- 1Department of Biological and Environmental Science, University of Jyväskylä, Jyväskylä, Finland
- 2Department of Zoology, Stockholm University, Stockholm, Sweden
- 3Zoological Institute and Museum, University of Greifswald, Greifswald, Germany
- 4CIBIO/InBIO, Research Center in Biodiversity and Genetic Resources, University of Porto, Porto, Portugal
- 5Department of Biological Sciences, University of South Carolina, Columbia, SC, United States
- 6Ecologie Systématique Evolution, CNRS, AgroParisTech, Université Paris-Saclay, Université Paris-Sud, Orsay Cedex, France
Sperm quantity and quality are key features explaining intra- and interspecific variation in male reproductive success. Spermatogenesis is sensitive to ionizing radiation and laboratory studies investigating acute effects of ionizing radiation have indeed found negative effects of radiation on sperm quantity and quality. In nature, levels of natural background radiation vary dramatically, and chronic effects of low-level background radiation exposure on spermatogenesis are poorly understood. The Chernobyl region offers a unique research opportunity for investigating effects of chronic low-level ionizing radiation on reproductive properties of wild organisms. We captured male bank voles (Myodes glareolus) from 24 locations in the Chernobyl exclusion zone in 2011 and 2015 and collected information on sperm morphology and kinetics. The dataset is limited in size and there overall was a relatively weak correlation between background radiation and sperm quality. Still, some correlations are worth discussing. First, mid-piece segments of spermatozoa tended to be smaller in bank vole males from areas with elevated background radiation levels. Second, we demonstrated a significant positive relationship between background radiation dose rates and the proportion of static spermatozoa among males within and among study locations after 10 as well as 60 min of incubation. Our results provide novel evidence of damaging effects of low dose ionizing radiation on sperm performance in wild rodent populations, and highlight that this topic requires further study across the natural gradients of background radiation that exist in nature.
Introduction
The quality and quantity of sperm are key drivers of male reproductive success across taxa and strongly impact fitness (Anderson and Dixson, 2002; Firman and Simmons, 2010; Tourmente et al., 2011; Fisher et al., 2016). Producing high-quality sperm is costly (Hosken et al., 2003; Lüpold et al., 2016), and, therefore, sperm quantity and quality is subject to trade-offs in environments with competing stressors (Vladicì et al., 2002). One such stressor is background radiation, which exists at varying levels across natural habitats and results from multiple sources (Figure 1a), such as for instance atmospheric UV radiation and earth crust radon radiation (Cinelli et al., 2019).
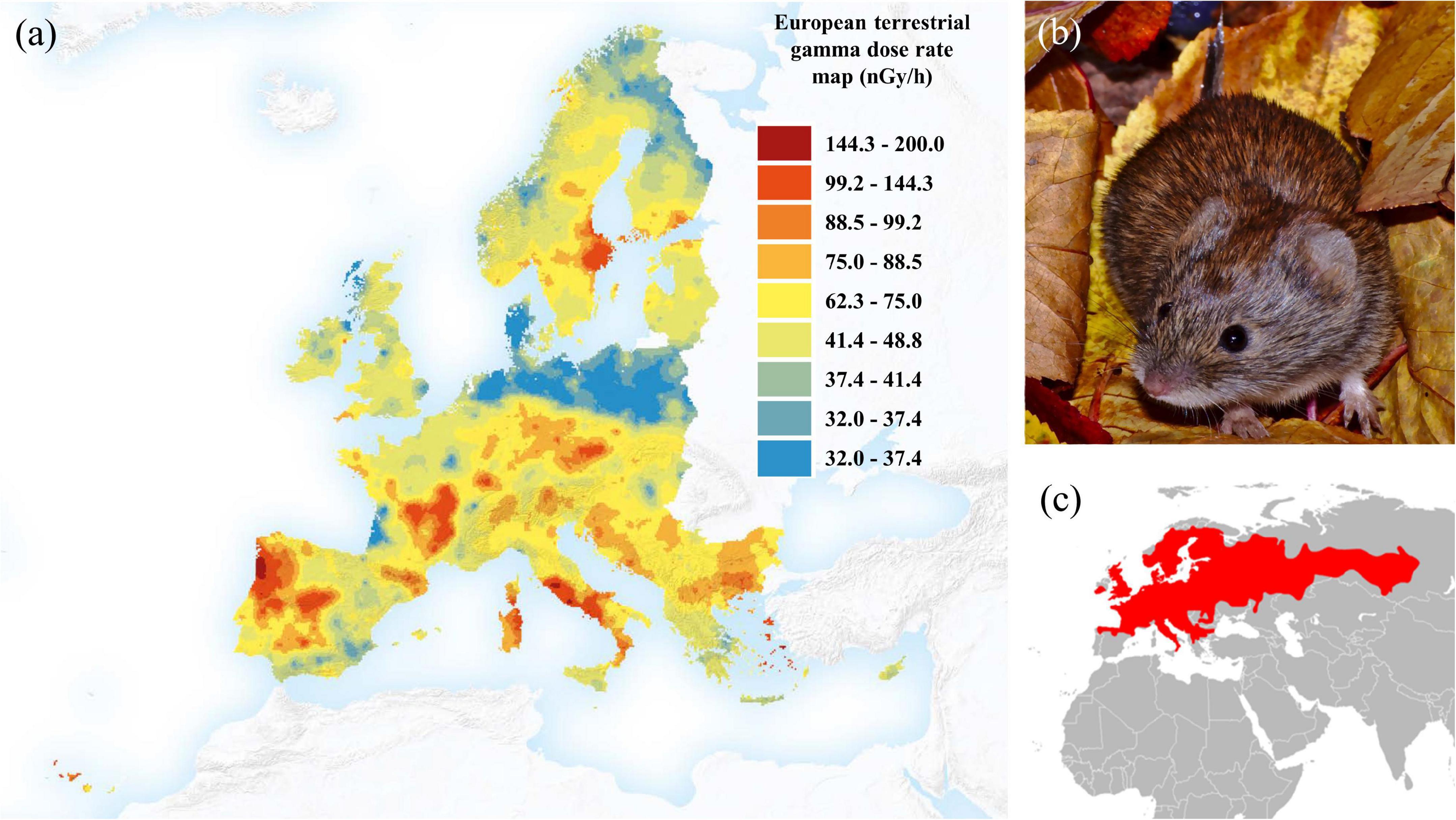
Figure 1. Panel (a) shows the dose rate, in nGy/h that a person would receive from terrestrial radiation if he/she spends all the reference time in a location outdoor in which the soil has fixed U, Th and K concentrations (modified based on Cinelli et al., 2019). Panel (b) shows the study organism, the bank voles Myodes glareolus (picture by Z. Boratyński), while panel (c) displays the distribution range of M. glareolus across most of Europe, shown in red (data from www.iucnredlist.org).
