- 1Department of Biodiversity, Conservation and Attractions, Kununurra, WA, Australia
- 2Research Institute for the Environment and Livelihoods, Charles Darwin University, Darwin, NT, Australia
- 3Southern Flora Wildflower Nursery, Bridgetown, WA, Australia
- 4Department of Biodiversity, Conservation and Attractions, Manjimup, WA, Australia
- 5World Wide Fund for Nature, Broome, WA, Australia
Northern Australia has undergone significant declines among threatened small and medium-sized mammals in recent decades. Conceptual models postulate that predation by feral cats is the primary driver, with changed disturbance regimes from fire and feral livestock in recent decades reducing habitat cover and exacerbating declines. However, there is little guidance on what scale habitat and disturbance attributes are most important for threatened mammals, and what elements and scale of fire mosaics actually support mammals. In this study, we test a series of hypotheses regarding the influence of site-scale (50 × 50 m) habitat and disturbance attributes, as well as local-scale (1 km radius), meta-local scale (3 km), landscape-scale (5 km) and meta-landscape scale (10 km) fire mosaic attributes on mammal abundance and richness. We found that habitat cover (rock, perennial grass, and shrub cover) at the site-scale had a positive effect, and disturbance factors (feral cats, fire, feral livestock) had a negative influence on mammal abundance and richness. Models supported site-scale habitat and disturbance factors as more important for mammals than broader-scale (local up to meta-landscape scale) fire mosaic attributes. Finally, we found that increasing the extent of ≥ 4 year unburnt habitat, and having an intermediate percentage (ca. 25%) of recently burnt (1-year burnt) habitat within the mosaic, were the most important functional elements of the fire mosaic at broad scales for mammals. Contrary to expectations, diversity of post-fire ages (‘pyrodiversity’) was negatively associated with mammal abundance and richness. These results highlight the need for management to promote retention of longer unburnt vegetation in sufficient patches across savanna landscapes (particularly of shrub and fruiting trees), maintain low-intensity patchy fire regimes, reduce the extent of intense late dry season wildfires, and to reduce the impact of feral livestock. This study provides further evidence for the role of feral cats in northern Australian mammal declines, and highlights the need for increased research into the efficacy of cat control methodologies in reducing biodiversity impacts in these extensive landscapes.
Introduction
Australia has the dubious distinction during the previous two centuries of having the highest number of mammalian extinctions; 21 out of the 230 non-volant mammals present at European colonization are now gone, and another 23 have disappeared from most of their former range (Woinarski et al., 2014). While many of these extinctions occurred in the early years of European colonization in southern and arid Australia (Johnson, 2006), more recent declines in the tropical savannas of northern Australia have occurred in areas that had relatively intact mammal assemblages right up until the 1990’s and early 2000’s, up to a century after many of the previous declines occurred (Braithwaite and Muller, 1997; Woinarski et al., 2001, 2010).
In Northern Australia it has been unclear what the primary mechanisms behind declines might be, and a range of hypotheses have been raised (Woinarski et al., 2001, 2010, 2011; Andersen et al., 2005; Ziembicki et al., 2015). These include predation by feral cats (Frank et al., 2014; McGregor et al., 2016), changes in the influence of dingoes, either directly on mammals, or via impacts on feral cats (Kennedy et al., 2012), changed fire regimes and management (Woinarski et al., 2010; Lawes et al., 2015; Radford et al., 2015, Radford et al., 2020a), pastoralism and feral livestock (Legge et al., 2011, 2019; Radford et al., 2015), cane toad invasion (Ziembicki et al., 2015; Radford et al., 2020b), declining ecosystem productivity due to changed disturbance regimes (McKenzie et al., 2007), disease or pathogens (Ziembicki et al., 2015) and climate change (Braithwaite and Muller, 1997; Ziembicki et al., 2015). However, no single mechanism explains mammal declines across northern Australia.
Australia wide, mammalian extinctions and declines have primarily been attributed to introduced predators, particularly the feral cat (Felis catus) and the red fox (Vulpes vulpes) based on the historical coincidence of arrival of predators with mammal declines and extinctions (Dickman, 1996; Johnson, 2006) and also on fenced cat exclosure experiments (Moseby et al., 2009; Frank et al., 2014; Tuft et al., 2021). The most parsimonious explanation for mammal declines in northern Australia is that cats are driving this decline too (though not foxes as they do not persist in the tropics). However, cats have co-existed in northern Australia with intact mammal faunas for ca. a century since they first established in the 1890’s (Abbott, 2002).
What then could have led to increased predation impacts of feral cats in northern Australia leading to the most recent savanna mammals declines? Recent studies have shown that cats preferentially hunt (McGregor et al., 2014, 2015), have higher kill rates (McGregor et al., 2016) and have greater impacts on small mammal prey in open, recently burnt savannas, or in habitats heavily disturbed by feral livestock (McGregor et al., 2015; Leahy et al., 2016; Shaw et al., 2021). Feral cat activity is thus greatest in areas under severe disturbance regimes, such as frequent high severity fires and/or high feral livestock activity; which is mechanistically linked to repeated removal of ground-layer vegetation and suppression of plant regrowth (McGregor et al., 2015; Legge et al., 2019; Davies et al., 2020). Conceptual models explaining northern Australian mammal declines now postulate predation by feral cats as the primary driver, but that changed disturbance regimes in recent decades (e.g., fire regimes and feral livestock grazing pressure) have reduced habitat cover and productivity, thereby exacerbating predation impacts on small mammals (Johnson, 2006; McKenzie et al., 2007; Radford et al., 2014; Ziembicki et al., 2015; Legge et al., 2019; Stobo-Wilson et al., 2020a).
Studies have now linked regional patterns of mammal abundance and richness with cat occupancy, disturbance regimes and vegetation cover, providing some support for these conceptual models (Davies et al., 2017, 2020; Radford et al., 2020a; Stobo-Wilson et al., 2020a; Penton et al., 2021). However from a management perspective, it is still unclear what site-scale habitat or disturbance features support or threaten mammal assemblages. Although we know that applying low intensity prescribed burning mosaics to savannas can lead to declines in the extent of damaging late dry season wildfires, and benefits to savanna mammals (Radford et al., 2020a), it is unclear which fire mosaic attributes specifically are important (or functional as per Parr and Andersen, 2006) in supporting local population increases or decreases. Moreover, it is unclear at what scale (local, landscape, regional) these functional mosaic attributes are essential for savanna mammals. Such questions are crucially important if conservation managers are to design the most appropriate interventions for threatened species at the local, landscape and regional scale across vast savanna landscapes in northern Australia.
In this study we use repeated measures analysis to test for the relative importance of site-linked habitat and disturbance attributes, and landscape-scale fire mosaics, in driving site-based mammal abundance and richness in north-west Australia. Uniquely, this longitudinal study spans nine years (2011–2019) and includes study sites stratified between rocky and non-rocky savannas, due to previously described compositional differences between mammal communities in these habitats (Radford et al., 2014; Radford et al., 2020a). Repeated measures analysis allows us to assess the importance of dynamic habitat and disturbance factors, including episodic fire events, in driving mammal population trends at the site scale. This contrasts with recent single time-step analyses (Radford et al., 2015; Davies et al., 2017; Stobo-Wilson et al., 2020a). By examining site-level fixed and dynamic habitat and disturbance factors, we can further scrutinize fine-scale vegetation and disturbance dynamics most relevant to on-ground management for improving mammal abundance and richness. Additionally, by testing for the relationship between fire regime attributes from local up to landscape and sub-regional scales, we can elucidate the most important functional components of fire mosaics for threatened savanna mammals.
Here, we test the following hypotheses: (1) That mammal abundance and richness is highest with increasing site-scale ground layer habitat cover/complexity (Radford et al., 2015, Radford et al., 2020a; Davies et al., 2017, 2020; Stobo-Wilson et al., 2020a); (2) that mammal abundance and richness is highest at sites where predator (feral cat and dingo), fire and cattle ‘disturbance’ is least prevalent or abundant (McGregor et al., 2014, 2015, 2016; Lawes et al., 2015; Radford et al., 2015; Leahy et al., 2016; Stobo-Wilson et al., 2020a,b; Shaw et al., 2021); (3) that site- and local-scale fire mosaic attributes will be more influential on mammal abundance and richness than broader landscape- or subregional-scale mosaic attributes due to recolonization/dispersal limitations for some mammal species (Leahy et al., 2016; Shaw et al., 2021); and (4) the most important attributes of local and landscape fire mosaics will be presence of longer unburnt habitat based on previous observations (e.g., Legge et al., 2008; Radford et al., 2015, Radford et al., 2020a) and that pyrodiversity (diversity of post-fire habitat age) will be positively associated with mammal abundance and richness based on patch mosaic burning theory (critiques by Parr and Andersen, 2006; Jones and Tingley, 2021).
Materials and Methods
Study Area
The study area is the Northern Kimberley biogeographic region of north-western Western Australia (Figure 1). The region experiences a tropical monsoonal climate, with high temperatures year-round (daily mean maximum 29.6–36.0°C) and high rainfall (900–1550 mm) occurring predominantly during the warmer months (i.e., November to April). Savanna vegetation is characteristic of this region, with Eucalypt species making up the tree canopy in most areas and C4 grasses dominating the understory. Vegetation ranges from savanna forest (30–50% tree cover) through to woodland and open woodland (10–30% cover) and shrubland (<10% cover) depending on substrates. Tussock and hummock grass cover ranges from 5 to almost 100%. Small patches of rainforest and riparian gallery forest with closed canopies (>70% cover) and zero grass cover occur within the savanna matrix. Substrates range from relatively fertile clay soil on igneous rock, through laterite derived loam and gravel substrates, to sandy or skeletal soils on rugged sandstone. Due to the annual cycle of a wet season followed by an extended dry season (>6 months) in which grasses cure, savannas in the region are subject to annual grass fueled fire regimes (Russell-Smith et al., 2003b). The Northern Kimberley region has not been subject to extensive vegetation clearing; however, fire regimes changed to high-intensity wildfire dominated fire regimes during the 20th century with the breakdown of traditional Indigenous fire management (Russell-Smith et al., 2003b; Connor et al., 2018). Introduced herds of cattle, horses, and donkeys are widespread throughout the region as an additional critical disturbance factor.
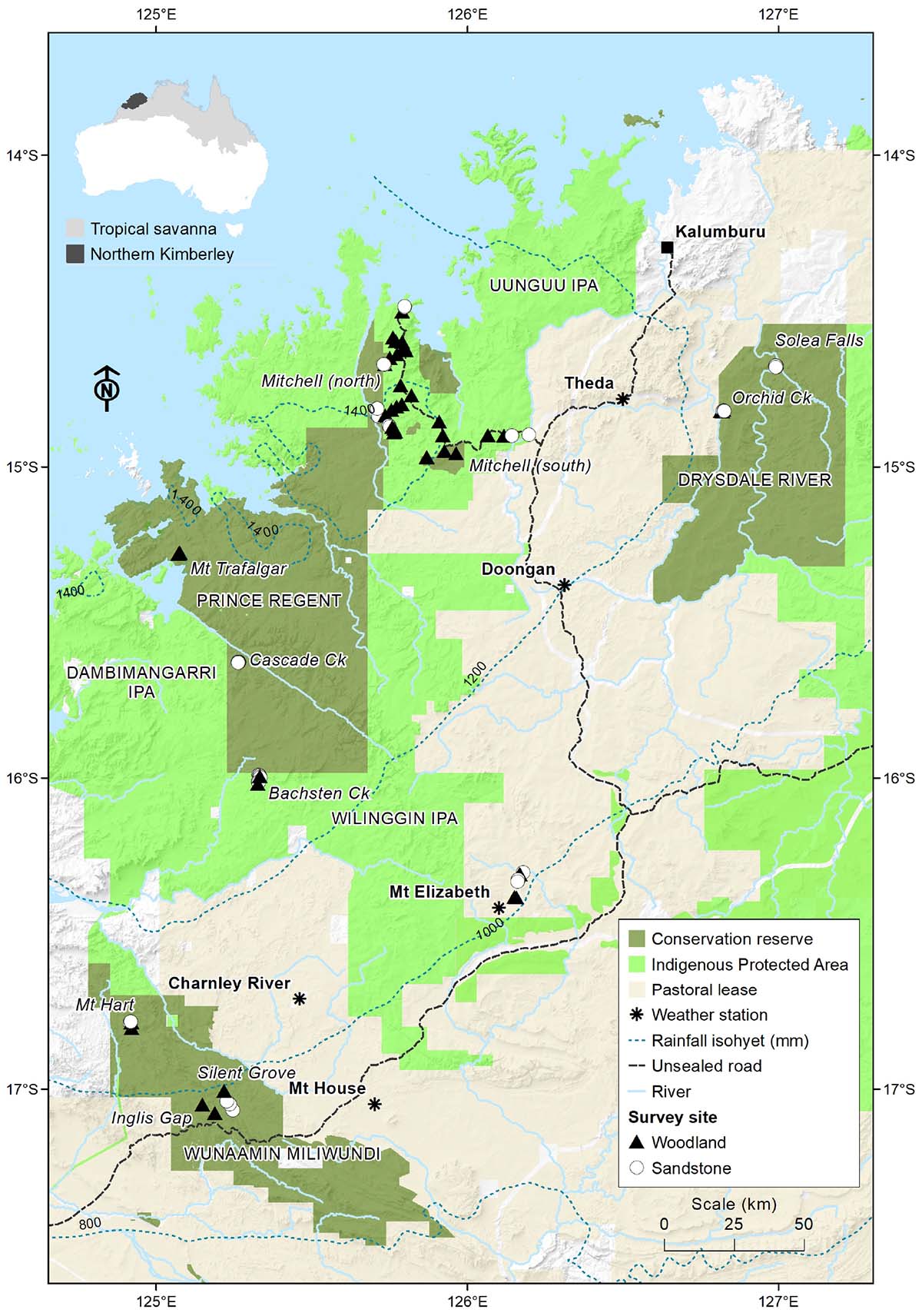
Figure 1. Locations of survey sites and locations in the Northern Kimberley region of northern Western Australia.
