- 1School of Life Sciences, Hebei University, Baoding, China
- 2State Key Laboratory of Integrated Management of Pest Insects and Rodents, Institute of Zoology, Chinese Academy of Sciences, Beijing, China
- 3Institute of Life Sciences and Green Development, Hebei University, Baoding, China
Phenological asynchrony is a common and important natural phenomenon that affects interspecific interaction, resource allocation, species survival, and range shift in sympatric species. However, the underpinnings for regulating phenological asynchrony at physiological and molecular levels remains less explored. We investigated the seasonal pattern of emergence period and abundance in three dominant grasshopper species, namely, Dasyhipus barbipes, Oedalus asiaticus, and Chorthippus dubius, which occur sympatrically in the Inner Mongolian steppe. The three grasshopper species decoupled their population occurrence phenology that occurred in a growing season between May and September and diverged into early, middle, and late seasonal species. We also examined the association of embryonic diapause and heat shock protein (Hsp) expression with phenological asynchrony in the three species. The species developed different embryonic diapause programs, i.e., obligate diapause, facultative diapause, and non-diapause, to control the timing of egg hatching and seasonality of population occurrence. The diapausing eggs exhibited significantly enhanced supercooling capacity compared with pre- and post-diapausing eggs. Gene expression analysis in the developmental process revealed that three Hsps, e.g., Hsp20.6, Hsp40, and Hsp90, were significantly upregulated in diapause state relative to that in pre- and post-diapause states; expression of these genes seems to be associated with the diapause program regulation. This study provides a possible mechanistic explanation for phenological differentiation among sympatric species in a typical steppe habitat and establishes a potential linkage among phenological asynchrony, diapause, and Hsp gene expression. The findings will facilitate our prediction of population dynamics and pest management.
Introduction
Phenology, the seasonal timing of biological activities of a species, is related to the development, reproduction, and survival of organisms and affects almost all aspects of ecology and evolution (Forrest and Miller-Rushing, 2010). Phenological asynchrony/synchrony of related species is a common phenomenon in nature and can affect inter-specific interaction, resource allocation, species survival, range shift, and evolutionary trajectory (Bradley et al., 1999; Warren Ii et al., 2011; Bartomeus et al., 2013). Phenological shift in insects can influence their synchrony with biotic resources (e.g., plant hosts) or abiotic cues (e.g., temperature fluctuation), alter voltinism pattern, and subject them to exposure to seasonal weather extremes during sensitive life stages. Taking herbivorous insects as an example, phenology asynchrony can avoid interspecific competition to a certain extent, adapt to the developmental phenology of host plants, and ensure the reproduction of their own population to the greatest extent (Singer and Parmesan, 2010; Johansson et al., 2015). Thus, the phenological pattern and varying extent in organism under changeable environmental conditions are crucial for ecological interactions and could be affected by strong natural selection.
Ecological factors, such as temperature cues, precipitation, resource availability, host-predator interaction, and inter-specific competition, affect phenological synchrony and asynchrony (Korpimaki et al., 2005; Johansson et al., 2015). For example, over the past decades, the phenology of many plant and animal species has been altered, and the shifts are related to climate change and anthropogenic disturbances (Visser and Both, 2005; Sherry et al., 2007; Kerby et al., 2012). Consequently, phenological differentiation has become an increasingly important topic worldwide (Ellwood et al., 2012).
Phenology of many arthropods could be controlled by diapause, a physiological state of dormancy with low metabolic activity and increased stress tolerance (King and MacRae, 2014). Diapausing insects slow or halt their development and enhance their tolerance to unfavorable environmental conditions (Denlinger, 2002). The diapause states among insect species can either be obligate or facultative but is generally restricted to one life history stage. Insects with obligate diapause program enter diapause on schedule no matter how the external environmental conditions are, while those with facultative diapause program can be induced into diapause state at certain stages or released from diapause arrest by specific external environmental signals (Denlinger, 2002). The onset and termination of diapause are determined by environmental cues, such as photoperiod, temperature, food, and population density; as such, diapause could be associated with phenological synchrony (Tauber and Tauber, 1976; van Asch and Visser, 2007). Normal development of diapausing individuals resumes only when they experience certain environmental signals, e.g., chilling below a threshold temperature for diapause termination. Thus, diapause is considered a key life history trait of insects to ensure the synchronization of their life cycle with seasonal changes in the environment (Tauber and Tauber, 1976). However, limited information is known regarding how distinct diapausing programs of different species in an ecosystem, e.g., sympatric species with similar trophic niches, are coupled to their phenological asynchrony to facilitate seasonal adaptation and resource competition.
Although several ecological and physiological mechanisms have been proposed for phenological asynchrony, the underlying molecular basis has been rarely investigated. Heat shock proteins (Hsps) exhibit several distinct properties that are closely associated with diapause and phenological changes (Denlinger, 2002; Rinehart et al., 2007; King and MacRae, 2014). Hsps are highly conserved molecular chaperones and can be elicited as active responses to environmental stimulation and adaptation to environmental stresses. Hsps consist of several families, namely, Hsp90, Hsp70, and small Hsps, which are classified based on their molecular weights. Hsps play various important roles in the successful folding, assembly, intracellular localization, secretion, regulation, and degradation of proteins (Feder and Hofmann, 1999). Hsps regulate stress resistance, cell development, and cell reproduction; hence, Hsp expression is crucial for insect survival during periods of stress and influences species abundance and overwintering in nature (Huang et al., 2007; Kang et al., 2009). During diapause the expression of Hsps and their protein products may increase, decrease, or remain unchanged (King and MacRae, 2014). As an example, in the flesh fly Sarcophaga crassipalpis, most, but not all, Hsps in diapaused larvae are upregulated (Rinehart et al., 2007). In contrast, Hsp90 is downregulated during diapause and upregulated before and after diapause. Hsps also affect cold tolerance that is a component of the diapause program of overwintering insects (Rinehart et al., 2007). These results represent an association between expression of Hsps and diapause occurrence. Nevertheless, the up-regulation of Hsps during diapause did not alter the decision to enter diapause or the duration of diapause (Rinehart et al., 2007). In all, the association between Hsp expression and diapause regulation is complex, and more direct evidence for the association is still lacking.
To address the underlying molecular mechanism for phenological synchrony, we here studied three dominant grasshopper species, namely, Dasyhipus barbipes, Oedalus asiaticus, and Chorthippus dubius, which are sympatric and abundant in the Inner Mongolian steppe (Figure 1A). According to the Department of Agriculture of China, the annual pest damaged area in Inner Mongolia grassland was as much as 4.6 million hm2, among which the seriously damaged area by the locusts and grasshoppers reached 2.3 million hm2 (Bai et al., 2012). These grasshoppers have frequent occurrence in the grassland that caused serious damage to grassland ecology and animal husbandry production in the northern China.
