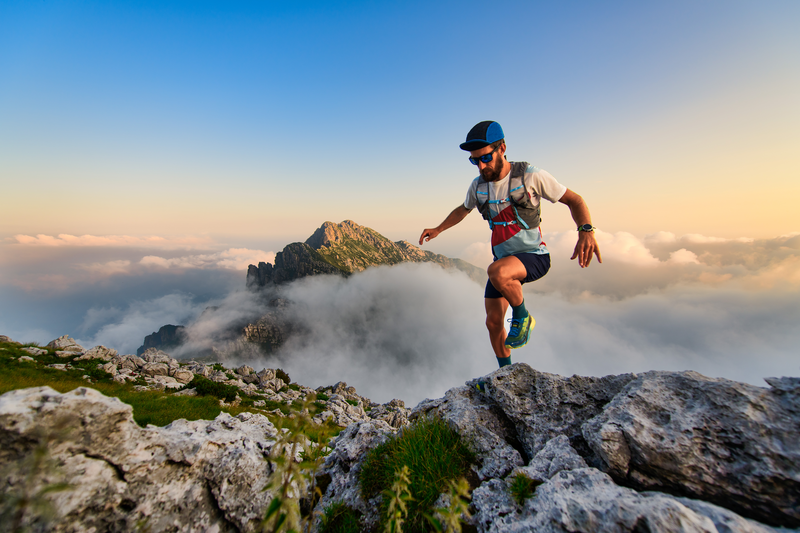
94% of researchers rate our articles as excellent or good
Learn more about the work of our research integrity team to safeguard the quality of each article we publish.
Find out more
ORIGINAL RESEARCH article
Front. Ecol. Evol. , 14 September 2021
Sec. Paleoecology
Volume 9 - 2021 | https://doi.org/10.3389/fevo.2021.734011
This article is part of the Research Topic Past Interactions Between Climate, Land Use, and Vegetation View all 22 articles
Global warming is having a profound influence on vegetation and biodiversity patterns, especially in alpine areas and high latitudes. The Qinling Mountain range is located in the transition zone between the temperate and subtropical ecosystems of central–east China and thus the vegetation of the area is diverse. Understanding the long-term interactions between plant diversity and climate change can potentially provide a reference for future landscape management and biodiversity conservation strategies in the Qinling Mountains region. Here, we use a pollen record from the Holocene sediments of Daye Lake, on Mount Taibai in the Qingling Mountains, to study regional vegetation changes based on biomes reconstruction and diversity analysis. Temperature and precipitation records from sites close to Daye Lake are used to provide environmental background to help determine the vegetation response to climate change. The results indicate that climate change was the main factor influencing vegetation and palynological diversity in the Qinling Mountains during the Holocene. The cold and dry climate at the beginning of the early Holocene (11,700–10,700 cal yr BP) resulted in a low abundance and uneven distribution of regional vegetation types, with the dominance of coniferous forest. During the early Holocene (10,700–7,000 cal yr BP), temperate deciduous broadleaf forest expanded, palynological diversity and evenness increased, indicating that the warm and humid climate promoted vegetation growth. In the middle Holocene (7,000–3,000 cal yr BP), the climate became slightly drier but a relatively warm environment supported the continued increase in palynological diversity. After ∼3,000 cal yr BP, palynological diversity and the evenness index commenced a decreasing trend, in agreement with the decreased temperature and precipitation in the Qinling Mountains. It’s noteworthy that human activity at this time had a potential influence on the vegetation. During the past few centuries, however, palynological diversity has increased along with the global temperature, and therefore it is possible that in the short-term ongoing climatic warming will promote vegetation development and palynological diversity in the area without human interference.
Climate change has a major impact on the global environment and biota, including humans (Shaver, 2000; Root et al., 2003; Peng et al., 2004; Parmesan, 2007). Global temperatures have risen substantially since the last glacial maximum (Xiao et al., 2020), especially during the past few decades, due to increasing atmospheric greenhouse gas concentrations. As an important component of global biodiversity, plant diversity plays an essential role in maintaining ecosystem stability and environmental protection (Ehrlich and Wilson, 1991; Thomas et al., 2004). However, plant diversity is currently undergoing rapid changes due to climatic warming and the increased frequency of extreme climatic events, as well as the reduction of living habitats (Walther et al., 2002; Wake and Vredenburg, 2008; Barnosky et al., 2011). However, several studies have shown that increased temperatures are conducive to plant growth at high latitudes (Peuelas and Filella, 2001), while at the same time, the vegetation at high latitudes and altitudes is more sensitive to climate change (Pepin et al., 2015; Ellis et al., 2021). Human activities also have important direct impacts on terrestrial ecosystems (Vitousek, 1994; Crutzen and Stoermer, 2000; Crutzen, 2002; Mottl et al., 2021). Humans altered the land surface, ecosystem dynamics and biodiversity by clearing the native vegetation for agriculture and living space, which has led to increased species extinctions, with rates accelerating during the past few centuries and with potentially irreversible effects (Braatz et al., 1992; Pimm et al., 2014; Lewis and Maslin, 2015). Therefore, the long-term paleovegetation and paleoclimatic records is of great significance to explore regional patterns of vegetation and climate change to distinguish the effects of climate change and human activity on vegetation. Such records may also provide a potential analogue of the interaction of climate change and vegetation in the future.
Pollen analysis has been widely used to study the history of vegetation and plant diversity on different timescales (Jackson and Blois, 2015; Birks et al., 2016a, b; Felde et al., 2020), and fossil pollen records can provide important information about the response of vegetation to past changes in climate and land use (Willis et al., 2004, 2010; Rudaya et al., 2020). The Qinling Mountain range is the natural geographic and climatic boundary between North and South China. The area is influenced mainly by the East Asian summer monsoon and the vegetation is highly sensitive to climate change (Liu et al., 2008; Zhang et al., 2021). Mount Taibai, which is the highest peak in the Qinling Mountain range, has numerous alpine lakes, and the pollen assemblages in the sediments of these lakes are a valuable archive of information on climate change and vegetation succession. Previous studies of the sediments have included analyses of biological indicators such as pollen and diatoms to reconstruct changes in vegetation cover and limnological conditions in the Mount Taibai area (Zhao et al., 1999; Zhang et al., 2001; Cui et al., 2003; Li et al., 2008, 2017; Cheng et al., 2017, 2020; Huang et al., 2020). Additionally, organic geochemical indicators have been used to characterize climate change on different timescales (Liu et al., 2005; Wang et al., 2016, 2019). However, these studies were not conducted on open-water lake sediments and were focused on the vegetation of the middle–late Holocene and the modern vegetation; moreover, they were low temporal resolution. Detailed investigations of the response of plant diversity to climate change based on high-resolution continuous sediments is important for biodiversity conservation in the area.
Lake sediments have the advantages of high stratigraphic continuity and resolution, which make them well suited for studies of regional climate and vegetation changes (Chen et al., 2000; Wagner et al., 2000; Rioual et al., 2001). Daye Lake is an enclosed alpine lake above the forest line on Mount Taibai, and here we report the results of a high-resolution biomes and palynological diversity reconstruction from the Holocene sediments of the site. The principal aims of the study were as follows: (1) To investigate the Holocene evolution of vegetation and climate based on the pollen records from Daye Lake in the Qinling Mountains region; (2) to reveal changes in the palynological diversity and infer the plant diversity changes; and (3) to explore the relationship between vegetation and climate change, to provide a basis for predicting possible future changes in the vegetation and plant diversity of the region.
Mount Taibai, with a maximum altitude of 3,767 m a.s.l., is the highest part of the Qinling Mountain range and the highest mountain in central and eastern China. The lithology is granite formed by the large-scale incursion of acidic magma during the Yanshan Movement in the Cretaceous (Tian and Huang, 1990). There are numerous Quaternary relict glacial landforms in the Mount Taibai area, with periglacial landforms generally developed in areas below the snow line of ∼2,600 m a.s.l. (Pang, 1998). However, the snow line has risen above Baxiantai, which is the highest peak of Mount Taibai (3,767 m a.s.l.).
There are pronounced differences in precipitation, temperature, topography, and vegetation distribution on the southern and northern sides of the Mount Taibai. Moreover, diverse temperate and subtropical plant species, as well as numerous medicinal herbs, survive in the region (Pang, 1998). The combination of thermal and hydrological conditions results in a vertical distribution of vegetation belts in the area. The area below 780 m a.s.l. is extensively cultivated and the natural vegetation has been substantially altered by human activities. Above this cultivated zone, the following vegetation zones on the northern slope of Mount Taibai can be defined, with increasing altitude: Quercus spp. (Quercus wutaishanica and Quercus aliena var. acuteserrata) forest (780–2,200 m a.s.l.), Betula spp. forest dominated by Betula albosinensis (2,200–2,730 m a.s.l.), coniferous forest divided into Abies fargesii-forest and Larix chinensis-forest (2,730–3,400 m a.s.l.), and alpine shrubs and meadow (>3,480 m a.s.l.).