Survey Design
Survey sites were distributed across 11 subregions within the Northern Kimberley (Figure 1 and Table 1). Study sites were stratified between two major geological landscapes: sandstone and volcanic (Figure 1), based on previous analyses which show that mammal assemblages diverge strongly between these habitats (Bradley et al., 1987; Start et al., 2007, 2012; Radford et al., 2014; Radford et al., 2020a). Habitat structure in sandstone landscapes was characterized by abundant rock cover and crevices due to the rugged rocky sandstone substrate, with vegetation ranging from hummock grassland to shrubland, woodland, and vine thicket. Volcanic landscapes had relatively few rock crevices, though sometimes with high rock cover, and vegetation ranging from open woodland on basalt to open forest on lateritic substrates. Location of survey sites was based primarily on the presence of historical survey sites (Bradley et al., 1987; Start et al., 2007, 2012; Radford et al., 2014). However, where historical sites were not available, additional survey sites were added in suitable habitats. Sites were located both inside and outside National Parks to account for the possible influences of differing tenure, management and disturbance factors within the region. Despite close proximity of some sites due to remote access constraints (ca. within 100 m in some areas) sites are considered independent due to the relatively few occasions (6%) where recaptures occurred among nearby sites.
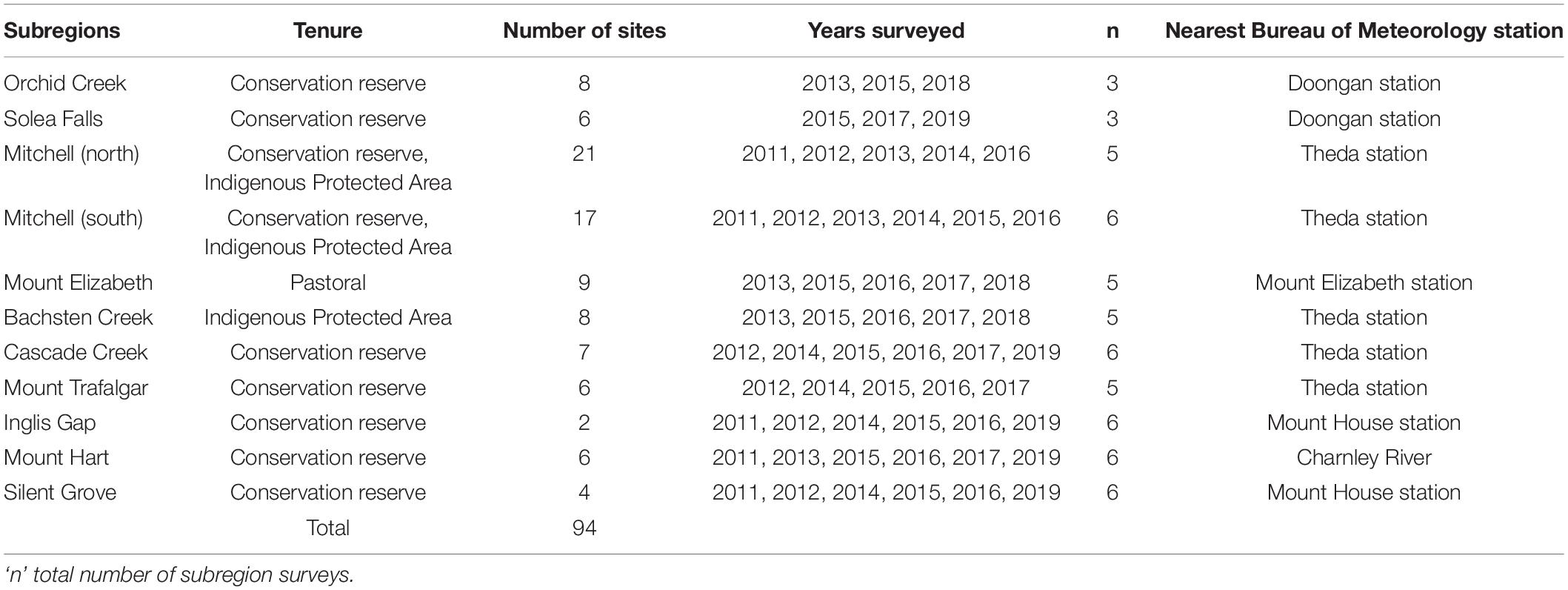
Table 1. Summary of survey sites, tenure, and survey frequency and Bureau of Meteorological Stations used for rainfall data.
In order to account for inherent variation among sites this study used repeated surveys to account for fixed site differences. In total, 407 surveys were undertaken at 94 sites between 2011 and 2019; 44 sites on sandstone and 50 in volcanic habitats (Table 1). Most sites were surveyed five times (n = 47), 18 sites were surveyed six times, while another 16 sites were surveyed three times. Ten sites were surveyed only once. Due to logistical constraints, selecting and surveying sites was a staged process, with not all sites surveyed every year (Table 1). Trap effort varied between the first and all other years of the study and is included as an offset within our analyses. Site trap effort was either 72 or 144 trap nights in 2011 (24 traps open for 3 or 6 nights) and was standardized from 2012 onward at 120 trap nights (24 traps open 5 nights).
Mammal Data
Mammal data were collected from 50 × 50 m quadrats (survey site) similar to those used as a standard monitoring plot in other areas of northern Australia (Woinarski et al., 2010; Legge et al., 2011; Radford et al., 2015). A total of 20 large (15 × 15.5 × 46 cm) and medium (9 × 10 × 33 cm) metal box traps (Elliotts), were alternately placed around the perimeter of each quadrat. Four larger wire cage traps (25 × 30 × 73 cm) were placed at the corners. Traps were baited with a mixture of peanut butter and rolled oats. Traps were shaded using grass, leaves or hessian sacks and checked early each morning to prevent overheating. Mammals were identified to species and marked using ISO FDX-B Microchips (Mychip) for larger species or permanent marker pens on small rodents’ ears. Site mammal data were described in terms of abundance (total number of individuals captured not including recaptures) and species richness (total number of species captured). Sandstone and volcanic habitat mean (±se) mammal captures per site were 9.5 (±1.1) and 7.2 (±1.0) respectively and site species richness was 2.7 (±0.1) and 2.1 (±0.1) respectively.
Survey Site Habitat and Productivity Attributes
Each survey site (50 × 50 m) was assessed for both fixed and dynamic habitat structural attributes during the mammal surveys (Table 2). Tree (height > 4 m) and shrub (height < 4 m) projected canopy cover was estimated using a 1% Bitterlich gauge (Lindsey et al., 1958). Each plant canopy was assessed as 1% cover if the 10 cm cross-bar, held 50 cm from the observer’s eye, was narrower than the canopy width when standing at the quadrat’s central point (37 m along the diagonal from the quadrat corner post). Assessing each canopy in a 360° arc from the central point gives a total percentage value for canopy cover. Separate canopy cover was estimated for trees which produced fleshy fruits eaten by arboreal savanna mammals as an index of habitat suitability for these species. Tree basal area was estimated using a Basal Area Factor 1 Metric Wedge Prism (CruiseMaster). Ground vegetation cover attributes were assessed using a 50 m transect run diagonally from the corner post through the survey site. The accumulated distance under the transect tape was used to estimate percentage cover of perennial grasses (tussock or hummock), annual grass (Sorghum spp.), herbaceous forbes (non-grasses) and leaf/branch litter. The fixed percentage of exposed rock and/or gravel cover was estimated using the same transect method. Combined introduced plant cover ‘weeds’ was estimated as a combined percentage projected ground cover of exotic tree, shrub and ground-layer vegetation cover.
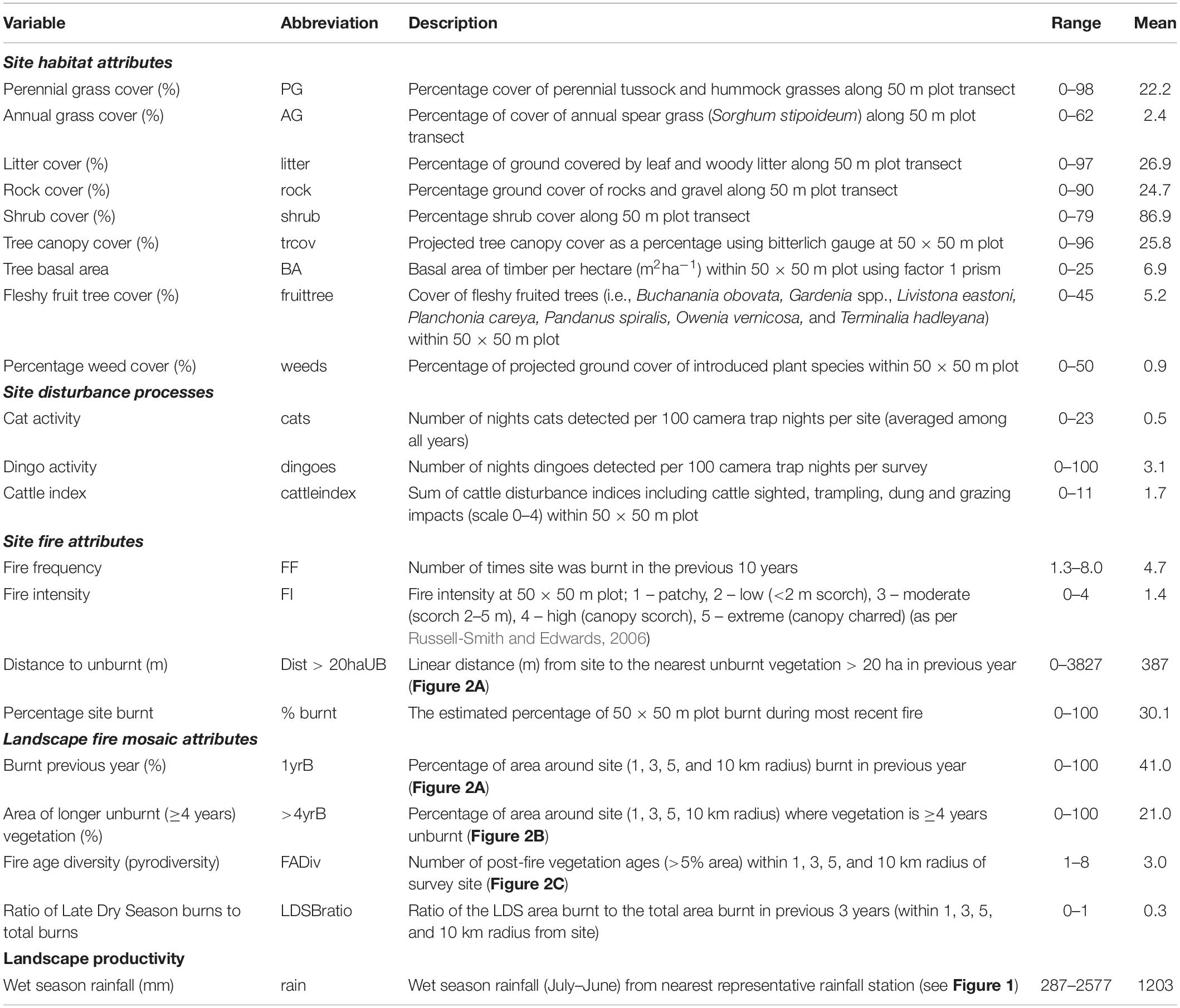
Table 2. Explanatory variables measured at and around survey sites, their definitions and summary statistics.
