- 1MTA-PE Evolutionary Ecology Research Group, University of Pannonia, Veszprém, Hungary
- 2Behavioral Ecology Research Group, Center for Natural Sciences, University of Pannonia, Veszprém, Hungary
- 3Department of Life Sciences, National Cheng Kung University, Tainan, Taiwan
- 4Jacana Ecological Education Park, Tainan, Taiwan
- 5Department of Ecology, University of Veterinary Medicine Budapest, Budapest, Hungary
- 6Milner Centre for Evolution, Department of Biology and Biochemistry, University of Bath, Bath, United Kingdom
- 7Department of Evolutionary Zoology and Human Biology, University of Debrecen, Debrecen, Hungary
In a few species, males invest more than females in parental care while the females invest in mating competition and producing multiple broods for several mates. Species in the family Jacanidae are commonly used for studying this type of breeding system (called sex-role reversal), and previous studies found discrepancies and variation between species in the expected characteristics of reversed sex roles. Yet, a better understanding of sex role differences in breeding behavior in such species is crucial for disentangling possible evolutionary mechanisms leading to this peculiar breeding system. Sex-role reversal in the pheasant-tailed jacana Hydrophasianus chirurgus has been documented long time ago. Since the very early observation of this species, however, there was no attempt to provide a comprehensive and quantitative description of their breeding. This study aims to fill these knowledge gaps by investigating the sex role differences in the breeding behavior of pheasant-tailed jacanas, by observing and monitoring a breeding population in Taiwan. We focused on three main characteristics of sex-role reversal: (1) competition between females for access to males, such as agonistic and courtship behaviors, (2) polyandrous mating, and (3) male-only care. As expected, we found that females provide most of the territory defense toward conspecifics. Males also participated in agonistic behaviors, although less frequently than females. Furthermore, contrary to what was expected, we found that males spent more time than females on courtship behavior. Polyandrous females performed mating and laying sequentially with different mates but maintained the pair bonds simultaneously with multiple males. For the first time for the species, we could estimate that the average number of mates per female (i.e., degree of polyandry) was 2.4 and that at least 81.8% of the females in the population were polyandrous. Finally, our observations corroborated that brood care is predominantly provided by males, nevertheless females were also participating to some degree in brood attendance but never in direct care (i.e., brooding). This study highlights that some aspects of polyandrous breeding might deviate from stereotyped view on sex-role reversal, and stress the importance of further within species and comparative studies in order to fully understand the mechanisms leading to sex-role reversal.
Introduction
In species providing parental care the sex difference in parental investment, as defined by Trivers (1972), varies widely. In most of the species, on average, females invest more in parental care (Clutton-Brock, 1991; Cockburn, 2006; Székely et al., 2013), which is often termed as conventional sex-roles. However, in a few species, we observe the opposite: males invest more than females in parental care while the females invest in mating competition and breeding with several mates. This is the case in species that have male-only parental care with a polyandrous type of mating system, called sex-role reversal (Trivers, 1985; Andersson, 1994). Occurring only in 1–2% of the species (Cockburn, 2006), sex-role reversal is the rarest type of breeding system in birds.
It was proposed that the sex providing care will be the one limiting the reproduction of the other sex (Emlen and Oring, 1977). In other words, the sex having higher potential reproductive rate will invest more in mating and territoriality (which is defined as a “masculine” role sometimes; Barlow, 2005). In a sex-role reversed species, thus, the females are expected to take upon territoriality and compete with other females for access to mates through aggressiveness. The dominance of females on males in sex-role reversed species is indeed confirmed by their reversed sexual size dimorphism with females being bigger than males and thus expected to be also more aggressive (Székely et al., 2007).
In term of caring for the young, precocial bird species are more flexible than altricial ones as often a single parents can take care of this task while the other parent deserts (Maynard Smith, 1977). This opportunity for uniparental care can lead to sex-role reversal in some cases, where males take most or all care of the young. It is hypothesized that males accept the care provider role because the cost of finding a new mate may be higher than the costs of investing in the current brood, for example due to the shortage of females, thus males may maximize fitness benefits by staying with their mates and provide care for existing offspring (Kokko and Jennions, 2008; Liker et al., 2013). In such populations the opposite is true for females, i.e., they have the opportunity to obtain multiple mates, thus may be selected to invest more in mating and less in care. Although classical polyandrous mating systems and sex-role reversal has been in the scope of many evolutionary studies, yet it is still uncertain from an evolutionary point of view why some lineages have adopted this breeding system (Betts and Jenni, 1991; Andersson, 2005). One reason for the lack of explanation might be the low level of knowledge and inconsistent information that we have about the ecology and behavior of species having such type of breeding.
The Jacanidea is one of the famous group of birds known for their sex-role reversal. All jacana species except the lesser jacana Microparra capensis seem to have indeed females breeding with several partners and males taking care of the brood. The polyandrous mating has been confirmed for 6 out of the 8 jacana species. The lesser jacana is monogamous (Tarboton and Fry, 1986; Hustler and Dean, 2002) and the level of information on the Madagascar jacana Actophilornis albinucha is insufficient to confirm its supposed polyandrous mating (D’Urban Jackson et al., 2019). However, within the other 6 polyandrous jacana species, the type of copulation pattern (i.e., simultaneous or sequential with different males) and degree of polyandry (i.e., average number of mates per female) vary a lot across the species (see Table 1 for detailed review). Their territoriality has been described in several species as a super-territory defended by the female which includes several sub-territories defended by her mates. In sex role reversed species, we do expect the females to provide most territorial defense, and thus to be more aggressive than males toward conspecifics. Even though this has been verified in the African jacana Actophilornis africanus (Tarboton, 1995), this idea has been challenged with the wattled jacana, the northern jacana and with the bronze winged jacana as in these species males were more likely to be the first one to respond to a conspecific intruder on the territory (Butchart et al., 1999a; Emlen and Wrege, 2004a; Lipshutz, 2017).
In jacanas, male-only care has been confirmed in six species. Yet, the complete absence of females’ participation in the care has been challenged: female wattled jacanas and northern jacanas have been observed participating at low level in parental care, even doing some brooding of the chicks, when the male is busy with incubating another clutch (Jenni and Betts, 1978; Emlen and Wrege, 2004a). The authors of the latter study even defined the females as “backup providers of chick care.” Yet in other jacanas species the male is readily described as the solely care provider (Tarboton, 1992, 1993; Mace, 2000; Butchart, 2008).
The pheasant-tailed jacana Hydrophasianus chirurgus is a classic example of polyandry and sex-role reversal since the very early studies by Hoffmann (1949, 1950), although only a few later studies investigated the breeding biology of the species. Unfortunately, details about their pair bonding is not extensively available: some of the descriptions are anecdotal (Serrao and Shekar, 1962) or based only on the observations of a single female (Thong-aree et al., 1995; Chen et al., 2008a). The fact that this species is polyandrous is well known and observed repeatedly, but the degree of polyandry is yet unknown (Table 1). Details about territoriality, female mating competition and male care are also very scarce (Thong-aree et al., 1995; Chen et al., 2008a).
This study aims at a better understanding of sex role differences in the breeding behavior of pheasant-tailed jacanas, by observing and monitoring a breeding population in Taiwan. We focus on the three parts of the breeding: (1) mate acquisition: first we study the amount of sex differences in agonistic behavior, predicting that females are involved more often than males in territorial defense. Then we investigate possible sex differences in the courtship behavior (i.e., time spent on displays), where we would expect females investing more in courtship when starting a new clutch, since the benefits of producing additional offspring should be higher for the females than for the males with already existing brood. (2) Then we investigate the pair bonding patterns and dynamics in order to calculate the degree of polyandry in this species and proportion of polyandrous females in the population. (3) Finally we study brood care (direct: brooding, and indirect: brood attendance) to understand the extent of the role division between males and females, expecting males to be the solely sex taking care of the brood, whilst females are deserting the male and the brood for creating a new clutch with a new mate.
Materials and Methods
Study Site and Population
The study was carried out from late June to early September 2019 in the Pheasant-tailed Jacana Educational Park (Guantian, Tainan City, Taiwan; 23°10′58.0”N 120°18′41.2”E; called Park henceforward). The pheasant-tailed jacana has been classified as an endangered species in Taiwan since 1989. In the last 10 years, the Tainan region has seen an increase in the population as the result of a successful conservation project (from 284 individuals in 2010 to 1024 individuals in 2019; Forestry, 2019), and ca. 80% of this population is found on water chestnut ponds in and around the Park. The Park (i.e., study site) was established in 2007 to promote and educate about this bird as well as providing breeding sites protected from farming activities. Jacanas breed in the reserve from mid-April until the end of September. However, we were able to conduct fieldwork only from mid-June until early September in 2019 due to logistic reasons, so the study period covered the second half of the breeding season. The total area of the study site was about 0.15 km2, which was divided into 25 ponds (Supplementary Figure 1). Although the whole area was studied to monitor the breeding of the birds, due to time restriction and poor visibility of birds on some of the ponds only 7 of the ponds were used for behavioral observation and individual identification (Supplementary Figure 1: P-3.1, P-3.2, P-5, P-6, P-7.1, P-7.2, and P-7.3, called hereafter “focal ponds”). The majority of the observations were conducted on pond P-5 as it was the biggest pond with a good visibility to the human observers, which allowed the identification of most resident individuals. Observations in P-3.1, P-3.2, P-5, and P-6 were made from behind wooden hides installed by the Park for visitors. Birds were habituated to the presence of human visitors and observers in these hides that made observations possible, even from short distance, without sign of disturbance. Observations in P-7.1, P-7.2, and P-7.3 were conducted from a handmade mobile bamboo hide. The hide was not moved during the observation and birds were habituated to its presence before the observations.
Individual Identification
Only two males in the focal ponds were ringed so we used detailed drawings of plumage differences (in the black head patch, white wing patch, and tail length, Supplementary Figures 2, 3) to ensure reliable identification of the individuals (Byrkjedal et al., 1997; Liker and Székely, 1999). Within-individual consistency of these patterns were checked several times during the season by drawing detailed patterns of identification and re-drawing them blindly again to see the consistency. The drawings were used for identification only in a single season, thus changes in plumage between years was not a problem (the birds molt after the breeding season and the consistency of breeding plumage between years has not been tested). Even if individuals were quite territorial, territory location in itself was not sufficient for identification as change in territory locations was observed through the season. For instance, two different males (M13 and M10, see Table 2) were observed nesting at exactly the same spot at different times of the season. The use of plumage differences allowed us to identify 12 females and 23 males. However, one female and one male left the focal ponds shortly after being identified and thus are not appearing in any of the results.
