- 1Laboratório de Diversidade Animal/Sistemática de Mamíferos, Departamento de Biologia, Universidade Federal de São Carlos (UFSCAR), Sorocaba, Brazil
- 2Departamento de Zoologia, Instituto de Biociências, Universidade de São Paulo (USP), São Paulo, Brazil
- 3Museu de Zoologia, Universidade de São Paulo (MZUSP), São Paulo, Brazil
The Cerrado biome is one of the global hotspots of biodiversity, and non-volant small mammals represent a significant portion of Cerrado species richness (45%) and endemism (86%). Nevertheless, we still lack a comprehensive picture of small mammal diversity patterns and drivers throughout the Cerrado. Here we surveyed small mammals across 45 sites to address species richness, abundance, and composition patterns and their drivers within and across sites, habitats, and localities at the world’s most diverse tropical savanna. As hypothesized, we found: (1) rich assemblages (12–21 species) characterized by few abundant and several intermediate-level and rare species; dominated by oryzomyine and akodontine cricetid rodents, and thylamyine and marmosine within marsupials, each tribe showing distinct habitat requirements; (2) strong habitat selectivity, with assemblages composed of forest dwellers, savanna specialists, and grassland inhabitants; and (3) similar species richness (α-diversity) but high species turnover (β-diversity) across sites, habitats, and localities, suggesting that horizontal stratification (within localities) and geographic location (across the Cerrado) are key drivers of small mammal diversity in tropical savannas. Thus, habitat heterogeneity and geographic location can be inferred as the main factors shaping species richness, abundance, and composition across the analyzed multiple spatial scales. Moreover, we found that geographical distance as well as the distance to neighbor biomes better explained species turnover, indicating landscape history and phylogenetic constraints as the major determinants of Cerrado small mammal diversity, as also evidenced for plants and other animal groups. These data highlight the need to preserve the mosaic of habitats across the different regions of the biome to conserve most of the Cerrado biodiversity.
Introduction
Understanding how species richness, composition, and endemism vary across space is a long-standing, central question in ecology and biogeography (Rosenzweig, 1995; Webb et al., 2002; Lomolino et al., 2006), which can also support setting priorities for biodiversity conservation (Wiens and Graham, 2005; McKnight et al., 2007; Jetz and Fine, 2012). In particular, knowledge on large-scale variations in species richness (α-diversity, the number of species in a given site), and species turnover (β-diversity, changes in species composition across sites), helps creating a comprehensive picture of diversity patterns and their drivers—critical to the understanding of the biogeographic history and diversification of biomes and/or species groups (Melo et al., 2009; Maestri and Patterson, 2016), as well as to pinpoint regions with high diversity and distinct composition for conservation (Socolar et al., 2016; Gianuca et al., 2017; Françoso et al., 2020).
Species richness in tropical savannas is influenced by a variety of factors, including natural fires, herbivore density, precipitation levels, and soil fertility—which ultimately determine habitat heterogeneity among open and forest habitats within these biomes (e.g., Solbrig et al., 1996; Radford et al., 2014; Pringle et al., 2016; Andersen, 2020)—as key drivers. Efforts to understand species turnover and changes in community composition across savannas have shown the importance of both environmental factors, such as bioclimatic gradients (e.g., Bond et al., 2001; Rugemalila et al., 2016; Morales-Martinez et al., 2018), and evolutionary constraints, such as niche conservatism, as drivers of β-diversity (e.g., Campos et al., 2011; Schmidt et al., 2017; Schoeman and Monadjem, 2018).
Tropical savannas are characterized by a highly seasonal climate and a mosaic of grasslands, savannas, woodlands, and forests (Cole, 1986; Solbrig et al., 1996). They represent unique ecosystems, harboring high numbers of endemic plants and animals (Pennington et al., 2018) that have evolved and adapted to their particular environmental conditions (Scholes and Archer, 1997; Mishra and Young, 2020). Although these open biomes represent a large portion of the world’s area and biodiversity (Furley, 2006; Dinerstein et al., 2017), savannas have been neglected in scientific research in comparison to tropical forests (Werneck, 2011). These rich and distinctive ecosystems have also been undervalued in terms of conservation (Colli et al., 2020; Dudley et al., 2020). They are currently threatened by human development, representing a great part of the world’s agricultural and livestock area (Suttie et al., 2005; Ryan et al., 2016), besides facing high rates of native vegetation loss (Van der Walt et al., 2015; Alencar et al., 2020).
The Cerrado, the largest and most diverse of South American savannas (Cole, 1986; McNaughton et al., 1993), is characterized by high species richness and endemism (Ribeiro et al., 2007; Silva et al., 2007; Nogueira et al., 2011; Carmignotto et al., 2012; Valdujo et al., 2012). Yet, only 8.3% of Cerrado native remnants are legally protected (Françoso et al., 2015), thus setting the Brazilian savannas as one of the 36 global hotspots for biodiversity conservation (Myers et al., 2000; Myers, 2003). Cerrado richness is known to vary according to the number and type of habitats in the landscape, reinforcing the importance of habitat heterogeneity, as in savannas worldwide (e.g., Colli et al., 2002; Batalha and Martins, 2007; Camargo et al., 2018). The Cerrado is also characterized by high β-diversity, with compositional changes strongly influenced by the distance to neighboring biomes, environmental conditions (climate and topography), and evolutionary constraints (e.g., Ratter et al., 1996, 2003; Silva, 1996; Brown and Gifford, 2002; Silva and Bates, 2002; Nogueira et al., 2011; Valdujo et al., 2013).
Despite an increase in the number of studies in the last decades, there are still large gaps in our knowledge of Cerrado landscapes and biota (Colli et al., 2020). For Cerrado small mammals, the composite nature of their assemblages, characterized by open vegetation and forest specialists, has long been highlighted (e.g., Alho et al., 1986; Bonvicino et al., 1996; Santos-Filho et al., 2012), as well as the role of gallery forests (GFs) in increasing community diversity due to their higher vertical complexity, allowing access to different forest strata and resources for scansorial and arboreal species (e.g., Redford and Fonseca, 1986; Johnson et al., 1999; Hannibal and Cáceres, 2010). While for lizards the open formations of the Cerrado have shown to be richer than forests (Colli et al., 2002; Nogueira et al., 2009), for birds and mammals, studies advocate the opposite (Silva and Bates, 2002; Camargo et al., 2018). Indeed, the relative contribution of horizontal (number of habitats) and vertical (habitat complexity) stratification for species richness and composition within and across sites and localities has not yet been properly accessed for small mammals. As known to researches for quite some time, field research in large biomes such as the Cerrado is not geographically balanced, being largely concentrated around the core area of the biome, near Brasília, the capital of Brazil, where main university campi are located (see also Mendonça et al., 2018). Research has also been in large part limited to single localities and/or habitat types, focusing on local-scale questions (e.g., Ribeiro and Marinho-Filho, 2005; Bezerra et al., 2009; Godoi et al., 2010), or varying in sampling design and effort, which compromise the strength of comparative analyses across the Cerrado (Marinho-Filho et al., 1994; Vieira and Palma, 2005; Ribeiro et al., 2020).
We conducted a field study to address these shortcomings by covering a much broader geographical extent compared to previous studies in the Cerrado biome, to assess small mammal diversity within and across distinct spatial scales. Our dataset comprises a standardized sampling effort across 45 sites and 7 localities encompassing distinct habitats and portions of the Cerrado, including its core area as well as areas nearby all adjacent biomes. We aim to verify the importance of habitat complexity and heterogeneity, large-scale environmental variables, and phylogenetic constraints, on small mammal richness, abundance, and composition within and across sites, habitats, and localities. We hypothesize that richness will vary across habitats, with forests being richer than savannas, and grasslands presenting the lowest number of species, according to the vertical complexity hypothesis (e.g., Camargo et al., 2018). We also anticipate a high species turnover across habitats, with assemblages dominated by both forest dwellers and open formation specialists, as previously found based on high habitat selectivity and distributional data of small mammals (e.g., Carmignotto et al., 2012; Ribeiro et al., 2020). As such, richness at localities should be strongly influenced by habitat heterogeneity (McCleery et al., 2018; Loggins et al., 2019). Across localities, we expect assemblage turnover to be influenced by the distance to neighbor biomes, large-scale environmental variables, and phylogenetic constraints, as previously shown for anurans (Valdujo et al., 2012, 2013), lizards (Nogueira et al., 2009, 2011), and plants (Françoso et al., 2016, 2020). Consequently, total richness in the Cerrado should have a greater contribution from species turnover across habitats and localities than from average species richness within sites. Finally, we hypothesize that different clades, represented by lineages descended from open habitat or forest dwellers’ ancestors, will present different habitat requirements, as found for lizards and birds at the Cerrado (Silva, 1995, 1997; Nogueira et al., 2009).
Materials and Methods
Study Area
The Cerrado, the largest Neotropical savanna, covers an area of about 2 million km2 at the center of South America (Oliveira and Marquis, 2002; Veblen et al., 2007), being limited by other dry and/or highly seasonal vegetation formations such as the Caatinga and Chaco (Prado and Gibbs, 1993). The Cerrado is also delimited by the two major South America’s rainforests: the Amazonian to the north, and the Atlantic Forest in its southern and eastern limits (Ab’Saber, 1977; Hueck and Seibert, 1981; Figure 1). The Cerrado is characterized by a defined seasonal climate, with a wet (October to April) and a dry (May to September) season, with the former concentrating around 80–90% of annual rainfall (Coutinho, 2006). Annual precipitation varies from 1,200 to 2,300 mm, with mean annual temperature ∼22°C (Nimer, 1989; Alvares et al., 2013; Figure 1). Major drainages cut the Cerrado across the central Brazilian plateau, where altitudes around 1,000–1,300 m dominate, descending to adjacent valleys and depressions (Parada and Andrade, 1977). The ancient plateaus, now covered by Cenozoic sediments, gave rise to sandy soil and lato soil, which are poor in nutrients and rich in minerals (Furley and Ratter, 1988).
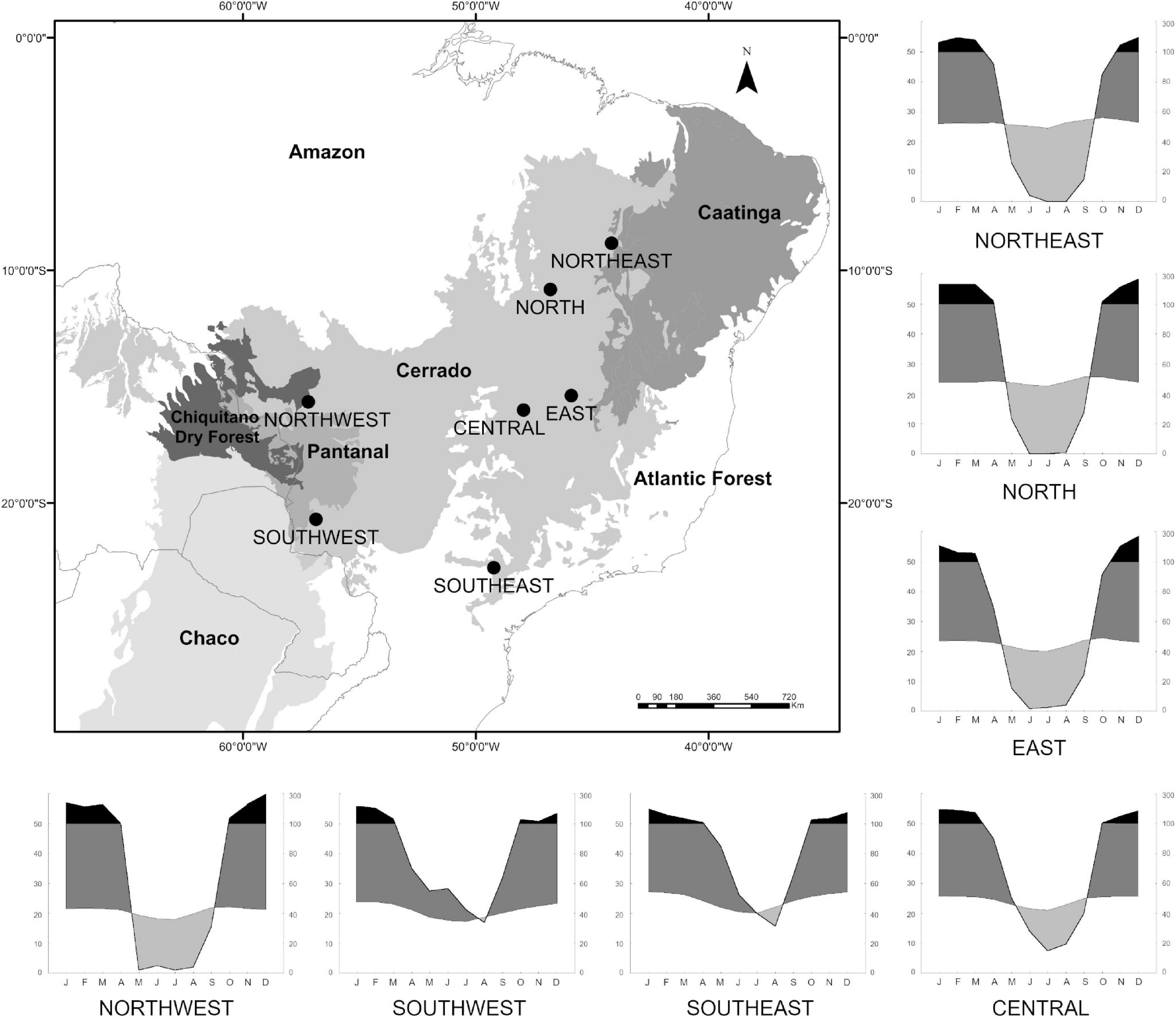
Figure 1. The seven localities surveyed across the Cerrado biome. Limits of the Cerrado and neighboring biomes in gray tones based on the ecoregion approach of Dinerstein et al. (2017). Climatic diagrams showing variations in annual temperature (left vertical axis) and rainfall regime (right vertical axis) within each locality surveyed in the Cerrado. In black, periods of rainfall above 100 mm; in dark gray, periods of rainfall above the temperature line, both periods usually associated with the rainy season; in light gray, periods of water deficiency, associated with the dry season. CENTRAL = Área Alfa Cerrado Reserve, EAST = Grande Sertão Veredas National Park, SOUTHEAST = Santa Bárbara Ecological Station, NORTHWEST = Serra das Araras Ecological Station, SOUTHWEST = Serra da Bodoquena National Park, NORTH = Serra Geral do Tocantins Ecological Station, NORTHEAST = Uruçuí-Una Ecological Station.
As a biome, the Cerrado presents the development of several facies as a result of the interaction between climate, soil types, and drainage (Furley, 1999; Oliveira-Filho and Ratter, 2002). These facies represent distinct habitats that were considered in the sampling design of the study as follows: “campo úmido” (CU)—flooded grasslands; “campo limpo” (CL)—a grass-dominated habitat; “campo sujo” (CS)—open grasslands with some trees and shrubs; “campo cerrado” (CC)—shrubby vegetation with a higher density of trees and the intervening ground covered by grasses; “cerrado sensu stricto” (CE)—woodlands with an open canopy and some grass covering the ground; “cerrado rupestre” (RC)—woodlands at rocky landscapes; “carrasco” (CAR)—woodlands with denser understories at transitional areas with Caatinga; “cerradão” (CD)—dense woodlands with a closed canopy; GF—forest formations along watercourses; seasonal forests (SF)—forest formations usually located at hillsides; and wet gallery forests (WGF)—open-canopy forests composed of palms and trees in a water-saturated soil (Ribeiro and Walter, 1998; Figure 2). These habitats were also classified into the three major habitat types present in the Cerrado: grasslands—comprising the different grassland facies (CU, CL, CS, and CC); savannas—representing the woodlands (CE, RC, and CAR); and forests—all surveyed forest formations (CD, GF, SF, and WGF; Supplementary Table 1).
