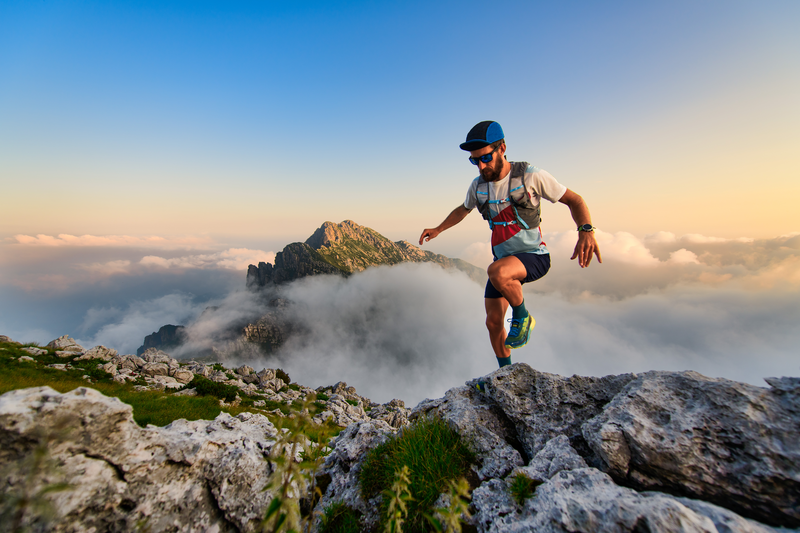
94% of researchers rate our articles as excellent or good
Learn more about the work of our research integrity team to safeguard the quality of each article we publish.
Find out more
ORIGINAL RESEARCH article
Front. Ecol. Evol. , 20 January 2022
Sec. Behavioral and Evolutionary Ecology
Volume 9 - 2021 | https://doi.org/10.3389/fevo.2021.756204
Differences among groups in collective behavior may arise from responses that all group members share, or instead from differences in the distribution of individuals of particular types. We examined whether the collective regulation of foraging behavior in colonies of the desert red harvester ant (Pogonomyrmex barbatus) depends on individual differences among foragers. Foragers lose water while searching for seeds in hot, dry conditions, so colonies regulate foraging activity in response to humidity. In the summer, foraging activity begins in the early morning when humidity is high, and ends at midday when humidity is low. We investigated whether individual foragers within a colony differ in the decision whether to leave the nest on their next foraging trip as humidity decreases, by tracking the foraging trips of marked individuals. We found that individuals did not differ in response to current humidity. No ants were consistently more likely than others to stop foraging when humidity is low. Each day there is a skewed distribution of trip number: only a few individuals make many trips, but most individuals make few trips. We found that from one day to the next, individual foragers do not show any consistent tendency to make a similar number of trips. These results suggest that the differences among colonies in response to humidity, found in previous work, are due to behavioral responses to current humidity that all workers in a colony share, rather than to the distribution within a colony of foragers that differ in response.
Evolution shapes how organisms respond to changing conditions (West-Eberhard, 2003; Sultan, 2015). The evolution of behavioral response to changing conditions relies on plasticity in behavior, or behavioral reaction norms (Dingemanse et al., 2010; Réale and Dingemanse, 2010). The starting point for natural selection on behavior is the variation among individuals (Lott, 1991) or personality (Sih et al., 2015; Loftus et al., 2020) that leads to variation in developmental trajectories and in responses to environmental fluctuations (Ratikainen and Kokko, 2019).
A fundamental question throughout biology is how much differences among components contribute to the phenotypic plasticity of a natural system. For example, how do changes in the function of cells during development depend on inherent differences among cells or on interactions among cells (Haraway, 1976; O’Brien and Bilder, 2013)? How does neural function arise from particular specialized neurons or from networks that assign neurons temporary roles (Anderson, 2010)? In general, how does the function of a biological system depend on the performance of inherently different component types or on context-dependent responses of equivalent components?
Understanding the evolution of collective behavior raises these questions about how variation contributes to plasticity. Collective response to fluctuation in environmental conditions varies among groups (Wray et al., 2011; Bengston and Jandt, 2014), for example, in colonies of harvester ants (Gordon et al., 2011), pods of dolphins (Lewis and Schroeder, 2003; Mann et al., 2012), and packs of African wild dogs (Creel and Creel, 1995) and wolves (Cassidy et al., 2015). Natural selection may shape differences in the distribution of particular types within a group, or instead differences in how all group members engage in collective behavior (Loftus et al., 2020). For example, the relations that determine access to resources may depend on persistent differences among individuals, or instead shift as conditions change (Chase et al., 2002; Desjardins et al., 2012).
The study of social insects has been dominated by this question: is variation among colonies explained by differences in the distribution of particular types of individuals within a colony, or by differences shared by all members of each colony? These two views represent the extremes of a gradient (Gordon, 2016). One view, associated with the idea of division of labor, is that specialized individuals carry out particular functions independently, and colonies differ in the distribution of particular types of individuals. The other view, associated with the idea of task allocation, is that equivalent individuals are allocated to different functions through interactions with each other and changing conditions.
