- 1Department of Animal Ecology, Netherlands Institute of Ecology (NIOO-KNAW), Wageningen, Netherlands
- 2Groningen Institute for Evolutionary Life Sciences, University of Groningen, Groningen, Netherlands
- 3Institute of Biodiversity, Animal Health and Comparative Medicine, University of Glasgow, Glasgow, United Kingdom
Artificial light at night (ALAN) has been recognized as a biodiversity threat due to the drastic effects it can have on many organisms. In wild birds, artificial illumination alters many natural behaviors that are important for fitness, including chick provisioning. Although incubation is a key determinant of the early developmental environment, studies into the effects of ALAN on bird incubation behavior are lacking. We measured nest temperature in nest boxes of great tits during the incubation period in two consecutive years. Nest boxes were located in eight previously dark field sites that have been experimentally illuminated since 2012 with white, green, or red light, or were left dark. We tested if light treatment affected mean nest temperature, number of times birds leave the nest (off-bout frequency), and off-bout duration during the incubation period. Subsequently, we investigated if incubation behavior is related to fitness. We found that birds incubating in the white light during a cold, early spring had lower mean nest temperatures at the end of incubation, both during the day and during the night, compared to birds in the green light. Moreover, birds incubating in white light took fewer off-bouts, but off-bouts were on average longer. The opposite was true for birds breeding in the green light. Low incubation temperatures and few but long off-bouts can have severe consequences for developing embryos. In our study, eggs from birds that took on average few off-bouts needed more incubation days to hatch compared to eggs from birds that took many off-bouts. Nevertheless, we found no clear fitness effects of light treatment or incubation behavior on the number of hatchlings or hatchling weight. Our results add to the growing body of literature that shows that effects of ALAN can be subtle, can differ due to the spectral composition of light, and can be year-dependent. These subtle alterations of natural behaviors might not have severe fitness consequences in the short-term. However, in the long term they could add up, negatively affecting parent condition and survival as well as offspring recruitment, especially in urban environments where more environmental pollutants are present.
Introduction
Artificial light at night (ALAN) is considered a polluter of natural environments due to the profound effects it has on wildlife (Hölker et al., 2010). Organisms use natural light and dark cycles as a cue to time important biological processes, and possess endogenous circannual and circadian biological clocks synchronized to these cycles (Dunlap, 1999). Light is thus an important physiological signal, providing ALAN with ample targets to affect physiological processes and behavior (Falcón et al., 2020). To effectively mitigate negative effects of light pollution, we need to understand how ALAN affects different species, and how light intensity and light spectrum modulate these effects (Gaston et al., 2015).
Relatively few studies have assessed the effects of ALAN on parental behavior, while such effects could have important implications for fitness as the early developmental environment can have long-term effects on the behavior and physiology of offspring (De Kogel, 1997; van Oers et al., 2015). In birds, ALAN can affect nestling condition, with experimentally exposed nestlings having lower body mass (Raap et al., 2016a) and increased immune responses (Raap et al., 2016b; Saini et al., 2019). Nestling condition might be further affected through parental behavior, as ALAN can increase parental provisioning rates (Titulaer et al., 2012), but this is not always observed (Welbers et al., 2017).
ALAN could also affect the developmental environment through effects on parental condition. Birds breeding under white and red light were found to have higher baseline stress levels, and birds with higher baseline stress levels tended to produce fewer fledglings (Ouyang et al., 2015). Additionally, birds have been found to be more active at night under white light, increasing their sleep debt (Ouyang et al., 2017), although cavity breeders might be shielded from such negative effects on sleep (Raap et al., 2018).
Negative effects of ALAN on parental condition could affect incubation behavior. Parents in poor condition are expected to be less effective incubators, as they need to leave the nest more often for self-maintenance (DuRant et al., 2013). For example, lower ambient temperatures result in parents taking longer off-bouts, presumably due to higher energetic demands (Schöll et al., 2020). This can have important implications for developing embryos, as leaving the nest can result in considerable reductions in nest temperature (Greeney, 2009). Regular cooling periods can lower embryo mass and reduce yolk reserves (Olson et al., 2006), and can adversely affect incubation period length and hatching success (Olson et al., 2006; Nord and Nilsson, 2011). Decreased nest attendance is especially detrimental late in incubation, as thermal tolerance of embryos decreases with age, to which parents respond by taking more frequent but shorter off-bouts (Cooper and Voss, 2013). Parental condition during incubation can also indirectly affect offspring, as parents with increased stress levels produce eggs with higher corticosterone concentrations, which affects their hatching success as well as offspring condition (Saino et al., 2005). In captivity, constant light pollution can interrupt incubation in commercially bred turkey hens (Proudman and Opel, 1981). However, studies that investigate the effects of ALAN on incubation behavior in wild birds are lacking.
