- 1Department of Biomedical Sciences, Section of Physiology, University of Cagliari, Cagliari, Italy
- 2Department of Life and Environmental Sciences, University of Cagliari, Cagliari, Italy
Like other animals, echinoderms rely on chemical senses to detect and localize food resources. Here, we evaluate the chemical sensitivity of the sea urchin Paracentrotus lividus to a number of stimuli possibly related to food, such as a few sugars, compared to the blue-green algae Spirulina (Arthrospira platensis). To do this we developed a simple, innovative method based on the recording of “urchinograms” estimating the movements of spines, pedicellariae, tube feet, and eventually of the whole sea urchin, in response to chemicals, while keeping both the whole animal and the stimulus in their natural environment, underwater. Our results show that Spirulina is a highly stimulating compound for the sea urchin, by acting in a dose-dependent manner. The animals resulted also sensitive, even if to a lesser extent, to some sugars, such as the monosaccharide glucose, but not to its isomer fructose, while among disaccharides, they sensed cellobiose, but not sucrose or trehalose. From an applied point of view, any insight into the chemical sensitivity of sea urchins toward potential food-related compounds may lead to the discovery of key chemicals that would help improve the efficiency and reduce the costs of dietary substrates for optimization of intensive rearing strategies. Although this method has been developed for P. lividus, it will be suitable to evaluate the chemical sensitivity of other echinoderms and other marine invertebrates characterized by low mobility.
Introduction
The ability of animals to perceive and respond to chemical cues is essential in all environments, including aquatic habitats, where changes in behavior after detection of waterborne molecules have been extensively documented for many species and in many behavioral contexts (Bargmann, 2006; Kamio and Derby, 2017; Mollo et al., 2017).
Like other echinoderms, sea urchins are slow-moving, broadcast spawning marine invertebrates which rely on chemical signals to produce appropriate behavioral responses, such as avoidance of predators or damaged conspecifics, spatial orientation, identification of suitable habitats, localization of potential food sources and conspecific mates (for a review, see Lawrence, 2013). Pioneering studies have shown that crushed sea urchins and nearby predators may trigger activation of spines, tube feet and pedicellariae in conspecifics (Snyder and Snyder, 1970; Campbell, 1983). Besides, it is known that Strongylocentrotus sp. is attracted by algae recognized as food (Vadas, 1977), while the echinoid Lytechinus variegatus can orient to chemicals emanating from potential food resources over distance even under turbulent water flow conditions (Pisut, 2004). Sea urchins have been reported to respond to distant feeding stimuli with upstream orientation, using odor-guided rheotaxis for chemtrail navigation and odor source localization (Atema, 2012). In this respect, their slow speed may facilitate temporal sampling of chemical cues, and the widely distributed array of chemosensory organs may enhance spatial resolution (Weissburg, 2000).
Sea urchins also use chemicals in the fine-tuning of breeding aggregations and spawning synchrony strategies, in order to increase the probability of gamete encounter in animals with external fertilization (Mercier and Hamel, 2009; Reuter and Levitan, 2010). Despite the broad chemical sensitivity of both larvae and adults of sea urchins, chemoreceptive organs have not been identified with certainty yet. On the basis of behavioral and histological studies, three main systems have been reported to respond to chemical cues: the “spine system,” the tube feet and the pedicellariae, with responses ranging from simple, local reflex reactions of these systems to a fully coordinated chemotaxis in which the whole animal moves toward or away from a stimulus source (Sloan and Campbell, 1982; Campbell et al., 2001). Sea urchin chemoreceptors belong to the family of the G-protein-coupled receptors (GPCRs) and their high number - up to several hundreds - is comparable with that identified in many other animals, thus suggesting that sea urchins possess a sophisticated chemosensory system (Burke et al., 2006; Raible et al., 2006).
The common sea urchin Paracentrotus lividus (Lamarck, 1816) (Echinoidea: Parechinidae) is a regular, edible echinoid, which is very widespread throughout the Mediterranean coasts. The species is a key herbivore of the shallow subtidal rocky habitats, playing a central role in the marine trophic cascade (Micheli et al., 2005; Giakoumi et al., 2012). Its high-density populations, due to the lack of natural predators (for example in overexploited areas), can have dramatic effects on rocky macroalgal communities, producing barren grounds (Guidetti and Dulcić, 2007; Gianguzza et al., 2011; Boada et al., 2017). On the other hand, P. lividus is the main echinoid consumed in Mediterranean and Atlantic Europe (FAO, 2015). In these regions unregulated harvesting pressures on the wild stock, has caused a drastic reduction of populations that cannot recover on a yearly base (Pais et al., 2007; Ceccherelli et al., 2011; Addis et al., 2012; Carboni et al., 2014; FAO, 2020) and some regions are currently experimenting restoking plans using reared juveniles P. lividus from experimental hatcheries (Giglioli et al., 2021). Only a few studies attribute the loss of the species to global warming and scarcity of seaweed (Yeruham et al., 2015, 2019). Aquaculture is probably the best way to satisfy the increasing demand and many efforts are currently in progress in the attempt to optimize formulation or integration of effective feeds able to guarantee fast growth of adults and gonadal maturation.
Spirulina is a blue-green filamentous cyanobacteria with a growth rate faster than most other plants (Shay, 1993), that contains high-quality protein content (Velasquez et al., 2016) and bioactive components such as essential fatty acids, vitamins, minerals and pigments with antioxidant properties (Vonshak, 1997). Spirulina has been recently considered as a potential integrator in the formulation of fish feeds and enhances the growth performance of various fish species depending on the amount in the diets (Zhang et al., 2020). Despite Spirulina is not a natural food item for P. lividus, this valuable compound can be a potential integrator of prepared diets for sea urchins.
Based on these considerations, in this study we evaluated the chemical sensitivity of the sea urchin P. lividus to a set of stimuli possibly related to food, such as a few sugars, compared to the blue-green algae Spirulina. To do this we developed a simple, but innovative method, based on the recording of an “urchinogram” that estimates the movements of spines, pedicellariae, tube feet, and eventually of the whole sea urchin, in response to chemicals, while keeping both the entire animal and the stimulus in their natural environment, underwater.
From an applied point of view, any insight into the chemosensitivity of the sea urchin toward potential food-related compounds may lead to the discovery of key chemicals that would help improve the efficiency and reduce the costs of dietary substrates for the optimization of intensive rearing strategies. Even if this method has been developed on the sea urchin P. lividus, it can be easily adapted to other echinoderms or marine invertebrates of commercial interests.