Social insect colonies vary in collective behavior (Gordon et al., 2011; Jeanson and Weidenmüller, 2014; Bockoven et al., 2015). Variation among colonies is the starting point for natural selection, because social insect colonies function as reproductive individuals (Jandt and Gordon, 2016). If colony behavior is due to the distribution of individuals performing particular behavior, then the important variation among colonies, on which selection acts, is in this distribution (Oster and Wilson, 1978). By contrast, when colony behavior operates as a distributed process, based on the responses of equivalent individuals to interactions, then the important variation among colonies, on which selection acts, is in these responses, shared by colony members (Gordon, 2013).
Here we consider how the foraging behavior of colonies of the red harvester ant (Pogonomyrmex barbatus) depends on behavioral differences among individual foragers in response to humidity. Colonies must spend water to get water, because ants lose water to evaporation when searching for seeds, but gain water by metabolizing the fats from the seeds they eat (Lighton and Feener, 1989). Thus, colonies regulate foraging activity in response to the tradeoff between losing water to evaporation and obtaining food and water.
A colony’s foraging activity changes over the course of the morning activity period (Gordon, 1984). In the summer, foraging activity begins in the early morning when humidity outside the nest is high, and ends at midday when humidity is low, while humidity and temperature are constant inside the nest (Pagliara et al., 2018). Foraging activity also varies from day to day (Gordon et al., 2008), depending on humidity, as well as other factors (Gordon et al., 2013; Pagliara et al., 2018). Further, colonies differ in how they regulate foraging in response to changes in humidity (Gordon et al., 2011, 2013): some colonies reduce foraging more in dry conditions, while others continue to forage despite low humidity (Gordon, 2013). Such colony differences persist from year to year, although workers live only a year (Gordon, 1991; Gordon et al., 2011), and are associated with colony differences in dopamine neurophysiology and differences in sensitivity to water loss through evaporation (Friedman et al., 2018, 2019, 2020). The colonies that sacrifice food intake to conserve water, by restricting foraging activity that entails evaporative water loss, show higher reproductive success in offspring colonies (Gordon, 2013).
To learn how differences among workers contribute to a colony’s regulation of foraging activity, we examined differences among marked individuals in foraging behavior. A forager goes out on its first trip each morning in response to olfactory encounters with returning patrollers (Greene and Gordon, 2003). After that, each forager may make many successive trips during the day (Beverly et al., 2009). A forager is stimulated to leave the nest on its next trip by an olfactory encounter with returning foragers with food, in which it responds to the odors of both the cuticular hydrocarbon profile of foragers and the food they carry (Greene et al., 2013; Pinter-Wollman et al., 2013; Pless et al., 2015; Davidson et al., 2016). Each forager travels away from the nest with a stream of other foragers, then leaves the trail to search for seeds, and almost always continues to search until it finds a seed. Once the forager finds a seed, it returns immediately to the nest, so the duration of a foraging trip depends on search time (Gordon, 1991; Beverly et al., 2009). Seeds are scattered and often buried, not patchy, so a forager does not have cues that indicate food abundance. However, the rate of forager return that an outgoing forager experiences inside the nest is associated with overall food availability: the higher the food availability, the shorter the search time, and the higher the rate of forager return (Beverly et al., 2009).
The strong association among foraging activity, worker sensitivity to water loss (Friedman et al., 2019), and current humidity (e.g., Gordon et al., 2013), all indicate that an outgoing forager’s response to its encounters with returning foragers, and its decision to leave the nest on its next trip, depend on the humidity conditions it experienced on previous trips outside the nest (Pagliara et al., 2018).
Here we examine whether foragers within a colony differ in how their decision to leave the nest on the next trip is influenced by the current humidity, in addition to their rate of encounter with returning foragers with food. We considered two alternatives. One is that particular individuals are less likely than others to leave the nest on their next trip when humidity is low. In this case, the higher the proportion of foragers that do not leave the nest in low humidity, the more likely would be the colony to reduce foraging in dry conditions. The ecologically important differences among colonies in response to humidity would be due to differences in this distribution. The other alternative is that individuals within colonies do not differ in response to humidity. In this case, the ecologically important differences among colonies in the regulation of foraging activity in response to humidity arise from responses to conditions that are shared among foragers. To distinguish these alternatives, we compared the responses of marked individuals to decreasing humidity in the course of the morning activity period, and asked whether particular foragers are more likely than others to stop leaving the nest on their next trip when humidity is low.
We observed foraging activity in four colonies (Colony ID: 859, 1107, 1242, and 1368) of the red harvester ant (P. barbatus) under natural conditions at the site of a long-term study near Rodeo, New Mexico, United States. Two colonies (Colony ID: 859 and 1107) were observed in August 2017, and two (Colony ID: 1242 and 1368) in August 2018. The colonies were all at least four years old (Colony ID [age in years]: 859 [19], 1107 [12], 1242 [8], 1368 [4]). Observations began early in the day when humidity was high (70–90%), and continued throughout the morning foraging period as humidity declined. Observations usually continued until both humidity and foraging activity declined (Figure 1). However, on August 20 and 21, 2017, the humidity declined less and eventually leveled off through midday (Figures 1A–D), and observations ended without a sharp decline in humidity.
