- 1Division of Behavioural Ecology, Institute of Ecology and Evolution, University of Bern, Hinterkappelen, Switzerland
- 2Conservation, Ecology, Evolution, and Behaviour Research Group, Ecology and Environment Research Centre, Department of Natural Sciences, Manchester Metropolitan University, Manchester, United Kingdom
- 3Leibniz Institute for Animal Biodiversity, Zoologisches Forschungsmuseum Alexander Koenig, Bonn, Germany
How can individuals obtain a breeding position and what are the benefits associated with philopatry compared to dispersal? These questions are particularly intriguing in polygamous cooperative breeders, where dispersal strategies reflect major life history decisions, and routes to independent breeding may utterly differ between the sexes. We scrutinized sex-dependent life-history routes by investigating dispersal patterns, growth rates and mortality in a wild colony of the cooperatively breeding cichlid Neolamprologus savoryi. Our data reveal that female helpers typically obtain dominant breeding positions immediately after reaching sexual maturity, which is associated with strongly reduced growth. In contrast, males obtain breeder status only at twice the age of females. After reaching sexual maturity, males follow one of two strategies: (i) they may retain their subordinate status within the harem of a dominant male, which may provide protection against predators but involves costs by helping in territory maintenance, defence and brood care; or (ii) they may disperse and adopt a solitary status, which diminishes survival chances and apparently reflects a best-of-a-bad-job strategy, as there are no obvious compensating future fitness benefits associated with this pathway. Our study illustrates that sex-dependent life history strategies strongly relate to specific social structures and mating patterns, with important implications for growth rates, the age at which breeding status is obtained, and survival.
Introduction
Understanding the evolutionary mechanisms underlying variation in dispersal and life-history decisions is a major challenge in evolutionary biology and behavioural ecology (Clobert et al., 2012; Li and Kokko, 2019). Resource competition between relatives, risk of inbreeding, and environmental stochasticity are major factors affecting natal dispersal (Hamilton and May, 1977; Bonte et al., 2012; Clobert et al., 2012). Delayed dispersal is common in animals, and it is often caused by ecological constraints, such as a lack of suitable breeding vacancies or enhanced predation risk. These constraints prevent individuals from leaving home to acquire an independent breeding position elsewhere (“ecological constraints hypothesis”; Emlen, 1982; Pruett-Jones and Lewis, 1990; Komdeur, 1992; Heg et al., 2004; Tanaka et al., 2016). Individuals may furthermore gain higher fitness returns from remaining in the natal territory and reaping benefits from group membership and the potential territory inheritance (“benefits of philopatry hypothesis”; Stacey and Ligon, 1991; Kokko and Ekman, 2002; Kingma et al., 2014). Nevertheless, dispersal is the only possibility to expand one’s range beyond the natal territory and to potentially access new resources such as breeding positions. Elucidating why, when and where individuals disperse is hence crucial if we aim to comprehend the evolution of complex sociality and cooperative breeding (Koenig and Dickinson, 2016; Rubenstein and Abbot, 2017; Kingma et al., 2021).
Dispersal decisions are selected to take account of various life-history traits such as growth, maturation and survival probability, which feedback on the breeding system by influencing resource access, mate choice, and parental care (McNamara et al., 2000; Kokko and Jennions, 2008; Mabry et al., 2013; Székely et al., 2014). A close relationship between the predominant mating pattern in a population and sex-specific dispersal has been hypothesised, with male-biased dispersal being associated with polygynous mating, and female-bias or no bias going along with monogamous mating (Greenwood, 1980; Perrin and Mazalov, 2000; Mabry et al., 2013; Li and Kokko, 2019). In other words, a particular mating system is likely to coincide with sex-specific life-history strategies that are characterised, for instance, by differences in growth and size, age at maturity, adult life-span, and dispersal (Badyaev, 2002; Hamilton and Heg, 2008; Székely et al., 2014; Trochet et al., 2016). For example, competition for territories and reproductive opportunities is typically stronger in males than in females due to sex-specific differences in the possibility to economically monopolise resources and mates (Emlen and Oring, 1977). This may select for a delayed start of reproduction in males in order to attain a large body size providing superiority in contests over resources and mating partners (Warner, 1984; Taborsky, 2016). The reproductive success of females, on the other hand, depends on brood size and hence the volume of the body cavity, but also on the number of broods she can produce and successfully raise (sensu fecundity selection; Hernaman and Munday, 2005). These fitness correlates in females can be strongly affected by the quality of the mate and his contribution to care (Webster, 1991; Huk and Winkel, 2006), which again may be size-dependent. Optimal dispersal decisions of females may consequently diverge from those of males (Promislow et al., 1992; Pakanen et al., 2016; Suh et al., 2020).
In cooperatively breeding species, groups are usually composed of individuals belonging to different generations, which implies divergence in important life history parameters such as growth, maturation, survival, and offspring care (Devillard et al., 2004; Koenig and Dickinson, 2016; Rubenstein and Abbot, 2017). The routes to independent breeding in such highly social animals are influenced by the likelihood to inherit resources from the breeders (Stiver et al., 2006; Leadbeater et al., 2011), reproductive skew within social groups (Johnstone and Cant, 1999; Taborsky, 2009), and costs of alloparental care (Taborsky and Grantner, 1998; Heinsohn and Legge, 1999), which all may affect dispersal decisions of group members (Clutton-Brock et al., 2002; Kingma et al., 2016). Furthermore, ecological factors such as the availability of suitable territories (Hatchwell and Komdeur, 2000), predation risk (Tanaka et al., 2016), and climatic conditions (Jetz and Rubenstein, 2011) may select for delayed dispersal and alternative routes to independent breeding (Eikenaar et al., 2009; Rubenstein, 2011). In many cooperative breeders individuals leaving their natal group go through a solitary phase before starting to breed independently (Kokko and Ekman, 2002; Koenig and Dickinson, 2016). The occurrence and duration of such “floating” phases often differs between the sexes (Eikenaar et al., 2009) and may be associated with survival costs due to increased predation risk (Taborsky, 1984; Ridley et al., 2008). Sex-specific costs and benefits of philopatry and dispersal (e.g., increased risk of mortality or reduced lifetime reproductive success) may yield alternative ways to become a breeder in highly social animals (Stiver et al., 2007; Kingma et al., 2016). To understand the evolutionary mechanisms underlying dispersal decisions in cooperative breeders it is hence important to trace individual life-histories and relate them to the way by which group members obtain breeder status (Kingma et al., 2016).
Here we studied the costs and benefits associated with different life-history routes to obtain breeder status in the cooperatively breeding cichlid, Neolamprologus savoryi (Heg et al., 2005). In this species, polygynous males monopolize several females that defend their own sub-territories, often together with brood care helpers (Josi et al., 2020a,b). Male territories usually cluster together and form colonies with complex relatedness structures (Josi et al., 2021). As in many other polygynous systems males exceed females in size (Heg et al., 2005). Offspring delay dispersal and support the breeder female in egg care, territory maintenance, and defence (Heg et al., 2005; Josi et al., 2019, 2020a,b). With increasing helper age, relatedness to the dominants decreases due to breeder turn-over, dispersal, and extra-pair parentage (Josi et al., 2021). Before reaching sexual maturity male and female helpers are of similar body shape and colour (Figure 1). Sexes take different life-history routes to reproduction: Females become breeders either by taking over their natal or a foreign territory, or by establishing a new territory (Josi et al., 2021). Males usually remain as helpers in a sub-territory until reaching a body size similar to the breeder female. Thereafter, they show two alternative trajectories before eventually becoming breeder: (i) They defend an own sub-territory, which has overlap with a dominant male’s territory and with the territories of females in that male’s harem. Here, they continue helping in territory defence and show submission to the dominant male (in the following these males are termed “subordinate males”; Figure 2). (ii) They disperse and defend an independent territory with little overlap to any other territories. These males (termed “solitary males”; Figure 2) do not show helping behaviour or submission to other males. Both trajectories may eventually result in either establishing a new harem or taking over a territory of a harem owner. Males and females that lose their breeder position were never observed to remain as a helper in the territory, but usually got evicted and most likely predated eventually.