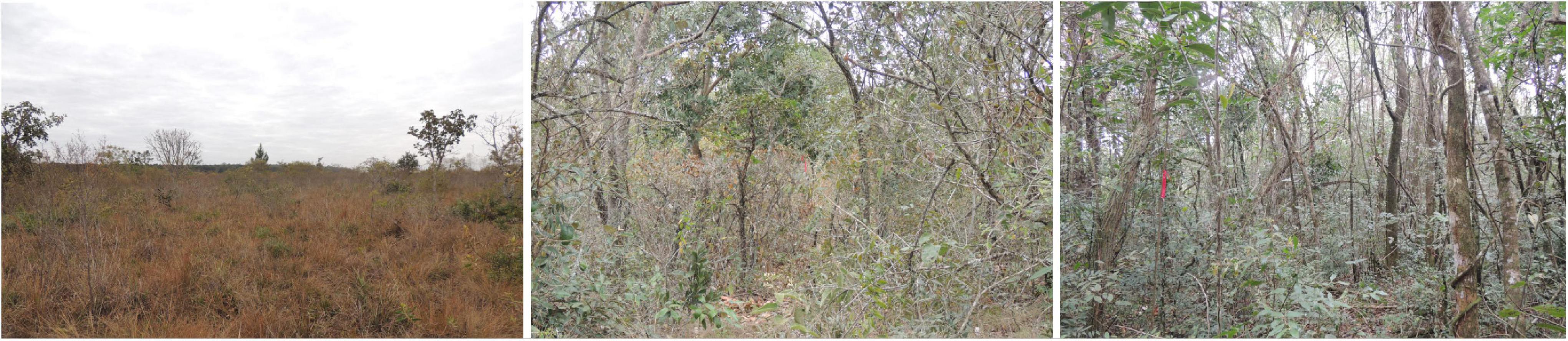
Figure 2. The three main habitat types surveyed within the Cerrado. Grassland [left, “campo sujo (CS)” at the Santa Bárbara Ecological Station], Savanna [center, “cerrado sensu stricto (CE)” at the Santa Bárbara Ecological Station], and Forest [right, “cerradão (CD)” at the Santa Bárbara Ecological Station].
Standardized Sampling Design
Small non-volant mammals were surveyed across seven distinct protected areas chosen across the entire range of the Cerrado and at different distances to the adjacent open or forest biomes, as follows: (1) Uruçuí-Una Ecological Station (a locality situated at northeastern Cerrado, hereafter NORTHEAST) in February/March 2000; (2) Grande Sertão Veredas National Park (a locality situated at eastern Cerrado, hereafter EAST) in October 2001; (3) Área Alfa Cerrado Reserve (a locality situated at central Cerrado, hereafter CENTRAL) in January/February 2002; (4) Serra da Bodoquena National Park (a locality situated at southwestern Cerrado, hereafter SOUTHWEST) in April 2002; (5) Serra das Araras Ecological Station (a locality situated at northwestern Cerrado, hereafter NORTHWEST) in October 2002; (6) Santa Bárbara Ecological Station (a locality situated at southeastern Cerrado, hereafter SOUTHEAST) in January/February 2003; and (7) Serra Geral do Tocantins Ecological Station (a locality situated at northern Cerrado, hereafter NORTH) in March/April 2003 (Figure 1). All localities were sampled for 10 consecutive days of capture during the rainy season (October to April), with ∼2,000 pitfall trap (PT) nights and ∼2,000 live trap (LT) nights per locality (except NORTHEAST, surveyed for 19 days, with ∼3,500 PT nights and ∼3,500 LT nights) (Supplementary Table 1). At each locality, sampling sites comprising both PTs and LTs were set at the different habitats representing the vegetation mosaic typical of the Cerrado (Figure 2), at least 1 km apart. All sampling sites in a given locality were distributed around a 20-km radius from a central point (usually the reserve lodge). The number of sampling sites varied from 5 to 7 per locality, according to the number of habitats available (from 4 to 7), including at least one sampling site in each major habitat type: grassland, savanna, and forest per locality (except for NORTHEAST and SOUTHWEST localities where grassland patches were not available) (Figure 3 and Supplementary Table 1).
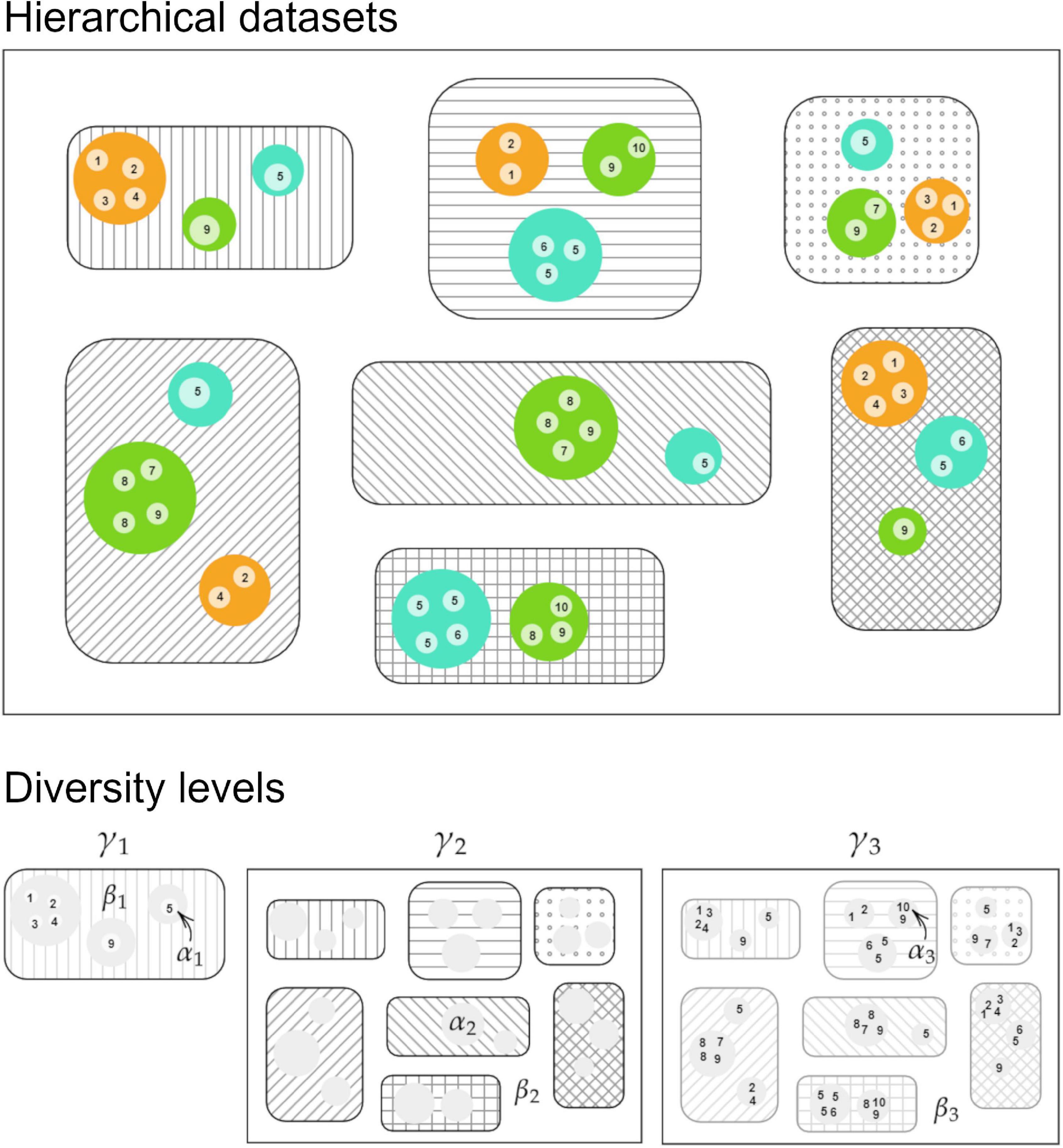
Figure 3. A schematic figure showing the hierarchical datasets: sites (45 points), habitats (10 numbers), habitat types (3 circles), and localities (7 squares) and their relationship with each diversity level analyzed in this study, where α1; α3 = mean species richness at sites, β1 = species richness across sites at localities, γ1; α2 = mean species richness at localities, β2 = species richness across localities; β3 = species richness across sites at the entire sample, γ2; γ3 = species richness of the entire sample.
Data Collection and Sampling Effort
Each sampling site was comprised of two transects: 40 PTs and 30–40 LTs (except at humid and rocky habitats generally surveyed only by LTs—Supplementary Table 1), spaced ∼15 m from each other. LTs were set at the ground to ∼1.5 m high, in line, with Sherman (7.5 cm × 8.5 cm × 23.0 cm; H.B. Sherman Traps, Tallahassee, FL, United States) and mesh-wire LTs (19.5 cm × 20.0 cm × 32.0 cm) interspersed (20–27 Sherman and 10–13 mesh-wire traps) and spaced 15 m from each other, comprising transects 450–600 m long, and a capture effort of ∼350 trap nights (Supplementary Table 1). LTs were baited with a mixture of peanut butter, sardine, and ground maize, adding a slice of manioc in the mesh wire traps. PTs were set in a “Y” configuration, with four 35-L buckets buried in the ground and connected with 50 cm high × 5 m long plastic drift fences at each capture station, with 10 stations spaced 15 m from each other, comprising a transect around 250 m long, and a capture effort of ∼400 trap nights (see also Nogueira et al., 2009; Supplementary Table 1). PT and LT transects were checked daily. Capture effort was estimated by multiplying the total number of traps with the number of nights they remained open in the field for each trap transect, site, habitat, and locality (Supplementary Table 1). A total of 45 sampling sites representing the mosaic of habitats of the Cerrado, which were classified as grasslands (15 sites), savannas (13 sites), and forests (17 sites) across the 7 localities, were surveyed, summing 15,963 PT nights and 16,121 LT nights (Supplementary Table 1).
Voucher specimens were deposited at the mammal collection of the Museu de Zoologia da Universidade de São Paulo (MZUSP), São Paulo, Brazil. When capture numbers exceeded 20, individuals were temporarily marked (with non-toxic colored enamel) and released at the capture site, to identify recaptures. Additionally, tissue samples were obtained for all vouchers and preserved in the above-mentioned collection, and chromosome preparations were made in the field for most collected specimens. The capture and collection of small mammals were approved by the IBAMA (permits no. 172/99; 065/2001; 353/2001; 355/2001; 150/2002; and 002/2003), and they followed the ASM guidelines for the use of wild mammals in research (Sikes and The Animal Care and Use Committee of the American Society of Mammalogists, 2016). Taxonomic identifications were based on morphological, karyological, and/or molecular data. Nomenclature and classification follow Voss and Jansa (2009) for the marsupials and Patton et al. (2015) for the rodents, and also follow recently published arrangements and species descriptions (Nascimento et al., 2013; Bonvicino et al., 2014a; Hurtado and Pacheco, 2017; Weksler et al., 2017; Suárez-Villota et al., 2018; Silva et al., 2019; Brandão et al., 2021; Fegies et al., 2021).
Data Analyses
Taxonomic α-Diversity Across Scales
To evaluate sampling effort and to estimate and compare species richness across sites, habitats, and localities, we used abundance data to calculate the coverage estimator and to generate sample size-based rarefaction and extrapolation sampling curves (Chao and Jost, 2012; Colwell et al., 2012). The coverage estimator represents the proportion of the total number of individuals in an assemblage belonging to a species represented in the sample, estimated in percentage, as a measure of completeness. We used the online iNEXT platform (Hsieh et al., 2016) with 1,000 bootstraps, considering as an endpoint setting for extrapolation curves, the double reference sample size (Chao et al., 2014), and treating the reference sample as the mean sample size for localities, as 50 individuals for sites, and as the small sample size for habitat types, to obtain extrapolated data for all samples. CIs of 95% were used as threshold in comparisons (Chao and Chiu, 2016).
iNEXT platform also estimates diversity measures based on the parameter “q” of the Hill numbers (Hill, 1973; Jost, 2006), which takes into account species richness and relative abundance data, with increasing sensitivity for dominant species (Chiu and Chao, 2014), and are recommended for biologically interpretable comparisons among samples (Moreno et al., 2018). Thus, besides species richness (q = 0—abundance data not considered), we also estimated the Shannon–Wiener diversity index (q = 1; the exponential of Shannon entropy—same weight for all species abundance data) and the Simpson diversity index (q = 2; the inverse of Simpson concentration—common species more important). Species richness was compared across habitats and habitat types (grasslands, savannas, and forests) to verify the contributions of habitat diversity and complexity in assemblage’s diversity.
Species were classified by abundance data, based on Whittaker plots, as rare (summing up to 10% of total abundance), abundant (summing up to 50%), and intermediate level, and also by family, subfamily and tribe as follows: marsupials from Didelphidae family were classified into Caluromyinae and Didelphinae subfamilies, and this latter into Didelphini, Marmosini, and Thylamyini tribes; rodents from Cricetidae family into Akodontini, Oryzomyini, Phyllotini, Thomasomyini, and Wiedomyini tribes; and rodents from Echimyidae family into Eumysopinae subfamily, and Caviidae family into Caviinae subfamily.
Taxonomic β-Diversity Across Scales
Our dataset is hierarchical and encompasses sites (n = 45), localities (n = 7), habitats (n = 10: CU, CL, CS, CC, CE, RC/CAR, CD, GF, SF, and WGF), and habitat types (n = 3: grasslands, savannas, and forests; Figure 3 and Supplementary Table 1). As such, we applied the additive partitioning concept of diversity: γ-diversity = α-diversity + β-diversity (Loreau and Hector, 2001; Veech et al., 2002; Chao et al., 2012) to investigate the contribution of species richness (α-diversity) and species turnover (β-diversity) across scales, i.e., within and across sites, habitats, and localities. α-diversity (α1) was treated as the mean observed and extrapolated number of species recorded in each site, β-diversity (β1) as the species richness found across sites (or habitats), and γ-diversity (γ1) as the mean number of species in a given locality. α-diversity (α2) was treated as the mean observed and extrapolated number of species recorded in each locality (α2 = γ1), β-diversity (β2) as the species richness found across localities, with γ-diversity (γ2) as the observed and estimated number of species found in the entire sample. α-diversity (α3) was also treated as the mean observed and extrapolated number of species recorded in each site (α3 = α1), β-diversity (β3) as the number of species recorded across all sites (or habitats) encompassing all localities, with γ-diversity (γ3) as the observed and estimated number of species found in the entire sample (γ3 = γ2) (see Figure 3). Sample-based rarefaction and extrapolation curves for sites (or habitats) and localities were used to estimate species richness (q = 0) across scales. Additionally, to evaluate β-diversity for the three main habitat types: grasslands, savannas, and forests, we also generated the curves based on species richness (q = 0), the Shannon–Wiener (q = 1), and Simpson (q = 2) diversity indices considering all sites grouped by habitat type (Figure 3).
Community Structure
We used non-metric multidimensional scaling (NMDS) ordination based on the Bray–Curtis similarity index (Clarke, 1993; Moreno et al., 2018) to graphically illustrate differences in community structure across sites, habitats, and localities; a Shepard plot was performed to verify the goodness of fit of the data in the NMDS analysis. The capture success for each species per site was computed as the total number of individuals divided by the capture effort and used in this analysis. Only species with at least five captures considering all sites were included, to prevent biasing the results due to a small sample size (see Linzey and Kesner, 1997; Carvalho et al., 2020). NMDS ordination was performed with the “vegan” R package (Oksanen et al., 2020), function metaMDS; and the Shepard plot (or stress plot) using the stressplot R function.
The Jaccard similarity index based on the presence/absence of data between pairwise localities was used to investigate spatial autocorrelation due to its robustness, where rare and abundant species are similarly weighted, being sensitive to species turnover in rare species and less biased regarding the presence of a few dominant species in assemblages (Moreno et al., 2018). The pairwise matrix of dissimilarity (1 − Jaccard index) was correlated with the pairwise matrix of geographic distances (Ludwig and Reynolds, 1988; Nekola and White, 1999) via the Mantel test (Manly, 1994).
Previous studies have shown the influence of the biota of neighboring biomes in the formation of the species pool of the Cerrado (Valdujo et al., 2012, 2013; Françoso et al., 2016, 2020). Species currently occurring in the Cerrado, which have originated from ancestors that occupied adjacent biomes, would—through niche conservatism—be restricted to areas in the Cerrado with similar environmental conditions to those required by their ancestors, that is, similar to those found in the neighboring biomes. Environmental variables are also known to influence species turnover in animal groups in the Cerrado (Brown and Gifford, 2002 for butterflies; Nogueira et al., 2009 for lizards; and Valdujo et al., 2013 for anurans). Based on these findings, we performed Mantel tests to investigate the relationship between species composition and: (1) geographic distance to neighboring Cerrado biomes: Atlantic Forest, Amazonian Forest, Caatinga, Chaco, and Pantanal. Geographic distances, in kilometer, were calculated in ArcMap 10.1 (Environmental Systems Research Institute [ESRI] Inc, 2012) based on the Dinerstein et al. (2017) ecoregion-based approach map for the biome limits; (2) large-scale environmental variables categorized, as follows, to homogenize the different types of variables for comparisons (McCune and Allen, 1985; Urban, 2000): (1) the length of dry season (1/2 months = 1; 3/4 months = 2; and 5 months = 3), (2) rainfall deficit (1 = 0–50 mm; 2 = 50–100 mm; 3 = 100–150 mm; 4 = 150–200 mm; and 5 = 200–250 mm), (3) annual mean temperature (1 = 20–22°C; 2 = 22–24°C; 3 = 24–26°C; and 4 = 26–28°C), (4) temperature variation between means of the hottest and coldest months (1 = 2–4°C; 2 = 4–6°C; and 3 = 6–8°C), (5) dominant habitat (1 = grasslands; 2 = savannas; and 3 = forests), (6) dominant soil type (1 = sand; 2 = lato soil; 3 = rendzine soil; and 4 = lito soil), and (7) relief (1 = smooth, 2 = mixed; and 3 = steep), and (8) altitude (1 = 0–500 m; 2 = 500–1,000 m; and 3 = >1,000 m). The chosen environmental variables were those revealed as the most significant in previous studies. Climatic data were extracted from Leemans and Cramer (1991) and were also used as the basis for the Walter’s climatic diagrams (Figure 1). Geomorphological data were based on RADAMBRASIL (1982a,b, c). The environmental variable matrix was based on Euclidian relative distances (Ludwig and Reynolds, 1988; Urban, 2000). The Mantel tests were performed in PAST software (Hammer et al., 2008).