Figure 1. Foraging rate and humidity. Smoothed outgoing foraging rates for all ants are shown in light blue. Relative humidity is shown in dark blue. Each row of two panels shows the data for Days 1 and 2 for one colony: (A) Colony 859, August 20, 2017; (B) Colony 859, August 21, 2017; (C) Colony 1107, August 20, 2017; (D) Colony 1107, August 21, 2017; (E) Colony 1242, August 22, 2018; (F) Colony 1242, August 23, 2018; (G) Colony 1368, August 24, 2018; (H) Colony 1368, August 25, 2018.
In each of the four colonies of P. barbatus, we collected about 200 foragers on the foraging trail and marked each one with a unique combination of three colors on the head, thorax and abdomen using Uni-Paint markers, as in Beverly et al. (2009). This marking method does not affect the subsequent foraging behavior (Brown and Gordon, 1997). The marked foragers were returned to their nests the same afternoon, and observed on the mornings of the following two days. Observations began at about 7:00 a.m. or after the first wave of foragers had returned from their first trip so that there were ants both leaving and returning to the nest (Figure 1). We chose to observe the same ants on two consecutive days because previous work with marked ants (e.g., Gordon, 1989) showed that the paint can be removed within 2–3 days, for example peeled off by one ant as it grooms another. We chose colonies that did not show any apparent reduction of foraging activity on dry days, using preliminary counts of foraging activity as in Gordon (2013). To make it possible to count all foraging trips by all ants, we chose colonies that consistently used only one main foraging trail. The numbers of foraging trips recorded for marked and unmarked workers are shown in Table 1.
To measure overall foraging rates, observers noted the time and direction of each forager on the trail crossing an imaginary line perpendicular to the trail, marked with rocks on either side of the trail, about 1 m from the edge of the nest mound. To estimate instantaneous outgoing foraging rates from these counts, in units of ants/sec, for each colony and day of observation, we used a sliding window filter, as in Pagliara et al. (2018).
To track the foraging trips of marked ants, one or more observers called out the color marks identifying each marked forager returning to or leaving the nest, crossing the indicated line across the trail, while another observer recorded the time on a tablet. We counted a complete foraging trip for a particular ant when it was observed both traveling out from and returning to the nest (Table 1). In analyzing the data, we considered only the trips for which we had the time at which the forager left and returned to the nest. When an ant had been seen twice leaving the nest, we deleted the first of the two observations, assuming that an intervening return to the nest had been missed. When an ant had been seen twice returning to the nest, we deleted the second of the two observations, assuming that an intervening departure had been missed. For further analysis we used only data from complete round trips.
Previous work at the study site using measurements inside and outside of nests showed that humidity inside the nest in the entrance chamber, where foragers decide whether to leave the nest, is remarkably constant throughout the day, and similar among colonies (Pagliara et al., 2018). Thus, we examined how a forager’s decision to leave depends on recent humidity conditions experienced outside the nest on the previous trip or trips, rather than the current conditions inside the nest which do not change in the course of the day.
Data on relative humidity at 5-min intervals were obtained from the Citizen Weather Observer Program at station E8703 and accessed through Weather Underground.1 The station is located in Rodeo, New Mexico, United States, about 1.7 miles from the study site, and previous work indicates that humidity measures at the site on the ground correspond to those obtained from this station (Pagliara et al., 2018). Relative humidity declined during the morning foraging activity period, ranging from 90 to 45% (Figure 1). For each recorded foraging trip of each marked worker, we found the average humidity over all 5-min intervals during the trip as in Pagliara et al. (2018).
We performed all statistical analyses for each colony and day of observation (i.e., colony-day) separately, because the range of relative humidity values differs from day to day. Other work in progress suggests that colonies respond to relative, not absolute, changes in humidity from day to day. All statistical analyses were conducted using R version 3.5.1 (R Core Team, 2018).
We first tested whether the marked foragers, a subset of all foragers, are representative of the response to humidity of all foragers, in each colony for each of the two days of observation. For each colony-day, we compared the distributions of the outgoing foraging rate as a function of humidity in all ants including the marked ants, and in the marked ants only, using a Kolmogorov-Smirnov test. This is a non-parametric test that assesses the null hypothesis that the two samples, all observed foragers and marked foragers, originate from the same population of foragers.
First, to confirm that in the days we performed this study, as in previous studies (e.g., Gordon et al., 2013), ants tend to stop foraging when humidity is low, we performed a Poisson regression of the total number of trips per ant on the humidity during its final trip of the day, when humidity is low. If a forager tends to perform its last trip when humidity is low, then the regression will have a significantly negative slope. If not, the slope will be positive or flat. We used the total number of trips as a measure of the number of times a forager decides to leave the nest on another trip. We used this measure to focus on how forager decisions to leave the nest depend on current humidity conditions. We did not evaluate total time spent foraging outside the nest because this does not reflect the number of foraging trips. An ant tends to stay outside until it finds food, so trip duration depends on search time (Beverly et al., 2009), and variation among ants in total time foraging outside the nest is strongly influenced by variation in food availability at the places they search.
For the regression, we used a generalized linear model (GLM): log(Total Number of Trips) ∼ slope × Relative Humidity on Last Trip + intercept, where the response variable (i.e., total number of trips) represents non-negative count data that can be approximated by a Poisson distribution. We fitted the Poisson GLM model using the stats package in R. We checked for overdispersion using the AER package. Regressions were performed for each of the 8 colony-days. We performed analyses for each colony and day using separate models, because both humidity and foraging activity differ strongly from day to day (Gordon et al., 2008).
