- 1Institute of Evolution and Ecology, School of Life Sciences, Central China Normal University, Wuhan, China
- 2Behavioral and Physiological Ecology, Groningen Institute for Evolutionary Life Sciences (GELIFES), Faculty of Science and Engineering, University of Groningen, Groningen, Netherlands
Life-history theory predicts that increased resource allocation in current reproduction comes at the cost of survival and future reproductive fitness. In taxa with biparental care, each parent can adjust investment on current reproduction according to changes in their partner’s effort, but these adjustments may be different for males and females as they may have different reproductive strategies. Numerous theoretical and empirical studies have proposed the mechanism underlying such adjustments. In addition, the value of the brood or litter (brood size) has also been suggested to affect the amount of care through manipulation of brood size. While the two conditions have been studied independently, the impact of their interplay on potential sex-dependent future reproductive performance remains largely unknown. In this study, we simultaneously manipulated both care system (removal of either parent vs. no removal) and brood size in a burying beetle (Nicrophorus vespilloides) to understand their joint effect on reproductive allocation and trade-off between current and future reproduction. Our results show that males compensated for mate loss by significantly increasing the level of care regardless of brood size, while females exhibited such compensation only for small brood size. Additionally, with an increase in allocation to current reproduction, males showed decreased parental investment during the subsequent breeding event as a pair. These findings imply a dual influence of parental care system and brood size on allocation in current reproduction. Moreover, the impact of such adjustments on sex-dependent differences in future reproduction (parental care, larvae number, and average larval mass at dispersal) is also demonstrated. Our findings enhance the understanding of sex roles in parental investment and highlight their importance as drivers of reproductive allocation.
Introduction
Life-history theory predicts that individuals trade off the reproductive investment between current and future reproduction, because an increased allocation to the current breeding attempts means fewer resources available to future breeding attempts (Williams, 1966; Reznick, 1985; Stearns, 1989; Clutton-Brock, 1991; Wolf et al., 2007). Under the constraints of time and energy, an increase in current parental effort can incur future fitness costs, such as reduced survival or reproductive performance. To maximize lifetime reproductive success, each parent should therefore adjust its investment allocation between current and future reproduction (Rivalan et al., 2005; Creighton et al., 2009; Bleu et al., 2013). In species with biparental care, the net result of this reproductive trade-off is sexual conflict over parental effort between parents as the fitness benefits depend on the combined effort of both parents, whereas the fitness costs are determined by individual effort (Parker, 2006; Szentirmai et al., 2007; Harrison et al., 2009; Royle et al., 2010; Lessells, 2012; Bebbington and Hatchwell, 2016). Each parent is expected to minimize its own current investment by shifting as much of the workload as possible over to its partner (Trivers, 1972; Lessells, 1999; Houston et al., 2005; Szentirmai et al., 2007; Harrison et al., 2009; Royle et al., 2010). The amount of contribution a parent provides to current reproduction is influenced by their partner’s effort (Markman et al., 1996; Sanz et al., 2000; Houston et al., 2005; Johnstone and Hinde, 2006; Harris and Uller, 2009; David et al., 2015). These adjustments may be different for males and females as they may have different reproductive strategies which include different resource allocation, mate selection, and parenting patterns due to sex-dependent pay-offs of certain reproductive behaviors (Gross, 1996; Sanz et al., 2000; Kotiaho and Simmons, 2003; Hoffman et al., 2008). Theoretically, how parents respond to changes in their partner’s effort depends on different evolutionarily stable strategies which include sealed-bid, negotiation (partial and full compensation) and matching strategy (Houston and Davies, 1985; McNamara et al., 1999; Johnstone and Hinde, 2006). Sealed-bid strategy assumes that parents do not respond to their partner’s investment changes, as they make an initial fixed decision about how much investment to provide (Houston and Davies, 1985). In contrast, negotiation and matching strategies propose that parents adjust their investment to their partners’ contribution (Johnstone and Hinde, 2006). Negotiation occurs when parents partially or fully compensate for the reduced care provided by their partners by increasing their effort (McNamara et al., 1999), whereas matching predicts that parents adjust their care to their partners’ contributions by matching any increase or reduction in the same direction (Johnstone and Hinde, 2006). Several studies have tested how parents adjust their contribution based on changes of their partner’s effort by handicapping or removing one parent and then assessing the response of the other parent. These studies showed mixed results: sealed-bid [house sparrow (Passer domesticus), Schwagmeyer et al., 2002; Nakagawa et al., 2007], negotiation [partial compensation: burying beetle (Nicrophorus vespilloides), Smiseth et al., 2005; burying beetle (N. orbicollis), Rauter and Moore, 2004; full compensation: great tit (Parus major), Sanz et al., 2000; magnificent frigatebird (Fregata magnificens), Osorno and Székely, 2004], and matching strategy [great tit, Hinde, 2006; zebra finch (Taeniopygia guttata), Mariette and Griffith, 2012, 2015; blue tits (Cyanistes caeruleus), Iserbyt et al., 2019] have all been found. Different strategies have also been found within the same species (great tit (P. major), full compensation and matching strategy) (Sanz et al., 2000; Hinde, 2006). Meanwhile, males and females respond differently to variation in their partner’s effort because of sex differences in reproductive strategies. Some studies show that males increase their investment in offspring provisioning after female removal, whereas females show no response to partner removal (Rauter and Moore, 2004; Suzuki and Nagano, 2009; Creighton et al., 2015; Cantarero et al., 2019). It is because females typically provide more care than males, and they may work to their physical limitations and there is no room to intensify the provisioning rates [e.g., burying beetle (Nicrophorus spp.), Rauter and Moore, 2004; Suzuki and Nagano, 2009; Creighton et al., 2015; rock sparrow (Petronia petronia), Cantarero et al., 2019]. However, other studies suggest that females compensate for their partner’s decrease in feeding rate, while males tend to do less because males focus more on the territory defense and lack confidence in paternity (great tit, Sanz et al., 2000).
In addition, brood or litter size may also affect the amount of care, as has been shown through manipulations of brood size. Parents adjust their effort by increasing their investment with increased brood sizes and decreasing it with decreased brood sizes (Wright and Cuthill, 1990; Sanz, 2001; Parejo and Danchin, 2006; Komdeur et al., 2007; Kokko and Jennions, 2008; Low et al., 2012; David et al., 2015; Griffioen et al., 2019). Although some parents can fully compensate for the effect of enlarged brood size by providing food at higher rates (Neuenschwander et al., 2003; García-Navas and Sanz, 2010), they may do so at a cost. It has been suggested that brood size manipulation affects future reproduction as enlarged broods decrease the survival of parents and the probability to produce subsequent broods (Tinbergen and Boerlijst, 1990; Parejo and Danchin, 2006). Several theoretical studies have been developed to explain the causes of parent-offspring conflict for parental care (Trivers, 1974; Godfray, 1995; Kilner and Hinde, 2012). These studies show that brood size has the potential to affect the allocation of resources between current and future reproduction, and the relative contribution of each sex to parental effort. Previous work has reported that there can be a sex difference in response to brood manipulation. In some studies, males increase their parental investment when brood size increases, whereas females do not seem as responsive as males to increases in brood size (MacGregor and Cockburn, 2002; Mock et al., 2005; Nakagawa et al., 2007; Low et al., 2012). However, others suggest that males show less increase in feeding when raising enlarged broods or more decrease in feeding when raising reduced broods than females (Ardia, 2007). That is, the allocation to current reproduction may be influenced both by partner’s effort and by brood size, and these adjustments in parental effort may be different for males and females as they have different reproductive strategies. Additionally, the evidence for the trade-off between current and future reproduction is mixed. Previous work on reproductive trade-offs have manipulated traits that affect levels of parental effort to current reproduction (such as brood size or clutch size) and then assessing the induced changes in future survival, life span, or reproductive performance. Some studies have found that increased allocation to current reproduction leads to a future cost, such as lower body condition (Ratz and Smiseth, 2018), lower fecundity (Billman et al., 2014), shorter life span (Daan et al., 1996), or reduced competitive ability over food resources (Richardson et al., 2020), whereas other studies failed to demonstrate the predicted trade-off relationships in future breeding attempts (Roff and Fairbairn, 2007; Santos and Nakagawa, 2012; Ratz et al., 2020). Several explanations have been proposed for whether the trade-off between current and future reproduction are predictable or not. One such explanation is that this results from sex differences in allocation strategies. Considering that males and females of most species have different reproductive strategies and differ significantly in their cost of reproduction (Trivers, 1972; Santos and Nakagawa, 2012), because, for example, females provide more care than males while males invest further in obtaining additional mating opportunities (Queller, 1997), males and females may thus evolve distinct parental allocation strategies.
Although we have a good understanding of the impacts of partner’s effort and brood size manipulations on the parental effort of each sex separately, little is known about their joint effects. In addition, males and females respond to partner’s effort and brood size differently and thus may exhibit different allocation strategies. In order to test the combined effects of partner’s effort and brood size on reproductive allocation between current and future reproduction, we use the burying beetle (N. vespilloides) in which we experimentally manipulated brood size and parental care system (experimental removal of a parent of either sex or no removal). To our knowledge, this is the first study to provide experimental evidence for the combined effects of parental care system and brood size on parental investment and reproductive trade-off between current and future reproduction.
Burying beetles (Nicrophorus spp.) are excellent model for studying the combined effects of flexible parental care system and parental investment due to brood size on reproductive trade-offs, because both uniparental and biparental care occurs and brood size is highly variable (Eggert et al., 1998; Scott, 1998; Ratz and Smiseth, 2018; Woelber et al., 2018). In these species, extended parental care is provided, consisting of the carcass maintenance stage and the larvae provisioning stage (Fetherston et al., 1990; Scott, 1990, 1998; Müller et al., 1998). Both parents prepare the carcass by burying it, removing any hair or feather from it, rounding it into a ball, and preserving it with anti-microbial secretions (Rozen et al., 2008; Trumbo, 2017). Typically, females spend more time provisioning larvae and care for longer than males, whereas males are more involved in carcass maintenance and defense, and desert the brood earlier than females because they have greater residual reproductive value than females (Bartlett, 1988; Smiseth and Moore, 2004; Ward et al., 2009; Ratz and Smiseth, 2018). The carcass serves as the sole source of food for both parents and larvae during breeding (Scott, 1998; Trumbo and Xhihani, 2015; Pilakouta et al., 2016), thus female burying beetles benefit from male desertion by feeding more from the carcass (Boncoraglio and Kilner, 2012). Both parents gain weight during the entire breeding, and the weight gained can serve as a proxy for investment in future reproduction (Creighton et al., 2009; Billman et al., 2014; Pilakouta et al., 2016; Richardson et al., 2020). Likewise, the weight loss during the larvae provisioning is an indicator of the reproductive investment to current reproduction (Trumbo and Xhihani, 2015). Prior work shows that both male and female beetles respond to brood size manipulations by increasing their parental care toward enlarged broods (Rauter and Moore, 2004). However, male but not female provisioning increased with brood size when caring for very large broods (Rauter and Moore, 2004; Smiseth and Moore, 2004). The difference in how males and females respond to brood size manipulation is in line with the difference in how males and females respond to the partner removal. Studies on several species of burying beetles have also found that males and females respond differently to partner removal. Males adjust their efforts to partner removal, while females generally show no response because they typically provide more care than males and their degree of compensation behavior is limited (Rauter and Moore, 2004; Smiseth and Moore, 2004; Smiseth et al., 2005; Suzuki and Nagano, 2009; Creighton et al., 2015). These findings suggest that there might be interaction between the effects of partner’s presence and brood size on the amount of parental investment provided by each sex, yet previous studies did not allow us to tease apart these effects.
In this study, we aim to examine (i) how each sex adjusts its parental investment based on its partner’s presence, brood size and their combined effects, (ii) whether the adjustments incur a sex difference in reproductive trade-offs. For the first question, we predicted that uniparental parents provide more care to the brood than biparental parents and that parents provide more care to larger broods than to smaller ones. We also expected an effect of the interaction between partner’s presence and brood size on the amount of parental care provided by each sex. We predicted that males may compensate for mate removal regardless of brood size, whereas females show no response to mate loss when raising a large brood compared to raising smaller broods, because females typically provide more care than males and their ability of compensation may be restricted by physical limitations than males. In addition, we predicted that uniparental parents may gain the same weight from the carcass as biparental parents, and that parents gain more weight when caring for small broods than larger broods. Because uniparental parents have chance to feed more from the carcass but provide more care than biparental parents, whereas parents caring for large broods share resource with more offspring and provide more care than parents caring for small broods. We predicted that males may gain more weight than females as they typically provide less care than females. For the second question, we predicted that parents that allocated more resources in current reproduction reduce their parental effort and reproductive outcome in future reproduction. We expected that uniparental parents and parents that had cared for large broods may suffer a future cost in subsequent parental care and reproductive outcome (i.e., larvae number and average larval mass). We also predicted that the carry-over effects of increased allocation to current reproduction may diminish with time as parents gain benefits from the carcass. The carry-over effects of parental care system and brood size may be more obvious during the carcass maintenance stage than during the larvae provisioning stage.