While it has been demonstrated that chronic radiation exposure can have negative effects on reproductive traits in several organisms (Yamashiro et al., 2013; Møller and Mousseau, 2015; Lehmann et al., 2016; Fukunaga et al., 2017; Mappes et al., 2019; Mousseau and Møller, 2020), the mechanisms behind these effects, or their transferability across species and sexes, remain unclear. It could involve indirect effects through radiation-induced oxidative stress (a response to increased level of reactive oxygen species), direct alteration of molecular structures in cells, or DNA damage from high doses of radiation (Azzam et al., 2012; Einor et al., 2016). Consequently, changes in chronic radioactive exposure can cause stress and reduce resources allocated to these reproductive traits (Congdon et al., 2001). Changes in swimming behavior and morphology of sperm in wild birds have been reported (Møller et al., 2008; Bonisoli-Alquati et al., 2011; Hermosell et al., 2013), but whether or not this effect is evident in mammals, with potential for translation to humans, is currently unknown.
We investigated this question using an ecological model system, the bank vole (Myodes glareolus), which is a common rodent species across forested temperate habitats in Eurasia (Figure 1b; Boratyński et al., 2014a). We use the Chernobyl exclusion zone, where bank voles are abundant across gradients of background radiation, as a natural laboratory, to investigate effects of background radiation on important male life-history traits. Previous research has shown that female bank voles have smaller litter sizes as a consequence of high radiation exposure (Lehmann et al., 2016), but whether similar patterns are found in the much more dispersive male voles (Kozakiewicz et al., 2007; Boratyński et al., 2020) is unclear. The sperm mid-piece is believed to be responsible for generating the energy needed for sperm mobility (Turner, 2006) and the volume of sperm mid-piece correlates positively with sperm swimming velocity and endurance in rodents (Firman and Simmons, 2010; Montoto et al., 2011). We therefore hypothesized that overall performance of sperm, including fitness-related morpho-physiological traits (e.g., the volume of the energetic engine of spermatozoids, the mid-piece; Fisher et al., 2016) and mobility capability (i.e., swimming velocity and endurance; Tourmente et al., 2015), will be reduced in males exposed to habitats with high background radiation.
Materials and Methods
Study System
The bank vole (Myodes/Clethrionomys glareolus) is widespread throughout Eurasia (Figure 1c) and abundant in a variety of forest habitats (Spitzenberger, 1999). Rapid reproduction and population turnover make this species ideal for evolutionary-ecological studies (Ledevin et al., 2010). Multiple mating (Boratyński and Koteja, 2010) is an important reproductive tactic in both male and female bank voles, which emphasizes the importance of sperm quality and sperm competition (Mokkonen et al., 2012).
Forty-nine sexually mature bank vole males were captured during summer (June–July) 2011 (33 individuals) and 2015 (16 individuals) from a total of 24 locations. The home range of male bank voles is 0.09 ha on average (Boratyński et al., 2020), which is relatively small considering the spatial variation of background radiation present in the Chernobyl Exclusion Zone. Trapping locations were chosen across known background radiation gradients within and around the Chernobyl Exclusion Zone (1–50 km from the nuclear power plant) (Supplementary Figure 1 and Supplementary Table 1). Animals were captured with Ugglan live traps (Grahnab, Sweden) baited with sunflower seeds and potatoes. Lines of 20 traps were set for one night at study locations. Environmental radiation level was measured near each trap using a hand dosimeter (Gamma-Scout w/ALERT Radiation detector/Geiger Counter, Gamma-Scout GmbH & Co. KG, Germany, with shielding set to detect only gamma radiation) and mean values were calculated for each study location. Radiation levels measured using dosimeters have high repeatability among days and years and are strongly positively correlated with doses measured from soil samples, plants and animals bodies (Møller and Mousseau, 2013; Galván et al., 2014; Boratyński et al., 2016; Lavrinienko et al., 2020).
Males captured in 2011 were sacrificed by cervical dislocation after capture, and body measurements recorded. After this, animals were frozen at −20°C, transported to Jyväskylä, Finland (frozen), and morphometric measurements were done in autumn 2013. The same was done to animals captured in 2015 (analyzed in 2017 after 2 years in the freezer). We did not fix the sperm since they were extracted from frozen animals (for morphology analyses) or used fresh (for kinetic analyses) as described below. All experiments complied with the legal requirements and adhered closely to international guidelines for the use of animals in research. The necessary permissions were obtained from the Animal Experimentation Committee for these experiments (permission no. ESAVI/3834/04.10.03/2011 and ESAVI/7256/04.10.07/2014). All samples were given an arbitrary code, from which sampling year could be read, but sample location and background radiation could not. The analyses described below were performed by the same person (the first author) blindly with respect to radiation levels at the site of collection.
Sperm Morphometrics and Performance
To record sperm morphology, testes (n = 49) were dissected from thawed individuals and testis, preputial gland, prostate gland and seminal vesicle mass measured with an analytic balance (Mettler Toledo, XS105 DualRange, precision: 0.01 g). Mature spermatozoa were collected from cauda epididymis in phosphate buffered saline (PBS, pH 7.4) and this sperm suspension was used for assessment of sperm numbers and sperm morphology. Sperm numbers were quantified from a hemocytometer using Leitz Diaplan microscope (Leitz, Wetzlar, Germany) with 500× magnification as described previously (Wang, 2002). Then dimensions of 30 sperm per male were measured under 1000× magnitude (LEICA DM-RBE Fluorescence Microscope with Retica 2000R Fast camera) for each sperm cell using ImageJ v.1.50i Software (National Institutes of Health, Bethesda, MD, United States). Measurements included: head length, width and area (length x width, μm2), mid-piece length, width and volume (calculated as cylinder: π × length × width, μm3), length of the principal piece and length of whole spermatozoon. A repeatability test was performed to ensure low measurement variability, by re-measuring 10 cells. The repeatability estimates were obtained using the package rptR-package for R R 4.1.0 (RCoreTeam, 2016; Stoffel et al., 2017).
We investigated abnormalities of the sperm, and all individuals had some proportion of deformed sperm (e.g., broken acrosome, bubbles in the mid-piece, bent tail). However, since rates were very low across the radiation gradients, we assumed that freezing of the animals could have caused some of these abnormalities. Therefore, we did not pursue this analysis further, even though it in hindsight might have been wise.