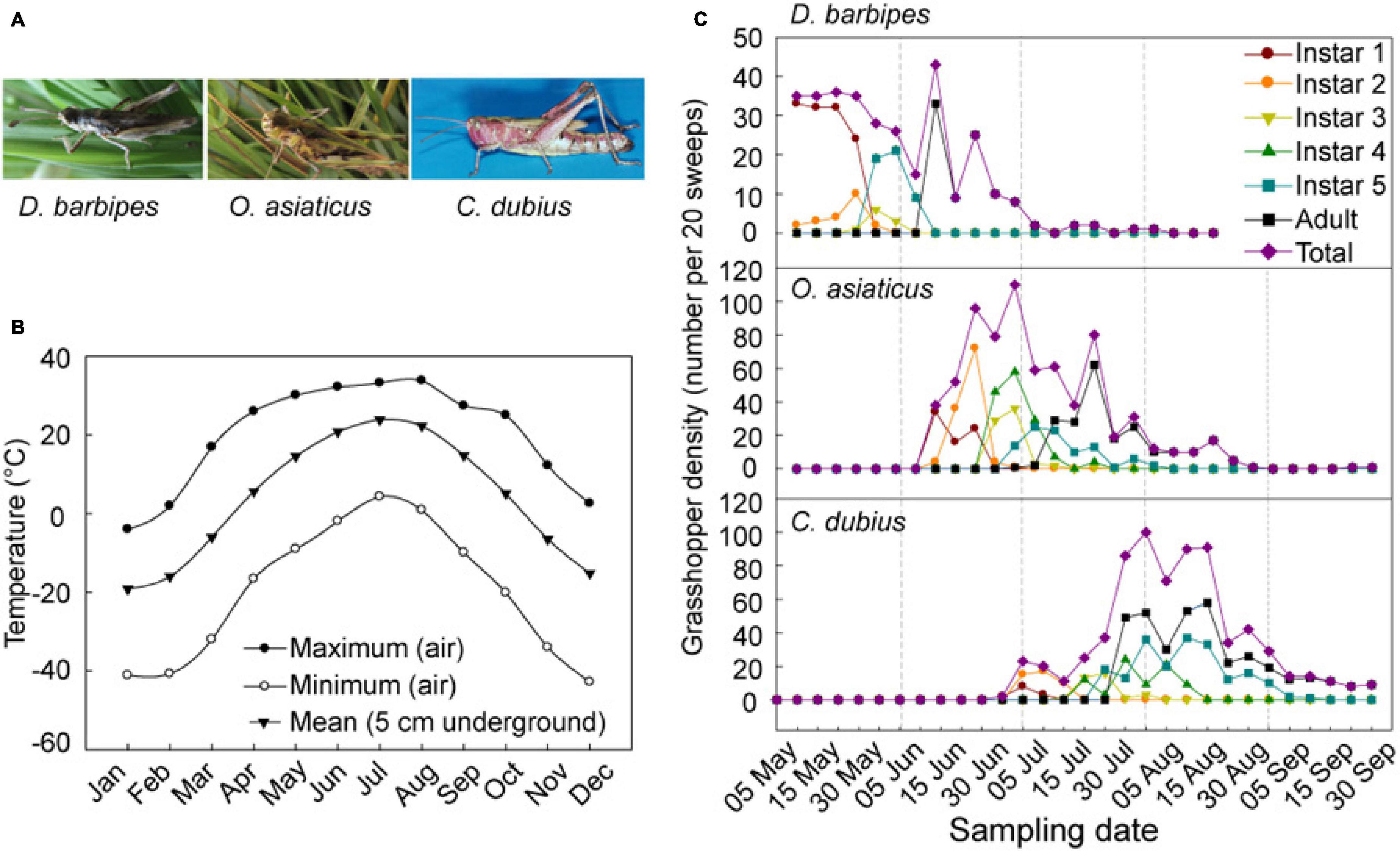
Figure 1. Seasonal temperature fluctuations and population dynamics of the three grasshopper species in the Inner Mongolia steppe of China. (A) Images of adults three grasshopper species, namely, D. barbipes, O. asiaticus, and C. dubius. (B) Monthly maximum and minimum temperatures in air and mean temperatures in soil in the Inner Mongolia Grassland Ecosystem Research Station (1970–2000). The mean temperatures in soil were recorded in 5 cm below the ground surface, where most eggs are deposited by grasshopper adults. (C) Phenological pattern of population abundance of the three grasshopper species. The survey period was between May 05 and Sept. 30 in 2002. The grasshoppers were sampled every 5–6 days. The seven different lines represent six developmental stages (i.e., nymphs of instar 1, 2, 3, 4, and 5, and adults) and the total populations (i.e., the sum of individuals at all developmental stages).
The three dominant grasshopper species have fast embryonic developmental rate with low developmental thresholds and sum of effective temperature (Hao and Kang, 2004a,c). Analysis of trophic niche and food resource utilization of the three grasshoppers showed that they possess similar host preferences. The former represents graminivorous species, the latter two belongs to mixed graminivorous species, but these species are mainly feeding on Poaceae plant, such as the grass Leymus chinensis and Stipa spp. (Li et al., 1987; Kang and Chen, 1994b). Despite the sympatric food competition, the three species exhibit prominent phenological asynchrony with their occurrence. Such asynchrony has been considered to be a good strategy to avoid interspecific competition and contribute to greater destruction in grassland (Kang and Chen, 1994a). However, the mechanisms underlying the asynchrony of seasonal occurrence of the three species remains a mystery.
In this study, we investigated the regulation of the asynchrony of the three grasshoppers in the Innner Mongolian steppe. We propose that the phenological asynchrony of the three grasshopper species is associated with diapausing program, which is related to Hsp expression. To test this hypothesis, we examined the developmental process and diapause events in the embryonic developmental progress. This study will improve our understanding of interspecific phenological asynchrony and facilitates our prediction of phenological responses to climate changes.
Materials and Methods
Study Sites
The grasshopper, D. barbipes, O. asiaticus, and C. dubius (Figure 1A) are widely distributed in the Inner Mongolian grassland of China (Li et al., 1987, 2007; Kang and Chen, 1995). Phenology survey and sampling of the grasshoppers were carried out near the Inner Mongolia Grassland Ecosystem Research Station of the Chinese Academy of Sciences (43°38′N, 116°42′E, 1187 m above sea level). The station is located in typical steppe region, which is characterized by dark chestnut soil with relatively homogeneous physiochemical properties. The dominant plants include Leymus chinensis, Stipa grandis, Artemisia frigida, Cleistogenes squarrosa, Agropyron cristatum, and Potentilla acaulis (Li et al., 2007).
Grasshopper Phenology Survey
The annual phenology of the three grasshopper species was monitored continuously for field sampling by using net sweep method. One sweep means a net (35 cm, diameter; 1.2 m, handle) return that traverses an arc of 180° through the vegetation (Evans, 1992). Sampling was conducted between 09:30 and 16:30 when the weather is sunny with light wind. Field sampling started in early May and continued with an interval of 5–6 days until late September in 2002. Each sample containing 20 sweeps was collected in a 20 m transect. Twenty samples were tessellated in grazing and ungrazing fields in each sampling period. All grasshoppers in each sample were sealed in a plastic bag and transferred to a laboratory. The insects were killed in a freezer at −18°C. Each specimen was then checked for its species and nymph instar.
Egg Collection and Incubation
Adults of the three grasshopper species were caught in field during adult peak periods of their occurrence. The adults were reared in a wooden cage (60 cm × 60 cm × 60 cm) at a density of approximately 200 individuals per cage (♀:♂=11). Each cage had five holes at the bottom to fit in with a cup (diameter and height of 12 cm); the cup was filled with sterilized sand (40 meshes) with 8% humidity (w/w) for adult oviposition. All grasshoppers were fed with bran and fresh grasses (L. chinenses and S. grandis) cut from the field every day. Light was provided using two 40 W incandescent lamps at the top of cage with a photoperiod of 14: 10 h (L: D). The temperature was controlled at 30 ± 2°C during daytime and 20 ± 2°C at night. Egg pods were collected from the oviposition cup every 2 days and kept in a plastic cup (diameter, 6 cm; height, 15 cm) filled with sterilized sand with 8% humidity. The cups with egg pods were sealed with plastic film and maintained in a low-temperature chamber at 5 ± 1°C and 50% humidity to ensure that all eggs were in the same embryonic stage when they were used for subsequent measurements.
Examination of Embryonic Development and Diapause
To reveal the process of embryonic development and possible diapausing events in the three grasshopper species, we examined the embryonic developmental stages and diapause-related traits (Supplementary Figure 1). A total of 36 replicate plastic cups with egg pods of each grasshopper species were incubated in the climate chambers at 30 ± 1°C for 10, 20, and 30 days. At each time interval, egg pods with approximately 100 eggs was removed from one plastic cup and dissected to determine the embryonic developmental stages. Another five replicates with 100 eggs were sampled for RNA preparation and gene expression assay. Each replicate of eggs was sealed in a 5 ml polypropylene tube, frozen in liquid nitrogen, and stored in −80°C freezer. According to the embryo examination, D. barbipes and O. asiaticus entered into diapause within 30 days (see section “Results”). Another 18 replicates of egg pods in plastic cups for each grasshopper species were transferred to 8°C freezer after 30 days of incubation and maintained for 60 days to terminate the diapause program. Afterward, the eggs were transferred back to the climate chamber at 30 ± 1°C to recover embryonic development. The eggs were cultured for another 10, 20, and 30 days, and the embryonic development or RNA preparation was subsequently examined, as stated previously (Supplementary Figure 1). Embryonic developmental stage was determined under a microscope (Leica Application Suite M205C). The entire developmental period of grasshopper embryos was classified into 27 stages according to a quantitative staging system (Van Horn, 1966). The great majority of embryos enter diapause at stage 19 that is characterized by developmental arrest, revolution halt and deposition of eye pigmentation (Van Horn, 1966; Hao and Kang, 2004c).