Materials and Methods
Study Animals
All burying beetles (N. vespilloides) used for this study were first-generation laboratory-reared offspring of adults collected at the field station of the University of Groningen in estate “de Vosbergen,” Eelde, The Netherlands. Up to six same-sex adult beetles that descended from the same broods were kept in plastic boxes (length: 15 cm; width: 10 cm; height: 8.5 cm) filled with 2 cm of moist soil under a 16:8 h light: dark cycle and a temperature of 21°C. All adult beetles were fed with mealworms (Tenebrio molitor) twice a week with 2–3 mealworms per beetle each time.
Experimental Design
In order to test the combined impact of partner’s presence and brood size on reproductive allocation of each sex and sex-dependent reproductive trade-offs, we performed an experiment which consisted of two parts (Supplementary Figure 1).
Experiment 1: Effects of Parental Care System and Brood Size and Their Interaction on the Amount of Parental Care and Weight Change of Parents
In the first experiment, we investigated how each sex adjusts its investment based on (1) partner’s presence by manipulating the parental care system through experimental removal of a parent of either sex (uniparental male, uniparental female) or no removal (biparental parents) after all the eggs were laid, and (2) brood size (5 vs. 15 larvae), and (3) their interaction during the larvae provisioning stage. We allowed a pair of beetles to establish and breed on a carcass. Unrelated (i.e., non-sibling), virgin adult beetles, aged approx. 2 weeks old at posteclosion, were randomly selected for use in our experiments. Each pair was placed in a breeding box (length: 19 cm; width: 23 cm; height: 12.5 cm) filled with 2 cm of soil and provided with a previously frozen mouse (15 g).
In N. vespilloides, females start egg-laying about 21 h and the eggs hatch about 81 ∼ 111 h at 20 ± 1°C after parents are given access to a carcass (Smiseth et al., 2006). After 3–4 days, shortly before the hatching of the larvae, we moved the parents and the carcass to a new breeding box with fresh soil, and at this point, randomly removed one parent to generate three treatment groups: uniparental (male care, N = 40; female care, N = 40), or biparental beetles (male and female care, N = 40). To make sure that the parents did not have eggs in the new breeding boxes, we checked each box three times daily (06:30–08:00 am, 14:00–15:30 pm, 21:30–23:00 pm, 6-h intervals) from the bottom of the boxes and renewed the soil when new eggs were found (biparental parents: 3 pairs). Because the larvae would crawl to the carcass after hatching, we kept the eggs in the soil of the old boxes to avoid the hatched larvae entering the carcass before we manipulated brood size. After egg laying, we started checking each old box three times daily for larvae hatching. When the eggs started to hatch (ca 3 days after egg-laying), we transferred 5 or 15 newly hatched larvae to the new boxes with parents to generate experimental broods (20 repetitions of both 5 and 15 larvae groups). We chose brood size of 5 and 15 larvae as treatment levels because they are within the natural range for this species when providing a 15 g mouse carcass under laboratory conditions (range larvae produced: 1–32, mean ± SE = 16.1 ± 6.3, N = 271; range larvae surviving to adult stage: 1–28, mean ± SE = 13.9 ± 5.2, N = 245, W. Wang, unpublished data). We manipulated brood size to 15 larvae which is very close to the average as a large treatment level to avoid filial cannibalism (Bartlett, 1987), and 5 larvae as a small treatment level to ensure that not all offspring died during breeding. As parents cannot directly recognize their offspring as long as the larvae are at the same developmental stage (Müller and Eggert, 1990; Oldekop et al., 2007), we added larvae that were produced by other females when needed. When the larvae dispersed (ca 6 days after larvae-hatching), we moved larvae from the same nest together into new boxes for pupation. We transferred all surviving parents into new boxes for 1 week, which were used for the second experiment.
Once larvae were added to the carcass, we recorded parental care activity of female and male parents three times daily (instant scanning) until larvae dispersal (the larvae provisioning stage) by visual inspection. In burying beetles, presence on the carcass is a strong indicator of parental care (Smiseth and Moore, 2004; Smiseth et al., 2005; Walling et al., 2008; Head et al., 2014). We checked presence or absence of parents by carefully removing the surface soil of the carcass. We recorded no parental care as when a parent was invisible (when in the soil), and parental care as when it was present on or inside the carcass. We estimated the amount of parental care as the proportion of times that parents spent on the carcass during the entire observation period, and then we calculated the parental care per larvae by dividing this proportion by brood size. In addition, we recorded combined biparental care as either or both of the parents being present on or inside the carcass. We defined the day of terminating care when the parents were absent from the carcass for three consecutive observations (Benowitz et al., 2013; Head et al., 2014). We then calculated the caring days as the duration from the day of larvae hatching until the time of terminating care (Parker et al., 2015). At the start of the experiment, the body size of each parent was recorded by measuring the pronotum width (accuracy: 0.01 mm), because body size may influence the amount of parental care (Pilakouta et al., 2015). Each beetle was weighed (accuracy: 0.0001 g) three times during breeding: at the start of the experiment (w0), at the time of egg hatching (wi) and at the time of larval dispersal (wii). For each parent, we recorded the weight change during the carcass maintenance stage (wi-w0, Table 1) to examine any effects on the amount of parental care during the larvae provisioning stage, because the resource acquisition at the onset of breeding and the nutritional status of parents affects their reproductive performances (Trumbo and Robinson, 2004; Richardson and Smiseth, 2019). We recorded the weight change during the larvae provisioning stage (wii-wi, Table 1), because it is a good indicator of the reproductive investment to current reproduction (Trumbo and Xhihani, 2015). We recorded the weight change during the entire reproductive period (wii-w0, Table 1). This is important because parents can increase in weight by feeding from the carcass during breeding and the weight gain can benefit future reproduction (Creighton et al., 2009; Billman et al., 2014; Pilakouta et al., 2016; Richardson et al., 2020). We excluded trials from our analyses in which females failed to produce eggs (N = 2; biparental female, brood 5: N = 1, brood 15: N = 1), or either of the parents died before larvae dispersal, which yielded the following final sample sizes of our first experiment: uniparental female care (brood 5: N = 19, brood 15: N = 18), uniparental male care (brood 5: N = 18, brood 15: N = 16), biparental care (brood 5: N = 15, brood 15: N = 16).
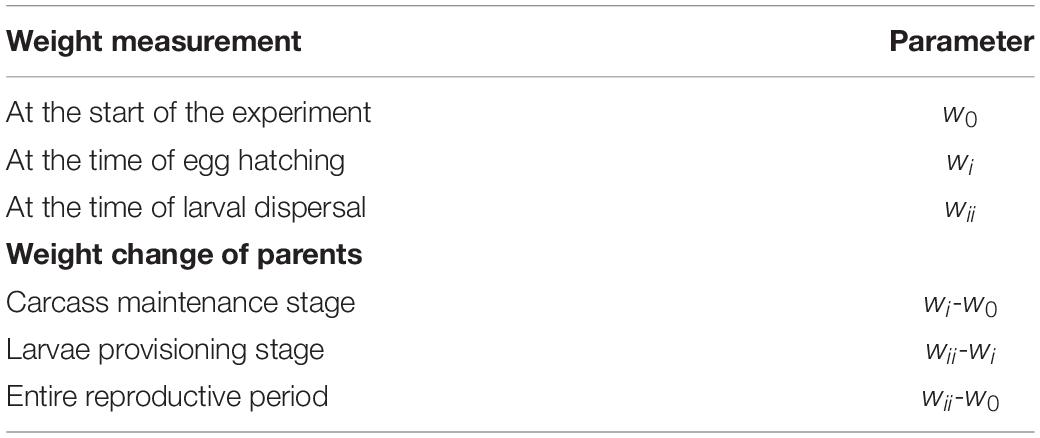
Table 1. Measurements of weight and the calculation of weight change of parents during the first brood.
Experiment 2: Sex-Dependent Reproductive Trade-Offs Between Current and Future Reproduction: Effects of Parental Care System and Brood Size
In the second experiment we experimentally investigated the sex-dependent reproductive trade-offs between current and future reproduction. We paired the surviving parents with the same partners as during the first experiment to exclude any potential effects due to changes in partner’s body size on parental care, because burying beetle parents adjust their contribution toward care based on both their own and their partner’s size (Pilakouta et al., 2015). All parents that were removed from the boxes in the first experiment for the uniparental care treatment, were kept in isolation and fed with mealworms twice a week until they were used in this experiment. We provided each pair of beetles with a new carcass to initiate their second breeding event. We recorded their parental care from the onset of breeding until larvae dispersal (the carcass maintenance stage and the larvae provisioning stage) three times daily as described above. The observations were performed blindly from the experimental conditions in the first breeding attempt. We also recorded their reproductive outcome (the number of larvae and the total mass of larvae at dispersal) in the second brood. We calculated the average larval mass by dividing the brood mass by the number of larvae. We excluded all trials in which females failed to produce eggs (N = 5; uniparental female, brood 5: N = 1; uniparental male, brood 5: N = 2; biparental female, brood 15 = 2), or either or both of the parents died from our further analyses, which yielded the following final sample sizes of our second experiment: uniparental female care (brood 5: N = 17, brood 15: N = 17), uniparental male care (brood 5: N = 14, brood 15: N = 15), biparental care (brood 5: N = 13, brood 15: N = 12). There was no difference in body size (pronotum width) of parents among the different parental groups both during the initial and subsequent breeding (Supplementary Table 1). We thus excluded any potential effects due to variation in body size of parents on the amount of parental care given that body size influences the amount of care (Pilakouta et al., 2015). There was no difference in weight change of parents during the carcass maintenance stage and thus its potential effect on differences in the amount of larvae provisioning were excluded (Supplementary Table 1).
Statistical Analyses
All statistical tests were performed using R version 4.0.3 (R Core Team, 2020) loaded with packages car (Fox and Weisberg, 2019), lme4 (Bates et al., 2015), multcomp (Hothorn et al., 2008), emmeans (Lenth, 2021), DHARMa (Hartig, 2021), and pwr (Champely, 2020). Body size (pronotum width) of parents did not differ among treatments and was therefore excluded from our analysis. We excluded the effect of mixing or non-mixing offspring because parents cannot recognize their offspring when the larvae are at the same stage (Müller and Eggert, 1990; Oldekop et al., 2007). Larvae number was significantly correlated with average larval mass and brood mass (larvae number vs. average larval mass: Pearson’s correlation, t = −6.178, r = −0.554, P < 0.001; larvae number vs. brood mass: Pearson’s correlation, t = 9.544, r = 0.717, P < 0.001; average larval mass vs. brood mass: Pearson’s correlation, t = 1.544, r = 0.164, P = 0.126). Therefore, larvae number and mean larval mass at the larval dispersal time were used to assess the reproductive benefits of parents. We used linear (mixed) models for traits that had a normal error structure (LMs: average larval mass at dispersal; LMMs: weight change of parents), and generalized (mixed) linear models for traits that had a binomial error distribution (GLMMs: the amount of parental care) and Poisson error structure (GLMs: larvae number; GLMMs: number of caring days, lme4 package). All models included the fixed factors parental care system (uniparental males, uniparental females, and biparental males, biparental females for parents’ traits or combined biparental care for larvae traits) and brood size (small vs. large) and the interaction between them. Group identity was included as a random factor in (G)LMMs. Biparental males and females of the same pair share the same group identity, whereas uniparental males and females all have different identity. We used post hoc Tukey contrasts to test for differences whenever parental care system or the interaction had a significant effect on the variable of interest (multcomp and emmeans package). The over/under-dispersion of the models was estimated using the ‘testDispersion’ function and the good fit of the models was verified by plotting the residuals using the ‘simulateResiduals’ function (DHARMa package). Some models were over- or underdispersed or did not have good fits (i.e., weight change during the larvae provisioning stage, larvae number, caring days, the amount of parental care during the carcass maintenance stage). For weight change analyses during the larvae provisioning stage, we compared models that included or excluded four outliers (four biparental males gained weight during the larvae provisioning stage, weight change > 0). Additional analyses of larvae number, caring days and the amount of parental care during the carcass maintenance stage were conducted by running L(M)Ms. Although excluding the four individuals and fitting alternative models solved problems with dispersion and improved the model fits, the results of the models were qualitatively similar to those obtained when using all data or GL(M)Ms, except for the interaction between parental care system and brood size on the amount of parental care during the carcass maintenance stage (see below). Therefore, we present the results from the analysis including all data, and using GLMMs because these allowed us to account for number of observations in parental care measures. Finally, we calculated the statistical power by using ‘pwr.chisq.test’ in pwr package. We used a desired power of 0.8, a significance level of 0.05 and chi-square test with four groups to determine the effect size (w). The w values of 0.3 are commonly considered to represent a medium effect size (Cohen, 1977). The complete dataset and R code used for the analyses are provided in Supplementary Material.
