Evol. Syst. 42, 289–311. doi: 10.1146/annurev-ecolsys-102710-145006
Carlsson, G., and Huss-Danell, K. (2003). Nitrogen fixation in perennial forage legumes in the field. Plant Soil 253, 353–372.
Casper, B. B., and Jackson, R. B. (1997). Plant competition underground. Annu. Rev. Ecol. Syst. 28, 545–570. doi: 10.1146/annurev.ecolsys.28.1.545
Catorci, A., Cesaretti, S., Velasquez, J. L., and Zeballos, H. (2011). Plant-plant spatial interactions in the dry Puna (southern Peruvian Andes). Alp. Bot. 121:113. doi: 10.1007/s00035-011-0097-1
Chen, B. J. W., Xu, C., Liu, M., Huang, Z. Y. X., Zhang, M., Tang, J., et al. (2020). Neighbourhood-dependent root distributions and the consequences on root separation in arid ecosystems. J. Ecol. 108, 1635–1648. doi: 10.1111/1365-2745.13360
Chen, Y., and Nelson, R. L. (2006). Variation in early plant height in wild soybean. Crop Sci. 46, 865–869. doi: 10.2135/cropsci2005.07-0202
Chesson, P. (2000). Mechanisms of maintenance of species diversity. Annu. Rev. Ecol. Syst. 31, 343–366. doi: 10.1146/annurev.ecolsys.31.1.343
Chu, C., Chen, L., Fan, P., He, Z., Li, Y., Liao, J., et al. (2021). Conceptual and theoretical dimensions of biodiversity research in China: examples from plants. Natl. Sci. Rev. 8:nwab060.
Craine, J. M., and Dybzinski, R. (2013). Mechanisms of plant competition for nutrients, water and light. Funct. Ecol. 27, 833–840. doi: 10.1111/1365-2435.12081
Cui, K., Peng, S., Ying, Y., Yu, S., and Xu, C. (2004). Molecular dissection of the relationships among tiller number, plant height and heading date in rice. Plant Prod. Sci. 7, 309–318. doi: 10.1626/pps.7.309
Day, K. J., John, E. A., and Hutchings, M. J. (2003). The effects of spatially heterogeneous nutrient supply on yield, intensity of competition and root placement patterns in Briza media and Festuca ovina. Funct. Ecol. 17, 454–463. doi: 10.1046/j.1365-2435.2003.00758.x
Dong, B., Wang, J., Liu, R., Zhang, M., Luo, F., and Yu, F. (2015). Soil heterogeneity affects ramet placement of Hydrocotyle vulgaris. J. Plant Ecol. 8, 91–100. doi: 10.1093/jpe/rtu003
Dufour, A., Gadallah, F., Wagner, H. H., Guisan, A., and Buttler, A. (2006). Plant species and environmental heterogeneity in a mountain landscape: effects of variability and spatial configuration. Ecography 29, 573–584. doi: 10.1111/j.0906-7590.2006.04605.x
Fichtner, A., Hardtle, W., Bruelheide, H., Kunz, M., Li, Y., and von Oheimb, G. (2018). Neighbourhood interactions drive overyielding in mixed-species tree communities. Nat. Commun. 9:1144.
Fransen, B., de Kroon, H., and Berendse, F. (2001). Soil nutrient heterogeneity alters competition between two perennial grass species. Ecology 82, 2534–2546. doi: 10.1890/0012-9658(2001)082[2534:snhacb]2.0.co;2
Garlick, K., Drew, R. E., and Rajaniemi, T. K. (2021). Root responses to neighbors depend on neighbor identity and resource distribution. Plant Soil 467, 227–237. doi: 10.1007/s11104-021-05083-9
Grilli, J., Barabas, G., Michalska-Smith, M. J., and Allesina, S. (2017). Higher-order interactions stabilize dynamics in competitive network models. Nature 548, 210–213. doi: 10.1038/nature23273
Harpole, W. S., and Tilman, D. (2007). Grassland species loss resulting from reduced niche dimension. Nature 446, 791–793. doi: 10.1038/nature05684
Hart, S. P., Freckleton, R. P., and Levine, J. M. (2018). How to quantify competitive ability. J. Ecol. 106, 1902–1909. doi: 10.1111/1365-2745.12954
Hodge, A. (2004). The plastic plant: root responses to heterogeneous supplies of nutrients. New Phytol. 162, 9–24. doi: 10.1111/j.1469-8137.2004.01015.x
Hou, F., and Shen, Y. (1999). A preliminary analysis on the spatial pattern of landscape of salinized grassland in Linze county. Acta Agrestia Sin. 7, 263–270.