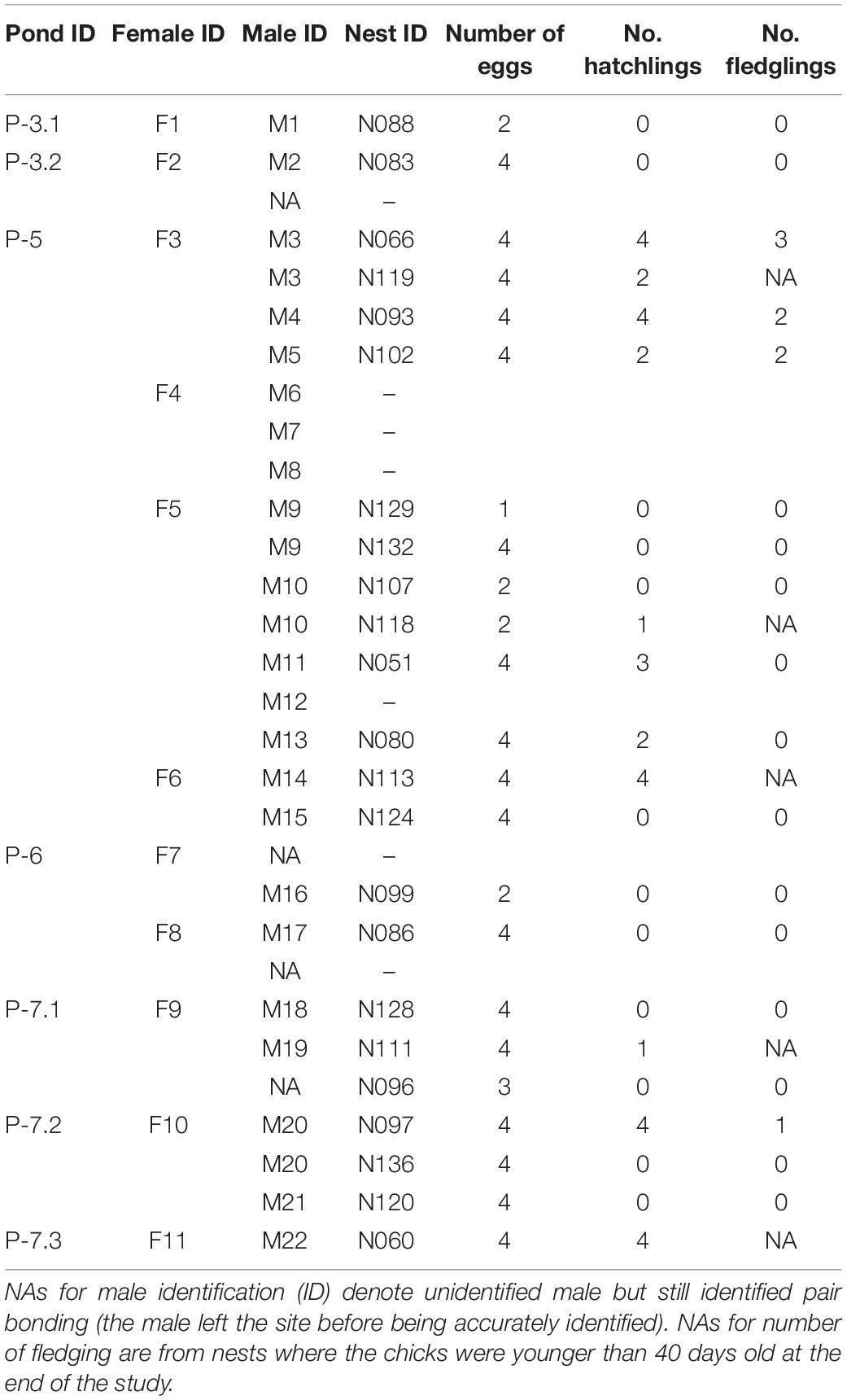
Table 2. Breeding performance of pheasant-tailed jacana: details of monitored nests and couples (female – male pairs).
Mating System
Once an individual was identified, its pair bonding, nesting attempts and breeding success were monitored by regular observations through the breeding season. Two individuals were considered to be paired when (1) they were repeatedly observed engaging in courtship behavior (i.e., various ground and aerial displays and vocalizations), copulation or nest building behavior, and (2) they were observed actively feeding next to each other without showing agonistic behavior (see below, these criteria are similar to those used in Butchart et al. (1999a)). Since the bond between the female and male usually persisted for long period (up to several weeks), we were able to infer the pair-bonds from multiple observations for most birds. Extra-pair paternity may occur in jacana (Emlen et al., 1998; Haig et al., 2003), however, in this study we use the term polyandry to describe the social mating system.
Nest and Brood Monitoring
When a nest was found, we considered the male performing egg care (incubation and shading) as the father. The female that was associated (paired) with that male was considered as the mother. Each nest found in the focal ponds was checked with a scope every day during egg laying, at least every three days during incubation and every day around the expected hatching date (about 23 days after the first egg laying). A nest was considered successful when at least one of the eggs hatched. After hatching, broods were monitored at least every three days and were considered successful when at least one chick reached the age of 40 days. The exact age of fledging has not been determined for this species (Jenni and Kirwan, 2020) but our observations suggest that after 40 days the chicks are quite independent and can fly at least for short distance. We followed a total of 23 nests and 11 broods produced by 19 couples (i.e., female – male pair) on the territories of 11 females (Table 2).
Behavioral Observations
We made three types of behavioral observations: agonistic interaction counting, pair bonding behavior observation and brood attendance behavior observation. All behavioral observation were made using a scope (Kowa TSN-601 with a 30x Kowa TSE-14WD eyepiece magnification), behind wooden fence hide or mobile hide (see above) and at least 30 min after arrival to the site for avoiding recording any possible disturbance consequences from the observer’s arrival. Agonistic interaction counting was done only in P-5 (see below), pair bonding behavior observations were done in P-3.1, P-3.2, P-5, P-6, P-7.1, P-7.2, and P-7.3 and brood attendance behavior observation were done in P-5, P-7.1, and P-7.2 as the other focused pond did not have brood. All observations were made by one observer (NF) to avoid observer bias. Only identified individuals were targeted for behavioral observation.
During behavioral observation we divided the birds’ behaviors into nine categories [some of these postures are also described for the African jacana in Tarboton (1992) and Bonkewitzz (1997)]: (1) agonistic behaviors: threat displays including ‘upright threat display,’ ‘wing spur display,’ and ground and aerial attack, (2) courtship behaviors: head down posture (often accompanied by vocalizations), mounting, and copulation, (3) nest-building behaviors: pulling and throwing vegetation toward (potential or existing) nest site and manipulating vegetation on a nest site, (4) egg care behaviors: incubation (i.e., sitting on the eggs) or egg shading (standing above the egg giving them some shade), (5) brooding behaviors: standing or sitting with chicks under the wing, (6) foraging behaviors: walking and pecking at vegetation in water, (7) maintenance behaviors: preening, scratching, stretching or bathing, (8) vigilance behaviors (i.e., alert): standing in an upright posture with the neck extended, without the tail upright and without the nape feather upright (as in agonistic behavior), (9) movement behaviors: walking, running or flying.
Agonistic interactions counting was made only in pond P-5 as it was the pond with a larger number of individuals identified allowing enough interactions with identified participants for statistical analysis. In this pond, the visibility allows to see the majority of the individuals at the same time, so individuals were observed about the same amount of time. It means that when spending time to observe some focal individuals on P-5, any agonistic behavior happening between other known individuals at the same time could also be noticed and counted (number of males on P-5: 11; number of females on P-5: 4). We counted all types of agonistic behavior as described previously. Every agonistic interaction occurrence was recorded throughout the day (even during other behavioral observation, i.e., during pair bonding and brood attendance observations) if it was between two identified individuals. We counted as one occurrence of agonistic interaction from the moment it starts until one or both individuals flew or walk to another area of the pond and the interaction stopped. We divided the agonistic interactions in four categories: Female–Female (FF), Male–Female (MF), whereas Male–Male interactions were split into between males sharing (i.e., had been paired to) the same female (MMP) and between males not sharing the same female (MMN). We did not create two categories in Male–Female interaction as all except one were observed between a female and a male that were not paired.
Pair bonding behavior observations and brood attendance behavior observations consisted of an instantaneous scan sampling of focal individual’s behavior for 30 min every 20 s and for 60 min every 30 s, respectively. When an individual was hidden (e.g., behind high grasses) or not visible in the pond we gave a “NA” (i.e., no data) to that record. We calculated the proportion of time spent on each category during the observation (excluding the NA observation). We also noted at every 5 min the approximate distance between the focal individuals. The distances between individuals were estimated using reference objects with known size, e.g., the birds themselves and the surrounding floating lotus and water chestnuts leaves. Individuals were rarely more than 100 m away from the observer. Furthermore, all distance estimations were made by the same observer allowing a good consistency in these estimations. We considered the proximity distance to about 3 m, i.e., if couples were within 3 m distance of each other they were considered in proximity of each other. In the same way if an adult was within 3 m of its brood it was considered in proximity of its brood (“brood attendance” henceforward). We used this distance because, according to our observation, it is the distance where the individuals interact between each other (i.e., vocalization or specific behavioral signal as head-down position or upright threat display) without one flying to the other.
Pair bonding behavior observations were made only on identified pairs observed in proximity of each other on the day of observation. Both male and female behavior were observed at the same time (if both were visible). A total of 44 courtship observations were made, involving 16 different males pair-bonded with 10 different females. Five couples were observed only once, the other 11 were observed at least two times with one couple observed nine times. The reason for the uneven observation time per couple was that some pairs were more often spending time together than others, furthermore, it was also dependent of the observer availability.