We performed two sets of analyses. In the first set of analysis, we used data from the first experiment (initial breeding) to examine the combined effects of parental care system and brood size on reproductive investment: the amount of parental care, weight change of parents during the larvae provisioning stage and during the entire reproductive period. We also examined the effect of the amount of parental care on weight change of parents during the larvae provisioning stage and during the entire reproductive period. To this end, we included the amount of parental care as an additional fixed factor in the model for weight change of parents. We then compared this model to a model that excluded the amount of parental care. We also compared the amount of parental care provided per larva and the number of caring days among different treatments.
In the second set of analysis, we used the data of the second experiment (subsequent breeding) to examine whether the increased allocation to current reproduction carried-over into reduced investment in the subsequent breeding: the amount of parental care, larvae number and average larval mass at dispersal. In the model for parental care, we included partner’s effort as a covariate, because whether partner had experienced larvae provisioning or not in the first breeding may affect their effort in subsequent breeding (Supplementary Table 1), and parents may adjust their investment based on their partner’s contribution. These covariates were included in the final models when they significantly improved the fit and the variance inflation factor (VIF, anova’ and ‘vif’ function in car package) was smaller than 2, indicating no problems with collinearity (Zuur et al., 2010). We then examined the impact of prior care and weight change of parents during the entire reproductive period in the first breeding on the amount of parental care in the second breeding. We did this by comparing models in which the amount of prior care and weight change of parents during the entire reproductive period in the first breeding were included or excluded as additional effects.
Results
Experiment 1: Effects of Parental Care System and Brood Size and Their Interaction on the Amount of Parental Care and Weight Change of Parents
We found that parents adjusted their care based on their partner’s presence. Both males and females spent significantly more time providing care when their partner was removed. The parental care system had a significant effect on the amount of parental care (Table 2). Uniparental parents compensated for mate removal by providing significantly more care than when they shared care with a partner (Figure 1 and Table 3). Within sexes, uniparental males spent a higher amount of care than biparental males, but less than biparental parents combined (Figure 1A and Table 3). Likewise, uniparental females provided more care than biparental females, but less than biparental parents combined (Figure 1B and Table 3). Across sexes, females spent significantly more time than males provisioning larvae in both uni- and biparental care groups (Table 3), and terminated caring for offspring later than males in both uni- (females vs. males: Estimate ± SE = 0.315 ± 0.117, z = 2.686, P = 0.026) and biparental care groups (females vs. males: Estimate ± SE = 0.366 ± 0.128, z = 2.870, P = 0.015).

Table 2. Effects of parental care system (uniparental male, uniparental female, biparental male, biparental female) and brood size (5 vs. 15), and their interaction on the amount of parental care and weight change of burying beetle parents during the larvae provisioning stage and the entire reproductive period in the first breeding.
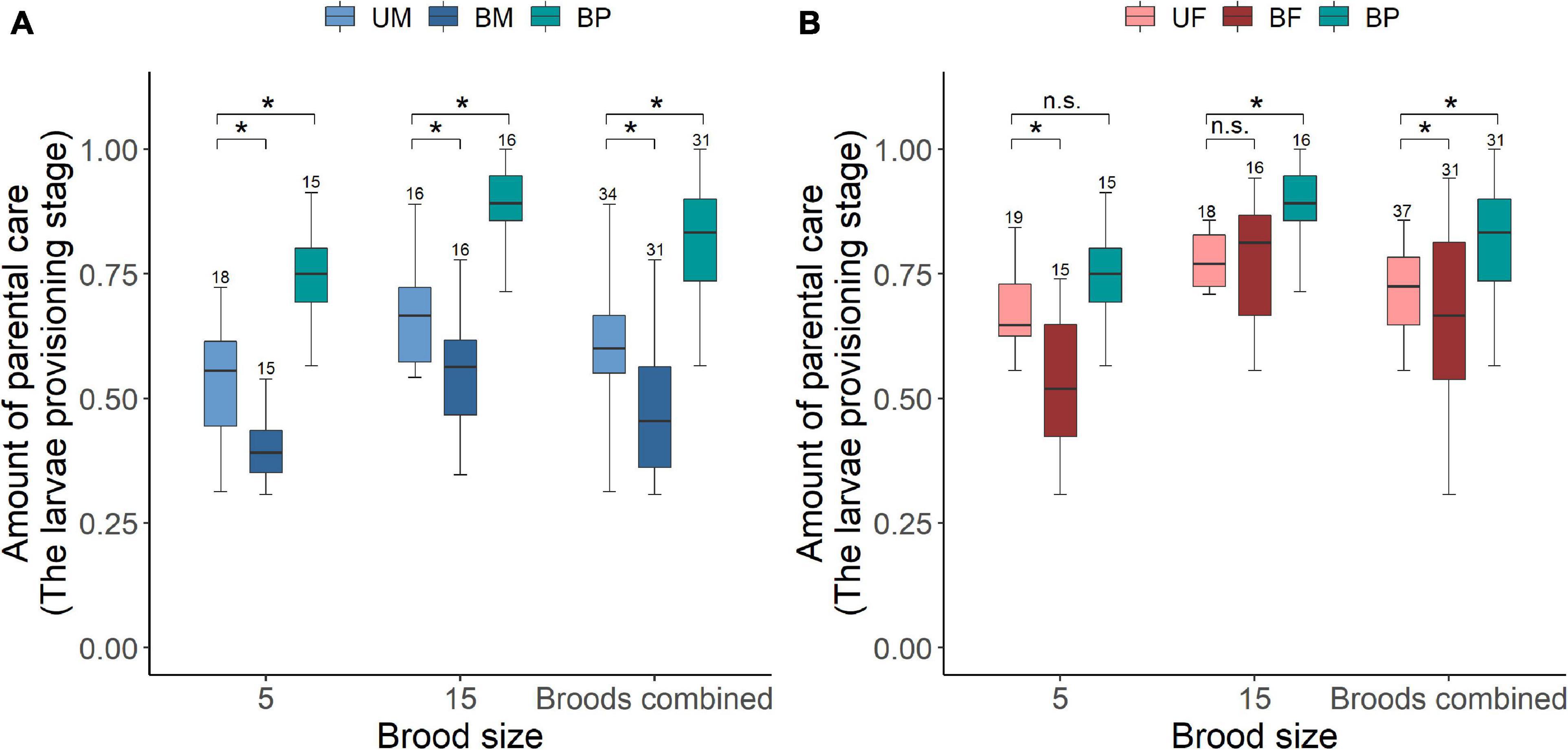
Figure 1. The effect of parental care system (uniparental male, uniparental female, biparental male, and biparental female) and brood size (5 vs. 15 larvae) on the amount of parental care provided by (A) male and (B) female burying beetles in the first breeding. Boxplots show median, interquartile range, and minimum/maximum range. Numbers above error bars are sample sizes. For statistical analyses see Tables 2–4. Asterisks, significant (P < 0.05); n.s., not significant; UM, uniparental male; UF, uniparental female; BM, biparental male; BF, biparental female; BP, the total amount of parental care for biparental male and biparental female combined.
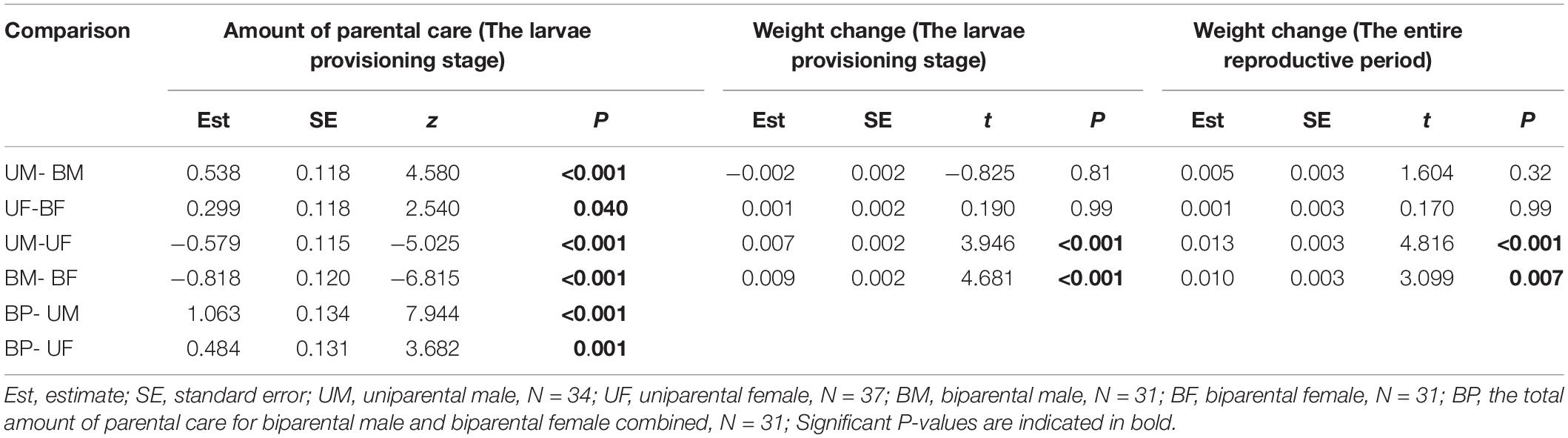
Table 3. Pairwise comparison (Tukey post hoc test) for the effect of parental care system (uniparental male, uniparental female, biparental male, and biparental female) on the amount of parental care and weight change of burying beetle parents during the larvae provisioning stage and the entire reproductive period in the first breeding.
In addition, we found that brood size had a significant positive effect on the amount of time spent providing care (Figure 1 and Table 2). Parents that cared for large broods provided more care than parents that cared for small broods (Figure 1, Table 4, and Supplementary Table 2). However, the amount of parental care provided per larva was less in large broods than in small broods (brood 5, mean ± SE = 0.108 ± 0.029; brood 15, mean ± SE = 0.046 ± 0.009; Estimate ± SE = 4.423 ± 0.185, t = 23.974, P < 0.001). Furthermore, there was a significant effect of the interaction between parental care system and brood size on the amount of parental care (Table 2). The post hoc pairwise comparisons showed that males and females differed significantly in response to mate removal when brood size increased from 5 to l5 larvae. Specifically, uniparental males partially compensate for mate removal by spending more time providing care than biparental males regardless of brood size (Figure 1A and Table 4). However, uniparental females fully compensated for mate removal by providing more care than biparental females when caring for small broods, but provided the same amount of care as biparental females when caring for large broods (Figure 1B and Table 4).