Results
We captured 792 individuals of 58 small mammals across sites, habitats, and localities in the Cerrado. PTs captured 299 individuals (38%) of 38 species (66%), while LTs captured 499 individuals (62%) of 47 species (81%) (Supplementary Table 2), highlighting the importance of using both traps in small mammal surveys: 11 species (19%) were recorded exclusively by PTs and 20 species by LTs (34%). Capture success also varied among taxonomic groups (i.e., marsupials from the Order Didelphimorphia, Family Didelphidae, and rodents from the Order Rodentia, Families Cricetidae, Caviidae, and Echimyidae). Cricetid rodents were the dominant group, with 500 (63%) individuals and 30 (52%) species, followed by didelphids, with 175 (22%) individuals and 17 (30%) species, echimyids with 113 (14%) individuals and 9 (15%) species, and cavies with 4 (1%) individuals and 2 (3%) species (Supplementary Table 2). Didelphids and cavies were mostly captured by PTs while LTs captured most cricetids and echimyids. Body size played an important role in differential capture rates, with LTs capturing, in general, larger species, such as echimyids, which were almost exclusively captured by this trap (Supplementary Table 2).
Species Richness and Diversity Across Scales
Analyzing the 45 sampling sites, species richness varied from 2 to 11 species (mean ± SD = 5.44 ± 2.56, Table 1), with the estimated richness ranging from 2.00 to 15.71 (mean ± SD = 7.28 ± 4.02). The sample-based rarefaction and extrapolation curves showed inflection points between 20 and 40 individuals, and a high overlap in species richness (q = 0) were observed across sites at the seven localities (Supplementary Figure 1). There was also a great variation in the number of individuals captured per site, ranging from 2 to 65 (mean ± SD = 17.60 ± 12.81), with sampling coverage varying accordingly, from 0.00 to 1.00 (mean ± SD = 0.82 ± 0.19, Table 1). However, most sites (n = 38; 84%) presented high levels of sampling coverage, above 0.75, evidencing that sites were properly surveyed (mean effort = 355 PT nights and 358 LT nights, Supplementary Table 1). Sites also showed a high overlap in species diversity considering both the Shannon–Wiener (q = 1) and the Simpson diversity (q = 2) indices (not shown), with most species represented by a few individuals—less than 10—across sites.
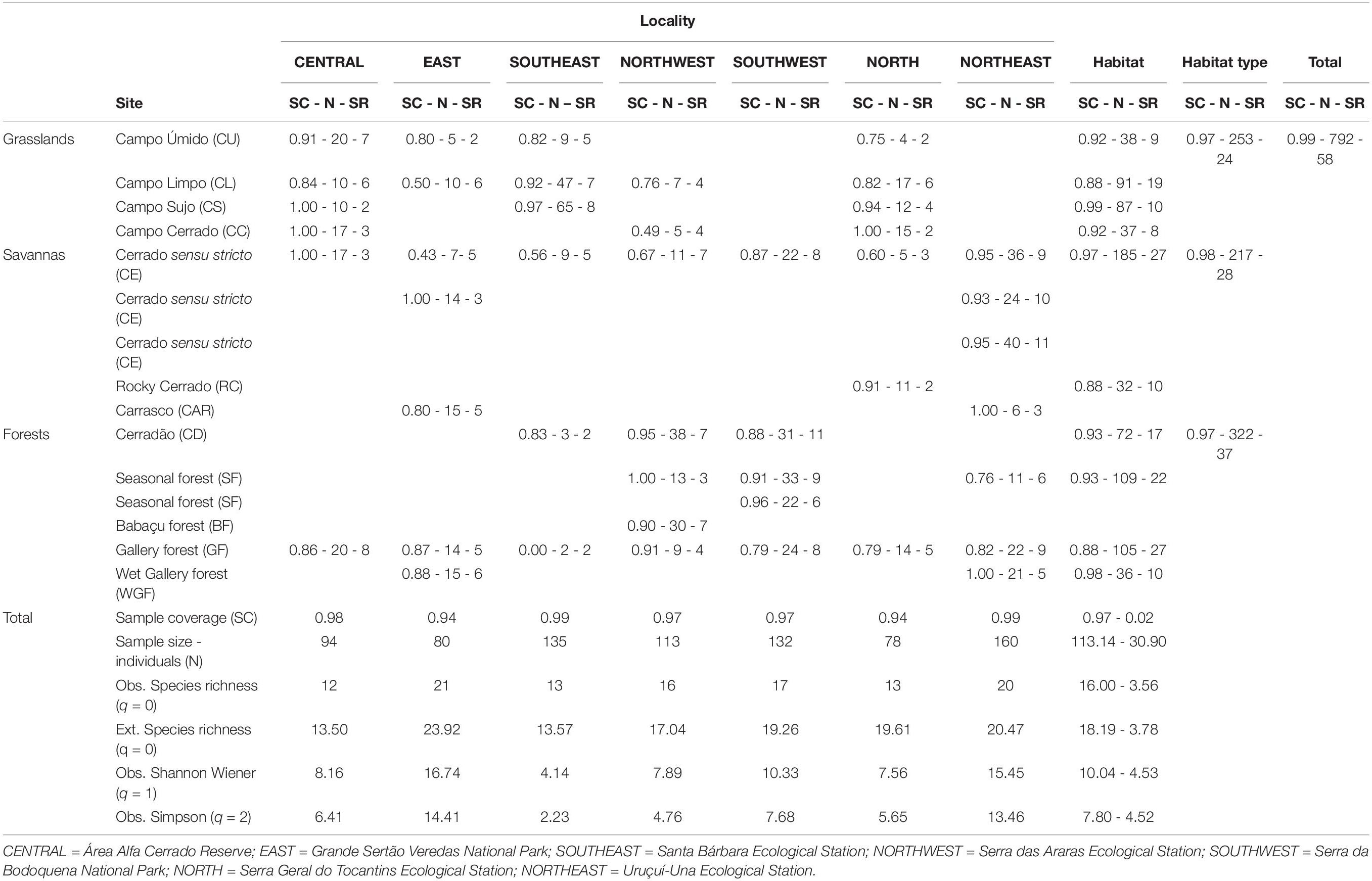
Table 1. Sampling coverage (SC), sample size (n, number of individuals), and the observed species richness (SR, q = 0), at each site, habitat, habitat type, and locality; and extrapolated SR (q = 0), the observed Shannon index (q = 1), and Simpson index (q = 2) for each locality, and mean and SD for all sampled localities.
Grouping the 45 sites by habitat, we also observed a high overlap in the observed species richness (q = 0), which did not increase from grasslands to forests (Figure 4A). The mean observed richness varied from 3.00 ± 1.00 (in the areas of “CC”) to 6.67 ± 4.51 (in the areas of “CD,” Table 1). Analyzing the sites by the three major habitat types (grasslands, savannas, and forests), they also presented similar mean observed richness (mean ± SD = 4.53 ± 2.07 for grasslands; mean ± SD = 5.69 ± 3.01, for savannas; and mean ± SD = 6.06 ± 2.51, for forests; Figure 4B). This is consistent with the results from rarefaction and extrapolation curves that show overlapping across habitats and higher mean species richness values associated with distinct habitats across localities (Supplementary Figure 1). The number of individuals was found to be higher in sites representing different habitats, such as “CL,” “CS,” “CE,” “CD,” GF, and SF, with no relationship to specific habitats (Table 1).
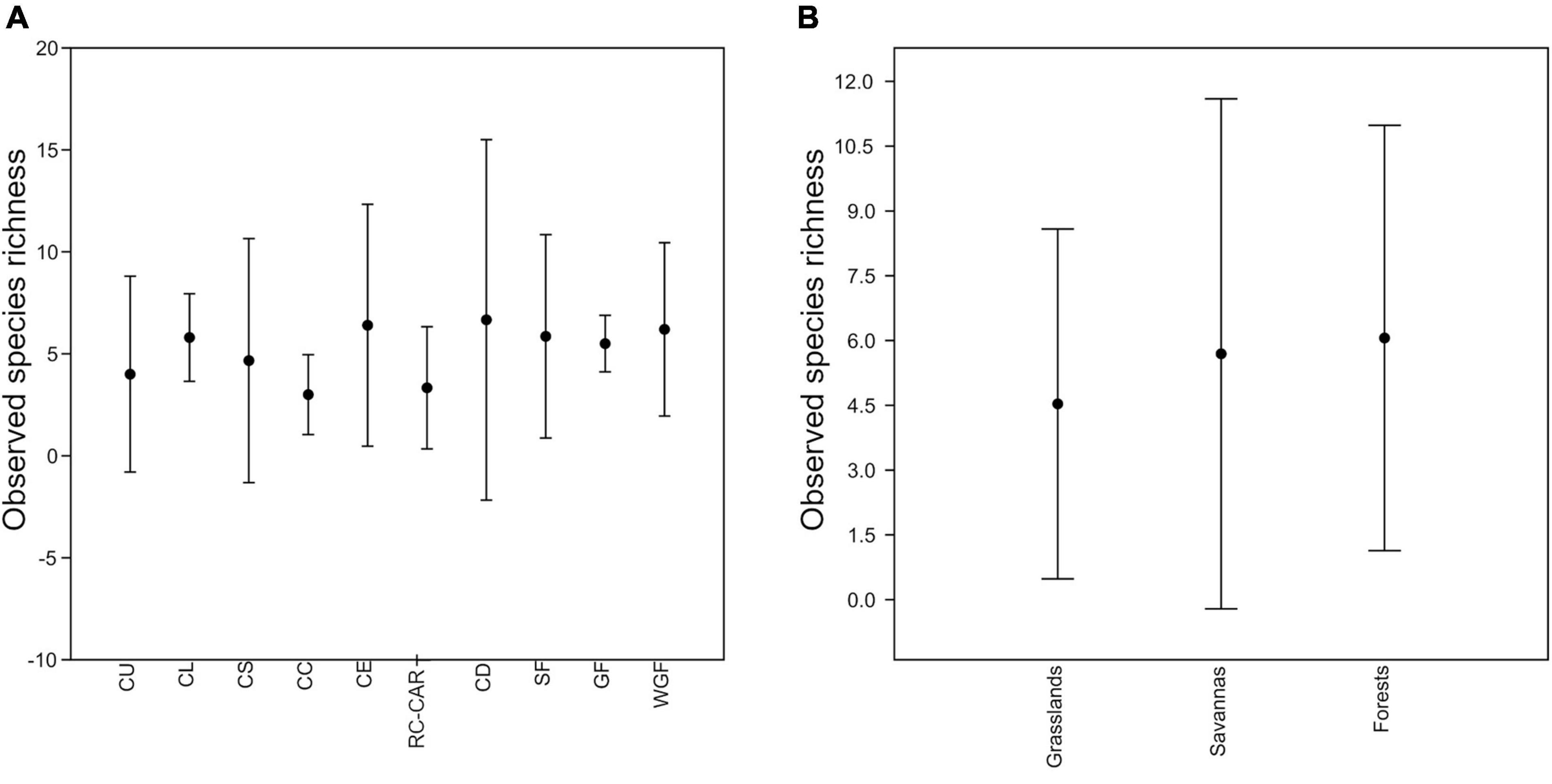
Figure 4. Mean observed species richness across the different habitats (A) and the three main habitat types (B) based on the 45 sites surveyed within the Cerrado. Point = mean, lines = SD, CU = “campo úmido,” CL = “campo limpo,” CS = “campo sujo,” CC = “campo cerrado,” CE = “cerrado sensu stricto,” RC = “cerrado rupestre,” CAR = “carrasco,” CD = “cerradão,” GF = gallery forest, SF = seasonal forest, WGF = wet gallery forest.
Sample-based rarefaction and extrapolation curves showed high richness (q = 0) and also an overlap across habitat types, especially between grasslands (mean ± SD = 27.73 ± 6.31) and savannas (mean ± SD = 30.48 ± 5.79), with forests showing a somewhat higher richness (mean ± SD = 39.69 ± 5.74) (Supplementary Figure 2A). The results for the Shannon diversity (q = 1), however, point savannas and forests as more diverse than grasslands, and savannas as the most diverse habitat type considering the Simpson index (Supplementary Figures 2B,C). This is consistent with the fact that grasslands were hyper dominated by one species (Necromys lasiurus), whereas savannas have shown the most even distribution across species (Supplementary Table 3).
While small mammal diversity greatly overlapped across sites, habitats, and habitat types, taxonomic representativeness was strongly related to habitat. Forests were dominated by oryzomyines, with echimyids and marmosines also comprising an important component of these denser habitats, while thylamyines were more associated with savannas, as well as phyllotines and wiedomyines, with akodontines predominating in grasslands (Figure 5 and Supplementary Table 3).
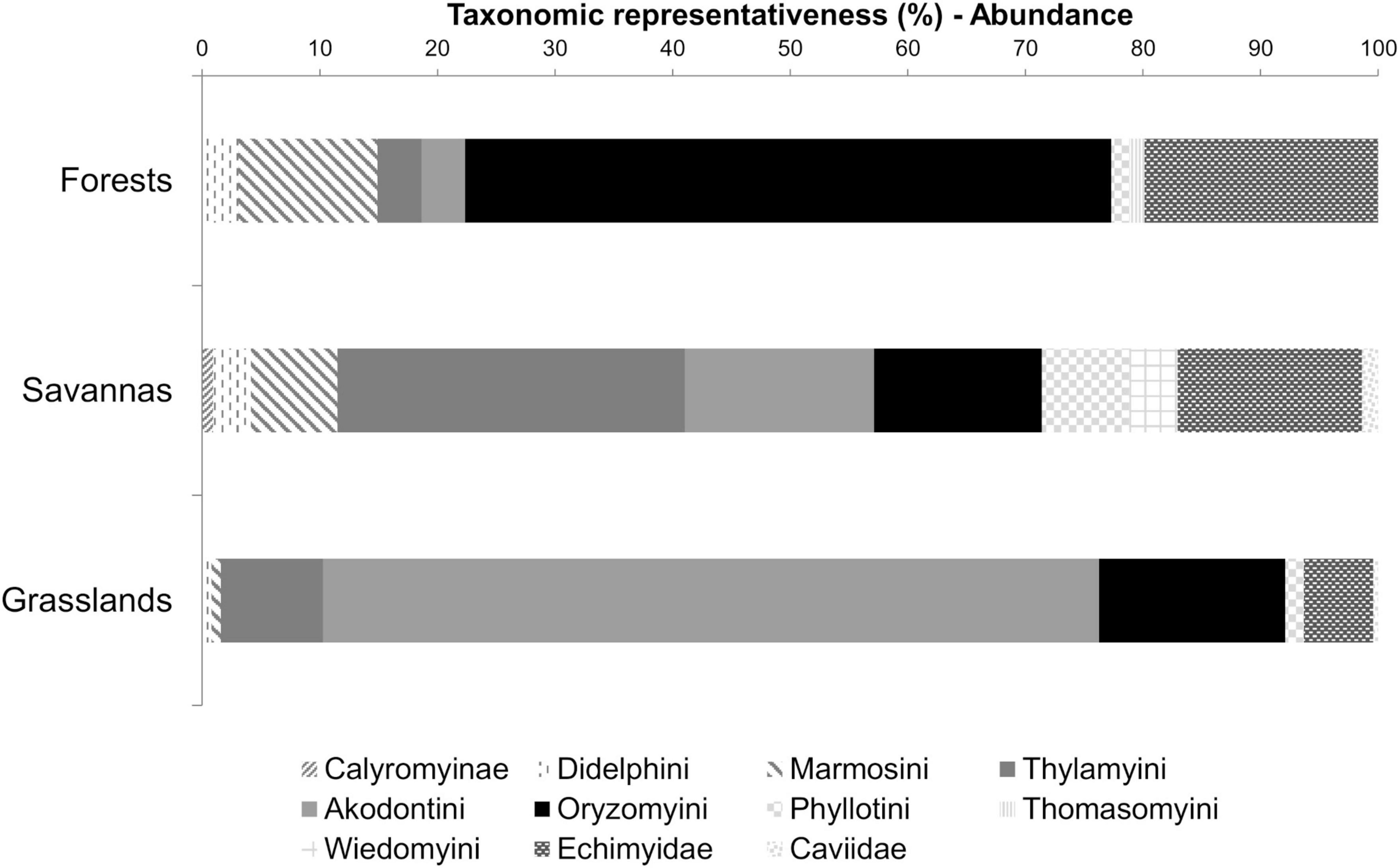
Figure 5. Percentage of taxonomic groups based on the number of individuals surveyed at the three main habitat types across the 45 sites in the Cerrado.