Brood attendance behavior observations were made only for identified individuals in the presence of their known chicks, whose number and approximate age were known from earlier observations of the family. Brood identity was assessed from proximity of the chicks to the identified parent and their corresponding age (i.e., size of the chicks). For each observation, we calculated the amount of time that the brood spent in proximity (i.e., within 3 m) of the male only, female only, or both parents, or in the absence of parents. Brood attendance behavior observation were observed for a total of 10 different broods which includes five females and eight males. One brood was observed only once at age of 39 days old (N066), while the rest of the broods were observed at least twice (see Supplementary Table 1). In 11 brood observations we did not have data for the female as the females did not appear in sight during the observation. We observed brood attendance (i.e., parent being within 3 m away from the chicks) in 8 out of 22 observations by the female and in 21 out of 22 observations by the male. In one of the observations, behaviors were recorded but neither the male not the female approached the chick close enough to be considered as brood attendance.
Data Analysis
Agonistic interactions counting was analyzed using generalized linear mixed models with a Poisson distribution including individuals’ identification as random factor. We first tested if there was a sex difference by constructing a model with the number of interactions as a response variable against the sex of the individual and the type of interaction (intrasexual interaction (FF and MM) or intersexual interaction MF) as well as their two-way interaction as explanatory variables. We then analyzed the males’ interactions separately with the number of interactions as response variable against the type of interaction (intersexual interaction: MF, Male–Male interaction sharing same female: MMP and Male–Male interaction not sharing the same female MMN) as explanatory variable.
In order to measure sex difference in time spent on various behavioral categories in the pair bonding behavior observations, we used generalized linear mixed models using a Gaussian distribution, with the proportion of time spent in different behavioral categories (after square-root transformation) as response variable and sex, time of the day (i.e., if the observation was made in the morning or the afternoon) and the time spent in the proximity (i.e., within 3 m) of the mate as well as the two-way interactions between the sex and the two other variable separately as explanatory variables. Couple identification nested in female identification were included as a random intercept. We did this analysis, with separate models, only for the following behavioral categories: (1) agonistic behaviors, (2) courtship behaviors, (3) nest-building behaviors, (4) foraging behaviors, (5) maintenance behaviors, (6) vigilance behaviors, (7) movement behaviors. Egg care behaviors and brooding behaviors were not analyzed as there were not enough occurrence during the pair bonding observations.
In the analyses of brood attendance behavior observations, first, we measured sex difference in time spent on brood attendance (in proximity of the chicks within the 60 min observation) using a generalized linear mixed model with a Gaussian distribution with the proportion of time spent in proximity of the chicks (i.e., brood attendance) as a response variable. We included the sex of the parent (here divides in three categories: male only, female only or both parents are present), the age of the offspring (divided in four categories: less than 10 days old, between 10 and 20 days old, between 20 and 30 days old and more than 30 days old), the time of the day (i.e., if the observation was made in the morning or the afternoon) as well as the two-way interactions between the sex and the two other variables separately as explanatory variables. Couple identification nested in female identification were included as a random intercept. Secondly, to measure sex difference in time spent on different behavior during brood attendance (i.e., only when in proximity of the chicks), we analyzed the proportion of time spent on each behavioral categories separately, as response variable, against the sex of the parents, the age of the offspring and the time of the day of observation as well as the two-way interactions between the sex of the parent and the time of the day as explanatory variables. Interaction between the sex and the age of the offspring could not be included in the models as there was not enough data in each category to be analyzed. We used couple identification nested in female identification as random effects. For this part we focused only on four behavioral categories: (1) agonistic behaviors, (2) foraging behaviors, (3) maintenance behaviors, (4) vigilance behaviors. Courtship behaviors, nest-building behaviors, egg care behaviors, and movement behaviors, were not analyzed here as their low occurrence during brood attendance could not allow it. Sex difference was not analyzed in brooding behavior as it was exclusively performed by the males. Brooding was observed rarely thus we had not enough data to analyze any time or age of the offspring effect.
In all cases we performed stepwise backward model selection procedures starting from the full model. Fixed effects in the models fitted with the maximum likelihood (ML) were tested by comparing a model with and without the fixed effect using likelihood ratio tests (LRTs) against a chi-square distribution (χ2). Non-significant fixed effects (P > 0.05) were removed one by one from the model starting with the least significant. The final model was fitted with restricted maximum likelihood (REML) to obtain the estimates for the fixed effects (Zuur et al., 2009). All statistics were performed in R version 3.5.0 (R Development Core Team, 2018), using the nlme package (Pinheiro et al., 2013) and lme4 package (Bates et al., 2015). Shapiro–Wilk tests were used to analyze normality and Bartlett tests to analyze homogeneity of variances. Tukey method was used for post hoc analyses, using emmeans and emtrends from the emmeans R package (Lenth, 2019).
Results
Agonistic Interactions
Agonistic interactions were observed between 15 of the 17 identified individuals that were resident on pond P-5: 4 females and 11 males. Males were significantly less frequently involved in agonistic interaction compared to females [estimate ± SE (male) = –1.84 ± 0.51, χ2 = 9.64, P = 0.002, Figure 1]. Both males and females were involved in more intra-sexual interactions than in inter-sexual interactions [estimate ± SE (intra-sexual) = 0.86 ± 0.17, χ2 = 27.10, P < 0.001, Figure 1]. The interaction between sex and type of interaction (intra- or inter- sexual) was not significant (χ2 = 2.94, P = 0.09). When males were analyzed separately, they tended to be more aggressive toward males pair-bonded with another females (MMN) than toward males pair-bonded to the same female (MMP), and they were significantly more aggressive toward males pair-bonded with a different female (MMN) than toward females (MF) (interaction type: χ2 = 10.92, P = 0.004; post hoc test: MF-MMN: –0.78 ± 0.25, P = 0.006; MF-MMP: –0.24 ± 0.28, P = 0.66; MMN-MMP: 0.54 ± 0.23, P = 0.05; Figure 1). All except one male-female interactions were between male and female involved in different couples.
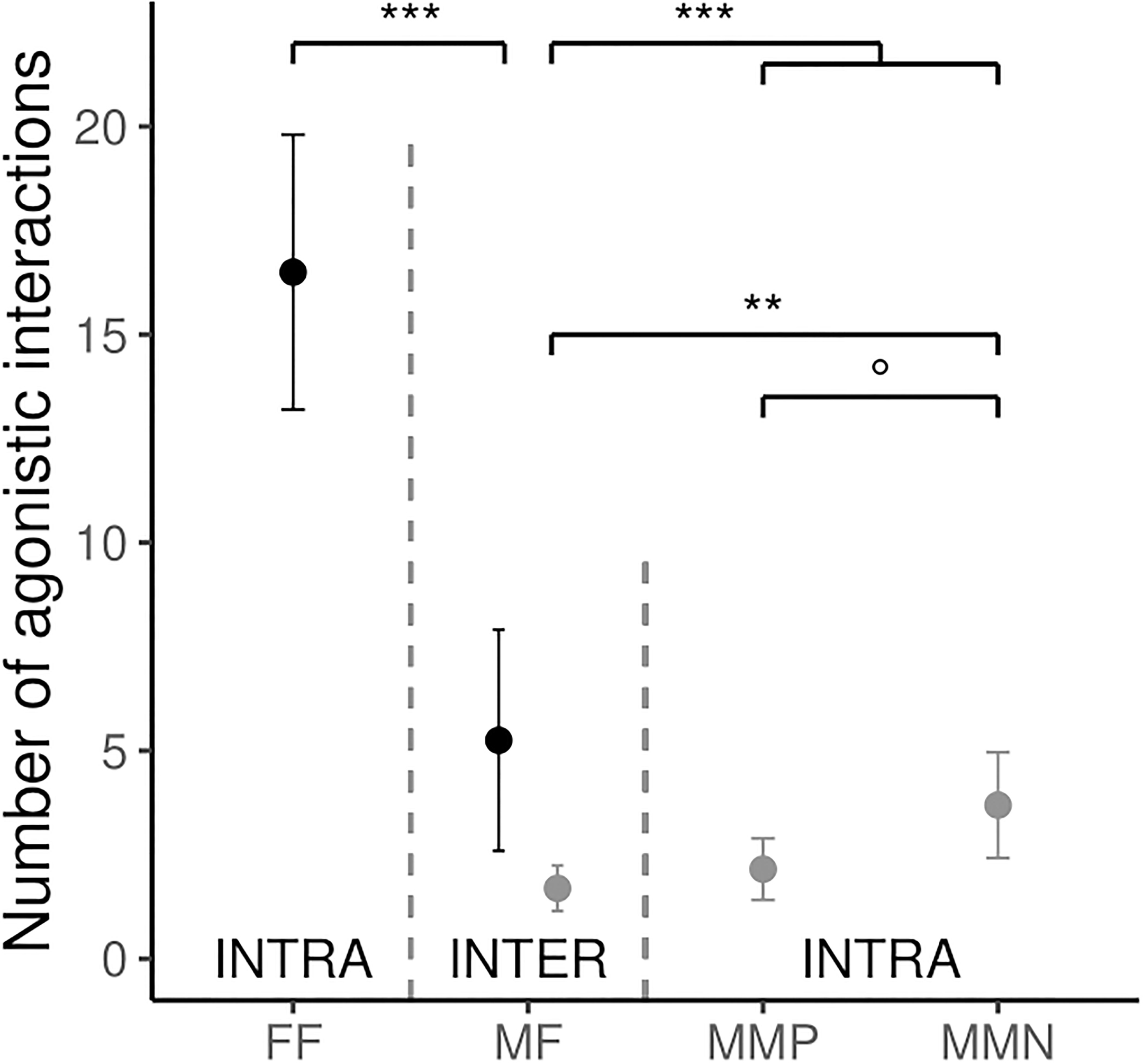
Figure 1. Average number of agonistic interactions for female (in black) and male (in gray) jacanas for intra-sexual (INTRA) and inter-sexual (INTER) interactions. FF, Female–Female; MF, Male–Female; MMP, Male–Male from same Female; MMN, Male–Male from different Female. °P = 0.06, **P < 0.01, ***P < 0.001. Females: N = 4; Males: N = 11. Error bars denote standard errors.