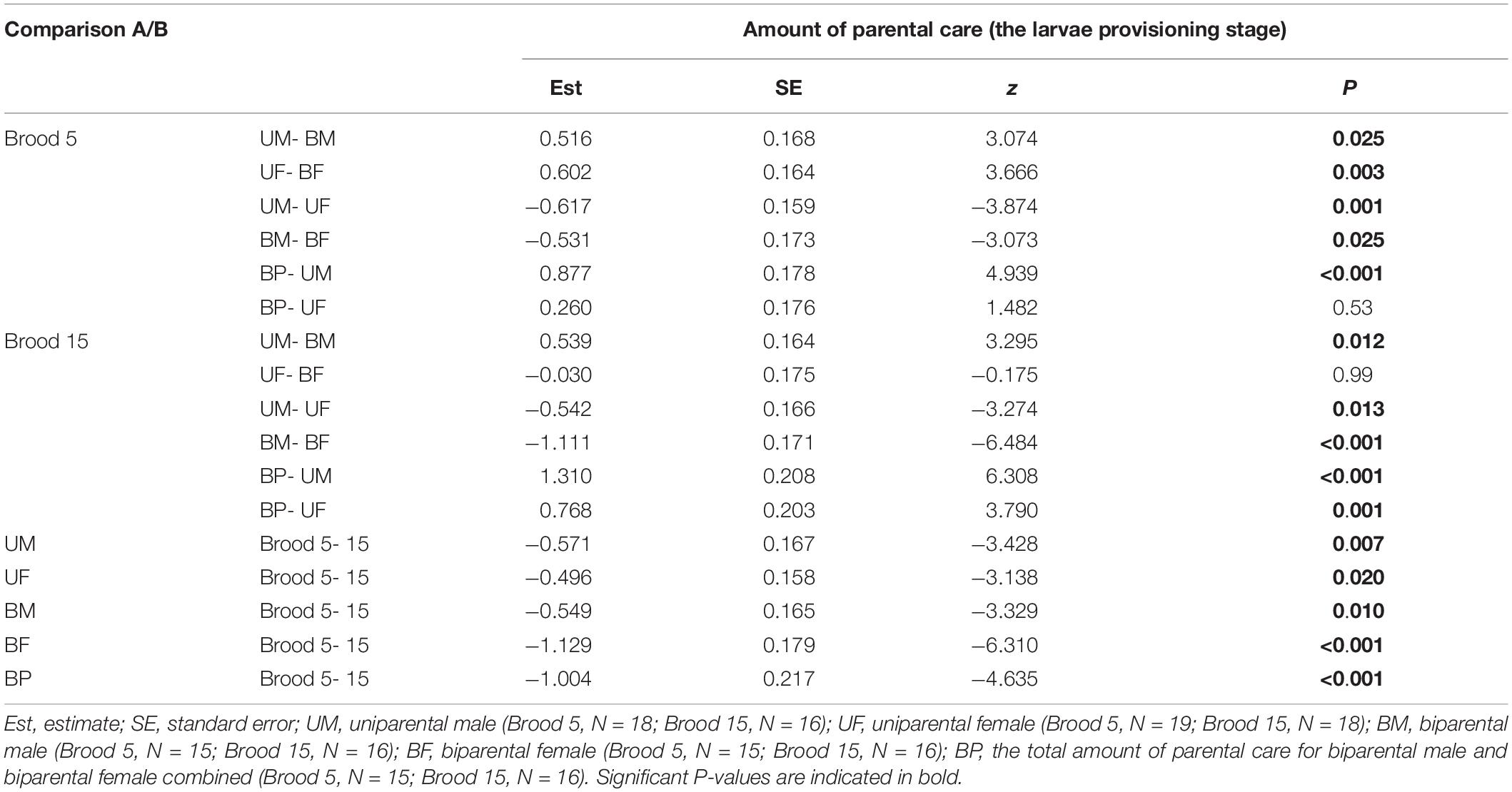
Table 4. Pairwise comparisons (Tukey post hoc test) for the interaction of parental care system (A: uniparental male, uniparental female, biparental male, biparental female; B: uniparental male, uniparental female, biparental male and female combined) and brood size (5 vs. 15) on the amount of parental care during the larvae provisioning stage in the first breeding.
We found that parental care system had a significant effect on weight change of parents during the larvae provisioning stage and the entire reproductive period (Table 2). Males lost less weight during the larvae provisioning stage (Figures 2A,B) and gained more weight during the entire reproductive period (Figures 2C,D) than females in both uniparental and biparental care groups (Table 3). However, there were no significant differences between uni-and biparental parents (Figure 2 and Table 3). In addition, brood size had a significant effect on weight change of parents during the larvae provisioning stage and the entire reproductive period (Table 2). Parents that cared for large broods lost more weight during the larvae provisioning stage and gained less weight during the entire reproductive period than parents that cared for small broods (Figure 2 and Supplementary Table 2). Finally, we found that the amount of parental care had a significant effect on weight change of parents during the larvae provisioning stage (χ2 = 8.812, P = 0.003), and the entire reproductive period (χ2 = 13.194, P < 0.001). Parents that provided more care for their offspring lost more weight during the larvae provisioning stage (Figures 3A,B) and gained less weight during the entire reproductive period than parents that provided less care (Figures 3C,D). No significant effects of interactions were observed on the weight change of parents during the larvae provisioning stage and the entire reproductive period (Table 2).
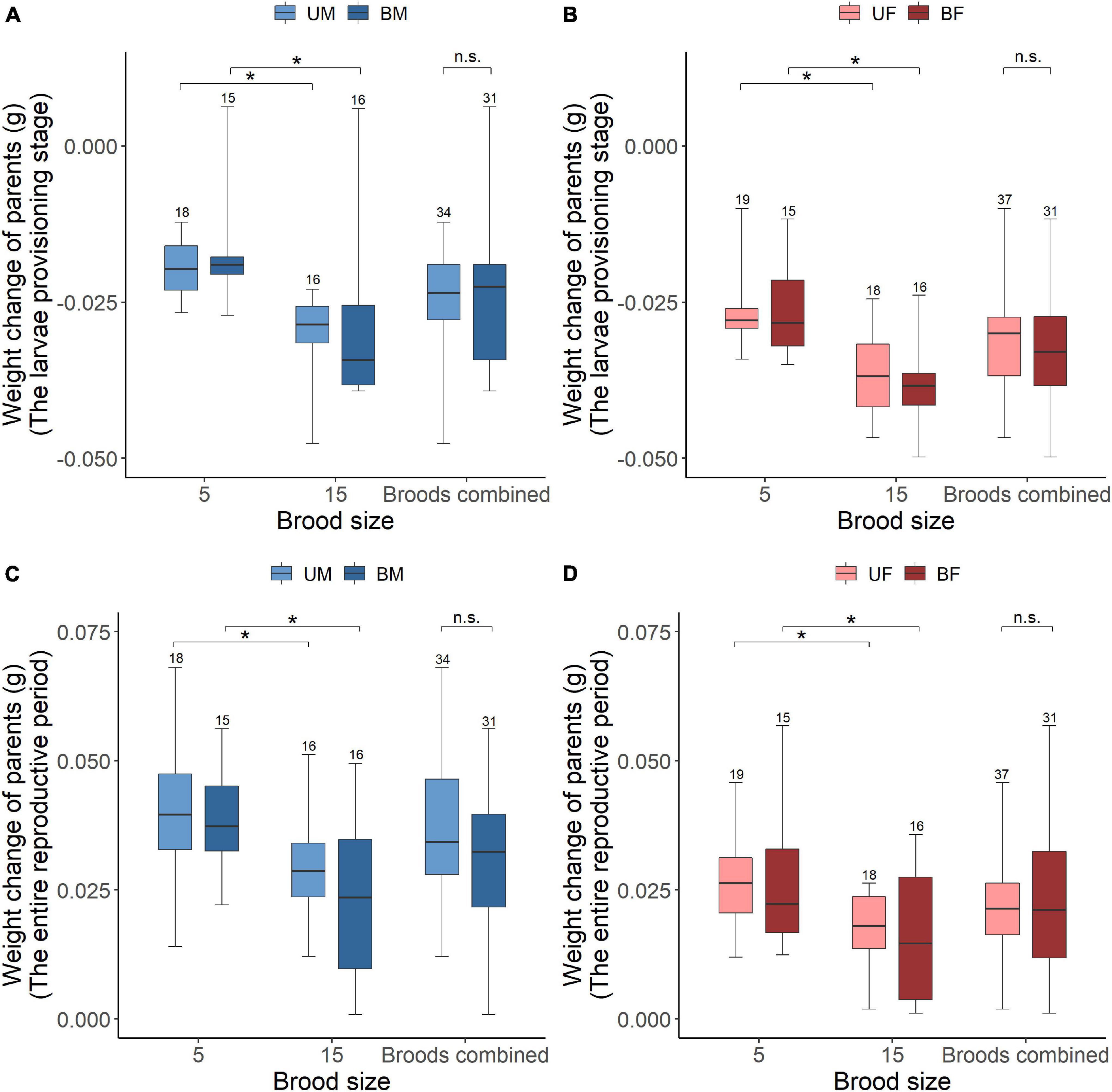
Figure 2. The effect of parental care system (uniparental male, uniparental female, biparental male, and biparental female) and brood size (5 vs. 15 larvae) on weight change of burying beetle during the larvae provisioning stage [values below 0 indicate weight loss; (A) male, (B) female] and the entire reproductive period [values above 0 indicate weight gain; (C) male (D) female] in the first breeding. Boxplots show median, interquartile range, and minimum/maximum range. Numbers above error bars are sample sizes. For statistical analyses see Tables 2, 3. Asterisks, significant (P < 0.05); n.s., not significant; UM, uniparental male; UF, uniparental female; BM, biparental male; BF, biparental female.
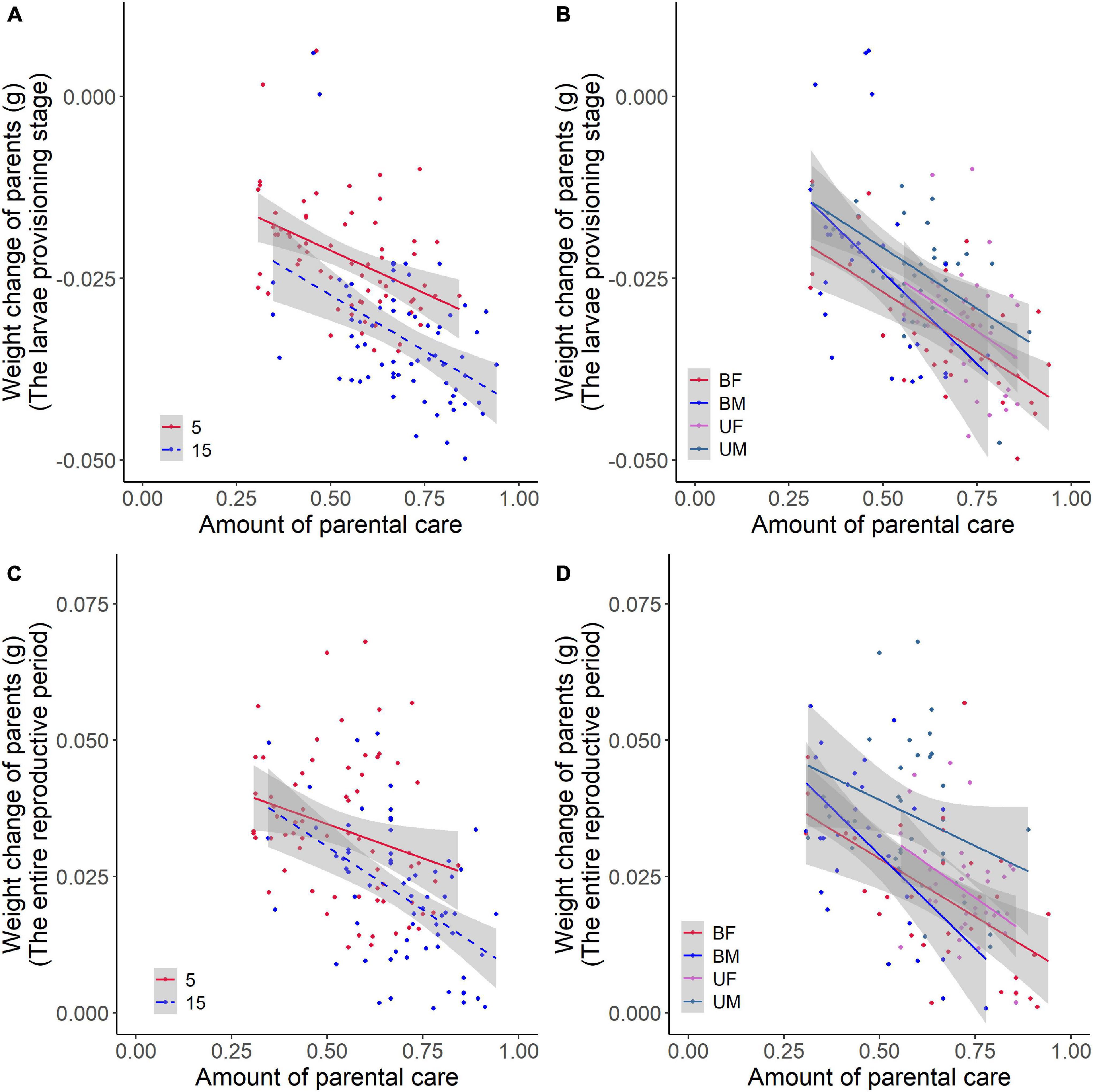
Figure 3. Effects of the amount of parental care on weight change of burying beetle parents during the larvae provisioning stage and the entire reproductive period in the first breeding. The colored regression lines represent the relationship for each treatment (±95% confidence intervals). The larvae provisioning stage (values below 0 indicate weight loss) for (A) brood size manipulations (Brood 5, y = –0.024x – 0.010, R2 = 0.173, N = 67, P = 0.001; Brood 15, y = –0.031x – 0.012, R2 = 0.200, N = 66, P < 0.001) and (B) parental care system treatments (UM, uniparental male, y = –0.033x – 0.004, R2 = 0.329, N = 34, P < 0.001; UF, uniparental female, y = –0.035x – 0.006, R2 = 0.112, N = 37, P = 0.0243; BM, biparental male, y = –0.050x – 0.001, R2 = 0.201, N = 31, P = 0.007; BF, biparental female, y = –0.033x – 0.011, R2 = 0.425, N = 31, P < 0.001). The entire reproductive period (values above 0 indicate weight gain) for (C) brood size manipulations (Brood 5, y = –0.025x + 0.047, R2 = 0.061, N = 67, P = 0.025; Brood 15, y = –0.046x + 0.054, R2 = 0.254, N = 66, P < 0.001) and (D) parental care system treatments (UM, uniparental male, y = –0.034x + 0.056, R2 = 0.070, N = 34, P = 0.071; UF, uniparental female, y = –0.049x + 0.058, R2 = 0.159, N = 37, P = 0.008; BM, biparental male, y = –0.069x + 0.063, R2 = 0.319, N = 31, P = 0.001; BF, biparental female, y = –0.043x + 0.049, R2 = 0.300, N = 31; P = 0.001) in the first breeding.