Next, to address the question whether individuals differ in response to humidity, we compared the number of trips made by a forager on Day 1 to the number of trips it made on Day 2. If certain individuals are more sensitive to low humidity than others, and thus likely to stop foraging earlier in the day, those individuals would tend to make fewer trips each day. We used number of trips rather than the value of humidity because response to humidity changes from day to day (Gordon et al., 2008, 2013). Previous work and recent work in progress suggest that colony response to humidity depends on changes in humidity over the past days and weeks rather than on the absolute value of relative humidity. Trip number within a day reflects forager response to the change in conditions on that day.
We tested whether certain individuals tend to make more trips by asking whether a given individual made the same number of trips on both days of observation. To do this, we treated as independent the number of foraging trips for each of the two days of observation of a given colony. For each of the four colonies, we compared the number of trips made by each ant that went out on both days, using a non-parametric Friedman test (Daniel, 1990). This test yields non-significant results if the number of trips overall is the same from day to day. This could occur either when most individual ants consistently make a similar number of trips from one day to the next, or when the number of trips increases for some ants and decreases for others. To distinguish these two possibilities, we further asked whether individual ants tend to make the same number of foraging trips from one day to the next, by examining the coefficient of correlation from day to day in number of trips.
Next, we asked whether there is a significant tendency for some ants to forage at lower humidity than other ants, using a permutation test (Curran-Everett, 2012) that compared the observed values of the humidity at which ants made their last trips on Days 1 and 2 with a null distribution, which was obtained by randomly permuting the humidity values associated with the ants’ last trips. This tests the null hypothesis that ants are similar in the humidity level at which they stop foraging. We performed this permutation test for each of the four colonies observed. In each colony, we considered only the behavior of those foragers that were observed foraging on both days of observation.
For each colony, and for each ant, we found the average of the humidity on its last trip over the two days. We then found the maximum of these average values, i.e., the value associated with the ant that stopped foraging at the highest average humidity value for each colony. This maximum value of average humidity was our test statistic. We then calculated the distribution of possible values for this statistic under the null hypothesis that ants are similar in the humidity level at which they stop foraging. To obtain the null distribution, we permuted the Days 1 and 2 humidity values associated with last trip of each ant, calculated the new averages for each ant, and obtained the maximum of these averages, and repeated this procedure 10,000 times. We then compared the observed maximum average humidity with the null distribution. The more likely an ant is to stop foraging when humidity is low, the lower its average. Of the 10,000 values in the null distribution, we counted how many were equally or more extreme than the observed value for each colony. The P-value was calculated as the proportion of times the values were equally or more extreme than the observed value (Curran-Everett, 2012).
The relation of foraging activity and humidity in the marked ants was similar to that of the rest of the foragers (Figure 2 and Table 2). Marked ants made 2.5–7.4% and 1.9–5.1% of all outgoing and returning trips recorded, respectively. For all 8 colony-days of observation, there was no significant difference between the marked foragers and all foragers, marked and unmarked, in the distribution of outgoing foragers per minute as a function of humidity (Kolmogorov-Smirnov test P-values > 0.1; Table 2).
Figure 2. Distributions of outgoing foraging rate as a function of relative humidity. Data for all ants are shown in solid blue, for marked ants in red. The distribution of foraging rate (i.e., the number of outgoing foragers per minute) in response to humidity for marked foragers is similar to that of all foragers (Table 2). Each row of two panels shows the data for Days 1 and 2 for one colony: (A) Colony 859, August 20, 2017; (B) Colony 859, August 21, 2017; (C) Colony 1107, August 20, 2017; (D) Colony 1107, August 21, 2017; (E) Colony 1242, August 22, 2018; (F) Colony 1242, August 23, 2018; (G) Colony 1368, August 24, 2018; (H) Colony 1368, August 25, 2018.
Table 2. Results from two-sample Kolmogorov-Smirnov tests, comparing the distributions of marked ants and of all ants of outgoing foragers per minute as a function of humidity (Figure 2).
Individual foragers do not tend to make the same number of trips from one day to the next (Figure 3); particular ants do not tend to continue foraging even when humidity is low. Instead, as previously observed (e.g., Gordon et al., 2013), ants tend to stop foraging as humidity declines. While humidity differed from day to day (Figure 1), all regressions of number of trips against relative humidity on the forager’s last trip had a significantly negative slope (P < 0.01; Table 3 and Figure 4), confirming that the more foraging trips an ant makes, the lower the humidity on its last trip.
Figure 3. Frequency distribution of number of foraging trips on two consecutive days in colonies 859 (A), 1107 (B), 1242 (C), and 1368 (D). Dark green bars represent Day 1; light green bars represent Day 2.
Table 3. The slope coefficients of Poisson regression of total trip number per ant on relative humidity are significantly negative (95% confidence intervals provided in parentheses).