Pair Bonding Behaviors
During pair bonding behavior observations, males spent significantly more time on courtship behaviors than females (χ2 = 25.41, P < 0.001, Table 3 and Supplementary Figure 4A). They also spent more time moving around (movement behaviors) than females (χ2 = 5.46, P = 0.02, Supplementary Figure 4A). Analysis showed a significant interaction between sex and proximity on maintenance behaviors: the more time the male and female spend together during the observation the more time the females spent on maintenance while it was the opposite for the males, i.e., the less the males spent time on maintenance (post hoc analysis of the interaction: slope estimation for females 0.15 ± 0.13, slope estimation for males –0.24 ± 0.13, χ2 = 4.63, P = 0.03, Table 3). We found a significant effect of the interaction between sex and time of the days on the time spent on nest building behaviors (χ2 = 6.46, P = 0.01). Post hoc analysis shows that males spend significantly more time on nest building than females during the afternoon only (post hoc analysis: female – male difference during afternoon observations t69 = –3.29, P = 0.01, Table 3 and Supplementary Figure 4A). There was no difference in time spent on foraging, vigilance and agonistic behavior between males and females.
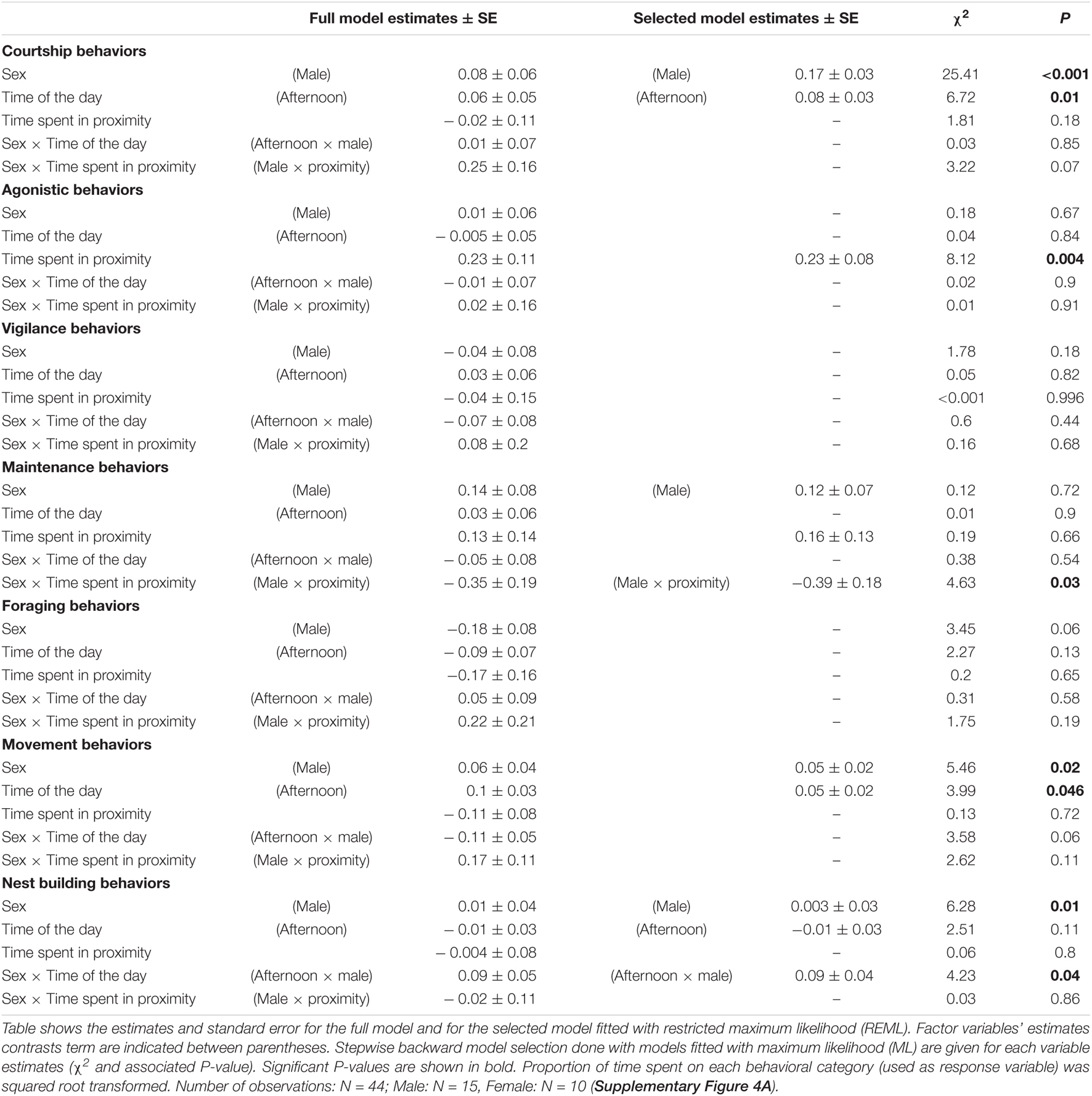
Table 3. Sex difference in amount of time spent on specific group of behaviors during pair bonding behaviors observation: courtship, agonistic behavior, vigilance, maintenance, foraging, movements, and nest building (see methods for details of these behavioral categories).
Courtship behavior and movements behavior significantly happened more in the afternoon (Courtship: χ2 = 6.72, P = 0.01; Movement: χ2 = 3.99, P = 0.046 Table 3 and Supplementary Figure 3A). The more time males and females spent together, the more time they both spent on agonistic behavior (χ2 = 8.12, P = 0.004, Table 3).
We observed a total of 22 copulations for seven females and nine males. On pond P-5, we observed 17 copulations for three females and five males (details are given in Figure 2). Copulations were observed exclusively during the afternoon. Copulations were observed from 4 to 3 days before the first egg laying and the day before each egg laying, except for one couple (F3-M3) where 2 copulations the same day were observed 7 days before the egg laying. However, we cannot exclude the possibility that the female had a nest attempt, but the nest was not found. We never observed more than 2 copulations per couple per day.
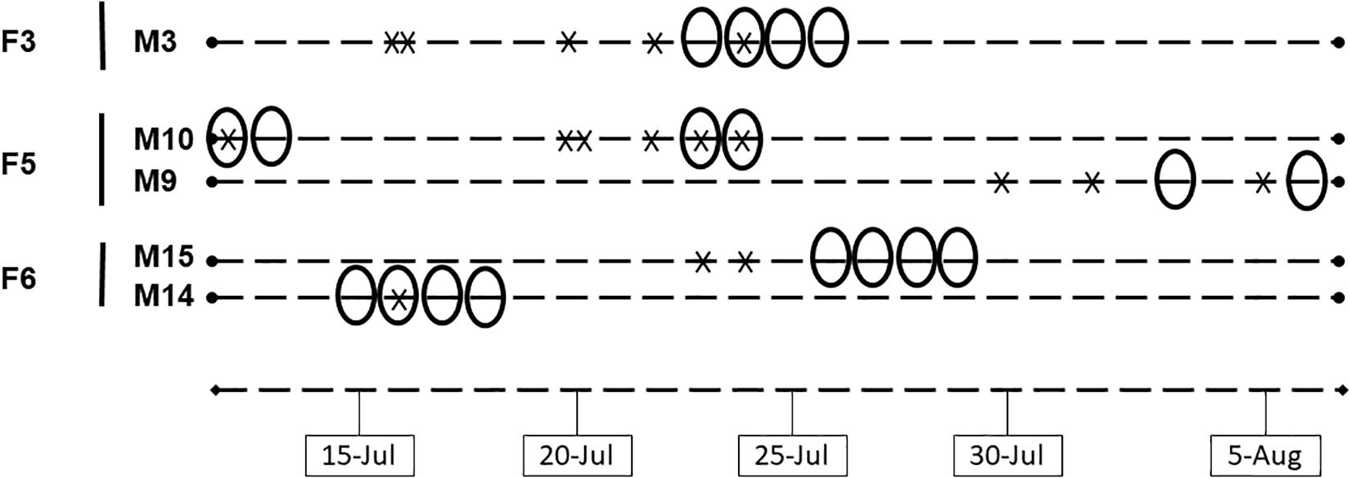
Figure 2. Observed copulations (cross) in relation to egg laying (ovals) for five different couples (three females) during a 26 days period (each dash represent a day). Copulations were not monitored every day.
Mating System
Out of the 11 focal females, 9 females paired with more than one male (81.8%, Table 2 and Figure 3). Out of the 18 males observed only one was seen changing mate by pair bonding with two different females: M6, who was seen pair bonding with F4 several times without successfully nesting but as soon as the female F4 left the breeding site permanently (i.e., was not seen anymore), the female F3 was seen actively performing displaying behavior toward M6. No nesting attempts were seen from either the couple F4-M6 or F3-M6. Females had 1–5 different males with an average of 2.4 males per females.