Methods
Animal Collection and Rearing Conditions
Sea urchins used in the trials were collected from the batch of juveniles produced in the hatchery of the University of Cagliari (Italy), cultured using the methods described by Carboni et al. (2014) and Hannon et al. (2017). For the experiments, specimens of about 30 mm test in diameter were selected, corresponding to the third age class.
Sea Urchin Bioassay
Sea urchins were individually exposed to test compounds in a small, rectangular Plexiglas® tank (12.5 cm long × 7 cm wide × 7 cm high; Figure 1), containing about 350 mL of seawater (SW; 18–19°C, water depth ∼4 cm). The tank was connected, by way of two flexible plastic tubes (length 40 cm, internal diameter 0.4 cm) opening at its two opposite short sides, to two different channels of a peristaltic pump (Gilson, Minipuls Evolution®) operating at a flow rate of 10 ml/min and thus ensuring a constant flow within the tank. The two tubes acted as the inflow and outflow terminals through which SW and chemical stimuli could be, respectively, delivered into and removed from the tank. The outflow terminal was connected to a system for waste collection. At the beginning of each test, animals were immersed in the experimental tank and allowed to acclimatize until becoming motionless, typically within 15 min. Before the stimulus was supplied, the response of each animal to the same aliquot of SW (blank control) was monitored for 5 min.
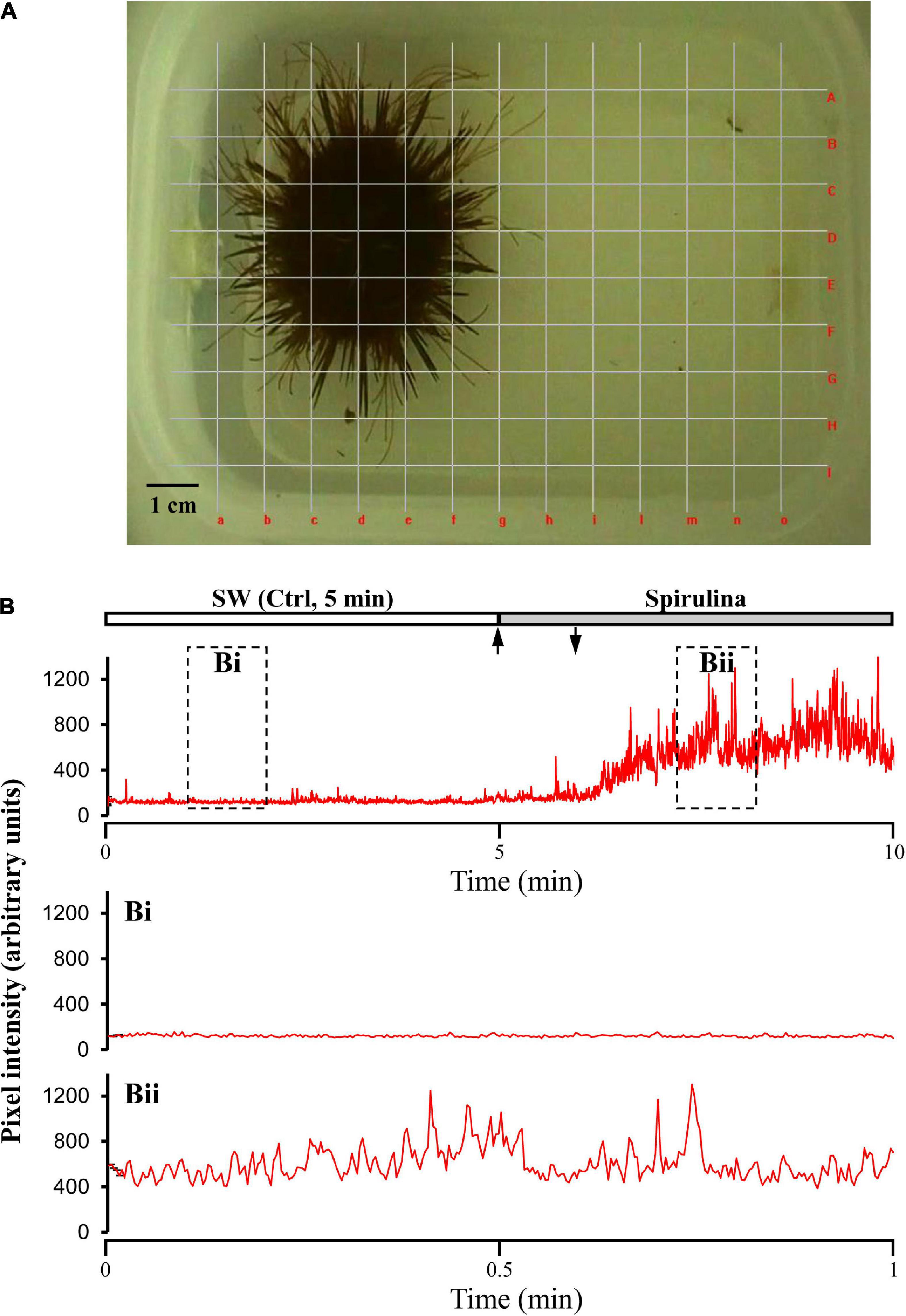
Figure 1. Recording layout of the sea urchin movements. (A) Video photogram showing the experimental tank with the superimposed grid, composed by 13 vertical (a–o) + 9 horizontal (A–I) evenly spaced lines, used to cover the entire area of sea urchin activity. (B) Sample recording of an “urchinogram,” comprising all summated visible movements of spines, pedicellariae, tube feet, and eventually of the whole sea urchin, in response to the blue-green algae Spirulina. Movements were estimated by changes in the mean squared difference in light intensity between successive frames determined by means of the Aviline software analysis (Data were recorded at 5 frames/s). Arrows indicate the time of stimulus supply. Bi and Bii represent the two enlarged portions of the recording within the dashed rectangles.
Stimuli were added to the tank for 1 min by switching the inflow terminal from SW to a different reservoir and each sea urchin was allowed 4 min to respond, starting from the time the stimulus entered the experimental tank (typically 45 s after switching). This time frame was selected on the basis of previous observations on dye diffusion in the experimental tank. Dye tests were also performed in order to verify the effectiveness of the perfusion/stimulation device. Trials were video-recorded for later analysis, using a Samsung SMX-F34 (Samsung, Seoul, South Korea) color digital camera mounted above the test tank. Video recordings were analyzed by an independent observer blind to the experimental treatment.
The behavioral response was determined by measuring the movement rate of spines, tube feet and the fully coordinated responses, if any, in which the whole organism moves toward or away from the outlet of stimulus supply. Animals were not fed for 24 h preceding the experiments. At the end of the experiments the sea urchins were returned to the holding tank.