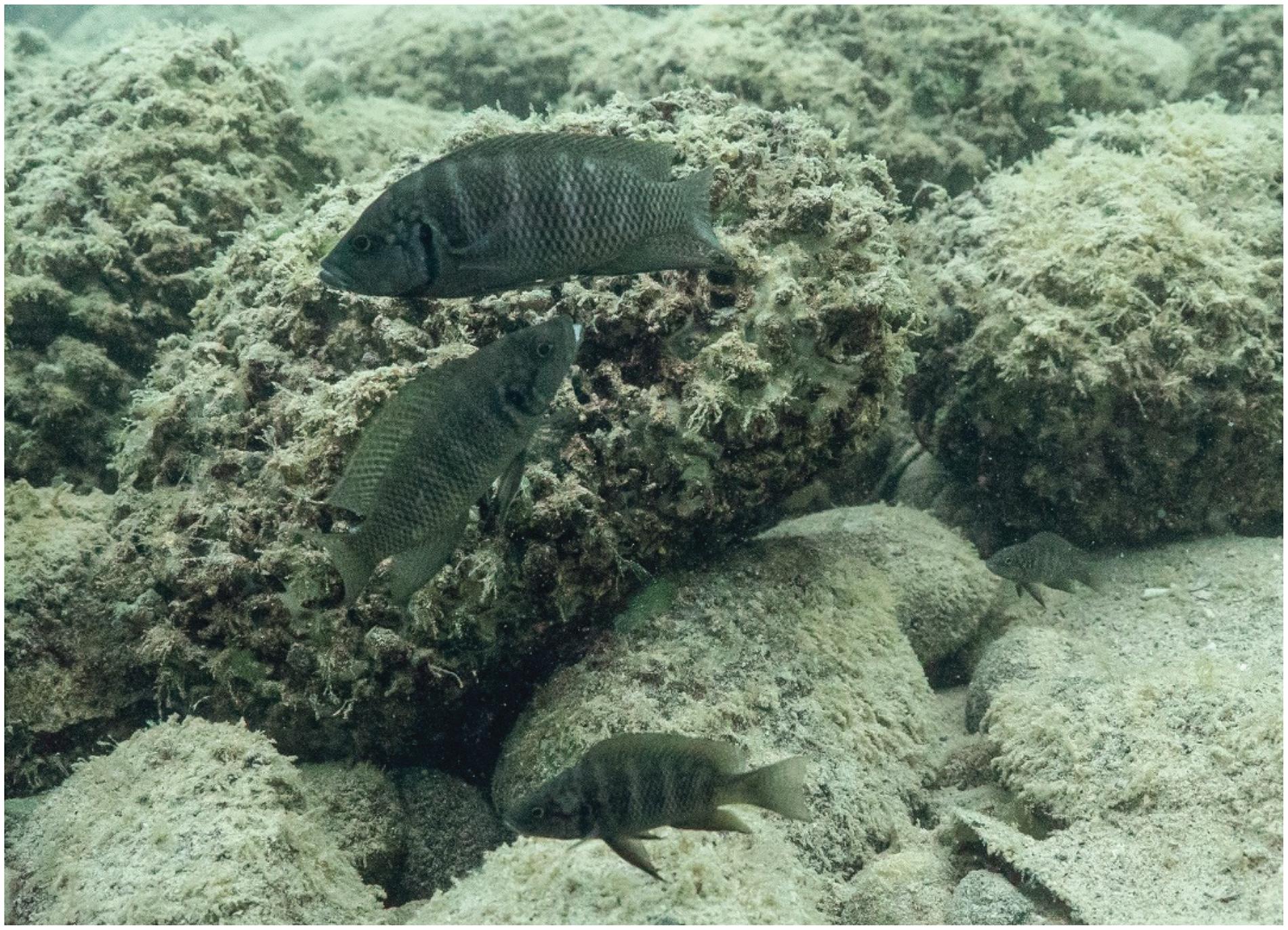
Figure 1. A breeding group of Neolamprologus savoryi consisting of a breeder male (largest fish on top), a breeder female (second largest fish below), as well as one large and one small helper of unknown sex. The group defends the female’s sub-territory, with the main shelter being under the rock in the centre of the picture.
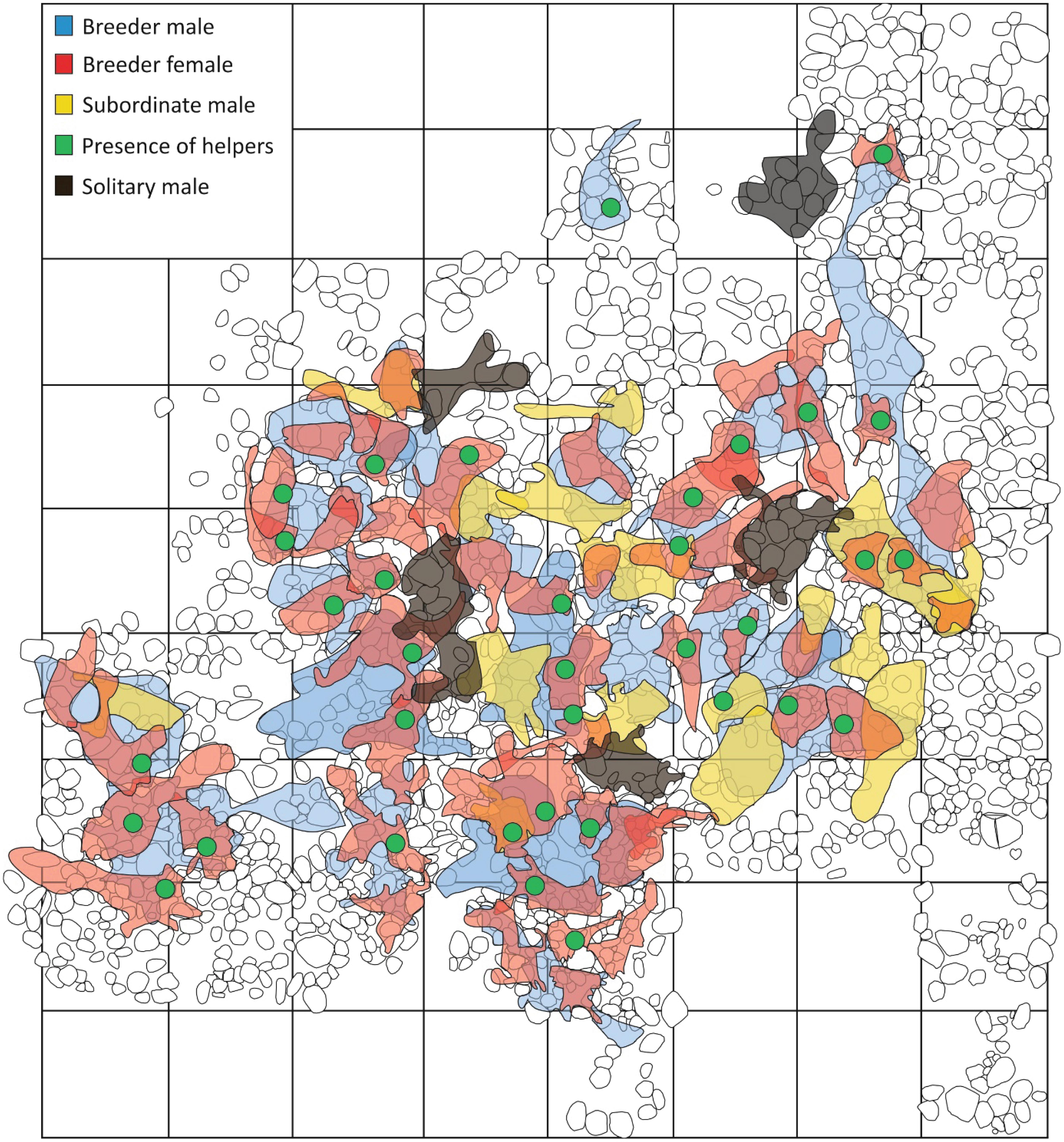
Figure 2. Map of the home ranges of individuals belonging to different social classes of the focal colony in 2016. Different colours indicate different social classes. Green dots indicate helper home ranges, which were small and always within the respective dominant female’s home range. Cobble and rock outlines are by depicted in black lines. Each square measures 1 × 1 m.