Measurement of Supercooling Points
To discover seasonal variations in cold tolerance of eggs in the three grasshopper species, we examined the supercooling points (SCP) of eggs buried in the field (Supplementary Figure 1). Embryo can survive at subzero temperatures by supercooling body fluid to a temperature at which spontaneous nucleation occurs; consequently, fluid freezing at 0°C is prevented (Duman, 2001). SCP of eggs is a reliable indicator of cold tolerance for grasshoppers (Jing and Kang, 2003; Hao and Kang, 2004b). The eggs came from the wild grasshopppers whose adults were caught in the field and reared in the laboratory and laid eggs for embryo assay. The three replicates of plastic cups with grasshopper egg pods were embedded in the field and covered with 3–5 cm local soil in 30 July, 30 August, and 30 September for D. barbipes, O. asiaticus, and C. dubius, in accordance with the egg laying period of each grasshopper species. One replicated cup with egg pods was retrieved at different time point according to embryonic developmental process for each grasshopper: 31 August, 30 September, and 15 April for D. barbipes; 30 September, 31 October, and 15 May O. asiaticus; 31 October, 15 May, and 15 June for C. dubius. S and developmental states of eggs were examined in laboratory (Supplementary Figure 1). The SCP of each egg was measured using a thermal couple attached to an automatic recorder (μR100, Model 4152, Yologawa Electric Co., Seoul) (Hao and Kang, 2004b). The egg was dissected immediately after the SCP measurement to determine the embryonic developmental stages and diapause states. At least 18 eggs were examined at each time point.
RNA Extraction, cDNA Synthesis, Gene Cloning, and Gene Sequencing
RNA sample was purified using the RNeasy Mini Kit (Qiagen, Germany) according to the manufacturer’s instructions. Two micrograms of purified total RNA were reverse transcribed with an oligo-dT adaptor primer to synthesize the first-single-stranded cDNA with M-MLV reverse transcriptase according to the manufacturer’s instructions (Promega). The synthesized first-strand cDNA was used as a template for cloning of the four Hsp genes, i.e., Hsp20.6, Hsp40, Hsp70, and Hsp90. Degenerate oligonucleotide primers for genes encoding the Hsps were designed on the basis of conserved amino acid sequences of insect Hsp orthologs. The degenerate primers were used to amplify the middle region of the Hsp genes. The resultant PCR products of about 500 base pairs were cloned into the PCR-II vector using the TA cloning system (Invitrogen). The identity of the cloned Hsp transcripts was validated via sequencing and BLAST search. All the degenerate primers for gene cloning are listed in Supplementary Table 1.
Measurement of mRNA Level by Real-Time Quantitative PCR
Gene expression was determined by quantitative real-time PCR using SYBR Green I kit (Roche, Switzerland) according to the manufacturer’s instructions. PCR was performed on the Light Cycler® 480 Real-Time PCR System. Rp49 was used as internal control (Chen et al., 2015). The thermocycler conditions were 95°C for 30 s, followed by 40 cycles at 95°C for 5 s and 60°C for 34 s. The specificity of amplification was confirmed through the melting curve analysis. The relative expression was quantified by using the comparative cycle threshold method (2–Δ Ct method) and normalized against that of the internal control (Chen and Wagner, 2012). At least three biological replicates of eight eggs were prepared for each treatment. All reactions were performed in triplicate. Primers for quantitative PCR are listed in Supplementary Table 1.
Statistical Analysis
One-way ANOVA and post hoc multiple comparisons using Tukey HSD T-test were performed to evaluate differences in mean SCPs of low-SCP and high-SCP group, and the expression of each Hsp gene. In non-parametric tests, Kruskal-Wallis one-way ANOVA for 3 groups and Mann-Whitney U-test for 2 group of frequency of individual SCPs were performed. Differences were considered significant at P < 0.05. Data were analyzed using SPSS 16.0 software (SPSS, Chicago).
Results
Phenological Asynchrony in the Three Grasshopper Species
The mean annual temperature in the grassland near the research station is 0.3°C, and the mean monthly temperature ranges from −21.6°C in January to 19.0°C in July (Bai et al., 2004). The mean temperature at 5 cm below ground, where most grasshopper eggs are oviposited, is about −20.5°C in January; this temperature is the minimum throughout the year. Subsequently, the temperature increases to above 10°C in April, which reaches the developmental threshold temperature of embryo for all grasshopper eggs (Hao and Kang, 2004a,c). The temperature reaches the maximum of 25°C in July, begins to decrease from August, and becomes close to zero in October (Figure 1B and Supplementary Table 2).
We investigated the population dynamics of D. barbipes, O. asiaticus, and C. dubius in the Inner Mongolian steppe during May–September (Figure 1C and Supplementary Table 3). The results showed that populations of D. barbipes started egg hatching earlier than May 5th, with peaks of hatchling occurrence on May 5–15, and ended on July 5th. The most abundant occurrence of the adult grasshopper was in 10–20 June. The total populations of the species at different developmental stages remained abundant from early May to middle June, with an active season of more than 60 days. For O. asiaticus, the first instar nymph was observed on June 10. The adults peaked on 20 July and end on 30 August. The species maintained high population abundance at different developmental stages from middle June to late July. The total populations of O. asiaticus remained active for a season of 82 days. The egg hatching of C. dubius didn’t occurred till on 30 June. The number of adults remained high in August. The abundant season for this grasshopper was between middle July and late August. The whole populations of C. dubius lasted for 92 days (Figure 1C). Therefore, the three grasshopper species possessed a similar nymph–adult duration of no more than 1.5 months in the Inner Mongolian steppe. However, C. dubius has a longer growth season than the other two species. The species formed a temporal sequential development in the local ecosystem; thus, no apparent temporal and trophic niches overlapped in the year. This result revealed the interspecific phenological asynchrony of emergence and abundance in the three grasshopper species. Hence, we defined D. barbipes, O. asiaticus, and C. dubius as early, middle, and late species, respectively.
Embryonic Development and Diapause
We investigated the embryonic development and diapause program of the three grasshopper species by first culturing newly laid eggs at 30°C; afterward, development of embryos was examined (Figure 2A). The embryo of D. barbipes, developed to the average stage of 10.6 (n = 92), 15.4 (n = 122), and 18.6 (n = 67) after 10, 20, and 30 days of incubation, respectively (Figure 2B). Eggs stop their development after the 30 days of incubation. We then subjected the eggs to cooling treatment at 8°C to terminate diapause development. The embryos resumed to their development and reached stage 21.5, 22.6, and 25.1 after another 10, 20, and 30 days of incubation at 30°C, respectively. The results indicated that D. barbipes is an obligate diapause species and survives the autumn–winter season by entering diapause at stage 19 (Figure 2B).