Daye Lake (33°57’25.90″ N, 107°45’38.39″ E; 3,615 m a.s.l.; Figure 1) is a small, closed glacier lake with a surface area of 0.5 ha and maximum water depth of 18 m, formed after the retreat of the Quaternary glacier activity, located above the tree line on the northern slope of Mount Taibai. It is mainly supplied by precipitation which is concentrated in summer. The regional climate is influenced by the East Asian summer monsoon, and the annual mean air temperature and precipitation are 8.1°C and 720 mm, respectively. The coldest month (January) and warmest month (July) have respective average temperatures of −4 and 19.3°C, based on a 30–year meteorological dataset (CE 1981–2010) from Taibai county meteorological station (1,545 m a.s.l) (see Figure 2 in Zhang et al., 2021). Based on the lapse rates for the northern slopes of the Qinling Mountains proposed by Ma et al. (2017), the mean annual, January and July temperatures are 2.8, −7.3, and 13.6°C, respectively. Daye Lake is surrounded by alpine shrubland and meadow. The shrub taxa are dominated by Salix cupularis, Rhododendron capitatum, and Spiraea, and the local herb taxa are mainly Ranunculaceae, Brassicaceae, and Poaceae (Zhang et al., 2021).
Figure 1. (A) Location of the study site (DY: Daye Lake) and other sites (S1–S4) in the surrounding region that are mentioned in the text (S1: Daihai Lake; S2: Gonghai Lake; S3: Qinghai Lake; S4: Dajiuhu wetland). The vegetation zones in China (https://geodata.pku.edu.cn) are also shown; (B) digital elevation map of the study area; (C) the picture of the Daye Lake.
Figure 2. AMS 14C dates for core DYH16A from Daye Lake based on the R package rbacon, version 2.5.0 (Blaauw and Christen, 2011) and the resulting age-depth model. The red line represents mean calendar years. The gray shading and the blue line indicate calendar ages with the 95% confidence range and the age errors, respectively.
A 2.35 m–long sediment core (DYH16A) was retrieved from Daye Lake in April 2016 using a UWITEC piston corer. The undisturbed core was transported to the laboratory and subsampled at 1 cm–intervals. Seventeen samples of bulk organic sediments were analyzed by accelerator mass spectrometry (AMS) at Arizona University, United States, and Peking University, China (Table 1). The ages of bulk sediment samples may be influenced by old carbon due to the complexity of carbon sources. Therefore, we used the intercept method after fitting a 2nd-order polynomial curve to get the carbon reservoir age which is comparable to the age difference between pollen concentrates and bulk organic from the surface sample (see the Supplementary Material). Finally, 1540 years was selected as the reservoir age of Daye Lake. A chronological model (Figure 2) was developed using the R package rbacon, version 2.5.0 (Blaauw and Christen, 2011) based on calendar ages before present (BP = 1950 CE) and calibrated with the IntCal20 dataset (Northern Hemisphere) (Reimer et al., 2020). Note that only the top 1.26 m of the core were discussed in this study.
Table 1. AMS 14C dates and their calibrated ages from the upper 1.26 m of core DYH16A from Daye Lake.
The top 1.26 m of core DYH16A at 1 cm–intervals were analyzed in this study. A known number of Lycopodium spores were added to 0.5 g of dried sediment to calculate pollen concentrations (grains g–1). Samples were then treated sequentially with HCl, KOH, and HF, following the procedures described in Fægri and Iversen (1989) and Moore et al. (1991). Pollen identification was carried out under a light microscope at 400× magnification with reference keys (e.g., Wang et al., 1995; Tang et al., 2016). At least 500 terrestrial pollen grains were counted each sample. Pollen percentages were calculated based on the sum of terrestrial taxa, excluding aquatic taxa and spores. Pollen diagrams were draw and constrained incremental sum of squares (CONISS) analysis was conducted using Tilia software of version 2.0 (Grimm, 1987).
A lower-resolution pollen record from the top 1.03 m of the core DYH16A has been published by Zhang et al. (2021) to reconstruct the regional plant abundances based on the REVEALS model. This record spans the past ∼7000 years which the sediment accumulation rate was relatively uniform. The higher-resolution pollen record presented here spans the entire Holocene and extends to the base of the core where the sediment accumulation rate was lower. But the entire core is continuously deposited which can reflect the Holocene changes of vegetation and climate in the region.
The pollen record from Daye Lake was used for biomes reconstruction in order to explore the vegetation response to Holocene climate change. The procedures used for biomization analysis follow those described in Prentice et al. (1996). First, pollen types (taxa) were assigned to one or more plant functional types (PFTs) (Table 2), and the PFTs were then classified into biomes (Table 3). Finally, the affiliated scores for each biome were calculated based on the pollen percentages and the PFT sets and were then used to represent the abundance of a particular biome. Assignment of the identified pollen taxa from Daye Lake to PFTs and biomes follows Yu et al. (2000) and Ni et al. (2014) for China, together with reference to the modern regional vegetation distribution. Biome definition depends on the influence of the dominant PFTs with the highest scores. Biome affinity scores provide an objective evaluation of the vegetation composition and have been applied to several surface and fossil pollen datasets (Tarasov et al., 1998; Yu et al., 2000; Rudaya et al., 2008; Ni et al., 2014). Biome modeling was conducted using PPPBase software (Guiot and Goeury, 1996), based on three matrixes (PFT × taxon, biome × PFT and biome × taxon matrix) which comprised 51 pollen types, 13 PFTs and 5 biomes in this study (Tables 2, 3).
Table 2. Assignment of the pollen taxa from Daye Lake to plant functional types (PFTs) used in the biomization model.
The reconstructions of palynological diversity can be used to directly interpret the composition and structure of the vegetation.
In order to reconstruct the palynological diversity history over the Holocene in Qingling Mountains region from different aspects, Hill’s indices (Hill, 1973) and rarefaction analysis (Hurlbert, 1971) are used to estimate the diversity richness including palynological richness, Shannon diversity, and Simpson diversity. Palynological richness is the simple count of the number of taxa which represents the total number of taxa in a community to describe the vegetation community; the number of common taxa is known as the Shannon diversity index; and the number of dominant taxa is a measure of the Simpson diversity (Rudaya et al., 2020; Kulkarni et al., 2021). We also calculated evenness index to reflect the community structure and to assess palynological diversity at the regional scale and variations in vegetation composition (Pielou, 1966). The value of evenness is between 0 (only one species in the pollen assemblage) and 1 (each species with the same abundance in the pollen assemblage), the low value indicates the greater difference in the abundance of the plant types in pollen assemblages (Li and Zhao, 2018). All of these indices were calculated using the “vegan” package, version 2.5–7, in R (Oksanen et al., 2020).
The pollen assemblages from Daye Lake (Figure 3) were conducted with CONISS analysis and divided into five significant zones, i.e., three pollen zones and two subzones, suggested by broken stick model. The zones are described below.
Figure 3. Pollen percentage diagram for core DYH16A from Daye Lake. Only the major taxa are shown (average > 1% and present within at least 70% of the total number of samples). The abundance of arboreal and non-arboreal pollen, the pollen concentration of terrestrial taxa, and the CONISS cluster analysis results are also shown. The dotted shading represents an exaggeration of the scale (×5). Quercus_D: Quercus_deciduous; Quercus_E: Quercus_evergreen.
Zone I (11,700–10,700 cal yr BP). This zone is characterized by the highest percentages of coniferous trees (average of 42.18%). Among them, Pinus, Abies, and Picea have high percentages, with averages of 27.60, 9.18, and 3.23%, respectively, while Tsuga has the lowest (average of 2.17%). Deciduous broadleaf trees are relatively poorly represented (30. 65%). Among the shrub taxa, Ephedra is well represented (average of 1.26%), while Rosaceae, Fabaceae, and Euphorbiaceae are poorly represented. Herbs are dominated by Artemisia which has an average of 21.45%. The pollen concentration is the lowest during the entire Holocene.