Stimuli and Supply Protocol
The following compounds, most of which already known for their potential feeding significance (Galasso et al., 2018; Casal-Porras et al., 2021), were selected as stimuli: the disaccharides trehalose, cellobiose, and sucrose, the monosaccharides glucose and fructose and the Spirulina Arthrospira platensis (Cyanobacteria). All sugars used were obtained from Sigma-Aldrich (Milan, Italy), while Spirulina was purchased from Livegreen Società Agricola (Italy) as a commercial pellet preparation.
All sugars were first dissolved in SW at 10–1 mol/L and were then stored frozen as stock solutions. On the day of the experiments, stock solutions were thawed and serially diluted in SW to be used at three different concentrations: 10–5, 10–3, and 10–1 mol/L, and, in all cases, were supplied at increasing concentrations. Spirulina was prepared by suspending the finely hashed power in SW at 5 mg/mL and then accurately filtered (Whatman Filter Paper, Sigma-Aldrich, Milan Italy) in order to remove any particulate which could mechanically stimulate the sea urchins. Then, Spirulina was serially diluted in SW to be used at 1, 0.1, and 0.01 mg/mL.
Owing to the procedure adopted for stimulus supply, actual concentrations to which sea urchins were exposed and to which they responded likely may have been less than those indicated, that is, the concentration diffusing from the inflow terminal to the tank containing the sea urchin tank. Therefore, we used a static system in such a way that each sea urchin was exposed to a blank stimulation for 5 min and then to three different increasing concentrations of a same compound (four in the case of Spirulina). During this stimulation sequence, the water was not replaced (i.e., stepwise stimulations were used, according to Solari et al., 2015, 2017a).
Experiments were performed on 15 sea urchins for each tested compound and concentration, except for trehalose (11) and cellobiose (12) and each animal was tested with only one chemical at a time.
Detection of Sea Urchin Movements: The “Urchinogram”
All visible movements of the sea urchin spines and tube feet as well as the fully coordinated responses, when present, of the whole animal within the experimental tank, were captured by video-recordings followed by computer analysis of the movements from frame to frame according to the procedure adopted by Middleton et al. (2006) and Solari et al. (2017b). This approach produces an “urchinogram” in which the movements at several sites and levels on the same animal can be recorded and compared. The video recordings were converted to a resolution of 640 × 480 pixels, at 5 frames/sec (300 frames/min), so that each frame could account for the instantaneous “movement state” of the sea urchin at 200 msec intervals. Each video was analyzed using a custom program (AviLine, 20051) while the computer mouse was used to overlay lines on the video frames so that each line crossed the light/dark boundary between the animal (dark) and the background (clear) (Figure 1). We adopted a grid with a total of 22 (13 vertical + 9 horizontal) evenly spaced lines, in order to cover the entire area of the experimental tank everywhere the animal had moved (Figure 1A). The mean square difference in intensity at each point of the lines in the grid between successive pairs of frames was plotted during the whole experiments. Therefore, the movements of the dark animal on the clear background, generated changes in pixel intensity along the lines, which was used as index of the movement rate of spines, tube feet and locomotion of each sea urchin.
Recording the mean square difference in intensity provides a great sensitivity and also good discrimination of movement, as it takes into consideration the change in every pixel along the line.
This analysis protocol recorded the displacement in the focus plane, but any movement in the vertical direction was not measured. Data were saved in a Microsoft Excel format and mean peak height and intervals between peaks were calculated. For each frame, the sum of values for all lines was calculated, in order to pick all movements of spine, tube feet and whole animal anywhere within the experimental tank. In this way the amplitude of the sea urchin movements could be evaluated before and after supply of the different stimuli. Significant differences were evaluated by means of the paired T-test with a 95% confidence level (p ≤ 0.05).
Results
After being acclimated in the experimental tank, the sea urchins became virtually motionless. In the absence of chemical stimulation, they displayed only a basal, negligible level of activity, usually limited to oscillations of few spines and tube feet. Conversely, in the presence of a stimulating compound, the sea urchins responded by increasing the movement of their spines and tube feet and sometimes this behavior was associated with a fully coordinated locomotion of the animal within the experimental tank and this was considered as an index of its chemical sensitivity.
Among the tested compounds, Spirulina resulted the most stimulating one, affecting the sea urchin motility in a dose-dependent manner. In details, as shown by the urchinograms in Figure 2, at the lowest tested dose (0.01 mg/mL) Spirulina was ineffective (p > 0.05, T-test, n = 15) as compared to the SW control (mean value of square differences in light intensity = 102959 ± 3423, 100% of the response). Conversely, at 0.1 mg/mL the blue-green algae evoked a significant increase in the movement rate of the animals to 133.5 ± 5.5% (p = 0.009, T-test, n = 15) and further increased the sea urchin response to 202.65 ± 9.1% (p = 0.0001, T-test, n = 15) when tested at 1 mg/mL.