Houseman, G. R. (2014). Aggregated seed arrival alters plant diversity in grassland communities. J. Plant Ecol. 7, 51–58. doi: 10.1093/jpe/rtt044
Hutchings, M. J. (1997). “The structure of plant populations,” in Plant Ecology, ed. M. J. Crawley (Oxford: Blackwell Science), 325–358. doi: 10.1002/9781444313642.ch11
Janecek, S., Janeckova, P., and Leps, J. (2004). Influence of soil heterogeneity and competition on growth features of three meadow species. Flora 199, 3–11. doi: 10.1078/0367-2530-00127
Johansson, M. E., and Keddy, P. A. (1991). Intensity and asymmetry of competition between plant pairs of different degrees of similarity: an experimental study on two guilds of wetland plants. Oikos 60, 27–34. doi: 10.2307/3544988
Kelly, V. R., and Canham, C. D. (1992). Resource heterogeneity in oldfields. J. Veg. Sci. 3, 545–552. doi: 10.2307/3235811
Lawson, C. R., Vindenes, Y., Bailey, L., and van de Pol, M. (2015). Environmental variation and population responses to global change. Ecol. Lett. 18, 724–736. doi: 10.1111/ele.12437
Lepik, A., Abakumova, M., Davison, J., Zobel, K., and Semchenko, M. (2021). Spatial mapping of root systems reveals diverse strategies of soil exploration and resource contest in grassland plants. J. Ecol. 109, 652–663. doi: 10.1111/1365-2745.13535
Levine, J. M., Bascompte, J., Adler, P. B., and Allesina, S. (2017). Beyond pairwise mechanisms of species coexistence in complex communities. Nature 546, 56–64. doi: 10.1038/nature22898
Li, H., Wang, X., Brooker, R. W., Rengel, Z., Zhang, F., Davies, W. J., et al. (2019). Root competition resulting from spatial variation in nutrient distribution elicits decreasing maize yield at high planting density. Plant Soil 439, 219–232. doi: 10.1007/s11104-018-3812-5
Li, M., Wei, Z., Wang, J., Jousset, A., Friman, V. P., Xu, Y., et al. (2018). Facilitation promotes invasions in plant-associated microbial communities. Ecol. Lett. 22, 149–158. doi: 10.1111/ele.13177
Li, Y., Mayfield, M. M., Wang, B., Xiao, J., Kral, K., Janik, D., et al. (2021). Beyond direct neighbourhood effects: higher-order interactio9ns improve modelling and predicting tree survival and growth. Natl. Sci. Rev. 8:nwaa244.
Li, Y., Xiao, J., Liu, H., Wang, Y., and Chu, C. (2020). Advances in higher-order interactions between organisms. Biodivers. Sci. 28, 1333–1344. doi: 10.17520/biods.2020217
Liu, Y., De Boeck, H. J., Wellens, M. J., and Nijs, I. (2017b). A simple method to vary soil heterogeneity in three dimensions in experimental mesocosms. Ecol. Res. 32, 287–295. doi: 10.1007/s11284-017-1435-6
Liu, Y., Bortier, M. F., De Boeck, H. J., and Nijs, I. (2017a). Root distribution responses to three-dimensional soil heterogeneity in experimental mesocosms. Plant Soil 421, 353–366. doi: 10.1007/s11104-017-3472-x
Liu, Y., Bortier, M. F., Nijs, I., Fu, Y., Li, Z., Hou, F., et al. (2021). Three-dimensional soil heterogeneity modulates responses of grassland mesocosms to an experimentally imposed drought extreme. Oikos 130, 1209–1223. doi: 10.1111/oik.07810
Liu, Y., De Boeck, H. J., Li, Z., and Nijs, I. (2019). Unimodal relationship between three-dimensional soil heterogeneity and plant species diversity in experimental mesocosms. Plant Soil 436, 397–411. doi: 10.1007/s11104-019-03938-w
Liu, Y., and Hou, F. (2021). Effects of three-dimensional soil heterogeneity on seed germination in controlled experiments. J. Plant Ecol. 14, 1–9. doi: 10.1093/jpe/rtaa070
Lortie, C. J., and Turkington, R. (2008). Species-specific positive effects in an annual plant community. Oikos 117, 1511–1521. doi: 10.1111/j.0030-1299.2008.16664.x
Maestre, F. T., and Cortina, J. (2002). Spatial patterns of surface soil properties and vegetation in a Mediterranean semi-arid steppe. Plant Soil 241, 279–291.