This intersexual and intrasexual variation in the routes to independent breeding renders N. savoryi an intriguing model to elucidate sex-specific costs and benefits of alternative dispersal strategies. To that end we traced a wild colony of N. savoryi over two consecutive years and monitored dispersal patterns of males and females. Repeated capture of all colony members enabled us to estimate growth, age, dispersal trajectories and survival in and around the entire colony. With these data we aimed at answering the following questions: (1) How does a polygynous mating system in a cooperatively breeding species relate to sex-specific differences in life-histories (i.e., growth, age at maturity, and survival) and routes to independent breeding? The polygynous mating pattern suggests that the higher competition for a breeding position among males selects for delaying the onset of independent reproduction to attain large size for competitive superiority, as compared to females. This predicts intersexual differences in growth rates, age at maturity, dispersal decisions, survival, and the routes to independent breeding. (2) How does the sex-specific role of subordinate group members (i.e., brood care helpers) affect fundamental life history decisions, such as dispersal? The potential to inherit the territory should be greater in females than in males due to the polygynous group structure, causing more places to fill in the territory of residence for females than for males. Hence, the cooperative breeding pattern suggests that females should remain philopatric and reach a breeding position earlier than males. Furthermore, male helpers are a greater risk to male breeders than female helpers are to female breeders, due to the sex-specific costs of reproductive parasitism (Josi et al., 2021). This implies that males should be forced to leave their natal territory more readily than females, which may favour a solitary phase. Interestingly, regarding the timing of dispersal the mating pattern and the cooperative breeding system predict contrasting sex-specific decisions.
Materials and Methods
Data were collected by SCUBA diving at the southern tip of Lake Tanganyika at Kasakalawe Point, Republic of Zambia, in September and October 2016 and 2017. Here, groups of N. savoryi defend territories containing shelters under rocks in 9–11 m depth (Figure 1; Heg et al., 2005). In both study years a topographic map was established to assess group structures and to mark territories (Figure 2; see Josi et al., 2020b for details). Home ranges of all individuals larger than 1.5 cm were assessed by observing individuals continuously for 20 min (cf. Tanaka et al., 2015; Josi et al., 2020b). Group compositions were determined based on home ranges, social interactions, and breeding chamber visits. Afterward, all fish were captured to determine their sex and standard length (SL). A small piece of fin tissue (approx. 1 mm2) was collected for genetic analyses. In all cases the tissue re-grew after a short period of time. Within years, individuals could be reliably identified using a combination of their size, individual colour patterns and location (Josi et al., 2020b). We re-identified individuals between the 2 years using 14 polymorphic microsatellites (Josi et al., 2019). Additionally, we took genetic samples from individuals in all isolated territories scattered around the focal colony in 2017 in search for potential long-distance dispersers. In both years, group compositions were repeatedly checked to identify potential dispersers. Details on microsatellites and genetic analyses are given in Josi et al. (2019).
We sampled fish from 48 different harems. The social structure of the colony changed between years. In 2016 we captured 182 individuals from which 9 were captured twice within a 3-months period (Table 1). In 2017 we captured 177 individuals in the focal colony and in close-by isolated territories to identify recaptures (Table 1). Of this sample, 34 individuals were recaptures from 2016 (recapture rate between years = 18.57%). In 2017 we also recaptured most individuals within the 3-months observation period (Table 1). We only included individuals as recaptures if a minimum interval of 20 days was given between the catches. In both years combined we recaptured 82 females and 94 males. In total, nine individuals were too small to be reliably sexed (Table 1).
Statistical Analysis
The individual growth rate (mm/day) was calculated as the difference in SL between the two capture events, divided by the number of days in between. A linear mixed effect model (LMM) was fitted to compare the growth rates of males (N = 94) and females (N = 82) in relation to the initial size [ln(SL)] to account for exponentially diminishing growth over time. Growth rate was set as response variable and the initial size and sex as well as the respective interaction were included as predictors. Additionally, Harem ID and Catcher ID were included as random factors to account for non-independence of group members and for potential differences in size measurements by different persons catching the fish. Using the intercept and the slope from the model, the age at which females and males became breeders was calculated (cf. Skubic et al., 2004). To analyse sex-dependent survival rates between the years, we fitted a generalized models (GLM) with binomial error distribution with recapture (yes/no) as response variable and sex as predictor. For comparing the growth rates among the different male types [subordinate males (N = 20), solitary males (N = 6) and breeder males (N = 28)] we fitted a LMM using the daily growth rate as response variable, with body size and male type as predictors. Harem ID and Catcher ID were included as random effects.
To compare the survival rates between male types living either solitary or associated with a group (subordinate and dominant males) over a period of 37 days in 2016 and 2017 (beginning and end of each field season), we sampled the entire focal colony and all surrounding territories in the area. Predation risk is high at the study side, and long-distance dispersal is scarce in cooperatively breeding cichlids (see Taborsky, 2016 for review). Therefore, we conclude that males that disappeared were most likely dead. A GLM with a binomial error distribution was fitted with survival as response variable and social status (solitary males and group males) as predictor.
Differences in transition probability between the sexes from helper stage to solitary living were tested with Fisher’s exact test.
Data were analysed using R version 3.3.3 (R Core Team, 2017). We fitted LMMs, and binomial GLMs using the package lme4 (Bates et al., 2013). Models were checked for overdispersion and normality. Parameter significances were tested using likelihood ratio tests.