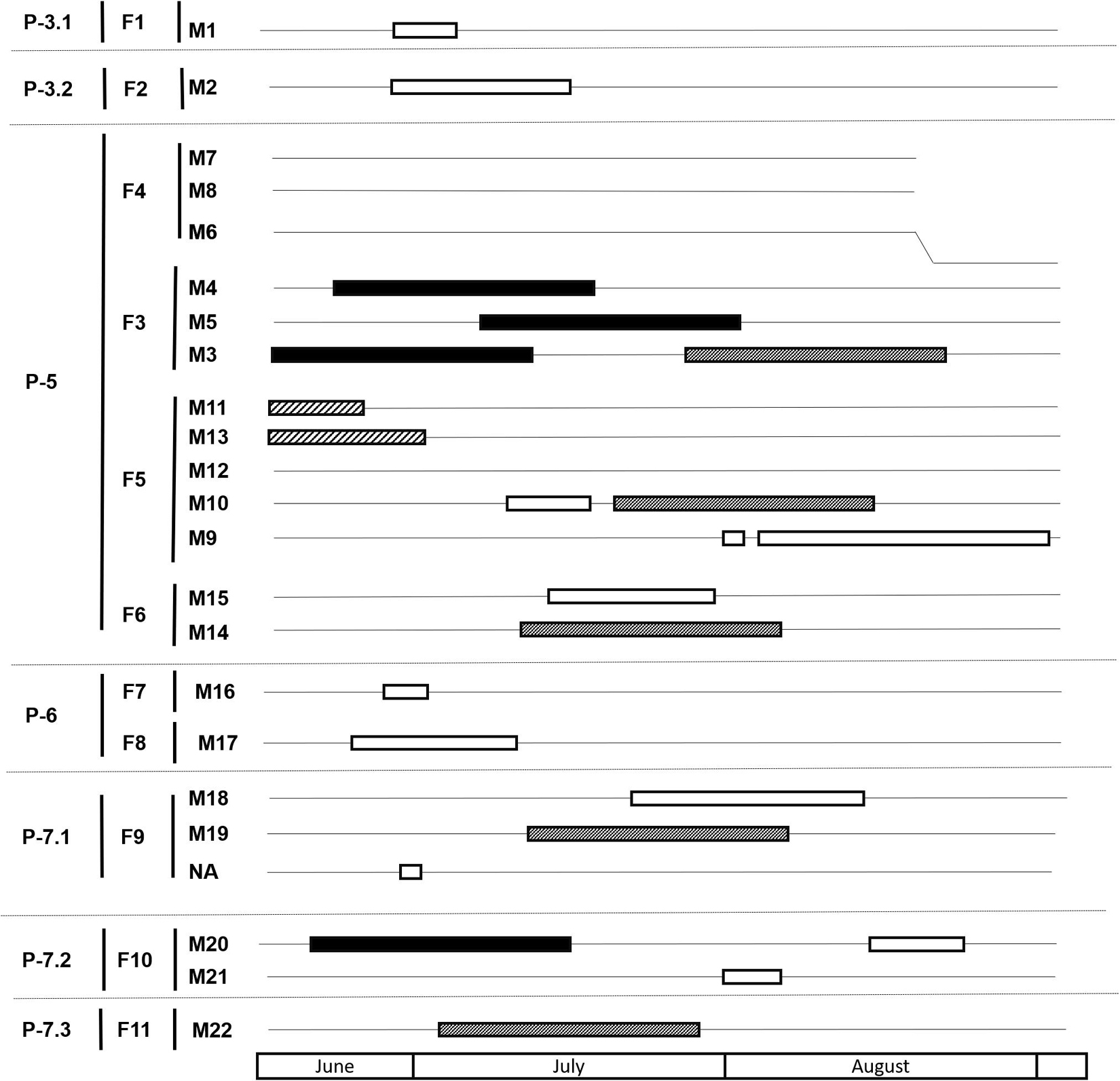
Figure 3. Dynamics of pair bonding and breeding by females (F) and males (M) observed. Horizontal lines represent pair bonding. Each rectangle represents the incubation period for a clutch (performed by the male). White filled rectangles represent clutches that did not hatch. Bold striped filled rectangles represent clutches that hatched but the chicks did not survive until fledging, whereas black filled rectangles represent successful clutches (hatched and fledged at least one chick). Light striped rectangles represent clutches that hatched but chicks did not reach the age of 40 days before the end of the fieldwork, so the success of the brood is unknown. Female F4 formed pair-bonds with three different males but did not make any nest attempt during the studied period, and one of her males (M6) subsequently established a pair-bond with F3. NA denote unidentified male that left before being able to identify him. Details are also given in Table 2.
Brood Attendance Behaviors
On average, males performed brood guarding (i.e., stayed in proximity of the chicks) during 54.2 ± 6.9% of the observation time whereas females stayed in proximity during 5.7 ± 2.2% of the time. Mixed models analysis showed that male-only brood attendance (i.e., in proximity of the chicks without the female around) was significantly more frequent than female-only brood attendance or both parents brood attendance (χ2 = 70.30, P < 0.001; post hoc test: Male-only – Female-only: t56 = –9.41, P < 0.001, Male-only – Both parents: t56 = –8.86, P < 0.001, Female-only – Both parents: t56 = 0.55, P = 0.84; Figure 4 and Table 4). Time spent on brood attendance was significantly higher during the first 10 days of the offspring age compared to when the offspring are more than 20 days old (χ2 = 10.48, P = 0.01; post hoc test, age 0–9 – 20–29: t56 = 2.94, P = 0.02; Figure 4 and Table 4). There was no significant difference in time spent on brood attendance according to the time of observation (morning or afternoon) (Table 4). Male and female did not differ in the time spent on brood attendance according to the time of the day nor the age of the offspring (i.e., there were no significant sex × time of the day, and sex × age of offspring interactions, respectively; Table 4).
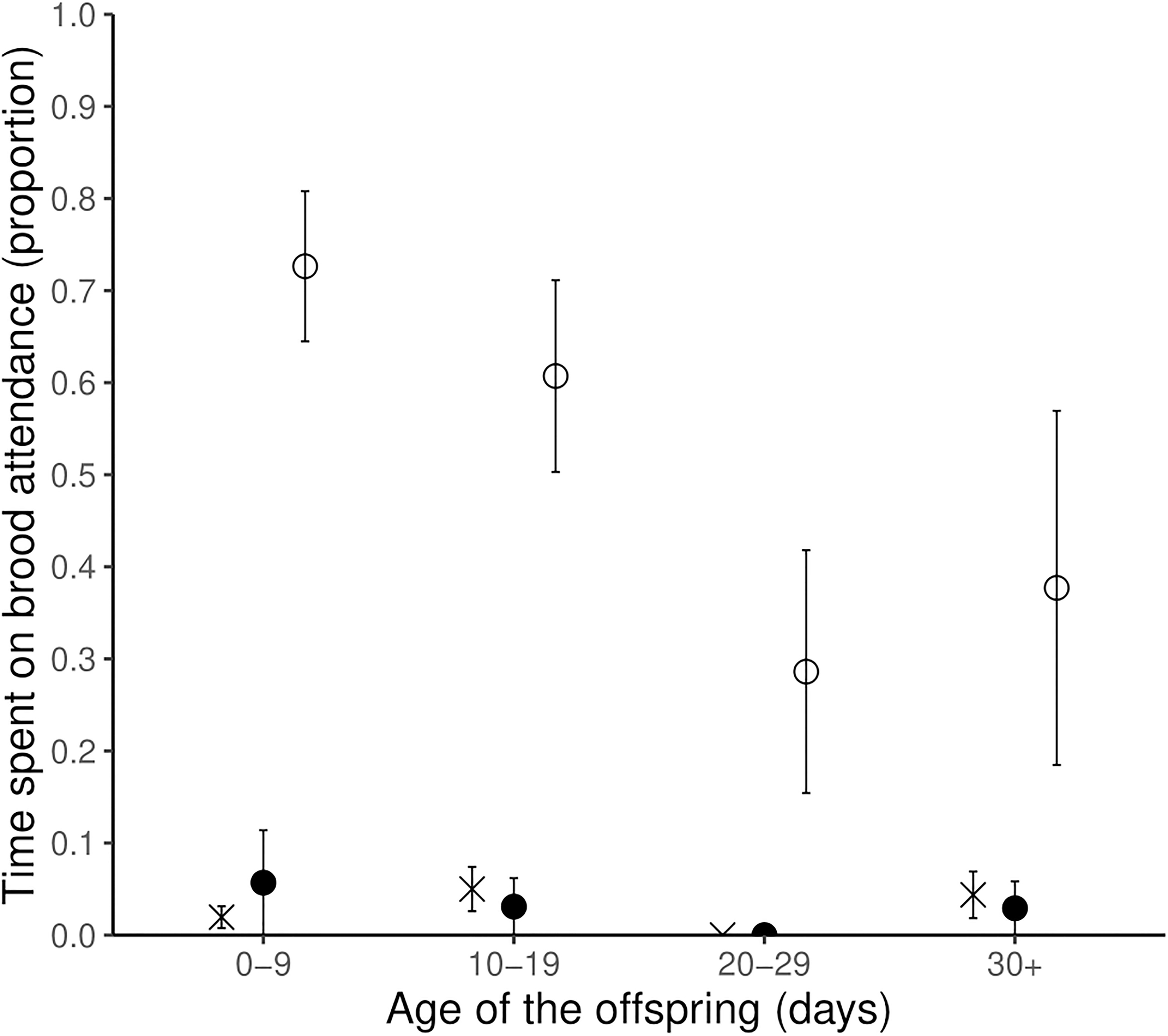
Figure 4. Proportion of time spent on brood attendance (i.e., within 3 m from the chicks) during brood care observation by the male alone (empty circle), female alone (full circle), and both parents (cross). Mean ± SE. Sample size: number of observations: N = 23, age 0–9: N = 6, age 10–19: N = 7, Age 20–29: N = 6, Age 30+: N = 4. Error bars denote standard errors.
Brooding behavior was done exclusively by males (Supplementary Figure 5). The behaviors performed during brood attendance were unrelated to the age of chicks, the sex of the parent nor the time of the day (i.e., morning or afternoon) for vigilance, maintenance and foraging behaviors (Table 4), however, males performed significantly more agonistic behaviors during brood attendance than females (Table 4).
Breeding Success
Out of 23 clutches produced by these couples (1–6 clutches per female and 1–2 clutches per male), 11 clutches (47.8%) hatched successfully, the other clutches failed. Out of 11 broods, we know the fate of six broods: at least four of them successfully fledged at least one chick, so that a clutch has approximately 18% chance of producing a fledged chick (four successful brood out of 18 clutches with known fate). Reason of clutch and brood failure was difficult to determine as it was not directly observed. We suspect that predation by large fishes (which are abundant in the ponds), water snakes and black shouldered kites were the main reasons, and flooding after heavy rain may also has contributed to nest losses.
Discussion
This study highlights several major characteristics of the breeding behavior of the sex-role reversed pheasant-tailed jacanas: (1) We did show as expected that the females participate more than the males in territory defense, but we found that males were also substantially involved in this behavior. (2) Contrary to what is expected males were performing courtship behaviors significantly more often than females. (3) Females were involved in some brood attendance however never performed any direct brood care, for example chick brooding. Finally our study based on observations of the largest number of identified individuals to date provides new data on the frequency and dynamics of polyandrous mating of the pheasant-tailed jacana. We show that this species has a simultaneous pair bonding system with a strict sequential copulation pattern. Although some aspects of breeding behavior of the pheasant-tailed jacana have been investigated by previous studies (e.g., Hoffmann, 1950; Thong-aree et al., 1995; Chen et al., 2008a) our study provides to date the most comprehensive assessment of reproductive roles of the sexes in the species, including sex differences in agonistic behavior, courtship, and parental care. Below we discuss each of these results in detail, and explain their importance within the broader frame of the evolution of sex-role reversal.