Figure 4. Total number of trips made by each ant as a function of the humidity on its last trip. The dark green line shows the result of the Poisson regression model: log(No. of Trips) ∼ slope × Relative Humidity on Last Trip + intercept (coefficient values in Table 3). Each row of two panels shows the data in light green for Day 1 and Day 2 for one colony: (A) Colony 859, August 20, 2017; (B) Colony 859, August 21, 2017; (C) Colony 1107, August 20, 2017; (D) Colony 1107, August 21, 2017; (E) Colony 1242, August 22, 2018; (F) Colony 1242, August 23, 2018; (G) Colony 1368, August 24, 2018; (H) Colony 1368, August 25, 2018.
There was no evidence for day-to-day similarity in the number of trips a forager made; the results of the Friedman tests were not significant for any colony (Colony ID, P-value: 859, P = 0.22; 1107, P = 0.08; 1242, P = 0.08; 1368, P = 0.18). The correlation coefficients indicate no relationship between the number of foraging trips from one day to the next (Figure 5). The correlation coefficients were 0.13, 0.16, 0.28, and 0.26 for colonies 859, 1107, 1242, and 1368, respectively. Thus, the points on the tail of the distributions shown in Figure 3 do not necessarily represent the same ants on both days.
Figure 5. Number of foraging trips on Day 1 by number of trips on Day 2 for colonies (A) 859, (B) 1107, (C) 1242, and (D) 1368 (correlation coefficients 0.13, 0.16, 0.28, and 0.26, respectively). Each dot represents a forager. The black line is the y = x line representing a model yielding a correlation coefficient of one.
There were no differences among individual ants in the humidity level at which they chose to stop foraging. No ants were more likely than others to stop foraging when humidity is low. The results of the permutation tests were not statistically significant for any of the four colonies (Colony ID, P-value: 859, P = 0.77; 1107, P = 0.42; 1242, P = 0.32; 1368, P = 0.13), supporting the null hypothesis that workers within a colony are similar in the humidity level at which they stop foraging.
Colony response to changing humidity is not due to the responses of particularly sensitive individuals that tend to stop foraging when humidity is low, or particularly impervious individuals that tend to continue despite low humidity. There were no consistent day-to-day trends in the number of trips made by about 100 individual foragers in each of four colonies as humidity declines (Figure 3). Instead, when humidity drops, all foragers are less likely to leave the nest on their next trip (Figure 4). This leads to the observed overall decrease in foraging activity in the course of each morning activity period as temperature rises and humidity decreases. Further work is needed to learn how forager decisions about when conditions are too dry to forage may shift with individual experience and colony food supply.
These results are consistent with previous work. One study showed no significant correlation from day to day in the time an individual first leaves the nest (Gordon et al., 2008), and our study shows no such correlation in the total number of trips per day. In another study, we removed on Day 2 the foragers that had made the most trips on Day 1, and found there was no change in the distribution of foraging trips, indicating that a different set of ants were likely to make many trips on Day 2 (Beverly et al., 2009).
Further work is needed to learn how foragers integrate both the rate of encounter with returning foragers, and their assessment of the current risk of water loss. Because humidity inside the nest stays constant while it declines outside (Pagliara et al., 2018), it seems likely that forager response to humidity (Figure 4) is influenced by the conditions it encountered on its previous trip outside the nest (Pagliara et al., 2018). A forager’s decision to leave the nest depends on its olfactory encounters with returning foragers (e.g., Pinter-Wollman et al., 2013), which is a cue to food availability. A forager’s response to these encounters with returning foragers, mediated by its assessment of current humidity conditions, is related to its sensitivity to water loss (Friedman et al., 2019) and the neurophysiology of biogenic amines such as dopamine (Friedman et al., 2018). To learn how these factors combine, a first step would be to examine encounters inside the nest at a range of humidity conditions.
In some ant species, genetic diversity is associated with colony performance of particular tasks (Wiernasz et al., 2008; Saar et al., 2018). Overall genetic diversity may correspond to variation among individuals in task performance, as has been demonstrated in some species (Snyder, 1992; Waddington et al., 2010). However, the results here suggest that by contrast, ecologically important differences among colonies in foraging activity do not arise from differences among individuals within colonies. Instead, our results suggest that the day-to-day differences among colonies in response to changing humidity are due to neurophysiological processes that differ among all workers in a colony (Friedman et al., 2020). The raw material for natural selection on the regulation of foraging in response to humidity (Gordon, 2013) appears to be differences among colonies in behavior common to all workers, rather than variation among colonies in the diversity, range, or distribution of types of workers within a colony.
It is an open question how much of the variation among ant colonies that is ecologically important, and shaped by natural selection, is the result of variation in the distribution of individuals of different types within colonies. Some studies show that colony differences do not depend on differences in distribution of types (e.g., Beshers and Traniello, 1996; Pinter-Wollman et al., 2012). For example, allocation of workers to foraging or brood care in Pogonomyrmex californicus (Holbrook et al., 2011), and Rhytidoponera metallica (Thomas and Elgar, 2003), both depend on colony size, not which type of individuals are present.
There is much to learn, for all animal groups, about how collective behavior depends on variation among individuals. In some social groups, the distribution of individuals showing particular behavior is important; for example, the distribution of age and gender of wolf pack members (Cassidy et al., 2015) is crucial in interactions between groups or packs. By contrast, in other social groups, behavior common to all group members leads to differences among groups, for example in the foraging behavior of pods of bottlenose dolphins (Lewis and Schroeder, 2003; Mann and Sargeant, 2003; Mann et al., 2012).
