- 1Laboratory of Zoology, Research Institute for Biosciences, University of Mons, Mons, Belgium
- 2Quality and Authentication of Agricultural Products Unit, Knowledge and Valorization of Agricultural Products Department, Walloon Agricultural Research Centre, Gembloux, Belgium
- 3Laboratory of Cell Biology, Research Institute for Biosciences, University of Mons, Mons, Belgium
- 4Evolutionary Biology and Ecology, Université Libre de Bruxelles, Brussels, Belgium
Climate change is related to an increase in the frequency and intensity of extreme events such as heatwaves. In insect pollinators, heat exposure is associated with direct physiological perturbations, and in several species, could lead to a decrease of fitness related to a decrease in fertility. Here we developed a new experimental protocol in controlled conditions to assess if the exposure to high temperatures could modify the attractiveness and fertility of Bombus terrestris males. Our results show that virgin queens of B. terrestris do not have preferences between the pheromonal secretions of heat-exposed and control males. Moreover, mating with a heat-exposed male has no impact on the copulation behavior and the development of the nest (brood composition). We advise to extend trials to cover a range of wild and heat-sensitive species on multiple generations to better understand the impact of heat waves on the bumblebee communities.
Introduction
Climate change is threatening worldwide biodiversity for many years in various direct (e.g., heat stress, drought) and indirect (e.g., habitat modification) ways (Bálint et al., 2011; Bellard et al., 2012; Dirzo et al., 2014). One of the consequences of exposure to heat stress is a loss of fertility (Walsh et al., 2019). In insects, heat exposure can impact the viability, motility, competitiveness, and the DNA integrity of spermatozoa (Sales et al., 2018; McAfee et al., 2020; Martinet et al., 2021b). With the increase in frequency and severity of heat waves (Meehl and Tebaldi, 2004; Hallmann et al., 2017) and hence the heat stress experienced by insects, this impact will worsen in the future and may cause negative population trends (Sales et al., 2018, 2021). We need to develop experimental protocols to assess and understand direct impact of heat stress on insect model species.
With an annual economic value of 153 billion dollars (USD) for crop pollination (Potts et al., 2016), insect pollinators, wild and domesticated, are a key functional group to conserve (Williams, 1994). Recently, some species have been studied to assess the impact of environmental stresses (e.g., extreme temperatures, pesticides) on individual survival and fertility (McAfee et al., 2020; Minnameyer et al., 2021; Strobl et al., 2021), but the potential consequences of sublethal effects on resulting offspring have been poorly studied (Sales et al., 2018).
Here, we explore this question using bumblebees as a model species for eusocial pollinators. Bumblebees are particularly sensitive to environmental stressors, especially to extreme high temperatures as they are originally adapted to cold climate (Heinrich, 2005). Unlike most insects, bumblebees can individually regulate their body temperature to a certain species-specific point (CTmin, CTmax) regardless of the air temperature (Heinrich and Heinrich, 1983; Oyen et al., 2016). Moreover, temperature can also be regulated by workers at the colony level, around an optimal temperature of 30°C (Heinrich and Heinrich, 1983; Livesey et al., 2019). However, as emerged males live outside the nest, they cannot benefit from social thermoregulation. This exposure can lead to several physiological perturbations like a decrease in sperm viability and an increase in chromatin degradation (Martinet et al., 2021b). Degradation of sperm quality in bumblebee males can potentially be harmful because most studied bumblebee queens are monandrous (Baer, 2003). After storing sperm in their spermatheca for several months during the hibernation, queens use it to fertilize their eggs and produce diploid workers while unfertilized eggs produce males (Lecocq et al., 2017). If a queen mates with a heat-exposed male, it may receive a low-quality stock of spermatozoa. This could lead to a bias in favor of males in the sex ratio in the resulting colony and thus impact the reproductive efficiency of the colony (Beekman and van Stratum, 1998; also in solitary bees, Zaragoza-Trello et al., 2021).
To avoid mating with males with low fertility, females of bumblebees could be able to detect this change in fertility by an equally changed pheromone signal following heat stress. In bumblebees, the courtship behavior (e.g., patrolling) involves a release of pheromones known as Cephalic Labial Gland Secretions (CLGS; Calam, 1969; Ayasse and Jarau, 2014; Valterová et al., 2019). These species-specific secretions are intended to attract females before mating (Bergström et al., 1981). It has previously been shown that extreme heat temperatures could impact those secretions in heat sensitive bumblebee species (i.e., Martinet et al., 2021b).
In the present study, we present a new experimental approach to assess the impact of heat stress on the attractiveness, the mating behavior and the offspring of males of Bombus terrestris (Figure 1). As a model species, we used Bombus terrestris, a Euro-Mediterranean species domesticated for crop pollination (Rasmont et al., 2008). This species is highly tolerant to environmental changes and is expanding globally (Inari et al., 2005; Torretta et al., 2006; Rasmont et al., 2015; Martinet et al., 2021a). It has previously been shown that for this heat-tolerant species, no effect of heat exposure was observed on sperm viability, sperm DNA integrity and composition of the attractive secretions of males (Martinet et al., 2021b). However, other non-assessed parameters related to bumblebee reproduction (e.g., sperm mobility, attractiveness of pheromonal mixture) could have a key role in the fertility of males. These factors are thus considered here.