Competition for Access to Mates: Agonistic Behaviors
Our study shows that in this species not only females are involved in agonistic behavior toward conspecifics. Even though we indeed see that females were more often involved than males in this kind of interaction, males also showed significant number of agonistic interactions against conspecifics suggesting that both sexes are actively involved in territoriality. This is an interesting finding because in sex-role reversed species it is often thought that only females are territorial (Jenni, 1974; Andersson, 2005). Interestingly, we observed only one agonistic interaction between a female and one of its mates, otherwise all male-female agonistic interaction were between males and females involved in different pairs, which corroborates again that males participate in territorial defense. In their paper Chen et al. (2008b) also reported that male pheasant-tailed jacanas performed some aggressive behaviors, but this study did not differentiate between conspecific and heterospecific interactions, neither if the conspecific individual included in the interaction was female or male. Involvement of the males in the territorial and thus resources defense is thought to be yet another benefits that the female could gain by mating with multiple males (Fedorka and Mousseau, 2002; Slatyer et al., 2012), because the more mates the female would have the larger territory and more resource could be defended. In the facultative social polyandrous mammals siamang, polyandrous groups have access to larger territory with a higher food density (Lappan et al., 2017).
Furthermore, we found that male-male fights were usually between males not sharing the same female’s territory. This latter observation could be explained by several mechanisms. First, once the pair bonding is made with a female, the males of the same female would compete for the female’s reproductive investment through other means than fighting (see below). Furthermore, since the males pair bonded with the same female live close to each other, there might be a dominance hierarchy among them based on initial interactions. This might reduce the frequency of fights within the social group and reduce the costs of competition which could ultimately lead to a decline of reproductive success (McDonald et al., 2017). In addition, it has been shown that male cooperation in reproduction could lead to several direct and indirect benefits (Díaz-Muñoz et al., 2014). Finally, the presence of an external male on the territory could not only represent an intruder but also a new potential co-mate and thus competitor for access to the female and resources of the territory, as well as a higher number of nests on the territory attracting more predators. We could then imagine that according to the quality of the habitats of the female, male could tolerate up to only a certain amount of co-mates (Lappan et al., 2017). More studies would be needed in order to measure the frequency and temporal dynamics of such social interactions within the polyandrous groups.
Both males and females increased their aggressiveness (toward other individuals) with the time spent together, the more time they spent together the more they were aggressive. This could be a result of an increase of synchronization in territoriality when they defend together against some intruders. Indeed, we observed several cases when more than two individuals were engaging in aggressive interaction at the same place, perhaps on territory boundaries (NF and AL, personal observations), which may the results of such joint defense by the couples.
Competition for Access to Mates: Courtship Behaviors
Interestingly, males were spending more time in courtship behaviors than females which goes against what we expected for a sex-role reversed species. In polyandry, we would expect the females to invest more into producing a new clutch than the male, since females could maximize their breeding success by obtaining multiple mates whereas the males’ success is determined in a large part by the successful rising of the current offspring. One potential explanation for this result is that we did not observe courtship at the beginning of the breeding season when competition between females for mates should be much intensive. Contrary to this explanation, in a previous study Chen et al. (2008b) observed that the time spent by both males and females on breeding behavior increased through the breeding season, which included courtship behavior (although they did not separate it from other activities such as parental care). Alternatively, the courtship behavior we observed may not only serve to establish new or re-establish earlier pair bonds, but may also signal the readiness of males to start a new breeding. Since both nest loss and brood loss were frequent in the study population (see section “Results”), males may compete with each other for the egg laying potential of the female, especially when there are several resident males on the female’s territory (as in the case of several females in our study). This high within-male competition for female’s attention was observed in the bronze-winged jacana (Butchart et al., 1999b). In such situation the male may benefit from intensively signaling his capacity for a new clutch as well as its quality (Kotiaho, 2002; Pariser et al., 2010), because otherwise he may wait for long periods until female finishes laying for other males. Male–male competition, here shown through courtship can have a strong impact on sexual selection as it has been shown in mammals (e.g., Lührs and Kappeler, 2014), insects (e.g., Russell et al., 2018), and other birds (McDonald et al., 2017). To get a better idea of the relevance of the above explanations, further data on the occurrence of courtship are needed, especially from the earlier part of the breeding season and with precise information on their timing relative to the initiation of the new clutches.
During the courtship behavior observations we found that females spent more time in maintenance behavior than males, which could show that preening might also be used as part of the courtship behaviors. In the same way, we observed the males doing more nest building behaviors than the female, especially in the afternoon, when copulation happens. Nest building by the males may also be used as part of the courtship behaviors, as it has been suggested in other jacana species (e.g., Bonkewitzz, 1997).
Polyandrous Mating
Our study confirmed that most female pheasant-tailed jacanas are polyandrous, although with a great variance in the number of mates (ranging from 1 to 5). The degree of polyandry (mean number of mates per females) was 2.4 and at least 81.8% of the females bred polyandrously. This put the characteristics of the mating system of the pheasant-tailed jacana (in term of degree and proportion of polyandry) close to the northern jacana. Which, interestingly is one of the closest jacana species to the pheasant-tailed jacana on the phylogenetic tree (Whittingham et al., 2006; D’Urban Jackson et al., 2019).
Pheasant-tailed jacanas were originally classified by Oring (1986) as a classical simultaneous polyandrous species. In more recent papers, the term sequential polyandrous is sometimes used for the species (Thong-aree et al., 1995; Chen et al., 2008b). In a sense, our study inferred the sequential nature of breeding with several males by the females: females produced clutches in a sequential order for different males, having a turnover of about 7 days between the end of laying a clutch for one male and the starting of a new clutch with another male. On the other hand, several females maintained pair bonds with multiple males simultaneously. For example, female F3 had three males simultaneously incubating separate clutches on her territory (Figure 3). Our observation suggest that the females regularly visit the territory and nest sites of their mates after laying the clutch, both during incubation and brood care, even during periods when the female is engaged in courtship with another male (NF and AL, personal observations). This suggests that the pair bond is maintained for a longer period between the female and the males resident on her territory, which is also indicated by the laying of new clutches for the same males after clutch or brood losses (see Figure 3 for examples). It has been observed in northern China that males become receptive to female after hatching of a clutch much sooner than in other species, with some copulation observed with males having only 2 weeks old brood (Jenni and Kirwan, 2020). This is something that we also witnessed in this population. These observations highlights the particularity of the mating system of pheasant-tailed jacanas as it is different from a strictly sequential polyandry, since in this latter system females usually desert her mate and offspring before initiate a new pair bond with another male (as in the Kentish plover or in the dotterel, Owens et al., 1994; Székely and Williams, 1995). It is nevertheless also different from classical polyandry, which refers to simultaneous pair bonding associated with simultaneous mating. In this paper, we try to clearly differentiate the terms “sequential polyandry” and “sequential polyandrous copulation” which in our opinion describe different level of mating system. We propose that the mating system of this species is closer to the system of other jacanas classified as having classical or simultaneous polyandry, as previously stated by Oring (1986), with however a sequential polyandrous copulation pattern which is different from other jacanas such as bronze-winged jacana (Butchart et al., 1999b). Maintenance of such pair bonding with the different mates through the breeding season could lower the cost of female competition for access to males. Meanwhile, the sequential polyandrous copulation could lower the probability for the male to raise unrelated chick as it was found in the comb-crested jacana (Haig et al., 2003) where pairs were genetically monogamous with only 2.8% of the chicks were unrelated to the father compared to 17% in the wattled jacana (Emlen et al., 1998).
Female birds are able to store sperms for several days, thus sperm competition between the current mate and the previous one of female jacanas is expected to be strong (Dale et al., 1999). In a strong sperm competition context, we would expect a high rate of copulation in order to ensure male’s paternity. Our result shows the opposite: few copulations and mainly during egg laying. This observation goes in line with what was observed in the bronze-winged jacana (Butchart, 1999). Potential other strategies might be used by the males to reduce the chance of raising unrelated young. One strategy might be egg removal: indeed a previous paper on the pheasant-tailed jacana shows that males might remove the first egg laid in their clutch in order to reduce the possibility of cuckoldry (Chen et al., 2008a).
Male-Only Care
This study corroborated that parental care in pheasant-tailed jacanas was, as expected, essentially performed by males. This has been indeed already shown in this species (Serrao and Shekar, 1962; Chen et al., 2008b) and other polyandrous Jacanidae species (Jenni and Collier, 1972; Emlen and Wrege, 2004a; Butchart, 2008). Incubation was solely carried out by males and, contrary to what has been observed in wattled jacana (Jenni and Collier, 1972), female pheasant-tailed jacanas do not seem to perform any chick brooding, even when the males are busy with the incubation of a new clutch. Since the pheasant-tailed jacana population presents a strong male biased sex-ratio in the population, females have a more re-mating opportunity than males and are thus expected to invest more in re-mating than in parental care (Liker et al., 2013; Székely et al., 2013). This strong difference of investment could lead to the rigid sex role that we observe. The lack of flexibility by the female in her investment in parental care was observed in a mate removal experiment with polyandrous black coucal, Goymann (2019) showed that the female would not compensate the male absence in brood care.
However, we did observe some participation by the female in brood attendance, with and without the male around. Both males and females lower their time attending the brood with the age of the offspring. In the wattled jacana (Emlen and Wrege, 2004a) the female was observed attending especially young chicks (under 4 weeks). In their paper, Emlen and Wrege (2004a) described the females wattled jacana as “backup providers of chick care.” In their study they found indeed that they observed females giving care in only 3% of the observation and each case were exceptional as they occurred in only two types of context: the male was predated, or the male was attending a clutch. This is not what was observed here as we still observed some females attending the brood outside these two contexts, for example when the male was foraging elsewhere. The variance of time spent by the female attending the brood in this study is not enough to see if this investment is related to male quality, pair bonding situation or female quality. Ultimately, the occasional proximity to the broods by the female could also be related to maintaining mating opportunity as it was suggested by several studies in other polyandrous birds (Goymann et al., 2015; Zheng et al., 2021).