Our results here suggest that responses common to all foragers within a colony lead to variation among red harvester ant colonies in the regulation of foraging activity. To understand the evolution of collective behavior, we need to learn how much of the ecologically important differences among groups arise from differences in the groups in the distribution of particular types or from differences between one group and another that all group members share.
Data are available in the Stanford Digital Repository at https://purl.stanford.edu/gr652yp4782.
DMG designed the study and performed the data collection. DMG and RP performed the data curation. DMG and NN designed the analyses. NN performed the analyses and wrote the first draft of the manuscript. All authors contributed to manuscript revisions and gave approval for publication.
NN was supported by the Stanford Data Science Scholars program and the Philanthropic Educational Organization (PEO) Scholar Award, International Chapter of the PEO Sisterhood. DMG was supported by the Hewlett Foundation.
The authors declare that the research was conducted in the absence of any commercial or financial relationships that could be construed as a potential conflict of interest.
All claims expressed in this article are solely those of the authors and do not necessarily represent those of their affiliated organizations, or those of the publisher, the editors and the reviewers. Any product that may be evaluated in this article, or claim that may be made by its manufacturer, is not guaranteed or endorsed by the publisher.
We thank Tze Lai, Chenru Liu, and Jing Miaou for their statistical advice and help. We are grateful to Christopher Jackson, Jacob Kupperman, Becca Nelson, Rick Paul, Christian Roussilhon, Mikaela Wilson, Jules Wyman, and Michael Yang for their assistance in the field. We thank Sam Crow for developing software used to record data, and Daniel Friedman for marking the ants. We are grateful to the staff at the Southwestern Research Station for their help and support.
Anderson, M. L. (2010). Neural reuse: a fundamental organizational principle of the brain. Behav. Brain Sci. 33, 245–266. doi: 10.1017/S0140525X10000853
Bengston, S. E., and Jandt, J. M. (2014). The development of collective personality: the ontogenetic drivers of behavioral variation across groups. Front. Ecol. Evol. 2:81. doi: 10.3389/fevo.2014.00081
Beshers, S. N., and Traniello, J. F. A. (1996). Polyethism and the adaptiveness of worker size variation in the attine ant Trachymyrmex septentrionalis. J. Insect Behav. 9, 61–83. doi: 10.1007/BF02213724
Beverly, B. D., McLendon, H., Nacu, S., Holmes, S., and Gordon, D. M. (2009). How site fidelity leads to individual differences in the foraging activity of harvester ants. Behav. Ecol. 20, 633–638. doi: 10.1093/beheco/arp041
Bockoven, A. A., Wilder, S. M., and Eubanks, M. D. (2015). Intraspecific variation among social insect colonies: persistent regional and colony-level differences in fire ant foraging behavior. PLoS One 10:e0133868. doi: 10.1371/journal.pone.0133868
Brown, M. J. F., and Gordon, D. M. (1997). Individual specialisation and encounters between harvester ant colonies. Behaviour 134, 849–866. doi: 10.1163/156853997x00188
Cassidy, K. A., MacNulty, D. R., Stahler, D. R., Smith, D. W., and Mech, L. D. (2015). Group composition effects on aggressive interpack interactions of gray wolves in Yellowstone National Park. Behav. Ecol. 26, 1352–1360. doi: 10.1093/beheco/arv081
Chase, I. D., Tovey, C., Spangler-Martin, D., and Manfredonia, M. (2002). Individual differences versus social dynamics in the formation of animal dominance hierarchies. Proc. Natl. Acad. Sci. U.S.A. 99, 5744–5749. doi: 10.1073/pnas.082104199
Creel, S., and Creel, N. M. (1995). Communal hunting and pack size in African wild dogs, Lycaon pictus. Anim. Behav. 50, 1325–1339. doi: 10.1016/0003-3472(95)80048-4
Curran-Everett, D. (2012). Explorations in statistics: permutation methods. Adv. Physiol. Educ. 36, 181–187. doi: 10.1152/advan.00072.2012
Daniel, W. W. (1990). “Friedman two-way analysis of variance by ranks,” in Applied Nonparametric Statistics, ed. W. W. Daniel (Boston, MA: PWS-Kent).