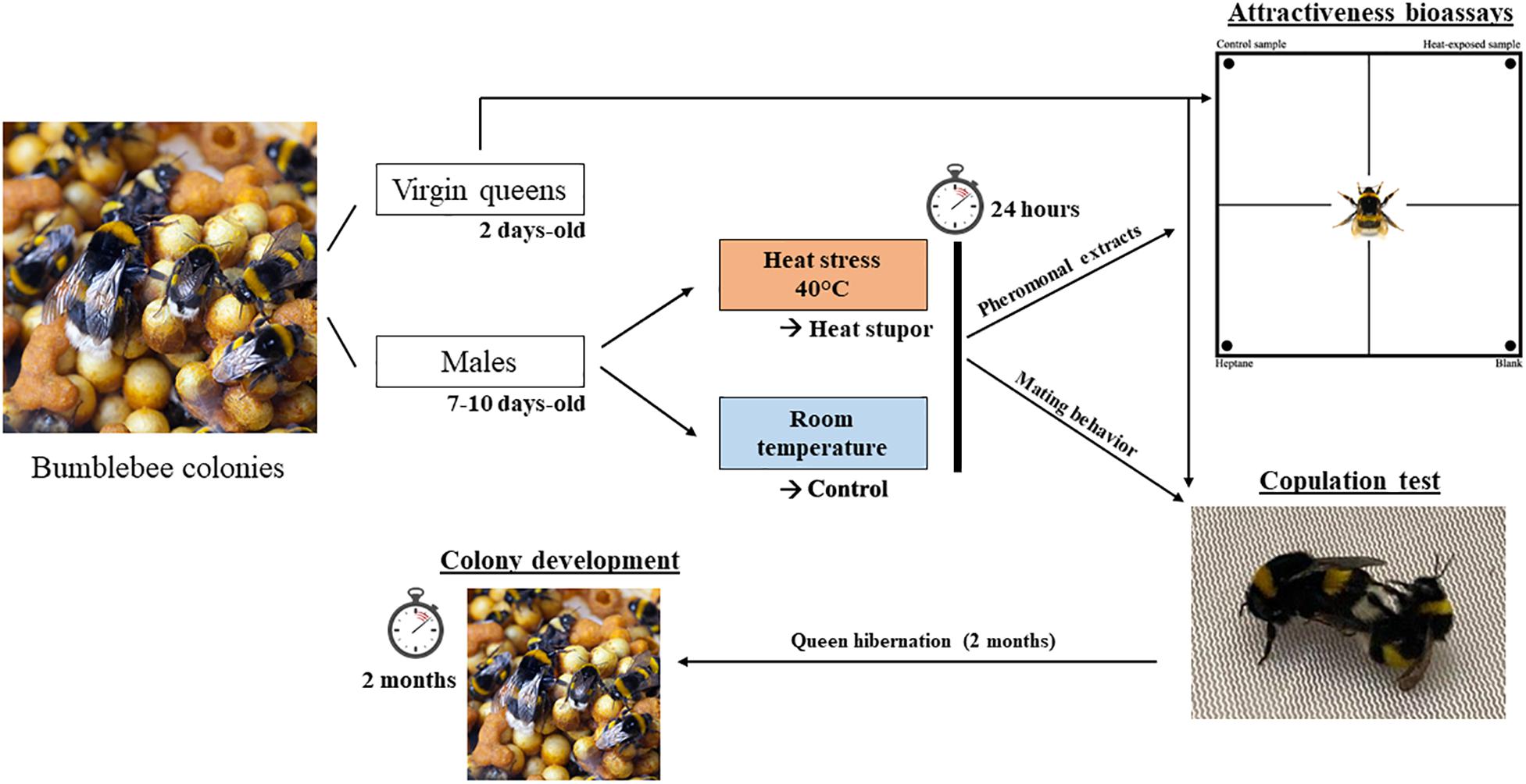
Figure 1. Experimental design. Virgin queens and males were produced from queen-right colonies. Males aged of 7–10 days were split in two condition groups: heat-stressed group (40°C) and control group (25°C). Each group were allowed to rest 24 h before the experiment for standardization. For each condition we used half of the group for the attractiveness bioassays (pheromonal extraction) and the other half for the copulation test. Mated queens were put in hibernation for 2 months before nesting. To assess developmental parameters (brood stages), resulting colonies were dissected 2 months after first eggs laid.
Materials and Methods
Model Species and Breeding Parameters
We used the buff-tailed bumblebee (B. terrestris) as a model species even though it is considered a resistant species (Martinet et al., 2021a) because it is easy to manage and available all year. Colonies were bought at Biobest NV (Westerlo, Belgium). We reared the different colonies in plastic boxes (8 cm × 16 cm × 16 cm) provided by Biobest NV (Westerlo, Belgium) at their optimal temperature of 26°C with 50–60% of relative humidity (e.g., Vanderplanck et al., 2019). Colonies were maintained in constant darkness and were fed with BIOGLUC (Biobest NV, Westerlo, Belgium) as sugar resources and pollen of Salix sp. as lipid/protein resources (Vanderplanck et al., 2019). Pollen loads were purchased from the “Ruchers de Lorraine” company. New pollen candy (pollen mixed with BIOGLUC) was provided every 2 days, with previous candies removed to prevent decay. Males were produced from queen-right colonies (colonies developing normally with a queen) and were isolated in plastic boxes without workers. To ensure that all males were exposed to the exact same conditions before our experiments, we maintained all males at 26°C and 50–60% relative humidity directly after emergence until their maturity. All males were 7–10 days old at the time of the experiment. This period corresponds to the peak of pheromones production of mature males (Baer, 2003; Coppée et al., 2011; Ayasse and Jarau, 2014).
All queens and males were conditioned in standard plastic box (16 cm × 11 cm× 9 cm) with ad libitum pollen candies and sugar under controlled lab conditions (26°C and 50–60% of humidity). Queens were isolated in a standard plastic box (16 cm × 11 cm × 9 cm) with ad libitum pollen candies and sugar solution (Biogluc, Biobest, Waterloo, Belgium) 1 day before the mating experiment to improve the mating success (Röseler, 1985).
Exposure of Males to Extreme Temperatures
Bumblebee males (n = 75) were thermally exposed following the protocol of Martinet et al. (2015). Briefly, males were placed in an incubator at 40°C (typical heatwave temperature) until the “Heat Stupor” state. This state is recognizable by some behaviors: (i) the bumblebee falls upside down and is unable to turn back on its legs, (ii) it loses all its reflexes, and (iii) it has muscular spams in its legs. After heat stress, survival males recovered 1 day in plastic boxes at 26°C with ad libitum access to nutritive resources (i.e., sugar) before being presented to virgin queen for the mating assays.
Quality Check of Cephalic Labial Gland Secretions
A subset of the control and heat stressed males (n = 15 and n = 15, respectively), were killed for pheromones quality check. Cephalic labial gland secretions (CLGS) were extracted in 400 mL of heptane following a protocol adapted from De Meulemeester et al. (2011). We used gas chromatography-mass spectrometry using a Finnigan GCQ quadrupole system (GC/MS) with a non-polar DB-5 ms capillary column [5% phenyl (methyl) polysiloxane stationary phase; column length 30 m; inner diameter 0.25 mm; film thickness 0.25 μm] to determine the qualitative composition of CLGS. The identification was confirmed with analytical standards. We performed a relative quantification for CLGS samples with a gas chromatograph Shimadzu GC-2010 system (GC-FID) equipped with a non-polar SLB-5 ms capillary column [5% phenyl (methyl) polysiloxane stationary phase; column length 30 m; inner diameter 0.25 mm; film thickness 0.25 μm] and a flame ionization detector.