The observed species richness (q = 0) varied from 12 to 21 species per locality (mean ± SD = 16.00 ± 3.56), with the estimated richness ranging from 13.50 to 23.92 species (mean ± SD = 18.19 ± 3.78, Table 1). The sample-based rarefaction and extrapolation curves showed inflection points between 100 and 150 individuals (Figure 6A), and, despite a varying number of individuals per locality (78–160, mean ± SD = 113.14 ± 30.90), all localities presented high values of sampling coverage, from 0.94 to 0.99 (mean ± SD = 0.97 ± 0.02, Table 1), evidencing the robustness of sampling effort (mean = 2,280 PT-nights and 2,303 LT-nights, Supplementary Table 1). Species richness rarefaction and extrapolation curves showed a great overlap, varying from 10 to 31 species, and not differing significantly across localities (Figure 6A). However, the results from both the Shannon–Wiener (q = 1) and the Simpson diversity (q = 2) indices, which consider species abundance, differed across localities, being possible to identify three distinct levels of diversity: the lowest values were found at SOUTHEAST, with four localities (NORTHWEST, NORTH, CENTRAL, and SOUTHWEST) showing intermediate diversity levels, and NORTHEAST and EAST representing the most species-rich localities (Figures 6B,C).
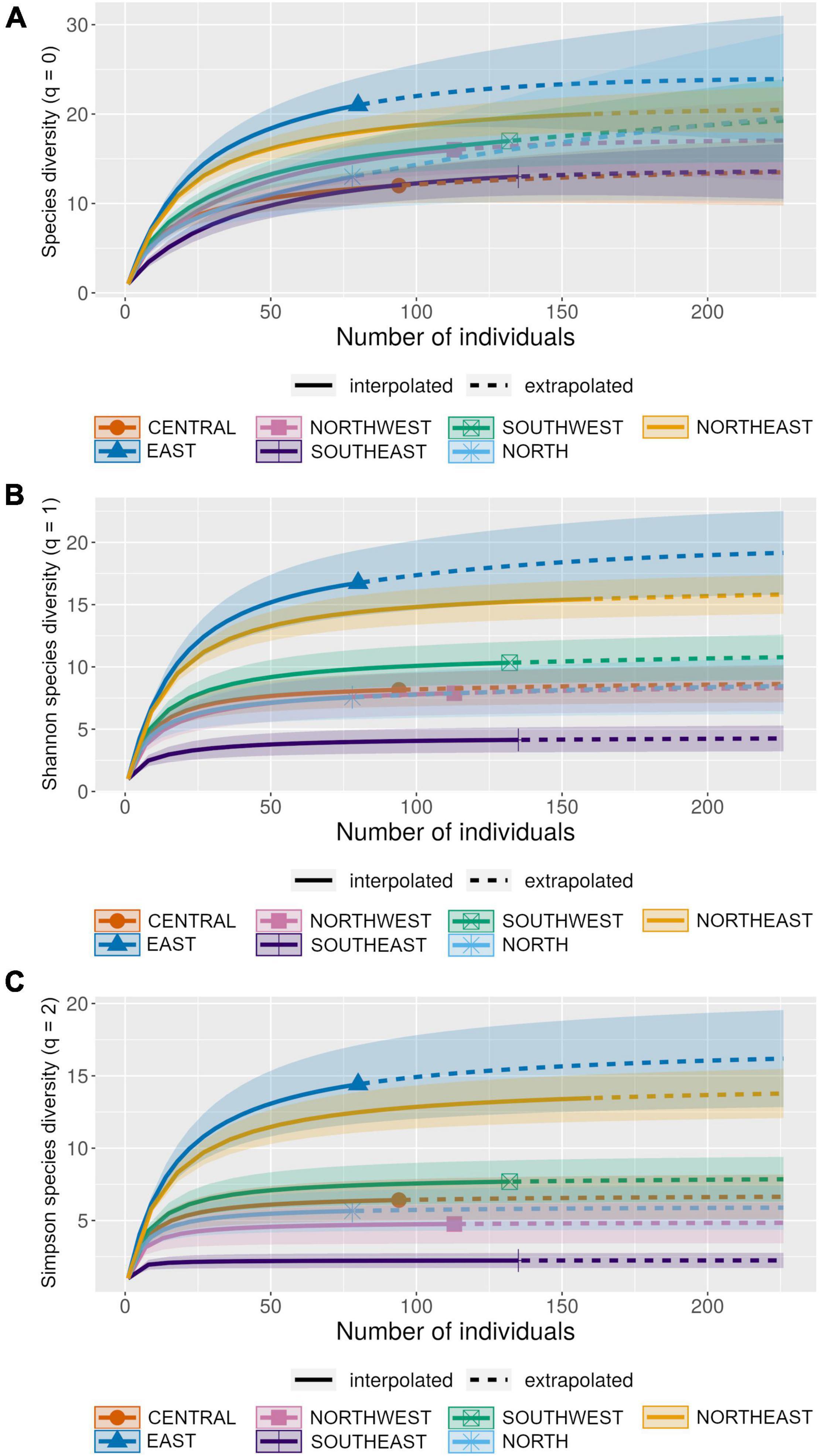
Figure 6. Rarefaction and extrapolation curves based on abundance data for each locality surveyed in the Cerrado. The sample-based curves were generated on the online iNEXT platform (Hsieh et al., 2016). (A) Observed species richness (q = 0), (B) observed Shannon index (q = 1), and (C) observed Simpson index (q = 2). CENTRAL = Área Alfa Cerrado Reserve, EAST = Grande Sertão Veredas National Park, SOUTHEAST = Santa Bárbara Ecological Station, NORTHWEST = Serra das Araras Ecological Station, SOUTHWEST = Serra da Bodoquena National Park, NORTH = Serra Geral do Tocantins Ecological Station, NORTHEAST = Uruçuí-Una Ecological Station.
Relative abundance varied greatly across species, with assemblages encompassing few abundant ones (from 1 to 6 species)—summing up from 8 to 29% of individuals per locality, and several species being either in the intermediate level (from 5 to 9 species)—comprising 38 to 46% of individuals, or rare (from 4 to 7 species)—representing between 29 and 46% of individuals (Supplementary Figure 3). Indeed, only 5 species (9% of all species) were frequently captured, with more than 20 individuals in a given locality, and most species not reached 10 individuals per locality (Supplementary Table 2). SOUTHEAST locality, the less diverse in relation to the Shannon–Wiener and Simpson indices (Figures 6B,C), also showed lower species richness and lower evenness in abundance distribution across species.
Taxonomic representativeness pointed didelphids (tribes Marmosini and Thylamyini), cricetids (tribes Akodontini, Oryzomyini, and Phyllotini), and echimyids (subfamily Eumysopinae) as the most representative groups. However, the importance of each group varied across localities (Supplementary Figure 4). While oryzomyines dominated in the number of species and individuals in most localities, akodontines were the major group in abundance in SOUTHEAST and NORTH. Echimyids, usually representing around 5–20% of species richness and abundance, summed 33% of NORTH individuals. Among didelphids, thylamyines were the most important group, but marmosines also contributed in NORTHWEST and SOUTHWEST. At NORTHEAST, for instance, didelphids represented 50% of all individuals. The two richest localities, EAST and NORTHEAST (Figures 6A–C), were also the ones with the highest taxonomic diversity, represented by 8 and 10 distinct taxonomic groups, respectively (Supplementary Figure 4).
Contributions of α- and β-Diversity Across Scales
Observed and estimated mean α1-diversity (at sites; varying from 2 to 11 species) represented a small portion (34–40%) of γ1-diversity (at localities; varying from 12 to 21 species), with β1-diversity (the species turnover across sites or habitats) mostly contributing (66–60%) (Supplementary Figure 5). Considering the entire sample for the Cerrado (γ2,3-diversity; 58 species), the importance of β2-diversity (72.4–70.9%—species turnover across localities) and β3-diversity (90.6–88.4%—species turnover across habitats and localities, all sites) was even greater compared to α2-diversity (27.6–29.1%—species richness per locality) and α3-diversity (9.4–11.6%—species richness per site; Supplementary Figure 5).
Community Structure
The NMDS analysis (stress = 0.15; see also the Shepard plot in Supplementary Figure 6) based on the Bray–Curtis similarity index shows distinct small mammal assemblages across sites, habitats, and localities. Considering only species with at least five captures during this study (n = 31; 53%), we found two major clusters, with all forests (except SOUTHEAST GF) segregated from grasslands and savannas (Figure 7). This community structure—of forest vs. open habitat—was observed across all seven localities. Community structure also differed within major habitat types, with the areas of “CU” clustering together irrespective of the locality, and the areas of dry grasslands being more similar to each other than with the areas of savannas, whereas savannas presented distinct community structures—two distinct clusters—evidencing a marked regionalization across savannas (Figure 7). This is consistent with a strong species turnover across localities (β2; Supplementary Figure 5), which is also pointed by the rarefaction and extrapolation curves of the three major habitat types (Supplementary Figure 2).
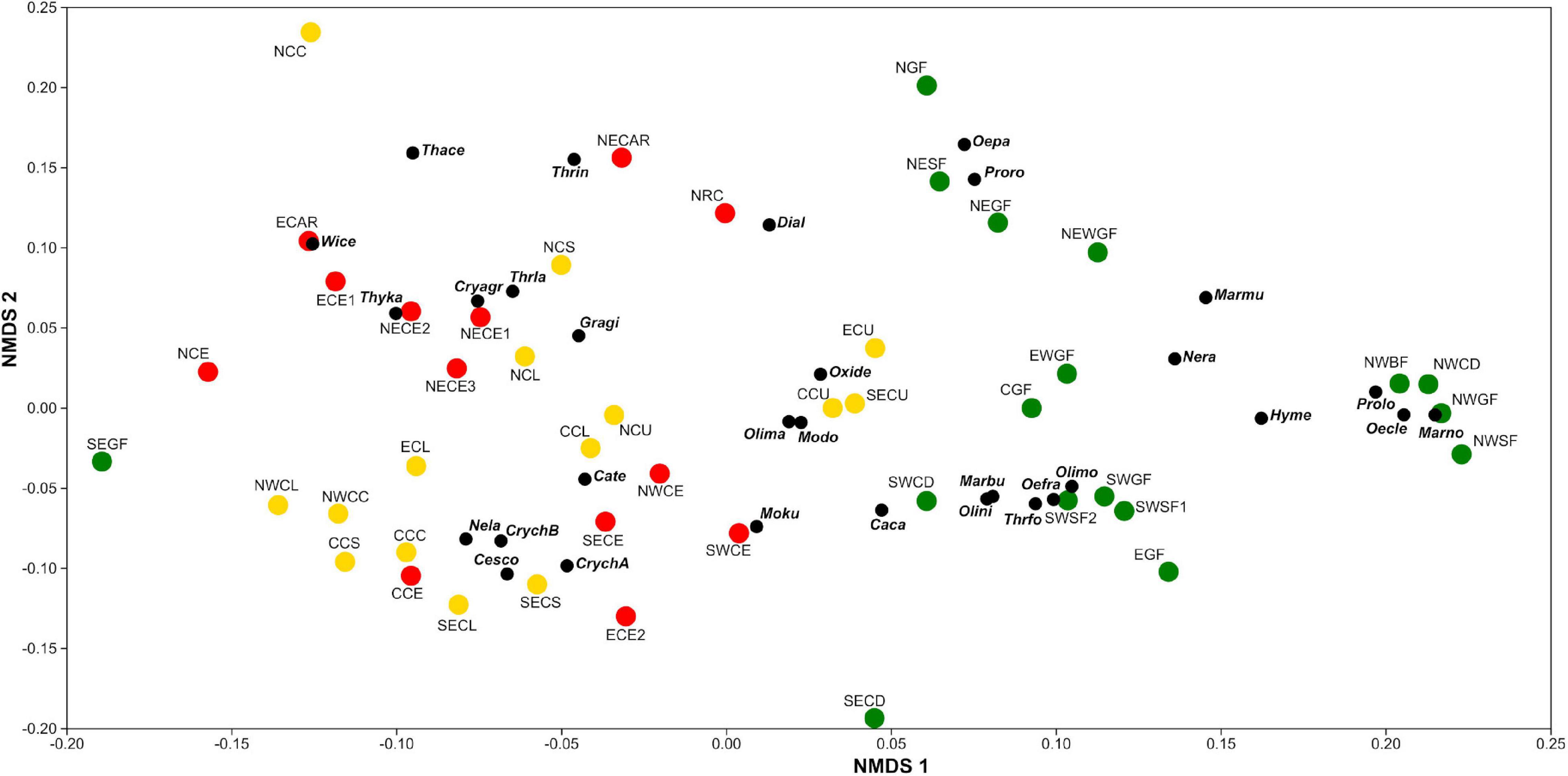
Figure 7. A non-metric multidimensional scaling (NMDS) ordination plot based on a Bray–Curtis similarity matrix, considering the capture success of species with at least five captures across the 45 sites surveyed in the Cerrado. Sites in color, with forests represented by green circles, savannas by red circles, and grasslands by yellow circles. Black circles represent the species. Site acronyms are the junctions of localities and habitats, as follows: CENTRAL (C) = Área Alfa Cerrado Reserve, EAST (E) = Grande Sertão Veredas National Park, SOUTHEAST (SE) = Santa Bárbara Ecological Station, NORTHWEST (NW) = Serra das Araras Ecological Station, SOUTHWEST (SW) = Serra da Bodoquena National Park, NORTH (N) = Serra Geral do Tocantins Ecological Station, NORTHEAST (NE) = Uruçuí-Una Ecological Station, CU = “campo úmido,” CL = “campo limpo,” CS = “campo sujo,” CC = “campo cerrado,” CE = “cerrado sensu stricto,” RC = “cerrado rupestre,” CAR = “carrasco,” CD = “cerradão,” GF = gallery forest, SF = seasonal forest, WGF = wet gallery forest, Species acronyms: Caca = Calomys callosus, Cate = Calomys tener, Cesco = Cerradomys scotti, Cryagr = Cryptonanus agricolai A, CrychA = Cryptonanus chacoensis A, CrychB = Cryptonanus chacoensis B, Dial = Didelphis albiventris, Gragi = Gracilinanus agilis, Hyme = Hylaeamys megacephalus, Marbu = Marmosa budini, Marmu = Marmosa murina, Marno = Marmosops noctivagus, Modo = Monodelphis domestica, Moku = Monodelphis kunsi, Nela = Necromys lasiurus, Nera = Nectomys rattus, Oecle = Oecomys cleberi, Oefra = Oecomys franciscorum, Oepa = Oecomys paricola, Olima = Oligoryzomys mattogrossae, Olimo = Oligoryzomys moojeni, Olini = Oligoryzomys nigripes, Oxide = Oxymycterus delator, Prolo = Proechimys longicaudatus, Proro = Proechimys roberti, Thace = Thalpomys cerradensis, Thrla = Thrichomys cf. laurentius, Thrfo = Thrichomys fosteri, Thrin = Thrichomys inermis, Thyka = Thylamys karimii, Wice = Wiedomys cerradensis.
Assemblages were characterized by high habitat selectivity, with 14 species associated to forests, and 17 species mostly occurring on grasslands and savannas. Among the first group, there are species clearly associated with different portions of the Cerrado, with species from NORTHWEST clustering together and apart from others (e.g., Marmosops noctivagus, Oecomys cleberi, and Proechimys longicaudatus), in the same manner as those from SOUTHWEST (e.g., Marmosa budini, Oecomys franciscorum, and Thrichomys fosteri), and those from NORTHEAST and EAST (e.g., Oecomys paricola and Proechimys roberti), demonstrating high regionalization also across forests. Oxymycterus delator and Oligoryzomys mattogrossae showed a strong relationship with “CU,” while others were more associated to dry grasslands (e.g., Cryptonanus chacoensis B, N. lasiurus, and Cerradomys scotti), and to savannas (e.g., Cryptonanus agricolai A, Thylamys karimii, and Thrichomys cf. laurentius). Distinct species composition was also found across localities, with NORTH, NORTHEAST, and EAST (e.g., Thalpomys cerradensis, Wiedomys cerradensis, and Thrichomys inermis) segregating from NORTHWEST, SOUTHWEST, and CENTRAL (e.g., C. chacoensis A, Monodelphis kunsi, and Calomys tener; Figure 7), reinforcing the species turnover pattern found in open habitats as well.
All taxonomic groups (didelphids, cricetids, and echimyids) showed similar patterns of habitat selectivity, with species segregation between forest and open habitats (Figure 7). Yet, thylamyines among didelphids, and wiedomyines and akodontines among rodents, were mostly associated with savannas and grasslands, respectively, as was also pointed by taxonomic representativeness in the three major habitat types (Figure 5).