Davidson, J. D., Arauco-Aliaga, R. P., Crow, S., Gordon, D. M., and Goldman, M. S. (2016). Effect of interactions between harvester ants on forager decisions. Front. Ecol. Evol. 4:115. doi: 10.3389/fevo.2016.00115
Desjardins, J. K., Hofmann, H. A., and Fernald, R. D. (2012). Social context influences aggressive and courtship behavior in a cichlid fish. PLoS One 7:e32781. doi: 10.1371/journal.pone.0032781
Dingemanse, N. J., Kazem, A. J. N., Réale, D., and Wright, J. (2010). Behavioural reaction norms: animal personality meets individual plasticity. Trends Ecol. Evol. 25, 81–89. doi: 10.1016/j.tree.2009.07.013
Friedman, D. A., Greene, M. J., and Gordon, D. M. (2019). The physiology of forager hydration and variation among harvester ant (Pogonomyrmex barbatus) colonies in collective foraging behavior. Sci. Rep. 9:5126. doi: 10.1038/s41598-019-41586-3
Friedman, D. A., Pilko, A., Skowronska-Krawczyk, D., Krasinska, K., Parker, J. W., Hirsh, J., et al. (2018). The role of dopamine in the collective regulation of foraging in harvester ants. iScience 8, 283–294. doi: 10.1016/j.isci.2018.09.001
Friedman, D. A., York, R. A., Hilliard, A. T., and Gordon, D. M. (2020). Gene expression variation in the brains of harvester ant foragers is associated with collective behavior. Commun. Biol. 3, 1–9. doi: 10.1038/s42003-020-0813-8
Gordon, D. M. (1984). Species-specific patterns in the social activities of harvester ant colonies (Pogonomyrmex). Insectes Soc. 31, 74–86. doi: 10.1007/BF02223693
Gordon, D. M. (1989). Dynamics of task switching in harvester ants. Anim. Behav. 38, 194–204. doi: 10.1016/S0003-3472(89)80082-X
Gordon, D. M. (1991). Behavioral flexibility and the foraging ecology of seed-eating ants. Am. Nat. 138, 379–411. doi: 10.1086/285223
Gordon, D. M. (2013). The rewards of restraint in the collective regulation of foraging by harvester ant colonies. Nature 498, 91–93. doi: 10.1038/nature12137
Gordon, D. M. (2016). From division of labor to the collective behavior of social insects. Behav. Ecol. Sociobiol. 70, 1101–1108. doi: 10.1007/s00265-015-2045-3
Gordon, D. M., Dektar, K. N., and Pinter-Wollman, N. (2013). Harvester ant colony variation in foraging activity and response to humidity. PLoS One 8:e63363. doi: 10.1371/journal.pone.0063363
Gordon, D. M., Guetz, A., Greene, M. J., and Holmes, S. (2011). Colony variation in the collective regulation of foraging by harvester ants. Behav. Ecol. 22, 429–435. doi: 10.1093/beheco/arq218
Gordon, D. M., Holmes, S., and Nacu, S. (2008). The short-term regulation of foraging in harvester ants. Behav. Ecol. 19, 217–222. doi: 10.1093/beheco/arm125
Greene, M. J., and Gordon, D. M. (2003). Cuticular hydrocarbons inform task decisions. Nature 423:32. doi: 10.1038/423032a
Greene, M. J., Pinter-Wollman, N., and Gordon, D. M. (2013). Interactions with combined chemical cues inform harvester ant foragers’ decisions to leave the nest in search of food. PLoS One 8:e52219. doi: 10.1371/journal.pone.0052219
Haraway, D. J. (1976). Crystals, Fabrics, and Fields: Metaphors that Shape Embryos. Available online at: https://philpapers-org.stanford.idm.oclc.org/rec/HARCFA-2 (accessed August 13, 2020).
Holbrook, C. T., Barden, P. M., and Fewell, J. H. (2011). Division of labor increases with colony size in the harvester ant Pogonomyrmex californicus. Behav. Ecol. 22, 960–966. doi: 10.1093/beheco/arr075
Jandt, J. M., and Gordon, D. M. (2016). The behavioral ecology of variation in social insects. Curr. Opin. Insect Sci. 15, 40–44. doi: 10.1016/j.cois.2016.02.012
Jeanson, R., and Weidenmüller, A. (2014). Interindividual variability in social insects–proximate causes and ultimate consequences. Biol. Rev. 89, 671–687. doi: 10.1111/brv.12074
Lewis, J. S., and Schroeder, W. W. (2003). Mud plume feeding, a unique foraging behavior of the bottlenose dolphin in the florida keys. Gulf Mex. Sci. 21, 92–97. doi: 10.18785/goms.2101.09
Lighton, J. R. B., and Feener, D. H. (1989). Water-loss rate and cuticular permeability in foragers of the desert ant Pogonomyrmex rugosus. Physiol. Zool. 62, 1232–1256. doi: 10.1086/physzool.62.6.30156211
Loftus, J., Perez, A., and Sih, A. (2020). Task syndromes: linking personality and task allocation in social animal groups. Behav. Ecol. 32, 1–17.
Lott, D. F. (1991). Intraspecific Variation in the Social Systems of Wild Vertebrates. Cambridge: Cambridge University Press.
Mann, J., and Sargeant, B. (2003). “Like mother, like calf: the ontogeny of foraging traditions in wild Indian ocean bottlenose dolphins (Tursiops sp.),” in The Biology of Traditions: Models and Evidence, eds D. M. Fragaszy and S. Perry (Cambridge: Cambridge University Press), 236–266.