Attraction of Virgin Females to Male Secretion
Virgin queens of B. terrestris are known to be very reactive to male pheromonal secretions in solution (Lecocq et al., 2015). We performed ethological tests based on the protocol established by Terzo and Sinkevich (2005) and updated by Lecocq et al. (2015). Virgin queens were stored individually in breeding conditions (i.e., 26°C, 50–60% of relative humidity and food resources ad libitum). To minimize potential external disturbances, ethological tests were carried out in the breeding room. All ethological tests were filmed using a camera (HC-100, Panasonic, Osaka, Japan) positioned at 81 cm above the experimental device. Individuals were introduced one by one into the apparatus (70 cm × 70 cm × 8 cm). The device was divided into four square areas (35 cm × 35 cm), henceforth referred to as “quarters.” A plastic basket (85 mm × 85 mm × 24 mm, VWR International, Fontenay-sous-Bois, France) was placed in the corner of each quarter of the apparatus containing a piece of filter paper inside (1.5 cm × 1.5 cm) soaked with a 2 μl sample of pheromonal secretions extract from control or heat-exposed males previously used for the CLGS quality check. Filter papers soaked in heptane (the solvent used for CLGS extraction) or not soaked were used as controls to test the attractiveness of the heptane itself. Therefore, the virgin queens were able to choose between four quarters during a same experiment: two quarters with either heptane or an unsoaked paper and the two other quarters with pheromonal secretions from either a control or heat-exposed male (Figure 1). Each young virgin queen was tested with a different sample from control or heat-exposed males randomly selected among the extracted pheromones of the 30 males. After each test, baskets and filter papers were removed, the apparatus was cleaned with ethanol and then ventilated for 5 min to ensure complete ethanol evaporation. Filter papers were placed randomly in the quarters to avoid a potential quarter preference factor. Each test was performed for a 5 min period under red-light conditions. The first 2 min correspond to the time required for virgin queens to be receptive to the new environment and were therefore removed from the analysis (Lecocq et al., 2015). During the last 3 min, we recorded the time spent by the queens in each quarter of the apparatus (we considered that the queen was in a quarter when more than half of its body was in this quarter). Before experiment, neutrality tests (n = 30) were carried out to check that no quarter was more attractive than others. Ethological bioassays were carried based on the CLGS of 30 males of B. terrestris (heat-exposed group n = 15; control group n = 15) and with 30 virgin queens, both from different colonies to avoid brother-sister mating.
Copulation
Reproduction and hibernation of bumblebee queens was performed following Lhomme et al. (2013). For the reproduction experiment, we used 50 virgin queens (2-day old) provided by Biobest (Westerlo, Belgium). Each queen was placed in a flight cage (35 cm × 35 cm × 60 cm) exposed to natural light at room temperature with three different males (7–10 days old) of the same condition (control or heat-stressed) to stimulate the mating. To avoid genetic and behavioral bias, we used non-related males to virgin queens from different standardized colonies provided by Biobest (Westerlo, Belgium). Once a queen was mated with a male, the other males were removed from the device to avoid any disruption during the mating. After a successful mating (±30 min), the queen was placed in a plastic box (16 cm × 11 cm × 9 cm) during 1 week with ad libitum pollen and sugar before hibernation.
For the hibernation, bumblebee queens (n = 50) were placed individually in a match box with a moist cotton to maintain humidity. Those boxes were maintained at 5°C for a period of 2 months (Beekman et al., 1998). After the hibernation, the queens were placed in a fly cage (Yoon et al., 2010) (30 cm × 30 cm × 30 cm) for 1 week at room temperature with ad libitum pollen and sugar. They were subjected to a photoperiod of 8 h of light and 16 h of dark for 3 days and then a phase of 24 h of full light to exit hibernation (Yoon et al., 2010). Finally, all the queens were placed individually in a rearing box (16 cm × 11 cm × 9 cm, Biobest NV, Westerlo, Belgium) to initiate their colony.
Colony Development
Queens were reared with optimum temperature conditions (26°C) and constant humidity (50–60%) in rearing plastic boxes (16 cm × 11 cm × 9 cm) in complete darkness. They were fed ad libitum with Salix sp. pollen (Ruchers de Lorraine), a diet of optimal quality (Vanderplanck et al., 2019) with sugar syrup (Biogluc, Biobest, Westerlo, Belgium). Prior to bumblebee feeding, pollen loads were crushed and mixed with sugar syrup (Biogluc, Biobest). Each pollen candy was weighed and replaced every 2 days and the removed candies were weighed to assess pollen consumption. During all the experimentations, syrup consumption was measured for each colony.
Colonies were dissected after 2 months of development (i.e., 2 months after the first eggs were laid). We selected this experiment time because it corresponds to a classic lifespan of commercial bumblebee colony and this time allows us to have a sufficiently grown colony to assess development parameters (Cnaani et al., 2002). We dissected the colonies using the following parameters defined by Taseï and Aupinel (2008): (a) total pollen and syrup consumption which gives a proxy on the larval development; (b) total mass of the brood; (c) number of new emergences (workers and possible reproductives) and (d) mortality (i.e., number of dead individuals divided by total individuals including initial ones and new-emerged ones) which gives indication on the workforce, brood care behavior and resilience (i.e., ability to respond to environmental stresses). We determined mass and number of each larval stages and emerged individuals (i.e., brood composition) considering separately the different brood stages, namely eggs, non-isolated larvae, isolated and pre-defecating larvae, isolated and post-defecating larvae, pupae, non-emerged and emerged males.
Statistical Analyses
For ethological data, all the videos were analyzed by using the BORIS v.7.4.7 software (Friard and Gamba, 2016). The time spent in each quarter of the apparatus was analyzed by using a non-parametric Chi2 as the normality and homoscedasticity were not respected. A Kruskal–Wallis test was performed to test the difference between the different quarters followed by a post hoc Kruskal–Wallis multiple comparison test to identify which quarters are different from the others.