Specimens examined for sperm swimming properties were captured in 2015 (N = 14). Sperm mobility was analyzed on fresh mature spermatozoa collected from the right cauda epididymis immediately after sacrificing animals. Previous research showed that sperm of muroid rodents stays viable up to 3 h (Tourmente et al., 2015) and our pre-examination with Finnish bank voles showed similar results (Kivisaari K, unpublished). We expected that radiation induced differences would emerge within the 1st hour. Spermatozoa were allowed to swim into pre-warmed medium (37°C, nutrient mixture F-10 Ham, Sigma Aldrich N6635, and 1% bovine serum albumin, BSA, Sigma Aldrich B4287, pH 7), incubated for 10 min and after that, a sample (30 μl) was used for assessment of sperm velocity parameters. Sperm velocity parameters were assessed using a computer assisted semen analysis system (CASA, ISAS v1, PROiSER R + D, Spain) with a phase contrast microscope (UB 203i Trinocular microscope with PROISER 782 monochrome camera). The sperm sample was placed into one well in a microscope slide (Erie Scientific Company: ER-308B-CE24, 10 wells, 6.7 mm) and examined using Ph-optics (50 images per second). Four videos of 4 s duration each were recorded for each male’s sperm sample. Sperm swimming mobility was assessed by collecting velocity data at two time points, after a short (10 min, reflecting sperm initial mobility) and a long (1 h, reflecting sperm endurance) incubation. The proportion of progressive (movement >80 μm/s), motile (20–80 μm/s) and static (non-mobile) sperm were recorded from each track. Note, however, that static sperm could include sperm that are still alive, and, therefore, should not be treated as dead with certainty. Additionally, sperm kinetic parameters: curvilinear and rectilinear speeds, average path velocity (μm/s), linearity index (%), average amplitude of the head lateral moving (μm) and beat frequency (Hz) were recorded from progressive and motile sperm (Mortimer, 2000).
Data Analysis
First, sperm numbers in the cauda were used as a dependent variable in a generalized linear mixed model (GLMM with Gaussian errors), where the main predictors were environmental radiation level (log10-transformed) and absolute testes mass as well as body mass (log10-transformed) as covariates to control for male reproductive investment (Parker and Ball, 2005), year as fixed effect and male identity nested within trapping location as random effect. Then two GLMM models (Gaussian errors) were set up testing effects of radiation level (log10-transformed) on preputial gland mass and prostate mass with absolute testes mass as well as body mass as covariates. We then tested for a functional relationship between sperm morphology and mobility from the males sampled 2015. For these males we performed a series of Pearson’s product-moment correlation analyses between morphology traits and mobility traits (log10-transformed) since both metrics were taken from the same individuals. To assess radiation effects on sperm morphology, dependent variables of head length or area, mid-piece length or volume, principal piece length and total sperm length, were analyzed in six separate GLMM models (Gaussian errors). The main predictors were background radiation level (log10-transformed) and absolute testes mass as well as body mass as covariates, year as fixed effect and male identity nested within trapping location as random effect. Second, sperm mobility was determined as the proportion progressive, motile and static sperm after a 10 min and a 60 min incubation. The factor “replicate”, describing the repeated estimates of mobility (after 10 and 60 min), was included in three separate repeated-measures GLMM models (assuming beta-distributed residuals). Other predictors in all these models were: radiation level (log10-transformed), absolute testes mass as well as body mass as covariates, and male identity nested within trapping location as a random effect. We then tested radiation effects on sperm kinetic parameters in six separate GLMM models with radiation level (log10-transformed) as main predictor, absolute testes mass as well as body mass as covariates, and male identity nested within trapping location as random effect. Statistical analyses were conducted using R 4.1.0 and packages: lme4, nlme, glmmADMB, ggplot2 (Wickham, 2009; Skaug et al., 2014; Bates et al., 2015; RCoreTeam, 2016; Pinheiro et al., 2021). Generally we applied Bonferroni correction, wherein we adjusted α by dividing standard (p = 0.05) significance level by the number of tests conducted for given group of analyses. These adjusted levels are reported in the tables.
Results
Reproductive Organ Size and Sperm Numbers
There was large variation in sperm numbers (Supplementary Table 2), and testis mass (representing the reproductive investment of males) was strongly positively correlated with sperm numbers (Supplementary Table 3). However, we did not find any significant correlations between radiation and sperm numbers (Supplementary Table 3). Testis mass was strongly positively correlated with prostate gland mass, but not with preputial gland mass (Supplementary Table 3). There was no significant relationship between radiation and testis mass (Table 1), nor between radiation and masses of preputial glands and prostate (prostate glands and seminal vesicles), after controlling for testis and body mass (Supplementary Table 3).
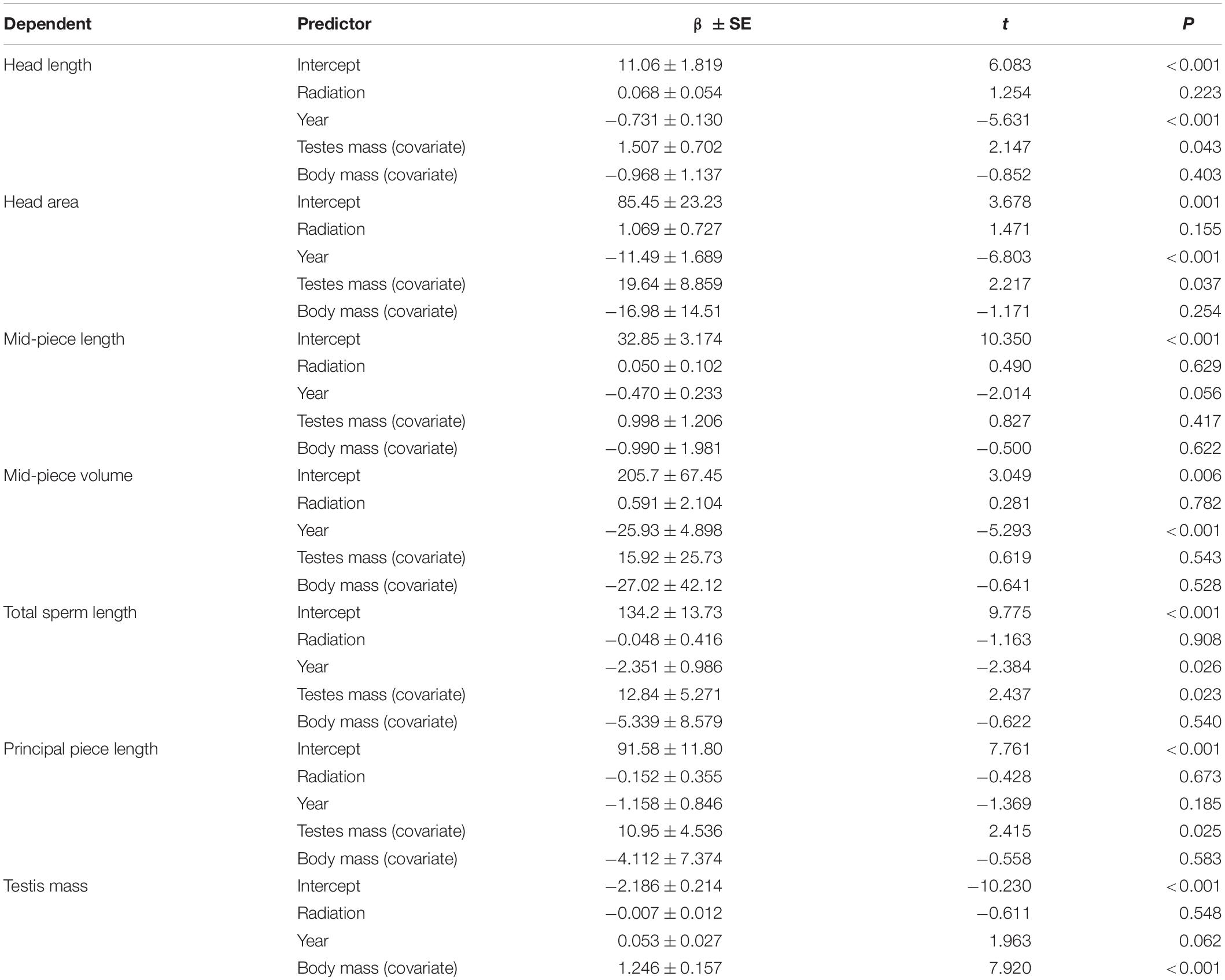
Table 1. Relationship of radiation and sperm morphology in 49 bank voles. We analyzed male sperm morphological traits in relation to radiation (log10) and testes as well as body mass (to account for the reproductive status of each male) in separate GLMMs (see material and methods for models). After correction for multiple testing, α is set at 0.00625.