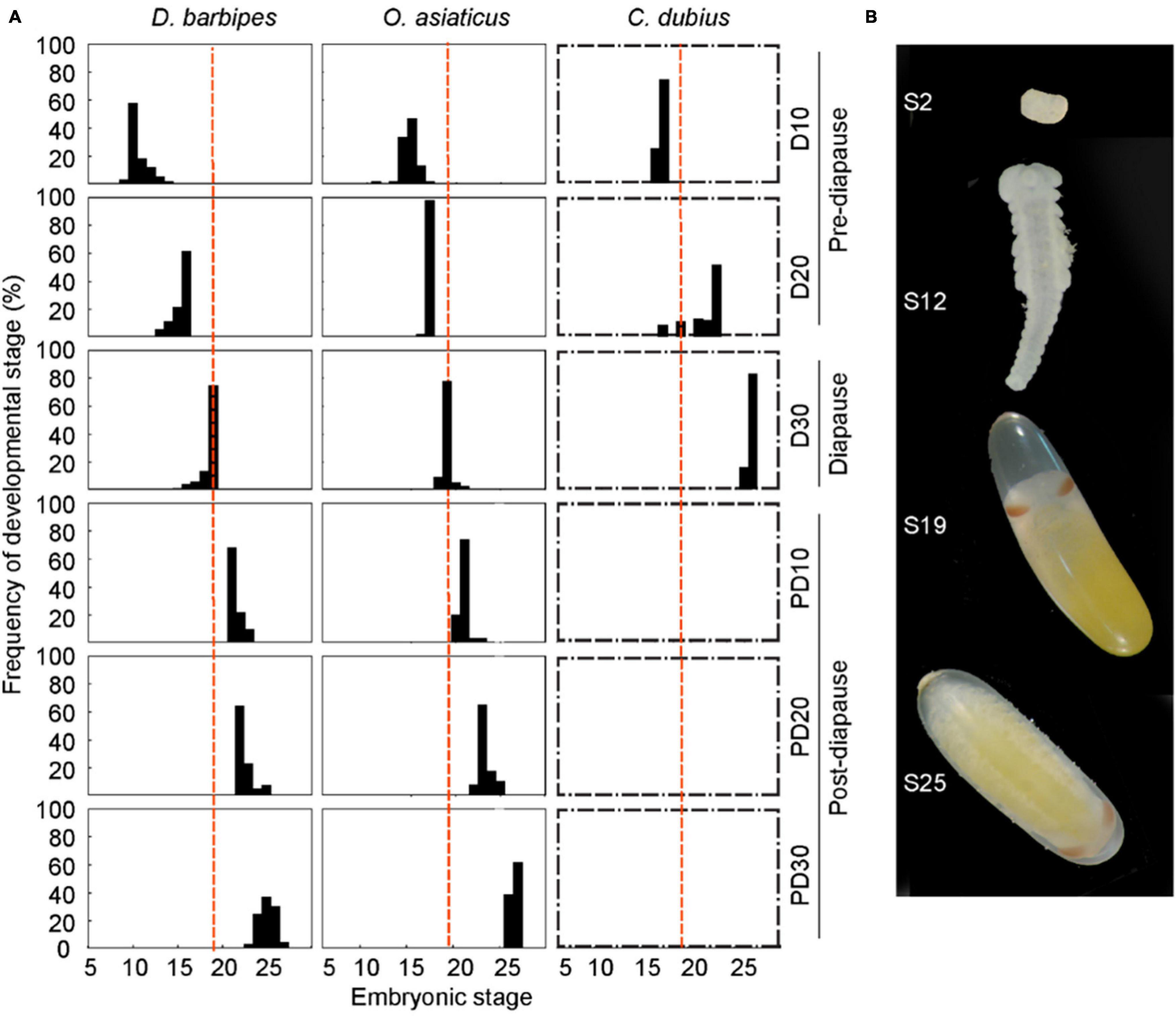
Figure 2. Pace of embryonic development associated with diapause programs in the three grasshopper species. (A) Divergence in the rate of embryonic development in the three grasshopper species with different diapause programs. Newly laid eggs were first incubated at 30 ± 1°C for 10, 20, and 30 days (i.e., D10, D20, and D30, respectively). Eggs were then kept at 8°C incubator for 60 days to terminate embryonic diapause in D. barbipes and O. asiaticus. Finally, the eggs were returned to 30 ± 1°C incubator and maintained for another 10, 20, and 30 days (i.e., PD10, PD20, and PD30, respectively). Red broken line represents the stage 19 of total 27 embryonic stages at which the egg embryos of the grasshopper enter diapause. The early species, D. barbipes, is an obligate diapause grasshopper, in which all embryos entered into diapause process at stage 19 at D30 and showed no continued development until cold treatment. The middle species, O. asiaticus, exhibits facultative diapause, in which approximately 5% of embryos developed beyond stage 19 and did not enter diapause. The later species, C. dubius, is non-diapause because all embryos exceeded stage 19 successfully and hatched at D30. Boxes with broken line indicate that the diapause program is not applicable to the species. One hundred eggs were dissected for embryonic developmental examination at each time point. (B) Photographs of embryos and eggs at different embryonic developmental stages in D. barbipes. Note that embryo at stage 19 entered into diapause but did not start anatrepsis.
In the middle species O. asiaticus, the embryo reached the average stage of 15.1, 16.9, and 19.1 after 10, 20, and 30 days, respectively. However, a small portion of embryos exceeded stage 19 and completed anatrepsis at day 30. After cold induction at 8°C, followed by another 10, 20, and 30 days at 30°C, the embryos developed to stage 20.9, 23.3, and 26.6, respectively (Figure 2). The middle species exhibited a characteristic of facultative diapause.
The late species C. dubius developed to the average stage of 16.7 (n = 188) and 21.5 (n = 97) after 10 and 20 days of incubation at 30°C (Figure 2A and Supplementary Table 4). At day 30, 70% (n = 120) of the embryos reached the final stage (stage 27), and approximately 20% of the embryos completed development and were hatched. Therefore, C. dubius displayed no diapause program during egg development.
The comparative analysis revealed an evolutionary divergence in the diapause program in the three sympatric species. Furthermore, the egg of late non-diapause species exhibited the fastest developmental rate, and the eggs of the early species showed the slowest rate before diapause.
Supercooling Capacity
We examined the SCPs of eggs in pre-diapause, diapause, and post-diapause states in the three grasshopper species. We then analyzed the distribution of frequency of individual SCPs in the three diapause states (Figure 3A and Supplementary Table 5). Non-parametric tests by Kruskal-Wallis ANOVA in the three species all showed that SCPs differ greatly significantly among the three diapause states (P < 0.05). Specifically, in the early species, the distribution of SCPs in pre-diapause eggs is significantly different from that in diapause eggs (Mann-Whitney U-test, P = 0.014), and that in diapause eggs is greatly significantly different from that in post-diapause eggs (P < 0.001). In the middle species, the distribution of SCPs in diapause eggs is also greatly significantly different from that in post-diapause eggs (P < 0.001). These results indicate that SCPs vary with diapause state in the three species.
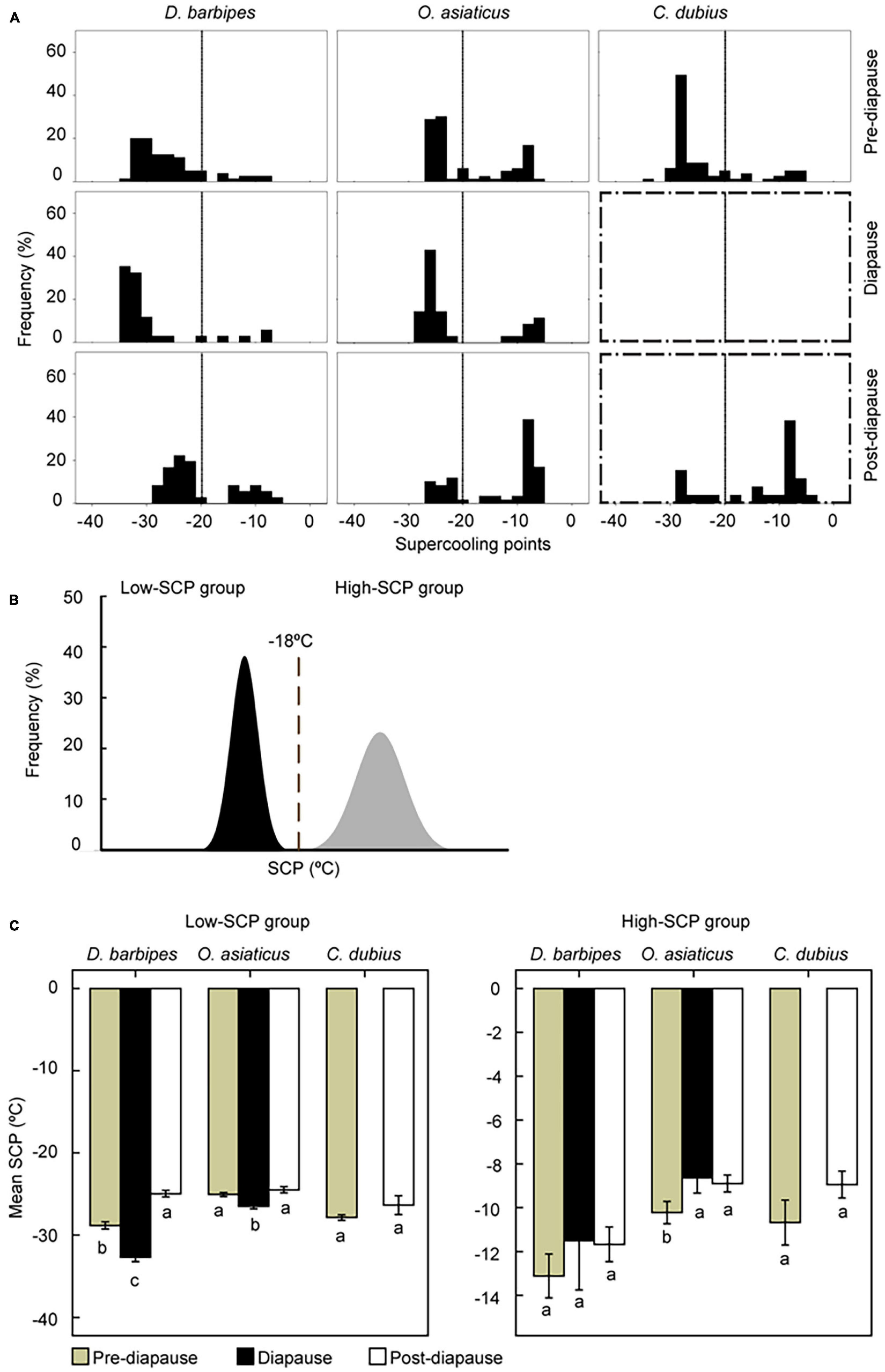
Figure 3. Variation of supercooling points (SCP) of eggs with diapause states in the three grasshopper species. (A) The frequency distribution in their SCPs of eggs with different diapause state in the three species. At least 60 eggs were subject to the measurement of SCPs in each treatment. Boxes with broken line dictate diapause program doesn’t apply to the species. The embryonic stages of grasshopper eggs subject to SCP measurement were at stage 13–15, stage 19 and stage 25–26 for pre-diapause, diapause and post-diapause stages. The total number of eggs in each group were normalized into 100 so that the distribution is comparable. (B) Schematic representation of the bimodal distribution of SCPs in a population. The SCPs of individuals in a natural population can be clearly divided into high-SCP and low-SCP groups at approximately −18°C. (C) SCP values of eggs in different diapause states. Averaged SCPs of eggs in low-SCP (left panel) and high-SCP (right panel) groups are demonstrated. Different letters below the columns mean significant difference at P < 0.05 via Turkey HSD test. The error bars represent 1 SE.