We performed statistical comparative analyses of the colony performances using R 3.5.1 environment (R Core Team, 2018). Significant tests (Wilcoxon Mann–Whitney) were run to assess differences between groups for each parameter. For the analysis of brood composition (relative mass of the different larval stages), we performed a permutational multivariate analysis of variance (perMANOVA) on arcsine-transformed data using the Bray-Curtis dissimilarity matrix and 1000 permutations (“adonis” command, R-package vegan, Oksanen et al., 2018). Prior to these tests, the multivariate homogeneity of within-group covariance matrices was verified using the “betadisper” function. When a significant difference was detected, we performed multiple pairwise comparisons with an adjustment of p-values (Bonferroni correction). Finally, separate t-test and Tukey post hoc tests were conducted to assess the effect of heat exposure on each developmental stage.
Results
Attraction of Virgin Females to Male Secretion
Quality check of CLGS revealed no difference among males and a sufficient load of pheromonal compounds including the main compound 2,3-dihydrofarnesol. Gas chromatography analyses revealed a typical chemical fingerprint of B. terrestris. Our results confirm that the 7–10 days-old males can be used to assess the attractiveness of their sexual attractive mixture.
Neutrality tests performed before the experiments showed no significant differences between quarters of the apparatus (Kruskal–Wallis multiple comparison test, p = 0.31), meaning that, without CLGS extract, young virgin queens have no preference for a specific quarter. During attractiveness tests, the time spent by the young virgin queens was not significantly different between the two control tests (i.e., performed with filter paper soaked in heptane or with unsoaked filter paper) (Kruskal–Wallis multiple comparison test, p = 0.19, Figure 2). However, a significant difference was detected between male secretions (from both control and heat-stressed groups) and heptane (Kruskal–Wallis multiple comparison test, p < 0.01) and between male secretions and non-impregnated filter paper (p < 0.01). Finally, no difference was detected between CLGS extracts from control and heat-exposed groups (p = 0.16).
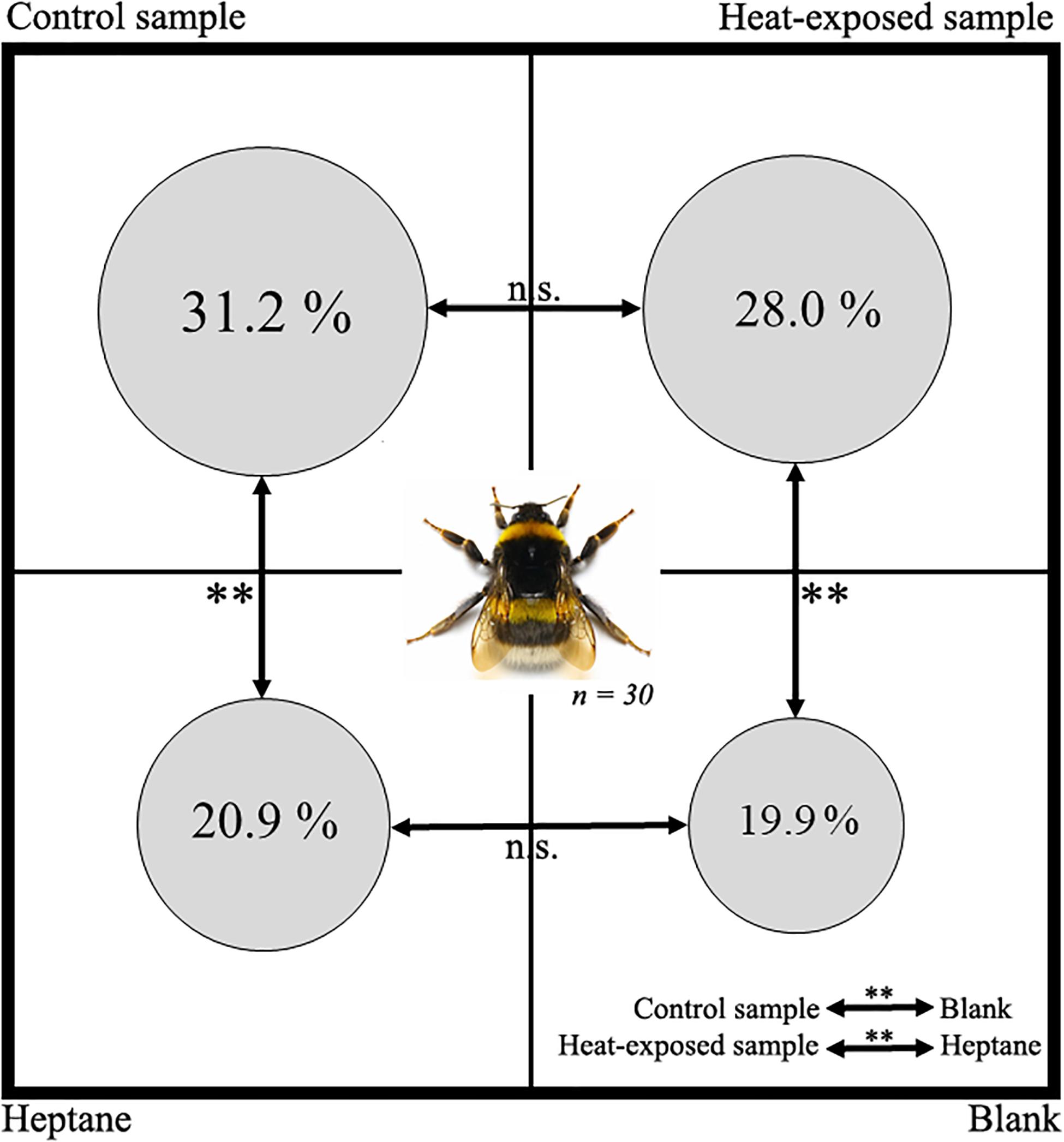
Figure 2. Ethological bioassays. Diagram showing the percentage of time spent by the virgin bumblebees queen (B. terrestris) in each quarter of the apparatus during ethological bioassays according to the solution present on the filter paper. Control sample, filter paper with pheromones from non-stressed males; Heptane, filter paper with heptane; Heat-exposed sample, filter paper with pheromones from heat-exposed males; Blank, unsoaked filter paper; bi-directional arrows represent statistical comparison between groups; n.s., non-significative difference; ∗∗, significative difference p < 0.01, Kruskal–Wallis multiple comparison test.