Correlation Between Sperm Morphology and Mobility
A number of Pearson’s product-moment correlation analyses between sperm morphometric traits and mobility traits were performed and while overall traits were not strongly correlated, some relationships do stand out. These include a positive correlation trend between mid-piece length and proportion static sperm after 1 h (r = 0.52, t = 2.099, df = 12, p = 0.058), a negative correlation between sperm mid-piece volume and proportion progressive sperm after 1 h (r = −0.63, t = −2.785, df = 12, p = 0.017), a positive correlation between sperm mid-piece volume and the proportion motile sperm after 10 min (r = 0.57, t = 2.396, df = 12, p = 0.034) and a positive correlation between sperm mid-piece volume and the proportion motile sperm after 1 h (r = 0.59, t = 2.566, df = 12, p = 0.025). While none of these results are upheld after correction for multiple comparisons, likely due to the limited sample size, they do imply a functional relationship between sperm morphology and mobility.
Sperm Morphology
We measured six sperm morphometric traits (and report high repeatability, intra-class correlation coefficients and its 95% CI, in our measurements): total sperm length [τ = 0.99 (0.95–1.00)], tail length [τ = 0.987 (0.95–1.00)], head length [τ = 0.92 (0.77–0.98)] and width [τ = 0.77 (0.33–0.95)], and mid-piece length [τ = 0.95 (0.84–0.99)] and width [τ = 0.98 (0.94–1.00)], and calculated sperm head area and mid-piece volume (Supplementary Table 2). Nearly all sperm morphometric measures were inter-correlated (Pearson: r = 0.18–0.97). Overall there were only weak correlations between morphological traits and radiation (Table 1). We further analysed the interaction between radiation and year for these traits and found it to be significant only for sperm mid-piece length (Supplementary Table 4). An analysis of the data partitioned by the year (either 2011 or 2015) revealed that the effect was driven by year 2011 (t = −2.627, β ± SE = −0.336 ± 0.128, p = 0.020), which contains the larger dataset (Figure 2A). After correcting for multiple testing this effect was no longer statistically significant, and should therefore be treated with caution.
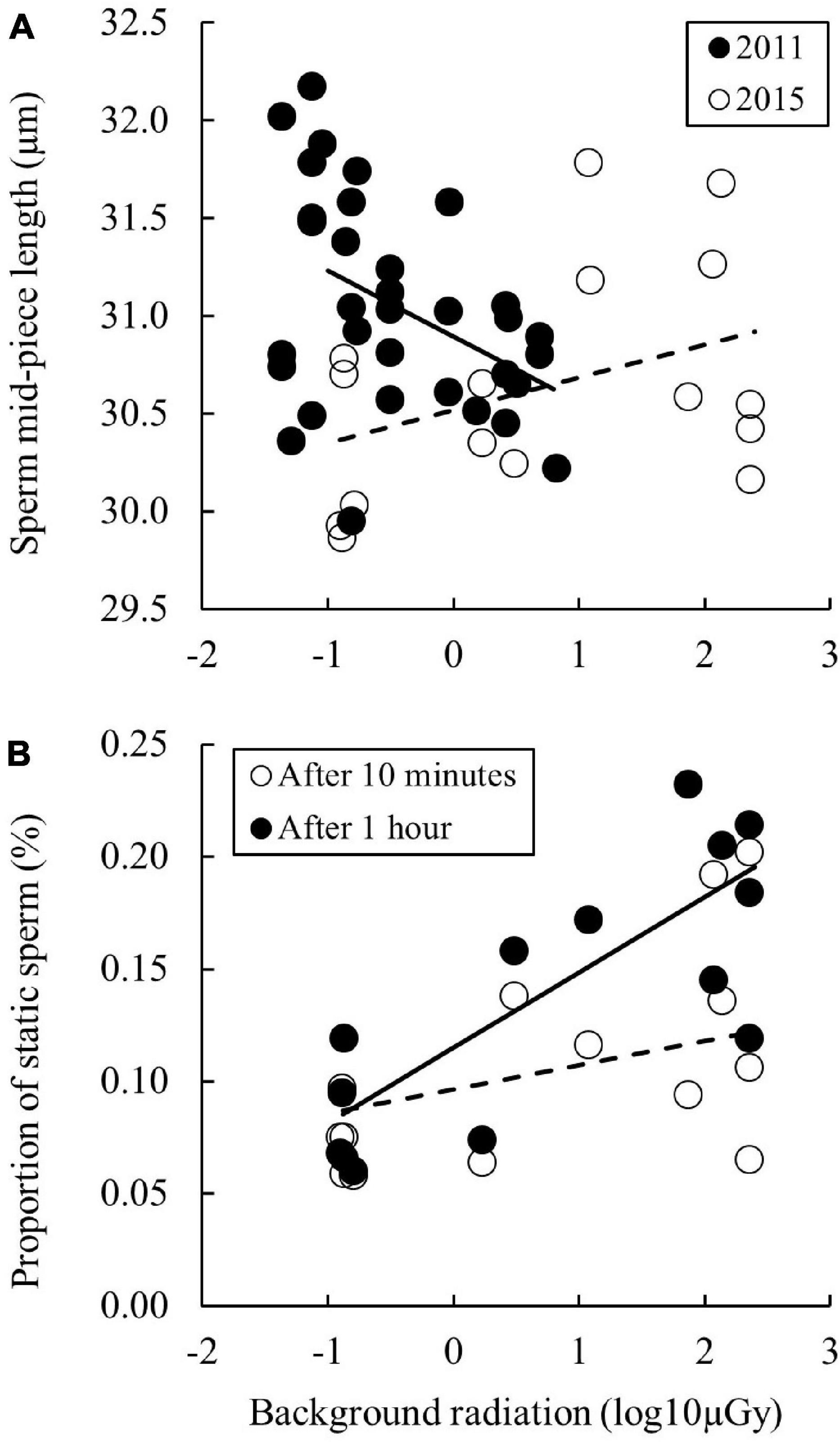
Figure 2. Figure showing how (A) sperm mid-piece length and (B) sperm mobility changes in male bank voles as a consequence of chronic exposure to radiation. In panel (A) data from 2015 (open symbols and dashed line) and 2011 (filled symbols and solid line) are plotted, while in panel (B) the first measurement (open symbols and dashed line) was taken after 10 min of incubation and the second (filled symbols and solid line) after 60 min of incubation. Lines represent predicted values from the mixed models, and sample sizes were 49 males in (A) and 14 males in (B).