The Jaccard similarity index based on the presence/absence of species between localities showed low values in most pairwise comparisons (Supplementary Table 4). These results point to distinct assemblages across the Cerrado, characterized by several exclusive species in a given locality (Supplementary Table 2). Despite a significant spatial correlation (Mantel test, r = 0.467, t = 1.988, p = 0.047), geographic distance between localities explained a small portion of the variation in species composition (R2 = 0.218; Supplementary Figure 7). The correlation between composition similarity and the distance to adjacent biomes was also significant (Mantel test, r = 0.557, t = 2.350, p = 0.019), pointing the importance of geographic location and the influence of neighbor biomes for Cerrado small mammal composition (R2 = 0.314). Large-scale environmental variables, however, showed no correlation with the composition similarity (Mantel test, r = 0.365, t = 1.852, p = 0.064). Although environmentally similar localities, such as NORTHWEST and NORTH, were more similar regarding species composition, CENTRAL and SOUTHEAST represent very distinct environments but presented quite similar faunas (Figure 1 and Supplementary Tables 4, 5).
Discussion
Species Richness and Diversity Across Scales
Species richness in the 45 sites (2–11, mean = 5.44) was very similar to the results reported by Vieira and Palma (2005; 2–10, mode = 5) in a study compiling 82 sites (the capture effort of at least 500 LT nights and 10 individuals) and by Mendonça et al. (2018; 1–26 species, mean = 5.82) in a literature review encompassing 446 sites. Although highly variable, α-diversity at sites (α1) has been properly accessed in most studies (e.g., Cáceres et al., 2010, 2011a; Bonvicino et al., 2012; Carmignotto et al., 2014; Gomes et al., 2015), contributing to the species richness found across habitats and localities at the Cerrado, as discussed below.
In relation to the habitat mosaic, however, we found unexpected results: similar species richness across habitats. According to the vertical complexity hypothesis, richness would vary across habitats, with forests being richer than savannas, and grasslands presenting the lowest number of species (e.g., Redford and Fonseca, 1986; Johnson et al., 1999; Hannibal and Cáceres, 2010). Although some authors have found similar species richness across habitats in a given locality (Bonvicino et al., 2005; Bezerra et al., 2009; Carmignotto and Aires, 2011), a positive relationship between habitat complexity and richness was mostly observed, with higher values associated to forests (Marinho-Filho et al., 1994; Vieira and Palma, 2005; Godoi et al., 2010; Santos-Filho et al., 2012; Gomes et al., 2015). Because savannas and forests are the best sampled habitats across the Cerrado (Mendonça et al., 2018), one explanation could be related to a sampling bias toward more forest habitat types, with grasslands being neglected in most studies and potentially influencing previous results. In fact, we obtained high richness for both forest and open habitats across sites and localities, indicating that horizontal heterogeneity is as important as vertical stratification for small mammal assemblages, as emphasized for other tropical savannas at both local and landscape scales (Price et al., 2010; Nyirenda et al., 2020).
At localities, sampling methodology and taxonomic resolution might explain the higher richness associated to forests found in previous studies. The survey of a significant portion of species by PTs here, including several open-habitat specialists (e.g., Cryptonanus spp., M. kunsi, and Microakodontomys transitorius), may explain the lower richness found in grasslands and savannas in studies not using this methodology. Also, some open-habitat taxa have been recently reviewed, evidencing sympatric species at the Cerrado (e.g., Cerradomys; Percequillo et al., 2008; Bonvicino et al., 2014a), as well as sympatric and morphologically cryptic species, identified by karyotype and/or molecular data (e.g., Cryptonanus and Oligoryzomys; Weksler et al., 2017; Fegies et al., 2021), revealing a hidden diversity for these groups, and increasing species richness at open habitats.
Moreover, the sampling methodology currently used was designed to capture terrestrial and scansorial species. Thus, similar to the relevance of using intense sampling effort and complementary trap types to effectively characterize the number of species in the Cerrado habitat mosaic (up to 7–11 species per site; Ribeiro and Marinho-Filho, 2005; Bezerra et al., 2009; Carmignotto et al., 2014), sampling the canopy should also increase species richness in forests (Camargo et al., 2018). In fact, arboreal marsupials (e.g., Caluromys lanatus and C. philander) and rodents (e.g., Phyllomys centralis) were not or rarely captured in our study, suggesting that these species are exclusively or mostly captured using traps set in the canopy (Hannibal and Cáceres, 2010; Machado et al., 2018).
Although less studied, fine-scale habitat variables (e.g., grass cover, canopy cover, number of termite mounds, and soil type) might also be related with Cerrado small mammal richness and abundance patterns at local scale (Vieira et al., 2005; Rocha C. R. et al., 2011; Furtado et al., 2021). Vegetation structure was shown to influence savanna vertebrate diversity, especially by altering the availability of food resources, refuge sites, presence of competitors, and/or predators, as well as their dispersal capacity (Kutt and Woinarski, 2007; Price et al., 2010; Sirami and Monadjem, 2012; Ribeiro et al., 2019). Such microhabitat selectivity, not measured in this study, may also contribute to the understanding of the great variation in abundance observed here across sites (2–65 individuals).
Species richness, in a similar manner as found across sites and habitats, overlapped across localities. This is the first study to analyze this variable in a standardized way, evidencing rich small mammal assemblages throughout the Cerrado, reinforcing the high richness previously found in independent surveys at different portions of the biome (12–24 species; e.g., Bonvicino et al., 2005, 2012, 2014b; Cáceres et al., 2007, 2011a; Bezerra et al., 2009; Pereira and Geise, 2009; Carmignotto and Aires, 2011; Rocha R. G. et al., 2011, 2014; Santos-Filho et al., 2012; Carmignotto et al., 2014; Gomes et al., 2015; Carmignotto, 2019; Ribeiro et al., 2020). Long-term inventories comprising the wet and dry seasons typical of the Cerrado may also yield a higher number of individuals and species, especially considering naturally rare species (e.g., 1–4 individuals across localities), and those that present a great variation in abundance between seasons (Bonvicino et al., 2014b; Gomes et al., 2015; Rocha et al., 2017; Ribeiro et al., 2019). In fact, higher sampling effort in the NORTH raised the previous species richness to 23 (Carmignotto and Aires, 2011), and the same was found for NORTHWEST (21 species; Santos-Filho et al., 2012); SOUTHEAST (18 species; Furtado et al., 2021), and CENTRAL (16 species; Carmignotto, 2005). These studies extrapolated the expected richness for these localities, and suggest that richer assemblages, around 18–24 species—as estimated here—are the rule for Cerrado. Similar results were obtained in standardized surveys for other terrestrial vertebrates, such as squamates and anurans (Nogueira et al., 2009; Valdujo et al., 2013), evidencing the high richness across this Neotropical savanna, and highlighting that the Cerrado is still a poorly sampled biome, with a few localities properly surveyed.
As hypothesized, richness at localities was strongly influenced by habitat heterogeneity, as advanced for other tropical savannas (McCleery et al., 2018; Loggins et al., 2019). The high number of habitats here surveyed (5–7) including at least one major habitat type (grasslands, savannas, and forests) represented well both the horizontal and vertical stratification at landscapes, and therefore, assemblages’ species richness. These results point to the importance not only of sampling methodology and capture effort, but also of sampling coverage, where the survey of multiple and distinct habitats plays a major role in species richness completeness (e.g., Nogueira et al., 2009; Price et al., 2010).
The Shannon and Simpson indices, on the other hand, showed that species richness together with evenness varied, with five of the seven localities presenting lower diversity indices, associated with lower species richness and a high number of individuals of few species (e.g., SOUTHEAST characterized by hyper dominance of one species, N. lasiurus). These results are in accordance with the abundance pattern of Cerrado assemblages, composed of a few abundant and several intermediate and rare species (Marinho-Filho et al., 1994; Mares and Ernest, 1995; Carmignotto et al., 2014). In fact, most species were represented by a few individuals, with only N. lasiurus—the most common species recorded in the Cerrado (Mendonça et al., 2018)—and Hylaeamys megacephalus, surpassing 35 individuals across localities. Although local abundance is related to distributional range (Vieira and Palma, 2005; Mendonça et al., 2018), and several locally abundant species in our study are widely distributed in the Cerrado (e.g., Oligoryzomys nigripes, C. scotti, and T. cerradensis), some species with restricted ranges (e.g., T. fosteri, P. roberti, and T. inermis) were also locally common.
Taxonomic representativeness varied across localities, but in general, cricetids dominated, followed by didelphids and echimyid rodents (Vieira and Palma, 2005; Carmignotto et al., 2012; Mendonça et al., 2018). In our study, however, didelphids were an important component of most assemblages both in terms of species richness (19–35%) and abundance (15–48%), suggesting that differences in sampling methodology, especially the use of PTs, uncovered more robust diversity patterns for this group and, consequently, for assemblages. In fact, PTs provided a higher number of individuals and species not only for didelphids but also for some small cricetids (e.g., Microakodontomys, Oligoryzomys, and Calomys) in comparison with LTs, including species exclusively recorded using this method (M. kunsi, three Cryptonanus species, Pseudoryzomys simplex, and Cavia aperea; see also Cáceres et al., 2011b; Ribeiro-Júnior et al., 2011). These results evidence that sampling methodology can also compromise the diversity patterns uncovered for small mammals as a substantial portion of individuals, and species, are exclusively caught by either live or PTs (e.g., Umetsu et al., 2006; Ardente et al., 2017; Bovendorp et al., 2017).
At the tribal level, we observed a strong relationship between landscape context—represented by the most common habitats in each locality—and taxonomic representativeness (see habitat selectivity mentioned below), attesting distinct habitat requirements by small mammal lineages, as hypothesized here and previously documented for birds (Silva, 1995, 1997) and lizards (Nogueira et al., 2009). Such groups represent distantly related lineages (distinct orders, in the case of marsupials and rodents; distinct families in the case of cricetids and echimyids; and even the tribes within each subfamily are not sister lineages) with very distinct biogeographical histories (e.g., Jansa et al., 2014; Steppan and Schenk, 2017; Courcelle et al., 2019; Percequillo et al., 2021), evidencing the importance of phylogenetic constraints shaping Cerrado small mammal current diversity (Carmignotto et al., 2012).
Contributions of α- and β-Diversity Across Scales
Because this is the first study on small mammals to apply standardized sampling across sites, habitats, and localities throughout the Cerrado, one important contribution concerns the critical relevance of species turnover—across scales—in shaping small mammal diversity in this biome. Although Cerrado harbors high α-diversity at sites (α1 = 40%; α3 = 10%) and at localities (α2 = 30%), β-diversity across habitats (β1 = 60%), and particularly across localities (β2 = 70%), and across both habitats and localities (β3 = 90%) represent most part of the diversity through all analyzed scales. Hence, as anticipated, total richness in the Cerrado has a greater contribution from species turnover across habitats and localities than from average species richness within sites.
Ribeiro et al. (2020), partitioning the two components of β-diversity, found that species replacement is much more important than nestedness, reinforcing species turnover and not the net gain or loss of species as a major driver of Cerrado small mammal diversity. A few small mammal studies assessing β-diversity have suggested savannas and forests as the habitats concentrating a higher variation across sites (Marinho-Filho et al., 1994; Vieira and Palma, 2005; Ribeiro et al., 2020). Despite not statistically different, we have also found mean higher richness for forests, evidencing a high species turnover within this habitat type across the Cerrado, mainly represented by restricted-range shared species with the neighboring forest biomes, as observed for plants and other vertebrates (e.g., Silva, 1997; Valdujo et al., 2012; Françoso et al., 2016). However, we also found high and similar richness for grasslands and savannas, stressing the importance of species turnover across these open habitats as well, mostly represented by restricted-range endemic species and species shared with adjacent open biomes (e.g., Nogueira et al., 2011; Valdujo et al., 2013), emphasizing the importance of all three major Cerrado habitat types for small mammals regional species pool.
High species richness obtained by summing the 45 sites in our study (representing ∼11% of the total effort reported for the Cerrado by Mendonça et al., 2018) represents 51% of the total richness obtained by these authors for GF, 47% for typical savanna (“CE”), and 36% for wet grasslands (“CU”), evidencing the spatially concentrated nature of Cerrado inventories performed so far, which did not properly access the high β-diversity, which is proving to be typical of the biome (Nogueira et al., 2011; Valdujo et al., 2013; Françoso et al., 2020; Ribeiro et al., 2020). In fact, 113 native small mammals were recorded along the 446 sites in the review of Mendonça et al. (2018), and 58 species were recorded in our study, reinforcing the distinctiveness among species composition we found across localities. Indeed, biogeographic regions based on differential plant and invertebrate and vertebrate community composition were previously proposed (Silva, 1995; Ratter et al., 1996, 2003; Brown and Gifford, 2002; Azevedo et al., 2016; Françoso et al., 2016, 2020; Amaral et al., 2017), stressing the importance of β-diversity in structuring plant and animal assemblages across the Cerrado.
Community Structure
As expected, a high species turnover across habitats can be associated with the strong habitat selectivity, with most species segregating between forest and open habitats (e.g., Bonvicino et al., 1996, 2005; Lacher and Alho, 2001; Santos-Filho et al., 2012; Ribeiro et al., 2020). Although didelphids have been often associated to forests (Vieira and Palma, 2005; Santos-Filho et al., 2012; Mendonça et al., 2018), we observed genera among marmosines (Monodelphis) and thylamyines (Cryptonanus and Thylamys) strongly associated with savannas and grasslands. This habitat dichotomy was also evident among oryzomyines, with Hylaeamys, Oecomys, Nectomys, and some Oligoryzomys species preferring forests while C. scotti and O. mattogrossae selected open habitats; among phyllotines, with Calomys callosus more frequently captured at forests and savannas, and C. tener at grasslands and savannas; and among echimyids, with Proechimys associated with forests and Thrichomys with open habitats. Akodontines and wiedomyines, on the other hand, preferred open formations. A strong historical component is the most likely explanation to this pattern as habitat preferences are related to the evolutionary history of lineages (Silva, 1997; Azevedo et al., 2016; Fenker et al., 2020). Carmignotto et al. (2012) have already advanced the composite nature of Cerrado endemic mammals, with lineages dispersed and diversified into the Cerrado descending from neighboring forest biomes (Amazonian or Atlantic Forests), where they occupy forests (e.g., niche conservatism, the colonization of similar habitats) or open habitats (e.g., ecological opportunity, the colonization of distinct habitats; see Lv et al., 2016), and those descended from adjacent open biomes (Caatinga, Chaco, or Seasonally Dry Forests) maintaining their specialized open-habitat preferences (e.g., niche conservatism). New data on systematics and biogeography of these lineages demonstrate the complex evolutionary history of Cerrado small mammals, presenting a remarkable heterogeneous diversification across landscapes and time periods, with ancient lineages associated with both forest and open formation ancestors, as well as recent diversification events occurring throughout the open and forest Cerrado habitats (Giarla and Jansa, 2014; Pavan et al., 2016; Courcelle et al., 2019; Fegies et al., 2021; Percequillo et al., 2021; Prado et al., 2021; Saldanha and Rossi, 2021).
Our study revealed that the distance between localities explained, in part, the dissimilarity in composition found across localities (see also Marinho-Filho et al., 1994; Vieira and Palma, 2005; Nogueira et al., 2009; Coelho et al., 2018), while the distance to adjacent biomes better contributed, as hypothesized. The influence of the distance to neighboring biomes in shaping regional species pools was shown to be mostly based on evolutionary constraints as several taxa colonized the Cerrado in areas similar to or nearby their ancestors’ range (e.g., niche conservatism; see Wiens and Graham, 2005), evidencing the importance of the historical component for anurans (Valdujo et al., 2012, 2013), squamates (Nogueira et al., 2009, 2011), birds (Silva, 1996, 1997; Silva and Bates, 2002) and now, small mammal assemblage composition. Although environmental correlates, mainly represented by rainfall gradient and topography, also constitute important determinants for species richness and turnover across savannas (Owen, 2013; Radford et al., 2014; Rugemalila et al., 2016), large-scale environmental variables tested here were not significantly related with composition dissimilarity. This result, contrary to our expectations, was also found by other authors working with Cerrado small mammals, leading them to consider local-scale processes, such as habitat selectivity and limited dispersal, as more important factors for species sorting in the Cerrado (e.g., Ribeiro et al., 2020). Our standardized surveys, however, have shown similar species richness patterns across sites, habitats, and localities, with a greater contribution given by species turnover across habitats, but especially across localities, evidencing the importance of large-scale evolutionary processes on local species composition (see Wiens and Graham, 2005). Given the fact that several organisms with very distinct ecological requirements (e.g., plants, anurans, squamates, and birds) present similar patterns across the Cerrado, we may agree with the authors who suggest the influence of large-scale historical, biogeographical processes, as the main drivers of Cerrado diversity (e.g., Nogueira et al., 2009; Fenker et al., 2020).