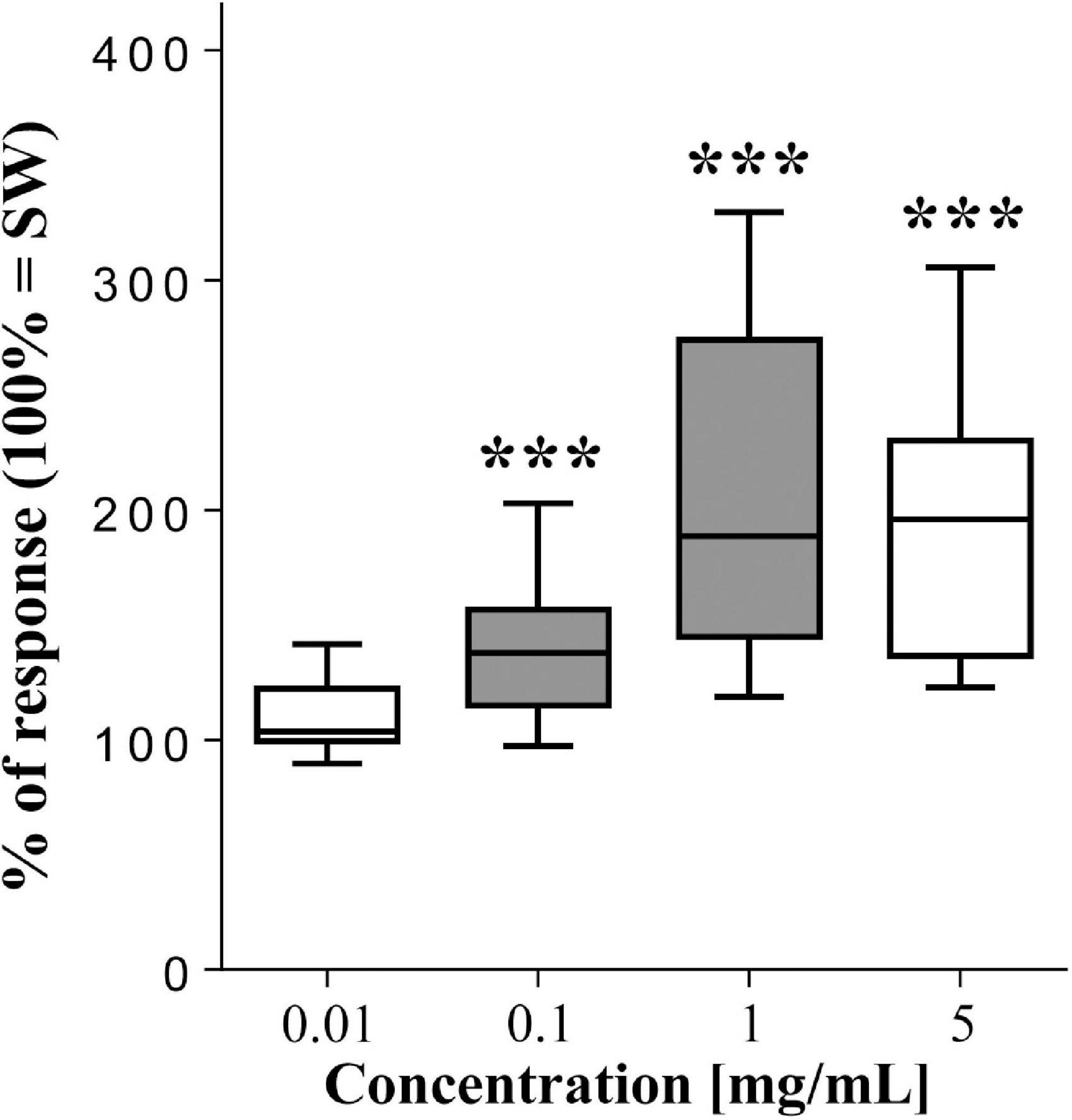
Figure 2. Normalized movement rate of sea urchins, recorded as mean square difference in light intensity (summed peak heights for each preparation during a 2-min stimulation) ± se (vertical bars), following supply of the blue-green algae Spirulina compared to seawater (SW = 100% of response). The top and bottom of the box and whisker plots show the upper and lower quartiles, respectively. The horizontal line in the middle indicates the median of the corresponding distribution, while the minimum and maximum observed values are indicated by the bars connected to the box. ∗∗∗ indicates a significant difference (p < 0.001, T-test) with respect to SW. Filled boxes indicate significant differences between a concentration and the next lower (p < 0.01, T-test). Data were obtained from 15 sea urchins.
At the highest dose (5 mg/mL) the algae enhanced the animal movement rate up to 184.8 ± 7.6% (p = 0.0001, T-test, n = 15) with respect to SW, but this response did not statistically differ from that detected at 1 mg/mL.
In general, the tested monosaccharides resulted less effective than Spirulina, but to different extents (Figure 3). In fact, although the sea urchins were completely insensitive to fructose (p > 0.05, T-test, n = 15), they responded to its isomer glucose, which significantly enhanced the movement rate of the animals to 113.3 ± 4.3% (p = 0.0075, T-test, n = 15), 120.6 ± 6.3% (p = 0.0078, T-test, n = 15), and 136.1 ± 10.4% (p = 0.0044, T-test, n = 15) with respect to the SW control (mean value of square differences in light intensity = 95738 ± 3598) at the three concentration 10–5, 10–3, and 10–1 mol/L, respectively.
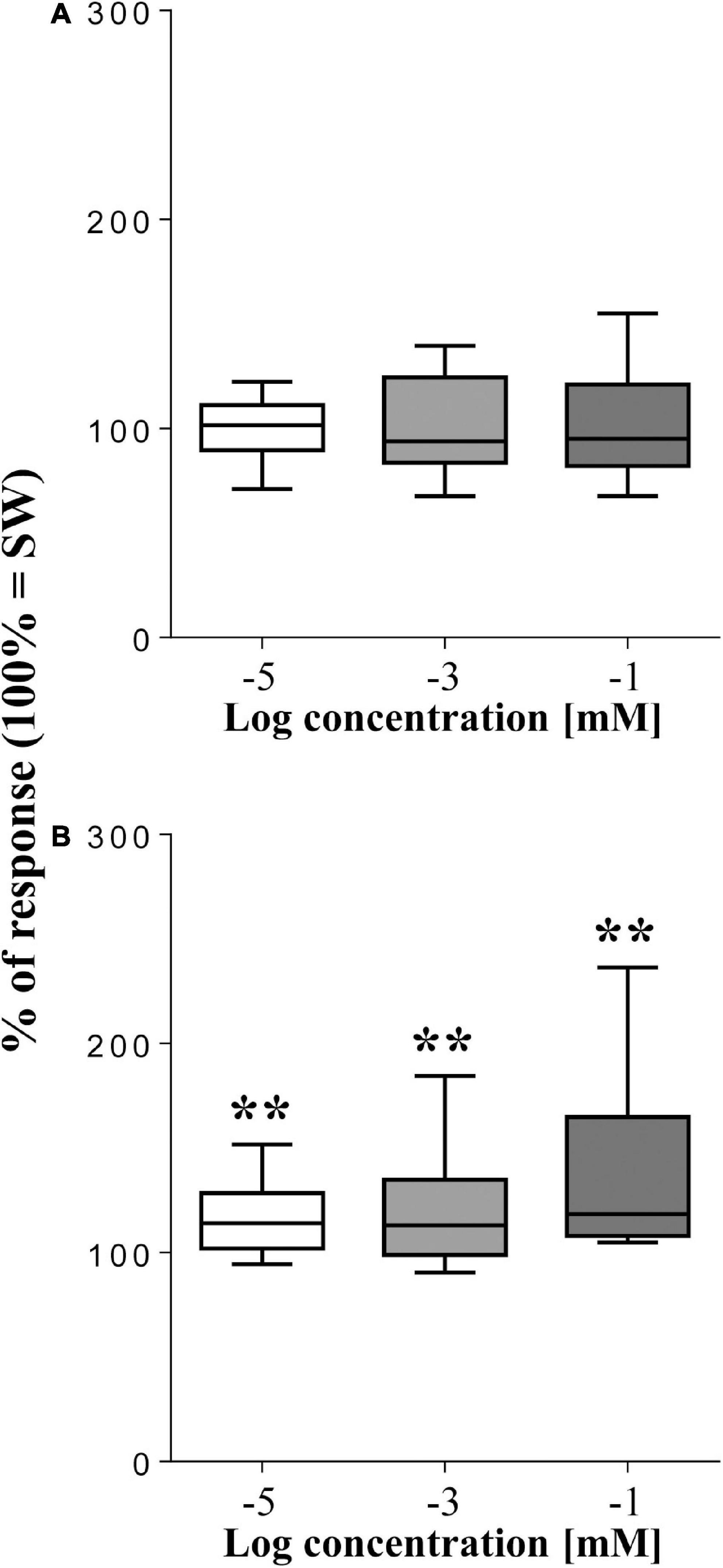
Figure 3. Normalized movement rate of sea urchins, recorded as mean square difference in light intensity (summed peak heights for each preparation during a 2-min stimulation) ± se (vertical bars), following supply of the monosaccharides fructose (A) and glucose (B) compared to seawater (SW = 100% of response). The top and bottom of the box and whisker plots show the upper and lower quartiles, respectively. The horizontal line in the middle indicates the median of the corresponding distribution, while the minimum and maximum observed values are indicated by the bars connected to the box. ∗∗ indicates a significant difference (p < 0.01, T-test) with respect to SW. Data were obtained from 15 sea urchins for both fructose and glucose.