The frequency of individual SCPs exhibited a clear bimodal distribution: SCPs of each species can be divided into discrete high-SCP and low-SCP groups, with a division at −18°C (Figure 3B). Specifically, the frequency of eggs in low-SCP group in pre-diapause state was 87.5, 66.27, and 81.48% for the early, middle, and late species, respectively. The frequency was still high for the early and middle species in diapause state. Nevertheless, the frequency of low-SCP eggs decreased to 69.44, 32.20, and 26.92% for the three grasshopper species in post-diapause state (Figure 3A). Therefore, the frequency distribution of eggs in low-SCP group shifted when the embryonic diapause program was terminated.
The averaged SCP in low-SCP group also varied with diapause state (Figure 3C). In the early species, the averaged SCP was significantly different among the three diapause states (One-way ANOVA, F = 120.43, n = 244, P < 0.001). The SCP was high (mean = −27.8°C, SE = 0.39, n = 88) in pre-diapause eggs, but it decreased considerably to −31.8°C (SE = 0.31, n = 88) in diapause eggs and increased to relatively higher temperature (mean = −24.2°C, SE = 0.26, n = 69) in post-diapaus eggs. In the middle species, the mean SCPs were −24.5°C (SE = 0.22, n = 66), −25.8°C (SE = 0.17, n = 74), and −23.7°C (SE = 0.14, n = 32) in pre-diapause, diapause, and post-diapause eggs, respectively; the diapaused eggs exhibited the lowest SCPs among the three diapause states (One-way ANOVA, F = 22.57, n = 172, P = 0.002). In the late species, the mean SCPs of eggs at the pre- and post-diapause eggs were the same (mean1 = −26.8°C, SE = 0.28; mean2 = −26.2°C, SE = 0.44; t-test, F = 0.01, n = 107, P = 0.319). By contrast, SCP values in the high-SCP group generally remained unchanged in different diapause states in the three species (One-way ANOVA: in the early species, F = 1.10, P = 0.34, n = 53; in the middle species, F = 4.91, P = 0.009, n = 125; in the late species, F = 2.74, P = 0.10, n = 85; Figure 3C). Thus, the supercooling capacity was enhanced in diapaused eggs.
Diapause Related Heat Shock Protein Expression
We also examined the expression of four Hsp genes, i.e., Hsp20.6, Hsp40, Hsp70, and Hsp90, in different developmental stages of embryos to determine whether Hsp expression was associated with the regulation of diapause and supercooling capacity of embryos in the grasshoppers (Figure 4 and Supplementary Table 6). In the early species, the expression of the three Hsp genes (except Hsp70) was significantly upregulated in diapaused eggs compared with that in pre-diapause and post-diapause eggs, although they differed significantly among developmental stages (one-way ANOVA, P < 0.001 for all the four Hsps). Specifically, the embryonic expression of Hsp20.6, Hsp40, and Hsp90 in diapause state (day 30) significantly increased by 3. 9-, 0. 7-, and 3.2-fold relative to that in pre-diapause state (day 20) (t-test, P < 0.001 for the three genes). The expression of the three Hsps significantly decreased to 2. 4-, 1. 3-, and 2.2-fold after diapause termination (Independent samples t-test, P < 0.001 for the three Hsps). However, Hsp70 expression significantly decreased in diapause eggs relative to that in the pre-diapause eggs (t-test, P < 0.001) and remained constant in the post-diapause stages (Figure 4). On the contrary, the embryonic expression of Hsp40, Hsp70, and Hsp90 remained unchanged in pre-diapause state (day 10 and 20, t-test, P > 0.05). The expression of the four Hsps also remained the same between day 10 and 30 in post-diapause eggs (t-test, P > 0.05). Therefore, the expression of Hsp20.6, Hsp40, and Hsp90 genes in D. barbipes was prominently upregulated when the embryo entered into diapause state. Moreover, the diapause-associated substantial upregulation of the three Hsps was independent of the developmental progress.
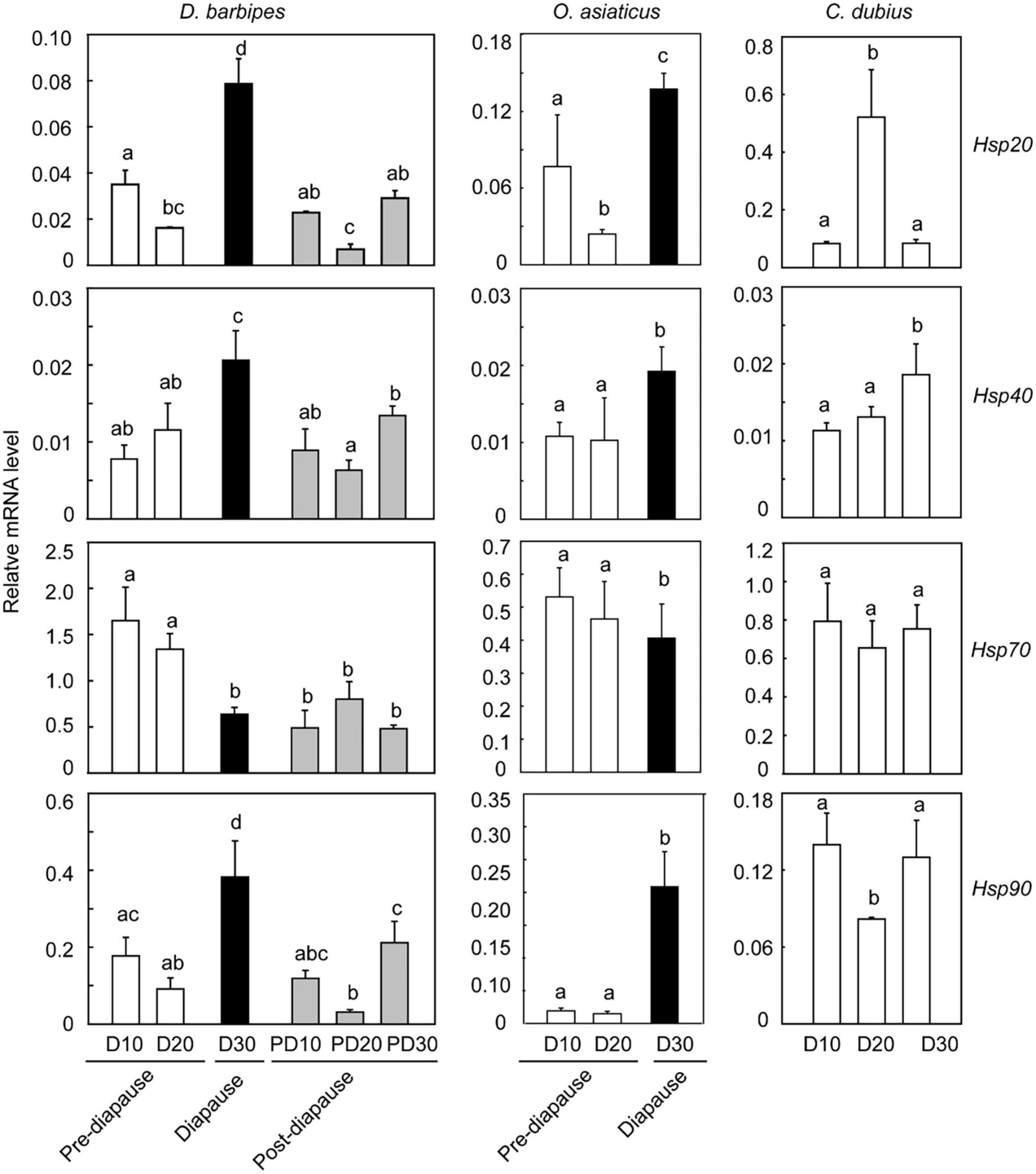
Figure 4. Association of the expression of four heat shock protein (Hsp) genes with embryonic development and diapause state in the three grasshopper species. The expression of four Hsp genes, i.e., Hsp20.6, Hsp40, Hsp70, and Hsp90, was measured in eggs at the six developmental points of time, as indicated in the X-axis. D10, D20, and D30 denote that newly deposited eggs were incubated at 30 ± 1°C for 10, 20, and 30 days, respectively. PD10, PD20, and PD30 indicate that D30 eggs were first cultured at 8°C for 60 days to terminate diapause, and then incubated at 30 ± 1°C for another 10, 20, and 30 days, respectively. The mRNA level was quantified by real-time PCR. The relative mRNA level was the mRNA level of Hsp genes normalized against that of the internal control gene, rp49. Each treatment has at least three biological replicates of eight eggs. Different letters above the columns mean significant difference at P < 0.05 via Turkey HSD test. The dark shading indicates diapaused eggs, and the gray shading indicates post-diapause eggs. The error bars represent 1 SE.