Zone II (10,700–3,800 cal yr BP). This zone is characterized by the lowest proportion of coniferous trees (average of 22.66%) among the three zones and the significant increase of deciduous broadleaf trees (from 30.65 to 48.62%). The obvious increase of Corylus/Carpinus (with average values of 18.51%) and evergreen oak (Quercus_evergreen) are at the expense of the decrease of Pinus (from 27.60 to 9.88%). However, the herb pollen proportion decrease, which is largely due to the decreases in Artemisia (from 9.31 to 5.30%) and Amaranthaceae (from 2.18 to 1.32%), whereas shrub taxa such as Rosaceae (from 2.16 to 5.66%) and Fabaceae (from 0.20 to 1.33%) increase. The pollen concentration is relatively high in this zone. Owing to the different trends of Pinus, Abies, Corylus/Carpinus, Fagus, and Artemisia within this zone, two subzones, i.e., Zone IIa (10,700–6,000 cal yr BP) and Zone IIb (6,000–3,800 cal yr BP) is further divided. Zone IIa shows the lowest abundance of coniferous trees (20.49%) and the highest value of deciduous broadleaf trees (51.48%) in the Holocene. In Zone IIb, Artemisia reach the lowest percentages in the entire record (averages of 5.04%).
Zone III (3,800–0 cal yr BP). There is a minor increase in the percentages of coniferous trees and herbs (average of 26.87 and 23.51%, respectively). The abundance of deciduous broadleaf trees and shrubs shows a decreasing trend (average of 40.80 and 6.10%, respectively). This zone can also be further divided into Zone IIIa (3,800–1,200 cal yr BP) and Zone IIIb (1,200–0 cal yr BP). Zone IIIa is characterized by continuous decrease in deciduous broadleaf trees, increase in coniferous trees and herbaceous plant (which is mainly due to increases in Artemisia and Amaranthaceae). However, Zone IIIb is characterized by the increase of coniferous trees, decrease of deciduous broadleaf trees, and a substantial increase in herbs, especially in Artemisia (from 7.99 to 13.62%, the maximum value over the Holocene) and Poaceae (from 2.04 to 3.09%). The pollen concentration in Zone IIIa is high whereas the one in Zone IIIb is low.
The biome reconstruction from Daye Lake (Figure 4) shows that the coniferous forests (CO) vegetation type displays the highest affinity scores during the first stage of the early Holocene (11,700–10,700 cal yr BP). This vegetation reconstruction suggests drought- and cold-tolerant needles communities played a major role in the regional vegetation cover. However, the temperate deciduous broadleaf forests (TEDE) become dominated during the Holocene interval after which suggests a warm and moist environment during the Holocene. There is a pronounced inverse relationship between TEDE and CO throughout the Holocene.
Figure 4. Biome scores for the Holocene pollen record from Daye Lake. The gray curve represents biome changes based on the original scores, and the colored lines are a 5-point smoothing data. Blue curve: coniferous forest (CO); purple curve: temperate deciduous broadleaf forest (TEDE); orange curve: warm mixed forest (WAMF); yellowish-green curve: steppe (STEP). And dominant biomes are also shown.
The Holocene period experiences drastic changes in the biome composition, characterized by a marked increase in TEDE, herbaceous vegetation (STEP) and warm mixed forest (WAMF) scores, which is accompanied by a decrease in the CO scores. The drastic decrease of CO (which is corresponding to the apparent reduce of Pinus, see Figure 3), and the visible increase of TEDE suggests a warm and moist environment during the period of∼10,700 and 6,000 cal yr BP. The period of 6,000–3,800 cal yr BP is characterized by the minimum scores of CO, decreased scores of TEDE, and the lowest scores of STEP, but a visible increased scores of WAMF. CO increase slightly compared with the previous stage from 3,800 to 1,200 cal yr BP. The increasing trends are observed in herbs and WAMF. During the past ∼1200 years, WAMF decrease and STEP increase from low to high values. There is an obvious low value of TEDE between 1000 and 600 years and then the scores increase significantly, whereas conifers show the opposite trend.
Diversity analysis based on pollen records from lake sediments can accurately reflect the richness and composition of the regional vegetation (Stirling and Wilsey, 2001; Birks et al., 2016a). The Shannon and Simpson diversity indexes show similar temporal trends and are positively correlated with evenness (Figure 5). The palynological richness shows an increasing trend, with pronounced fluctuations, throughout the Holocene. Therefore, Shannon diversity and evenness index are employed in the following discussion. During 11,700–10,700 cal yr BP, the palynological diversity increases substantially, corresponding to a trend of increasing evenness. Palynological richness, diversity and evenness index are in increasing trends until 6,000 cal yr BP; however, there is an interval of pronounced low palynological diversity and evenness index during 9,000–8,200 and 7,800–7,000 cal yr BP based on the raw data (gray line in Figure 5). Palynological diversity increases substantially from 6,000 to 3,800 cal yr BP, and then decreases slightly between 3,800 and 1,200 cal yr BP. The decreasing trend is observed from ∼1,200 cal yr BP, which is followed by another increase during the past ∼600 years.
Figure 5. Changes in palynological richness, Shannon and Simpson diversity, and evenness index at Daye Lake during the Holocene. The gray curves are the original data and the colored lines are a 5-point smoothing data. The red line indicates palynological richness based on the total number of taxa, the yellowish-green line is Shannon’s diversity based on the number of common taxa, and the blue and green lines are Simpson’s diversity based on the number of dominated taxa and evenness, respectively.
Climate change is a major factor influencing the distribution and abundance of vegetation (Bartlein et al., 1986; Ma et al., 2013); however, the response of vegetation and plant diversity to climate change shows strong regional variations. For example, temperature is the major influence on diversity in high-altitude mountains and high-latitude regions (Hou, 1983; Zhang et al., 2020), while precipitation is more important in arid and semi-arid regions such as the Loess Plateau of China (Shang and Li, 2010; Sun et al., 2013). Elsewhere, such as in southwest China, temperature and precipitation both have major influences on the vegetation (Wang et al., 2018; Trivedi et al., 2020). In the case of east-central China, the Qingling Mountains region, the relationship between climate change and vegetation over the Holocene need to be investigated.
The Qinling Mountain range is a typical region influenced by the East Asian summer monsoon, where plant growth is influenced predominantly by temperature and precipitation (Gansert, 2004; Zhang et al., 2006). The response of the vegetation at Daye Lake to climate change is manifested by changes in community composition and in the relative abundance of the dominant taxa (Figures 3, 4). Coniferous trees such as Abies and Picea can tolerate low temperatures and drought, and generally grow at high altitudes (Achuff and Roi, 1977; Liu Z. L. et al., 2002). By contrast, deciduous broadleaf forest (e.g., Quercus_deciduous, Carpinus_Corylus, and Ulmaceae) is favored by a warm and moist environment (Wang et al., 2018; Xu et al., 2019; Trivedi et al., 2020). Evergreen trees such as Quercus_evergreen and Cyclobalanopsis are widely distributed in subtropical and tropical regions, especially in locations with high temperatures and precipitation (Srivastava et al., 2021). Herbaceous plants such as Artemisia and Amaranthaceae are drought–tolerant (Kolb and Sperry, 1999; Uotila et al., 2021).
In order to determine the vegetation response at Daye Lake to Holocene climate change, we compared the pollen assemblages, biome scores, Shannon diversity and evenness index with paleoclimatic records from other sites in the region. The cited records in the study comprise the following: monsoon precipitation and temperature reconstructions based on pollen records from Gonghai and Daihai Lakes, both to the north of the Qinling Mountains (Xu et al., 2010; Chen F. H. et al., 2015); temperature and precipitation reconstructions based on records of branched fatty alcohol ratio BNA15 and pollen assemblages from the Dajiuhu wetland to the south of the Qinling Mountains, respectively (Huang et al., 2013; Sun et al., 2019); alkenone-based temperature and pollen-based precipitation records from Qinghai Lake, to the northwest of the Qinling Mountains (Herzschuh et al., 2010; Hou et al., 2016; Figure 6).
Figure 6. Comparison of vegetation changes in the Qinling Mountains region with paleoclimatic records from other sites in China. (A) Pollen-based temperature reconstruction from Daihai Lake (Xu et al., 2010). (B) Pollen–based precipitation reconstruction from Gonghai Lake (Chen F. H. et al., 2015). (C) Temperate deciduous broadleaf forest scores based on the biome reconstruction from Daye Lake. (D) Shannon index of palynological diversity from Daye Lake. (E) Evenness index from Daye Lake. (F) Temperature reconstruction based on the branched fatty alcohol ratio BNA15 from the Dajiuhu wetland (Huang et al., 2013). (G) Pollen-based precipitation reconstruction from the Dajiuhu wetland (Sun et al., 2019). (H) UK37-inferred temperature record from Qinghai Lake (Hou et al., 2016). (I) Pollen-based precipitation reconstruction from Qinghai Lake (Herzschuh et al., 2010).