Among the tested disaccharides (Figure 4), only cellobiose resulted effective, but only at the lowest concentration (10–5 mol/L), which enhanced the sea urchin movement rate to 127.6 ± 9.4% (p = 0.0137, T-test, n = 12) compared to SW (mean value of square differences in light intensity = 95738 ± 3598). Conversely, at the two highest concentration cellobiose did not evoke any significant response (p > 0.05, T-test, n = 15). Similarly, both sucrose and trehalose resulted ineffective and never affected the movement rate of the sea urchin, which therefore resulted insensitive to them, regardless of the concentration used.
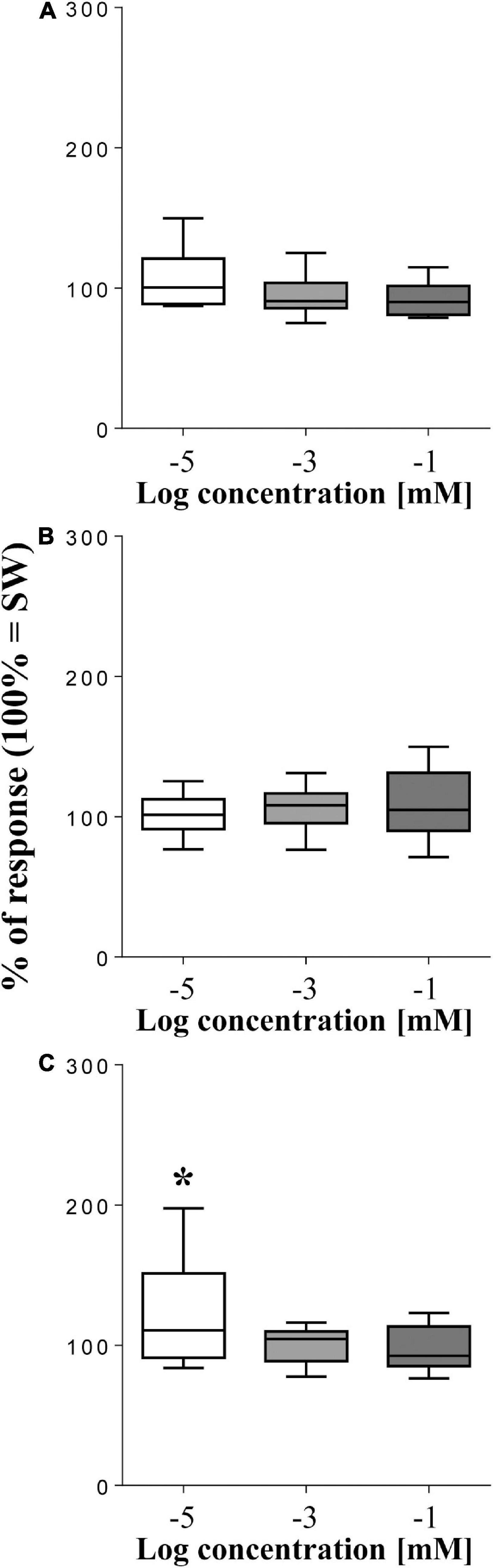
Figure 4. Normalized movement rate of sea urchins, recorded as mean square difference in light intensity (summed peak heights for each preparation during a 2-min stimulation) ± se (vertical bars), following supply of the disaccharides sucrose (A), trehalose (B), and cellobiose (C) compared to seawater (SW = 100% of response). The top and bottom of the box and whisker plots show the upper and lower quartiles, respectively. The horizontal line in the middle indicates the median of the corresponding distribution, while the minimum and maximum observed values are indicated by the bars connected to the box. ∗ indicates a significant difference (p < 0.05, T-test) with respect to SW. Data were obtained from 15 sea urchins for sucrose, 11 for trehalose, and 12 for cellobiose.
Discussion and Conclusion
The present behavioral study extends our knowledge about the chemoreceptive features of sea urchins, by providing data on the ability of P. lividus to detect several food-related compounds. We based on the assumption that the overall movements of spines, pedicellariae, tube feet, and eventually locomotion of the whole animal are classically regarded as a behavioral indicator of chemical detection in sea urchins (Sloan and Campbell, 1982; Campbell et al., 2001). Therefore, in the present study we developed a simple and innovative method, which consists in recording the “urchinograms,” that estimate the movements of the animals in response to chemical stimulation while keeping both the whole sea urchin and the stimulus in their natural environment, underwater.