Experiment 2: Sex-Dependent Reproductive Trade-Offs Between Current and Future Reproduction: Effects of Parental Care System and Brood Size
We found that increased allocation to current reproduction carried-over into reduced future investment for males but not for females. Brood size manipulations in the first breeding had a significant effect on the amount of parental care during the carcass maintenance stage in the second breeding (Table 5). Parents that had cared for small broods in their first breeding provided more care during the carcass maintenance stage in subsequent breeding than parents that had cared for large broods (Figure 4A). Brood size had no effect on the amount of parental care during the larvae provisioning stage (Table 5). In addition, parental care system treatments in the first breeding had a significant effect on the amount of parental care during the carcass maintenance stage and the larvae provisioning stage in the second breeding (Table 5). Males provided less care than females during the carcass maintenance stage in the second breeding for both beetles that had uniparental and biparental care in the first breeding (Figure 4B and Table 6). However, there was no significant difference between males and females during the larvae provisioning stage (Figure 4C and Table 6). There were no significant differences in the amount of parental care between uni- and biparental parents during the carcass maintenance stage and the larvae provisioning stage (Figures 4B,C and Table 6). No significant effect of the interaction was observed, although for parental care during the carcass maintenance stage it approached significance (Table 5). The interaction was significant (χ2 = 40.443, P < 0.001) when we ran a LMM on parental care during carcass maintenance stage. However, pairwise comparisons on the interaction were qualitatively similar and suggested that only males exhibit a significant difference between small and large broods in the second breeding (uniparental male, 5 vs. 15: Estimate ± SE = 0.859 ± 0.276, z = 3.106, P = 0.023; biparental male, 5 vs. 15: Estimate ± SE = 1.025 ± 0.330, z = 3.101, P = 0.023). In contrast, there was no significant difference in subsequent parental care between females that had cared for small and large broods (uniparental female, 5 vs. 15: Estimate ± SE = 0.098 ± 0.307, z = 0.320, P = 0.99; biparental female, Estimate ± SE = 0.055 ± 0.351, z = 0.156, P = 0.99). Finally, we found that weight gain of parents during the entire reproductive period in the first breeding period had a significant positive effect on the amount of parental care during the carcass maintenance stage (χ2 = 5.886, P = 0.015) but not during the larvae provisioning stage (χ2 = 1.818, P = 0.18) in the subsequent breeding. However, prior care in the first breeding did not affect the amount of parental care during the carcass maintenance stage (χ2 = 1.148, P = 0.28) and the larvae provisioning stage (χ2 = 0.081, P = 0.78) in the subsequent reproduction.

Table 5. Effects of parental care system (parent traits: uniparental male, uniparental female, biparental male, biparental female; larvae traits: uniparental male, uniparental female, and biparental care) and brood size (5 vs. 15), and their interaction on the amount of parental care during the carcass maintenance stage and the larvae provisioning stage, and larvae number, average larval mass at dispersal in the second breeding.
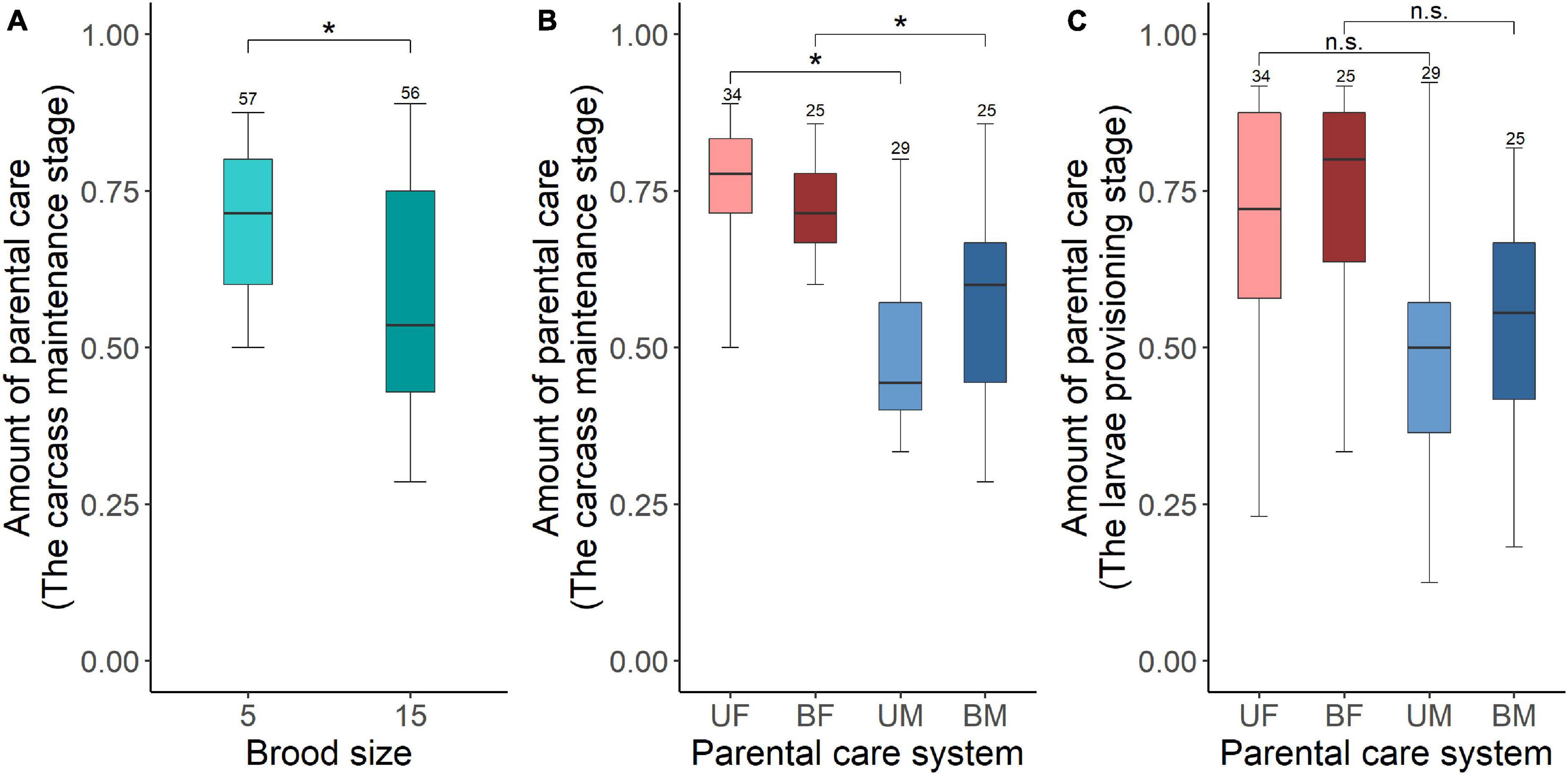
Figure 4. The carry over effects of parental care system (uniparental male, uniparental female, biparental male, biparental female) and brood size (5 vs. 15 larvae) in the first breeding on the amount of parental care during the carcass maintenance stage [(A) brood size (B) parental care system] and the larvae provisioning stage [(C) parental care system] in the second breeding. Boxplots show median, interquartile range, and minimum/maximum range. Numbers above error bars are sample sizes. For statistical analyses see Tables 5, 6. Asterisks, significant (P < 0.05); n.s., not significant; UM, uniparental male; UF, uniparental female; BM, biparental male; BF, biparental female.
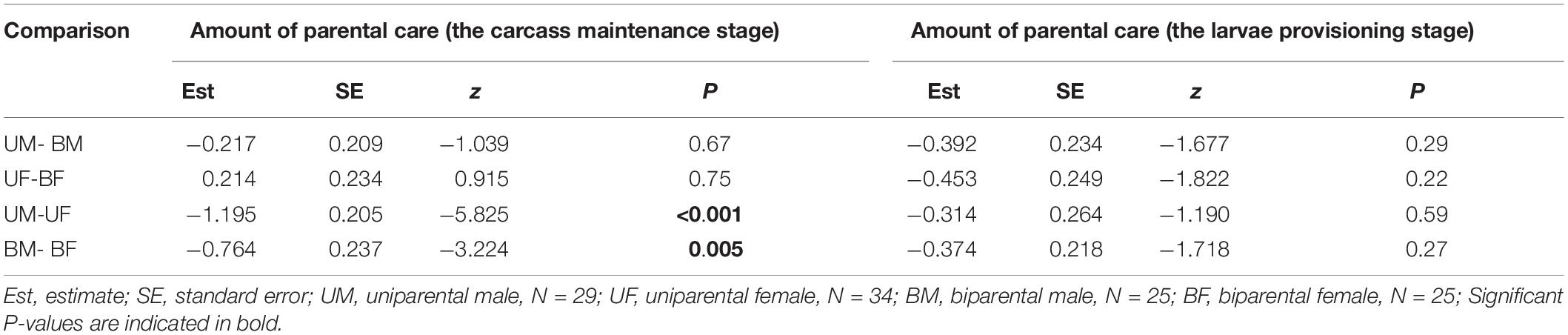
Table 6. Pairwise comparison for the effect of parental care system (uniparental male, uniparental female, biparental male, and biparental female) in the first breeding on the amount of parental care during the carcass maintenance stage and the larvae provisioning stage in the second breeding.
We also found that brood size and parental care system and their interaction had no effects on subsequent larvae number and average larval mass at dispersal (Table 5 and Supplementary Table 3). Increased allocation to current reproduction did not affect the performance of offspring produced during subsequent reproduction event as there was no significant difference in larvae number and average larval mass at dispersal between different parental care system treatments or brood size categories (Figures 5A,B). Our power analysis indicated that both the first (N = 133, w = 0.286) and second set of analysis (N = 113, w = 0.311) was adequate to detect a medium effect size.
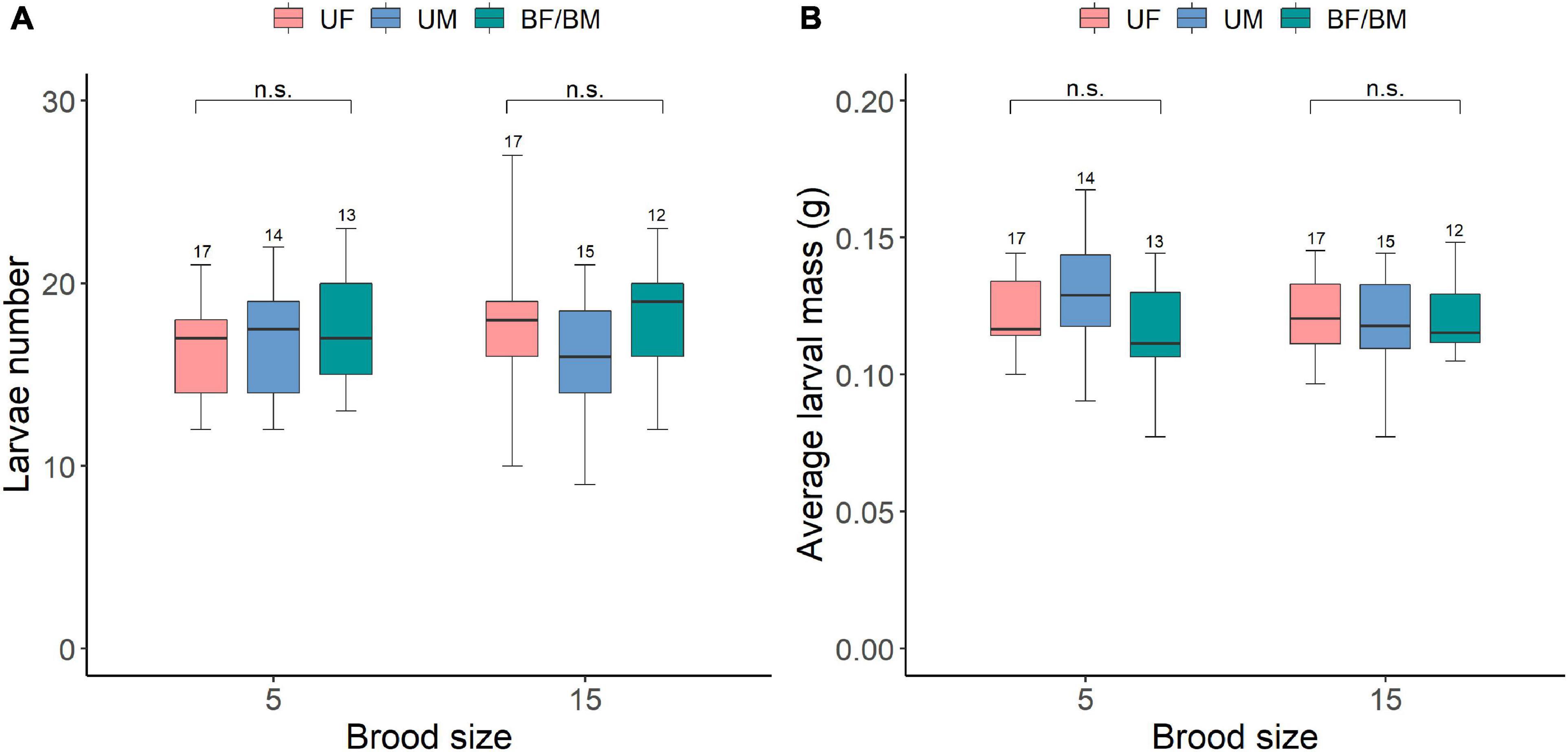
Figure 5. The carry over effects of parental care system (uniparental male, uniparental female, biparental care) and brood size (5 vs. 15 larvae) in the first breeding on (A) larvae number and (B) average larval mass at dispersal in the second breeding. Boxplots show median, interquartile range, and minimum/maximum range. Numbers above error bars are sample sizes. For statistical analyses see Table 5. n.s., not significant; UM, uniparental male; UF, uniparental female; BM, biparental male; BF, biparental female.