Conclusion
Based on standardized surveys, we evidenced that habitat heterogeneity is a major determinant of small mammal species richness at multiple spatial scales here analyzed, pointing to a general pattern across tropical savannas. We also found high β-diversity across habitats and localities, emphasizing a greater contribution of species turnover in structuring small mammal communities across the biome. The replacement of open-area specialists as well as forest dwellers across localities throughout the Cerrado highlights the importance of evolutionary constraints shaping small mammal communities, revealing the complex and distinct history of this Neotropical savanna. Summing to similar results found for other animals and plants at the Cerrado, our study contributes to the understanding of the biogeographic history of the biome, evidencing the need to preserve the habitat mosaic typical of the Cerrado landscapes, as well as different portions of the biome to properly preserve the diversity of small mammals at this Neotropical savanna. Taken the fact that almost 50% of the biome has been lost or converted into agriculture and pasture in the last decades, the continuing rate of landscape change, and a few conservation units across the biome (Beuchle et al., 2015; Françoso et al., 2015; Strassburg et al., 2017; Alencar et al., 2020), it is urgent to implement new conservation units at different portions of the Cerrado.
Inventories based on standardized sampling design and high capture effort, with the use of complementary sampling methodologies in poorly surveyed areas, are highly recommended [e.g., central and eastern Mato Grosso (Brandão et al., 2019); southeastern and western Goiás (Hannibal et al., 2021); central Minas Gerais and eastern Mato Grosso do Sul (Mendonça et al., 2018)], as shown here, to provide robust datasets and increased knowledge on species diversity and distribution in the biome. The collection of vouchers and tissue samples, as well as karyotype data from the surveyed small mammals, is the basis for the description of new taxa (e.g., Bonvicino et al., 2014a; Brandão et al., 2021). Indeed, improvements on the systematics of several genera have contributed with the high richness found in open habitats, as well the high β-diversity found across localities, as previously widely distributed taxa are currently represented by distinct restricted-range species [e.g., former concepts of Oligoryzomys fornesi (Weksler and Bonvicino, 2005, 2015); Oryzomys subflavus (Percequillo et al., 2008); Thrichomys apereoides (Nascimento et al., 2013); and Micoureus demerarae (Silva et al., 2019)]. This diversity will also continue to increase based on recent findings of cryptic lineages in didelphid and cricetid genera (e.g., Suárez-Villota et al., 2018; Fegies et al., 2021; Prado et al., 2021), reinforcing the existence of yet higher taxonomic richness than have been recorded so far in the Cerrado.
Data Availability Statement
The original contributions presented in the study are included in the article/Supplementary Material, further inquiries can be directed to the corresponding author.
Ethics Statement
Ethical review and approval were not required for this animal study because during the time the research was conducted, in the years of 2000–2003, the animal ethics committees were being implemented at the universities, and the current laws regulating this type of research, with wild animals, followed by the committees (CEUAs – Federal law 11.794/2008 and State law 11.977/2009), are not yet in effect.
Author Contributions
AC collected and analyzed the data and wrote the initial draft of the manuscript. All authors helped to design the study, made a critical contribution to the data analysis and manuscript writing and review, and gave final approval for submission.
Funding
This study was funded by FAPESP grants #98/05075-7 BIOTA program to MV and #00/06642-4 to AC; and CNPq research fellowship #311051/2018-9 to RP.
Conflict of Interest
The authors declare that the research was conducted in the absence of any commercial or financial relationships that could be construed as a potential conflict of interest.
Publisher’s Note
All claims expressed in this article are solely those of the authors and do not necessarily represent those of their affiliated organizations, or those of the publisher, the editors and the reviewers. Any product that may be evaluated in this article, or claim that may be made by its manufacturer, is not guaranteed or endorsed by the publisher.
Acknowledgments
This work was only possible with the collaboration of several people, and we thank especially Cristiano Nogueira, who shared with APC the enthusiasm to study the Cerrado terrestrial vertebrates, aid to define the standardized sampling design, the selection of surveyed localities and sites, and of course, in field work, being responsible for all PT transects across field trips, as part of his Ph.D. thesis; Roberta Paresque, who also participated in most of the field trips, as part of her Master dissertation, for her friendship, strength, and joy during field work, and for providing karyotype data for several marsupials and rodents surveyed; Maria José de Jesus Silva and Renata Cecília Amaro, who participated in the NORTHEAST field trip, also contributing with karyological data; Edmara Gonçalves, Renato Gregorin, Rogério Rossi, Felipe Mucci, researchers Talitha M. Pires, Daniel F. Jelin, Karine Abati, the MZUSP employees “Seu” Ageu, Júlio (drivers), and Luis (taxidermist) who have made all field trips easier and more fun; Hussam E. D. Zaher (MZUSP) for inviting APC to participate in field trips to NORTHEAST; all the staff of the Conservation Units visited; IBAMA for approving the small mammal surveys; João Alves de Oliveira, Miguel Trefaut Rodrigues, and Jader Marinho-Filho for their valuable comments during the APC Ph.D. trial.
Supplementary Material
The Supplementary Material for this article can be found online at: https://www.frontiersin.org/articles/10.3389/fevo.2021.739919/full#supplementary-material
References
Ab’Saber, A. N. (1977). Os domínios morfoclimáticos na América do Sul – primeira aproximação. Geomorfologia 52, 1–22.
Alencar, A., Shimbo, J. Z., Lenti, F., Marques, C. B., Zimbres, B., Rosa, M., et al. (2020). Mapping three decades of changes in the Brazilian savanna native vegetation using landsat data processed in the Google Earth engine platform. Remote Sens. 12:924. doi: 10.3390/rs12060924
Alho, C. J. R., Pereira, L. A., and Paula, A. C. (1986). Patterns of habitat utilization by small mammal populations in cerrado biome of central Brazil. Mammalia 50, 447–460. doi: 10.1515/mamm.1986.50.4.447
Alvares, C. A., Stape, J. L., Sentelhas, P. C., Goncalves, J. L. D., and Sparovek, G. (2013). Köppen’s climate classification map for Brazil. Meteorol. Z. 22, 711–728. doi: 10.1127/0941-2948/2013/0507
Amaral, A. G., Munhoz, C. B., Walter, B. M., Aguirre-Gutiérrez, J., and Raes, N. (2017). Richness pattern and phytogeography of the Cerrado herb–shrub flora and implications for conservation. J. Veg. Sci. 28, 848–858. doi: 10.1111/jvs.12541
Andersen, A. N. (2020). Faunal responses to fire in Australian tropical savannas: Insights from field experiments and their lessons for conservation management. Divers. Distrib. 27, 828–843. doi: 10.1111/ddi.13198
Ardente, N. C., Ferreguetti, A. C., Gettinger, D., Leal, P., Martins-Hatano, F., and Bergallo, H. G. (2017). Differencial efficiency of two sampling methods in capturing non-volant small mammals in an area in eastern Amazonia. Acta Amaz. 47, 123–132. doi: 10.1590/1809-4392201602132
Azevedo, J. A. R., Valdujo, P. H., and de Nogueira, C. C. (2016). Biogeography of anurans and squamates in the Cerrado hotspot: coincident endemism patterns in the richest and most impacted savanna on the globe. J. Biogeogr. 43, 2454–2464. doi: 10.1111/jbi.12803
Batalha, M. A., and Martins, F. R. (2007). The vascular flora of the cerrado in Emas National Park (Central Brazil): a savanna flora summarized. Braz. Arch. Biol. Tech. 50, 269–277. doi: 10.1590/S1516-89132007000200012
Beuchle, R., Grecchi, R. C., Shimabukuro, Y. E., Seliger, R., Eva, H. D., Sano, E., et al. (2015). Land cover changes in the Brazilian Cerrado and Caatinga biomes from 1990 to 2010 based on a systematic remote sensing sampling approach. Appl. Geogr. 58, 116–127. doi: 10.1016/j.apgeog.2015.01.017
Bezerra, A. M. R., Carmignotto, A. P., and Rodrigues, F. H. G. (2009). Small non-volant mammals of an ecotone region between the Cerrado hotspot and the Amazonian rainforest, with comments on their taxonomy and distribution. Zool. Stud. 48, 861–874.
Bond, W. J., Smythe, K.-A., and Balfour, D. A. (2001). Acacia species turnover in space and time in an African savanna. J. Biogeogr. 28, 117–128. doi: 10.1046/j.1365-2699.2001.00506.x
Bonvicino, C. R., Casado, F., and Weksler, M. (2014a). A new species of Cerradomys (Mammalia: Rodentia: Cricetidae) from Central Brazil, with remarks on the taxonomy of the genus. Zoology 31, 525–540. doi: 10.1590/S1984-46702014000600002
Bonvicino, C. R., Cerqueira, R., and Soares, V. A. (1996). Habitat use by small mammals of upper Araguaia river. Rev. Bras. Biol. 56, 761–767.
Bonvicino, C. R., Lazar, A., Corrêa, M. M. O., Weksler, M., Paula, A. C., and Bezerra, A. M. R. (2014b). Conservation units in the core area of the Cerrado domain: an overview on the small nonvolant mammals (Rodentia and Didelphimorphia). Hering. 8, 202–221.
Bonvicino, C. R., Lemos, B., and Weksler, M. (2005). Small mammals of Chapada dos Veadeiros National Park (Cerrado of Central Brazil): ecologic, karyologic, and taxonomic considerations. Rev. Bras. Biol. 65, 395–406. doi: 10.1590/S1519-69842005000300004
Bonvicino, C. R., Lindbergh, S. M., Faria, M. B., and Bezerra, A. M. R. (2012). The eastern boundary of the Brazilian Cerrado: a hotspot region. Zool. Stud. 51, 1207–1218.
Bovendorp, R. S., MCCleery, R. A., and Galetti, M. (2017). Optimising sampling methods for small mammal communities in Neotropical rainforests. Mamm. Rev. 47, 148–158. doi: 10.1111/mam.12088
Brandão, M. V., Garbino, G. S. T., Semedo, T. B. F., Feijó, A., Nascimento, F. O., Fernandes-Ferreira, H., et al. (2019). Mammals of Mato Grosso, Brazil: annotated species list and historical review. Mastozool. Neotrop. 26, 263–307. doi: 10.31687/saremMN.19.26.2.0.03
Brandão, M. V., Percequillo, A. R., D’Elía, G., Paresque, R., and Carmignotto, A. P. (2021). A new species of Akodon Meyen, 1833 (Rodentia: Cricetidae: Sigmodontinae) endemic from the Brazilian Cerrado. J. Mammal. 102, 101–122. doi: 10.1093/jmammal/gyaa126
Brown, K. Jr., and Gifford, D. R. (2002). “Lepidoptera in the Cerrado landscape and the conservation of vegetation, soil, and topographical mosaics,” in The Cerrados of Brazil, eds P. S. Oliveira and R. J. Marquis (New York, NY: Columbia University Press), 201–222. doi: 10.7312/oliv12042-010
Cáceres, N. C., Bornschein, M. R., Lopes, W. H., and Percequillo, A. R. (2007). Mammals of the Bodoquena Mountains, southwestern Brazil: an ecological and conservation analysis. Rev. Bras. Zool. 24, 426–435. doi: 10.1590/S0101-81752007000200021
Cáceres, N. C., Godoi, M. N., Hannibal, W., and Ferreira, V. L. (2011a). Effects of altitude and vegetation on small-mammal distribution in the Urucum Mountains, western Brazil. J. Trop. Ecol. 27, 279–287. doi: 10.1017/S0266467410000854
Cáceres, N. C., Nápoli, R. P., and Hannibal, W. (2011b). Differential trapping success for small mammals using pitfall and standard cage traps in a woodland savannah region of southwestern Brazil. Mammalia 75, 45–52. doi: 10.1515/mamm.2010.069
Cáceres, N. C., Nápoli, R. P., Casella, J., and Hannibal, W. (2010). Mammals in a fragmented savannah landscape in south-western Brazil. J. Nat. Hist. 44, 491–512. doi: 10.1080/00222930903477768
Camargo, N. F., Sano, N. Y., and Vieira, E. M. (2018). Forest vertical complexity affects alpha and beta diversity of small mammals. J Mammal. 99, 1444–1454. doi: 10.1093/jmammal/gyy136
Campos, R. I., Vasconcelos, H. L., Andersen, A. N., Frizzo, T. L. M., and Spena, K. C. (2011). Multi-scale ant diversity in savanna woodlands: an intercontinental comparison. Austral Ecol. 36, 983–992. doi: 10.1111/j.1442-9993.2011.02255.x
Carmignotto, A. P. (2005). Pequenos Mamíferos Terrestres Do Bioma Cerrado: Padrões Faunísticos Locais E Regionais. Ph.D. thesis. São Paulo: Universidade de São Paulo.
Carmignotto, A. P. (2019). Effects of damming on a small mammal assemblage in Central Brazilian Cerrado. Bol. Soc. Bras. Mastozool. 85, 63–73.
Carmignotto, A. P., and Aires, C. C. (2011). Mamíferos não voadores (Mammalia) da estação ecológica serra geral do tocantins. Biota Neotrop. 11, 307–322. doi: 10.1590/S1676-06032011000100029
Carmignotto, A. P., Bezerra, A. M. R., and Rodrigues, F. H. G. (2014). Nonvolant small mammals from a southwestern area of Brazilian Cerrado: diversity, habitat use, seasonality, and biogeography. Therya 5, 535–558. doi: 10.12933/therya-14-197
Carmignotto, A. P., de Vivo, M., and Langguth, A. (2012). “Mammals of the cerrado and caatinga: distribution patterns of the tropical open biomes of central South America,” in Bones, Clones, And Biomes: The History And Geography Of Recent Neotropical Mammals, eds B. D. Patterson and L. P. Costa (Chicago, IL: University of Chicago Press), 307–350.
Carvalho, W. D., Meyer, C. F. J., Xavier, B. S., Mustin, K., Castro, I. J., Silvestre, S. M., et al. (2020). Consequences of replacing native savannahs with Acacia plantations for the taxonomic, functional, and phylogenetic α- and β-diversity of bats in the northern Brazilian Amazon. Front. Ecol. Evol. 8:609214. doi: 10.3389/fevo.2020.609214
Chao, A., and Chiu, C.-H. (2016). “Species richness: estimation and comparison,” in Wiley StatsRef: Statistics Reference Online, eds Balakrishnan, N., Colton, T., Everitt, B., Piegorsch, W., F. Ruggeri and Teugels, J. L. (Hoboken, NJ: JohnWiley & Sons, Ltd), 1–26. doi: 10.1002/9781118445112.stat03432.pub2
Chao, A., and Jost, L. (2012). Coverage-based rarefaction and extrapolation: standardizing samples by completeness rather than size. Ecology 93, 2533–2547. doi: 10.1890/11-1952.1
Chao, A., Chiu, C.-H., and Hsieh, T. C. (2012). Proposing a resolution to debates on diversity partitioning. Ecology 93, 2037–2051. doi: 10.1890/11-1817.1
Chao, A., Gotelli, N. J., Hsieh, T., Sander, E. L., Ma, K., Colwell, R. K., et al. (2014). Rarefaction and extrapolation with Hill numbers: a framework for sampling and estimation in species diversity studies. Ecol. Monogr. 84, 45–67. doi: 10.1890/13-0133.1
Chiu, C.-H., and Chao, A. (2014). Distance-based functional diversity measures and their decomposition: a framework based on Hill numbers. PLoS One 9:e113561. doi: 10.1371/journal.pone.0100014
Clarke, K. (1993). Non-parametric multivariate analyses of changes in community structure. Austral Ecol. 18, 117–143. doi: 10.1111/j.1442-9993.1993.tb00438.x
Coelho, M. S., Carneiro, M. A. A., Branco, C. A., Borges, R. A. X., and Fernandes, G. W. (2018). Species turnover drives β-diversity patterns across multiple spatial scales of plant-galling interactions in mountaintop grasslands. PLoS One 13:e0195565. doi: 10.1371/journal.pone.0195565
Colli, G. R., Bastos, R. P., and Araújo, A. F. B. (2002). “The character and dynamics of the Cerrado herpetofauna,” in The Cerrados of Brazil, eds P. S. Oliveira and R. J. Marquis (New York, NY: Columbia University Press), 223–241. doi: 10.7312/oliv12042-011
Colli, G. R., Vieira, C. R., and Dianese, J. C. (2020). Biodiversity and conservation of the Cerrado: recent advances and old challenges. Biodivers. Conserv. 29, 1465–1475. doi: 10.1007/s10531-020-01967-x
Colwell, R. K., Chao, A., Gotelli, N. J., Lin, S.-Y., Mao, C. X., Chazdon, R. L., et al. (2012). Models and estimators linking individual-based and sample-based rarefaction, extrapolation and comparison of assemblages. J. Plant Ecol. 5, 3–21. doi: 10.1093/jpe/rtr044
Courcelle, M., Tilak, M.-K., Leite, Y. L. R., Douzery, E. J. P., and Fabre, P.-H. (2019). Digging for the spiny rat and hutia phylogeny using a gene capture approach, with the description of a new mammal subfamily. Mol. Phylogenet. Evol. 136, 241–253. doi: 10.1016/j.ympev.2019.03.007
Coutinho, L. M. (2006). O conceito de bioma. Acta Bot. Bras. 20, 13–23. doi: 10.1590/S0102-33062006000100002
Dinerstein, E., Olson, D., Joshi, A., Vynne, C., Burgess, N. D., Wikramanayake, E., et al. (2017). An ecoregion-based approach to protecting half the terrestrial realm. BioScience 67, 534–545. doi: 10.1093/biosci/bix014
Dudley, N., Eufemia, L., Fleckenstein, M., Periago, M. E., Petersen, I., and Timmers, J. F. (2020). Grasslands and savannahs in the UN decade on ecosystem restoration. Restor. Ecol. 28, 1313–1317. doi: 10.1111/rec.13272
Environmental Systems Research Institute [ESRI] Inc (2012). ArcMap [GIS software]. Version 10.1. Redlands, CA: ESRI.