Sperm Mobility
There was a significant positive relationship between background radiation and proportion of static sperm (Table 2 and Figure 2B), but not between background radiation and progressive or motile sperm. We also found an increased proportion of static sperm between replicate measures after 10 min and 1 h (t = 3.58, β ± SE = 0.334 ± 0.093, p < 0.001). The interactions between replicate and radiation for progressive (t = −0.63, β ± SE = −0.056 ± 0.088, p = 0.52), motile (t = −0.04, β ± SE = −0.003 ± 0.086, p = 0.97) or static sperm (t = 1.13, β ± SE = 0.088 ± 0.078, p = 0.26) were non-significant. Thus, higher radiation is associated with a higher proportion of static sperm in both the 10 and 60 min replicate measures. These results were upheld after correcting for multiple testing. Further, testis mass was significantly related to both the proportion progressive and static sperm but not to the proportion motile sperm (Table 2). Sperm kinetic parameter tests did not display any significant correlations to radiation (Supplementary Table 5).
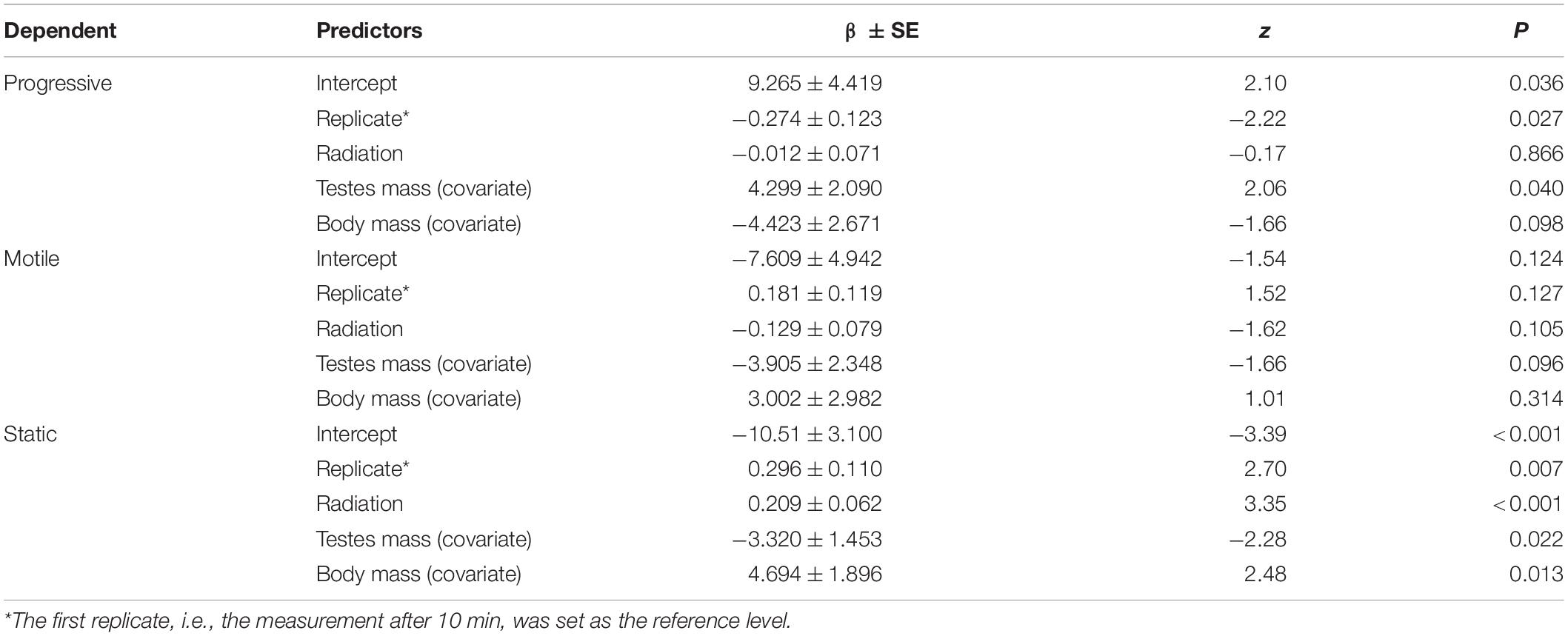
Table 2. Test of radiation effects on sperm mobility (progressive, motile and static) in 14 bank voles. Replicate reflects an internal correlation between motility after 10 and after 60 min. All interactions between this variable and the predictors were tested and found to be non-significant. After correction for multiple testing, α is set at 0.01666667.
Discussion
Sperm quality and quantity are key elements of male reproductive success, and hence fitness (Tourmente et al., 2011; Fisher et al., 2016). The results of the present study overall indicate weak correlations between background radiation and sperm quality, possibly suggesting ample compensatory mechanisms, e.g., in the form of antioxidant production (Boratyński et al., 2014b). However, for sperm swimming endurance, a link between chronic low-dose radiation exposure and key fitness-related traits could be observed. Thus, sperm quality is one possible causal mechanism underlying the smaller litter sizes previously observed in bank voles from areas with high levels of background radiation (Lehmann et al., 2016). These results should motivate further studies of the mechanisms driving relationships between natural background radiation and life-history traits.
Sperm mid-piece size has been shown to correlate positively with sperm swimming velocity and endurance in rodents (Firman and Simmons, 2010; Montoto et al., 2011), implying that a reduction in mid-piece size could reduce fertility in bank voles that engage in sperm competition. A possible mechanism causing mid-piece shortening could be radiation induced mitochondrial damage (Kim et al., 2006). Mitochondrial DNA is particularly sensitive to oxidative damage due to limited DNA repair mechanisms and the lack of histones (Kim et al., 2006). Smaller mid-pieces could thus indicate mitochondrial damage and explain the sperm mobility results, as also indicated by the correlation analyses. However, since ATP content can actually be lower in sperm with longer mid-pieces in birds (Bennison et al., 2016; Mendonca et al., 2018), it would be important to follow up morphological analyses with kinetic, or whole-organism studies of the fitness consequences of sperm morphological variation.