Productivity is directly linked to rainfall in seasonal tropical savannas of northern Australia (McKenzie et al., 2007; Radford et al., 2014). Wet season rainfall (July–June) was calculated for survey sites within each sub-region (Table 1 and Figure 1) based on monthly rainfall totals from the nearest weather station within the same rainfall isohyet with a complete monthly rainfall data set during the study period (Table 1 and Figure 1).
Disturbance Attributes
Predator Activity
Predator trap success data was recorded at survey sites through the use of remote infrared cameras at each site during live-trapping surveys (see above). Single cameras were placed out for five nights per survey. In addition, each site was trapped once using a 5-camera array (as per Einoder et al., 2018; Stobo-Wilson et al., 2020a) over a 30–50 days period. Reconyx PC900 Hyperfire Professional Infra-red (IR) or PC950 Hyperfire Security IR cameras were used in camera surveys, both of which are successful in detecting mammals > 1 kg. Cameras were baited using universal bait (peanut butter and oats). The presence of predators cannot, therefore, be attributed primarily to the bait. Rather the presence of predators is likely to reflect natural visitation of sites in savanna landscapes, with the omnivorous bait providing a short distance cue to encourage the predator to come close to the camera. Dingoes were frequently detected at many survey sites during the study period and trap success (number of detections per 100 trap nights) was recorded for each site during each year of survey. Cats were not detected at the majority of survey sites despite multiple surveys over a 9 years study period. However, cats were detected at some sites and at these sites were sometimes frequently recorded. This suggests that cats had a preference for some sites over others despite all sites being equally available to cats as they are ubiquitous throughout the region. To account for this site-specific preference (possibly related to local disturbance regimes), cat trap success (number of detections per 100 trap nights) was calculated as a fixed variable accounting for the relative preference cats had of visiting each monitoring site.
Fire Mosaics at Survey Site Up to Landscape Scales
Fire mosaics were measured at multiple pre-defined scales within and around survey sites to test for the effects of disturbance attributes from survey site up to broad landscape scales (Figure 2). Mosaic scales were defined as site (50 × 50 m), local (1 km radius from survey site; total area of 3 km2), meta-local (3 km radius; total area of 28 km2), landscape (5 km radius; total area of 79 km2), and meta-landscape scales (10 km radius; total area of 314 km2).
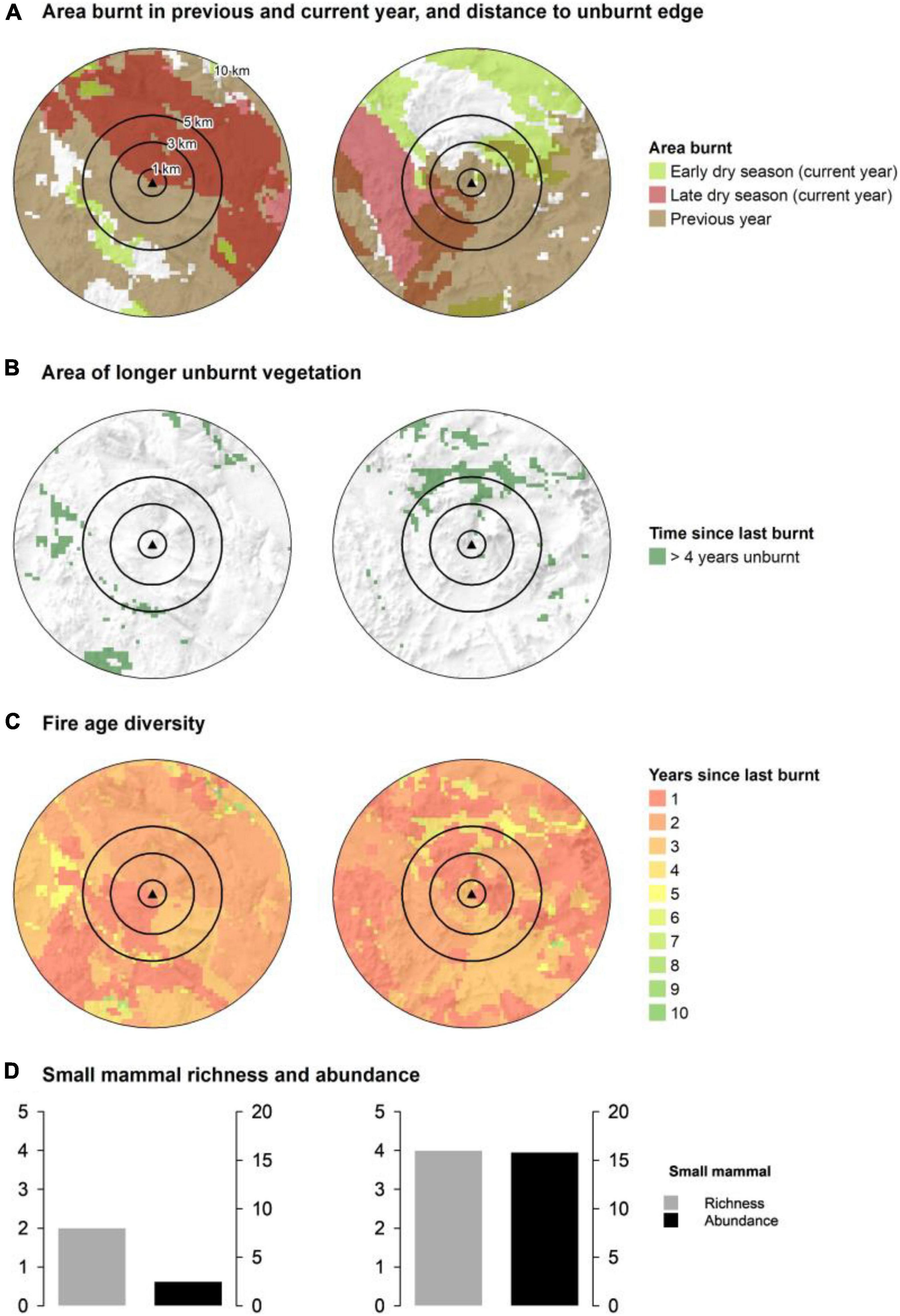
Figure 2. Fire mosaic elements within the landscape context of two of the mammal survey sites, LCI 205 at Inglis Gap (left) and LCI 210 at Mt Hart (right). The graphs show concentric spatial areas around sites defined by rings at 1, 3, 5, and 10 km from the center of the location of the survey site and provides a partial view of the fire mosaic context within which these sites are placed. (A) Shows the spatial extent of early (March–July) and late dry season fires (August–December) during the year of the survey and the total extent of fire in the previous year. (B) Shows the spatial extent of habitat unburnt for four or more years. (C) Shows the patterns of ‘pyrodiversity,’ here defined as the number of post-fire habitat ages, within the landscape context of the survey sites. (D) Shows a histogram of mammal abundance (black; number of individuals per 100 trap nights) and richness (grey; number of different species) for the two sites, with the Mt Hart site LCI 210 supporting much greater mammal abundance and richness (right) than the Inglis Gap site LCI 205 (left).
Site-scale mosaic attributes included fire intensity (based on leaf scorch height as per Russell-Smith and Edwards, 2006) and percentage of site burnt in the current year were estimated at the time of each survey (Table 2). Remote sensing data were used to derive other fire mosaic attributes (Table 2). Analyses are based on fire scars derived from Moderate Resolution Imaging Spectroradiometer (MODIS) imagery at 250 m resolution1. MODIS data for each year were obtained from the North Australia and Rangelands Fire Information (NAFI) website2. Site scale fire frequency was calculated over the previous 10 years based on fire scars intersecting with site locations during each year of the study period and also based on direct observations during the study period. As an indication of fire size at the site scale (year previous to survey), raster analysis was used to determine the distance from each survey site to the nearest unburnt vegetation patch > 20 ha. This size patch was chosen to represent the minimum size an unburnt patch could be to support a single home range for savanna mammal species (e.g., Cook, 2010; Hohnen et al., 2015, 2016a; Penton et al., 2020).
Local up to meta-landscape scale mosaic attributes within radial areas included percentage of area burnt in the previous year, the area unburnt for ≥ 4 years, fire age diversity (pyrodiversity) and the ratio of late dry season burning (Table 2 and Figure 2). All spatial analyses were carried out in ArcMap 10.1 using tools in the Spatial Analyst extension.
Survey Site Feral Herbivore Disturbance
Feral livestock disturbance (mainly cattle) was qualified as cattle sighted [none (0), single individual (1), several (2), groups (3)], grazing level and evidence of tracks/trampling [no evidence (0), light (1), moderate (2), heavy (3)] and cattle dung [none (0), some sighted (1), scattered (2), extensive (3)]. These four herbivore disturbance attributes were added together to give an index of cattle disturbance impact at the site.
Data Analysis
To examine the relationship between mammal richness or mammal abundance and potential predictor variables (Table 1), we fitted generalized linear mixed models (GLMM) (R package glmmTMB: Brooks et al., 2017) with Poisson or negative binomial distribution and log-link, offset for trap effort, and with site included as a random effect using statistical software program R (R Core Team, 2021). All continuous variables were centered and standardized (Gelman, 2008) and included as quadratic polynomial terms where non-linear trends were detected during data exploration. Collinearity between variables was tested using Variance Inflation Factors (VIF) with cutoff for inclusion of 3 and Pearson’s correlations with cutoff for inclusion of 0.7 (Zuur et al., 2010). Due to collinearity (VIF > 3), fire frequency was dropped from analyses for volcanic habitat.
Because mammal species assemblages differ between habitats (Radford et al., 2014; Radford et al., 2020a), separate analyses were conducted for each habitat type, i.e., sandstone and volcanic. These analyses were structured according to three main hypotheses being tested (1) site-level habitat, (2) site-level disturbance and (3) pyrodiversity at 1, 3, 5, and 10 km radii around a particular site (Table 1 and Figure 2). To identify highly influential predictors in each of these models, we used an information-theoretic model-averaging approach (Burnham and Anderson, 2002). A top (95% confidence) model set was selected according to AICc (Akaike Information Criterion corrected for small sample size), i.e., the top models with cumulative sum of Akaike weights less than 0.95 (R package MuMIn: Barton, 2020). The best models included only highly influential variables from the top model set. These variables are defined as having a relative variable importance (sum of Akaike weights for all models containing a given predictor variable) ≤ 0.73, which is equivalent to an AICc difference of <2 (Richards, 2005). Model assumptions were verified by normal probability plots (QQ-plots) of fixed versus random effects, plotting residual versus fitted values versus each covariate in the model, and versus each covariate not in the model. We tested for overdispersion, zero-inflation, temporal autocorrelation (based on Durbin-Watson test) and spatial autocorrelation (based on Moran’s I) (R package DHARMa: Hartig, 2021). Model validation indicated no problems.
Results
Mammal Abundance
In sandstone habitats, the site-scale attributes (50 × 50 m) most strongly associated with increased mammal abundance were (1) habitat (positive relationship): rock cover and tree canopy cover, (2) disturbance (negative relationship): cat activity, fire frequency and proportion of the site burnt in the current year (Table 2). Mosaic attributes, including fire age diversity, proportion of the site burnt in the previous year (negative relationships), and proportion of ≥4-year old unburnt vegetation (positive relationship) were associated with increased mammal abundance at the local and meta-local scales (≤3 km) around a site (Table 3 and Figure 3). Previous wet season rainfall within sub-regions (≥5 km) was also a strong predictor of increased mammal abundance (Table 3).