Our results show that the sea urchins are sensitive to Spirulina Arthrospira platensis (Cyanobacteria) and to a few sugars, such as glucose and cellobiose, that elicit, even though to different extents, an increase in the animal motility. Among the tested compounds, Spirulina resulted the most effective stimulus, acting in a dose-dependent manner within the range 0.01–1 mg/mL and evoking in P. lividus strong responses that were mainly characterized by a robust activation of the spine system and tube feet. Apart from the antioxidant, pain-relief, anti-inflammatory, and brain-protective properties, Spirulina is known as a potent source of nutrients due to its chemical composition, which includes proteins, carbohydrates, essential amino acids, minerals, essential fatty acids, vitamins, and pigments (Gutiérrez-Salmeán et al., 2015). Recent studies on sea urchins confirmed several benefits of Spirulina-enriched diets which improved gonadic growth and gamete production in P. lividus (Cirino et al., 2017) and enhanced the content of astaxanthin, a carotenoid with antioxidant properties and beneficial effects for various degenerative diseases, in the egg of the sea urchin Arbacia lixula (Galasso et al., 2018). Although the present technique does not allow to correlate the animal sensitivity to the attractive degree exerted by the cyanobacteria, such a marked sensitivity of the animal is likely coupled to activation of a search strategy aimed at localizing Spirulina. In our experiments, the sea urchins resulted also sensitive, although to a lesser extent than Spirulina, to some, but not all, sugars. In fact, they responded to the monosaccharide glucose, but not to its isomer fructose. Among the tested disaccharides, the animals were sensitive to the lowest concentration of cellobiose, but they sensed neither sucrose nor trehalose. Like most sea urchins, P. lividus appears to be an opportunistic generalist consumer even though it prefers seaweed and seagrass (Lawrence, 2013). For this reason, a reduced or partial sensitivity to sugars was unexpected as carbohydrates are likely good indicators for a vegetarian diet counterpart (Mohr and Schopfer, 1995). Instead, trehalose represents an anomalous carbohydrate; in fact, although it chemically belongs to the class of the sugars, it represents an indicator of a protein diet, as it is a haemolymph sugar commonly found in the body fluid of invertebrates (Fairbairn, 1958) which belong to the sea urchin diet and are essential to complete the assortment of the ingested nutritional principles (Zupo, 1993). The lack of any concentration-response relationship found in response to glucose also suggests that the sensitivity of P. lividus may not allow resolution of concentration differences as great as 100-fold step concentration increases for the same compound, at least under the experimental conditions (stepwise stimulations) we adopted. Therefore, it is likely that the chemoreceptive apparatus of this animal may act as a detector of relative rather than absolute intensity of a stimulus by resetting the response threshold to a zero-level in the presence of constant background chemical noise, similar to what observed in other animals like crustaceans (Borroni and Atema, 1988; Solari et al., 2017a).
More investigation is needed to discover whether P. lividus is sensitive to a potentially wider range of carbohydrates. It is known that different species of sea urchins may be adapted to local ecological conditions so as to evolve specific dietetic patterns which can also include allochthonous species (Pusceddu et al., 2021), but differences and/or plastic rearrangement in sensitivity may also appear intraspecifically, due to availability and distribution of food resources in a given habitat or depending on changes in life cycle, age, and size of the animal (Lawrence, 2013).
In conclusion, the method here presented for evaluation of chemical sensitivity in sea urchins revealed a high chemosensitivity level of P. lividus to Spirulina and this information gains further importance in the light of the commercial potential of this echinoderm for human consumption and for its growing importance in the aquaculture practise worldwide (Stefánsson et al., 2017). The knowledge on the chemoreceptive abilities of the sea urchin to detect chemicals and the discovery of key compounds with attractant and/or phagostimulant activity might be strategic in developing formulation or supplementation of effective feeds suitable for intensive aquaculture systems. In fact, any formulation of a nutritionally balanced feed will be inappropriate if the animal cannot effectively locate and willingly consume it. For these reasons, further investigations are needed to elucidate the optimal dosage with which Spirulina is detected, in order to act as a phagostimulant in relation to the benefit it may confer in supplemented diets. Eventually, it could be useful to screen among its numerous components those key compounds, if any in particular, which trigger the prompt sensory-motor response in sea urchins, with the aim to limit the water soaking of the prepared diets (Secci et al., 2020). Several studies performed on both larvae and adult of P. lividus have recently focused on fresh and formulated diets in the attempt to increase their nutritional value (Cyrus et al., 2015; Castilla-Gavilán et al., 2018; Prato et al., 2018; Zupo et al., 2019) and a new contribution in this field may rise from the knowledge of their stimulating effectiveness that could also help improving the palatability for animals.
Finally, even if this method has been developed on the sea urchin P. lividus, it can be easily adapted to other echinoderms or marine invertebrates of commercial interests for which the understanding of chemosensitivity is paramount for intensive aquaculture practices.
Data Availability Statement
The raw data supporting the conclusions of this article will be made available by the authors, without undue reservation.
Author Contributions
PS, RC, and PA: conceptualization and resources. PS: investigation. PS, VP, MS, and AG: formal analysis and data curation. PS and PA: supervision and writing – original draft preparation. PS, VP, MS, AG, RC, and PA: writing – review and editing. All the authors reviewed and approved the manuscript.
Funding
This work was supported by a grant from the University of Cagliari (Universitá degli Studi di Cagliari; Fondo Integrativo per la Ricerca, FIR 2019).
Conflict of Interest
The authors declare that the research was conducted in the absence of any commercial or financial relationships that could be construed as a potential conflict of interest.
Publisher’s Note
All claims expressed in this article are solely those of the authors and do not necessarily represent those of their affiliated organizations, or those of the publisher, the editors and the reviewers. Any product that may be evaluated in this article, or claim that may be made by its manufacturer, is not guaranteed or endorsed by the publisher.
Acknowledgments
The authors thank Marco Melis, Department of Biomedical Sciences, University of Cagliari, for his helpful cooperation and technical support in the experimental part of the study. The protocol and procedures are full in accordance with the European Directive 2010/63/EU on the protection of animals used for scientific purposes.
Supplementary Material
The Supplementary Material for this article can be found online at: https://www.frontiersin.org/articles/10.3389/fevo.2021.749493/full#supplementary-material
Supplementary Video 1 | Video recording of an integral 10-min experiment (8 × speed) showing the enhancement of the movement rate of the sea urchin following Spirulina supply as compared to seawater (control).
Footnotes
References
Addis, P., Secci, M., Angioni, A., and Cau, A. (2012). Spatial distribution patterns and population structure of the sea urchin Paracentrotus lividus (Echinodermata: Echinoidea), in the coastal fishery of western Sardinia: a geostatistical analysis. Sci. Mar. 76, 733–740. doi: 10.3989/scimar.03602.26B
Atema, J. (2012). “Chemical Ecology,” in Aquatic Systems, eds C. Bronmark and L. A. Hansson. New York: Oxford University Press, 1–18.