Mann, J., Stanton, M. A., Patterson, E. M., Bienenstock, E. J., and Singh, L. O. (2012). Social networks reveal cultural behaviour in tool-using using dolphins. Nat. Commun. 3:980. doi: 10.1038/ncomms1983
O’Brien, L. E., and Bilder, D. (2013). Beyond the niche: tissue-level coordination of stem cell dynamics. Annu. Rev. Cell Dev. Biol. 29, 107–136. doi: 10.1146/annurev-cellbio-101512-122319
Oster, G. F., and Wilson, E. O. (1978). Caste and ecology in the social insects. Monogr. Popul. Biol. 12, 1–352. doi: 10.2307/2530130
Pagliara, R., Gordon, D. M., and Leonard, N. E. (2018). Regulation of harvester ant foraging as a closed-loop excitable system. PLoS Comput. Biol. 14:e1006200. doi: 10.1371/journal.pcbi.1006200
Pinter-Wollman, N., Bala, A., Merrell, A., Queirolo, J., Stumpe, M. C., Holmes, S., et al. (2013). Harvester ants use interactions to regulate forager activation and availability. Anim. Behav. 86, 197–207. doi: 10.1016/j.anbehav.2013.05.012
Pinter-Wollman, N., Hubler, J., Holley, J. A., Franks, N. R., and Dornhaus, A. (2012). How is activity distributed among and within tasks in Temnothorax ants? Behav. Ecol. Sociobiol. 66, 1407–1420. doi: 10.1007/s00265-012-1396-2
Pless, E., Queirolo, J., Pinter-Wollman, N., Crow, S., Allen, K., Mathur, M. B., et al. (2015). Interactions increase forager availability and activity in harvester ants. PLoS One 10:e0141971. doi: 10.1371/journal.pone.0141971
R Core Team (2018). R: A Language and Environment for Statistical Computing. Vienna: R Foundation for Statistical Computing.
Ratikainen, I. I., and Kokko, H. (2019). The coevolution of lifespan and reversible plasticity. Nat. Commun. 10:538. doi: 10.1038/s41467-019-08502-9
Réale, D., and Dingemanse, N. J. (2010). “Personality and individual social specialisation,” in Social Behaviour: Genes, Ecology and Evolution, eds T. Székely, A. J. Moore, and J. Komdeur (New York, NY: Cambridge University Press), 417–441.
Saar, M., Eyer, P. A., Kilon-Kallner, T., Hefetz, A., and Scharf, I. (2018). Within-colony genetic diversity differentially affects foraging, nest maintenance, and aggression in two species of harvester ants. Sci. Rep. 8:13868. doi: 10.1038/s41598-018-32064-3
Sih, A., Mathot, K. J., Moirón, M., Montiglio, P. O., Wolf, M., and Dingemanse, N. J. (2015). Animal personality and state-behaviour feedbacks: a review and guide for empiricists. Trends Ecol. Evol. 30, 50–60. doi: 10.1016/j.tree.2014.11.004
Snyder, L. E. (1992). The genetics of social behavior in a polygynous ant. Naturwissenschaften 79, 525–527. doi: 10.1007/BF01135776
Sultan, S. E. (2015). Organism and Environment: Ecological Development, Niche Construction, and Adaptation. Oxford: Oxford University Press. doi: 10.1093/acprof:oso/9780199587070.001.0001
Thomas, M. L., and Elgar, M. A. (2003). Colony size affects division of labour in the ponerine ant Rhytidoponera metallica. Naturwissenschaften 90, 88–92. doi: 10.1007/s00114-002-0396-x
Waddington, S. J., Santorelli, L. A., Ryan, F. R., and Hughes, W. O. H. (2010). Genetic polyethism in leaf-cutting ants. Behav. Ecol. 21, 1165–1169. doi: 10.1093/beheco/arq128
West-Eberhard, M. J. (2003). Developmental Plasticity and Evolution. New York, NY: Oxford University Press.
Wiernasz, D. C., Hines, J., Parker, D. G., and Cole, B. J. (2008). Mating for variety increases foraging activity in the harvester ant, Pogonomyrmex occidentalis. Mol. Ecol. 17, 1137–1144. doi: 10.1111/j.1365-294X.2007.03646.x
Keywords: foraging, harvester ants, humidity, individual variation, phenotypic plasticity, Pogonomyrmex barbatus, reaction norm, task allocation
Citation: Nova N, Pagliara R and Gordon DM (2022) Individual Variation Does Not Regulate Foraging Response to Humidity in Harvester Ant Colonies. Front. Ecol. Evol. 9:756204. doi: 10.3389/fevo.2021.756204
Received: 10 August 2021; Accepted: 15 December 2021;
Published: 20 January 2022.
Edited by:
Mingbo Yin, Fudan University, ChinaReviewed by:
Qike Wang, The University of Melbourne, AustraliaCopyright © 2022 Nova, Pagliara and Gordon. This is an open-access article distributed under the terms of the Creative Commons Attribution License (CC BY). The use, distribution or reproduction in other forums is permitted, provided the original author(s) and the copyright owner(s) are credited and that the original publication in this journal is cited, in accordance with accepted academic practice. No use, distribution or reproduction is permitted which does not comply with these terms.
*Correspondence: Nicole Nova, bmljb2xlLm5vdmFAc3RhbmZvcmQuZWR1; Deborah M. Gordon, ZG1nb3Jkb25Ac3RhbmZvcmQuZWR1
Disclaimer: All claims expressed in this article are solely those of the authors and do not necessarily represent those of their affiliated organizations, or those of the publisher, the editors and the reviewers. Any product that may be evaluated in this article or claim that may be made by its manufacturer is not guaranteed or endorsed by the publisher.
Research integrity at Frontiers
Learn more about the work of our research integrity team to safeguard the quality of each article we publish.