Here, we investigate if ALAN of different spectra affects incubation behavior. In two consecutive years, we monitored the incubation of great tits breeding in nest boxes that were spread over eight previously dark field sites, which have been experimentally illuminated since 2012 with white, green, or red light, or were left dark. For each nest box, we recorded general life-history data including hatching date and number of hatchlings, and measured mean nest temperature, off-bout frequency, and off-bout duration per day during the incubation period. Subsequently, we investigated if incubation behavior related to offspring condition and fitness. We expected different colors of ALAN to affect incubation behavior and fitness to different extents. Several studies have shown that the response of birds to ALAN is often wavelength-specific (de Jong et al., 2017; Alaasam et al., 2018; Aulsebrook et al., 2020), likely because the photoreceptors involved in several physiological processes, such as reproduction and hormone production, are more sensitive to certain wavelengths (Rani and Kumar, 2000; Hunt et al., 2009; Grubisic et al., 2019). Specifically, we predicted ALAN to negatively affect incubation in at least the white light treatment compared to the dark as previous research shows that white light can negatively affect bird condition (Ouyang et al., 2015, 2017). We therefore expected birds in the white light to take longer off-bouts and have lower incubation temperatures. As incubation is a key determinant of the prenatal environment, we furthermore expected incubation behavior to affect life-history traits: we expected birds that showed suboptimal incubation behavior (e.g., low nest temperatures) to have a longer incubation length and/or lower hatching success.
Materials and Methods
Experimental Set-Up
To investigate the effects of ALAN, we made use of a standardized experimental field set-up in which eight previously dark forest edges have been illuminated from sunset to sunrise since 2012 (see for details Spoelstra et al., 2015). In short, each field site consists of four forest edge transects with five lampposts, emitting either ClearField red, Fortimo white, or ClearSky green LED light (Philips, Amsterdam, the Netherlands), or no light, with the order of transects randomly assigned at the start of the experiment. As ALAN is meant for civil use, light intensities were standardized to lux (8.2 ± 0.3 lux measured directly under the lamp at ground level). All three light colors have a full color spectrum, but with a different composition: green lamps emit more short and less long wavelengths, while red lights emit less short and more long wavelengths. In each transect, nine nest boxes were placed in the forest edge around the lamp post in a standardized pattern, differing in the distance to the nearest light post (36 nest boxes per field site, see for details de Jong et al., 2015).
Incubation Behavior Data Collection
In two consecutive breeding seasons (2016 and 2017), we measured nest temperature and life-history traits for great tits breeding in the nest boxes around the lamp posts at the eight different field sites. Temperature loggers (Maxim Integrated, DS1922L-F5#, United Kingdom) were covered with panty hose and attached to a piece of wire to prevent birds from removing it from the nest. Loggers were then placed in the nest cup alongside the eggs, either just before or during incubation (Figure 1), with similar sample size distributions between light treatments for when during incubation loggers were placed. Loggers recorded nest temperature every 2 min at a resolution of 0.0625°C and were read out in the field every 4–5 days, until removed completely on the day of hatching of the first egg. Read-out lasted on average 4 min, and if females were present at read-out and subsequently left, they returned on average after 6–8 min (estimated from nest temperature data, see next section). At two sites, we also recorded ambient temperature on the outside of a nest box with temperature loggers. Ambient temperature data were supplemented with hourly average temperatures downloaded from a nearby weather station (Royal Dutch Meteorological Institute (KNMI) stations Hoogeveen and Deelen).