AviLine (2005). Available online at: http://biolpc22.york.ac.uk/drosophila/ovary/ (accessed Sep 05, 2005)
Bargmann, C. I. (2006). Comparative chemosensation from receptors to ecology. Nature 444, 295–301. doi: 10.1038/nature05402
Boada, J., Arthur, R., Alonso, D., Pages, J. F., Pessarrodona, A., Oliva, S., et al. (2017). Immanent conditions determine imminent collapses: nutrient regimes define the resilience of macroalgal communities. Proc. R. Soc. B 284:20162814. doi: 10.1098/rspb.2016.2814
Borroni, P. F., and Atema, J. (1988). Adaptation in chemoreceptor cells. I. Self adapting backgrounds determine threshold and cause parallel shift of response function. J. Comp. Physiol. A 164, 67–74. doi: 10.1007/BF00612719
Burke, R. D., Angerer, L. M., Elphick, M., Humphrey, R., Yaguchi, G. W., Liang, S., et al. (2006). A genomic view of the sea urchin nervous system. Dev. Biol. 300, 434–460. doi: 10.1016/j.ydbio.2006.08.007
Campbell, A. C., Coppard, S., D’Abreo, C., and Tudor-Thomas, R. (2001). Escape and aggregation responses of three echinoderms to conspecific stimuli. Biol. Bull. 201, 175–185. doi: 10.2307/1543332
Carboni, S., Kelly, M. S., Hughes, A. D., Vignier, J., Atack, T., and Migaud, H. (2014). Evaluation of flow through culture technique for commercial production of sea urchin (Paracentrotus lividus) larvae. Aquac.Res. 45, 768–772. doi: 10.1111/are.12019
Casal-Porras, I., Jimenez-Ramos, R., Zubia, E., and Brun, F. G. (2021). Importance of the chemical defenses and sugars in the feeding preference of Paracentrotus lividus over two sympatric template seagrass species. Estuar. Coast. Shelf. Sci. 259:107466. doi: 10.1016/j.ecss.2021.107466
Castilla-Gavilán, M., Buzin, F., Cognie, B., Dumay, J., Turpin, V., and Decottignies, P. (2018). Optimising microalgae diets in the sea urchin Paracentrotus lividus larviculture to promote aquaculture diversification. Aquaculture 490, 251–259. doi: 10.1016/j.aquaculture.2018.02.003
Ceccherelli, G., Pais, A., Pinna, S., Sechi, N., and Chessa, L. A. (2011). Human impact on Paracentrotus lividus: the result of harvest restrictions and accessibility of locations. Mar. Biol. 158, 845–852. doi: 10.1007/s00227-010-1611-5
Cirino, P., Ciaravolo, M., Paglialonga, A., and Toscano, A. (2017). Long-term maintenance of the sea urchin Paracentrotus lividus in culture. Aquac. Rep. 7, 27–33. doi: 10.1016/j.aqrep.2017.04.003
Cyrus, M. D., Bolton, J. J., Scholtz, R., and Macey, B. M. (2015). The advantages of Ulva (Chlorophyta) as an additive in sea urchin formulated feeds: effects on palatability, consumption and digestibility. Aquac. Nutr. 21, 578–591. doi: 10.1111/anu.12182
Fairbairn, D. (1958). Trehalose and glucose in helminths and other invertebrates. Can. J. Zool. 36, 787–795. doi: 10.1139/z58-065
FAO. (2020). FAO yearbook of fishery and aquaculture statistics capture production 2018. Rome: FAO Food and Agriculture Organization of the United Nations.
Galasso, C., Orefice, I., Toscano, A., Vega Fernandez, T., Musco, L., Brunet, C., et al. (2018). Food modulation controls astaxanthin accumulation in eggs of the sea urchin Arbacia lixula. Mar. Drugs 16:186. doi: 10.3390/md16060186
Giakoumi, S., Cebrian, E., Kokkoris, G. D., Ballesteros, E., and Sala, E. (2012). Relationships between fish, sea urchins and macroalgae: The structure of shallow rocky sublittoral communities in the Cyclades, Eastern Mediterranean. Estuar. Coast. Shelf. Sci. 109, 1–10. doi: 10.1016/j.ecss.2011.06.004
Gianguzza, P., Agnetta, D., Bonaviri, C., Di Trapani, F., Visconti, G., Gianguzza, F., et al. (2011). The rise of thermophilic sea urchins and the expansion of barren grounds in the Mediterranean Sea. Chem. Ecol. 27, 129–134. doi: 10.1080/02757540.2010.547484
Giglioli, A. A., Addis, P., Pasquini, V., Secci, M., and Hannon, C. (2021). First assessment of restocking efficacy of the depleted sea urchin Paracentrotus lividus populations in two contrasted sites. Aquac. Res. 52, 2896–2900. doi: 10.1111/are.15098
Guidetti, P., and Dulcić, J. (2007). Relationships among predatory fish, sea urchins and barrens in Mediterranean rocky reefs across a latitudinal gradient. Mar. Environ. Res. 63, 168–184. doi: 10.1016/j.marenvres.2006.08.002
Gutiérrez-Salmeán, G., Fabila-Castillo, L., and Chamorro-Cevallos, G. (2015). Nutritional and toxicological aspects of Spirulina (Arthrospira). Nutr. Hosp. 32, 34–40. doi: 10.3305/nh.2015.32.1.9001
Hannon, C., Officer, R. A., and Chamberlain, J. (2017). Evaluation of the efficacy of algal-conditioned substrates for inducing settlement of Paracentrotus lividus larvae. Aquac. Res. 48, 1968–1973. doi: 10.1111/are.12959
Kamio, M., and Derby, C. D. (2017). Finding food: how marine invertebrates use chemical cues to track and select food. Nat. Prod. Rep. 34, 514–528. doi: 10.1039/c6np00121a
Lawrence, J. M. (2013). Sea urchins: Biology and ecology. Developments in aquaculture and fisheries science. Amsterdam: Elsevier.
Mercier, A., and Hamel, J.-F. (2009). Endogenous and exogenous control of gametogenesis and spawning in echinoderms. Adv. Mar. Biol. 55, 1–302. doi: 10.1016/S0065-2881(09)55001-8
Micheli, F., Benedetti-Cecchi, L., Gambaccini, S., Bertocci, I., Borsini, C., Osio, G. C., et al. (2005). Cascading human impacts, marine protected areas, and the structure of Mediterranean reef assemblages. Ecol. Monogr. 75, 81–102. doi: 10.1890/03-4058
Middleton, C. A., Nongthomba, U., Parry, K., Sweeney, S. T., Sparrow, J. C., and Elliott, C. J. H. (2006). Neuromuscular organization and aminergic modulation of contractions in the Drosophila ovary. BMC Biol. 4:17. doi: 10.1186/1741-7007-4-17
Mollo, E., Garson, M. J., Polese, G., Amodeo, P., and Ghiselin, M. T. (2017). Taste and smell in aquatic and terrestrial environments. Nat. Prod. Rep. 34, 496–513. doi: 10.1039/c7np00008a
Pais, A., Chessa, L. A., Serra, S., Ruiu, A., Meloni, G., and Donno, Y. (2007). The impact of commercial and recreational harvesting for Paracentrotus lividus on shallow rocky reef sea urchin communities in North-western Sardinia. Italy. Estuar. Coast. Shelf. Sci. 73, 589–597. doi: 10.1016/j.ecss.2007.02.011
Pisut, D. P. (2004). The distance chemosensory behavior of the sea urchin Lytechinus variegatus. Georgia: Georgia Institute of Technology.