Maestre, F. T., and Reynolds, J. F. (2007). Amount or pattern? Grassland responses to the heterogeneity and availability of two key resources. Ecology 88, 501–511. doi: 10.1890/06-0421
Mayfield, M. M., and Levine, J. M. (2010). Opposing effects of competitive exclusion on the phylogenetic structure of communities. Ecol. Lett. 13, 1085–1093. doi: 10.1111/j.1461-0248.2010.01509.x
McKane, R., Johnson, L. C., Shaver, G. R., Nadelhoffer, K. J., Rastetter, E. B., Fry, B., et al. (2002). Resource-based niches provide a basis for plant species diversity and dominance in arctic tundra. Nature 415, 68–71. doi: 10.1038/415068a
Mommer, L., van Ruijven, J., Jansen, C., van de Steeg, H. M., and de Kroon, H. (2012). Interactive effects of nutrient heterogeneity and competition: implications for root foraging theory? Funct. Ecol. 26, 66–73. doi: 10.1111/j.1365-2435.2011.01916.x
Nakamura, R., Kachi, N., and Suzuki, J. (2008). Effects of nutrient distribution pattern and aboveground competition on size of individuals in Ipomoea tricolor populations. Botany 86, 1260–1265. doi: 10.1139/b08-082
Novoplansky, A. (2009). Picking battles wisely: plant behavior under competition. Plant Cell Environ. 32, 726–741. doi: 10.1111/j.1365-3040.2009.01979.x
O’Brien, E. E., Brown, J. S., and Moll, J. D. (2007). Roots in space: a spatially explicit model for belowground competition in plants. P. Roy. Soc. B Biol. Sci. 274, 929–934. doi: 10.1098/rspb.2006.0113
Pierik, R., Mommer, L., and Voesenek, L. A. (2013). Molecular mechanisms of plant competition: neighbor detection and response strategies. Funct. Ecol. 27, 841–853. doi: 10.1111/1365-2435.12010
Poorter, H., and Nagel, O. (2000). The role of biomass allocation in the growth response of plants to different levels of light, CO2, nutrients and water: a quantitative review. Aust. J. Plant Physiol. 27, 595–607. doi: 10.1071/pp99173
Raath-Krüger, M. J., McGeoch, M. A., Schöb, C., Greve, M., and le Roux, P. C. (2019). Positive plant-plant interactions expand the upper distributional limits of some vascular plant species. Ecosphere 10:e02820.
Rajaniemi, T. K. (2011). Competition for patchy soil resources reduces community evenness. Oecologia 165, 169–174. doi: 10.1007/s00442-010-1710-5
Rajaniemi, T. K., Turkington, R., and Goldberg, D. (2009). Community-level consequences of species interactions in an annual plant community. J. Veg. Sci. 20, 836–846. doi: 10.1111/j.1654-1103.2009.01086.x
Ravenek, J. M., Mommer, L., Visser, E. J. W., van Ruijven, J., van der Paauw, J. W., Smit-Tiekstra, A., et al. (2016). Linking root traits and competitive success in grassland species. Plant Soil 407, 39–53. doi: 10.1007/s11104-016-2843-z
Robinson, D., Hodge, A., Griffiths, B. S., and Fitter, A. H. (1999). Plant root proliferation in nitrogen-rich patches confers competitive advantage. P. Roy. Soc. B Biol. Sci. 266, 431–435. doi: 10.1098/rspb.1999.0656