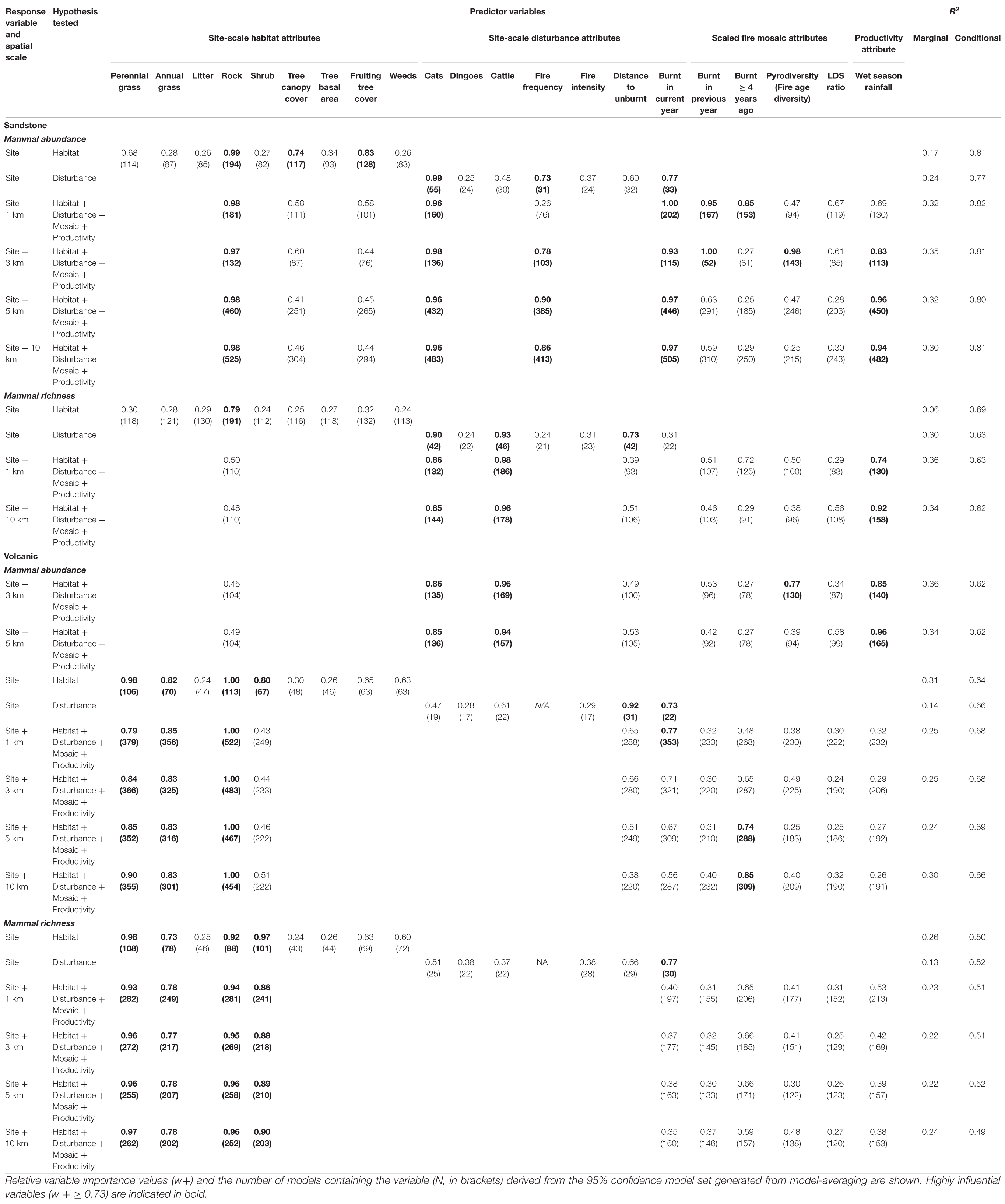
Table 3. The relative importance of habitat, disturbance, pyrodiversity, and productivity variables derived from general linear mixed models (GLMM) modeling the effect of these variables on mammal abundance or richness.
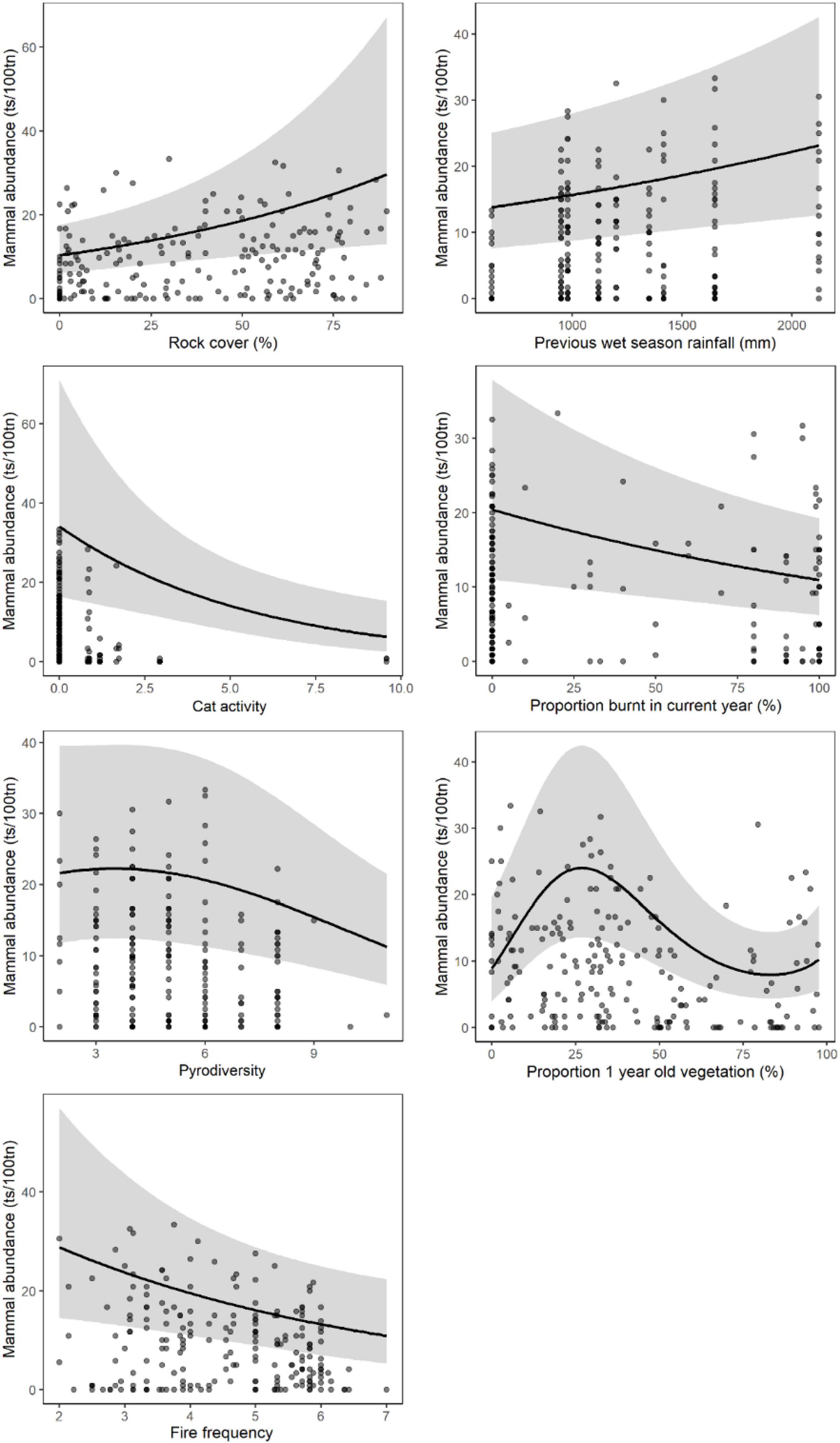
Figure 3. Mammal abundance [trap success per 100 trap nights (ts/100 tn)] in sandstone habitat shown only for highly influential predictor variables sampled at the 3 km scale around each site (see Table 2). Relationships for each variable were derived from GLMM predictions while holding other explanatory variables constant at their median level. Model fit is shown by a black line and the gray band represents the 95% confidence interval. Gray circles indicate observed data values and are darker when repeated observations occur at the same point. Cat activity is cat detections per 100 camera trap nights, pyrodiversity is the number of post fire vegetation ages within 3 km of the site, fire frequency is the number of times the site was burnt in the previous 10 years.
At the local/meta-local scale (≤3 km) around a site, the disturbance + mosaic model, together with the fixed habitat attribute of rock cover, was the best model for mammal abundance in sandstone habitats (Table 3 and Figure 3). At the landscape and meta-landscape scale (≥5 km), mosaic attributes were less important and the disturbance model, together with habitat attribute rock cover, and sub-regional rainfall was the best model of mammal abundance in sandstone habitats (Table 3).
In volcanic habitats, the site-scale attributes most strongly associated with increased mammal abundance were (1) habitat (positive relationships): rock cover, shrub cover, perennial grass cover, and negatively with annual grass (Sorghum stipoideum) cover; (2) site-scale disturbance (negative relationships): distance to the nearest unburnt patch > 20 ha and proportion of the site burnt in the current year (Table 3). Mosaic attribute (proportion of ≥ 4-year old unburnt vegetation) was associated with increased mammal abundance at the landscape and meta-landscape scale (≥5 km; Table 3 and Figure 4).
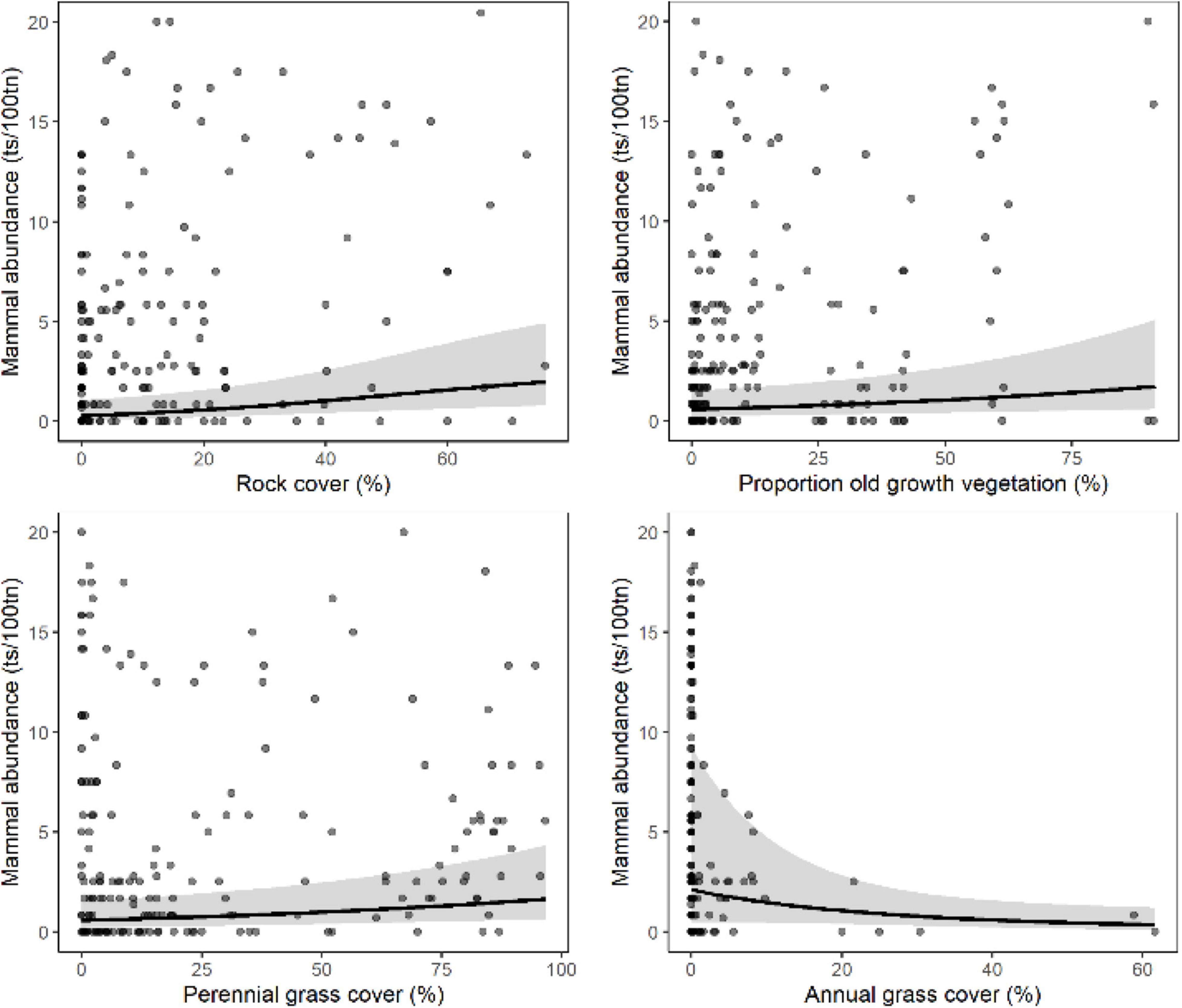
Figure 4. Mammal abundance [trap success per 100 trap nights (ts/100tn)] in volcanic habitat shown only for highly influential predictor variables sampled at the 3 km scale around each site (see Table 2). Relationships for each variable were derived from GLMM predictions while holding other explanatory variables constant at their median level. Model fit is shown by a black line and the gray band represents the 95% confidence interval. Gray circles indicate observed data values and are darker when repeated observations occur at the same point.
At the site-scale, the local-scale and at the meta-local scales (≤3 km), disturbance attributes were less important and the habitat model, was the best model of mammal abundance in volcanic habitats (Table 3). At the landscape- and meta-landscape-scales, the habitat model and mosaic attribute, the proportion of ≥4 years old unburnt vegetation, was the best model for mammal abundance in volcanic habitats (Table 3 and Figure 4).