Prato, E., Fanelli, G., Angioni, A., Biandolino, F., Parlapiano, I., Papa, L., et al. (2018). Influence of a prepared diet and a macroalga (Ulva sp.) on the growth, nutritional and sensory qualities of gonads of the sea urchin Paracentrotus lividus. Aquaculture 493, 240–250. doi: 10.1016/j.aquaculture.2018.05.010
Pusceddu, A., Mikhno, M., Giglioli, A., Secci, M., Pasquini, V., Moccia, D., et al. (2021). Foraging of the sea urchin Paracentrotus lividus (Lamarck, 1816) on invasive allochthonous and autochthonous algae. Mar. Environ. Res. 170:105428. doi: 10.1016/j.marenvres.2021.105428
Raible, F., Tessmar-Raible, K., Arboleda, E., Kaller, T., Bork, P., Arendt, D., et al. (2006). Opsins and clusters of sensory G-protein-coupled receptors in the sea urchin genome. Dev. Biol. 300, 461–475. doi: 10.1016/j.ydbio.2006.08.070
Reuter, K. E., and Levitan, D. R. (2010). Influence of sperm and phytoplankton on spawning in the echinoid Lytechinus variegatus. Biol. Bull. 219, 198–206. doi: 10.1086/BBLv219n3p198
Secci, M., Ferranti, M. P., Giglioli, A., Pasquini, V., Sicurelli, D., Fanelli, G., et al. (2020). Effect of temperature and duration of immersion on the stability of prepared feeds in echinoculture. J. Appl. Aquac. 33, 150–164. doi: 10.1080/10454438.2020.1724845
Shay, E. G. (1993). Diesel fuel from vegetable oils: status and opportunities. Biomass Bioener. 4, 227–242. doi: 10.1016/0961-9534(93)90080-N
Sloan, N. A., and Campbell, A. C. (1982). “Perception of food,” in Echinoderm Nutrition, eds M. Jangoux and J. N. Lawrence. Rotterdam: A. A. Balkema.
Snyder, N., and Snyder, H. (1970). Alarm response of Diadema antillarum. Science 168, 276–278. doi: 10.1126/science.168.3928.276
Solari, P., Melis, M., Sollai, G., Masala, C., Palmas, F., Sabatini, A., et al. (2015). Sensing with the legs: contribution of pereiopods in the detection of food-related compounds in the red swamp crayfish Procambarus clarkii. J. Crustac. Biol. 35, 81–87. doi: 10.1163/1937240X-00002291
Solari, P., Sollai, G., Masala, C., Loy, F., Palmas, F., Sabatini, A., et al. (2017a). Antennular morphology and contribution of aesthetascs in the detection of food-related compounds in the shrimp Palaemon adspersus Rathke, 1837 (Decapoda: Palaemonidae). Biol. Bull. 232, 110–122. doi: 10.1086/692696
Solari, P., Rivelli, N., De Rose, F., Picciau, L., Murru, L., Stoffolano, J. G., et al. (2017b). Opposite effects of 5-HT/AKH and octopamine on the crop contractions in adult Drosophila melanogaster: Evidence of a double brain-gut serotonergic circuitry. PLoS One 12:e0174172. doi: 10.1371/journal.pone.0174172
Stefánsson, G., Kristinsson, H., Ziemer, N., Hannon, C., and James, P. (2017). Markets for sea urchins: A review of global supply and markets, Technical Report 10-17. Reykjavtßk: Matís ohf/Matis - Food Research, Innovation & Safety.
Vadas, R. L. (1977). Preferential feeding: an optimization strategy in sea urchins. Ecol. Monogr. 47, 337–371.
Velasquez, S. F., Chan, M. A., Abisado, R. G., Traifalgar, R. F. M., Tayamen, M. M., Maliwat, G. C. F., et al. (2016). Dietary Spirulina (Arthrospira platensis) replacement enhances performance of juvenile Nile tilapia (Oreochromis niloticus). J. Appl. Phycol. 28, 1023–1030. doi: 10.1007/s10811-015-0661-y
Vonshak, A. (1997). Spirulina Platensis (Arthrospira): Physiology, Cell-biology, and Biotechnology. London: Taylor & Francis.
Weissburg, M. J. (2000). The fluid dynamical context of chemosensory behavior. Biol. Bull. 198, 188–202. doi: 10.2307/1542523
Yeruham, E., Abelson, A., Rilov, G., Ben Ezra, D., and Shpigel, M. (2019). Energy budget of cultured Paracentrotus lividus under different temperatures. Aquaculture 501, 7–13. doi: 10.1016/j.aquaculture.2018.11.006
Yeruham, E., Rilov, G., Shpigel, M., and Abelson, A. (2015). Collapse of the echinoid Paracentrotus lividus populations in the Eastern Mediterranean - Results of climate change? Sci. Rep. 5:13479. doi: 10.1038/srep13479
Zhang, F., Bon Man, Y., Mo, W. Y., and Wong, M. H. (2020). Application of Spirulina in aquaculture: a review on wastewater treatment and fish growth. Rev. Aquac. 12, 582–599. doi: 10.1111/raq.12341
Zupo, V. (1993). The use of feeding indices for the study of food webs: An application to a Posidonia oceanica ecosystem. Coenoses 8, 85–95.
Keywords: aquatic chemoreception, Spirulina, sugars, spines, tube feet, aquaculture, behavior
Citation: Solari P, Pasquini V, Secci M, Giglioli A, Crnjar R and Addis P (2021) Chemosensitivity in the Sea Urchin Paracentrotus lividus (Echinodermata: Echinoidea) to Food-Related Compounds: An Innovative Behavioral Bioassay. Front. Ecol. Evol. 9:749493. doi: 10.3389/fevo.2021.749493
Received: 29 July 2021; Accepted: 27 September 2021;
Published: 12 October 2021.
Edited by:
Sara Invitto, University of Salento, ItalyReviewed by:
Muki Spiegel, University of Haifa, IsraelMariachiara Chiantore, University of Genoa, Italy
Copyright © 2021 Solari, Pasquini, Secci, Giglioli, Crnjar and Addis. This is an open-access article distributed under the terms of the Creative Commons Attribution License (CC BY). The use, distribution or reproduction in other forums is permitted, provided the original author(s) and the copyright owner(s) are credited and that the original publication in this journal is cited, in accordance with accepted academic practice. No use, distribution or reproduction is permitted which does not comply with these terms.
*Correspondence: Paolo Solari, c29sYXJpQHVuaWNhLml0