We found that the behaviors performed by the females when attending the brood is almost the same as the males when attending the chicks with the exception of brooding behavior and agonistic behaviors. The latter result can be explained by longer time the males spend with the brood thus they may be present more often when the brood needs defense, for example from conspecifics or from other species.
Conclusion
In conclusion, our study shows a clear sex-role reversal pattern in the pheasant-tailed jacana with females being highly polyandrous and males taking most of the care of the offspring. However, we highlight some patterns that are important to take in account: (1) males also participated in territorial defense especially toward males that were not paired with the same female and males invested more in courtship behaviors than females. This led us to conclude that male pheasant-tailed jacana might undergo strong male competition for access to female through courtship, but may also cooperate with or tolerate co-mates in order to increase mate and resource defense. (2) Females also participated in brood attendance yet to a low degree confirming that female pheasant-tailed will invest more into mating than providing care, (3) polyandry in this species involves the simultaneous maintenance of bonds with several males by a female which will sequentially copulate with them. These deviations from what is expected in a sex-role reversal species suggest that the mating system shows subtle variations even among closely related species that all exhibit classical polyandry. Our study was conducted on a relatively small number of individuals and only in the second half of the breeding season, that may limit the generality of some our conclusions (e.g., for sex differences in courtship, see above). Thus, a longer study through several years would be useful for further corroboration of our findings. Ultimately, such results on sexual difference in mating and parental investment in a sex-role reversal species can help us to understand better the evolutive mechanisms leading to this unconventional sex-roles.
Data Availability Statement
The raw data supporting the conclusions of this article will be made available by the authors, without undue reservation.
Ethics Statement
The animal study was reviewed and approved by the Forestry Bureau of Taiwan.
Author Contributions
AL, TS, AK, and NF conceived and developed the study. W-CL and Y-FL took care of the organization and administration regarding the project and the study site in Taiwan. NF collected the data with the help of Y-FL and W-CL. NF analyzed the data. NF and AL wrote the manuscript with input from TS, AK, and Y-FL. All authors contributed to the final manuscript.
Funding
The project was funded by the National Research, Development and Innovation Office of Hungary (NKFIH, grant KH130430). NF was supported by NHKFIH PD134414 grant, AK was supported by NKFIH NN125642 grant, and Y-FL was supported by the Forestry Bureau of Taiwan (108 FD-9.1-C-36 and 109 FD-9.1-C-32). TS was funded by The Royal Society (Wolfson Merit Award WM170050, APEX APX\R1\191045) and by the National Research, Development and Innovation Office of Hungary (ÉLVONAL KKP-126949, K-116310).
Conflict of Interest
The authors declare that the research was conducted in the absence of any commercial or financial relationships that could be construed as a potential conflict of interest.
The handling editor declared a past co-authorship with one of the authors TS.
Publisher’s Note
All claims expressed in this article are solely those of the authors and do not necessarily represent those of their affiliated organizations, or those of the publisher, the editors and the reviewers. Any product that may be evaluated in this article, or claim that may be made by its manufacturer, is not guaranteed or endorsed by the publisher.
Acknowledgments
We thank the Forestry Bureau of Taiwan, and the City of Tainan for permission to work on their property. We thank the Jacana Ecological and Educational Park for allowing us to work in their park and we are especially grateful to the park employee for kindly welcoming us and helping with the fieldwork handling. We also thank the ÉLVONAL shorebirds community, especially Vojtěch Kubelka for giving extremely good insights and suggestions in the development of this project methodology and fieldwork organization. Two reviewers and the associate editor are thanked for their constructive comments which improved the manuscript.
Supplementary Material
The Supplementary Material for this article can be found online at: https://www.frontiersin.org/articles/10.3389/fevo.2021.742588/full#supplementary-material
Supplementary Figure S1 | Map of the study site surveyed: The Pheasant-tailed Jacana educational park in Guantian, Tainan, Taiwan. Dark green indicates the ponds used for behavioral observation.
Supplementary Figure S2 | Pictures of different pheasant-tailed jacana individuals from the study population. Pictures (A,B) show the sexual size dimorphism between males and females (A: male M3 on the left/front and female F3 on the right/back; B: male M20 on the left and female F10 on the right). Pictures (C–F) illustrate the variation between individuals in their plumage: note the differences in the black patch on the top of the head, in the white wing patch pattern, and in tail length (C: male M3 and one of his chicks; D: female F10 from another angle; E: male M16 and his eggs; F: male M17 and his egg).
Supplementary Figure S3 | Examples of individual variability in pheasant-tailed jacana drawn from field sketches made in the study area. (A) Male M12. (B) Male M1. (C) Female F4. (D) Female F3.
Supplementary Figure S4 | Proportion of time spent on different focused categories of behaviors by the males (in black) and females (in gray) during (A) courtship observation and (B) brood attendance (i.e., in proximity of the chicks). Mean ± SE. Sample size: (A) Number of observations: N = 44; Male: N = 15, Female: N = 10 and (B) Number of observations: N = 21; Male: N = 8, Female: N = 5. *P < 0.05; ***P < 0.00. Error bars denote standard errors. Details of the results are shown in Tables 2, 3, respectively.
Supplementary Figure S5 | Proportion of time of the observation spent on brooding the offspring by the male according to the age of the offspring (less than 10 days old, between 10 and 20 days old, between 20 and 30 days old, and more than 30 days old). Each dot represents one brood care observation.
References
Andersson, M. (2005). Evolution of classical polyandry: three steps to female emancipation. Ethology 111, 1–23. doi: 10.1111/j.1439-0310.2004.01057.x
Barlow, G. W. (2005). How do we decide that a species is sex-role reversed? Q. Rev. Biol. 80, 28–35. doi: 10.1086/431022
Bates, D., Mächler, M., Bolker, B., and Walker, S. (2015). Fitting linear mixed-effects models using lme4. J. Stat. Softw. 67, 1–48. doi: 10.18637/jss.v067.i01
Betts, B. J., and Jenni, D. A. (1991). Time budgets and the adaptiveness of polyandry in Northern Jacanas. Wilson Bull. 103, 578–597. doi: 10.2307/4163086
Bonkewitzz, A. N. (1997). Behavioural and Social Organization of the African Jacana Actophilornis Africanus. Ph.D. dissertation. Pietermaritzburg: University of Natal.
Butchart, S. H. M. (1999). Sexual conflicts and copulation patterns in polyandrous bronze-winged jacanas (Metopidius indicus). Behaviour 136, 443–468.
Butchart, S. H. M. (2008). Population structure and breeding system of the sex-role reversed, polyandrous Bronze-winged jacana Metopidius indicus. IBIS 142, 93–102. doi: 10.1111/j.1474-919x.2000.tb07688.x
Butchart, S. H. M., Seddon, N., and Ekstrom, J. M. M. (1999a). Polyandry and competition for territories in bronze-winged jacanas. J. Anim. Ecol. 68, 928–939. doi: 10.1046/j.1365-2656.1999.00341.x
Butchart, S. H. M., Seddon, N., and Ekstrom, J. M. M. (1999b). Yelling for sex: harem males compete for female access in bronze-winged jacanas. Anim. Behav. 57, 637–646. doi: 10.1006/anbe.1998.0985
Byrkjedal, I., GrØnstØl, G. B., Lislevand, T., Magne Pedersen, K., Sandvik, H., and Stalheim, S. (1997). Mating systems and territory in Lapwings Vanellus vanellus. IBIS 139, 129–137. doi: 10.1111/j.1474-919x.1997.tb04512.x
Chen, T. C., Lin, Y. S., Deng, P. L., and Ding, T. S. (2008a). Male pheasant-tailed jacanas commit infanticides to avoid cuckoldry when paternity of eggs is doubtful. J. Nat. Hist. 42, 2991–3000. doi: 10.1080/00222930802389817
Chen, T. C., Lin, Y. S., and Ding, T. S. (2008b). Time budget of polyandrous Pheasant-tailed Jacana (Hydrophasianus chirurgus) during breeding season in Taiwan. Taiwania 53, 107–115.
Clutton-Brock, T. H. (1991). The Evolution of Parental Care. Princeton, NJ: Princeton University Press.
Cockburn, A. (2006). Prevalence of different modes of parental care in birds. Proc. R. Soc. B. Biol. Sci. 273, 1375–1383. doi: 10.1098/rspb.2005.3458
Dale, J., Montgomerie, R., Michaud, D., and Boag, P. (1999). Frequency and timing of extrapair fertilisation in the polyandrous red phalarope (Phalaropus fulicarius). Behav. Ecol. Sociobiol. 46, 50–56. doi: 10.1007/s002650050591
Díaz-Muñoz, S. L., DuVal, E. H., Krakauer, A. H., and Lacey, E. A. (2014). Cooperating to compete: altruism, sexual selection and causes of male reproductive cooperation. Anim. Behav. 88, 67–78. doi: 10.1016/J.ANBEHAV.2013.11.008
D’Urban Jackson, J., Zefania, S., Moehy, S., Bamford, A., Bruford, M., and Székely, T. (2019). Ecology, conservation, and phylogenetic position of the Madagascar Jacana Actophilornis albinucha. Ostrich 90, 315–326. doi: 10.2989/00306525.2019.1662508
Emlen, S. T., and Oring, L. W. (1977). Ecology, sexual selection, and the evolution of mating systems. Science 197, 215–223. doi: 10.2307/1744497
Emlen, S. T., and Wrege, P. H. (2004a). Division of labour in parental care behaviour of a sex-role-reversed shorebird, the wattled jacana. Anim. Behav. 68, 847–855. doi: 10.1016/j.anbehav.2003.08.034
Emlen, S. T., and Wrege, P. H. (2004b). Size dimorphism, intrasexual competition, and sexual selection in wattled jacana (Jacana Jacana), a sex-role-reversed shorebird in panama. Auk 121, 391–403. doi: 10.1093/auk/121.2.391
Emlen, S. T., Wrege, P. H., and Webster, M. S. (1998). Cuckoldry as a cost of polyandry in the sex-role-reversed wattled jacana, Jacana jacana. Proc. R. Soc. B. Biol. Sci. 265, 2359–2364. doi: 10.1098/rspb.1998.0584
Fedorka, K. M., and Mousseau, T. A. (2002). Material and genetic benefits of female multiple mating and polyandry. Anim. Behav. 64, 361–367. doi: 10.1006/anbe.2002.3052
Forestry, B. (2019). Looking Back on the Pheasant-Tailed Jacana’s Journey of Restoration - Heartwarming Premiere of Jacana in the Water Caltrop Field Documentary. Arlington, TX: Nature Conservation.