In the first stage of the early Holocene (11,700–10,700 cal yr BP), coniferous forests with Pinus, Abies and Picea were abundant in the study area, as well as steppe habitats dominated by Artemisia. Deciduous broadleaf taxa were poorly represented in the vegetation (Figures 3, 4). The dominance of coniferous forest indicates that the climate during this period was cold and dry, which is confirmed by the paleoclimatic record from sites elsewhere in the region (Figure 6).
During the early Holocene (10,700–7,000 cal yr BP), there were significant decreases in Pinus and Artemisia, and evergreen trees such as Quercus_evergreen began to appear (Figure 3), and there was a substantial increase in deciduous broadleaf and warm mixed forests. The pollen percentages and biome results indicate that the region was occupied by deciduous broadleaf forests, suggesting that the climate in the early Holocene was warm and humid. At the same time, temperature and precipitation commenced an increasing trend and reached their highest levels in the region of the southern and northern China, and the northeastern Qinghai-Tibet Plateau (Figure 6). However, the climate change at this stage is not stable, and there are fluctuations on a centennial time scale. For example, the abundance of deciduous broadleaf taxa decreased at 9,000–8,200 cal yr BP and 7,800–7,000 cal yr BP in Daye Lake, revealing deteriorating climate, while it corresponded to the declining of temperature and precipitation in Qinghai Lake and Dajiuhu wetland, respectively. And the Gonghai Lake in the northern China also has a low precipitation.
In the middle Holocene (7,000–3,000 cal yr BP), coniferous forest expanded slightly, as indicated by increases in Pinus and Picea pollen percentages, while percentages of thermophilous deciduous broadleaf taxa gradually decreased. The representation of Artemisia reached the lowest level in the profile. The hydrothermal combination at this time were probably worser than during the previous stage; nevertheless, the climate was still conducive to the growth of warm-temperate mixed forest (Figure 4). The substantial climatic differences existed between the northern, western, and southern parts of China at this time. At Qinghai Lake in the northeastern Tibetan Plateau, to the northwest of the Qinling Mountains, there was strong decrease in temperature which reached its lowest level during the Holocene, probably because of intensification of the Westerlies (Hou et al., 2016); while precipitation decreased substantially. However, temperatures at sites to the north of the Qinling Mountains were close to those sites to the south. At Daihai Lake, temperatures reached their highest level during the Holocene, while in the Dajiuhu wetland the temperature remained at a high level. This dipolar pattern of precipitation is a feature of the climate of China on various timescales in north and south of China and is discussed in detail in Chen J. H. et al. (2015). Overall, our results of pollen percentages and biome reconstruction may indicate that the changes of precipitation pattern at Daye Lake during the Holocene were between northern and southern China.
During the late Holocene, especially after 3,000 cal yr BP, Pinus and Artemisia commenced a pronounced increasing trend and there was the increased representation of herbaceous vegetation (Figure 3). The biome reconstruction shows an increase in coniferous forests and herb-dominated vegetation, accompanied by decreases in deciduous broadleaf and warm mixed taxa (Figure 4), indicating a climatic deterioration. It is noteworthy that decreases in temperature and precipitation occurred at other sites adjacent to Qingling Mountains region (Figure 6).
Pollen is an important indicator for the reconstruction of past plant diversity. There have been many studies on plant diversity based on the palynological diversity (Xiao et al., 2008; Liang et al., 2019; Rudaya et al., 2020; Mottl et al., 2021). Even though there are several uncertainties involved in reconstructing plant diversity from fossil pollen records. For example, palynological diversity cannot represent the full range of plant diversity due to the limitations of pollen identification, sample size, and the complexity of depositional processes (Odgaard, 2001; Weng et al., 2006; Meltsov et al., 2011; Birks et al., 2016a; Liang et al., 2019). Several taxa can only be identified to family level, and poorly represented entomophilous taxa can lead to the underestimation of plant diversity. However, the vegetation of the Qinling Mountains is dominated by woody plants which are identified mainly at the genus level (4 families + 16 genera of trees and shrubs in Figure 3). And at least 500 pollen grains were counted for each sample in this study to ensure a statistically adequate and representative sample of observations. Moreover, Zhang et al. (2021) suggested that the pollen loading in Daye Lake is predominantly wind–transported, indicating that the palynological diversity reconstructed in this study is representative of the vegetation of the Qinling Mountains region. Nevertheless, several studies have shown that palynological diversity is positively correlated with plant diversity (Odgaard, 1999; Meltsov et al., 2011; Birks et al., 2016a; Felde et al., 2016). Overall, despite these potential limitations of palynological diversity, we assume that the pollen records from Daye Lake can provide a reliable record of changes in plant diversity in the Qingling Mountains region during the Holocene.
The record of palynological diversity at Daye Lake is in good agreement with records of regional climate change (Figure 6), indicating that palynological diversity was highly dependent on the evolution of the regional climate. The low values of palynological diversity and evenness index at the beginning of the early Holocene are consistent with the relatively low temperature and precipitation indicated by the paleoclimate reconstructions. The increased palynological diversity and evenness index during the early Holocene is also consistent with the inferred warm and humid climate, and numerous studies have demonstrated that intervals of warm and humid climate are characterized by high vegetation cover and high palynological diversity in northwest, southwest and Loess Plateau regions of China (Fang et al., 2003; Zhang et al., 2005; Xiao et al., 2008; Zhao and Ding, 2014). Notably, palynological diversity and evenness index at Daye Lake continued to increase during the middle Holocene (7,000–3,000 cal yr BP), even though the precipitation decreased; temperatures remained at a high level (Figures 6A, F), the environmental conditions in the Qingling Mountains continued to favor a high degree of palynological diversity, indicating that the vegetation in the Qinling Mountains was more sensitive to temperature than to precipitation. The palynological diversity also commenced a decreasing trend after ∼3,000 cal yr BP, and it’s clear that the environmental conditions in the area became less favorable for certain plant species, leading to their decreased representation or disappearance. Cheng et al. (2017) also suggested that the alpine timberline on Mount Taibai moved upward to a higher altitude during ∼4,200–2,000 cal yr BP and started to move downward from ∼2,000 cal yr BP. The visible decrease in the palynological diversity of the Daye Lake between 1,200 and 600 cal yr BP may also be resulted from the cold and dry environment which was consist with the sudden decrease in temperature and precipitation in the northern and southern of the Qinling Mountains within the recognizable range of the age error. At the same time, archeological evidence indicated that this period corresponded to the cultural and economic prosperity of the Song Dynasty (Archaeological research institute of Sui-Tang Dynasties of Shanxi Academy of Archaeology, 2018) which revealed the possible influence of human activities on the vegetation. Interestingly, during the past few centuries, palynological diversity increased, accompanied by an increase in temperature and a decrease in precipitation (Figure 6). This seems to support evidence that temperature had been a major factor affedting vegetation evolution in Qinling Mountains region, and that the rising temperatures resulting from global warming had a positive effect on palynological diversity in the region. However, the possible roles played by human activities should also be considered. For example, during the past several hundred years, the increases in Artemisia, Amaranthaceae, and Poaceae pollen percentages in the sediments can be attributed to the development of agriculture in low-altitude areas and open land may be one of the reasons for the increase in palynological diversity (Woodbridge et al., 2021).
Overall, the results of this study indicate that plant diversity in the Qinling Mountains during the Holocene was driven primarily by climate change. The relatively warm and humid environment to the south of the Qinling Mountains has resulted in a higher plant diversity in southern China than in northern China (Sun et al., 2009; Shang and Xing, 2016). Sun et al. (2013) concluded during the warm period of the Holocene, subtropical vegetation in the south expanded and migrated northward into the Qinling Mountains region. The impact of climate on vegetation is also exerted via extreme climatic events such as droughts, floods, and abrupt cooling, which lead to species extinctions and the reduction of biodiversity (Srivastava et al., 2021). Park et al. (2019) showed that in coastal East Asia successive droughts during the ∼8.2 ka caused forest damage and a major reduction of plant diversity. Additionally, Tao et al. (2013) demonstrated that in northwest China the cold and dry climatic event at 4.2 ka resulted in the conversion of grassland to desert, with a greatly reduced plant diversity and vegetation structure. However, the pollen record from Daye Lake seems not to contain clear evidence for the influence of abrupt climatic events on palynological diversity (Figure 6). Notably, climate change will affect the vegetation phenology which will subsequently impact the plant diversity. For example, changes in seasonal temperatures will alter the flowering time of plants, with increases in annual average temperature overall resulting in an earlier flowering time (Hart et al., 2014; Yu et al., 2016).