Copulation and Colony Development
Overall, our results show that heat exposure on males did not have an impact on mating time or copulation behavior. During nest development and colony growth, behavioral differences between both conditions (i.e., heat-exposed and control groups) as well as in colony dynamics and brood development were not observed. We evaluated the performance of bumblebee colonies based on colony growth, composition of brood, mortality, total pollen, and syrup collection. For both conditions (i.e., colonies from a heat-exposed male and colonies from a control male), all these parameters were not significantly affected by heat stress applied beforehand to the males (Kruskal–Wallis multiple comparison test, respectively, p = 0.67; p = 0.89; p = 0.73 and p = 0.37; Figures 3A,B). Finally, the dissection of the colony showed no significative difference in brood composition for all assessed developmental stages (Figure 3C, perMANOVA, p = 0.29).
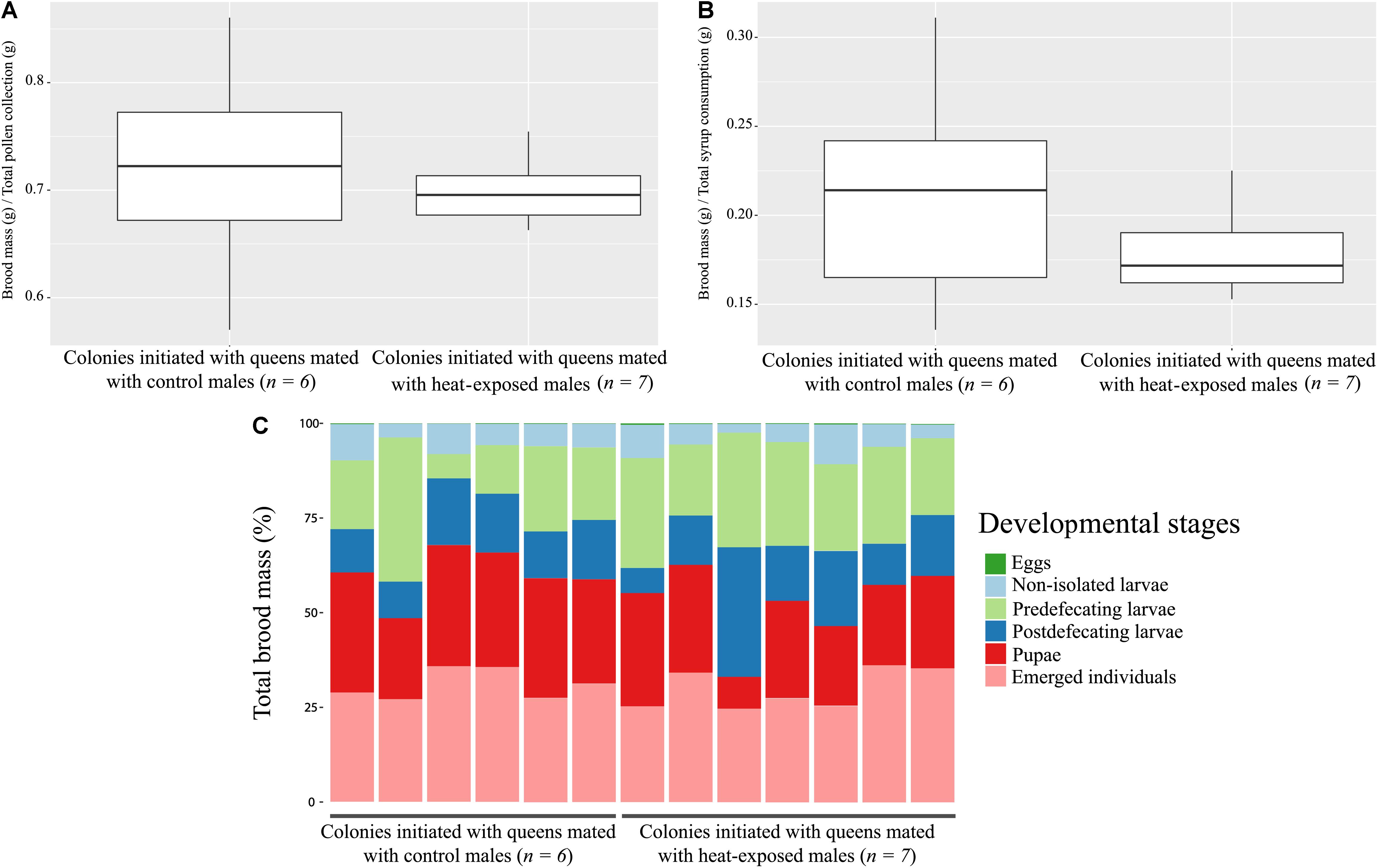
Figure 3. (A,B) Resource collection. Brood mass/pollen collection (A), brood mass/syrup collection (B) for colonies initiated with queens mated with heat-exposed males (right) and control males (left). No significative difference has been detected between the two groups [Wilcoxon Mann–Whitney, respectively, (A) p = 0.73 and (B) p = 0.37]. (C) Colony dynamics. Brood composition at different developmental stages expressed as percentage of total brood mass for colonies initiated with queens mated with control or heat-exposed males. No significant difference has been detected in brood composition between the two groups (perMANOVA, p = 0.29).
Discussion
In insects, heat stupor is associated to neuro-muscular potential, water balance, or physiological disruptions (Kingsolver et al., 2013). We performed multiple bioassays to investigate if heat-exposed males (i.e., in heat stupor with) are able to attract and mate with virgin queens and produce viable offspring. Our results demonstrate that virgin queens of B. terrestris are unable to distinguish secretions of heat-exposed males from secretions of non-exposed individuals. A previous study in another hymenopteran (i.e., Nasonia vitripennis, a parasitic wasp) also showed that females are not able to distinguish a heat-exposed male from a non-exposed male (Chirault et al., 2015). As previously demonstrated (Martinet et al., 2021b) it has been shown that there is no significant impact of heat shock on the histology of cephalic labial glands and the composition of the produced pheromones in males of B. terrestris (i.e., a warm-adapted species). Our bioassays are consistent with these results.