Mammal Richness
In sandstone habitats, the site-scale attributes most strongly associated with increased mammal richness were (1) habitat: rock cover, and (2) disturbance (negative relationship): cat activity, cattle index and distance to the nearest unburnt patch > 20 ha (Table 3). Mosaic attribute, fire age diversity was associated with decreased mammal richness at the local- and meta-local scales (<3 km; Table 3). Previous wet season rainfall within sub-regions was a strong predictor of increased mammal richness (Table 3). The site-scale disturbance model, together with sub-regional rainfall, was the best model of mammal richness in sandstone habitats (Table 3 and Figure 5).
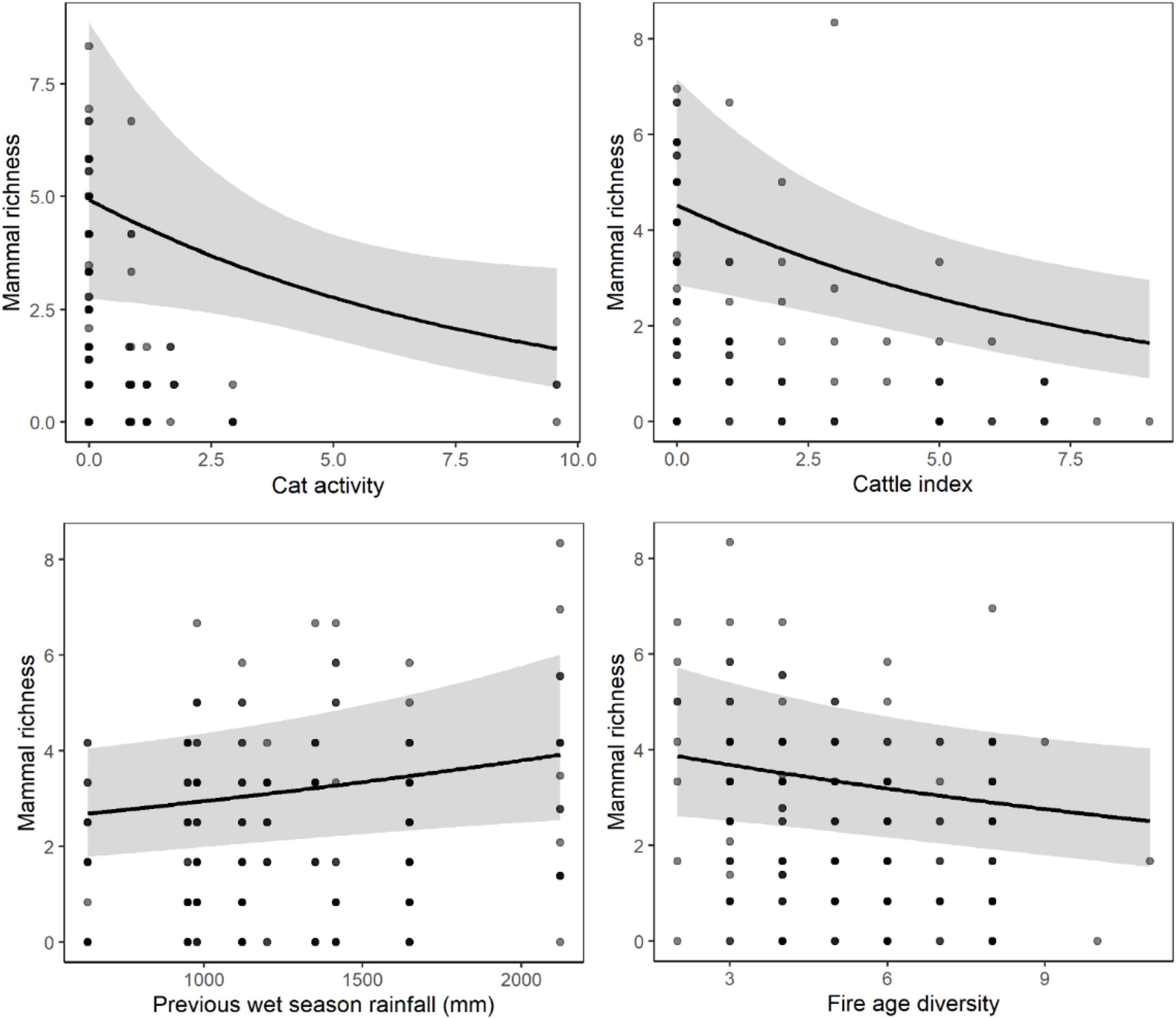
Figure 5. Mammal richness [species per 100 trap nights (tn)] in sandstone habitat shown only for highly influential predictor variables sampled at the 1, 5, and 10 km scale around each site (see Table 2). Relationships for each variable were derived from GLMM predictions while holding other explanatory variables constant at their median level. Model fit is shown by a black line and the gray band represents the 95% confidence interval. Gray circles indicate observed data values and are darker when repeated observations occur at the same point.
In volcanic habitats, the site-scale attributes most strongly associated with increased mammal richness were (1) habitat: rock cover, shrub cover, perennial grass cover, and negatively with annual grass (Sorghum stipoideum) cover (2) disturbance (negative relationships): proportion of the site burnt in the current year (Table 3). Fire mosaic attributes were not strong predictors of mammal richness at any scale (Table 3). The habitat model was the best model of mammal richness in volcanic habitats (Table 3 and Figure 6).
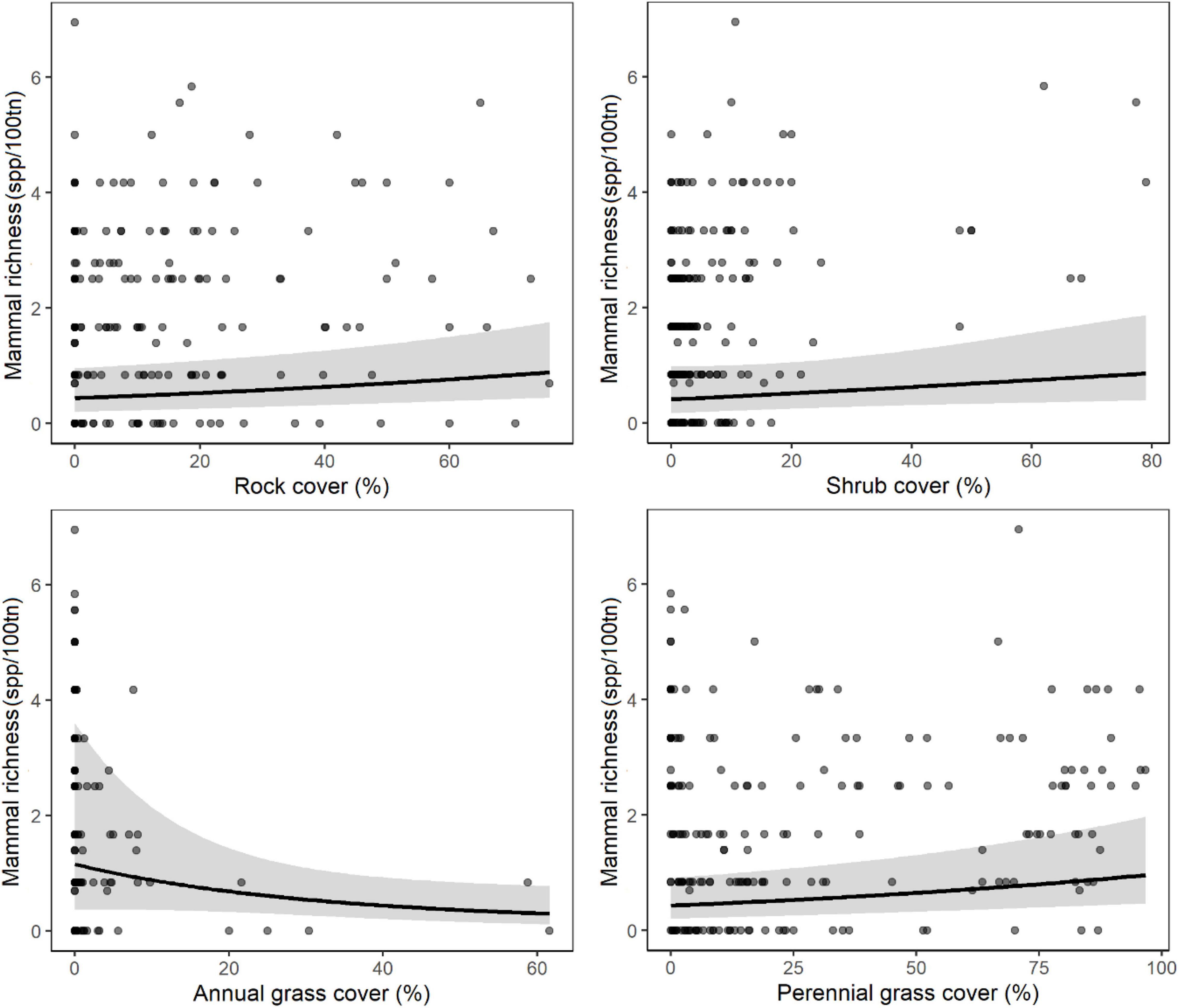
Figure 6. Mammal richness [species per 100 trap nights (tn)] in volcanic habitat shown only for highly influential predictor variables sampled at all scales around each site (see Table 2). Relationships for each variable were derived from GLMM predictions while holding other explanatory variables constant at their median level. Model fit is shown by a black line and the gray band represents the 95% confidence interval. Gray circles indicate observed data values and are darker when repeated observations occur at the same point.
Discussion
Our study supports the hypotheses that habitat values, both fixed (rock cover) and dynamic (perennial grass and shrub cover), disturbance (site-based feral cat activity, percentage of recently burnt habitat, feral livestock) are fundamentally significant in determining savanna mammal abundance and richness at the site scale. This supports previous work showing relationships between mammals and vegetation productivity (Stobo-Wilson et al., 2020a), shrub and grass cover (Radford et al., 2015; Davies et al., 2017; Penton et al., 2021), cat occupancy (Davies et al., 2017; Stobo-Wilson et al., 2020a), fire ‘activity’ or extent (Lawes et al., 2015; Radford et al., 2015; Stobo-Wilson et al., 2020a) and the presence of feral livestock (Legge et al., 2019). Our study also supported the hypothesis that site-scale disturbance and habitat features are more directly important to local mammal populations than broader landscape-scale (see above) or regional-scale (as per Radford et al., 2020a; Stobo-Wilson et al., 2020a) fire mosaic attributes. As far as we are aware this is the first study published in northern Australia which has explicitly tested for the importance of fire mosaic attributes at different spatial scales on threatened mammal assemblages. Finally our study identifies ≥ 4 years old unburnt habitat as the most important functional element (as per Parr and Andersen, 2006) of both site-scale and broad-scale fire mosaics for savanna mammals, not pyrodiversity per se. Such information has significant implications on how prescribed burning and other management actions should be applied at multiple spatial scales across northern Australian savanna landscapes.
The Positive Influence of Site-Scale Habitat Cover
Habitat cover at the site-scale was the most consistently identified attribute supporting mammal abundance and richness. The fixed mosaic habitat element, rock cover, was strongly supported in habitat and combined models for rocky sandstone habitats, and the abundance and richness of mammals in volcanic habitats. Rocks and rock crevices have been identified as critical habitat features supporting persistence of savanna mammals (Ibbett et al., 2017; Stobo-Wilson et al., 2020a) not least because feral cats have lower occupancy and hunt less effectively in rock habitats (McGregor et al., 2015; Hohnen et al., 2016b). Our study supports the importance of rocks as a key habitat attribute for threatened savanna mammals. Dynamic ground layer vegetation cover attributes (vegetation cover, perennial grass, and shrub cover) were also strongly supported in models for mammal richness and abundance in non-rocky, but high productivity, volcanic savannas. This corroborates Stobo-Wilson et al. (2020a) in finding that vegetation productivity was positively associated with mammal richness at broad scales except for when fires removed the cover. The importance of shrub cover as a model attribute for volcanic habitats also support previous findings for the threatened mammal Conilurus penicillatus in Melville Island savannas (Davies et al., 2017; Penton et al., 2021). The dynamic nature of ground layer vegetation highlights a key implied threat to savanna mammals in these open habitats. Total consumption of ground-layer vegetation after high intensity, extensive fires, has been shown to increase feral cat visits and hunting activity to burnt areas (McGregor et al., 2014, 2016), and result in elevated predation related mortality (Leahy et al., 2016), and the need for mammals to disperse and recolonize from remote refuge habitats in order to re-establish local populations (Shaw et al., 2021). A key feature of sites that retained mammal populations after fire were that they had been burnt in lower intensity, more patchy fires which retained local vegetation cover (Shaw et al., 2021). Such areas act as refugia for local mammals after extensive fires (Legge et al., 2008). As such it is crucial that prescribed burning for conservation outcomes in savannas retain frequent unburnt patches (ca. 2–20 ha), equivalent of threatened mammal home ranges (e.g., Oakwood, 2002; Pardon et al., 2003; Cook, 2010; Hohnen et al., 2015, 2016a; Leahy et al., 2016; Penton et al., 2020) throughout the burnt landscape if mammal populations are to persist at the local scale.