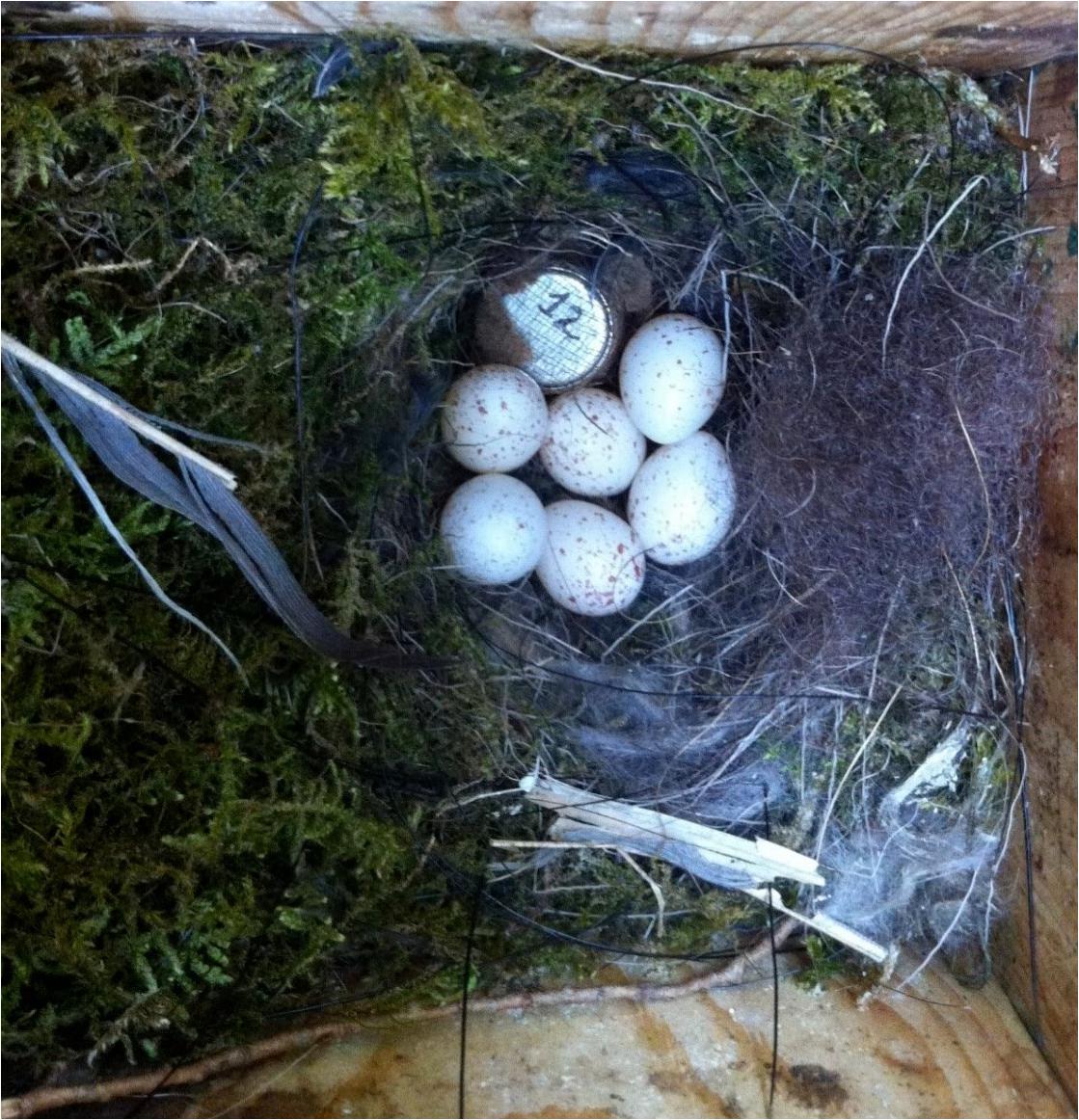
Figure 1. Example of temperature logger placed in the nest cup just before or during incubation. Loggers were covered with panty hose and attached to a piece of wire to prevent birds from removing it from the nest.
We checked each nest on average twice a week and recorded lay date of the first egg, clutch size, hatch date, and the number of hatchlings. In 2016, for a subset of the nests, chicks were also weighed 2 days after hatching as a measure of chick condition. Incubation length was calculated from the life-history data, assuming great tits lay one egg a day from the first lay date onward and start incubation on the day the last egg was laid. As clutch size was noted down at every nest check, we corrected incubation length for any observed lay delays.
For each nest box, nest temperature data from the last 13 days before hatching were used for the analysis of incubation behavior, as this is the average incubation length of great tits (Van Balen, 1973). We included boxes for which we measured at least the last 3 days of incubation in the analysis, as decreased nest attendance is especially detrimental late in incubation (Cooper and Voss, 2013). Boxes for which the last 3 days of incubation were missing were excluded from the analysis (2017 N = 2). Boxes that were abandoned (2016 N = 10, 2017 N = 5) or predated (2017 N = 1) before hatching were also excluded. One other box was excluded from analysis, because eggs were unfertilized and did not hatch (2016 N = 1), and one box was excluded because of misplacement of the temperature logger, which only recorded ambient temperature (2016 N = 1). Table 1 reports sample sizes of boxes included in the analysis for each treatment in each year. Sample sizes showed similar distributions between light treatments for when during the season and for when during incubation nests were sampled (Supplementary Figures 1, 2).