Roiloa, S. R., and Retuerto, R. (2006). Small-scale heterogeneity in soil quality influences photosynthetic efficiency and habitat selection in a clonal plant. Ann. Bot. 98, 1043–1052. doi: 10.1093/aob/mcl185
Roiloa, S. R., Sanchez-Rodrigues, P., and Retuerto, R. (2014). Heterogeneous distribution of soil nutrients increase intra-specific competition in the clonal plant Glechoma hederacea. Plant Ecol. 215, 863–873. doi: 10.1007/s11258-014-0338-7
Schenk, H. J. (2006). Root competition: beyond resource depletion. J. Ecol. 94, 725–739. doi: 10.1111/j.1365-2745.2006.01124.x
Wassmuth, B. E., Stoll, P., Tscharntke, T., and Thies, C. (2009). Spatial aggregation facilitates coexistence and diversity of wild plant species in field margins. Perspect. Plant Ecol. 11, 127–135. doi: 10.1016/j.ppees.2009.02.001
Weigelt, A., and Jolliffe, P. (2003). Indices of plant competition. J. Ecol. 91, 707–720. doi: 10.1046/j.1365-2745.2003.00805.x
Wijesinghe, D. K., John, E. A., and Hutchings, M. J. (2005). Does pattern of soil resource heterogeneity determine plant community structure? An experimental investigation. J. Ecol. 93, 99–112. doi: 10.1111/j.0022-0477.2004.00934.x
Xiao, S., Chen, S., and Wang, G. (2007). Does the ESS height of plant populations still exist with the inclusion of spatial structure?—an individual-based model research. Ecol. Model. 204, 213–218. doi: 10.1016/j.ecolmodel.2007.01.025
Yang, L., and Rudolf, V. H. W. (2010). Phenology, ontogeny and the effects of climate changes on the time of species interactions. Ecol. Lett. 13, 1–10. doi: 10.1111/j.1461-0248.2009.01402.x
Ying, Z., Ge, G., and Liu, Y. (2018). The effects of clonal integration on the responses of plant species to habitat loss and habitat fragmentation. Ecol. Model. 384, 290–295. doi: 10.1016/j.ecolmodel.2018.06.016
Yu, H., Shen, N., Yu, D., and Liu, C. (2019). Effects of temporal heterogeneity of water supply and spatial heterogeneity of soil nutrients on the growth and intraspecific competition of Bolboschoenus yagara depend on plant density. Front. Plant Sci. 9:1987. doi: 10.3389/fpls.2018.01987
Zaller, J. G., Frank, T., and Drapela, T. (2011). Soil sand content can alter effects of different taxa of mycorrhizal fungi on plant biomass production of grassland species. Eur. J. Soil Biol. 47, 175–181. doi: 10.1016/j.ejsobi.2011.03.001
Zhang, L., Mi, X., Harrison, R. D., Yang, B., Man, X., Ren, H., et al. (2020). Resource heterogeneity, not resource quantity, plays an important role in determining tree species diversity in two species-rich forests. Front. Ecol. Evol. 8:224. doi: 10.3389/fevo.2020.00224
Zhou, J., Dong, B., Alpert, P., Li, H., Zhang, M., Lei, G., et al. (2012). Effects of soil nutrient heterogeneity on intraspecific competition in the invasive, clonal plant Alternanthera philoxeroides. Ann. Bot. 109, 813–818. doi: 10.1093/aob/mcr314
Zhu, X., Shen, Y., Yan, S., and Zhao, Y. (1997). The base research of Linze grassland ecological test station. Pratacultural Sci. 14, 14–19.