Fegies, A. C., Carmignotto, A. P., Perez, M. F., Guilardi, M. D., and Lessinger, A. C. (2021). Molecular phylogeny of Cryptonanus (Didelphidae: Thylamyini): evidence for a recent and complex diversification in South American open biomes. Mol. Phylogenet. Evol. 162, 107213. doi: 10.1016/j.ympev.2021.107213
Fenker, J., Domingos, F. M. C. B., Tedeschi, L. G., Rosauer, D. F., Werneck, F. P., Colli, G. R., et al. (2020). Evolutionary history of Neotropical savannas geographically concentrates species, phylogenetic and functional diversity of lizards. J. Biogeogr. 47, 1130–1142. doi: 10.1111/jbi.13800
Françoso, R. D., Brandão, R., Nogueira, C. C., Salmona, Y. B., Machado, R. B., and Colli, G. R. (2015). Habitat loss and the effectiveness of protected areas in the Cerrado Biodiversity Hotspot. Nat. Conserv. 13, 35–40. doi: 10.1016/j.ncon.2015.04.001
Françoso, R. D., Dexter, K. G., Machado, R. B., Pennington, R. T., Pinto, J. R. R., Brandão, R. A., et al. (2020). Delimiting floristic biogeographic districts in the Cerrado and assessing their conservation status. Biodivers. Conserv. 29, 1477–1500. doi: 10.1007/s10531-019-01819-3
Françoso, R. D., Haidar, R. F., and Machado, R. B. (2016). Tree species of South America central savanna: endemism, marginal areas and the relationship with other biomes. Acta Bot. Bras. 30, 1–9. doi: 10.1590/0102-33062015abb0244
Furley, P. A. (1999). The nature and diversity of Neotropical savanna vegetation with particular reference to the Brazilian Cerrados. Glob. Ecol. Biogeogr. 8, 223–241. doi: 10.1046/j.1466-822X.1999.00142.x
Furley, P. A. (2006). Tropical savannas. Progr. Phys. Geogr. 30, 105–121. doi: 10.1191/0309133306pp474pr
Furley, P. A., and Ratter, J. A. (1988). Soil resources and plant communities of the central Brazilian Cerrado and their development. J. Biogeogr. 15, 97–108. doi: 10.2307/2845050
Furtado, L. O., Felício, G. R., Lemos, P. R., Christianini, A. V., Martins, M., and Carmignotto, A. P. (2021). Winners and losers: how woody encroachment is changing the small mammal community structure in a Neotropical savanna. Front. Ecol. Evol. 9:774744. doi: 10.3389/fevo.2021.774744
Gianuca, A., Declerck, S., Lemmens, P., and De Meester, L. (2017). Effects of dispersal and environmental heterogeneity on the replacement and nestedness components of β -diversity. Ecology 98, 525–533. doi: 10.1002/ecy.1666
Giarla, T. C., and Jansa, S. A. (2014). The role of physical geography and habitat type in shaping the biogeographical history of a recent radiation of Neotropical marsupials (Thylamys: Didelphidae). J. Biogeogr. 41, 1547–1558. doi: 10.1111/jbi.12320
Godoi, M. N., Cunha, N. L., and Cáceres, N. C. (2010). Efeito do gradiente floresta-cerrado-campo sobre a comunidade de pequenos mamíferos do Alto do Maciço do Urucum, oeste do Brasil. Mastozool. Neotrop. 17, 263–277.
Gomes, L. P., Rocha, C. R., Brandão, R. A., and Marinho-Filho, J. (2015). Mammal richness and diversity in Serra do Facão region, southeastern Goiás state, central Brazil. Biota Neotrop. 15:4. doi: 10.1590/1676-0611-BN-2015-0033
Hammer, Ø, Harper, D., and Ryan, P. (2008). PAST: paleontological statistics software package for education and data analysis. Paleontol. Eletron. 4, 1–9.
Hannibal, W., and Cáceres, N. C. (2010). Use of vertical space by small mammals in gallery forest and woodland savannah in south-western Brazil. Mammalia 74, 247–255. doi: 10.1515/mamm.2010.007
Hannibal, W., Zortéa, M., Calaça, A. M., Carmignotto, A. P., Bezerra, A. M. R., Carvalho, H. G., et al. (2021). Checklist of mammals from Goiás, central Brazil. Biota Neotrop. 21:e20201173. doi: 10.1590/1676-0611-BN-2020-1173
Hill, M. O. (1973). Diversity and evenness: a unifying notation and its consequences. Ecology 54, 427–432. doi: 10.2307/1934352
Hsieh, T. C., Ma, K. H., and Chao, A. (2016). iNEXT: an R package for rarefaction and extrapolation of species diversity (Hill numbers). Meth. Ecol. Evol. 7, 1451–1456. doi: 10.1111/2041-210X.12613
Hueck, K., and Seibert, P. (1981). Vegetationskarte Von Südamerika. Stuttgart: Gustav Fischer Verlag.
Hurtado, N., and Pacheco, V. (2017). Revision of Neacomys spinosus (Thomas, 1882) (Rodentia: Cricetidae) with emphasis on Peruvian populations and the description of a new species. Zootaxa 4242, 401–440. doi: 10.11646/zootaxa.4242.3.1
Jansa, S. A., Barker, F. K., and Voss, R. S. (2014). The early diversification history of didelphid marsupials: a window into South America’s “Splendid Isolation”. Evolution 68, 684–695. doi: 10.1111/evo.12290
Jetz, W., and Fine, P. V. A. (2012). Global Gradients in Vertebrate Diversity Predicted by Historical Area-Productivity Dynamics and Contemporary Environment. PLoS Biol. 10:e1001292. doi: 10.1371/journal.pbio.1001292
Johnson, M. A., Saraiva, P. M., and Coelho, D. (1999). The role of gallery forests in the distribution of Cerrado mammals. Rev. Bras. Biol. 59, 421–427. doi: 10.1590/S0034-71081999000300006
Kutt, A., and Woinarski, J. (2007). The effects of grazing and fire on vegetation and the vertebrate assemblage in tropical savanna woodland in north-eastern Australia. J. Trop. Ecol. 23, 95–106. doi: 10.1017/S0266467406003579
Lacher, T. E. Jr., and Alho, C. J. R. (2001). Terrestrial small mammal richness and habitat associations in an Amazon Forest-Cerrado contact zone. Biotropica 33, 171–181. doi: 10.1111/j.1744-7429.2001.tb00166.x
Leemans, R., and Cramer, W. P. (1991). “The IIASA database for mean monthly values of temperature, precipitation, and cloudiness on a global terrestrial grid. RR-91-18,” in Proceedings of the International Institute for Applied Systems Analysis, Laxenburg.
Linzey, A. V., and Kesner, M. D. (1997). Small mammals of a woodland-savannah ecosystem in Zimbabwe. I. Density and habitat occupancy patterns. J. Zool. 243, 137–152. doi: 10.1111/j.1469-7998.1997.tb05760.x
Loggins, A., Monadjem, A., Kruger, L., Reichert, B., and McCleery, R. (2019). Vegetation structure shapes small mammal communities in African savannas. J. Mammal. 100, 1243–1252. doi: 10.1093/jmammal/gyz100
Lomolino, M. V., Riddle, B. R., and Brown, J. H. (2006). Biogeography, 3rd Edn. Sunderland: Sinauer Associates, Inc.
Loreau, M., and Hector, A. (2001). Partitioning selection and complementarity in biodiversity experiments. Nature 412, 72–76. doi: 10.1038/35083573
Ludwig, J. A., and Reynolds, J. F. (1988). Statistical Ecology: A Primer On Methods And Computing. New York, NY: John Wiley & Sons.
Lv, X., Xia, L., Ge, D., Wu, Y., and Yang, Q. (2016). Climatic niche conservatism and ecological opportunity in the explosive radiation of arvicoline rodents (Arvicolinae, Cricetidae). Evolution 70, 1094–1104. doi: 10.1111/evo.12919
Machado, L. F., Loss, A. C., Paz, A., Vieira, E. M., Rodrigues, F. P., and Marinho-Filho, J. (2018). Phylogeny and biogeography of Phyllomys (Rodentia: Echimyidae) reveal a new species from the Cerrado and suggest Miocene connections of the Amazon and Atlantic Forest. J. Mammal. 99, 377–396. doi: 10.1093/jmammal/gyy015
Maestri, R., and Patterson, B. D. (2016). Patterns of species richness and turnover for the South American rodent fauna. PLoS One 11:e0151895. doi: 10.1371/journal.pone.0151895
Mares, M. A., and Ernest, K. A. (1995). Population and community ecology of small mammals in a gallery forest of central Brazil. J. Mammal. 76, 750–768. doi: 10.2307/1382745
Marinho-Filho, J., Reis, M. L., Oliveira, P. S., Vieira, E. M., and Paes, M. N. (1994). Diversity standards and small mammal numbers: conservation of the Cerrado biodiversity. An. Acad. Bras. Ciênc. 66, 149–157.
McCleery, R. M., Monadjem, A., Baiser, B., Fletcher, R., Vickers, K., and Kruger, L. (2018). Animal diversity declines with broad-scale homogenization of canopy cover in African savannas. Biol. Conserv. 226, 54–62. doi: 10.1016/j.biocon.2018.07.020
McCune, B., and Allen, T. F. H. (1985). Will similar forests develop on similar sites? Can. J. Bot. 63, 367–376. doi: 10.1139/b85-043
McKnight, M., White, P., Mcdonald, R., Lamoreux, J., Sechrest, W., Ridgely, R., et al. (2007). Putting beta-diversity on the map: broad-scale congruence and coincidence in the extremes. PLoS Biol. 5:e272. doi: 10.1371/journal.pbio.0050272
McNaughton, S. J., Sala, O. E., and Oesterheld, M. (1993). “Comparative ecology of African and South American arid to subhumid ecosystems,” in Biological Relationships Between African And South America, ed. P. Goldblatt (New Haven, CT: Yale University Press), 548–567. doi: 10.2307/j.ctt22726mc.22
Melo, A. S., Rangel, T. F. L., and Diniz-Filho, J. A. F. (2009). Environmental drivers of beta-diversity patterns in New World birds and mammals. Ecography 32, 226–236. doi: 10.1111/j.1600-0587.2008.05502.x
Mendonça, A., Percequillo, A. R., Camargo, N. F., Ribeiro, J. F., Palma, A. R. T., Oliveira, L. C., et al. (2018). CERRADO SMALL MAMMALS: abundance and distribution of marsupials, lagomorphs, and rodents in a Neotropical savanna. Ecology 99:1900. doi: 10.1002/ecy.2367
Mishra, N. B., and Young, K. R. (2020). “Savannas and grasslands,” in Terrestrial Ecosystems and Biodiversity, 1st Edn, ed. Y. Wang (Boca Raton, FL: CRC Press), 235–247. doi: 10.1201/9780429445651
Morales-Martinez, D., Rodríguez-Posada, M. E., Fernández-Rodríguez, C., Calderón-Capote, M., and Gutierrez, D. (2018). Spatial variation of bat diversity between three foodplain-savanna ecosystems of the Colombian Llanos. Therya 9, 41–52. doi: 10.12933/therya-18-537
Moreno, C. E., Calderón-Patrón, J. M., Martín-Regalado, N., Martínez-Falcón, A. P., Ortega-Martínez, I. J., Rios-Díaz, C. L., et al. (2018). Measuring species diversity in the tropics: a review of methodological approaches and framework for future studies. Biotropica 50, 929–941. doi: 10.1111/btp.12607
Myers, N. (2003). Biodiversity hotspots revisited. Bioscience 53, 916–917. doi: 10.1641/0006-3568(2003)053[0916:BHR]2.0.CO;2
Myers, N., Mittermeier, R. A., Mittermeier, C. G., da Fonseca, G. A. B., and Kent, J. (2000). Biodiversity hotspots for conservation priorities. Nature 403, 853–858. doi: 10.1038/35002501
Nascimento, F. F., Lazar, A., Menezes, A. N., Durans, A. M., Moreira, J. C., Salazar-Bravo, J., et al. (2013). The role of historical barriers in the diversification processes in open vegetation formations during the Miocene/Pliocene using an ancient rodent lineage as a model. PLoS One 8:e61924. doi: 10.1371/journal.pone.0061924
Nekola, J. C., and White, P. S. (1999). The distance decay of similarity in biogeography and ecology. J. Biogeogr. 26, 867–878. doi: 10.1046/j.1365-2699.1999.00305.x
Nimer, E. (1989). Climatologia Do Brasil. Rio de Janeiro: Instituto Brasileiro de Geografia e Estatística.
Nogueira, C., Colli, G. R., and Martins, M. (2009). Local richness and distribution of the lizard fauna in natural habitat mosaics of the Brazilian Cerrado. Austral Ecol. 34, 83–96. doi: 10.1111/j.1442-9993.2008.01887.x
Nogueira, C., Ribeiro, S., Costa, G. C., and Colli, G. R. (2011). Vicariance and endemism in a Neotropical savanna hotspot: distribution patterns of Cerrado squamate reptiles. J. Biogeogr. 38, 1907–1922. doi: 10.1111/j.1365-2699.2011.02538.x
Nyirenda, V. R., Namukonde, N., Simwanda, M., Phiri, D., Murayama, Y., Ranagalage, M., et al. (2020). Rodent assemblages in the mosaic of habitat types in the zambezian bioregion. Diversity 12:365. doi: 10.3390/d12100365
Oksanen, J., Blanchet, F. G., Friendly, M., Kindt, R., Legendre, P., McGlinn, D., et al. (2020). Package ‘vegan’ Community Ecology Package. R Package Version 2.5-7. Available online at: https://CRAN.R-project.org/package=vegan (accessed November 28, 2020).
Oliveira, P. S., and Marquis, R. J. (2002). The Cerrados of Brazil: Ecology And Natural History Of A Neotropical Savanna. New York, NY: Columbia University Press. doi: 10.7312/oliv12042
Oliveira-Filho, A. T., and Ratter, J. A. (2002). “Vegetation physiognomies and woody flora of the Cerrado biome,” in The Cerrados of Brazil: Ecology And Natural History Of A Neotropical Savanna, eds P. S. Oliveira and R. J. Marquis (New York, NY: Columbia University Press), 91–120. doi: 10.7312/oliv12042-005
Owen, R. D. (2013). Ecology of small terrestrial mammals in an isolated Cerrado patch, eastern Paraguay: communities, species, and effects of enso, precipitation, and fire. Mastozool. Neotrop. 20, 97–112.
Parada, J. M., and Andrade, S. M. (1977). “Cerrados: recursos minerais,” in IV Simpósio sobre o Cerrado: Bases Para Utilização Agropecuária, ed. M. G. Ferri (São Paulo: Editora da Universidade de São Paulo, EDUSP), 195–209.