Results
Growth and Survival
Growth rates differed between males and females, depending on initial body size (LMM: body size × sex: = 4.62, p = 0.03; Figure 3A). In both sexes, growth rate decreased with increasing body size/age (males: intercept: 0.481, size: β ± SE = –0.12 ± 0.008, = 110.06, p < 0.001; females: intercept: 0.553, size: β ± SE = –0.145 ± 0.013, = 76.7, p < 0.001), but at a size where females already decreased growth and started to breed males continued growing at a higher rate and for a longer period of time (see Figure 3B). The smallest breeder female measured 38 mm, which corresponds to an age of 412 ± 41 days (Figure 3B). The smallest breeder male measured 52 mm (age estimate 1,160 ± 200 days, Figure 3B).
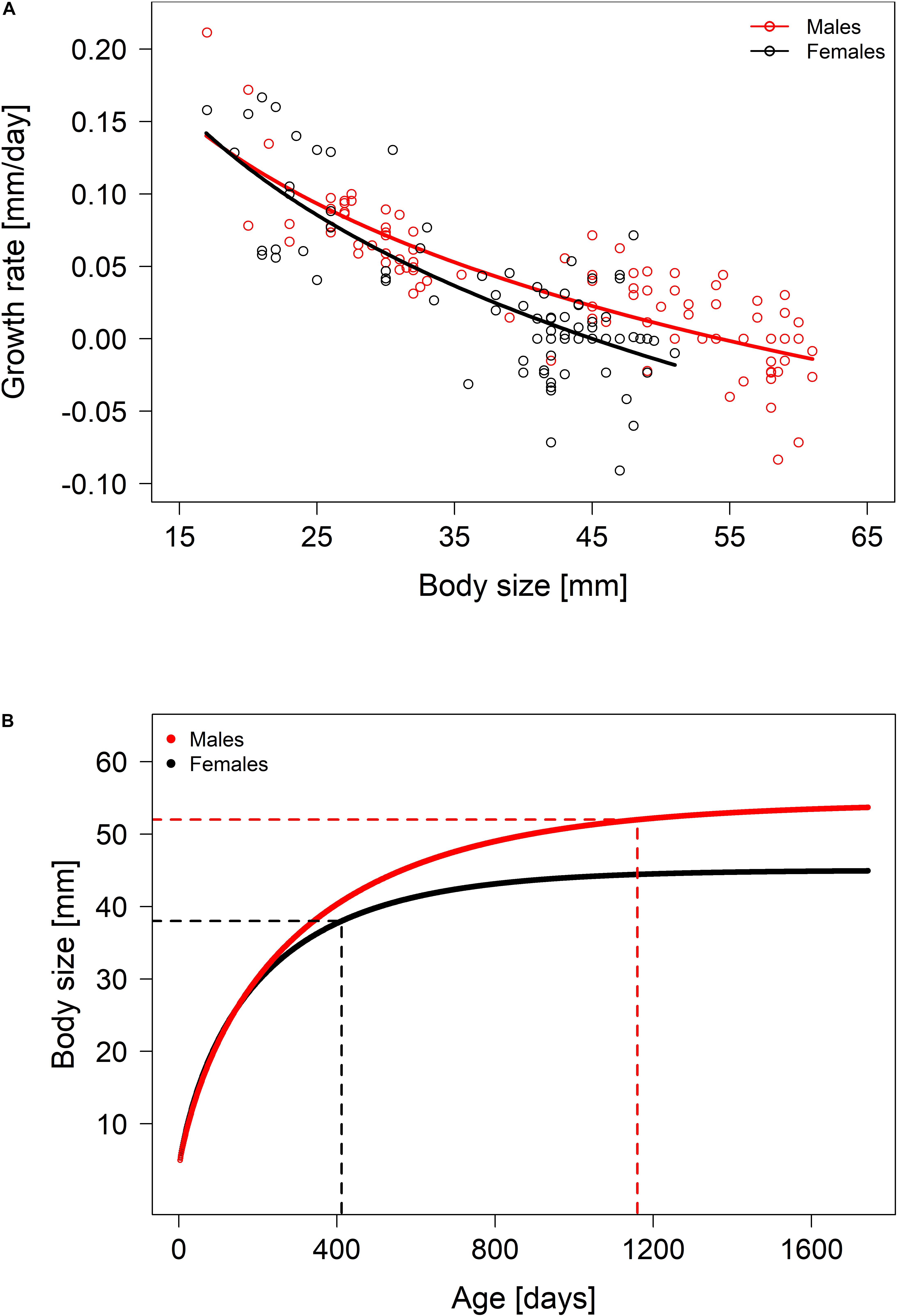
Figure 3. (A) Growth rate in relation to initial body size (SL) of males (red) and females (black). Lines represent the model predicted means. Points represent original data; negative values arise due to measuring errors within and between observers. (B) Size-dependent mean predicted ages of males and females. Dashed lines mark the earliest point in time at which individuals became breeders.
The growth rates did not differ significantly between the three male types ( = 1.63, p = 0.44). Initial body size differed slightly between the categories and seemed to explain more variation in growth than male status (body size: = 1.44, p = 0.23). Solitary males had a lower survival probability compared to males living in a group ( = 5.15, p = 0.02, Figure 4).
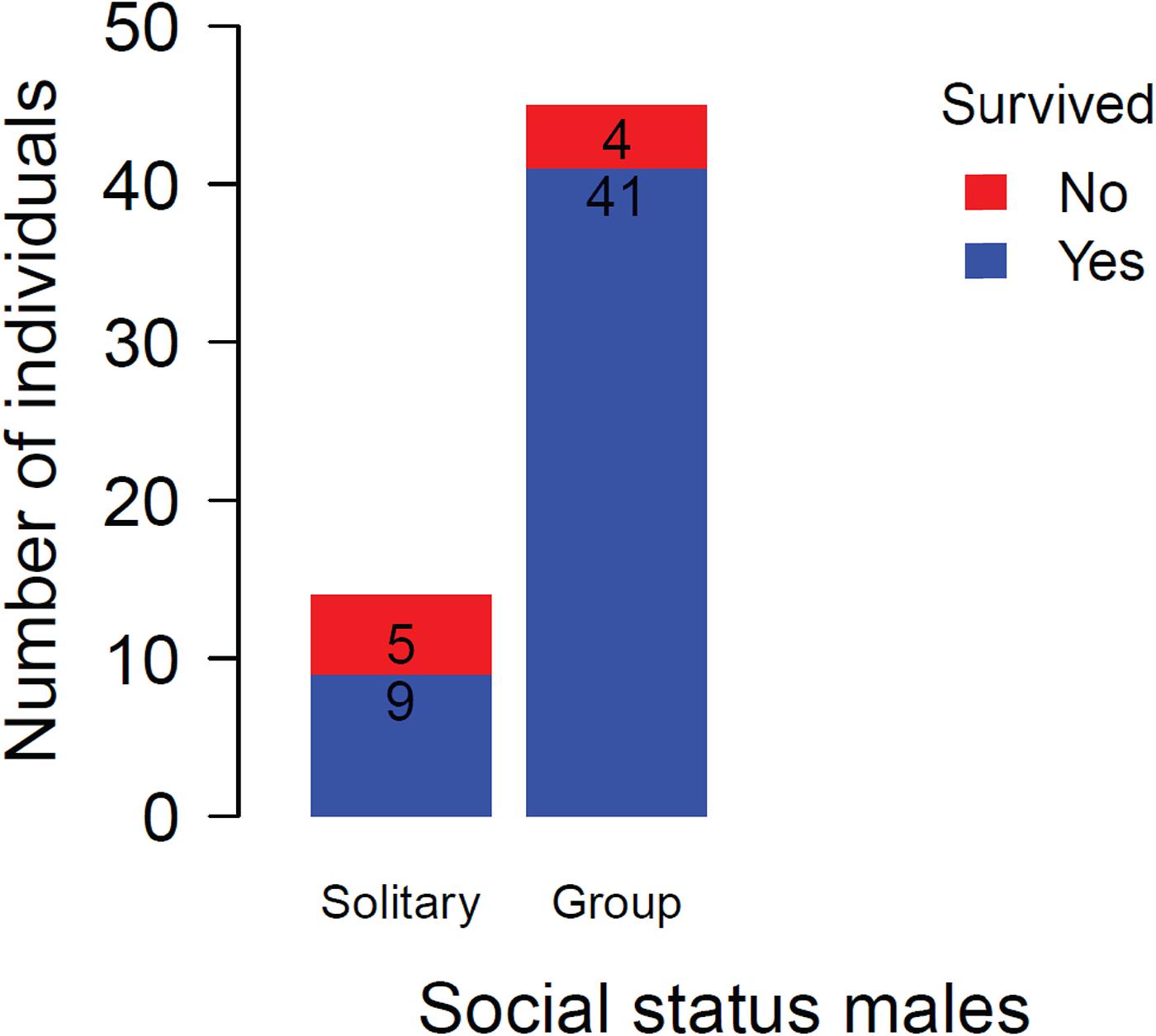
Figure 4. Survival rates of males associated with a group versus those living solitarily over 37 days (from beginning to end of the respective field season). Numbers indicate sample sizes.