Discussion
Here, we report empirical and experimental evidence from a burying beetle that can have uni- or biparental care showing that parents adjust their contribution based on both partner’s presence and brood size, and that this adjustment differs between the sexes. Specifically, males exhibited partial compensation behavior for mate loss regardless of brood size, whereas females fully compensated for mate loss in small broods but showed no response when caring for large broods. We found that sexes differ in their reproductive trade-off between current and future reproduction. Increased allocation to current reproduction due to brood size incurred a cost of reproduction in terms of reduced future parental care for males, but not for females. However, there was no evidence that increased allocation to current reproduction resulted in observed fitness costs during the subsequent breeding event in terms of larvae number and average larval mass at dispersal.
Effects of Parental Care System and Brood Size and Their Interaction on the Amount of Care and Weight Change
We found that parental care system had a significant effect on the amount of parental care provided. Both uniparental males and uniparental females provided more care than biparental males and females, yet they spent less time on care than two parents together, indicating incomplete compensation. Parents respond to mate removal by compensating the amount of care, but also the kind of care if parental roles are sex-dependent. Although both male and female burying beetles are able to perform all parental duties, they focus on different tasks (Smiseth and Moore, 2004; Ratz and Smiseth, 2018). Therefore, one likely explanation may be that the incomplete compensation behavior for provisioning was caused by the sex-dependent roles in parental care and the ability of each sex to carry out the role normally performed by its partner. This is supported by the fact that males focus more on carcass maintenance and defense, whereas females spend more time provisioning larvae (Smiseth and Moore, 2004; Ratz and Smiseth, 2018). We also showed that females spent significantly more time on caring than males and terminated caring later than males. Other studies on this and other species of burying beetles showed that males normally desert the broods earlier and provide less care than females (Bartlett, 1988; Scott and Traniello, 1990; Trumbo, 1991). In our study, males had higher residual reproductive value than females after their first breeding because they provided less care and gained more weight at the entire reproductive period than females. It has been suggested that residual reproductive value predicts brood desertion in burying beetles (Ward et al., 2009). We therefore suggest that the total amount of care provided by males is expected to be lower than females, and thus males provide less care and terminate caring earlier than females. In addition, we found that both males and females respond to brood size manipulations by increasing their provisioning toward larger broods, whereas the amount of parental care per larva was less in large broods than in small broods, indicating incomplete compensation for increased brood size. This is consistent with previous studies on this and other species (Sargent, 1988; Rauter and Moore, 2004; Karino and Arai, 2006; Ratz and Smiseth, 2018; Richardson et al., 2020), suggesting that parents usually provide more care toward larger broods because of higher benefits from providing care. However, our manipulation included both small and large broods which allowed us to test the effect of brood size on the compensation behavior of parents. We found that the amount of parental care was also significantly affected by the interaction between parental care system and brood size manipulation. Uniparental males exhibited partial compensation behavior for mate removal by increasing their food provisioning in both small and large broods compared to biparental males, whereas uniparental females fully compensated for mate removal in small broods but did not show compensation behavior when caring for large broods as they provided the same amount of care as biparental females. A previous mate removal study on the same species has also demonstrated that only males compensate for mate loss (Smiseth et al., 2005). However, this study did not investigate the combined effects of brood size and parental care system, nor did they manipulate brood size experimentally. Additionally, we found that the sex difference in response to mate removal corresponded with the previously found sex difference in parental care in response to larval begging. Males provided more care in response to higher larval begging, whereas females, in contrast, did not change their care (Smiseth and Moore, 2004). Considering that females normally stay longer and provide more care than males (Bartlett, 1988; Fetherston et al., 1990, 1994; Scott, 1998), we suggest that the sex difference in response to mate loss and larval begging may be due to the difference in reaching their maximum capacity of providing care. Females may not show compensation behavior when caring for large broods because they have already worked near their maximum capacity and thus their ability to increase their contribution after male removal is limited. This suggestion is supported by the results showing that females caring for small broods fully compensated for mate removal, whereas females caring for large broods did not. Meanwhile, parents lost more weight when providing higher amount of care during the larvae provisioning stage. This is in agreement with biparental birds in which incomplete compensation is often the result of physical limitations (Drent and Daan, 1980; Jones et al., 2002; Matysioková and Remeš, 2014; Cones and Crowley, 2020; Williams and DeLeon, 2020). In contrast, males exhibited compensation behavior regardless of brood size, and this may be because they provided less care and worked at a lower level than females and gained more weight than females.
We also found that in the first breeding females lost more weight during the larvae provisioning stage and gained less weight during the entire reproductive period than males, and this is consistent with the fact that females provided more care than males, suggesting that males expend less energy than females during breeding or they feed more from the carcass than females. Meanwhile, we found that parents lost more weight during the larvae provisioning stage and thus gained less weight during the entire reproductive period when caring for larger broods, reflecting that larger broods require more care and resource from parents.
Sex-Dependent Reproductive Trade-Offs Between Current and Future Reproduction: Effects of Parental Care System and Brood Size
We found a trade-off between current and future reproductive investment for males but not for females. In males, higher amount of care due to larger brood size resulted in less weight gain and in turn lower future reproductive investment. In females, increased allocation due to larger brood size resulted in less weight gain but did not incur future costs in terms of reduced parental care in the second breeding. The sex-dependent reproductive trade-offs may be explained by the sex difference in the functions of parental care. In burying beetles, males are more involved in the carcass maintenance stage, while females often spend more time on the larvae provisioning stage than males (Müller et al., 1998; Scott, 1998; Smiseth and Moore, 2004; Ratz and Smiseth, 2018). Therefore, the carcass maintenance stage should be the most stressful for males. The benefits gained by feeding from the carcass during the subsequent breeding might be not enough to cancel out the costs of prior reproduction for males, and thus they exhibited reproductive trade-offs. However, females focused on the larvae provisioning stage thus they might have a higher probability to recover energy and have more chance to offset the costs of prior reproduction by feeding from the carcass during the carcass maintenance stage. Additionally, we demonstrated the predicted reproductive trade-off during the carcass maintenance stage, but not during the larvae provisioning stage. It has been suggested that the weight gained during the initial breeding attempt can serve as a proxy for investment in future reproduction (Creighton et al., 2009; Billman et al., 2014; Pilakouta et al., 2016; Richardson et al., 2020). In our experiment, all parents gained weight during breeding, which improved their own body condition. Therefore, a likely explanation is that the costs of reproduction were offset to some degree by the benefits of feeding from the carcass during the carcass maintenance stage, then resulting in undetectable costs in subsequent stages. That is, the carry-over effects of reproductive costs may diminish with time as parents gained benefits from the carcass. Another explanation is that the costs of reproduction were reduced under laboratory conditions compared with natural conditions, because burying beetles did not have to search and compete for food, carcasses and mates between two breeding attempts. The breeding opportunities for N. vespilloides are typically limited by the availability of resources in the field and it is costly to compete for and protect the carcasses (Scott, 1998). By providing them with food, carcasses and mates before the subsequent breeding attempt, the potential cost for not storing enough energy during their initial breeding was canceled out to some degree and thus leading to the non-significant results during the larvae provisioning stage. We found no evidence that increased allocation to current reproduction resulted in future costs for reproductive performances in subsequent reproduction as there were no differences in larvae number and average larval mass at dispersal among different treatments. The weight change during the entire reproductive period in the first breeding had no effect on larvae number and average larval mass at dispersal in the second breeding. It has been reported that pre-hatching care (i.e., the carcass maintenance stage) did not affect offspring number and brood mass in burying beetles (Capodeanu-Nägler et al., 2016). In the subsequent breeding, although males caring for small and large broods provided different amount of care during the carcass maintenance stage, there were no differences in post-hatching care (i.e., the larvae provisioning stage) among the different treatments. Considering the fact that males are more involved in carcass preparation and brood guarding, and females spend more time provisioning food for larvae (Smiseth and Moore, 2004), we suggest that offspring performances were more likely to be influenced by post-hatching care and female care in burying beetles. Finally, we showed that weight gain during the entire reproductive period, but not the amount of prior care of parents, had a significant effect on parental allocation in subsequent breeding. This may be the reason for our findings that the observed increased allocation due to the variation in parental care system did not incur future costs, as parental care system (uniparental vs. biparental) was of no effect on weight change for both male and female parents. However, brood size may affect the reproductive trade-off through the weight change of parents during the entire reproductive period, because parents that had cared for large broods provided more care and gained less weight in the initial breeding, and provided less parental care in the subsequent breeding. Prior work on the same species demonstrated that increased allocation to current reproduction resulted in reduced weight gain during the initial breeding and incurred a future cost of reproduction in terms of reduced competitive ability (Richardson et al., 2020). Our results are in line with these findings and provide further evidence for reproductive trade-offs by demonstrating that an increase in current parental effort can incur costs in future reproduction. Furthermore, our experimental design included a novel manipulation that allowed us to tease apart effects of parental care system and brood size.
Conclusion
In this study, we experimentally demonstrated the effects of parental care system and brood size and the interaction between these two factors on parental investment and reproductive trade-off between current and future reproduction. Males and females differ in compensation behavior: males compensated for mate loss by significantly increasing the level of care regardless of brood size, while females exhibited such compensation only for small brood size. The effects of brood size manipulation but not of parental care system carried-over into sex-dependent reproductive allocation. With an increase in allocation to current reproduction due to larger brood size, males but not females showed decreased parental investment during subsequent breeding. However, increased investment due to parental care system did not incur future costs in terms of reduced parental care. This is, to our knowledge, the first study on the combined effects of parental care system and brood size on reproductive allocation and future fitness, and the results enhanced our understanding of sex roles in parental investment and furthered our knowledge of sex-dependent reproductive trade-offs in burying beetles. However, we tested the trade-off under laboratory conditions where the potential future costs for searching and competing for food, carcasses, and mates was not paid. It is unclear what the consequence will be when breeding in natural situations where the inter- and intra-specific competition for limited food and mates is higher. In addition, considering that the benefits gained by feeding from the resource may mask the costs of reproduction and thus have important consequence for subsequent reproduction, we suggest that future work on reproductive trade-offs in such species should consider the potential impact of resource variability and how much benefits they could gain when reproductive resource changes.
Data Availability Statement
The raw data supporting the conclusions of this article will be made available by the authors, without undue reservation.
Author Contributions
WW, LM, JK, and HW conceived and designed the study. WW performed the experiments and wrote the initial manuscript. MV and WW analyzed the data with significant help of LM. JK led the manuscript writing and editing. All authors contributed to the article and approved the submitted version.
Funding
This study was supported by a Ph.D. grant from the China Scholarship Council (CSC, 201906770043) and a Dobberke foundation from the Royal Netherlands Academy of Arts and Sciences (KNAWWF/Dobberke/2785) to WW, an Ecology Fund of the Royal Netherlands Academy of Arts and Sciences (KNAWWF/807/19021) to LM, and NWO grants (854.11.003 and 823.01.014) to JK.