In the middle species, the four Hsp genes represented a diapause-associated expression pattern, which was similar to that of the early species. The expression levels of Hsp20.6, Hsp40, and Hsp90 significantly increased when the embryo entered into diapausing stage (t-test, P < 0.001) (Figure 4). The expression of Hsp70 showed no significant change (t-test, P > 0.05, Figure 4). By contrast, the embryonic expression of Hsp40, Hsp70, and Hsp90 in pre-diapause state remained unchanged (t-test, P > 0.05). The expression of Hsp20.6 significantly reduced at day 20 (t-test, P = 0.002).
In the late species, Hsp20.6 exhibited the highest embryonic expression at day 20, and Hsp90 presented the lowest expression at this stage. Hsp40 was significantly upregulated at day 30 (t-test, P = 0.012). The expression of Hsp70 remained unchanged in the three developmental stages (t-test, P > 0.05, Figure 4).
In summary, the three Hsps (Hsp20.6, Hsp40, and Hsp90) exhibited the same pattern of gene expression in response to diapause progression; regardless of different diapausing strategies utilized by the three species. These Hsps prominently upregulated their expression in diapausing embryos. Such significant upregulation of Hsp genes is independent of embryonic development.
Discussion
Ecological Significance of Phenological Asynchrony
Both herbivores and plants in the Inner Mongolia steppe exhibit seasonal timing in their activities because of the clear seasonality of their environment. The Inner Mongolia steppe of China is part of the Eurasian grassland and located in south of the Mongolian Plateau. The monthly minimum air temperature is over 0°C only in June, July, and August, and the mean daily temperature at 5 cm below ground increases above 0°C from April to October. The frost-free period lasts for about 110 days from early May to the end of August (Figure 1). Furthermore, the grasshopper species generally exhibit a threshold temperature for development above 10°C (Hao and Kang, 2004a,c). Therefore, the period within a year (∼5 months) when climates are favorable for the three species to grow and reproduce successfully is limited. Any growth or reproduction occurring outside this window could lead to severe fitness consequences (Visser and Both, 2005). Thus, sympatric species adjust their temporal phenology to complete their life cycles and avoid competition for shared resources.
Different grasshoppers develop considerably distinct phenological oscillation. The early grasshopper species, D. barbipes, presents a low development threshold temperature at about 10°C (Hao and Kang, 2004a). The first instar nymph of the grasshopper starts emerging by the end of April. Instead, embryos of this species develop more slowly than those of the middle and later species when incubated at 30°C (Figure 2). This result implied an adaptation of the species to low-temperature condition to survive. The early species requires less effective accumulative temperature than middle and late grasshopper species; such accumulation ensures the completion of its life cycle within less than 2 months at early season of the year. The middle species, O. asiaticus, shows a high developmental threshold temperature at 13.1°C, which is consistent with the soil temperature at the season (Figure 1). The late species, C. dubius, possesses a low development threshold temperature at 11.4°C (Hao and Kang, 2004a,c). The embryos of this species develop fast at 30°C without entering into diapause so as to avoid confronting a harmful low temperature for adult survival and embryonic development in autumn in the steppe. However, the embryos of the late species only develop to approximately stage 13 before overwintering, a stage before embryo revolution. Afterward, all eggs of the late species will appear dormancy to overwinter in the soil. Thus, the eggs require a long time to finish embryonic development in the subsequent year. Consequently, the late species can prevent the competition of temporal and trophic niches with other species in the year.
The phenological divergence among the three grasshoppers could be consequential for herbivore–resource interactions, and cause a comprehensive influence on their life cycle traits and some fitness components. Trophic interactions between herbivores and plants are considered dominant synchronization factors. Resource quality and abundance can influence the strength of a trophic match/mismatch (Kerby et al., 2012). The trophic requirement may drive change in a consumer’s phenology at the same rate as that of its food phenology (Visser and Both, 2005). However, multiple species coexist and compete at the same trophic level in an ecosystem; therefore, divergence in phenology of emergence time could be an adaptive response. In the current study, the seasonality of life cycle differs so that the maximal food resources can be utilized, and interspecific competition among the three grasshopper species can be reduced (Kang et al., 2007). Nonetheless, the shift in phenology could cause life cycle tradeoff, such as in reproductive fitness and stress tolerance. In addition to trophic and temperature factors, herbivore populations can be structured by indirect competition. For example, temporal asynchrony in emergence time of different species at same trophic level could lead to apparent competition via shared natural enemies (Blitzer and Welter, 2011). In a long-term evolution, the direct competition for limited resources results in a sequence of phenological pattern of varied grasshopper species, and shapes the specific grasshopper communities in the Inner Mongolian steppe.
Association of Phenological Asynchrony With Diapause
A few theories of phenological asynchronization have been proposed and tested to relate diapause and cold hardiness; however, only few empirical studies on the mechanisms of phenological asynchrony regulation were conducted to date (Yurk and Powell, 2009; Pau et al., 2011). Our data provide strong evidence that the sympatric grasshopper species in Inner Mongolia steppe could have developed their phenological asynchrony through taking advantage of differential diapause programs. The results showed that all embryos in the early species halted their development at stage 19, at which the revolution of embryo ceased for months (Van Horn, 1966; Hao and Kang, 2004c). Embryo development resumed only after experiencing the cold winter or via chilling treatments, as shown in our study. By contrast, the non-diapause late species presented a regular embryonic development and the fastest developmental rate at 30°C among the three grasshopper species. Diapause can ensure that insects will not occur too late during the season to complete development and reproduction before the onset of winter, thereby holding individual synchronization and keeping the univoltine for early species (Denlinger, 2002). For most insects, diapause is evoked by short-day length that signals the advent of winter. However, different from most insects, D. babipes initiates diapause at early summer time, which is not a response to short-day length. This diapause strategy may prevent an additional generation, which can lead to considerable increases of population size, and present the potential to create an outbreak or avoid an unfinished generation, which can lead to population extinction.
Embryonic diapause in grasshoppers can enhance supercooling capacity and stress tolerance in anticipation of adverse environmental condition. The grasshopper eggs of all species in Inner Mongolia grassland are susceptible to freezing, and cold tolerance is associated with developmental stage and diapause states (Hao and Kang, 2004b; Zhao et al., 2006). Only the eggs in the pre-diapause and diapause states could overwinter safely. We observed that the averaged SCPs in low SCP group significantly decreased compared with that of eggs in pre-diapause and post-diapause states in the two grasshoppers. When the diapausing program was terminated, SCPs significantly increased, which probably represented a reduced cold hardiness in post-diapausing eggs. Thus, the diapausing program in the early and middle species promotes cold hardiness of the eggs and restarts embryonic development to hold egg embryos at a safe stage during overwintering.
We observed a bimodal distribution of SCPs of eggs within each of the three species. Based on the bimodal distribution, the individuals can be separated into “high” and “low” group of SCPs. Our results showed that the mean SCP of the eggs overwintering in the soil can be decreased to below −26 and −8°C in the low-SCP and high-SCP groups, respectively (Figure 3C). The similar phenomena of SCP bimodality were reported in many other insect species (Sinclair et al., 2003; Hao and Kang, 2004c; Zhao et al., 2006). The mechanism for the SCP bimodal distribution still remains unresolved. The bimodal distributions might represent a bet hedging strategy that would allow animals to survive unexpected cold (Chown and Klok, 1998). Variation analysis of averaged SCPs of individuals in natural populations of a leafminer species Liriomyza sativae indicates that the high-group SCPs varied with populations but the low-group SCPs remain unchanged (Chen et al., 2005). Hence the low-group SCPs may be characteristic of a species’ supercooling capacity. We observed that the diapaused eggs in low-SCP group presented a significant decrease of SCP in D. barbip and O. asiaticus species, whereas their eggs in high-SCP group showed no significant difference of SCP between diapause states. Our study demonstrated a potential link between diapause and cold tolerance that are commensurate with the asynchronization in phenology of the three grasshopper species.