Dispersal Events
Within Year
In total, we observed 15 dispersal events occurring within the two observation periods of 3 months each. Eight of these were not associated with a change in social status (four helpers and four breeder females; Table 2). The helpers were two males and two females, which dispersed and took up a helper position in a group of a different female within the same harem. All four dispersing breeder females moved to a breeder male that was larger than their original partner. The dispersal of the other seven individuals was associated with a change in social status. Four of these cases involved subordinate males that either became solitary (n = 3) or took over a harem (n = 1; Table 2). The other three individuals included one solitary male that became a breeder, one helper female changing to breeder status by establishing an own sub-territory within the original harem, and one helper female obtaining a breeder position in another harem defended by a larger male (Table 2).
Between Years
Out of the 34 recaptures between years, 19 individuals (56%) had changed their social status: Three out of 17 subordinate males caught in 2016 had become breeders in 2017 (18%), and eight male helpers had become solitary (47%; Table 3). One out of five solitary males captured in 2016 changed to breeder status in the subsequent year (20%). Seven out of 26 female helpers caught in 2016 had become breeders in 2017, either in the same or a different harem (27%; Table 3). Between years, we further recaptured six helpers (8%) that had not changed social status (five males and one female; Table 3), 5 of which had remained in the same location. The recapture rate of breeders between years was low (7/52 females, 2/32 males) and did not differ between the sexes (GLM; = 0.36, p = 0.55). Most recaptured female breeders (6/7) had changed the harem between years (86%; Table 3).
Territory Inheritance and Status Persistence of Helpers
Male and female helpers differed in their routes to independent breeding (Tables 2, 3). In 2016 we caught 24 male and 22 female helpers. Three of 24 male helpers (12.5%) had become breeders in the following year (one inherited the territory), whereas 7 of 22 female helpers (32%) attained breeder status in the same time interval (three inherited the territory). Most of the male helpers became solitary (8/24; 33%) or remained as helpers/subordinates (5/24; 21%; in 4/5 cases they remained in the same territory), whereas only one female remained as a helper (in the same territory; 5%). The transition from helper to solitary living was more likely in males than in females (females: 0/22; males: 8/24 Fisher’s exact test; p = 0.02). The remaining 8 male helpers and 16 female helpers most likely did not survive between years.
Discussion
In cooperative breeders, understanding dispersal decisions and their fitness consequences is essential to explain why individuals continue to stay and help raising offspring of others instead of dispersing early to breed on their own (Clutton-Brock et al., 2002; Koenig and Dickinson, 2016; Suh et al., 2020). Our results show that in N. savoryi different routes to independent breeding exist for males and females, which are associated with divergent growth rates and survival probabilities. Helpers of both sexes grow similarly before reaching sexual maturity and start developing functional gonads at a comparable size (Josi et al., 2021). Most females become breeders right after maturation and concomitantly decelerate growth. Males, in contrast, continue to grow after reaching sexual maturity and either remain as subordinates in the harem and help the dominant breeders, or they disperse or get evicted from the territory and become solitary (Figure 2). This was never observed in females and is a risky strategy, exemplified by the reduced survival chances of solitary males.
The first objective of this study was to elucidate how a polygynous mating system relates to sex-specific differences in life-history strategies after reaching sexual maturity. As predicted, females start to reproduce at a younger age than males (Figure 3B). Even though a large body size yields a reproductive advantage due to enhanced fecundity, investing in early reproduction seems to pay off for females (Josi et al., 2020b). This is partly because N. savoryi females generally lay small clutches (Josi et al., 2019) that are probably not overly constrained by a small body size. Furthermore, like many Lake Tanganyika cichlids, N. savoryi has no distinct breeding season (Heg et al., 2005; Josi et al., 2021) and can produce clutches at a high rate (approx. 1 per month under good conditions). These conditions diminish the trade-off between investment in growth versus reproduction (Josi et al., 2019). Therefore, the early attainment of breeding status by females may not be associated with high costs. In contrast, the polygynous mating system involves enhanced competition for breeding territories among males (cf. Limberger, 1983; Tanaka et al., 2015; Jungwirth et al., 2016), which selects for large size in order to gain competitive superiority. This comes at the cost of a delayed onset of reproduction, long after reaching sexual maturity. Indeed, our results show that males reached a breeder position at larger body size and more than twice the age of females.
Overall, our results do not indicate sex-dependent survival differences, but we found that breeder males were more philopatric than females after establishing a territory; 85% of the dispersal events of breeders occurred in females, which dispersed in 91% of these cases to a territory owned by a lager male. Breeder females may benefit from dispersing to a larger territory owner in multiple ways: First, they might gain genetic benefits for their offspring by pairing up with a high-quality male (Andersson, 1994). Second, they might gain increased predator protection from larger male breeders (Wiegmann and Baylis, 1995). These results are corroborated by molecular data suggesting that N. savoryi males keep their established breeding territory for longer compared to breeder females (Josi et al., 2021).
Once a breeding position is obtained, the growth rate strongly declines in both sexes, apparently reflecting a trade-off between investing energy in growth or reproduction. This seems to be at odds with some other cooperatively breeding vertebrates, where growth rate was shown to increase after a breeding position was reached (Russell et al., 2004; Heg, 2010; Young and Bennett, 2010), which in turn may increase fertility or reinforce status (Clutton-Brock et al., 2006). In N. savoryi, starting to breed independently may be particularly costly, as at this stage helpers are often not present. Hence, costly duties such as digging out a breeding chamber and defending against predators and competitors are carried out exclusively by the breeders when establishing a new territory (Josi et al., 2020a,b). This may require that the available energy resources are primarily invested in reproductive purposes instead of growth, which might explain the outlined difference of growth patterns compared to other cooperatively breeding vertebrates. In summary the polygynous mating pattern of N. savoryi can explain important life history parameters such as growth and the age at start of breeding, which relates to sex-specific dispersal decisions.