Conflict of Interest
The authors declare that the research was conducted in the absence of any commercial or financial relationships that could be construed as a potential conflict of interest.
Publisher’s Note
All claims expressed in this article are solely those of the authors and do not necessarily represent those of their affiliated organizations, or those of the publisher, the editors and the reviewers. Any product that may be evaluated in this article, or claim that may be made by its manufacturer, is not guaranteed or endorsed by the publisher.
Acknowledgments
We thank VB and two reviewers for their constructive comments on the manuscript.
Supplementary Material
The Supplementary Material for this article can be found online at: https://www.frontiersin.org/articles/10.3389/fevo.2021.739396/full#supplementary-material
References
Ardia, D. R. (2007). Site-and sex-level differences in adult feeding behavior and its consequences to offspring quality in tree swallows (Tachycineta bicolor) following brood-size manipulation. Can. J. Zool. 85, 847–854. doi: 10.1139/Z07-070
Bartlett, J. (1987). Filial cannibalism in burying beetles. Behav. Ecol. Sociobiol. 21, 179–183. doi: 10.2307/4600076
Bartlett, J. (1988). Male mating success and paternal care in Nicrophorus vespilloides (Coleoptera: Silphidae). Behav. Ecol. Sociobiol. 23, 297–303. doi: 10.1007/bf00300576
Bates, D., Mächler, M., Bolker, B., and Walker, S. (2015). Fitting linear mixed-effects models using lme4. J. Stat. Softw. 67, 1–48. doi: 10.18637/jss.v067.i01
Bebbington, K., and Hatchwell, B. J. (2016). Coordinated parental provisioning is related to feeding rate and reproductive success in a songbird. Behav. Ecol. 27, 652–659. doi: 10.1093/beheco/arv198
Benowitz, K. M., Head, M. L., Williams, C. A., Moore, A. J., and Royle, N. J. (2013). Male age mediates reproductive investment and response to paternity assurance. Proc. R. Soc. B. 280:20131124. doi: 10.1098/rspb.2013.1124
Billman, E. J., Creighton, J. C., and Belk, M. C. (2014). Prior experience affects allocation to current reproduction in a burying beetle. Behav. Ecol. 25, 813–818. doi: 10.1093/beheco/aru051
Bleu, J., Le Galliard, J. F., Fitze, P. S., Meylan, S., Clobert, J., and Massot, M. (2013). Reproductive allocation strategies: a long-term study on proximate factors and temporal adjustments in a viviparous lizard. Oecologia 171, 141–151. doi: 10.1007/s00442-012-2401-1
Boncoraglio, G., and Kilner, R. M. (2012). Female burying beetles benefit from male desertion: sexual conflict and counter-adaptation over parental investment. PLoS One 7:e31713. doi: 10.1371/journal.pone.0031713
Cantarero, A., Plaza, M., Moreno, J., and Griggio, M. (2019). Parental feeding responses to experimental short-term partner removal in a species with male and female brood desertion. Behav. Ecol. Sociobiol. 73, 1–8. doi: 10.1007/s00265-019-2693-9
Capodeanu-Nägler, A., Keppner, E. M., Vogel, H., Ayasse, M., Eggert, A. K., Sakaluk, S. K., et al. (2016). From facultative to obligatory parental care: interspecific variation in offspring dependency on post-hatching care in burying beetles. Sci. Rep. 6, 1–10. doi: 10.1038/srep29323
Cones, A. G., and Crowley, P. H. (2020). Optimal maternal incubation strategies for altricial and precocial birds. Ecol. Model. 436:109290. doi: 10.1016/j.ecolmodel.2020.109290
Creighton, J. C., Heflin, N. D., and Belk, M. C. (2009). Cost of reproduction, resource quality, and terminal investment in a burying beetle. Am. Nat. 174, 673–684. doi: 10.1086/605963
Creighton, J. C., Smith, A. N., Komendat, A., and Belk, M. (2015). Dynamics of biparental care in a burying beetle: experimental handicapping results in partner compensation. Behav. Ecol. Sociobiol. 69, 265–271. doi: 10.1007/s00265-014-1839-z
Daan, S., Deerenberg, C., and Dijkstra, C. (1996). Increased daily work precipitates natural death in the kestrel. J. Anim. Ecol. 65, 539–544. doi: 10.2307/5734
David, M., Pinxten, R., Martens, T., and Eens, M. (2015). Exploration behavior and parental effort in wild great tits: partners matter. Behav. Ecol. Sociobiol. 69, 1085–1095. doi: 10.1007/s00265-015-1921-1
Drent, D. H., and Daan, S. (1980). The prudent parent: energetic adjustments in avain breeding. Ardea 68, 225–252. doi: 10.5253/arde.v68.p225
Eggert, A. K., Reinking, M., and Müller, J. K. (1998). Parental care improves offspring survival and growth in burying beetles. Anim. Behav. 55, 97–107. doi: 10.1006/anbe.1997.0588
Fetherston, I. A., Scott, M. P., and Traniello, J. F. A. (1990). Parental care in burying beetles: the organization of male and female brood−care behavior. Ethology 85, 177–190. doi: 10.1111/j.1439-0310.1990.tb00398.x
Fetherston, I. A., Scott, M. P., and Traniello, J. F. A. (1994). Behavioral compensation for mate loss in the burying beetle Nicrophorus orbicollis. Anim. Behav. 47, 777–785. doi: 10.1006/anbe.1994.1110
Fox, J., and Weisberg, S. (2019). An R Companion to Applied Regression, Third Edition. Available online at: https://socialsciences.mcmaster.ca/jfox/Books/Companion/(accessed date 2020 May 17).
García-Navas, V., and Sanz, J. J. (2010). Flexibility in the foraging behavior of blue tits in response to short−term manipulations of brood size. Ethology 116, 744–754. doi: 10.1111/j.1439-0310.2010.01788.x
Godfray, H. C. J. (1995). Evolutionary theory of parent-offspring conflict. Nature 376, 133–138. doi: 10.1038/376133a0
Griffioen, M., Müller, W., and Iserbyt, A. (2019). A fixed agreement-consequences of brood size manipulation on alternation in blue tits. Peer. J. 7:e6826. doi: 10.7717/peerj.6826
Gross, M. R. (1996). Alternative reproductive strategies and tactics: diversity within sexes. Trends. Ecol. Evol. 11, 92–98. doi: 10.1016/0169-5347(96)81050-0
Harris, W. E., and Uller, T. (2009). Reproductive investment when mate quality varies: differential allocation versus reproductive compensation. Phil. Trans. R. Soc. B. 364, 1039–1048. doi: 10.1098/rstb.2008.0299
Harrison, F., Barta, Z., Cuthill, I., and Székely, T. (2009). How is sexual conflict over parental care resolved? A meta-analysis. J. Evol. Biol. 22, 1800–1812. doi: 10.1111/j.1420-9101.2009.01792.x
Hartig, F. (2021). DHARMa: Residual Diagnostics for Hierarchical (Multi-Level/Mixed) Regression Models. R package version 0.4.3.
Head, M. L., Hinde, C. A., Moore, A. J., and Royle, N. J. (2014). Correlated evolution in parental care in females but not males in response to selection on paternity assurance behaviour. Ecol. Lett. 17, 803–810. doi: 10.1111/ele.12284
Hinde, C. A. (2006). Negotiation over offspring care? a positive response to partner-provisioning rate in great tits. Behav. Ecol. 17, 6–12. doi: 10.1093/beheco/ari092
Hoffman, C. L., Ruiz-Lambides, A. V., Davila, E., Maldonado, E., Gerald, M. S., and Maestripieri, D. (2008). Sex differences in survival costs of reproduction in a promiscuous primate. Behav. Ecol. Sociobiol. 62, 1711–1718. doi: 10.1007/s00265-008-0599-z
Hothorn, T., Bretz, F., and Westfall, P. (2008). Simultaneous inference in general parametric models. Biom. J. 50, 346–363. doi: 10.1002/bimj.200810425
Houston, A. I., and Davies, N. B. (1985). “The evolution of co-operation and life history in the dunnock, Prunella modularis,” in Behavioral ecology: ecological consequences of adaptive behavior, eds R. M. Sibly and R. H. Smith (Oxford: Blackwell Scientific), 471–487.
Houston, A. I., Székely, T., and McNamara, J. M. (2005). Conflict between parents over care. Trends Ecol. Evol. 20, 33–38. doi: 10.1016/j.tree.2004.10.008
Iserbyt, A., Griffioen, M., Eens, M., and Müller, W. (2019). Enduring rules of care within pairs-how blue tit parents resume provisioning behavior after experimental disturbance. Sci. Rep. 9, 1–9. doi: 10.1038/s41598-019-39139-9
Johnstone, R. A., and Hinde, C. A. (2006). Negotiation over offspring care-how should parents respond to each other’s efforts? Behav. Ecol. 17, 818–827. doi: 10.1093/beheco/arl009
Jones, K. M., Ruxton, G. D., and Monaghan, P. (2002). Model parents: is full compensation for reduced partner nest attendance compatible with stable biparental care? Behav. Ecol. 13, 838–843. doi: 10.1093/beheco/13.6.838
Karino, K., and Arai, R. (2006). Effect of clutch size on male egg-fanning behavior and hatching success in the goby, Eviota prasina (Klunzinger). J. Exp. Mar. Biol. Ecol. 334, 43–50. doi: 10.1016/j.jembe.2006.01.018
Kilner, R. M., and Hinde, C. A. (2012). “Parent-offspring conflict,” in The evolution of parental care, eds N. J. Royle, P. T. Smiseth, and M. Kölliker (Oxford: Oxford University Press), 119–132.
Kokko, H., and Jennions, M. D. (2008). Parental investment, sexual selection and sex ratios. J. Evol. Biol. 21, 919–948. doi: 10.1111/j.1420-9101.2008.01540.x
Komdeur, J., Janson, J., Magrath, M., Mulder, R., and Elgar, M. (2007). Provisioning adjustments by male and female fairy martins to short-term manipulations of brood size. Behav. 144, 1119–1132. doi: 10.1163/156853907781871815
Kotiaho, J. S., and Simmons, L. W. (2003). Longevity cost of reproduction for males but no longevity cost of mating or courtship for females in the male-dimorphic dung beetle Onthophagus binodis. J. Insect Physiol. 49, 817–822. doi: 10.1016/S0022-1910(03)00117-3
Lenth, R. V. (2021). emmeans: Estimated Marginal Means, aka Least-Squares Means. R package version 1.6.3.
Lessells, C. M. (1999). “Sexual conflict in animals,” in Levels of Selection in Evolution, ed. L. Keller (Princeton: Princeton University Press), 75–99.
Lessells, C. M. (2012). “Sexual conflict,” in The Evolution of Parental Care, eds N. J. Royle, P. T. Smiseth, and M. Kölliker (Oxford: Oxford University Press), 150–170.