Patton, J. L., Pardinas, U. F. J., and D’Elia, G. (2015). Mammals of South America, Volume 2, Rodents. Chicago, IL: The University of Chicago Press, doi: 10.7208/chicago/9780226169606.001.0001
Pavan, S. E., Jansa, S. A., and Voss, R. S. (2016). Spatiotemporal diversification of a low-vagility Neotropical vertebrate clade (short-tailed opossums, Didelphidae: Monodelphis). J. Biogeogr. 43, 1299–1309. doi: 10.1111/jbi.12724
Pennington, R. T., Lehmann, C. E. R., and Rowland, L. M. (2018). Tropical savannas and dry forests. Curr. Biol. 28, R527–R548. doi: 10.1016/j.cub.2018.03.014
Percequillo, A. R., Hingst-Zaher, E., and Bonvicino, C. R. (2008). Systematic review of genus Cerradomys Weksler, Percequillo and Voss, 2006 (Rodentia: Cricetidae: Sigmodontinae: Oryzomyini), with description of two new species from eastern Brazil. Am. Mus. Nov. 3622, 1–46. doi: 10.1206/495.1
Percequillo, A. R., Prado, J. R., Abreu, E. F., Dalapicolla, J., Pavan, A. C., Chiquito, E. A., et al. (2021). Tempo and mode of evolution of oryzomyine rodents (Rodentia, Cricetidae, Sigmodontinae): a phylogenomic approach. Mol. Phylogenet. Evol. 159:107120. doi: 10.1016/j.ympev.2021.107120
Pereira, L. G., and Geise, L. (2009). Non-flying mammals of Chapada Diamantina (Bahia, Brazil). Biota Neotrop. 9, 185–196. doi: 10.1590/S1676-06032009000300019
Prado, D. E., and Gibbs, P. E. (1993). Patterns of species distributions in the dry seasonal forests of South America. Ann. Missouri Bot. Garden 80, 902–927. doi: 10.2307/2399937
Prado, J. R., Knowles, L. L., and Percequillo, A. R. (2021). New species boundaries and the diversification history of marsh rat taxa clarify historical connections among ecologically and geographically distinct wetlands of South America. Mol. Phylogenet. Evol. 155:106992. doi: 10.1016/j.ympev.2020.106992
Price, B., Kutt, A., and Mcalpine, C. (2010). The importance of fine-scale savanna heterogeneity for reptiles and small mammals. Biol. Conserv. 143, 2504–2513. doi: 10.1016/j.biocon.2010.06.017
Pringle, R., Prior, K., Palmer, T., Young, T., and Goheen, J. (2016). Large herbivores promote habitat specialization and beta diversity of African savanna trees. Ecology 97, 2640–2657. doi: 10.1002/ecy.1522
RADAMBRASIL (1982a). Projeto RadamBrasil Levantamento De Recursos Naturais. Folha. SD. 21, Cuiabá, Vol. 26. Rio de Janeiro: Ministério das Minas e Energia, DNPM.
RADAMBRASIL (1982b). Projeto RadamBrasil Levantamento De Recursos Naturais. Folha SD. 23, Brasília, Vol. 29. Rio de Janeiro: Ministério das Minas e Energia, DNPM.
RADAMBRASIL (1982c). Projeto RadamBrasil Levantamento De Recursos Naturais. Folha SF. 21, Campo Grande, Vol. 28. Rio de Janeiro: Ministério das Minas e Energia, DNPM.
Radford, I. J., Dickman, C. R., Start, A. N., Palmer, C., Carnes, K., Carnes, K., et al. (2014). Mammals of australia’s tropical savannas: a conceptual model of assemblage structure and regulatory factors in the kimberley region. PLoS One 9:e92341. doi: 10.1371/journal.pone.0092341
Ratter, J. A., Bridgewater, S., and Ribeiro, J. F. (2003). Analysis of the floristic composition of the Brazilian cerrado vegetation III: comparison of the woody vegetation of 376 areas. Edinburgh J. Bot 60, 57–109. doi: 10.1017/S0960428603000064
Ratter, J. A., Bridgewater, S., Atkinson, R., and Ribeiro, J. F. (1996). Analysis of the floristic composition of the Brazilian Cerrado vegetation II: comparison of the woody vegetation of 98 areas. Edinburgh J. Bot. 53, 153–180. doi: 10.1017/S0960428600002821
Redford, K. H., and Fonseca, G. A. B. (1986). The role of gallery forests in the zoogeography of the Cerrado’s non-volant mammalian fauna. Biotropica 18, 126–135. doi: 10.2307/2388755
Ribeiro, J. F., and Walter, B. M. T. (1998). “Fitofisionomias do bioma Cerrado,” in Cerrado: Ambiente E Flora, eds S. M. Sano and S. P. Almeida (Planaltina, GO: EMBRAPA, CPAC), 89–166.
Ribeiro, J. F., Guaraldo, A., Nardoto, G. B., Santoro, G., and Vieira, E. M. (2019). Habitat type and seasonality influence the isotopic trophic niche of small mammals in a neotropical savanna. Hystrix 30, 30–38. doi: 10.4404/hystrix-00150-2018
Ribeiro, M. C. L. B., Britski, H., Ramos, A., Figueiredo, A., Silva, C. J., Fonseca, C. P., et al. (2007). “Biota Aquática,” in Biodiversidade Do Cerrado E Pantanal: Áreas E Ações Prioritárias Para Conservação, ed. R. B. Cavalcanti (Brasília, DF: Ministério do Meio Ambiente, Biodiversidade 17), 193–256.
Ribeiro, R., and Marinho-Filho, J. (2005). Estrutura da comunidade de pequenos mamíferos (Mammalia, Rodentia) da estação ecológica de águas emendadas, planaltina, distrito federal, Brasil. Rev. Bras. Zool. 22, 898–907. doi: 10.1590/S0101-81752005000400014
Ribeiro, R., Ricklefs, R. E., and Marinho-Filho, J. (2020). Partitioning beta diversity to unravel mechanisms underlying the distributions of nonvolant small mammls in Brazil’s Cerrado. J. Mammal. 101, 1438–1450. doi: 10.1093/jmammal/gyaa085
Ribeiro-Júnior, M. A., Rossi, R. V., Miranda, C. L., and Ávila-Pires, T. C. S. (2011). Influence of pitfall trap size and design on herpetofauna and small mammal studies in a Neotropical forest. Zoologia 28, 80–91. doi: 10.1590/S1984-46702011000100012
Rocha, C. R., Ribeiro, R., and Marinho-Filho, J. (2017). Influence of temporal variation and seasonality on population dynamics of three sympatric rodents. Mammal. Biol. 84, 20–29. doi: 10.1016/j.mambio.2017.01.001
Rocha, C. R., Ribeiro, R., Takahashi, F. S. C., and Marinho-Filho, J. (2011). Microhabitat use by rodent species in a central Brazilian cerrado. Mamm. Biol. 76, 651–653. doi: 10.1016/j.mambio.2011.06.006
Rocha, R. G., Ferreira, E., Costa, B. M. A., Martins, I. C. M., Leite, Y. L. R., Costa, L. P., et al. (2011). Small mammals of the mid-Araguaia river in central Brazil, with the description of a new species of climbing rat. Zootaxa 2789, 1–34. doi: 10.11646/zootaxa.2789.1.1
Rocha, R. G., Ferreira, E., Martins, I. C. M., Costa, L. P., and Fonseca, C. (2014). Seasonally flooded stepping stones: emerging diversity of small mammal assemblage in the Amazonia-Cerrado ecotone, central Brazil. Zool. Stud. 53:60. doi: 10.1186/s40555-014-0060-0
Rosenzweig, M. L. (1995). Species Diversity in Space and Time. Cambridge: Cambridge University Press, 436.
Rugemalila, D., Anderson, T., and Holdo, R. (2016). Precipitation and Elephants, Not Fire, Shape Tree Community Composition in Serengeti National Park, Tanzania. Biotropica 48, 476–482. doi: 10.1111/btp.12311
Ryan, C. M., Pritchard, R., McNichol, I., Owen, M., Fisher, J. A., and Lehmann, C. (2016). Ecosystem services from southern African woodlands and their future under global change. Phil. Trans. R. Soc. 371:20150312. doi: 10.1098/rstb.2015.0312
Saldanha, J., and Rossi, R. V. (2021). Integrative analysis supports a new species of the Oecomys catherinae complex (Rodentia, Cricetidae) from Amazonia. J. Mammal. 102, 69–89. doi: 10.1093/jmammal/gyaa145
Santos-Filho, M., Frieiro-Costa, F., Ára, I., and Silva, M. N. F. (2012). Use of habitats by non-volant small mammals in Cerrado in Central Brazil. Braz. J. Biol. 72, 893–902. doi: 10.1590/S1519-69842012000500016
Schmidt, F. A., Ribas, C. R., Sobrinho, T. G., Ubaidillah, R., Schoereder, J. H., Clough, Y., et al. (2017). Similar alpha and beta diversity changes in tropical ant communities, comparing savannas and rainforests in Brazil and Indonesia. Oecology 185, 487–498. doi: 10.1007/s00442-017-3960-y
Schoeman, M. C., and Monadjem, A. (2018). Community structure of bats in the savannas of southern Africa: influence of scale and human land use. Hystrix 29, 3–10. doi: 10.4404/hystrix-00038-2017
Scholes, R. J., and Archer, S. R. (1997). Tree-grass interactions in savannas. Ann. Rev. Ecol. Syst. 28, 517–544. doi: 10.1146/annurev.ecolsys.28.1.517
Sikes, R. S., and The Animal Care and Use Committee of the American Society of Mammalogists (2016). 2016 guidelines of the american society of mammalogists for the use of wild mammals in research and education. J. Mammal. 97, 663–688. doi: 10.1093/jmammal/gyw078
Silva, J. M. C. (1995). Biogeographic analysis of the South American Cerrado avifauna. Steenstrupia 21, 49–67.
Silva, J. M. C. (1996). Distribution of amazonian and atlantic birds in gallery forests of the cerrado region. South America. Ornitol. Neotrop. 7, 1–18.
Silva, J. M. C. (1997). Endemic bird species and conservation in the Cerrado region, South America. Biodivers. Conserv. 6, 435–450. doi: 10.1023/A:1018368809116
Silva, J. M. C., and Bates, J. M. (2002). Biogeographic patterns and conservation in the South American Cerrado: a tropical savanna hotspot. Bioscience 52, 225–234. doi: 10.1641/0006-3568(2002)052[0225:bpacit]2.0.co;2
Silva, J. M. C., Hass, A., Ferreira, A. A., Bianchi, C. A., Tubelis, D., Willis, E. O., et al. (2007). “Avifauna,” in Biodiversidade do Cerrado e Pantanal: Áreas E Ações Prioritárias Para Conservação, ed. R. B. Cavalcanti (Brasília, DF: Ministério do Meio Ambiente, Biodiversidade), 277–299.
Silva, L. G. L., Ferreira, D. C., and Rossi, R. V. (2019). Species diversity of Marmosa subgenus Micoureus (Didelphimorphia, Didelphidae) and taxonomic evaluation of the white-bellied woolly mouse opossum, Marmosa constantiae. Zool. J. Linn. Soc. 187, 240–277. doi: 10.1093/zoolinnean/zlz023
Sirami, C., and Monadjem, A. (2012). Changes in bird communities in Swaziland savannas between 1998 and 2008 owing to shrub encroachment. Div. Distrib. 18, 390–400. doi: 10.1111/j.1472-4642.2011.00810.x
Socolar, J. B., Gilroy, J. J., Kunin, W. E., and Edwards, D. P. (2016). How should beta-diversity inform biodiversity conservation? Trends Ecol. Evol. 31, 67–80. doi: 10.1016/j.tree.2015.11.005
Solbrig, O., Medina, E., and Silva, J. (1996). “Biodiversity and Savanna Ecosystem Processes: a Global Perspective,” in Functional Roles of Biodiversity: A Global Perspective, eds H. A. Mooney, J. H. Cushman, E. Medina, O. E. Sala, and E.-D. Shulze (Hoboken, NY: John Wiley & Sons), 185–211. doi: 10.1007/978-3-642-78969-4
Steppan, S. J., and Schenk, J. J. (2017). Muroid rodent phylogenetics: 900-species tree reveals increasing diversification rates. PLoS One 12:e0183070. doi: 10.1371/journal.pone.0183070
Strassburg, B. B. N., Brooks, T., Feltran-Barbieri, R., Iribarrem, A., Crouzeilles, R., Loyola, R., et al. (2017). Moment of truth for the Cerrado hotspot. Nat. Ecol. Evol. 1:3. doi: 10.1038/s41559-017-0099
Suárez-Villota, E. Y., Carmignotto, A. P., Brandão, M. V., Percequillo, A. R., and Silva, M. J. J. (2018). Systematics of the genus Oecomys (Sigmodontinae: Oryzomyini): molecular phylogenetics, cytogenetic, and morphological approaches reveal cryptic species. Biol. J. Linn. Soc. 184, 182–210. doi: 10.1093/zoolinnean/zlx095
Suttie, J. M., Reynolds, S. G., and Batello, C. (2005). Grasslands of the World. No. 34. Rome: Food and Agriculture Organization.
Umetsu, F., Naxara, L., and Pardini, R. (2006). Evaluating the efficiency of pitfall traps for sampling small mammals in the Neotropics. J. Mammal. 87, 757–765. doi: 10.1644/05-MAMM-A-285R2.1
Valdujo, P. H., Carnaval, A. C. O. Q., and Graham, C. H. (2013). Environmental correlates of anuran beta diversity in the Brazilian Cerrado. Ecography 36, 708–717. doi: 10.1111/j.1600-0587.2012.07374.x
Valdujo, P. H., Silvano, D. L., Colli, G., and Martins, M. (2012). Anuran species composition and distribution patterns in brazilian cerrado, a neotropical hotspot. South Am. J. Herp. 7, 63–78. doi: 10.2994/057.007.0209
Van der Walt, L., Cilliers, S. S., Du Toit, M. J., and Kellner, K. (2015). Conservation of fragmented grasslands as part of the urban green infrastructure: how important are species diversity, functional diversity and landscape functionality? Urban Ecosyst. 18, 87–113. doi: 10.1007/s11252-014-0393-9
Veblen, T. T., Young, K. R., and Orme, A. R. (2007). The Physical Geography Of South America. New York, NY: Oxford University Press.
Veech, J. A., Summerville, K. S., Crist, T. O., and Gering, J. C. (2002). The additive partitioning of species diversity: recent revival of an old idea. Oikos 99, 3–9. doi: 10.1034/j.1600-0706.2002.990101.x
Vieira, E. M., and Palma, A. R. T. (2005). “Pequenos mamíferos do Cerrado: distribuição dos gêneros e estrutura das comunidades nos diferentes hábitats,” in Biodiversidade, Ecologia e Conservação do Cerrado, eds A. Scariot, J. M. Felfili, and J. C. Sousa-Silva (Brasília, DF: EMBRAPA), 267–282.
Vieira, E. M., Iob, G., Briani, D. C., and Palma, A. R. T. (2005). Microhabitat selection and daily movements of two rodents (Necromys lasiurus and Oryzomys scotti) in Brazilian Cerrado, as revealed by a spool-and-line device. Mamm. Biol. 70, 359–365. doi: 10.1016/j.mambio.2005.08.002
Voss, R. S., and Jansa, S. A. (2009). Phylogenetic relationships and classification of didelphid marsupials, an extant radiation of New World metatherian mammals. Bull. Am. Mus. Nat. Hist. 322, 1–177. doi: 10.1206/322.1
Webb, C. O., Ackerly, D. D., McPeek, M. A., and Donoghue, M. J. (2002). Phylogenies and community ecology. Annu. Rev. Ecol. Syst. 33, 475–505. doi: 10.1146/annurev.ecolsys.33.010802.150448
Weksler, M., and Bonvicino, C. R. (2005). Taxonomy of pigmy rice rats (genus Oligoryzomys, Rodentia: Sigmodontinae) of the Brazilian Cerrado, with the description of two new species. Arq. Mus. Nac. 63, 113–130.
Weksler, M., and Bonvicino, C. R. (2015). “Genus Oligoryzomys Bangs, 1900,” in Mammals of South America, Vol. 2, Rodents, eds J. L. Patton, U. F. J. Pardinãs, and G. D’Elía (Chicago, IL: The University of Chicago Press), 417–437.
Weksler, M., Lemos, E. M. S., D’Andrea, P. S., and Bonvicino, C. R. (2017). The taxonomic status of Oligoryzomys mattogrossae (Allen, 1916) (Rodentia: Cricetidae: Sigmodontinae), reservoir of anajatuba hantavirus. Am. Mus. Novit. 3880, 1–32. doi: 10.1206/3880.1
Werneck, F. P. (2011). The diversification of eastern South American open vegetation biomes: historical biogeography and perspectives. Quat. Sci. Rev. 30, 1630–1648. doi: 10.1016/j.quascirev.2011.03.009
Keywords: abundance, composition, habitat selectivity, inventory, marsupials, rodents, species richness
Citation: Carmignotto AP, Pardini R and de Vivo M (2022) Habitat Heterogeneity and Geographic Location as Major Drivers of Cerrado Small Mammal Diversity Across Multiple Spatial Scales. Front. Ecol. Evol. 9:739919. doi: 10.3389/fevo.2021.739919
Received: 12 July 2021; Accepted: 24 December 2021;
Published: 17 February 2022.
Edited by:
Emerson M. Vieira, Universidade de Brasília, BrazilReviewed by:
Cássia Bitencourt, Royal Botanic Gardens, Kew, United KingdomRenan Maestri, Federal University of Rio Grande do Sul, Brazil
Copyright © 2022 Carmignotto, Pardini and de Vivo. This is an open-access article distributed under the terms of the Creative Commons Attribution License (CC BY). The use, distribution or reproduction in other forums is permitted, provided the original author(s) and the copyright owner(s) are credited and that the original publication in this journal is cited, in accordance with accepted academic practice. No use, distribution or reproduction is permitted which does not comply with these terms.
*Correspondence: Ana Paula Carmignotto, apcarmig@ufscar.br