There is a long history of human impacts on the composition and structure of vegetation, associated with the development of agriculture and the use of fire (Ruddiman, 2003; Rudaya et al., 2020; Kulkarni et al., 2021). In southern China significant agricultural activity began ∼6000 years ago (Cheng et al., 2018), while the large-scale alteration of the vegetation composition and ecosystems has occurred during the past few millennia (Lewis and Maslin, 2015; McMichael, 2021). In the Qinling Mountains, agricultural activity began as early as ∼8000 cal yr BP, associated with cultures such as the Peiligang and Yangshao (Zhang et al., 2018). However, even though a long history of human activities was documented in this area, there is no ambiguous evidence for human disturbance of the vegetation at Daye Lake, possibly due to its high altitude (3,615 m a.s.l.), location above the forest line (Zhang et al., 2021). Liu H. et al. (2002) suggested that the manifest influence of human activities on vegetation is concentrated on the low latitudes in Mount Taibai.
In summary, the temperature of the Qinling Mountains has increased in recent decades due to global warming, which will impact the regional vegetation composition and palynological diversity (Li et al., 2018; Huang et al., 2020). Additionally, the palynological diversity of the Qinling Mountains region may increase with the ongoing climate warming in the future when the direct human interference is absent.
Our results show that the climate was the main factor affecting regional vegetation changes and palynological diversity. At the beginning of the early Holocene (11,700–10,700 cal yr BP), the cold and dry climate of the region resulted in predominantly coniferous forest vegetation, with a low palynological diversity and evenness index. During the early Holocene (10,700–7,000 cal yr BP), the warm and humid climate with fluctuations on a centennial time scale promoted the growth and expansion of deciduous broadleaf forest, and there was a corresponding increase in palynological diversity. During the middle Holocene (7,000–3,000 cal yr BP), although the climate became drier, the relatively warm environmental conditions continued to promote vegetation growth and high palynological diversity. During the past ∼3000 years, the temperature and precipitation in the Qinling Mountains decreased substantially, which led to reduction in palynological diversity, and human activities may play a potential role. However, during the past few centuries palynological diversity has increased along with rising temperatures, which may indicate that ongoing warming in the region may have a beneficial effect on vegetation growth and palynological diversity and the natural plant diversity may be in an increasing trend without human interference in the future in the region.
The original contributions presented in the study are included in the article/Supplementary Material, further inquiries can be directed to the corresponding author/s.
YZ: conceptualization, methodology, analysis, and writing – original manuscript. QC: conceptualization, methodology, reviewing, and editing. YH: reviewing and editing. AZ: supervision, project administration, providing revisions and comments, and funding acquisition. DW: providing revisions and comments. All authors contributed to the article and approved the submitted version.
This research has received funding from the National Natural Science Foundation of China (Project Nos. 41771208 and 41790421), the Strategic Priority Research Program of the Chinese Academy of Sciences (#XDA20070101), and the National Key Research and Development Program of China (Grant No. 2016YFA0600501).
The authors declare that the research was conducted in the absence of any commercial or financial relationships that could be construed as a potential conflict of interest.
All claims expressed in this article are solely those of the authors and do not necessarily represent those of their affiliated organizations, or those of the publisher, the editors and the reviewers. Any product that may be evaluated in this article, or claim that may be made by its manufacturer, is not guaranteed or endorsed by the publisher.
We thank Xiaonan Zhang, Liying Yin, Tianlong Yan, and Zhihong Cao for assistance in the field, Lingyu Tang for supervising with pollen identification, and Feng Qin and Chen Liang for advice on statistical analyses. We gratefully acknowledge the two reviewers for helpful comments that greatly improved the manuscript. This research contributes to the DiverseK(a working group of PAGES) initiative.
The Supplementary Material for this article can be found online at: https://www.frontiersin.org/articles/10.3389/fevo.2021.734011/full#supplementary-material
Achuff, P., and Roi, G. (1977). Picea–Abies forests in the highlands of Northern Alberta. Vegetatio 33, 127–146. doi: 10.1007/BF00205908
Archaeological research institute of Sui-Tang Dynasties of Shanxi Academy of Archaeology (2018). A Summary of the Archaeology of the Three Kingdoms, Sui, Tang, Song, Yuan, Ming and Qing Dynasties of Shanxi province from 2008 to 2017 years. Archaeol. Cult. Relics 5, 111–147.
Barnosky, A. D., Matzke, N., Tomiya, T., Wogan, G. O. U., Swartz, B., Quental, T. B., et al. (2011). Has the Earth’s sixth mass extinction already arrived? Nature 471, 51–57. doi: 10.1038/nature09678
Bartlein, P. J., Prentice, I. C., and Webb, T. (1986). Climatic response surfaces from pollen data for some Eastern North American Taxa. J. Biogeogr. 13, 35–57. doi: 10.2307/2844848
Birks, H. J. B., Felde, V. A., Bjune, A. E., Grytnes, J. A., Seppä, H., and Giesecke, T. (2016a). Does pollen–assemblage richness reflect floristic richness? A review of recent developments and future challenges. Rev. Palaeobot. Palynol. 228, 1–25. doi: 10.1016/j.revpalbo.2015.12.011
Birks, H. J. B., Felde, V. A., and Seddon, A. W. R. (2016b). Biodiversity trends within the Holocene. Holocene 26, 994–1001. doi: 10.1177/0959683615622568
Blaauw, M., and Christen, J. A. (2011). Flexible paleoclimate age–depth models using an autoregressive gamma process. Bayesian Anal. 6, 457–474. doi: 10.1214/11-BA618
Braatz, S., Davis, G., Shen, S., and Rees, C. (1992). Conserving biological diversity: a strategy for protected areas in the Asia–Pacific Region. Environ. Plann. A. 193, 1–66.
Chen, F. H., Xu, Q. H., Chen, J. H., Birks, H. J. B., Liu, J. B., Zhang, S. R., et al. (2015). East Asian summer monsoon precipitation variability since the last deglaciation. Sci. Rep. 5:11186. doi: 10.1038/srep11186
Chen, J. A., Wan, G. J., and Tang, D. Q. (2000). Recent climate changes recorded by sediment grain sizes and isotopes in Erhai Lake. Prog. Nat. Sci. Mater. 10, 54–61. doi: 10.2183/pjab.76.7
Chen, J. H., Chen, F. H., Feng, S., Huang, W., Liu, J. B., and Zhou, A. F. (2015). Hydroclimatic changes in China and surroundings during the medieval climate anomaly and little ice age: spatial patterns and possible mechanisms. Quat. Sci. Rev. 107, 98–111. doi: 10.1016/j.quascirev.2014.10.012
Cheng, B., Adams, J., Chen, J. H., Zhou, A. F., and Mackay, A. (2020). Neoglacial trends in diatom dynamics from a small alpine lake in the Qinling Mountains of central China. Clim. Past. 16, 543–554. doi: 10.5194/cp-16-543-2020
Cheng, Y., Liu, H. Y., Wang, H. Y., Wang, H. Y., Piao, S. L., Yin, Y., et al. (2017). Contrasting effects of winter and summer climate on alpine timberline evolution in monsoon–dominated East Asia. Quat. Sci. Rev. 169, 278–287. doi: 10.1016/j.quascirev.2017.06.007
Cheng, Z. J., Weng, C. Y., Steinke, S., and Mohtadi, M. (2018). Anthropogenic modification of vegetated landscapes in southern China from 6,000 years ago. Nat. Geosci. 11, 939–944. doi: 10.1038/s41561-018-0250-1
Crutzen, P. J., and Stoermer, E. F. (2000). IGBP Newsletter 41. Stockholm: Royal Swedish Academy of Sciences.
Cui, Z. J., Tang, Y. X., Li, J. J., Liu, H. Y., and Liu, G. N. (2003). Information of Holocene environment changes from lacustrian deposits of the Foyechi lake section, Taibai Mountain. J. Geomech. 9, 330–336.
Ehrlich, P. R., and Wilson, E. O. (1991). Biodiversity studies: science and policy. Science 253, 758–762. doi: 10.1126/science.253.5021.758
Ellis, E. C., Gauthierb, N., Goldewijkd, K. K., Bird, R. B., Boiving, N., Díazi, S., et al. (2021). People have shaped most of terrestrial nature for at least 12,000 years. Proc. Natl. Acad. Sci. U.S.A. 118:2023483118. doi: 10.1073/pnas.2023483118
Fægri, K., and Iversen, J. (1989). Textbook of Pollen Analysis, 4th Edn. London: John Wiley and Sons, 328.