One dynamic ground layer vegetation attribute that had a negative relationship with savanna mammals was annual Sorghum grass cover. Unlike perennial grass, which spreads out and provides ground layer cover, annual Sorghum grass mostly grows vertically as a single very tall tiller (Weier et al., 2016) with very few leaves at ground level to provide cover. In this study, sites with high Sorghum cover (>10% projected canopy cover) had low mammal abundance and richness. Luckily Northern Kimberley savannas generally have relatively low Sorghum cover. Among our study sites, the mean annual Sorghum grass cover was 2% while perennial grass cover was 22% (Table 1). However, in other regions of northern Australia, savannas often have much greater annual Sorghum dominance (Russell-Smith et al., 2003a; Scott et al., 2010). Sorghum not only provides little cover for mammals, but it is also highly flammable once cured, leading to low patchiness and extensive fires even under milder fire weather conditions in the early dry season (Miles, 2020). Fire management which can reduce local fire frequency, will potentially also reduce Sorghum dominance (biomass, cover, seed set) and thereby benefit savanna mammals, through increasing competition from resprouting perennial grasses, shrubs and tree canopies (Radford and Fairman, 2015; Weier et al., 2018; Radford et al., 2021).
The Negative Influence of Site-Scale Disturbance – Feral Cats, Fires, and Cattle
Mammal abundance and richness was greatest where cats, fire extent and livestock disturbance was least. Cats are putatively the primary threat to savanna mammals in northern Australia (Johnson, 2006; Frank et al., 2014; Ziembicki et al., 2015; McGregor et al., 2016; Tuft et al., 2021). While feral cats are ubiquitous across the entire study region (Legge et al., 2017), the few survey sites where cats were recorded all had very low mammal abundance and richness compared to sites where cats were not recorded. This suggests a strong local influence of cat activity on mammal populations where cat activity is high. Stobo-Wilson et al. (2020b) found a negative relationship between vegetation productivity and cat occupancy, and these results are mirrored in our study with cat activity levels higher at low productivity (lower rainfall) sites (e.g., Mount Elizabeth, Solea Falls, Orchid Creek; Figure 1). High feral cat activity in savannas has also been linked with disturbance of ground layer vegetation either through frequent fire or extensive grazing (Davies et al., 2020; Penton et al., 2021). This is also reflected in this study, with feral cats more active at sites on one pastoral station (Mount Elizabeth) and sites subject to extensive wildfires during the study period (Solea Falls, Orchid Creek). Despite the failure of camera trapping to detect feral cats at most volcanic savanna sites, it is also likely that the overriding influence of habitat cover in these savannas relates directly to risk of predation by feral cats. Cats are known to have higher occupancy rates in open non-rocky savannas compared to rugged rocky savanna habitats (Hohnen et al., 2016b). Removal of vegetation ground cover in non-rocky savannas, through frequent fires or cattle disturbance, leads to much greater cat predation related mortality for savanna mammal species (McGregor et al., 2015; Leahy et al., 2016; Stobo-Wilson et al., 2020a,b).
Our study supports a predominantly negative relationship at the site-scale of fire disturbance on mammal abundance and richness. This is despite the positive influence of increased early dry season prescribed burning at regional scales in the same region (Radford et al., 2020a). The current study showed that at the site-scale, mammals were negatively associated with the extent of fire in the current year, with distance to unburnt habitat, and also with increased site fire frequency. These results mirror a number of previous studies from northern Australia showing predominantly negative influences of fires on savanna mammals (Andersen et al., 2005; Woinarski et al., 2010; Lawes et al., 2015; Radford et al., 2015; Stobo-Wilson et al., 2020a). However, the influence of early dry season prescribed burning at regional scales in modifying fire intensity, patchiness and extent at landscape and local scales may also allow greater site-scale vegetation cover to be maintained than under a late dry season wildfire regime dominated by high intensity, high consumption fires. Possibly the mechanism underlying mammal improvements at the regional-scale in the previous study (Radford et al., 2020a) was increased retention of habitat cover at the site-scale under managed compared to unmanaged fire regimes.
Despite previous studies reporting negative influences of cattle on savanna mammals in northern Australia (Legge et al., 2011, 2019; Radford et al., 2015; Davies et al., 2020; Mihailou and Massaro, 2021), cattle disturbance was associated with negative impacts in this study only for mammal richness in sandstone habitats. Surprisingly, the influence of cattle is detected only in sandstone habitats when these are known to have low carrying capacity for cattle in the Kimberley region (Speck et al., 1960). Volcanic woodlands are the only habitats considered suitable for cattle production in the Northern Kimberley (Speck et al., 1960). However, throughout much of the study period a cattle culling program was being undertaken which probably reduced cattle impacts on grass layer vegetation (Reid et al., 2020). One thing these results also highlight, however, is that despite cattle being at relatively low abundance in much of the study region, their impacts can still be high in low productivity sandstone sites where ground layer vegetation cover may take longer to recover. As shown in previous studies (Legge et al., 2011, 2019; Radford et al., 2015), our study highlights the need for ongoing cattle management if threatened mammal conservation is a priority for land owners.
Functional Local-Scale Up to Meta-Landscape-Scale Elements of Fire Mosaics
Combined models with both site- and broader-scale fire mosaic attributes emphasize that site-scale habitat and disturbance features are much more influential for savanna mammals than broader-scale fire mosaic elements. Site-scale attributes were strongly supported in all 16 combined habitat-disturbance-mosaic models. In contrast, only five models had broad-scaled mosaic attributes supported (Table 3). This emphasizes the importance of local-scale ecological processes in shaping savannas mammal population dynamics (Legge et al., 2008; Radford, 2012; Radford et al., 2015; Leahy et al., 2016; Shaw et al., 2021) over and above the over-arching influences of broader scale fire mosaic patterns from local up to landscape and regional scales (Lawes et al., 2015; Radford et al., 2020a; Stobo-Wilson et al., 2020a). The finding that site-scale, over broader landscape-scale factors, are more important for in situ persistence of mammal populations is also supported by recent studies from other biomes (Hale et al., 2021).
Nonetheless the results of this study highlight a number of key functional attributes of broader scale fire mosaics (as per Parr and Andersen, 2006) which do influence savanna mammal patterns. An important beneficial functional attribute of broader-scale fire mosaics verified in this study was the extent of ≥4-year old unburnt habitat (Table 2 and Figures 3, 5). Increasing unburnt habitat was beneficial for savanna mammals within 1 km of a site (local scale) in sandstone habitats and within 5–10 km of sites in volcanic savanna habitats (landscape and meta-landscape scales). The benefits of the presence within a fire mosaic of unburnt refuge habitat through multiple years at both local and landscape scales is likely to result from the ability of mammal populations to persist in association with unburnt habitat. Many mammal species use unburnt refugia in the short term following a fire (Legge et al., 2008). In addition, these refugia within broader frequently burnt landscapes facilitates lower predator related mortality (Leahy et al., 2016), local persistence of population and therefore greater population stability at the site- and local- and landscape-scales (Shaw et al., 2021). This result emphasizes further the importance of achieving persistence of longer unburnt vegetation (≥4 years) patches within savanna landscapes for the benefit of threatened mammal in northern Australia.
Mammal abundance in savannas on rocky sandstone was greatest after years with intermediate fire extent (ca. 25%) the previous year at both local and meta-local scales (≤3 km) within broader fire mosaics (Table 3 and Figure 3). This suggests that some burning at local/meta-local scales is beneficial for savanna mammals within sandstone habitats. However, given the benefits of unburnt habitat highlighted above, these benefits are probably derived through reduced total fire extent and retention of ground-layer vegetation cover at the site-scale due to protective networks of burnt patches, rather than due to the direct benefits of burnt habitat per se (though see Radford, 2012 for an example of benefits of burnt habitats for carnivous mammals). This suggests that benefits from prescribed burning mosaics (Radford et al., 2020a) might result more from the unburnt patches achieved at the site- and local/meta-local scale through low intensity burning, rather than some resource or habitat feature of the burnt habitat itself.
In their critique of the patch mosaic burning paradigm, Parr and Andersen (2006) and Andersen et al. (2014), emphasized the importance of functional elements within fire mosaics, rather than pyrodiversity (diversity of post-fire habitat age and fire frequency) per se. They argued that focus on diversity alone may cause conservation managers to miss crucial elements of mosaics needed by target biodiversity while focusing on superfluous (non-functional) mosaic elements. For instance, major variations in fire frequency within mosaics were found not to influence savanna ant assemblages, only very long unburnt habitat (>6 years) (Andersen et al., 2014). Our study provides another example, where pyrodiversity (diversity of post-fire age) at the local and meta-local scales (≤3 km from survey site) was negatively related to savanna mammal abundance and richness in sandstone habitats (Table 3 and Figure 4). This is likely related to pyrodiversity being directly correlated in this context with larger fire extent and therefore to less unburnt habitat. Either way, this finding is crucially important for conservation managers in showing that pyrodiversity per se (diversity of post-fire fuel ages) is not a useful target when applying prescribed burning management for target biodiversity – in this case because of a negative association with threatened savanna mammals. Instead, it is much more important for fire managers to focus on the establishment and deliberate retention of patches ≥ 4 years unburnt habitat through multiple years. The benefits therefore of establishing an intermediate extent (25%) of recent fire within mosaics, is in maintaining longer unburnt habitat refugia within the savanna matrix. Our study joins an increasing number of ecological studies which do NOT support a general finding that ‘pyrodiversity begets biodiversity’ (Jones and Tingley, 2021). Future studies need to move beyond simplistic ideas concerning pyrodiversity and biodiversity, and focus more on the key functional elements within fire mosaics which mechanistically support target species with particular ecological traits, if progress is to be made in use of prescribed fire mosaics for threatened species conservation.
Explanatory Habitat, Disturbance and Mosaic Features Not Supported
Dingoes have been postulated to have both positive and negative impacts on threatened savanna mammals in northern Australia. Stobo-Wilson et al. (2020a) found negative associations between savanna mammals and dingoes (postulated predation impacts). However there is also some evidence of positive effects of dingoes on savanna mammals via reductions in meso-predatory cat activity/occupancy (Kennedy et al., 2012; Leo et al., 2019). Our study provides no support for any influence of dingoes in our study area despite them being common throughout the region. The relative rockiness of Kimberley savanna could explain this as dingoes are negatively associated with increasing rockiness (Stobo-Wilson et al., 2020b).
Another hypothesis raised in some studies is that late dry season wildfires are much more damaging to savanna biodiversity and habitat, particularly mammals, and that low intensity prescribed burning in the early dry season can benefit mammals (Andersen et al., 2005; Lawes et al., 2015; Radford et al., 2020a, Radford et al., 2021). Despite this there was little support in our study for additional impacts of late dry season fires compared to early dry season fires. Neither fire intensity observed at the site-scale (which correlates with fire season) nor the proportion of late dry season fires within our broader-scale mosaics were supported in our models. Instead it was simply the extent of fire at site-scale and broader mosaic scales that influenced mammal abundance and richness. Late dry season fires may only be detrimental to savanna mammals (as per Radford et al., 2020a) because of their large extent and lack of patchiness compared to early dry season prescribed burns, rather than because of any inherent difference in the impacts of these fires on habitat/vegetation structure (Andersen, 2020). These findings emphasize the importance of prescribed fire in NOT removing key habitat cover values, rather than in any inherent value of the early dry season burnt habitat itself.
Management Implications
To implement fire management for explicit biodiversity conservation, we recommend the following:
Reduce fire extent via the application of judicious and strategic low-intensity prescribed burns (Andersen et al., 2005), which provide barriers to wildfires but maintains shrub and fruiting tree cover. This approach requires burning in the months where conditions (e.g., Fire Weather Index) is conducive to producing smaller, less intense fires (Perry et al., 2019).
Maintain more and larger patches (up to 5 km2) of longer unburnt vegetation (>4 years) across the landscape (Radford et al., 2020a) through the strategic application of prescribed fire (as above).
Reduce fire frequency by minimizing prescribed burning in areas that are naturally less fire-prone (Andersen et al., 2005). When implementing prescribed burning, use landscape features that maximize the stopping power of strategic fire scars (Fisher et al., 2021). Satellite-mapped fire histories, available from the North Australia and Rangelands Fire Information (see footnote 2) and Savanna Monitoring and Evaluation Framework3 websites, are particularly useful for identifying less fire-prone areas.