Heat Shock Protein Expression and Diapause Regulation
Our study also revealed association of Hsp expression and diapausing regulation in the grasshopper species. The expression of Hsp20.6, Hsp40, and Hsp90 was significantly upregulated in diapausing eggs compared with that in pre-diapause and post-diapause eggs in both early and middle species. The upregulation of Hsps often occurs in response to an environmental stress (Feder and Hofmann, 1999). Nevertheless, our results indicated that the diapause-associated upregulation of Hsps is neither solely a result of developmental changes in embryo nor a transient response to stress. First, although variation in Hsp expression level exists among developmental stages, the level of the three Hsps in diapausing eggs was considerably increased by approximately twofold the averaged level in non-diapausing eggs in the early and middle grasshopper species. The high expression could persist throughout diapause. Second, no consistent up- or downregulation of the four Hsps with embryonic development was observed in the non-diapausing grasshopper, C. dubius. In early embryos, the Hsp20.6 expression increased, but Hsp90 expression decreased; additionally, the Hsp40 and Hsp70 expression remained unchanged in the middle-term of embryo development. The concurrence of diapause initiation and upregulation, and the diapause termination and down regulation of Hsp suggested that the remarkable expression changes of Hsps are elicited in response to embryonic diapause but not to other developmental signals.
The implication for an involvement of Hsps in embryonic diapause regulation is supported by several previous studies (Denlinger, 2002; King and MacRae, 2014). A similar pattern of expression changes throughout diapause was observed in Hsp23 in S. crassipalpis. Hsp23 expression is elevated at the onset of diapause, and downregulated when the diapause is terminated (Yocum et al., 1998). Hsp40 is also upregulated in diapausing insects (Tachibana et al., 2005; Rinehart et al., 2007). However, the regulatory roles of Hsps differ among species and among Hsp members. Hsp90 is downregulated in the diapause state of S. crassipalpis and not regulated in the blow fly Lucilia sericata (Tachibana et al., 2005; Rinehart et al., 2007). In the onion maggot, Delia antiqua, Hsp90 is considerably upregulated, but Hsp70 is downregulated in response to summer diapause (Chen et al., 2005). Small Hsps often accumulate during insect diapause to increase protein binding capacity. Large Hsps, such as Hsp90 and Hsp70, help refold proteins released from small Hsps when diapause is terminates. Therefore, Hsps operate in networks of their client proteins, cochaperones, or other Hsps to participate in diapause-associated protein folding and degradation (King and MacRae, 2014).
Conclusion
This study revealed a complex and cascading changes of life cycle strategies related to phenological asynchrony. Three sympatric grasshopper species exhibited pronounced asynchronization in phenology in a short duration of growing seasons in the Inner Mongolia steppe. Distinct diapause programs evolved in the three species to control embryo development and cold tolerance. The phenology of the grasshoppers could evolve through natural selection via a combination of several factors, including climate change, seasonal variation in their food resources, life cycle tradeoff, natural enemy, and anthropic disturbance. Nonetheless, chaperones, such as Hsps, could regulate the physiological changes, e.g., diapausing, and buffer the phenological changes under global changes. Taken together, this study provides a mechanistic model for elucidating the phenological synchrony of grasshoppers, and will considerably facilitate pest management and our understanding of the ecological and evolutionary responses to climate change.
Data Availability Statement
The original contributions presented in the study are included in the article/Supplementary Material, further inquiries can be directed to the corresponding author/s.
Author Contributions
BC and SH designed the study and wrote the manuscript. BC, SG, KG, and SH performed the experiments. BC, SH, and CJ analyzed the data. BC, SG, KG, CJ, and SH revised the manuscript. All authors contributed to the article and approved the submitted version.
Funding
This work was supported by the National Natural Science Foundation of China (Grant Nos. 31872304 and 31572459) and the Science and Technology Project of Hebei Education Department (Grant No. SLRC2019019).
Conflict of Interest
The authors declare that the research was conducted in the absence of any commercial or financial relationships that could be construed as a potential conflict of interest.
Publisher’s Note
All claims expressed in this article are solely those of the authors and do not necessarily represent those of their affiliated organizations, or those of the publisher, the editors and the reviewers. Any product that may be evaluated in this article, or claim that may be made by its manufacturer, is not guaranteed or endorsed by the publisher.
Acknowledgments
We thank the Inner Mongolia Ecosystem Research Station for supplying facilities under laboratory and field conditions. We gratefully acknowledge Yongxia Hu for her wonderful works with grasshopper rearing and egg collection.
Supplementary Material
The Supplementary Material for this article can be found online at: https://www.frontiersin.org/articles/10.3389/fevo.2021.743872/full#supplementary-material
References
Bai, Y., Han, X., Wu, J., Chen, Z., and Li, L. (2004). Ecosystem stability and compensatory effects in the Inner Mongolia grassland. Nature 431, 181–184. doi: 10.1038/nature02850
Bai, Y. M., Liu, L., and Gao, S. H. (2012). Study on meteorological monitoring and prediction of grassland locusts and defense countermeasures. Beijing: Meteorology Press, 34.
Bartomeus, I., Park, M. G., Gibbs, J., Danforth, B. N., Lakso, A. N., and Winfree, R. (2013). Biodiversity ensures plant-pollinator phenological synchrony against climate change. Ecol. Lett. 16, 1331–1338. doi: 10.1111/ele.12170
Blitzer, E. J., and Welter, S. C. (2011). Emergence asynchrony between herbivores leads to apparent competition in the field. Ecology 92, 2020–2026. doi: 10.1890/11-0117.1
Bradley, N. L., Leopold, A. C., Ross, J., and Huffaker, W. (1999). Phenological changes reflect climate change in Wisconsin. Proc. Natl. Acad. Sci. U. S. A. 96, 9701–9704. doi: 10.1073/pnas.96.17.9701
Chen, B., Kayukawa, T., Monteiro, A., and Ishikawa, Y. (2005). The expression of the HSP90 gene in response to winter and summer diapauses and thermal-stress in the onion maggot, Delia antiqua. Insect Mol. Biol. 14, 697–702. doi: 10.1111/j.1365-2583.2005.00602.x
Chen, B., Li, S., Ren, Q., Tong, X., Zhang, X., and Kang, L. (2015). Paternal epigenetic effects of population density on locust phase-related characteristics associated with heat-shock protein expression. Mol. Ecol. 24, 851–862. doi: 10.1111/mec.13072
Chen, B., and Wagner, A. (2012). Hsp90 is important for fecundity, longevity, and buffering of cryptic deleterious variation in wild fly populations. BMC Evol. Biol. 12:25. doi: 10.1186/1471-2148-12-25
Chown, S. L., and Klok, C. J. (1998). Interactions between desiccation resistance, host-plant contact and the thermal biology of a leaf-dwelling sub-antarctic caterpillar, Embryonopsis halticella (Lepidoptera: Yponomeutidae). J. Insect Physiol. 44, 615–628. doi: 10.1016/S0022-1910(98)00052-3
Denlinger, D. L. (2002). Regulation of diapause. Annu. Rev. Entomol. 47, 93–122. doi: 10.1146/annurev.ento.47.091201.145137
Duman, J. G. (2001). Antifreeze and ice nucleator proteins in terrestrial arthropods. Annu. Rev. Physiol. 63, 327–357. doi: 10.1146/annurev.physiol.63.1.327
Ellwood, E. R., Diez, J. M., Ibanez, I., Primack, R. B., Kobori, H., Higuchi, H., et al. (2012). Disentangling the paradox of insect phenology: are temporal trends reflecting the response to warming?. Oecologia 168, 1161–1171. doi: 10.1007/s00442-011-2160-4
Evans, E. W. (1992). Absence of inter specific competition among tall grass prairie grasshoppers during a drought. Ecology 73, 1038–1044. doi: 10.2307/1940178
Feder, M. E., and Hofmann, G. E. (1999). Heat-shock proteins, molecular chaperones, and the stress response: evolutionary and ecological physiology. Annu. Rev. Physiol. 61, 243–282. doi: 10.1146/annurev.physiol.61.1.243
Forrest, J., and Miller-Rushing, A. J. (2010). Toward a synthetic understanding of the role of phenology in ecology and evolution. Philos. Trans. R. Soc. Lond. B Biol. Sci. 365, 3101–3112. doi: 10.1098/rstb.2010.0145
Hao, S. G., and Kang, L. (2004a). Effects of temperature on the post-diapause embryonic development and the hatching time in three grasshopper species (Orth., Acrididae). J Appl Entomol 128, 95–101. doi: 10.1046/j.1439-0418.2003.00810.x
Hao, S.-G., and Kang, L. (2004c). Postdiapause Development and Hatching Rate of Three Grasshopper Species (Orthoptera: Acrididae) in Inner Mongolia. Environ. Entomol. 33, 1528–1534. doi: 10.1603/0046-225X-33.6.1528
Hao, S. G., and Kang, L. (2004b). Supercooling capacity and cold hardiness of the eggs of the grasshopper Chorthippus fallax (Orthoptera: Acrididae). Eur. J. Entomol. 101, 231–236. doi: 10.14411/eje.2004.031
Huang, L. H., Chen, B., and Kang, L. (2007). Impact of mild temperature hardening on thermotolerance, fecundity, and Hsp gene expression in Liriomyza huidobrensis. J. Insect Physiol. 53, 1199–1205. doi: 10.1016/j.jinsphys.2007.06.011
Jing, X., and Kang, L. (2003). Geographical variation in egg cold hardiness: a study on the adaptation strategies of the migratory locust Locusta migratoria L. Ecol. Entomol. 28, 151–158. doi: 10.1046/j.1365-2311.2003.00497.x
Johansson, J., Kristensen, N. P., Nilsson, J. -Å, and Jonzén, N. (2015). The eco-evolutionary consequences of interspecific phenological asynchrony - a theoretical perspective. Oikos 124, 102–112. doi: 10.1111/oik.01909
Kang, L., Chen, B., Wei, J. N., and Liu, T. X. (2009). Roles of thermal adaptation and chemical ecology in Liriomyza distribution and control. Annu. Rev. Entomol. 54, 127–145. doi: 10.1146/annurev.ento.54.110807.090507
Kang, L., and Chen, Y. L. (1994b). Trophic niche of grasshoppers within steppe ecosystems in Inner Mongolia. Acta Ecol. Sin. 37, 178–189.