Regarding the experiment of copulation and colony development, our interpretations are limited to B. terrestris, a species known for its heat resistant capabilities. Additional experiments on more sensitive species still must be performed to test if virgin females are able to avoid copulation with heat-exposed males presenting potential physiological damages (Martinet et al., 2021a,b). Indeed, it has been emphasized that bumblebee species, like the declining Bombus jonellus, are more substantially impacted by heat exposure particularly in CLGS composition, sperm viability and sperm DNA integrity (Martinet et al., 2021b). In the present study, we investigated the effect of heat stress on the first generation only, although it is known that extreme high temperatures could cause a decrease in sperm viability and thereby transgenerational damages on different life stages in ectotherm insects, as it is the case for the red flour beetle Tribolium castaneum (Sales et al., 2018, 2021). However, while investigating transgenerational damages could be a key point to understand global pollinator decline, we did not detect any significant impact in B. terrestris while in the case of Tribolium castaneum, damages from thermal stress is observable in the first generation (Sales et al., 2018, 2021).
Different threats have been identified to explain the global decline of bumblebees. For several years, many studies have explored effects of microbiota, diet, temperature, and pesticide exposure on bumblebee’s health in lab conditions (Gill and Raine, 2014; Vanderplanck et al., 2019; Barraud et al., 2020; Rothman et al., 2020; Maebe et al., 2021a; Oyen et al., 2021). However, most of these studies used commercially available bumblebee species (i.e., Bombus impatiens in North America and B. terrestris in Europe, and Bombus hypocrita in Asia) which are known to be tolerant and resistant to environmental changes (Oyen and Dillon, 2018; Maebe et al., 2021b; Martinet et al., 2021a). Limiting studies to reared species may bias our overall view of the adaptive response of bumblebees to decline factors as these species are selected throughout this rearing process. We should extend further studies to more wild and sensitive species to better understand this impact on the global bumblebee’s biodiversity.
Data Availability Statement
The original contributions presented in the study are included in the article/supplementary material, further inquiries can be directed to the corresponding author.
Author Contributions
KP, EZ, AA, and BM conceived and designed the experiments, and performed the experiments. KP and BM analyzed the data. All authors wrote the manuscript.
Funding
KP is Ph.D. student “Aspirant” of F.R.S.-FNRS and BM is F.R.S.-FNRS grant fellowship “Chargé de Recherches” (Fonds de la Recherche Scientifique). DM contributed as researcher for the “Fonds de la Recherche Scientifique – FNRS,” the Research Foundation – Flanders (FWO) under EOS Project named CLIPS (n°3094785). The research was partly funded by the Institute of Biosciences (UMons) under “Bombstress project”.
Conflict of Interest
The authors declare that the research was conducted in the absence of any commercial or financial relationships that could be construed as a potential conflict of interest.
Publisher’s Note
All claims expressed in this article are solely those of the authors and do not necessarily represent those of their affiliated organizations, or those of the publisher, the editors and the reviewers. Any product that may be evaluated in this article, or claim that may be made by its manufacturer, is not guaranteed or endorsed by the publisher.
Acknowledgments
We acknowledge all people who helped us to carry out this experiment, especially Dimitri Evrard and Victor Lefebvre for their technical support and the maintenance of bumblebee breeding room. We thank Maryse Vanderplanck for her advice and discussions during the experimentations and Thomas J. Wood for the proofreading. We also thank the two reviewers for their constructive comments which have greatly improved the quality of the manuscript.
References
Ayasse, M., and Jarau, S. (2014). Chemical ecology of bumble bees. Annu. Rev. Entomol. 59, 299–319. doi: 10.1146/annurev-ento-011613-161949
Baer, B. (2003). Bumblebees as model organisms to study male sexual selection in social insects. Behav. Ecol. Sociobiol. 54, 521–533. doi: 10.1007/s00265-003-0673-5
Bálint, M., Domisch, S., Engelhardt, C. H. M., Haase, P., Lehrian, S., Sauer, J., et al. (2011). Cryptic biodiversity loss linked to global climate change. Nat. Clim. Change 1, 313–318. doi: 10.1038/nclimate1191
Barraud, A., Vanderplanck, M., Nadarajah, S., and Michez, D. (2020). The impact of pollen quality on the sensitivity of bumblebees to pesticides. Acta Oecol. 105:103552. doi: 10.1016/j.actao.2020.103552
Beekman, M., and van Stratum, P. (1998). Bumblebee sex ratios: why do bumblebees produce so many males? Proc. R. Soc. Lond. B 265, 1535–1543. doi: 10.1098/rspb.1998.0469
Beekman, M., Van Stratum, P., and Lingeman, R. (1998). Diapause survival and post-diapause performance in bumblebee queens (Bombus terrestris) Entomol. Exp. Appl. 89, 207–214. doi: 10.1046/j.1570-7458.1998.00401.x
Bellard, C., Bertelsmeier, C., Leadley, P., Thuiller, W., and Courchamp, F. (2012). Impacts of climate change on the future of biodiversity. Ecol. Lett. 15, 365–377. doi: 10.1111/j.1461-0248.2011.01736.x
Bergström, G., Svensson, B. G., Appelgren, M., and Groth, I. (1981). Complexity of bumble bee marking pheremones: biochemical, ecological and systematical interpretations. System. Assoc. 19:175.