Fang, J. Y., Piao, S. L., He, J. S., and Ma, W. H. (2003). Increasing terrestrial vegetation activity in China, 1982–1999. Sci. China Ser. C 47, 229–240. doi: 10.1360/03yc0068
Felde, V. A., Flantua, S. G. A., Jenks, C. R., Benito, B. M., de Beaulieu, J. L., Kuneš, P., et al. (2020). Compositional turnover and variation in Eemian pollen sequences in Europe. Veg. Hist. Archaeobot. 29, 101–109. doi: 10.1007/s00334-019-00726-5
Felde, V. A., Peglar, S. M., Bjune, A. E., Grytnes, J. A., and Birks, H. J. B. (2016). Modern pollen-plant richness and diversity relationships exist along a vegetational gradient in southern Norway. Holocene 26, 163–175. doi: 10.1177/0959683615596843
Gansert, D. (2004). Treelines of the Japanese Alps–altitudinal distribution and species composition under contrasting winter climates. Flora 199, 143–156. doi: 10.1078/0367-2530-00143
Grimm, E. C. (1987). CONISS: a FORTRAN 77 program for stratigraphically constrained cluster analysis by the method of incremental sum of squares. Comput. Geosci. 13, 13–35. doi: 10.1016/0098-3004(87)90022-7
Guiot, J., and Goeury, C. (1996). PPPBASE, a software for statistical analysis of paleoecological and paleoclimatological data. Dendrochronologia 14, 295–300.
Hart, R., Salick, J., Ranjitka, r S, and Xu, J. C. (2014). Herbarium specimens show contrasting phenological responses to Himalayan climate. Proc. Natl. Acad. Sci. U.S.A. 111, 10615–10619. doi: 10.1073/pnas.1403376111
Herzschuh, U., Birks, H. J. B., Liu, X. Q., Kubatzki, C., and Lohmann, G. (2010). What caused the mid-Holocene forest decline on the eastern Tibet-Qinghai Plateau? Glob. Ecol. Biogeogr. 19, 278–286. doi: 10.1111/j.1466-8238.2009.00501.x
Hill, M. O. (1973). Diversity and evenness: a unifying notation and its consequences. Ecology 54, 427–432. doi: 10.2307/1934352
Hou, H. Y. (1983). Vegetation of China with reference to its geographical distribution. Ann. Mo. Bot. Gard. 70, 509–549. doi: 10.2307/2992085
Hou, J. Z., Huang, Y. S., Zhao, J. T., Liu, Z. H., Colman, S., and An, Z. S. (2016). Large Holocene summer temperature oscillations and impact on the peopling of the northeastern Tibetan Plateau. Geophys. Res. Lett. 43, 1323–1330.
Huang, C. L., Yang, Q. K., Guo, Y. H., Zhang, Y. Q., and Guo, L. N. (2020). The pattern, change and driven factors of vegetation cover in the Qin Mountains region. Sci. Rep. 10:20591. doi: 10.1038/s41598-020-75845-5
Huang, X. Y., Meyers, P. A., Jia, C. L., Zheng, M., Xue, J. T., Wang, X. X., et al. (2013). Paleotemperature variability in central China during the last 13 ka recorded by a novel microbial lipid proxy in the Dajiuhu peat deposit. Holocene 23, 1123–1129. doi: 10.1177/0959683613483617
Hurlbert, S. H. (1971). The nonconcept of species diversity: a critique and alternative parameters.” Ecology 52, 577–586. doi: 10.2307/1934145
Jackson, S. T., and Blois, J. L. (2015). Community ecology in a changing environment: perspectives from the Quaternary. Proc. Natl. Acad. Sci. U.S.A. 112, 4915–4921. doi: 10.1073/pnas.1403664111
Kolb, K. J., and Sperry, J. S. (1999). Differences in drought adaptation between subspecies of sagebrush (Artemisia tridentata). Ecology 80, 2373–2384. doi: 10.2307/176917
Kulkarni, C., Finsinger, W., Anand, P., Nogué, S., and Bhagwat, S. A. (2021). Synergistic impacts of anthropogenic fires and aridity on plant diversity in the Western Ghats: implications for management of ancient social–ecological systems. J. Environ. Manage. 283, 111957–111969. doi: 10.1016/j.jenvman.2021.111957
Lewis, S. L., and Maslin, M. A. (2015). Defining the Anthropocene. Nature 519, 171–180. doi: 10.1038/nature14258
Li, Q., and Zhao, Y. (2018). Quantitative methods and progress of paleo-floristic diversity reconstruction based on pollen assemblages. Quat. Sci. 38, 821–829. doi: 10.11928/j.issn.1001-7410.2018.04.02
Li, S. S., Lu, J. Y., Yan, J. P., Liu, X. F., Kong, F., and Wang, J. (2018). Spatiotemporal variability of temperature in northern and southern Qinling Mountains and its influence on climatic boundary. Acta Geogr. Sinica 73, 13–24.
Li, Y. C., Xu, Q. H., Cao, X. Y., Yang, X. L., Li, Y. Y., and Zhou, L. P. (2008). Pollen influx and surface pollen assemblage on the northern slope of Taibai Mountain. Geogr. Res. 27, 536–546.
Li, Y. F., Li, C. H., Xu, L. Y., Xu, B., and Pang, Y. Z. (2017). Relationships between vegetation and stomata, and between vegetation and pollen surface soil in Taibai mountains. Acta Palaeontol. Sin. 56, 108–116.
Liang, C., Zhao, Y., Qin, F., Cui, Q. Y., Li, Q., Li, H., et al. (2019). Complex responses of vegetation diversity to Holocene climate change in the eastern Tibetan Plateau. Veg. Hist. Archaeobot. 28, 379–390. doi: 10.1007/s00334-018-0697-4
Liu, G. N., Fu, H. R., Cui, Z. J., and Tang, Y. X. (2005). An 8000 years environmental change record of lacustrine deposits of Foyechi Lake at Taibai Mountains. Res. Soil Water Conserv. 12, 1–5.
Liu, H., Tang, Z., Dai, J., Tang, Y., and Cui, H. (2002). Larch timberline and its development in North China. Mt. Res. Dev. 22, 359–367.
Liu, Y., Linderholm, H. W., Song, H., Cai, Q., Tian, Q., Sun, J., et al. (2008). Temperature variations recorded in Pinus tabulaeformis tree rings from the southern and northern slopes of the central Qinling Mountains, central China. Boreas 38, 285–291.
Liu, Z. L., Fang, J. Y., and Piao, S. L. (2002). Geographical distribution of species in genera Abies, Picea and Larix in China. Acta Geogr. Sinica 57, 577–586.
Ma, X. P., Bai, H. Y., Guo, S., Deng, C. H., and Han, S. S. (2017). Verification of temperature vertical lapse rate and mountain climate character–istics of Taibai Mountains in Qinling Mountains. J. Arid Land Resour. Environ. 31, 139–144.
Ma, Y. Z., Liu, K. B., Feng, Z. D., Meng, H. W., Sang, Y. L., Wang, W., et al. (2013). Vegetation changes and associated climate variations during the past 38,000 years reconstructed from the Shaamar eolian–paleosol section, northern Mongolia. Quat. Int. 311, 25–35. doi: 10.1016/j.quaint.2013.08.037
McMichael, C. N. H. (2021). Ecological legacies of past human activities in Amazonian forests. New Phytol. 229, 2492–2496. doi: 10.1111/nph.16888
Meltsov, V., Poska, A., Odgaard, B. V., Sammul, M., and Kull, T. (2011). Palynological richness and pollen sample evenness in relation to local floristic diversity in southern Estonia. Rev. Palaeobot. Palynol. 166, 344–351. doi: 10.1016/j.revpalbo.2011.06.008
Moore, P. D., Webb, J. A., and Collison, M. E. (1991). Pollen analysis. J. Ecol. 80, 876–877. doi: 10.2307/2260877
Mottl, O., Flantua, S. G. A., Bhatta, K. P., Felde, V. A., Giesecke, T., Goring, S., et al. (2021). Global acceleration in rates of vegetation change over the past 18,000 years. Science 372, 860–864. doi: 10.1126/science.abg1685
Ni, J., Cao, X. Y., Jeltsch, F., and Herzschuh, U. (2014). Biome distribution over the last 22,000 yr in China. Palaeogeogr. Palaeoclimatol. Palaeoecol. 409, 33–47. doi: 10.1016/j.palaeo.2014.04.023
Odgaard, B. V. (1999). Fossil pollen as a record of past biodiversity. J. Biogeogr. 26, 7–11. doi: 10.1046/j.1365-2699.1999.00280.x
Odgaard, B. V. (2001). Palaeoecological perspectives on pattern and process in plant diversity and distribution adjustments: a comment on recent developments. Divers. Distrib. 7, 197–201. doi: 10.1111/j.1472-4642.2001.00110.x
Oksanen, J., Blanchet, F. G., Kindt, R., Legendre, P., Minchin, P. R., O’Hara, R. B., et al. (2020). vegan Community Ecology Package Version 2.5–7 November 2020. vegan Community Ecology Package. 1–298.