It is generally accepted that a large proportion of static sperm can be indicative of low male fertility (Turner, 2006). Our data indeed show a significant positive correlation between the proportion of static sperm and increasing radiation level. The time lag between ejaculation and fertilization varies greatly between species and the female reproductive tract filters out sperm with inferior morphology and mobility (Orr and Brennan, 2015). For this reason, sperm swimming endurance can be more important to male fitness than initial mobility (Orr and Brennan, 2015).
Radiation can also affect sperm quality indirectly, through variation in life-history traits. For example, radiation can be viewed as an environmental stressor that causes trade-offs between investment in survival versus reproduction, resulting in low quality sperm (Congdon et al., 2001; Vladicì et al., 2002). Even low level chronic radiation causes small amounts of cellular damage, and resource allocation could be biased toward DNA and cellular repair mechanisms (Kim et al., 2006; Azzam et al., 2012). In this context it is important to note that damaged DNA in sperm cannot be repaired (Smith et al., 2016), thus highlighting impact of even moderate radiation-induced damage. Future approaches could include measuring oxidative phosphorylation from fresh sperm samples to further test the hypothesis of radiation-induced mitochondrial damage. In any case, future studies will require a significantly larger sample (Makin and Orban de Xivry, 2019), since the effect sizes discovered here, and in other studies on background radiation adaptation (Boratyński et al., 2021), are relatively small.
For sperm morphology, year had a major impact on the studied traits. While effort was taken in standardizing sampling time and effort (Supplementary Table 1) some factors influencing sperm quality warrant discussion. In birds, flagellum length and sperm velocity has been shown to increase during the mating season, whereas sperm numbers declined (Lüpold et al., 2012). The same study also found that sperm morphological traits correlated positively with the number of mates the male had and how much offspring it produced (Lüpold et al., 2012). In another study, sperm length decreased during the breeding season (Schmoll et al., 2018). In the current study, all sampling was done within a two-week period during the breeding season, and in Chernobyl, bank voles hardly ever live through two mating seasons (around 80% voles do not survive first winter, e.g., Boratyński et al., 2010). The aforementioned studies suggest seasonal changes occur over much longer time-periods (e.g., the whole breeding cycle) (Lüpold et al., 2012; Schmoll et al., 2018). In bank voles, research has shown that there is a difference in sperm numbers and testicle size between dominant and subordinate males where the former group has larger number of sperm and larger testes (Lemaître et al., 2012). However, the same study also shows that subordinate males do not compensate for this and do not have faster sperm and for example do not invest more in copulatory plugs (Lemaître et al., 2012). Taken together, we do not believe seasonality, age or mating history has major impact on the interpretation of the results presented here.
It has been demonstrated that animals may show radioadaptive responses to very high levels of background radiation, for instance in the Chernobyl Exclusion Zone (Galván et al., 2014). It is now important to investigate also the extent to which populations exposed to lower levels of radiation, across natural habitats show radioadaptive responses (Tapio and Jacob, 2007; Okano et al., 2016; Fuller et al., 2019). As levels of background radiation vary dramatically (Cinelli et al., 2019) it should constitute a significant, either direct (e.g., DNA-damage) or indirect (e.g., diverting resources to antioxidant production), selective factor. Yet, natural variation in background radioactivity is very rarely incorporated in life-history contexts.
Data Availability Statement
The original contributions presented in the study are included in the article/Supplementary Material, further inquiries can be directed to the corresponding author.
Ethics Statement
The animal study was reviewed and approved by Animal Experimentation Committee Finland Permit numbers ESAVI/3834/04.10.03/2011 and ESAVI/7256/04.10.07/2014.
Author Contributions
KK, ZB, SC, PL, TM, TAM, and AM: design. KK, ZB, PL, and TM: data collection. KK, ZB, PL, SC, and TM: statistical analyses. KK: write the manuscript. TAM, AM, PL, and ZB: critically appraised and edited the manuscript. All authors read and approved the manuscript before submission.
Funding
Academy of Finland to TM (Grant No. 268670), and Emil Aaltonen Foundation (Grant No. 160107) and Oskar Öflund Foundation to KK financially supported this study. The Samuel Freeman Charitable Foundation, The American Council of Learned Societies, and the CNRS (France) provided financial support for TAM and AM.
Conflict of Interest
The authors declare that the research was conducted in the absence of any commercial or financial relationships that could be construed as a potential conflict of interest.
Publisher’s Note
All claims expressed in this article are solely those of the authors and do not necessarily represent those of their affiliated organizations, or those of the publisher, the editors and the reviewers. Any product that may be evaluated in this article, or claim that may be made by its manufacturer, is not guaranteed or endorsed by the publisher.
Acknowledgments
We would like to thank Gennadi Milinevsky, Igor Chizhevsky, Serhii Kireyey, Anatoly Nosovsky, and Maksym Ivanenko for logistic support and help in organizing fieldwork and permits, and Anton Lavrinienko and Eugene Tukalenko for their efforts in the field.
Supplementary Material
The Supplementary Material for this article can be found online at: https://www.frontiersin.org/articles/10.3389/fevo.2021.736389/full#supplementary-material
References
Anderson, M., and Dixson, A. (2002). Motility and the midpiece in primates. Nature 416:496. doi: 10.1038/416496a
Azzam, E. I., Jay-Gerin, J. P., and Pain, D. (2012). Ionizing radiation-induced metabolic oxidative stress and prolonged cell injury. Cancer Lett. 327, 48–60. doi: 10.1016/j.canlet.2011.12.012
Bates, D., Mächler, M., Bolker, B., and Walker, S. (2015). Fitting linear mixed-effects models using lme4. J. Stat. Softw. 67, 1–48.