Calam, D. H. (1969). Species and sex-specific compounds from the heads of male bumblebees (Bombus spp.). Nature 221, 856–857. doi: 10.1038/221856a0
Chirault, M., Lucas, C., Goubault, M., Chevrier, C., Bressac, C., and Lécureuil, C. (2015). A combined approach to heat stress effect on male fertility in Nasonia vitripennis: from the physiological consequences on spermatogenesis to the reproductive adjustment of females mated with stressed males. PLoS One 10:e0120656. doi: 10.1371/journal.pone.0120656
Cnaani, J., Schmid-Hempel, R., and Schmidt, J. O. (2002). Colony development, larval development and worker reproduction in Bombus impatiens Cresson. Insectes Soc. 49, 164–170. doi: 10.1007/s00040-002-8297-8
Coppée, A., Mathy, T., Cammaerts, M. C., Verheggen, F. J., Terzo, M., Iserbyt, S., et al. (2011). Age-dependent attractivity of males’ sexual pheromones in Bombus terrestris (L.) [Hymenoptera, Apidae]. Chemoecology 21, 75–82. doi: 10.1007/s00049-011-0070-x
De Meulemeester, T., Gerbaux, P., Boulvin, M., Coppe, A., and Rasmont, P. (2011). A simplified protocol for bumble bee species identification by cephalic secretion analysis. Ins. Soc. 58, 227–236. doi: 10.1007/s00040-011-0146-1
Dirzo, R., Young, H. S., Galetti, M., Ceballos, G., Isaac, N. J., and Collen, B. (2014). Defaunation in the anthropocene. Science 345, 401–406. doi: 10.1126/science.1251817
Friard, O., and Gamba, M. (2016). BORIS: a free, versatile open−source event−logging software for video/audio coding and live observations. Methods Ecol. Evol. 7, 1325–1330. doi: 10.1111/2041-210X.12584
Gill, R. J., and Raine, N. E. (2014). Chronic impairment of bumblebee natural foraging behaviour induced by sublethal pesticide exposure. Funct. Ecol. 28, 1459–1471. doi: 10.1111/1365-2435.12292
Hallmann, C. A., Sorg, M., Jongejans, E., Siepel, H., Hofland, N., Schwan, H., et al. (2017). More than 75 percent decline over 27 years in total flying insect biomass in protected areas. PLoS One 12:e0185809. doi: 10.1371/journal.pone.0185809
Heinrich, B., and Heinrich, M. J. E. (1983). Size and caste in temperature regulation by bumblebees. Physiol. Zool. 56, 552–562. doi: 10.1086/physzool.56.4.30155878
Inari, N., Nagamitsu, T., Kenta, T., Goka, K., and Hiura, T. (2005). Spatial and temporal pattern of introduced Bombus terrestris abundance in Hokkaido, Japan, and its potential impact on native bumblebees. Pop. Ecol. 47, 77–82. doi: 10.1007/s10144-004-0205-9
Kingsolver, J. G., Diamond, S. E., and Buckley, L. B. (2013). Heat stress and the fitness consequences of climate change for terrestrial ectotherms. Funct. Ecol. 27, 1415–1423. doi: 10.1111/1365-2435.12145
Lecocq, T., Coppée, A., Mathy, T., Lhomme, P., Cammaerts-Tricot, M. C., Urbanová, K., et al. (2015). Subspecific differentiation in male reproductive traits and virgin queen preferences, in Bombus terrestris. Apidologie 46, 595–605. doi: 10.1007/s13592-015-0349-y
Lecocq, T., Gérard, M., Maebe, K., Brasero, N., Dehon, L., Smagghe, G., et al. (2017). Chemical reproductive traits of diploid Bombus terrestris males: consequences on bumblebee conservation. Insect Sci. 24, 623–630. doi: 10.1111/1744-7917.12332
Lhomme, P., Sramkova, A., Kreuter, K., Lecocq, T., Rasmont, P., and Ayasse, M. (2013). A method for year-round rearing of cuckoo bumblebees (Hymenoptera: Apoidea: Bombus subgenus Psithyrus). Ann. Soc. Entomol. Fr. 49, 117–125. doi: 10.1080/00379271.2013.774949
Livesey, J. S., Constable, C., Rawlinson, W. G., Robotham, A. M., Wright, C., Hampshire, A. E., et al. (2019). The power and efficiency of brood incubation in queenless microcolonies of bumble bees (Bombus terrestris L.). Ecol. Entomol. 44, 601–609. doi: 10.1111/een.12736
Maebe, K., De Baets, A., Vandamme, P., Vereecken, N. J., Michez, D., and Smagghe, G. (2021a). Impact of intraspecific variation on measurements of thermal tolerance in bumble bees. J. Therm. Biol. 99:103002. doi: 10.1016/j.jtherbio.2021.103002
Maebe, K., Hart, A. F., Marshall, L., Vandamme, P., Vereecken, N. J., Michez, D., et al. (2021b). Bumblebee resilience to climate change, through plastic and adaptive responses. Glob. Change Biol. 27, 4223–4237. doi: 10.1111/gcb.15751
Martinet, B., Zambra, E., Przybyla, K., Lecocq, T., Anselmo, A., Nonclercq, D., et al. (2021b). Mating under climate change: impact of simulated heatwaves on the reproduction of model pollinators. Funct. Ecol. 35, 739–752. doi: 10.1111/1365-2435.13738
Martinet, B., Dellicour, S., Ghisbain, G., Przybyla, K., Zambra, E., Lecocq, T., et al. (2021a). Global effects of extreme temperatures on wild bumblebees. Conserv. Biol. 35, 1507–1518. doi: 10.1111/cobi.13685
Martinet, B., Lecocq, T., Smet, J., and Rasmont, P. (2015). A protocol to assess insect resistance to heat waves, applied to bumblebees (Bombus Latreille, 1802). PLoS One 10:e0118591. doi: 10.1371/journal.pone.0118591
McAfee, A., Chapman, A., Higo, H., Underwood, R., Milone, J., Foster, L. J., et al. (2020). Vulnerability of honey bee queens to heat-induced loss of fertility. Nat. Sustain. 3, 367–376. doi: 10.1038/s41893-020-0493-x
Meehl, G. A., and Tebaldi, C. (2004). More intense, more frequent, and longer lasting heat waves in the 21st century. Science 305, 994–997. doi: 10.1126/science.1098704
Minnameyer, A., Strobl, V., Bruckner, S., Camenzind, D. W., Van Oystaeyen, A., Wäckers, F., et al. (2021). Eusocial insect declines: insecticide impairs sperm and feeding glands in bumblebees. Sci. Total Environ. 785:146955. doi: 10.1016/j.scitotenv.2021.146955
Oksanen, J., Blanchet, G. F., Friendly, M., Kindt, R., Legendre, P., McGlinn, D., et al. (2018). vegan: Community Ecology Package. R package version 2.5-1. Available online at: https://CRAN.R-project.org/package=vegan
Oyen, K. J., and Dillon, M. E. (2018). Critical thermal limits of bumblebees (Bombus impatiens) are marked by stereotypical behaviors and are unchanged by acclimation, age or feeding status. J. Exp. Biol. 221:jeb165589. doi: 10.1242/jeb.165589
Oyen, K. J., Giri, S., and Dillon, M. E. (2016). Altitudinal variation in bumble bee (Bombus) critical thermal limits. J. Therm. Biol. 59, 52–57. doi: 10.1016/j.jtherbio.2016.04.015
Oyen, K. J., Jardine, L. E., Parsons, Z. M., Herndon, J. D., Strange, J. P., Lozier, J. D., et al. (2021). Body mass and sex, not local climate, drive differences in chill coma recovery times in common garden reared bumble bees. J. Comp. Physiol. B 191, 843–854. doi: 10.1007/s00360-021-01385-7
Potts, S. G., Imperatriz-Fonseca, V., Ngo, H. T., Aizen, M. A., Biesmeijer, J. C., Breeze, T. D., et al. (2016). Safeguarding pollinators and their values to human well-being. Nature 540, 220–229. doi: 10.1038/nature20588
R Core Team (2018). R: A Language and Environment for Statistical Computing. Vienna: R Foundation for Statistical Computing.