Pang, D. Q. (1998). The basic features of Taibai Mountain in Qinling. J. Baoji Coll. Arts Sci. (Nat. Sci.) 18, 41–44.
Park, J., Park, J., Yi, S., Kim, J. C., Lee, E., and Choi, J. (2019). Abrupt Holocene climate shifts in coastal East Asia, including the 8.2 ka, 4.2 ka, and 2.8 ka BP events, and societal responses on the Korean peninsula. Sci. Rep. 9:10806. doi: 10.1038/s41598-019-47264-8
Parmesan, C. (2007). Influences of species, latitudes and methodologies on estimates of phenological response to global warming. Glob. Change Biol. 13, 1860–1872. doi: 10.1111/j.1365-2486.2007.01404.x
Peng, S. B., Huang, J. L., Sheehy, J. E., Laza, R. C., Visperas, R. M., Zhong, X. H., et al. (2004). Rice yields decline with higher night temperature from global warming. Proc. Natl. Acad. Sci. U.S.A. 101, 9971–9975. doi: 10.1073/pnas.0403720101
Pepin, N., Bradley, R. S., Diaz, H. F., Baraer, M., Caceres, E. B., Forsythe, N., et al. (2015). Elevation–dependent warming in mountain regions of the world. Nat. Clim. Change 5, 424–430. doi: 10.1038/NCLIMATE2563
Peuelas, J., and Filella, I. (2001). Responses to a warming world. Science 294, 793–795. doi: 10.1126/science.1066860
Pielou, E. C. J. (1966). The measurement of diversity in different types of biological collections. J. Theor. Biol. 13, 131–144. doi: 10.1016/0022-5193(66)90013-0
Pimm, S. L., Jenkins, C. N., Abell, R., Brooks, T. M., Gittleman, J. L., Joppa, L. N., et al. (2014). The biodiversity of species and their rates of extinction, distribution, and protection. Science 344:1246752. doi: 10.1126/science.1246752
Prentice, I. C., Guiot, J., Huntley, B., Jolly, D., and Cheddadi, R. (1996). Reconstructing biomes from palaeoecological data: a general method and its application to European pollen data at 0 and 6 ka. Clim. Dyn. 12, 185–194. doi: 10.1007/BF00211617
Reimer, P. J., Austin, W. E. N., Bard, E., Bayliss, A., Blackwell, P. G., Ramsey, C. B., et al. (2020). The IntCal20 Northern Hemisphere radiocarbon age calibration curve (0–55 kcal BP). Radiocarbon 62, 725–757. doi: 10.1017/RDC.2020.41
Rioual, P., Andrieu–Ponel, V., Rietti–Shati, M., Battarbee, R. W., de Beaulieu, J. L., Cheddadi, R., et al. (2001). High–resolution record of climate stability in France during the last interglacial period. Nature 413, 293–296. doi: 10.1038/35095037
Root, T. L., Price, J. T., Hall, K. R., Schneider, S. H., Rosenzweig, S., and Pounds, J. A. (2003). Fingerprints of global warming on wild animals and plants. Nature 421, 57–60. doi: 10.1038/nature01333
Rudaya, N., Krivonogov, S., Słowiński, M., Cao, X. Y., and Zhilich, S. (2020). Postglacial history of the Steppe Altai: climate, fire and plant diversity. Quat. Sci. Rev. 249:106616. doi: 10.1016/j.quascirev.2020.106616
Rudaya, N., Tarasov, P., Dorofeyuk, N., Solovieva, N., Kalugin, I., Andreev, A., et al. (2008). Holocene environments and climate in the Mongolian Altai reconstructed from the Hoton–Nur pollen and diatom records: a step towards better understanding climate dynamics in Central Asia. Quat. Sci. Rev. 28, 540–554. doi: 10.1016/j.quascirev.2008.10.013
Ruddiman, W. F. (2003). The anthropogenic greenhouse era began thousands of years ago. Clim. Change 61, 261–293. doi: 10.1023/B:CLIM.0000004577.17928.fa
Shang, S. H., and Xing, H. H. (2016). Comparison of the differences in the vegetation vertical distribution on the southern and northern slope of Qinling Mountains. Ji Lin Griculture. 114–115. doi: 10.14025/j.cnki.jlny.2016.01.057
Shang, X., and Li, X. Q. (2010). Holocene vegetation characteristics of the southern Loess Plateau in the Weihe River valley in China. Rev. Palaeobot. Palynol. 160, 46–52. doi: 10.1016/j.revpalbo.2010.01.004
Shaver, G. (2000). Global warming and terrestrial ecosystems: a conceptual framework for analysis. Bioscience 50, 871–882.
Srivastava, J., Manjunatha, B. R., Balakrishna, K., Prajith, A., Manjunatha, H. V., Jose, J., et al. (2021). Quantitative pollen–based reconstruction of the vegetation diversity in response to the late–Holocene climate change near Karwar, south–west coast of India. Quat. Int. 599-600, 95–106. doi: 10.1016/j.quaint.2021.03.026
Stirling, G., and Wilsey, B. (2001). Empirical relationships between species richness, evenness, and proportional diversity. Am. Nat. 158, 286–299. doi: 10.1086/321317
Sun, H., Bai, H. Y., Zhang, Q. Y., Luo, X. P., and Zhang, S. H. (2009). Responses of vegetation coverage to regional environmental change in the northern and southern Qinling Mountains. Acta Sci. Circumstantiae 29, 2635–2641.
Sun, J., Ma, C. M., Cao, X. Y., Zhao, Y., and Zhu, C. (2019). Quantitative precipitation reconstruction in the east–central monsoonal China since the late glacial period. Quat. Int. 521, 175–184. doi: 10.1016/j.quaint.2019.05.033
Sun, N., Li, X. Q., Dodson, J., Zhou, X. Y., Zhao, K. L., and Yang, Q. (2013). Plant diversity of the Tianshui Basin in the western Loess Plateau during the mid–Holocene–Charcoal records from archaeological sites. Quat. Int. 308–309, 27–35. doi: 10.1016/j.quaint.2013.05.014
Tang, L. Y., Mao, L. M., Shu, J. W., Li, C. H., Shen, C. M., and Zhou, Z. Z. (2016). Atlas of Quaternary Pollen and Spores in China. Beijing: Science Press.
Tao, S. C., An, C. B., Chen, F. H., Liu, K. B., and Tang, L. Y. (2013). An abrupt climatic event around 4.2 cal ka BP documented by fossil pollen of Tuolekule Lake in the eastern Xinjiang Uyghur autonomous region. Acta Palaeontol. Sinica 52, 234–242.
Tarasov, P. E., Webb, T., Andreev, A. A., Afanas’eva, N. B., Berezina, N. A., Bezusko, L. G., et al. (1998). Present–day and mid–Holocene biomes reconstructed from pollen and plant macrofossil data from the former Soviet Union and Mongolia. J. Biogeogr. 25, 1029–1053. doi: 10.1046/j.1365-2699.1998.00236.x
Thomas, C. D., Cameron, A., Green, R. E., Green, R. E., Bakkenes, M., Beaumont, L. J., et al. (2004). Extinction risk from climate change. Nature 427, 145–148. doi: 10.1038/nature02121
Tian, Z. S., and Huang, C. C. (1990). The glaciation process in Mt.Taibai of the Qinling Mountains and the climatic changes in the Loess Plateau. Geogr. Res. Aust. 9, 15–23.
Trivedi, A., Tangc, Y. N., Qin, F., Farooqui, A., Wortley, A. H., Wang, Y. F., et al. (2020). Holocene vegetation dynamics and climatic fluctuations from Shuanghaizi Lake in the Hengduan Mountains, southwestern China. Palaeogeogr. Palaeoclimatol. Palaeoecol. 560:110035. doi: 10.1016/j.palaeo.2020.110035
Uotila, P., Sukhorukov, A. P., Bobon, N., McDonald, J., Krinitsina, A. A., and Kadereit, G. (2021). Phylogeny, biogeography and systematics of Dysphanieae (Amaranthaceae). Taxon 70, 526–551.