Appendix
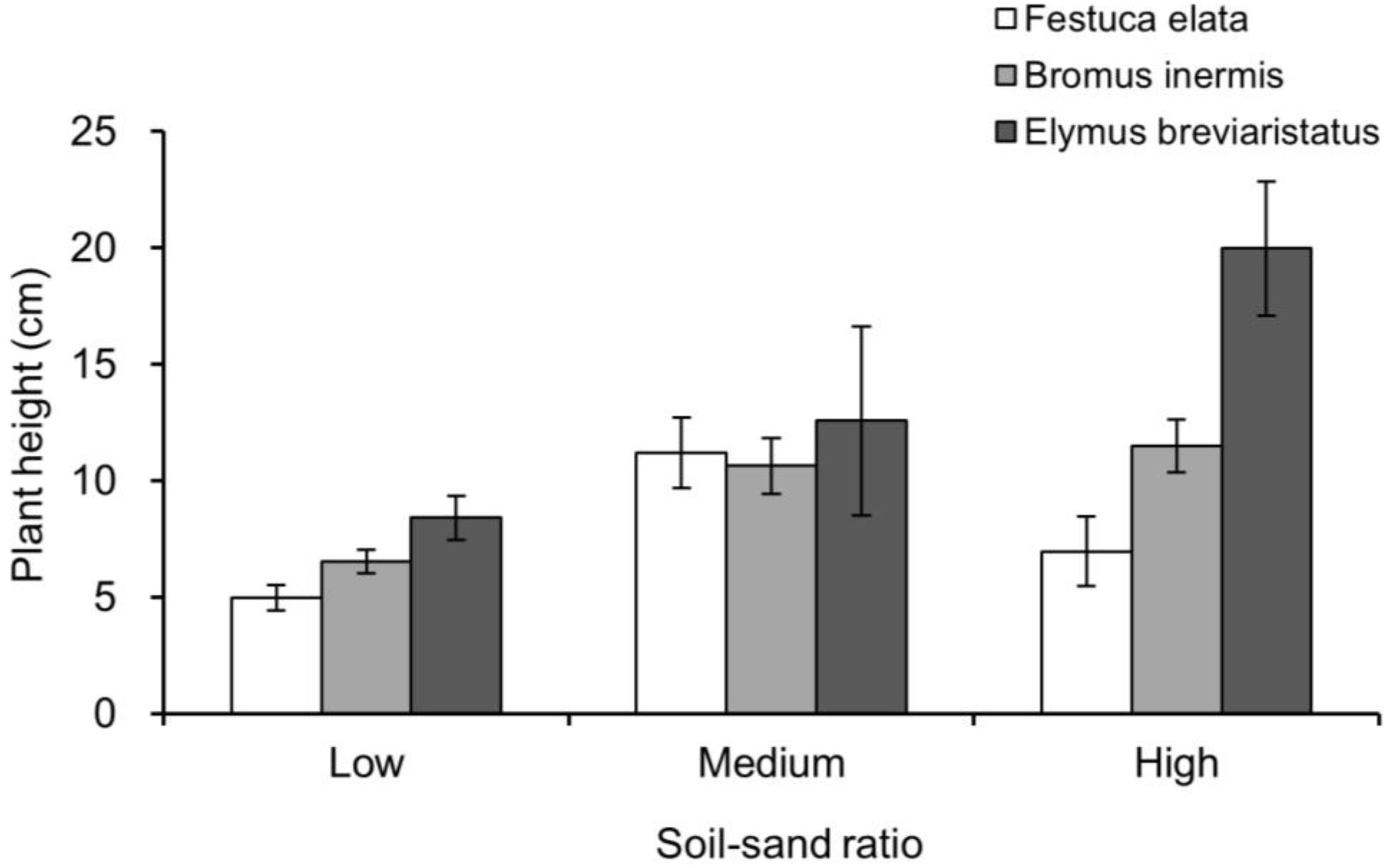
Appendix Figure 1. Plant height in pots with one individual growing along, which is separated by soil–sand ratio (i.e., low, medium, and high) and species (i.e., Festuca elata, Bromus inermis, and Elymus breviaristatus).