Rasmont, P., Coppée, A., Michez, D., and De Meulemeester, T. (2008). An overview of the Bombus terrestris (L. 1758) subspecies (Hymenoptera: Apidae). Ann. Soc. Entomol. Fr. 44, 243–250. doi: 10.1080/00379271.2008.10697559
Rasmont, P., Franzen, M., Lecocq, T., Harpke, A., Castro, L., Cederberg, B., et al. (2015). Climatic Risk and Distribution Atlas of European Bumblebees. Sofia: Pensoft, 236.
Röseler, P. F. (1985). A technique for year-round rearing of Bombus terrestris (Apidae, Bombini) colonies in captivity. Apidologie 16, 165–170. doi: 10.1051/apido:19850206
Rothman, J. A., Russell, K. A., Leger, L., McFrederick, Q. S., and Graystock, P. (2020). The direct and indirect effects of environmental toxicants on the health of bumblebees and their microbiomes. Proc. R. Soc. B 287:20200980. doi: 10.1098/rspb.2020.0980
Sales, K., Vasudeva, R., Dickinson, M. E., Godwin, J. L., Lumley, A. J., and Michalczyk, Ł, et al. (2018). Experimental heatwaves compromise sperm function and cause transgenerational damage in a model insect. Nat. Commun. 9:4771. doi: 10.1038/s41467-018-07273-z
Sales, K., Vasudeva, R., and Gage, M. J. (2021). Fertility and mortality impacts of thermal stress from experimental heatwaves on different life stages and their recovery in a model insect. R. Soc. Open Sci. 8:201717. doi: 10.1098/rsos.201717
Strobl, V., Albrecht, M., Villamar-Bouza, L., Tosi, S., Neumann, P., and Straub, L. (2021). The neonicotinoid thiamethoxam impairs male fertility in solitary bees, Osmia cornuta. Environ. Pollut. 284:117106. doi: 10.1016/j.envpol.2021.117106
Taseï, J. N., and Aupinel, P. (2008). Nutritive value of 15 single pollens and pollen mixes tested on larvae produced by bumblebee workers (Bombus terrestris, Hymenoptera: Apidae). Apidologie 39, 397–409. doi: 10.1051/apido:2008017
Terzo, M., and Sinkevich, V. (2005). “Development of an experimental protocol for the quantification of the attractivity of the male sexual pheromones of the Bombus terrestris,” in Proceedings of the 3rd European Congress on Social Insects, ed. V. E. Kypiatkov (St. Petersburg: St. Petersburg University Press), 204.
Torretta, J. P., Medan, D., and Arahamovich, A. H. (2006). First record of the invasive bumblebee Bombus terrestris (L.) (Hymenoptera, Apidae) in Argentina. T. Am. Entomol. Soc. 132, 285–289.
Valterová, I., Martinet, B., Michez, D., Rasmont, P., and Brasero, N. (2019). Sexual attraction: a review of bumblebee male pheromones. Z. Naturforsch. 74, 233–250. doi: 10.1515/znc-2019-0003
Vanderplanck, M., Martinet, B., Carvalheiro, L. G., Rasmont, P., Barraud, A., Renaudeau, C., et al. (2019). Ensuring access to high-quality resources reduces the impacts of heat stress on bees. Sci. Rep. 9:12596. doi: 10.1038/s41598-019-49025-z
Walsh, B. S., Parratt, S. R., Hoffmann, A. A., Atkinson, D., Snook, R. R., Bretman, A., et al. (2019). The impact of climate change on fertility. Trends Ecol. Evol. 34, 249–259. doi: 10.1016/j.tree.2018.12.002
Williams, I. H. (1994). The dependence of crop production within the European Union on pollination by honey bees. Agric. Zool. Rev. 6, 229–257.
Yoon, H. J., Lee, K. Y., Hwang, J. S., and Park, I. G. (2010). Chilling temperature and humidity to break diapause of the bumblebee queen Bombus terrestris. Int. J. Entomol. Res. 20, 93–98.
Keywords: climate change, heat exposure, bumblebee, reproduction, colony development
Citation: Przybyla K, Michez D, Zambra E, Anselmo A, Hennebert E, Rasmont P and Martinet B (2021) Effects of Heat Stress on Mating Behavior and Colony Development in Bombus terrestris (Hymenoptera: Apidae). Front. Ecol. Evol. 9:748405. doi: 10.3389/fevo.2021.748405
Received: 27 July 2021; Accepted: 30 September 2021;
Published: 21 October 2021.
Edited by:
Brad Metz, North Carolina State University, United StatesReviewed by:
Zhu Wanlong, Yunnan Normal University, ChinaHannah Levenson, North Carolina State University, United States
Copyright © 2021 Przybyla, Michez, Zambra, Anselmo, Hennebert, Rasmont and Martinet. This is an open-access article distributed under the terms of the Creative Commons Attribution License (CC BY). The use, distribution or reproduction in other forums is permitted, provided the original author(s) and the copyright owner(s) are credited and that the original publication in this journal is cited, in accordance with accepted academic practice. No use, distribution or reproduction is permitted which does not comply with these terms.
*Correspondence: Kimberly Przybyla, kimberly.przybyla@umons.ac.be; orcid.org/0000-0001-9663-4179