Bennison, C., Hemmings, N., Brookes, L., Slate, J., and Birkhead, T. (2016). Sperm morphology, adenosine triphosphate (ATP) concentration and swimming velocity: unexpected relationships in a passerine bird. Proc. R. Soc. B Biol. Sci. 283:1558. doi: 10.1098/rspb.2016.1558
Bonisoli-Alquati, A., Møller, A. P., Rudolfsen, G., Saino, N., Caprioli, M., Ostermiller, S., et al. (2011). The effects of radiation on sperm swimming behavior depend on plasma oxidative status in the barn swallow (Hirundo rustica). Comp. Biochem. Physiol. Mol. Integr. Physiol. 159, 105–112. doi: 10.1016/j.cbpa.2011.01.018
Boratyński, Z., and Koteja, P. (2010). Sexual and natural selection on body mass and metabolic rates in free-living bank voles. Funct. Ecol. 24, 1252–1261. doi: 10.1111/j.1365-2435.2010.01764.x
Boratyński, Z., Koskela, E., Mappes, T., and Oksanen, T. A. (2010). Sex-specific selection on energy metabolism – selection coefficients for winter survival. J. Evol. Biol. 23, 1969–1978. doi: 10.1111/j.1420-9101.2010.02059.x
Boratyński, Z., Melo-Ferreira, J., Alves, P. C., Berto, B., Koskela, E., Pentikäinen, O. T., et al. (2014a). Molecular and ecological signs of mitochondrial adaptation: consequences for introgression? Heredity 113, 277–286. doi: 10.1038/hdy.2014.28
Boratyński, Z., Lehmann, P., Mappes, T., Mousseau, T. A., and Møller, A. P. (2014b). Increased radiation from Chernobyl decreases the expression of red colouration in natural populations of bank voles (Myodes glareolus). Sci. Rep. 4:7141. doi: 10.1038/srep07141
Boratyński, Z., Arias, J. M., Garcia, C., Mappes, T., Mousseau, T. A., Møller, A. P., et al. (2016). Ionizing radiation from Chernobyl affects development of wild carrot plants. Sci. Rep. 6:39282. doi: 10.1038/srep39282
Boratyński, Z., Szyrmer, M., and Koteja, P. (2020). The metabolic performance predicts home range size of bank voles: a support for the behavioral-bioenergetics theory. Oecologia 193, 547–556. doi: 10.1007/s00442-020-04704-x
Boratyński, Z., Mousseau, T. A., and Møller, A. P. (2021). Individual quality and phenology mediate the effect of radioactive contamination on body temperature in Chernobyl barn swallows. Ecol. Evol. 11, 9039–9048. doi: 10.1002/ece3.7742
Cinelli, G., De Cort, M., and Tollefsen, T. (eds) (2019). European Atlas Of Natural Radiation. Luxembourg: Publication office of the European Union.
Congdon, J. D., Dunham, A. E., Hopkins, W. A., Rowe, C. L., and Hinton, T. G. (2001). Resource allocation-based life histories: a conceptual basis for studies of ecological toxicology. Environ. Toxicol. Chem. 20, 1698–1703. doi: 10.1002/etc.5620200811
Einor, D., Bonisoli-Alquati, A., Costantini, D., Mousseau, T. A., and Møller, A. P. (2016). Ionizing radiation, antioxidant response and oxidative damage: a meta-analysis. Sci. Total Environ. 54, 463–471. doi: 10.1016/j.scitotenv.2016.01.027
Firman, R. C., and Simmons, L. W. (2010). Sperm midpiece length predicts sperm swimming velocity in house mice. Biol. Lett. 6, 513–516. doi: 10.1098/rsbl.2009.1027
Fisher, H., Jacobs-Palmer, E., Lassance, J. M., and Hoekstra, H. E. (2016). The genetic basis and fitness consequences of sperm midpiece size in deer mice. Nat. Commun. 7:13652. doi: 10.1038/ncomms13652
Fukunaga, H., Butterworth, K. T., Yokoya, A., Ogawa, T., and Prise, K. M. (2017). Low-dose radiation-induced risk in spermatogenesis. Int. J. Radiat. Biol. 93, 1291–1298. doi: 10.1080/09553002.2017.1355579
Fuller, N., Smith, J. T., and Ford, A. (2019). Impacts of ionising radiation on sperm quality. DNA integrity and post-fertilisation development in marine and freshwater crustaceans. Ecotoxicol. Environ. Saf. 186:109764. doi: 10.1016/j.ecoenv.2019.109764
Galván, I., Bonisoli-Alquati, A., Jenkinson, S., Ghanem, G., Wakamatsu, K., Mousseau, T. A., et al. (2014). Chronic exposure to low-dose radiation at Chernobyl favours adaptation to oxidative stress in birds. Funct. Ecol. 28, 1387–1403. doi: 10.1111/1365-2435.12283
Hermosell, I. G., Laskemoen, T., Rowe, M., Møller, A. P., Mousseau, T. A., Albrecht, T., et al. (2013). Patterns of sperm damage in Chernobyl passerine birds suggest a trade-off between sperm length and integrity. Biol. Lett. 9:20130530. doi: 10.1098/rsbl.2013.0530
Hosken, D. J., Garner, T. W. J., Tregenza, T., Wedell, N., and Ward, P. I. (2003). Superior sperm competitors sire higher–quality young. Proc. R. Soc. B Biol. Sci. 270, 1933–1938. doi: 10.1098/rspb.2003.2443
Kim, G. J., Chandrasekaran, K., and Morgan, W. F. (2006). Mitochondrial dysfunction, persistently elevated levels of reactive oxygen species and radiation-induced genomic instability: a review. Mutagenesis 21, 361–368. doi: 10.1093/mutage/gel048
Kozakiewicz, M., Chołuj, A., and Kozakiewicz, A. (2007). Long-distance movements of individuals in a free-living bank vole population: an important element of male breeding strategy. Acta Theriologica 52, 339–348. doi: 10.1007/bf03194231
Lavrinienko, A., Tukalenko, E., Kesäniemi, J., Kivisaari, K., Masiuk, S., Boratyński, Z., et al. (2020). Applying the Anna Karenina principle for wild animal gut microbiota: temporal stability of the bank vole gut microbiota in a disturbed environment. J. Anim. Ecol. 89, 2617–2630. doi: 10.1111/1365-2656.13342
Ledevin, R., Michaux, J. R., Deffontaine, V., Henttonen, H., and Renaud, S. (2010). Evolutionary history of the bank vole Myodes glareolus: a morphometric perspective. Biological Journal of the Linnean Society 100, 681–694. doi: 10.1111/j.1095-8312.2010.01445.x
Lehmann, P., Boratyński, Z., Mappes, T., Mousseau, T. A., and Møller, A. P. (2016). Fitness costs of increased cataract frequency and cumulative radiation dose in natural mammalian populations from Chernobyl. Sci. Rep. 6:19974. doi: 10.1038/srep19974
Lemaître, J.-F., Ramm, S. A., Hurst, J. L., and Stockley, P. (2012). Sperm competition roles and ejaculate investment in a promiscuous mammal. J. Evol. Biol. 25, 1216–1225. doi: 10.1111/j.1420-9101.2012.02511.x
Lüpold, S., Birkhead, T. R., and Westneat, D. F. (2012). Seasonal variation in ejaculate traits of male red-winged blackbirds (Agelaius phoeniceus). Behav. Ecol. Sociobiol. 66, 1607–1617. doi: 10.1007/s00265-012-1415-3
Lüpold, S., Manier, M. K., Puniamoorthy, N., Schoff, C., Starmer, W. T., Buckley, S. H., et al. (2016). How sexual selection can drive the evolution of costly sperm ornamentation. Nature 533, 535–538. doi: 10.1038/nature18005
Makin, T. R., and Orban de Xivry, J. -J. (2019). Science Forum: ten common statistical mistakes to watch out for when writing or reviewing a manuscript. eLife 8:e48175.
Mappes, T., Boratyński, Z., Kivisaari, K., Lavrinienko, A., Milinevsky, G., Mousseau, T. A., et al. (2019). Ecological mechanisms can modify radiation effects in a key forest mammal of Chernobyl. Ecosphere 10:e02667.