Our second question concerned the importance of cooperative brood care for sex-specific life history trajectories. The data suggest that in N. savoryi, male helpers delay dispersal and provide alloparental care for a longer period than female helpers, which obtain a breeder position earlier. This corresponds with findings in cooperatively breeding birds, where males remain in the safe natal territory to grow until reaching a competitive size, while at the same time providing alloparental care (Pruett-Jones and Lewis, 1990; Cockburn et al., 2017). However, staying for a prolonged time comes with various costs, including the costly help itself (Taborsky and Grantner, 1998), the investment for acquiring a breeder position and the prolonged non-reproductive period. Hence alternative routes to independent breeding may evolve in males. Indeed, our results show a sex difference regarding the transition from helper to solitary status. While 38% of male helpers became solitary between years, this status is apparently never adopted by females. The alternative male trajectories have important implications for survival, as solitary males survived less likely than males living in groups (Figure 4). Also in other cooperatively breeding cichlids subordinates leaving their home territory face reduced survival chances due to lacking group protection (Taborsky, 1984; Heg et al., 2004; Tanaka et al., 2016). This prompts the question why male helpers leave their safe natal territory at all. If the solitary phase of subordinate males was a self-chosen strategy, the higher mortality risk should be compensated by future fitness benefits, such as enhanced growth or a higher probability to take over a harem. However, being solitary did not affect growth rate, which was comparable to subordinate males and breeder males. In addition, there was no indication that solitary males became breeders with a higher likelihood than subordinate helpers (Tables 2, 3). Therefore, it seems that leaving the home territory to switch to a solitary state reflects a best-of-a-bad-job strategy. This may result from reproductive competition with the harem owner, which may cause enhanced aggression of the latter, similar to other cooperatively breeding cichlids (Taborsky, 1985; Skubic et al., 2004; Mitchell et al., 2009). For subordinate male helpers, either expulsion from the territory or deliberate dispersal may be the consequence (Dierkes et al., 1999). Similarly, costly sex-specific life history trajectories have been observed also in other cooperative breeders. In pied babblers (Turdoides bicolor), for example, solitary males suffer from a continuous body mass loss compared to group members. In consequence, solitary floaters are unable to successfully compete for breeding positions (Ridley et al., 2008). Such sex-specific differences in life-histories and routes to independent breeding suggest that understanding the intrinsic attributes and specific ecology of a species is essential to grasp the evolutionary mechanisms responsible for delayed dispersal and alloparental care. Unfortunately, for many cooperative breeders such data are scarce.
To conclude, this study shows that in N. savoryi differences in dispersal decisions between and within the sexes are tightly linked to divergent life history trajectories, including different growth rates, the age at obtaining a breeder status, and survival. Apparently, dispersal is constrained by high levels of predation, and especially males benefit from philopatry through increased survival chances. We suggest that for a better understanding of the evolution of cooperative breeding and dispersal decisions, future studies should investigate the routes to independent breeding by incorporating individual life-history decisions and their associated costs and benefits, as this may elucidate alternative sex- and status-dependent pathways that are of importance for the development of complex social systems (Kingma et al., 2016).
Data Availability Statement
The raw data supporting the conclusions of this article will be made available by the authors, without undue reservation.
Ethics Statement
Ethical review and approval was not required for this study in accordance with the local legislation and institutional requirements.
Author Contributions
DJ, HT, and JFr planned the study. MT and JFr organized the funding. DJ, HT, JFl, and MR-C collected the data. DJ analysed the data and drafted the manuscript, which was revised by MT and JFr. DJ, JFl, MR-C, MT, and JFr approved the submitted version of the manuscript. All authors contributed to the article and approved the submitted version.
Funding
This study was supported by SNF-grants 31003A_156152 and 31003A_176174 to MT, and 31003A_166470 and 310030_185021 to JFr.
Conflict of Interest
The authors declare that the research was conducted in the absence of any commercial or financial relationships that could be construed as a potential conflict of interest.
Publisher’s Note
All claims expressed in this article are solely those of the authors and do not necessarily represent those of their affiliated organizations, or those of the publisher, the editors and the reviewers. Any product that may be evaluated in this article, or claim that may be made by its manufacturer, is not guaranteed or endorsed by the publisher.
Acknowledgments
We thank Celestine and the late Augustin Mwewa and their team for their extensive aid at the Tanganyika Science Lodge. We are grateful to the Department of Fisheries, Ministry of Agriculture and Livestock, Republic of Zambia for the permission to conduct this work, and especially Taylor Banda and Lawrence Makasa for their ongoing support. We are grateful to the referees for their thoughtful comments on the first draft of the manuscript.
References
Badyaev, A. V. (2002). Growing apart: an ontogenetic perspective on the evolution of sexual size dimorphism. Trends Ecol. Evol. 17, 369–378. doi: 10.1016/s0169-5347(02)02569-7
Bates, D., Maechler, M., and Bolker, B. (2013). lme4: Linear Mixed-Effects Models Using S4 Classes. R Packag. Version 1.1-7. Available online at: https://cran.r-project.org/web/packages/lme4/ (accessed June 22, 2021).
Bonte, D., Van Dyck, H., Bullock, J. M., Coulon, A., Delgado, M. M., Gibbs, M., et al. (2012). Costs of dispersal. Biol. Rev. 87, 290–312.
Clobert, J., Baguette, M., Benton, T. G., and Bullock, J. M. (2012). Dispersal Ecology and Evolution, First Edit Edn. Oxford: Oxford University Press.
Clutton-Brock, T. H., Hodge, S. J., Spong, G., Russell, A. F., Jordan, N. R., Bennett, N. C., et al. (2006). Intrasexual competition and sexual selection in cooperative mammals. Nature 444, 1065–1068.
Clutton-Brock, T. H., Russell, A. F., Sharpe, L. L., Young, A. J., Balmforth, Z., and McIlrath, G. M. (2002). Evolution and development of sex differences in cooperative behavior in meerkats. Science 297, 253–256. doi: 10.1126/science.1071412
Cockburn, A., Hatchwell, B. J., and Koenig, W. D. (2017). “Sociality in birds,” in Comparative Social Evolution, eds D. R. Rubenstein and P. Abbot (Cambridge: Cambridge University Press), 320–353. doi: 10.1017/9781107338319.012
Devillard, S., Allainé, D., Gaillard, J. M., and Pontier, D. (2004). Does social complexity lead to sex-biased dispersal in polygynous mammals? A test on ground-dwelling sciurids. Behav. Ecol. 15, 83–87. doi: 10.1093/beheco/arg099
Dierkes, P., Taborsky, M., and Kohler, U. (1999). Reproductive parasitism of broodcare helpers in a cooperatively breeding fish. Behav. Ecol. 10, 510–515. doi: 10.1093/beheco/10.5.510
Eikenaar, C., Richardson, D. S., Brouwer, L., Bristol, R., and Komdeur, J. (2009). Experimental evaluation of sex differences in territory acquisition in a cooperatively breeding bird. Behav. Ecol. 20, 207–214. doi: 10.1093/beheco/arn136
Emlen, S. (1982). The evolution of helping. I. An ecological constraints model. Am. Nat. 119, 29–39. doi: 10.1086/283888
Emlen, S. T., and Oring, L. W. (1977). Ecology, sexual selection, and the evolution of mating systems. Science 197, 215–223. doi: 10.1126/science.327542
Greenwood, P. J. (1980). Mating systems, philopatry and dispersal in birds and mammals. Anim. Behav. 28, 1140–1162.