Low, M., Makan, T., and Castro, I. (2012). Food availability and offspring demand influence sex-specific patterns and repeatability of parental provisioning. Behav. Ecol. 23, 25–34. doi: 10.1093/beheco/arr145
MacGregor, N. A., and Cockburn, A. (2002). Sex differences in parental response to begging nestlings in superb fairy-wrens. Anim. Behav. 63, 923–932. doi: 10.1006/anbe.2001.1991
Mariette, M. M., and Griffith, S. C. (2012). Nest visit synchrony is high and correlates with reproductive success in the wild zebra finch Taeniopygia guttata. J. Avian Biol. 43, 131–140. doi: 10.1111/j.1600-048X.2012.05555.x
Mariette, M. M., and Griffith, S. C. (2015). The adaptive significance of provisioning and foraging coordination between breeding partners. Am. Nat. 185, 270–280. doi: 10.1086/679441
Markman, S., Yom-Tov, Y., and Wright, J. (1996). The effect of male removal on female parental care in the orange-tufted sunbird. Anim. Behav. 52, 437–444. doi: 10.1006/anbe.1996.0188
Matysioková, B., and Remeš, V. (2014). The importance of having a partner: male help releases females from time limitation during incubation in birds. Front. Zool. 11, 1–10. doi: 10.1186/1742-9994-11-24
McNamara, J. M., Gasson, C. E., and Houston, A. I. (1999). Incorporating rules for responding into evolutionary games. Nature 401, 368–371. doi: 10.1038/43869
Mock, D. W., Schwagmeyer, P. L., and Parker, G. A. (2005). Male house sparrows deliver more food to experimentally subsidized offspring. Anim. Behav. 70, 225–236. doi: 10.1016/j.anbehav.2004.10.020
Müller, J. K., and Eggert, A. K. (1990). Time-dependent shifts between infanticidal and parental behavior in female burying beetles a mechanism of indirect mother-offspring recognition. Behav. Ecol. Sociobiol. 27, 11–16. doi: 10.1007/bf00183307
Müller, J. K., Eggert, A. K., and Sakaluk, S. K. (1998). Carcass maintenance and biparental brood care in burying beetles: are males redundant? Ecol. Entomol. 23, 195–200. doi: 10.1046/j.1365-2311.1998.00119.x
Nakagawa, S., Gillespie, D. O. S., Hatchwell, B. J., and Burke, T. (2007). Predictable males and unpredictable females: sex difference in repeatability of parental care in a wild bird population. J. Evol. Biol. 20, 1674–1681. doi: 10.1111/j.1420-9101.2007.01403.x
Neuenschwander, S., Brinkhof, M. W., Kölliker, M., and Richner, H. (2003). Brood size, sibling competition, and the cost of begging in great tits (Parus major). Behav. Ecol. 14, 457–462. doi: 10.1093/beheco/arg025
Oldekop, J. A., Smiseth, P. T., Piggins, H. D., and Moore, A. J. (2007). Adaptive switch from infanticide to parental care: how do beetles time their behavior? J. Evol. Biol. 20, 1998–2004. doi: 10.1111/j.1420-9101.2007.01364.x
Osorno, J. L., and Székely, T. (2004). Sexual conflict and parental care in magnificent frigatebirds: full compensation by deserted females. Anim. Behav. 68, 337–342. doi: 10.1016/j.anbehav.2003.06.027
Parejo, D., and Danchin, E. (2006). Brood size manipulation affects frequency of second clutches in the blue tit. Behav. Ecol. Sociobiol. 60, 184–194. doi: 10.1007/s00265-005-0155-z
Parker, D. J., Cunningham, C. B., Walling, C. A., Stamper, C. E., Head, M. L., Roy-Zokan, E. M., et al. (2015). Transcriptomes of parents identify parenting strategies and sexual conflict in a subsocial beetle. Nat. Commun. 6, 1–12. doi: 10.1038/ncomms9449
Parker, G. A. (2006). Sexual conflict over mating and fertilization: an overview. Philos. Trans. R. Soc. B. 361, 235–259. doi: 10.1098/rstb.2005.1785
Pilakouta, N., Richardson, J., and Smiseth, P. T. (2015). State−dependent cooperation in burying beetles: Parents adjust their contribution towards care based on both their own and their partner’s size. J. Evol. Biol. 28, 1965–1974. doi: 10.1111/jeb.12712
Pilakouta, N., Richardson, J., and Smiseth, P. T. (2016). If you eat, I eat: resolution of sexual conflict over consumption from a shared resource. Anim. Behav. 111, 175–180. doi: 10.1016/j.anbehav.2015.10.016
Queller, D. C. (1997). Why do females care more than males? Proc. R. Soc. Lond. B. 264, 1555–1557. doi: 10.1098/rspb.1997.0216
R Core Team (2020). R: A language and environment for statistical computing. Vienna: The R Foundation for Statistical Computing.
Ratz, T., Nichol, T. W., and Smiseth, P. T. (2020). Parental responses to increasing levels of handicapping in a burying beetle. Behav. Ecol. 31, 73–80. doi: 10.1093/beheco/arz157
Ratz, T., and Smiseth, P. T. (2018). Flexible parents: joint effects of handicapping and brood size manipulation on female parental care in Nicrophorus vespilloides. J. Evol. Biol. 31, 646–656. doi: 10.1111/jeb.13254
Rauter, C. M., and Moore, A. J. (2004). Time constraints and trade-offs among parental care behaviors: effects of brood size, sex and loss of mate. Anim. Behav. 68, 695–702. doi: 10.1016/j.anbehav.2003.09.018
Reznick, D. (1985). Costs of reproduction: an evaluation of the empirical evidence. Oikos 44, 257–267. doi: 10.2307/3544698
Richardson, J., and Smiseth, P. T. (2019). Effects of variation in resource acquisition during different stages of the life cycle on life−history traits and trade−offs in a burying beetle. J. Evol. Biol. 32, 19–30. doi: 10.1111/jeb.13388
Richardson, J., Stephens, J., and Smiseth, P. T. (2020). Increased allocation to reproduction reduces future competitive ability in a burying beetle. J. Anim. Ecol. 89, 1918–1926. doi: 10.1111/1365-2656.13242
Rivalan, P., Prevot-Julliard, A. C., Choquet, R., Pradel, R., Jacquemin, B., and Girondot, M. (2005). Trade-off between current reproductive effort and delay to next reproduction in the leatherback sea turtle. Oecologia 145, 564–574. doi: 10.1007/s00442-005-0159-4
Roff, D. A., and Fairbairn, D. J. (2007). The evolution of trade−offs: where are we? J. Evol. Biol. 20, 433–447. doi: 10.1111/j.1420-9101.2006.01255.x
Royle, N. J., Schuett, W., and Dall, S. R. (2010). Behavioral consistency and the resolution of sexual conflict over parental investment. Behav. Ecol. 21, 1125–1130. doi: 10.1093/beheco/arq156
Rozen, D. E., Engelmoer, D. J. P., and Smiseth, P. T. (2008). Antimicrobial strategies in burying beetles breeding on carrion. Proc. Natl. Acad. Sci. U.S.A 105, 17890–17895. doi: 10.1073/pnas.0805403105
Santos, E. S. A., and Nakagawa, S. (2012). The costs of parental care: a meta−analysis of the trade−off between parental effort and survival in birds. J. Evol. Biol. 25, 1911–1917. doi: 10.1111/j.1420-9101.2012.02569.x
Sanz, J. J. (2001). Experimentally reduced male attractiveness increases parental care in the pied flycatcher Ficedula hypoleuca. Behav. Ecol. 12, 171–176. doi: 10.1093/beheco/12.2.171
Sanz, J. J., Kranenbarg, S., and Tinbergen, J. M. (2000). Differential response by males and females to manipulation of partner contribution in the great tit (Parus major). J. Anim. Ecol. 69, 74–84. doi: 10.1046/j.1365-2656.2000.00373.x
Sargent, R. C. (1988). Paternal care and egg survival both increase with clutch size in the fathead minnow, Pimephales promelas. Behav. Ecol. Sociobiol. 23, 33–37. doi: 10.1007/bf00303055
Schwagmeyer, P. L., Mock, D. W., and Parker, G. A. (2002). Biparental care in house sparrows: negotiation or sealed bid? Behav. Ecol. 13, 713–721. doi: 10.1093/beheco/13.5.713
Scott, M. P. (1990). Brood guarding and the evolution of male parental care in burying beetles. Behav. Ecol. Sociobiol. 26, 31–39. doi: 10.1007/bf00174022
Scott, M. P. (1998). The ecology and behavior of burying beetles. Annu. Rev. Entomol. 43, 595–618. doi: 10.1146/annurev.ento.43.1.595
Scott, M. P., and Traniello, J. F. A. (1990). Behavioral and ecological correlates of male and female parental care and reproductive success in burying beetles (Nicrophorus spp.). Anim. Behav. 39, 274–283. doi: 10.1016/S0003-3472(05)80871-1
Smiseth, P. T., Dawson, C., Varley, E., and Moore, A. J. (2005). How do caring parents respond to mate loss? Differential response by males and females. Anim. Behav. 69, 551–559. doi: 10.1016/j.anbehav.2004.06.004
Smiseth, P. T., and Moore, A. J. (2004). Behavioral dynamics between caring males and females in a beetle with facultative biparental care. Behav. Ecol. 15, 621–628. doi: 10.1093/beheco/arh053
Smiseth, P. T., Ward, R. J. S., and Moore, A. J. (2006). Asynchronous hatching in Nicrophorus vespilloides, an insect in which parents provide food for their offspring. Funct. Ecol. 20, 151–156. doi: 10.1111/j.1365-2435.2006.01072.x
Stearns, S. C. (1989). Trade-offs in life-history evolution. Funct. Ecol. 3, 259–268. doi: 10.2307/2389364
Suzuki, S., and Nagano, M. (2009). To compensate or not? Caring parents respond differentially to mate removal and mate handicapping in the burying beetle, Nicrophorus quadripunctatus. Ethology 115, 1–6. doi: 10.1111/j.1439-0310.2008.01598.x
Szentirmai, I., Székely, T., and Komdeur, J. (2007). Sexual conflict over care: antagonistic effects of clutch desertion on reproductive success of male and female penduline tits. J. Evol. Biol. 20, 1739–1744. doi: 10.1111/j.1420-9101.2007.01392.x
Tinbergen, J. M., and Boerlijst, M. C. (1990). Nestling weight and survival in individual great tits (Parus major). J. Anim. Ecol. 59, 1113–1127. doi: 10.2307/5035
Trivers, R. L. (1972). “Parental investment and sexual selection,” in Sexual Selection and the Descent of Man, ed. B. Campbell (Chicago: Aldine Press), 136–179.
Trivers, R. L. (1974). Parent-offspring conflict. Amer. Zool. 14, 249–264. doi: 10.1093/icb/14.1.249
Trumbo, S. T. (1991). Reproductive benefits and the duration of paternal care in a biparental burying beetle, Necrophorus orbicollis. Behav. 117, 82–105. doi: 10.1163/156853991X00139
Trumbo, S. T. (2017). Feeding upon and preserving a carcass: the function of prehatch parental care in a burying beetle. Anim. Behav. 130, 241–249. doi: 10.1016/j.anbehav.2017.07.001
Trumbo, S. T., and Robinson, G. E. (2004). Nutrition, hormones and life history in burying beetles. J. Insect. Physiol. 50, 383–391. doi: 10.1016/j.jinsphys.2004.01.008
Trumbo, S. T., and Xhihani, E. (2015). Influences of parental care and food deprivation on regulation of body mass in a burying beetle. Ethology 121, 985–993. doi: 10.1111/eth.12413
Walling, C. A., Stamper, C. E., Smiseth, P. T., and Moore, A. J. (2008). The quantitative genetics of sex differences in parenting. Proc. Natl. Acad. Sci. U.S.A. 105, 18430–18435. doi: 10.1073/pnas.0803146105
Ward, R. J. S., Cotter, S. C., and Kilner, R. M. (2009). Current brood size and residual reproductive value predict offspring desertion in the burying beetle Nicrophorus vespilloides. Behav. Ecol. 20, 1274–1281. doi: 10.1093/beheco/arp132
Williams, G. C. (1966). Natural selection, the costs of reproduction, and a refinement of Lack’s principle. Am. Nat. 100, 687–690. doi: 10.2307/2459305
Williams, H. M., and DeLeon, R. L. (2020). Using artificial intelligence classification of videos to examine the environmental, evolutionary and physiological constraints on provisioning behavior. J. Avian. Biol. 51:8. doi: 10.1111/jav.02424
Woelber, B. K., Hall, C. L., and Howard, D. R. (2018). Environmental cues influence parental brood structure decisions in the burying beetle Nicrophorus marginatus. J. Ethol. 36, 55–64. doi: 10.1007/s10164-017-0527-7
Wolf, M., Van Doorn, G. S., Leimar, O., and Weissing, F. J. (2007). Life-history trade-offs favour the evolution of animal personalities. Nature 447, 581–584. doi: 10.1038/nature05835
Wright, J., and Cuthill, I. (1990). Manipulation of sex differences in parental care: the effect of brood size. Anim. Behav. 40, 462–471. doi: 10.1016/S0003-3472(05)80526-3
Keywords: Nicrophorus vespilloides, parental care system, brood size, sex difference, reproductive trade-offs
Citation: Wang W, Ma L, Versteegh MA, Wu H and Komdeur J (2021) Parental Care System and Brood Size Drive Sex Difference in Reproductive Allocation: An Experimental Study on Burying Beetles. Front. Ecol. Evol. 9:739396. doi: 10.3389/fevo.2021.739396
Received: 10 July 2021; Accepted: 14 September 2021;
Published: 05 October 2021.
Edited by:
Veronika Bókony, Plant Protection Institute, Centre for Agricultural Research, HungaryReviewed by:
Matthieu Paquet, Swedish University of Agricultural Sciences, SwedenAlejandro Cantarero, University of Turku, Finland
Copyright © 2021 Wang, Ma, Versteegh, Wu and Komdeur. This is an open-access article distributed under the terms of the Creative Commons Attribution License (CC BY). The use, distribution or reproduction in other forums is permitted, provided the original author(s) and the copyright owner(s) are credited and that the original publication in this journal is cited, in accordance with accepted academic practice. No use, distribution or reproduction is permitted which does not comply with these terms.
*Correspondence: Jan Komdeur, ai5rb21kZXVyQHJ1Zy5ubA==
†ORCID: Jan Komdeur, orcid.org/0000-0002-9241-0124; Hua Wu, orcid.org/0000-0002-9883-4091; Wenxia Wang, orcid.org/0000-0002-2376-0323; Long Ma, orcid.org/0000-0002-6911-0684