Mendonca, T., Birkhead, T. R., Cadby, A. J., Forstmeier, W., and Hemmings, N. (2018). A trade-off between thickness and length in the zebra finch sperm mid-piece. Proc. R. Soc. B 285:1883.
Mokkonen, M., Koskela, E., Mappes, T., and Mills, S. C. (2012). Sexual antagonism for testosterone maintains multiple mating behaviour. J. Anim. Ecol. 81, 277–283. doi: 10.1111/j.1365-2656.2011.01903.x
Mousseau, T. A., and Møller, A. P. (2020). Plants in the light of ionizing radiation: what have we learned From Chernobyl. Fukushima, and other “Hot” places? Front. Plant Sci. 11:552. doi: 10.3389/fpls.2020.00552
Møller, A. P., Mousseau, T. A., Lynnn, C., Ostermiller, S., and Rudolfsen, G. (2008). Impaired swimming behaviour and morphology of sperm from barn swallows Hirundo rustica in Chernobyl. Mutat. Res. 650, 210–216. doi: 10.1016/j.mrgentox.2007.12.006
Møller, A. P., and Mousseau, T. A. (2013). Assessing effects of radiation on abundance of mammals and predator-prey interactions in Chernobyl using tracks in the snow. Ecol. Indic. 26, 112–116. doi: 10.1016/j.ecolind.2012.10.025
Møller, A. P., and Mousseau, T. A. (2015). Strong effects of ionizing radiation from Chernobyl on mutation rates. Sci. Rep. 5:8363. doi: 10.1038/srep08363
Montoto, L. G., Sánchez, M. V., Tourmente, M., Martín-Coello, J., Luque-Larena, J. J., Gomendio, M., et al. (2011). Sperm competition differentially affects swimming velocity and size of spermatozoa from closely related muroid rodents: head first. Reproduction 142, 819–830. doi: 10.1530/REP-11-0232
Okano, T., Ishiniwa, H., Onuma, M., Shindo, J., Yokohata, Y., and Tamaoki, M. (2016). Effects of environmental radiation on testes and spermatogenesis in wild large Japanese field mice (Apodemus speciosus) from Fukushima. Sci. Rep. 6:23601. doi: 10.1038/srep23601
Orr, T. J., and Brennan, P. L. R. (2015). Sperm storage: distinguishing selective processes and evaluating criteria. Trends Ecol. Evol. 30, 261–272. doi: 10.1016/j.tree.2015.03.006
Parker, G. A., and Ball, M. A. (2005). Sperm competition, mating rate and the evolution of testis and ejaculate sizes: a population model. Biol. Lett. 1, 235–238. doi: 10.1098/rsbl.2004.0273
Pinheiro, J., Bates, D., DebRoy, S., and Sarkar, D. R Core Team (2021). Nlme: Linear And Nonlinear Mixed Effects Models. R package version 3. 1-153. Available online at: https://CRAN.R-project.org/package=nlme (accessed June 15, 2021).
RCoreTeam (2016). R: A language and environment for statistical computing. Available online at: Retrieved from https://www.r-project.org/ (accessed June 25, 2021).
Schmoll, T., Kleven, O., and Rusche, M. (2018). Individual phenotypic plasticity explains seasonal variation in sperm morphology in a passerine bird. Evol. Ecol. Res. 19, 561–574.
Skaug, H., Fournier, D., Nielsen, A., Magnusson, A., and Bolker, B. (2014). glmmADMB: Generalized Linear Mixed Models Using AD Model Builder. R package version 0.8.0.
Smith, Z., Sindhu, C., and Meissner, A. (2016). Molecular features of cellular reprogramming and development. Nat. Rev. Mol. Cell Biol. 17, 139–154. doi: 10.1038/nrm.2016.6
Spitzenberger, F. (1999). The Atlas Of European Mammals: Clethrionomys Glareolus, eds A. J. Mitchell-Jones, G. Amori, and W. Bogdanowicz (London: Academic Press).
Stoffel, M. A., Nakagawa, S., and Schielzeth, H. (2017). rptR: repeatability estimation and variance decomposition by generalized linear mixed-effects models. Methods Ecol. Evol. 8, 1639–1644. doi: 10.1111/2041-210x.12797
Tapio, S., and Jacob, V. (2007). Radioadaptive response revisited. Radiat. Environ. Biophys. 46, 1–12. doi: 10.1007/s00411-006-0078-8
Tourmente, M., Gomendio, M., and Roldan, E. R. S. (2011). Sperm competition and the evolution of sperm design in mammals. BMC Evol. Biol. 11:12. doi: 10.1186/1471-2148-11-12
Tourmente, M., Villar-Moya, P., Varea-Sánchez, M., Luque-Larena, J. J., Rial, E., and Roldan, E. R. S. (2015). Performance of rodent spermatozoa over time is enhanced by increased ATP concentrations: the role of sperm competition. Biol. Reprod. 93, 1–13. doi: 10.1095/biolreprod.114.127621
Turner, R. M. (2006). Moving to the beat: a review of mammalian sperm motility regulation. Reprod. Fertil. Dev. 18, 25–38. doi: 10.1071/rd05120
Vladicì, T. V., Afzelius, B. A., and Bronnikov, G. E. (2002). Sperm quality as reflected through morphology in salmon alternative life histories. Biol. Reprod. 66, 98–105. doi: 10.1095/biolreprod66.1.98
Wang, Y. (2002). Epididymal sperm count. Curr. Protoc. Toxicol. 16, 1–5. doi: 10.14421/biomedich.2015.41.1-3
Keywords: background radiation, Myodes glareolus, reproduction, performance, sperm morphometry
Citation: Kivisaari K, Calhim S, Lehmann P, Boratyński Z, Mousseau TA, Møller AP and Mappes T (2022) Chronic Background Radiation Correlates With Sperm Swimming Endurance in Bank Voles From Chernobyl. Front. Ecol. Evol. 9:736389. doi: 10.3389/fevo.2021.736389
Received: 05 July 2021; Accepted: 13 December 2021;
Published: 06 January 2022.
Edited by:
Elise Huchard, UMR 5554 Institut des Sciences de l’Evolution de Montpellier (ISEM), FranceReviewed by:
Arrilton Araujo, Federal University of Rio Grande do Norte, BrazilEmily Cramer, University of Oslo, Norway
Copyright © 2022 Kivisaari, Calhim, Lehmann, Boratyński, Mousseau, Møller and Mappes. This is an open-access article distributed under the terms of the Creative Commons Attribution License (CC BY). The use, distribution or reproduction in other forums is permitted, provided the original author(s) and the copyright owner(s) are credited and that the original publication in this journal is cited, in accordance with accepted academic practice. No use, distribution or reproduction is permitted which does not comply with these terms.
*Correspondence: Kati Kivisaari, a21raXZpc2FAZ21haWwuY29t