Hamilton, I. M., and Heg, D. (2008). Sex differences in the effect of social status on the growth of subordinates in a cooperatively breeding cichlid. J. Fish Biol. 72, 1079–1088. doi: 10.1111/j.1095-8649.2007.01787.x
Hamilton, W. D., and May, R. M. (1977). Dispersal in stable habitats. Nature 269, 578–581. doi: 10.1038/269578a0
Hatchwell, B. J., and Komdeur, J. (2000). Ecological constraints, life history traits and the evolution of cooperative breeding. Anim. Behav. 59, 1079–1086. doi: 10.1006/anbe.2000.1394
Heg, D. (2010). Status-dependent and strategic growth adjustments in female cooperative cichlids. Behav. Ecol. Sociobiol. 64, 1309–1316. doi: 10.1007/s00265-010-0945-9
Heg, D., Bachar, Z., Brouwer, L., and Taborsky, M. (2004). Predation risk is an ecological constraint for helper dispersal in a cooperatively breeding cichlid. Proc. R. Soc. B 271, 2367–2374. doi: 10.1098/rspb.2004.2855
Heg, D., Bachar, Z., and Taborsky, M. (2005). Cooperative breeding and group structure in the Lake Tanganyika cichlid Neolamprologus savoryi. Ethology 111, 1017–1043. doi: 10.1111/j.1439-0310.2005.01135.x
Heinsohn, R., and Legge, S. (1999). The cost of helping. Trends Ecol. Evol. 14, 53–57. doi: 10.1080/0300443880390105
Hernaman, V., and Munday, P. L. (2005). Life-history characteristics of coral reef gobies. II. Mortality rate, mating system and timing of maturation. Mar. Ecol. Prog. Ser. 290, 223–237. doi: 10.3354/meps290223
Huk, T., and Winkel, W. (2006). Polygyny and its fitness consequences for primary and secondary female pied flycatchers. Proc. R. Soc. B 273, 1681–1688. doi: 10.1098/rspb.2006.3485
Jetz, W., and Rubenstein, D. R. (2011). Environmental uncertainty and the global biogeography of cooperative breeding in birds. Curr. Biol. 21, 72–78. doi: 10.1016/j.cub.2010.11.075
Johnstone, R. A., and Cant, M. A. (1999). Reproductive skew and the threat of eviction: a new perspective. Proc. R. Soc. B 266:275. doi: 10.1098/rspb.1999.0633
Josi, D., Heg, D., Takeyama, T., Bonfils, D., Konovalov, D. A., Frommen, J. G., et al. (2021). Age- and sex-dependent variation in relatedness corresponds to reproductive skew, territory inheritance and workload in cooperatively breeding cichlids. Evolution doi: 10.1111/evo.14348 [Epub ahead of print].
Josi, D., Taborsky, M., and Frommen, J. G. (2019). First field evidence for alloparental egg care in cooperatively breeding fish. Ethology 125, 164–169.
Josi, D., Freudiger, A., Taborsky, M., and Frommen, J. G. (2020a). Experimental predator intrusions in a cooperative breeder reveal threat-dependent task partitioning. Behav. Ecol. 31, 1369–1378. doi: 10.1093/beheco/araa094
Josi, D., Taborsky, M., and Frommen, J. G. (2020b). Investment of group members is contingent on helper number and the presence of young in a cooperative breeder. Anim. Behav. 160, 35–42.
Jungwirth, A., Brena, P. F., Keller, I., and Taborsky, M. (2016). Polygyny affects paternal care, but not survival, pair stability, and group tenure in a cooperative cichlid. Behav. Ecol. 27, 592–600. doi: 10.1093/beheco/arv194
Kingma, S. A., Bebbington, K., Hammers, M., Richardson, D. S., and Komdeur, J. (2016). Delayed dispersal and the costs and benefits of different routes to independent breeding in a cooperatively breeding bird. Evolution 70, 2595–2610. doi: 10.1111/evo.13071
Kingma, S. A., Bebbington, K., Teunissen, N., Peters, A., and Komdeur, J. (2021). The evolution of delayed dispersal and different routes to breeding in social birds. Adv. Stud. Behav. 53, 163–224. doi: 10.1016/bs.asb.2021.03.003
Kingma, S. A., Santema, P., Taborsky, M., and Komdeur, J. (2014). Group augmentation and the evolution of cooperation. Trends Ecol. Evol. 29, 476–484. doi: 10.1016/j.tree.2014.05.013
Koenig, W. D., and Dickinson, J. L. (eds.). (2016). Cooperative Breeding in Vertebrates: Studies of Ecology, Evolution, and Behavior. Cambridge: Cambridge University Press. doi: 10.1017/CBO9781107338357
Kokko, H., and Ekman, J. (2002). Delayed dispersal as a route to breeding: territorial inheritance, safe havens, and ecological constraints. Am. Nat. 160, 468–484. doi: 10.2307/3079235
Kokko, H., and Jennions, M. D. (2008). Parental investment, sexual selection and sex ratios. J. Evol. Biol. 21, 919–948. doi: 10.1111/j.1420-9101.2008.01540.x
Komdeur, J. (1992). Importance of habitat saturation and territory quality for evolution of cooperative breeding in the Seychelles warbler. Nature 358, 493–495. doi: 10.1038/358493a0
Leadbeater, E., Carruthers, J. M., Green, J. P., Rosser, N. S., and Field, J. (2011). Nest inheritance is the missing source of direct fitness in a primitively eusocial insect. Science 333, 874–876. doi: 10.1126/science.1205140
Li, X. Y., and Kokko, H. (2019). Sex-biased dispersal: a review of the theory. Biol. Rev. 94, 721–736. doi: 10.1111/brv.12475
Limberger, D. (1983). Pairs and harems in a cichlid fish, Lamprologus brichardi. Z. Tierpsychol. 62, 115–144. doi: 10.1111/j.1439-0310.1983.tb02146.x
Mabry, K. E., Shelley, E. L., Davis, K. E., Blumstein, D. T., and van Vuren, D. H. (2013). Social mating system and sex-biased dispersal in mammals and birds: a phylogenetic analysis. PLoS One 8:e57980. doi: 10.1371/journal.pone.0057980
McNamara, J. M., Székely, T., Webb, J. N., and Houston, A. I. (2000). A dynamic game-theoretic model of parental care. J. Theor. Biol. 205, 605–623. doi: 10.1006/jtbi.2000.2093
Mitchell, J. S., Jutzeler, E., Heg, D., and Taborsky, M. (2009). Gender differences in the costs that subordinate group members impose on dominant males in a cooperative breeder. Ethology 115, 1162–1174. doi: 10.1111/j.1439-0310.2009.01705.x
Pakanen, V. M., Koivula, K., Orell, M., Rytkönen, S., and Lahti, K. (2016). Sex-specific mortality costs of dispersal during the post-settlement stage promote male philopatry in a resident passerine. Behav. Ecol. Sociobiol. 70, 1727–1733. doi: 10.1007/s00265-016-2178-z
Perrin, N., and Mazalov, V. (2000). Local competition, inbreeding, and the evolution of sex-biased dispersal. Am. Nat. 155, 116–127. doi: 10.2307/3079020
Promislow, D. E. L., Montgomerie, R., and Martin, T. E. (1992). Mortality costs of sexual dimorphism in birds. Proc. R. Soc. B 250, 143–150. doi: 10.1098/rspb.1992.0142
Pruett-Jones, S. G., and Lewis, M. J. (1990). Sex ratio and habitat limitation promote delayed dispersal in superb fairy-wrens. Nature 348, 541–542. doi: 10.1038/348541a0
R Core Team. (2017). R: A Language and Environment for Statistical Computing. Vienna: R Foundation for Statistical Computing.