Goymann, W. (2019). Males paving the road to polyandry? Parental compensation in a monogamous nesting cuckoo and a classical polyandrous relative. Ethology 126, 436–444. doi: 10.1111/eth.12988
Goymann, W., Makomba, M., Urasa, F., and Schwabl, I. (2015). Social monogamy vs. polyandry: ecological factors associated with sex roles in two closely related birds within the same habitat. J. Evol. Biol. 28, 1335–1353. doi: 10.1111/jeb.12657
Haig, S. M., Mace, T. R., and Mullins, T. D. (2003). Parentage and relatedness in polyandrous comb-crested jacanas using ISSRs. J. Hered. 94, 302–309. doi: 10.1093/jhered/esg072
Hoffmann, A. (1949). Uber die brutpflege des polyandrischen Wasserfasans Hydrophasianus chirurgus. Zool. Jahrbücher. 78, 367–403.
Hoffmann, A. (1950). Zur Brutbiologie des polyandrischen Wasserfasans Hydrophasianus chirurgus scop. Ornithol. Ber. 2, 119–126.
Hustler, K., and Dean, W. R. J. (2002). Observations on the breeding biology and behaviour of the Lesser Jacana, Microparra capensis. Ostrich 73, 79–82. doi: 10.1080/00306525.2002.11446733
Jenni, A. D., and Collier, G. (1972). Polyandry in the American jacana (Jacana spinosa). Auk 89, 743–765.
Jenni, D. A. (1974). Evolution of polyandry in birds. Integr. Comp. Biol. 14, 129–144. doi: 10.1093/icb/14.1.129
Jenni, D. A., and Betts, B. J. (1978). Sex differences in nest construction, incubation, and parental behaviour in the polyandrous American jacana (Jacana spinosa). Anim. Behav. 26, 207–218. doi: 10.1016/0003-3472(78)90020-9
Jenni, D. A., and Kirwan, G. M. (2020). “Pheasant-tailed Jacana (Hydrophasianus chirurgus), version 1,” in Birds of the World, eds J. del Hoyo, A. Elliott, J. Sargatal, D. A. Christie, and E. de Juana (Ithaca, NY: Cornell Lab of Ornithology).
Kokko, H., and Jennions, M. D. (2008). Parental investment, sexual selection and sex ratios. J. Evol. Biol. 21, 919–948. doi: 10.1111/j.1420-9101.2008.01540.x
Kotiaho, J. S. (2002). Sexual selection and condition dependence of courtship display in three species of horned dung beetles. Behav. Ecol. 13, 791–799. doi: 10.1093/beheco/13.6.791
Lappan, S., Andayani, N., Kinnaird, M. F., Morini, L., Nurcahyo, A., and O’Brien, T. (2017). Social polyandry among siamangs: the role of habitat quality. Anim. Behav. 133, 145–152. doi: 10.1016/J.ANBEHAV.2017.09.017
Lenth, R. (2019). Emmeans: Estimated Marginal Means, aka Least-Squares Means. R Packag version 1.4.3.01.
Liker, A., Freckleton, R. P., and Székely, T. (2013). The evolution of sex roles in birds is related to adult sex ratio. Nat. Commun. 4:1587. doi: 10.1038/ncomms2600
Liker, A., and Székely, T. (1999). Parental behaviour in the lapwing Vanellus vanellus. IBIS 141, 608–614. doi: 10.1111/j.1474-919x.1999.tb07368.x
Lipshutz, S. E. (2017). Divergent competitive phenotypes between females of two sex-role-reversed species. Behav. Ecol. Sociobiol. 71:106. doi: 10.1007/s00265-017-2334-0
Lührs, M. L., and Kappeler, P. M. (2014). Polyandrous mating in treetops: how male competition and female choice interact to determine an unusual carnivore mating system. Behav. Ecol. Sociobiol. 68, 879–889. doi: 10.1007/S00265-014-1701-3
Mace, T. R. (2000). Time budget and pair-bond dynamics in the comb-crested jacana Irediparra gallinacea : a test of hypotheses. EMU 100, 31–41. doi: 10.1071/MU9844
Maynard Smith, J. (1977). Parental investment: a prospective analysis. Anim. Behav. 25, 1–9. doi: 10.1016/0003-3472(77)90062-8
McDonald, G. C., Spurgin, L. G., Fairfield, E. A., Richardson, D. S., and Pizzari, T. (2017). Pre- and postcopulatory sexual selection favor aggressive, young males in polyandrous groups of red junglefowl. Evolution 71, 1653–1669. doi: 10.1111/EVO.13242
Osborne, D. R. (1982). Replacement nesting and polyandry in the wattled jacana. Wilson Bull 94, 206–208.
Owens, I. P. F., Burke, T., and Thompson, D. B. A. (1994). Extraordinary sex roles in the Eurasian dotterel: female mating arenas, female-female competition, and female mate choice. Am. Nat. 144, 76–100. doi: 10.1086/285662
Pariser, E. C., Mariette, M. M., and Griffith, S. C. (2010). Artificial ornaments manipulate intrinsic male quality in wild-caught zebra finches (Taeniopygia guttata). Behav. Ecol. 21, 264–269. doi: 10.1093/beheco/arp185
Pinheiro, J., Bates, D., DebRoy, S., and Sarkar, D. (2013). Nlme: Linear and Nonlinear Mixed Effects Models. R package.
R Development Core Team (2018). R: A Language and Environment for Statistical Computing. R Foundation for Statistical Computing. Vienna: R Development Core Team.
Russell, A. L., Buchmann, S. L., De Sabino, W. O., and Papaj, D. R. (2018). Brawls bring buzz: male size influences competition and courtship in Diadasia rinconis (Hymenoptera: Apidae). J. Insect Sci. 18:18. doi: 10.1093/JISESA/IEY083
Serrao, J. S., and Shekar, P. B. (1962). Pheasant-tailed jacana at Kalina. News Lett. Bird Watch 2, 4–6.
Slatyer, R. A., Jennions, M. D., and Backwell, P. R. Y. (2012). Polyandry occurs because females initially trade sex for protection. Anim. Behav. 83, 1203–1206. doi: 10.1016/J.ANBEHAV.2012.02.011
Székely, T., Lislevand, T., and Figuerola, J. (2007). “Sexual size dimorphism in birds,” in Sex, Size and Gender Roles, eds D. Fairbairn, W. Blanckenhorn, and T. Székely (Oxford: Oxford University Press), 27–37.
Székely, T., Remeš, V., Freckleton, R. P., and Liker, A. (2013). Why care? Inferring the evolution of complex social behaviour. J. Evol. Biol. 26, 1381–1391. doi: 10.1111/jeb.12148
Székely, T., and Williams, T. D. (1995). Costs and benefits of brood desertion in female kentish plovers, Charadrius alexandrinus. Behav. Ecol. Sociobiol. 37, 155–161. doi: 10.1007/BF00176712
Tarboton, W. R. (1993). Incubation behaviour of the African jacana. South African J. Zool. 28, 32–39. doi: 10.1080/02541858.1993.11448285
Tarboton, W. R. (1995). Polyandry in the African jacana: the roles of male dominance and rate of clutch loss. Ostrich 66, 49–60. doi: 10.1080/00306525.1995.9633759
Tarboton, W. R., and Fry, C. H. (1986). Breeding and other behaviour of the lesser jacana. Ostrich 57, 223–243. doi: 10.1080/00306525.1986.9633661
Thong-aree, S., Khobkhet, O., Lauhachinda, V., and Pong-umpai, S. (1995). Breeding biology of pheasant-tailed jacana, Hydrophasianus chirurgus in central Thailand. J Nat Hist Soc Siam 43, 289–302.
Trivers, R. L. (1972). “Parental investment and sexual selection,” in Sexual Selection and the Descent of Man First, ed. B. Campbell (Chicago, IL: Aldine), 53–95.
Whittingham, L. A., Sheldon, F. H., and Emlen, S. T. (2006). Molecular phylogeny of jacanas and its implications for morphologic and biogeographic evolution. Auk 117:22. doi: 10.1093/auk/117.1.22
Zheng, J., Komdeur, J., Székely, T., Versteegh, M. A., Li, D., Wang, H., et al. (2021). Males and females of a polygamous songbird respond differently to mating opportunities. Behav. Ecol. Sociobiol. 75:72. doi: 10.1007/s00265-021-03000-9
Keywords: polyandry, sex-role reversal, male-only care, Jacanidae, sexual competition
Citation: Fresneau N, Lee Y-F, Lee W-C, Kosztolányi A, Székely T and Liker A (2021) Sex Role Reversal and High Frequency of Social Polyandry in the Pheasant-Tailed Jacana (Hydrophasianus chirurgus). Front. Ecol. Evol. 9:742588. doi: 10.3389/fevo.2021.742588
Received: 16 July 2021; Accepted: 06 October 2021;
Published: 03 November 2021.
Edited by:
E. Tobias Krause, Institute of Animal Welfare and Animal Husbandry, Friedrich-Loeffler-Institute, GermanyReviewed by:
Eliane Gonçalves De Freitas, São Paulo State University, BrazilPiotr Matyjasiak, Cardinal Stefan Wyszyński University, Poland
Copyright © 2021 Fresneau, Lee, Lee, Kosztolányi, Székely and Liker. This is an open-access article distributed under the terms of the Creative Commons Attribution License (CC BY). The use, distribution or reproduction in other forums is permitted, provided the original author(s) and the copyright owner(s) are credited and that the original publication in this journal is cited, in accordance with accepted academic practice. No use, distribution or reproduction is permitted which does not comply with these terms.
*Correspondence: Nolwenn Fresneau, bm9sd2Vubi5mcmVzbmVhdUBnbWFpbC5jb20=