Finally, managers must implement concurrent conservation actions that reduce the impacts of feral livestock, cats and weeds to increase landscape productivity and maximize the benefits of fire management (Legge et al., 2019; Stobo-Wilson et al., 2020a).
We realize that such an approach across northern Australia’s vast and remote landscapes is both a formidable and expensive challenge. We note that significant fire regime benefits have been realized across some areas in the last ten years (e.g., Radford et al., 2020a; Edwards et al., 2021), but that further improvements are also required (e.g., Russell-Smith et al., 2017; Evans and Russell-Smith, 2019; Edwards et al., 2021).
Many of these improvements are being driven by emissions reductions schemes (‘savanna burning’) that incentivize the reduction of wildfires, and can provide the quantum of money needed to resource fire management for biodiversity conservation (Edwards et al., 2021). However, it is imperative that adequate and targeted monitoring, evaluation- and reporting are embedded within fire programs in an adaptive management context (see Corey et al., 2020) to better understand the biodiversity implications of fire management. Furthermore, fire management programs should have increasing patches of longer unburnt vegetation throughout the landscape as an explicit target for their management performance. Our results suggest that longer unburnt vegetation is more important than fire seasonality per se for threatened savanna mammals.
Data Availability Statement
The datasets presented in this study can be found in online repositories. The names of the repository/repositories and accession number(s) can be found below: https://naturemap.dbca.wa.gov.au.
Ethics Statement
The animal study was reviewed and approved by Department of Biodiversity, Conservation and Attractions Animal Ethics Committee.
Author Contributions
All authors listed have made a substantial, direct, and intellectual contribution to the work, and approved it for publication.
Conflict of Interest
The authors declare that the research was conducted in the absence of any commercial or financial relationships that could be construed as a potential conflict of interest.
Publisher’s Note
All claims expressed in this article are solely those of the authors and do not necessarily represent those of their affiliated organizations, or those of the publisher, the editors and the reviewers. Any product that may be evaluated in this article, or claim that may be made by its manufacturer, is not guaranteed or endorsed by the publisher.
Acknowledgments
We thank the Wunambal Gaambera, Dambimangari, Wilinggin, and Balanggarra Aboriginal Corporations for access to their Native Title lands, and the Uunguu, Wunggur, Dambimangari, and Balanggarra Rangers and Traditional Landowners for assistance during field surveys. We thank the Lacy (Mount Elizabeth) and Jane (Bachsten Creek) families for access to their respective properties. We also thank DBCA staff (R. Fairman, N. McKenzie, L. Baker, J. Hayward, G. Whelan, R. O’Donnell, G. Pearce, H. Corpus, B. Greatwich, D. Chemello, G. Murray, R. McPhail, and N. Godfrey) and numerous volunteers (H. Ricardo, J. Turpin, A. Loggins, L. Zhang, M. Olawsky, C. Davies, J. O’Connell, A. Stobo-Wilson, C. Penton, L. Ashburn, L. Ribeiro, L. Paolucci, and M. Taylor) for logistical support. The Department of Biodiversity, Conservation and Attractions provided funding to undertake the survey program. Finally, we thank the two reviewers whose comments improved this manuscript.
Footnotes
References
Abbott, I. (2002). Origin and spread of the cat, Felis catus, on mainland Australia, with a discussion of the magnitude of its early impact on native fauna. Wildl. Res. 29, 51–74. doi: 10.1071/wr01011
Andersen, A. N. (2020). Faunal responses to fire in Australian tropical savannas: insights from field experiments and their lessons for conservation management. Divers. Distrib. 27, 828–843. doi: 10.1111/ddi.13198
Andersen, A. N., Cook, G. D., Corbett, L. K., Douglas, M. M., Eager, R. W., Russell-Smith, J., et al. (2005). Fire frequency and biodiversity conservation in Australian tropical savannas: implications from the Kapalga fire experiment. Austral Ecol. 30, 155–167. doi: 10.1111/j.1442-9993.2005.01441.x
Andersen, A. N., Ribbons, R. R., Pettit, M., and Parr, C. L. (2014). Burning for biodiversity: highly resilient ant communities respond only to strongly contrasting fire regimes in Australia’s seasonal tropics. J. Appl. Ecol. 51, 1406–1413. doi: 10.1111/1365-2664.12307
Bradley, A. J., Kemper, C. M., Kitchener, D. J., Humphreys, W. F., and How, R. A. (1987). Small mammals of the Mitchell Plateau region, Kimberley, Western-Australia. Wildl. Res. 14, 397–413. doi: 10.1071/wr9870397
Braithwaite, R. W., and Muller, W. J. (1997). Rainfall, groundwater and refuges: predicting extinctions of Australian tropical mammal species. Aust. J. Ecol. 22, 57–67. doi: 10.1111/j.1442-9993.1997.tb00641.x
Brooks, M. E., Kristensen, K., van Benthem, K. J., Magnusson, A., Berg, C. W., Nielsen, A., et al. (2017). glmmTMB balances speed and flexibility among packages for zero-inflated generalized linear mixed modeling. R J. 9, 378–400. doi: 10.32614/rj-2017-066
Burnham, K. P., and Anderson, D. R. (2002). Model Selection and Multimodel Inference: A Practical Information-Theoretic Approach. New York: Springer-Verlag.
Connor, S. E., Schneider, L., Trezise, J., Rule, S., Barrett, R. L., Zawadzki, A., et al. (2018). Forgotten impacts of European land-use on riparian and savanna vegetation in northwest Australia. J. Veg. Sci. 29, 427–437. doi: 10.1111/jvs.12591
Cook, A. (2010). Habitat Use and Home-Range of the Northern Quoll, Dasyurus hallucatus: Effects of Fire. Master’s Thesis. Crawley WA: University of Western Australia.
Corey, B., Andersen, A. N., Legge, S., Woinarski, J. C., Radford, I. J., and Perry, J. J. (2020). Better biodiversity accounting is needed to prevent bioperversity and maximize co-benefits from savanna burning. Conserv. Lett. 13:e12685.
Davies, H. F., Maier, S. W., and Murphy, B. P. (2020). Feral cats are more abundant under severe disturbance regimes in an Australian tropical savanna. Wildl. Res. 47, 624–632. doi: 10.1071/wr19198
Davies, H. F., McCarthy, M. A., Firth, R. S., Woinarski, J. C., Gillespie, G. R., Andersen, A. N., et al. (2017). Top-down control of species distributions: feral cats driving the regional extinction of a threatened rodent in northern Australia. Divers. Distrib. 23, 272–283. doi: 10.1111/ddi.12522
Dickman, C. R. (1996). Overview of the Impacts of Feral Cats on Australian Native Fauna. Canberra, NSW: Australian Nature Conservation Agency, 1–92.
Edwards, A., Archer, R., De Bruyn, P., Evans, J., Lewis, B., Vigilante, T., et al. (2021). Transforming fire management in northern Australia through successful implementation of savanna burning emissions reductions projects. J. Environ. Manage. 290:112568. doi: 10.1016/j.jenvman.2021.112568
Einoder, L. D., Southwell, D. M., Lahoz-Monfort, J. J., Gillespie, G. R., Fisher, A., and Wintle, B. A. (2018). Occupancy and detectability modelling of vertebrates in northern Australia using multiple sampling methods. PLoS One 13:e0203304. doi: 10.1371/journal.pone.0203304
Evans, J., and Russell-Smith, J. (2019). Delivering effective savanna fire management for defined biodiversity conservation outcomes: an Arnhem Land case study. Int. J. Wildland Fire 29, 386–400. doi: 10.1071/wf18126
Fisher, R., Heckbert, S., and Garnett, S. (2021). Reframing wildfire simulations for understanding complex human–landscape interactions in cross-cultural contexts: a case study from Northern Australia. Fire 4:46. doi: 10.3390/fire4030046
Frank, A. S., Johnson, C. N., Potts, J. M., Fisher, A., Lawes, M. J., Woinarski, J. C., et al. (2014). Experimental evidence that feral cats cause local extirpation of small mammals in Australia’s tropical savannas. J. Appl. Ecol. 51, 1486–1493. doi: 10.1111/1365-2664.12323
Gelman, A. (2008). Scaling regression inputs by dividing by two standard deviations. Stat. Med. 27, 2865–2873. doi: 10.1002/sim.3107
Hale, S., Mendoza, L., Yeatman, T., Cooke, R., Doherty, T., Nimmo, D., et al. (2021). Evidence that post-fire recovery of small mammals occurs primarily via in situ survival. Divers. Distrib. 1–13. doi: 10.1111/ddi.13283
Hartig, F. (2021). DHARMa: Residual Diagnostics for Hierarchical (Multi-Level / Mixed) Regression Models. R Package Version 0.4.4.
Hohnen, R., Tuft, K. D., Legge, S., Radford, I. J., Carver, S., and Johnson, C. N. (2015). Post-fire habitat use of the golden-backed tree-rat (Mesembriomys macrurus) in the northwest Kimberley, Western Australia. Austral Ecol. 40, 941–952. doi: 10.1111/aec.12278
Hohnen, R., Tuft, K., Legge, S., Walters, N., Johanson, L., Carver, S., et al. (2016a). The significance of topographic complexity in habitat selection and persistence of a declining marsupial in the Kimberley region of Western Australia. Aust. J. Zool. 64, 198–216. doi: 10.1071/zo16015
Hohnen, R., Tuft, K., McGregor, H. W., Legge, S., Radford, I. J., and Johnson, C. N. (2016b). Occupancy of the invasive feral cat varies with habitat complexity. PLoS One 11:e0152520. doi: 10.1371/journal.pone.0152520
Ibbett, M., Woinarski, J. C. Z., and Oakwood, M. (2017). Declines in the mammal assemblage of a rugged sandstone environment in Kakadu National Park, Northern Territory, Australia. Aust. Mammal. 40, 181–187. doi: 10.1071/am17011
Johnson, C. (2006). Australia’s Mammal Extinctions: A 50,000-Year History. Cambridge: Cambridge University Press.
Jones, G. M., and Tingley, M. W. (2021). Pyrodiversity and biodiversity: a history, synthesis, and outlook. Divers. Distrib. 1–18. doi: 10.1111/ddi.13280
Kennedy, M., Phillips, B. L., Legge, S., Murphy, S. A., and Faulkner, R. A. (2012). Do dingoes suppress the activity of feral cats in northern Australia? Austral Ecol. 37, 134–139. doi: 10.1111/j.1442-9993.2011.02256.x
Lawes, M. J., Murphy, B. P., Fisher, A., Woinarski, J. C., Edwards, A. C., and Russell-Smith, J. (2015). Small mammals decline with increasing fire extent in northern Australia: evidence from long-term monitoring in Kakadu National Park. Int. J. Wildland Fire 24, 712–722. doi: 10.1071/wf14163
Leahy, L., Legge, S. M., Tuft, K., McGregor, H. W., Barmuta, L. A., Jones, M. E., et al. (2016). Amplified predation after fire suppresses rodent populations in Australia’s tropical savannas. Wildl. Res. 42, 705–716. doi: 10.1071/wr15011
Legge, S., Kennedy, M. S., Lloyd, R. A. Y., Murphy, S. A., and Fisher, A. (2011). Rapid recovery of mammal fauna in the central Kimberley, northern Australia, following the removal of introduced herbivores. Austral Ecol. 36, 791–799. doi: 10.1111/j.1442-9993.2010.02218.x
Legge, S., Murphy, B. P., McGregor, H., Woinarski, J. C., Augusteyn, J., Ballard, G., et al. (2017). Enumerating a continental-scale threat: how many feral cats are in Australia? Biological Conserv. 206, 293–303. doi: 10.1016/j.biocon.2016.11.032
Legge, S., Murphy, S., Heathcote, J., Flaxman, E., Augusteyn, J., and Crossman, M. (2008). The short-term effects of an extensive and high-intensity fire on vertebrates in the tropical savannas of the central Kimberley, northern Australia. Wildl. Res. 35, 33–43. doi: 10.1071/wr07016
Legge, S., Smith, J. G., James, A., Tuft, K. D., Webb, T., and Woinarski, J. C. (2019). Interactions among threats affect conservation management outcomes: livestock grazing removes the benefits of fire management for small mammals in Australian tropical savannas. Conserv. Sci. Pract. 1:e52.