Ridley, A. R., Raihani, N. J., and Nelson-Flower, M. J. (2008). The cost of being alone: the fate of floaters in a population of cooperatively breeding pied babblers Turdoides bicolor. J. Avian Biol. 39, 389–392.
Rubenstein, D. R. (2011). Spatiotemporal environmental variation, risk aversion, and the evolution of cooperative breeding as a bet-hedging strategy. Proc. Natl. Acad. Sci. U. S. A. 108(Suppl.), 10816–10822. doi: 10.1073/pnas.1100303108
Rubenstein, D. R., and Abbot, P. (2017). Comparative Social Evolution. Cambridge: Cambridge University Press.
Russell, A. F., Carlson, A. A., McIlrath, G. M., Jordan, N. R., and Clutton-Brock, T. (2004). Adaptive size modification by dominant female meerkats. Evolution 58, 1600–1607. doi: 10.1554/03-480
Skubic, E., Taborsky, M., McNamara, J. M., and Houston, A. I. (2004). When to parasitize? A dynamic optimization model of reproductive strategies in a cooperative breeder. J. Theor. Biol. 227, 487–501. doi: 10.1016/j.jtbi.2003.11.021
Stacey, P., and Ligon, J. (1991). The benefits-of-philopatry hypothesis for the evolution of cooperative breeding: variation in territory quality and group size effects. Am. Nat. 137, 831–846. doi: 10.1086/285196
Stiver, K. A., Desjardins, J. K., Fitzpatrick, J. L., Neff, B., Quinn, J. S., and Balshine, S. (2007). Evidence for size and sex−specific dispersal in a cooperatively breeding cichlid fish. Mol. Ecol. 16, 2974–2984. doi: 10.1111/j.1365-294x.2007.03350.x
Stiver, K. A., Fitzpatrick, J., Desjardins, J. K., and Balshine, S. (2006). Sex differences in rates of territory joining and inheritance in a cooperatively breeding cichlid fish. Anim. Behav. 71, 449–456. doi: 10.1016/j.anbehav.2005.06.011
Suh, Y. H., Pesendorfer, M. B., Tringali, A., Bowman, R., and Fitzpatrick, J. W. (2020). Investigating social and environmental predictors of natal dispersal in a cooperative breeding bird. Behav. Ecol. 31, 692–701. doi: 10.1093/beheco/araa007
Székely, T., Weissing, F. J., and Komdeur, J. (2014). Adult sex ratio variation: implications for breeding system evolution. J. Evol. Biol. 27, 1500–1512. doi: 10.1111/jeb.12415
Taborsky, M. (1984). Broodcare helpers in the cichlid fish Lamprologus brichardi: their costs and benefits. Anim. Behav. 32, 1236–1252. doi: 10.1016/s0003-3472(84)80241-9
Taborsky, M. (1985). Breeder-helper conflict in a cichlid fish with broodcare helpers: an experimental analysis. Behaviour 95, 45–75. doi: 10.1163/156853985x00046
Taborsky, M. (2009). “Reproductive skew in cooperative fish groups: virtue and limitations of alternative modeling approaches,” in Reproductive Skew in Vertebrates: Proximate and Ultimate Causes, eds R. Hager and C. Jones (Cambridge: Cambridge University Press), 265–304. doi: 10.1017/cbo9780511641954.012
Taborsky, M. (2016). “Cichlid fishes: a model for the integrative study of social behavior,” in Cooperative Breeding in Vertebrates, eds W. D. Koenig and J. L. Dickinson (Cambridge: Cambridge University Press), 272–293. doi: 10.1017/cbo9781107338357.017
Taborsky, M., and Grantner, A. (1998). Behavioural time–energy budgets of cooperatively breeding Neolamprologus pulcher (Pisces: Cichlidae). Anim. Behav. 56, 1375–1382. doi: 10.1006/anbe.1998.0918
Tanaka, H., Frommen, J. G., Takahashi, T., and Kohda, M. (2016). Predation risk promotes delayed dispersal in the cooperative breeding cichlid Neolamprologus obscurus. Anim. Behav. 117, 51–58. doi: 10.1016/j.anbehav.2016.04.019
Tanaka, H., Heg, D., Takeshima, H., Takeyama, T., Awata, S., Nishida, M., et al. (2015). Group composition, relatedness, and dispersal in the cooperatively breeding cichlid Neolamprologus obscurus. Behav. Ecol. Sociobiol. 69, 169–181. doi: 10.1007/s00265-014-1830-8
Trochet, A., Courtois, E. A., Stevens, V. M., Baguette, M., Chaine, A., Schmeller, D. S., et al. (2016). Evolution of sex-biased dispersal. Q. Rev. Biol. 91, 297–320.
Warner, R. R. (1984). Deferred reproduction as a response to sexual selection in a coral reef fish: a test of the life historical consequences. Evolution 38, 148–162. doi: 10.2307/2408554
Webster, M. S. (1991). Male parental care and polygyny in birds. Am. Nat. 137, 274–280. doi: 10.1086/285161
Wiegmann, D. D., and Baylis, J. R. (1995). Male body size and paternal behaviour in smallmouth bass, Micropterus dolomieui (Pisces: Centrarchidae). Anim. Behav. 50, 1543–1555. doi: 10.1016/0003-3472(95)80010-7
Keywords: benefits-of-philopatry, polygamy, delayed dispersal, territory inheritance, cooperation, cichlid fish, Neolamprologus savoryi, ecological constraints
Citation: Josi D, Flury JM, Reyes-Contreras M, Tanaka H, Taborsky M and Frommen JG (2021) Sex-Specific Routes to Independent Breeding in a Polygynous Cooperative Breeder. Front. Ecol. Evol. 9:750483. doi: 10.3389/fevo.2021.750483
Received: 30 July 2021; Accepted: 20 September 2021;
Published: 21 October 2021.
Edited by:
Olivia Roth, GEOMAR Helmholtz Center for Ocean Research Kiel, GermanyReviewed by:
Arrilton Araujo, Federal University of Rio Grande do Norte, BrazilCarl Soulsbury, University of Lincoln, United Kingdom
Copyright © 2021 Josi, Flury, Reyes-Contreras, Tanaka, Taborsky and Frommen. This is an open-access article distributed under the terms of the Creative Commons Attribution License (CC BY). The use, distribution or reproduction in other forums is permitted, provided the original author(s) and the copyright owner(s) are credited and that the original publication in this journal is cited, in accordance with accepted academic practice. No use, distribution or reproduction is permitted which does not comply with these terms.
*Correspondence: Joachim G. Frommen, ai5mcm9tbWVuQG1tdS5hYy51aw==
†Deceased