Kang, L., and Chen, Y. L. (1994a). Multidimentional analysis of resource utilization in assemblages of rangeland grasshoppers (Orthoptera: Acrididae). Ecol. Sin. 1, 264–282. doi: 10.1111/j.1744-7917.1994.tb00253.x
Kang, L., and Chen, Y. L. (1995). Dynamics of grasshopper community under different grazing intensities in Inner Mongolia. Acta Ecol. Sin. 2, 265–281. doi: 10.1111/j.1744-7917.1995.tb00048.x
Kang, L., Han, X., Zhang, Z., and Sun, O. J. (2007). Grassland ecosystems in China: review of current knowledge and research advancement. Philos. Trans. R. Soc. Lond. B Biol. Sci. 362, 997–1008. doi: 10.1098/rstb.2007.2029
Kerby, J. T., Wilmers, C. C., and Post, E. (2012). “Climate change, phenology, and the nature of consumer–resource interactions: advancing the match/mismatch hypothesis,” in Trait-mediated indirect interactions: ecological and evolutionary perspectives, eds T. Ohgushi, O. J. Schmitz, and R. D. Holt (Cambridge: Cambridge University Press), 508–525. doi: 10.1017/CBO9780511736551.032
King, A. M., and MacRae, T. H. (2014). Insect heat shock proteins during stress and diapause. Annu. Rev. Entomol. 60, 59–75. doi: 10.1146/annurev-ento-011613-162107
Korpimaki, E., Norrdahl, K., Huitu, O., and Klemola, T. (2005). Predator-induced synchrony in population oscillations of coexisting small mammal species. Proc. Biol. Sci. 272, 193–202. doi: 10.1098/rspb.2004.2860
Li, H. C., Hao, S. G., and Kang, L. (2007). Regional diferentiation of the Acridoidea ecofaunas in different vegetational zones(subzones)of Inner Mongolia region. Acta Ecol. Sin. 50, 361–375.
Li, H. C., Wang, Z., and Chen, Y. L. (1987). Food consumption and utilization by three species of acridoids (adult stage) in typical steppe. Acta Ecol. Sin. 7, 331–338.
Pau, S., Wolkovich, E. M., Cook, B. I., Davies, T. J., Kraft, N. J. B., Bolmgren, K., et al. (2011). Predicting phenology by integrating ecology, evolution and climate science. Glob. Change Biol. Bioenergy 17, 3633–3643. doi: 10.1111/j.1365-2486.2011.02515.x
Rinehart, J. P., Li, A., Yocum, G. D., Robich, R. M., Hayward, S. A., and Denlinger, D. L. (2007). Up-regulation of heat shock proteins is essential for cold survival during insect diapause. Proc. Natl. Acad. Sci. U. S. A. 104, 11130–11137. doi: 10.1073/pnas.0703538104
Sherry, R. A., Zhou, X., Gu, S., Arnone, J. A. III, Schimel, D. S., Verburg, P. S., et al. (2007). Divergence of reproductive phenology under climate warming. Proc. Natl. Acad. Sci. U. S. A. 104, 198–202. doi: 10.1073/pnas.0605642104
Sinclair, B. J., Jaco Klok, C., Scott, M. B., Terblanche, J. S., and Chown, S. L. (2003). Diurnal variation in supercooling points of three species of Collembola from Cape Hallett, Antarctica. J. Insect Physiol. 49, 1049–1061. doi: 10.1016/j.jinsphys.2003.08.002
Singer, M. C., and Parmesan, C. (2010). Phenological asynchrony between herbivorous insects and their hosts: signal of climate change or pre-existing adaptive strategy?. Philos. Trans. R. Soc. Lond. B. Biol. Sci. 365, 3161–3176. doi: 10.1098/rstb.2010.0144
Tachibana, S., Numata, H., and Goto, S. G. (2005). Gene expression of heat-shock proteins (Hsp23, Hsp70 and Hsp90) during and after larval diapause in the blow fly Lucilia sericata. J. Insect Physiol. 51, 641–647. doi: 10.1016/j.jinsphys.2004.11.012
Tauber, M. J., and Tauber, C. A. (1976). Insect seasonality: diapause maintenance, termination, and postdiapause development. Annu. Rev. Entomol. 21, 81–107. doi: 10.1146/annurev.en.21.010176.000501
van Asch, M., and Visser, M. E. (2007). Phenology of forest caterpillars and their host trees: the importance of synchrony. Annu. Rev. Entomol. 52, 37–55. doi: 10.1146/annurev.ento.52.110405.091418
Van Horn, S. N. (1966). Studies on the embryogenesis of Aulocara elliotti (Thomas) (Orthoptera, Acrididae). II. Developmental variability and the effects of maternal age and environment. J. Morphol. 120, 115–133. doi: 10.1002/jmor.1051200202
Visser, M. E., and Both, C. (2005). Shifts in phenology due to global climate change: the need for a yardstick. Proc. Biol. Sci. 272, 2561–2569. doi: 10.1098/rspb.2005.3356
Warren Ii, R. J., Bahn, V., and Bradford, M. A. (2011). Temperature cues phenological synchrony in ant-mediated seed dispersal. Glob. Change Biol. Bioenergy 17, 2444–2454. doi: 10.1111/j.1365-2486.2010.02386.x
Yocum, G. D., Joplin, K. H., and Denlinger, D. L. (1998). Upregulation of a 23 kDa small heat shock protein transcript during pupal diapause in the flesh fly, Sarcophaga, crassipalpis. Insect Biochem. Mol. Biol. 28, 677–682. doi: 10.1016/S0965-1748(98)00046-0
Yurk, B. P., and Powell, J. A. (2009). Modeling the evolution of insect phenology. Bull. Math. Biol. 71, 952–979. doi: 10.1007/s11538-008-9389-z
Keywords: grasshopper, phenological asynchrony, diapause, heat shock protein, Inner Mongolian steppe
Citation: Chen B, Jiang C, Guo S, Guo K and Hao S (2021) Phenological Asynchrony Is Associated With Diapause Program and Heat Shock Protein Expression in Three Grasshopper Species in the Inner Mongolian Steppe. Front. Ecol. Evol. 9:743872. doi: 10.3389/fevo.2021.743872
Received: 19 July 2021; Accepted: 16 September 2021;
Published: 04 October 2021.
Edited by:
Todd Jason McWhorter, University of Adelaide, AustraliaReviewed by:
Richelle Li Tanner, Chapman University, United StatesMatthew John Harke, Gloucester Marine Genomics Institute (GMGI), United States
Copyright © 2021 Chen, Jiang, Guo, Guo and Hao. This is an open-access article distributed under the terms of the Creative Commons Attribution License (CC BY). The use, distribution or reproduction in other forums is permitted, provided the original author(s) and the copyright owner(s) are credited and that the original publication in this journal is cited, in accordance with accepted academic practice. No use, distribution or reproduction is permitted which does not comply with these terms.
*Correspondence: Bing Chen, chenbing@hbu.edu.cn