Leo, V., Reading, R. P., Gordon, C., and Letnic, M. (2019). Apex predator suppression is linked to restructuring of ecosystems via multiple ecological pathways. Oikos 128, 630–639. doi: 10.1111/oik.05546
Lindsey, A. A., Barton, J. D., and Miles, S. R. (1958). Field efficiencies of forest sampling methods. Ecology 39, 428–444. doi: 10.2307/1931752
McGregor, H. W., Legge, S., Jones, M. E., and Johnson, C. N. (2014). Landscape management of fire and grazing regimes alters the fine-scale habitat utilisation by feral cats. PLoS One 9:e109097. doi: 10.1371/journal.pone.0109097
McGregor, H. W., Legge, S., Jones, M. E., and Johnson, C. N. (2016). Extraterritorial hunting expeditions to intense fire scars by feral cats. Sci. Rep. 6:22559. doi: 10.1038/srep22559
McGregor, H., Legge, S., Jones, M. E., and Johnson, C. N. (2015). Feral cats are better killers in open habitats, revealed by animal-borne video. PLoS One 10:e0133915. doi: 10.1371/journal.pone.0133915
McKenzie, N. L., Burbidge, A. A., Baynes, A., Brereton, R. N., Dickman, C. R., Gordon, G., et al. (2007). Analysis of factors implicated in the recent decline of Australia’s mammal fauna. J. Biogeogr. 34, 597–611.
Mihailou, H., and Massaro, M. (2021). An overview of the impacts of feral cattle, water buffalo and pigs on the savannas, wetlands and biota of northern Australia. Austral Ecol. 46, 699–712.
Miles, G. (2020). The Flames of Convenience: Early Dry Season Burning on Trial in Kakadu and the Western Top End of the Northern Territory. Port Adelaide, SA: Green Hill Publishing.
Moseby, K. E., Hill, B. M., and Read, J. L. (2009). Arid recovery–a comparison of reptile and small mammal populations inside and outside a large rabbit, cat and fox-proof exclosure in arid South Australia. Austral Ecol. 34, 156–169. doi: 10.1111/j.1442-9993.2008.01916.x
Oakwood, M. (2002). Spatial and social organization of a carnivorous marsupial Dasyurus hallucatus (Marsupialia: Dasyuridae). J. Zool. 257, 237–248. doi: 10.1017/s0952836902000833
Pardon, L. G., Brook, B. W., Griffiths, A. D., and Braithwaite, R. W. (2003). Determinants of survival for the northern brown bandicoot under a landscape-scale fire experiment. J. Anim. Ecol. 72, 106–115. doi: 10.1046/j.1365-2656.2003.00686.x
Parr, C. L., and Andersen, A. N. (2006). Patch mosaic burning for biodiversity conservation: a critique of the pyrodiversity paradigm. Conserv. Biol. 20, 1610–1619. doi: 10.1111/j.1523-1739.2006.00492.x
Penton, C. E., Woolley, L. A., Radford, I. J., and Murphy, B. P. (2020). Overlapping den tree selection by three declining arboreal mammal species in an Australian tropical savanna. J. Mammal. 101, 1165–1176. doi: 10.1093/jmammal/gyaa074
Penton, C. E., Davies, H. F., Radford, I. J., Woolley, L.-A., Rangers, T. L., and Murphy, B. P. (2021). A hollow argument: understory vegetation and disturbance determine abundance of hollow-dependent mammals in an Australian tropical savanna. Front. Ecol. Evol. 9:739550. doi: 10.3389/fevo.2021.739550
Perry, J. J., Cook, G. D., Graham, E., Meyer, C. M., Murphy, H. T., and VanDerWal, J. (2019). Regional seasonality of fire size and fire weather conditions across Australia’s northern savanna. Int. J. Wildland Fire 29, 1–10.
R Core Team (2021). R: A Language and Environment For Statistical Computing. Vienna: R Foundation for Statistical Computing.
Radford, I. J. (2012). Threatened mammals become more predatory after small-scale prescribed fires in a high-rainfall rocky savanna. Austral Ecol. 37, 926–935.
Radford, I. J., and Fairman, R. (2015). Fauna and vegetation responses to fire and invasion by toxic cane toads (Rhinella marina) in an obligate seeder dominated tropical savanna in the Kimberley, Northern Australia. Wildl. Res. 42, 302–314. doi: 10.1071/wr14259
Radford, I. J., Dickman, C. R., Start, A. N., Palmer, C., Carnes, K., Everitt, C., et al. (2014). Mammals of Australia’s tropical savannas: a conceptual model of assemblage structure and regulatory factors in the Kimberley region. PloS one 9:e92341. doi: 10.1371/journal.pone.0092341
Radford, I. J., Gibson, L. A., Corey, B., Carnes, K., and Fairman, R. (2015). Influence of fire mosaics, habitat characteristics and cattle disturbance on mammals in fire-prone savanna landscapes of the northern Kimberley. PLoS One 10:e0130721. doi: 10.1371/journal.pono.0130721
Radford, I. J., Oliveira, S. L. J., Byrne, B., and Woolley, L. A. (2021). Tree hollow densities reduced by frequent late dry-season wildfires in threatened Gouldian finch (Erythrura gouldiae) breeding habitat. Wildl. Res. 48, 511–520. doi: 10.1071/wr20108
Radford, I. J., Woolley, L. A., Corey, B., Hatherley, E., Vigilante, T., and Wunambal Gaambera Aboriginal Corporation (2020a). Prescribed burning benefits threatened mammals in northern Australia. Biodivers. Conserv. 29, 2985–3007. doi: 10.1371/journal.pone.0130721
Radford, I. J., Woolley, L. A., Dickman, C. R., Corey, B., Trembath, D., and Fairman, R. (2020b). Invasive anuran driven trophic cascade: an alternative hypothesis for recent critical weight range mammal collapses across northern Australia. Biol. Invasions 22, 1–16.
Reid, A. M., Murphy, B. P., Vigilante, T., and Wunambal Gaambera Aboriginal Corporation, and Bowman, D. M. (2020). Distribution and abundance of large herbivores in a northern Australian tropical savanna: a multi-scale approach. Austral Ecol. 45, 529–547. doi: 10.1111/aec.12860
Richards, S. A. (2005). Testing ecological theory using the information-theoretic approach: examples and cautionary results. Ecology 86, 2805–2814. doi: 10.1890/05-0074
Russell-Smith, J., and Edwards, A. C. (2006). Seasonality and fire severity in savanna landscapes of monsoonal northern Australia. Int. J. Wildland Fire 15, 541–550. doi: 10.1071/wf05111
Russell-Smith, J., Evans, J., Edwards, A. C., and Simms, A. (2017). Assessing ecological performance thresholds in fire-prone Kakadu National Park, northern Australia. Ecosphere 8:e01856. doi: 10.1002/ecs2.1856
Russell-Smith, J., Yates, C., Edwards, A., Allan, G. E., Cook, G. D., Cooke, P., et al. (2003b). Contemporary fire regimes of northern Australia, 1997–2001: change since Aboriginal occupancy, challenges for sustainable management. Int. J. Wildland Fire 12, 283–297. doi: 10.1071/wf03015
Russell-Smith, J., Whitehead, P. J., Cook, G. D., and Hoare, J. L. (2003a). Response of Eucalyptus-dominated savanna to frequent fires: lessons from Munmarlary, 1973–1996. Ecol. Monogr. 73, 349–375. doi: 10.1890/01-4021
Scott, K. A., Setterfield, S. A., Douglas, M. M., and Andersen, A. N. (2010). Environmental factors influencing the establishment, height and fecundity of the annual grass Sorghum intrans in an Australian tropical savanna. J. Trop. Ecol. 26, 313–322.
Shaw, R. E., James, A. I., Tuft, K., Legge, S., Cary, G. J., Peakall, R., et al. (2021). Unburnt habitat patches are critical for survival and in situ population recovery in a small mammal after fire. J. Appl. Ecol. 58, 1325–1335. doi: 10.1111/1365-2664.13846
Speck, N. H., Bradley, J., Lazarides, M., Twidale, C. R., Slatyer, R. O., Stewart, G. A., et al. (1960). No. 4 the lands and pastoral resources of the North Kimberley Area, WA. CSIRO Land Res. Surv. 2010, 1–116. doi: 10.1071/lrs04
Start, A. N., Burbidge, A. A., McDowell, M. C., and McKenzie, N. L. (2012). The status of non-volant mammals along a rainfall gradient in the south-west Kimberley, Western Australia. Aust. Mammal. 34, 36–48. doi: 10.1071/am10026
Start, A. N., Burbidge, A. A., McKenzie, N. L., and Palmer, C. A. R. O. L. (2007). The status of mammals in the north Kimberley, Western Australia. Aust. Mammal. 29, 1–16. doi: 10.1071/am07001
Stobo-Wilson, A. M., Stokeld, D., Einoder, L. D., Davies, H. F., Fisher, A., Hill, B. M., et al. (2020a). Bottom-up and top-down processes influence contemporary patterns of mammal species richness in Australia’s monsoonal tropics. Biol. Conserv. 247:108638. doi: 10.1016/j.biocon.2020.108638
Stobo-Wilson, A. M., Stokeld, D., Einoder, L. D., Davies, H. F., Fisher, A., Hill, B. M., et al. (2020b). Habitat structural complexity explains patterns of feral cat and dingo occurrence in monsoonal Australia. Divers. Distrib. 26, 832–842. doi: 10.1111/ddi.13065
Tuft, K., Legge, S., Anke, S. K., Frank, A. S. K., James, A. I., May, T., et al. (2021). Cats are a key threatening factor to the survival of local populations of native small mammals in Australia’s tropical savannas: evidence from translocation trials with Rattus tunneyi. Wildl. Res. 48, 654–662. doi: 10.1071/wr20193
Weier, A., Radford, I. J., Oliveira, S. L., and Lawes, M. J. (2016). Recently but infrequently burnt breeding sites are favoured by threatened Gouldian finches (Erythrura gouldiae). Int. J. Wildland Fire 25, 1281–1290. doi: 10.1071/WF16105
Weier, A., Radford, I. J., Woolley, L. A., and Lawes, M. J. (2018). Fire regime effects on annual grass seeds as food for threatened grass-finch. Fire Ecol. 14:8. doi: 10.1186/s42408-018-0019-3
Woinarski, J. C. Z., Legge, S., Fitzsimons, J. A., Traill, B. J., Burbidge, A. A., Fisher, A., et al. (2011). The disappearing mammal fauna of northern Australia: context, cause, and response. Conserv. Lett. 4, 192–201. doi: 10.1111/j.1755-263x.2011.00164.x
Woinarski, J. C. Z., Milne, D. J., and Wanganeen, G. (2001). Changes in mammal populations in relatively intact landscapes of Kakadu National Park, Northern Territory, Australia. Austral Ecol. 26, 360–370. doi: 10.1046/j.1442-9993.2001.01121.x
Woinarski, J. C., Armstrong, M., Brennan, K., Fisher, A., Griffiths, A. D., Hill, B., et al. (2010). Monitoring indicates rapid and severe decline of native small mammals in Kakadu National Park, northern Australia. Wildl. Res. 37, 116–126. doi: 10.1071/wr09125
Woinarski, J., Burbidge, A., and Harrison, P. (2014). The Action Plan for Australian Mammals 2012. Clayton, VIC: Commonwealth Scientific and Industrial Research Organization Publishing (CSIRO Publishing).
Woolley, L. A., Murphy, B. P., Radford, I. J., Westaway, J., and Woinarski, J. C. (2018). Cyclones, fire, and termites: the drivers of tree hollow abundance in northern Australia’s mesic tropical savanna. For. Ecol. Manage. 419, 146–159.
Ziembicki, M. R., Woinarski, J. C., Webb, J. K., Vanderduys, E., Tuft, K., Smith, J., et al. (2015). Stemming the tide: progress towards resolving the causes of decline and implementing management responses for the disappearing mammal fauna of northern Australia. Therya 6, 169–225. doi: 10.12933/therya-15-236
Keywords: critical weight range mammals, conceptual models, feral cats, fire mosaics, feral livestock, explanatory variables, population dynamics
Citation: Radford IJ, Corey B, Carnes K, Shedley E, McCaw L and Woolley L-A (2021) Landscape-Scale Effects of Fire, Cats, and Feral Livestock on Threatened Savanna Mammals: Unburnt Habitat Matters More Than Pyrodiversity. Front. Ecol. Evol. 9:739817. doi: 10.3389/fevo.2021.739817
Received: 12 July 2021; Accepted: 25 October 2021;
Published: 25 November 2021.
Edited by:
Christopher Richard Dickman, The University of Sydney, AustraliaReviewed by:
Viorel Dan Popescu, Ohio University, United StatesPeter Mcdonald, Northern Territory Government, Australia
Copyright © 2021 Radford, Corey, Carnes, Shedley, McCaw and Woolley. This is an open-access article distributed under the terms of the Creative Commons Attribution License (CC BY). The use, distribution or reproduction in other forums is permitted, provided the original author(s) and the copyright owner(s) are credited and that the original publication in this journal is cited, in accordance with accepted academic practice. No use, distribution or reproduction is permitted which does not comply with these terms.
*Correspondence: Ian J. Radford, ian.radford@dbca.wa.gov.au