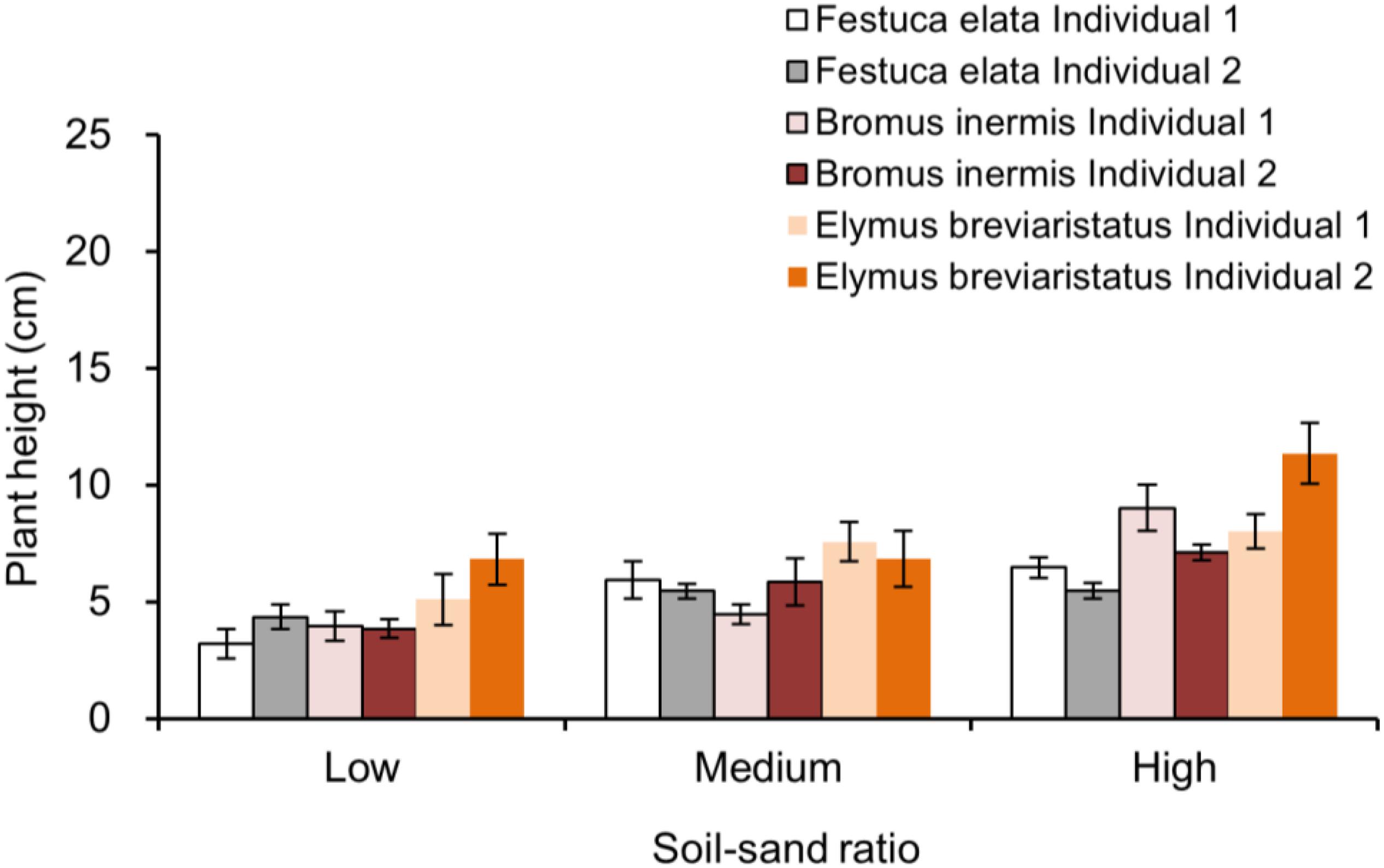
Appendix Figure 2. Plant height in pots with two individuals growing together, which is separated by the individual (i.e., individual identity in a pot), soil–sand ratio (i.e., low, medium, and high), and species (i.e., Festuca elata, Bromus inermis, and Elymus breviaristatus).
Keywords: relative competition intensity, soil–sand ratio, soil heterogeneity, higher-order interactions, plant interactions
Citation: Li G, Wang M, Ma C, Tao R, Hou F and Liu Y (2021) Effects of Soil Heterogeneity and Species on Plant Interactions. Front. Ecol. Evol. 9:756344. doi: 10.3389/fevo.2021.756344
Received: 10 August 2021; Accepted: 29 September 2021;
Published: 25 October 2021.
Edited by:
Alexei B. Ryabov, University of Oldenburg, GermanyReviewed by:
Hong Wei Yu, Key Laboratory of Drinking Water Science and Technology, Research Center for Eco-Environmental Sciences, Chinese Academy of Sciences (CAS), ChinaYong Zhou, Yale University, United States
Copyright © 2021 Li, Wang, Ma, Tao, Hou and Liu. This is an open-access article distributed under the terms of the Creative Commons Attribution License (CC BY). The use, distribution or reproduction in other forums is permitted, provided the original author(s) and the copyright owner(s) are credited and that the original publication in this journal is cited, in accordance with accepted academic practice. No use, distribution or reproduction is permitted which does not comply with these terms.
*Correspondence: Yongjie Liu, eWpsQGx6dS5lZHUuY24=
†These authors have contributed equally to this work