Vitousek, P. M. (1994). Beyond global warming: ecology and global change. Ecology 75, 1861–1876. doi: 10.2307/1941591
Wagner, B., Melles, M., Hahne, J., Hubberten, H. W., and Niessen, F. (2000). Holocene climate history of Geogra Phieal Society, East Greenland– evidence from lake sediments. Palaeogeogr. Palaeoclimatol. Palaeoecol. 160, 45–68. doi: 10.1016/S0031-0182(00)00046-8
Wake, D. B., and Vredenburg, V. T. (2008). Are we in the midst of the sixth massextinction? A view from the world of amphibians. Proc. Natl. Acad. Sci. U.S.A. 105, 11466–11473. doi: 10.1073/pnas.0801921105
Walther, G. R., Post, E., Convey, P., Menzel, A., Parmesan, C., Beebee, J. T. C., et al. (2002). Ecological responses to recent climate change. Nature 416, 389–395. doi: 10.1038/416389a
Wang, F. X., Qian, N. F., Zhang, Y. L., and Yang, H. Q. (1995). Pollen Flora of China. Beijing: Science Press.
Wang, H. Y., Cheng, Y., Luo, Y., Zhang, C. N., Deng, L., Yang, X. Y., et al. (2019). Variations in erosion intensity and soil maturity as revealed by mineral magnetism of sediments from an alpine lake in monsoon–dominated central east China and their implications for environmental changes over the past 5500 years. Holocene 29, 1835–1855. doi: 10.1177/0959683619865583
Wang, H. Y., Song, Y. Q., Cheng, Y., Luo, Y., Zhang, C. N., Gao, Y. S., et al. (2016). Mineral magnetism and other characteristics of sediments from a sub–alpine lake (3080 m a.s.l.) in central east China and their implications on environmental changes for the last 5770 years. Earth Planet Sci. Lett. 452, 44–59. doi: 10.1016/j.epsl.2016.07.031
Wang, X., Yao, Y. F., Wortley, A. H., Qiao, H. J., Blackmore, S., Wang, Y. F., et al. (2018). Vegetation responses to the warming at the Younger Dryas–Holocene transition in the Hengduan Mountains, southwestern China. Quat. Sci. Rev. 192, 236–248. doi: 10.1016/j.quascirev.2018.06.007
Weng, C., Hooghiemstra, H., and Duivenvoorden, J. F. (2006). Challenges in estimating past plant diversity from fossil pollen data: statistical assessment, problems, and possible solutions. Divers. Distrib. 12, 310–318. doi: 10.1111/j.1366-9516.2006.00230.x
Willis, K. J., Bailey, R. M., Bhagwat, S. A., and Birks, H. J. B. (2010). Biodiversity baselines, thresholds and resilience: testing predictions and assumptions using palaeoecological data. Trends Ecol. Evol. 25, 583–591. doi: 10.1016/j.tree.2010.07.006
Willis, K. J., Gillson, L., Brncic, T. M., and Figueroa-Rangel, B. L. (2004). Providing baselines for biodiversity measurement. Trends Ecol. Evol. 20, 107–108. doi: 10.1016/j.tree.2004.12.003
Woodbridge, J., Fyfe, R., Smith, D., Pelling, R., de Vareilles, A., Batchelor, R., et al. (2021). What drives biodiversity patterns? Using long-term multidisciplinary data to discern centennial-scale change. J. Ecol. 109, 1396–1410. doi: 10.1111/1365-2745.13565
Xiao, X. Y., Shen, J., Wang, S. M., Xiao, H. F., and Tong, G. B. (2008). The plant diversity and its relationship with paleoenvironment since 2.78 Ma revealed by pollen records in the Heqing deep drilling core. Sci. Bull. 53, 3686–3698. doi: 10.1007/s11434-008-0439-6
Xiao, X. Y., Yao, A., Hillman, A., Shen, J., and Haberle, S. G. (2020). Vegetation, climate and human impact since 20 ka in central Yunnan Province based on high–resolution pollen and charcoal records from Dianchi, southwestern China. Quat. Sci. Rev. 236:106297. doi: 10.1016/j.quascirev.2020.106297
Xu, D. K., Lu, H. Y., Chu, G. Q., Liu, L., Shen, C. M., Li, F. J., et al. (2019). Synchronous 500–year oscillations of monsoon climate and human activity in Northeast Asia. Nat. Commun. 10:4105. doi: 10.1038/s41467-019-12138-0
Xu, Q. H., Xiao, J. L., Li, Y. C., Tian, F., and Nakagawa, T. (2010). Pollen-based quantitative reconstruction of holocene climate changes in the Daihai Lake Area, Inner Mongolia, China. J. Clim. 23, 2856–2868. doi: 10.1175/2009JCLI3155.1
Yu, G., Chen, X., Ni, J., Cheddadi, R., Guiot, J., Han, H., et al. (2000). Palaeovegetation of China: a pollen data–based synthesis for the mid–Holocene and last glacial maximum. J. Biogeogr. 27, 635–664. doi: 10.1046/j.1365-2699.2000.00431.x
Yu, Q., Jia, D. R., Tian, B., Yang, Y. P., and Duan, Y. W. (2016). Changes of flowering phenology and flower size in rosaceous plants from a biodiversity hotspot in the past century. Sci. Rep. 6:28302. doi: 10.1038/srep28302
Zhang, B., Xu, J., Wu, H., Xiao, F., and Zhu, Y. (2006). Digital integration and pattern analysis of mountain altitudinal belts in China. J. Mt. Sci. 24, 144–149. doi: 10.1109/TPSD.2006.5507455
Zhang, D. L., Chen, X., Li, Y. M., and Zhang, S. R. (2020). Holocene vegetation dynamics and associated climate changes in the Altai Mountains of the Arid Central Asia. Palaeogeogr. Palaeoclimatol. Palaeoecol. 550:109744. doi: 10.1016/j.palaeo.2020.109744
Zhang, J. N., Xia, Z. K., Zhang, X. H., Storozum, M. J., Huang, X. Z., Han, J. Y., et al. (2018). Early–middle Holocene ecological change and its influence on human subsistence strategies in the Luoyang basin, north-central china. Quat. Res. 89, 446–458.
Zhang, J. P., Tong, M., Wang, S. B., and Tong, G. B. (2001). Palaeoclimatic reconstruction of sporopollen recorded in Fangxiangsi profile, Taibaishan Mountain. Int. J. Geomech. 7, 315–320.
Zhang, Y., Kong, Z. C., Yan, S., Yang, Z. J., and Ni, J. (2005). Palaeo-biodiversity at the northern piedmont of Tianshan mountains in Xinjiang during the middle to late holocene. Acta Phytoecol. Sinica 29, 836–844.
Zhang, Y., Marquer, L., Cui, Q. Y., Zheng, Z., Zhao, Y., Wan, Q. C., et al. (2021). Holocene vegetation changes in the transition zone between subtropical and temperate ecosystems in Eastern Central China. Quat. Sci. Rev. 253:106768. doi: 10.1016/j.quascirev.2020.106768
Zhao, S. J., and Ding, Z. L. (2014). Changes in plant diversity on the Chinese Loess Plateau since the Last Glacial Maximum. Sci. Bull. 59, 4096–4100. doi: 10.1007/s11434-014-0541-x
Keywords: pollen, palynological diversity, biomes, climate, Qinling Mountain range
Citation: Zhang Y, Cui Q, Huang Y, Wu D and Zhou A (2021) Vegetation Response to Holocene Climate Change in the Qinling Mountains in the Temperate–Subtropical Transition Zone of Central–East China. Front. Ecol. Evol. 9:734011. doi: 10.3389/fevo.2021.734011
Received: 30 June 2021; Accepted: 12 August 2021;
Published: 14 September 2021.
Edited by:
Niina Kuosmanen, University of Helsinki, FinlandReviewed by:
Petr Kuneš, Charles University, CzechiaCopyright © 2021 Zhang, Cui, Huang, Wu and Zhou. This is an open-access article distributed under the terms of the Creative Commons Attribution License (CC BY). The use, distribution or reproduction in other forums is permitted, provided the original author(s) and the copyright owner(s) are credited and that the original publication in this journal is cited, in accordance with accepted academic practice. No use, distribution or reproduction is permitted which does not comply with these terms.
*Correspondence: Qiaoyu Cui, cWlhb3l1LmN1aUBpZ3NucnIuYWMuY24=; Aifeng Zhou, emhvdWFmQGx6dS5lZHUuY24=
Disclaimer: All claims expressed in this article are solely those of the authors and do not necessarily represent those of their affiliated organizations, or those of the publisher, the editors and the reviewers. Any product that may be evaluated in this article or claim that may be made by its manufacturer is not guaranteed or endorsed by the publisher.
Research integrity at Frontiers
Learn more about the work of our research integrity team to safeguard the quality of each article we publish.