Statistical Analyses
Incubation Behavior
To analyze the recorded nest temperatures, we summarized the data for each nest into five incubation behavior parameters per incubation day measured. These parameters and their respective models were:
1. Mean day nest temperature | (Linear Mixed Model (LMM)) |
2. Mean night nest temperature | (LMM) |
3. Mean variation in temperature at night | (log transformed LMM) |
4. Mean off-bout frequency | (Poisson Generalized LMM (GLMM)) |
5. Mean off-bout duration | (log transformed LMM) |
Mean day and night nest temperature were chosen to reflect average incubation effort, mean variation in temperature at night to investigate ALAN effects related to restlessness at night (e.g., Ouyang et al., 2017), and mean off-bout frequency and duration to investigate ALAN effects related to food requirements and availability (e.g., Welbers et al., 2017). Incubation days with data gaps larger than 1 h due to temperature logger malfunctioning were excluded from analysis (86 days out of 1,118 for 63 boxes), as such gaps would exert a large influence on daily means. In total, we included 1,032 incubation days for 103 boxes in the analysis (see Table 1 for sample sizes per treatment).
Off-bout frequency and off-bout duration were inferred from the data with a running mean algorithm. This off-bout detection algorithm fits a running mean with a time window of 2 h through the nest temperature data (see Supplementary Figure 3 for an example). If the temperature dropped below the running mean, the start of an off-bout was detected, with the end of the off-bout detected when the minimum temperature was reached and the subsequent temperature measures increased again. Each off-bout’s start, minimum temperature, and duration were recorded, from which mean off-bout frequency and mean off-bout duration could be calculated for each nest per incubation day measured. The distributions of detected off-bouts were similar between treatments (Supplementary Figure 4) and showed a large peak for off-bouts with a very short duration and/or small drop in temperature (i.e., off-bout surface defined as the product of the difference from the running mean times off-bout duration). As these are most likely not true off-bouts, we excluded off-bouts with a duration < 5 min and a surface < 1.25 from the analysis, based on the peaks in the respective histograms (Supplementary Figure 5). We only analyzed off-bouts that occurred between sunrise and sunset as the majority of the drops below the running mean during the night are a result of much more stable incubation temperatures.
For each incubation parameter, we fit a linear or generalized linear mixed model (LMM or GLMM, see above) with R version 4.02. (R Core Team, 2020) packages lme4 and nlme (Bates et al., 2015; Pinheiro et al., 2021). Ambient temperature, clutch size, and Julian hatch date nested in year were included as covariates. All covariates were centered before inclusion in the model. As fixed effects, we used light treatment as a factor with year specific treatment levels, in interaction with (1) distance to lamp post, (2) incubation day, and (3) incubation day squared. Incubation day was defined as day until hatching, with hatching at day 0. Because effects of artificial illumination are often year-dependent (e.g., Dominoni et al., 2020), we chose year specific treatment levels to avoid having to fit three-way interactions. Interaction terms with P > 0.05 were dropped from the final model to be able to then test for the main effect of light treatment. Field site and nest box ID were included as random effects, to account for multiple incubation days per nest box and shared field site between nest boxes.
For three out of four LMM models, we used variance models because linear model assumptions were violated (see above, R package nlme). Variance decreased with higher nest temperatures, following the natural increase in incubation effort as eggs get closer to hatching (Haftorn, 1981). To account for this variance heterogeneity, we modeled variance by incubation day which resulted in models with more homogenous residual distributions and lower Akaike information criterion (AIC) values (dAIC > 10 compared to non-variance model). Mean variation in night nest temperature and mean off-bout duration were log transformed to meet model assumptions. Residuals from the Poisson GLMM for off-bout frequency were not overdispersed.
We performed post-hoc tests for models whose ANOVA indicated that light treatment was a significant explainer (α = 0.05). All post-hoc treatment comparisons were performed within breeding season year and were corrected for multiple comparisons with the Tukey method, using R package emmeans (Lenth, 2020). When the interaction between incubation day and treatment was significant, we first performed post-hoc tests to compare slopes between treatments. We then performed a broken-stick analysis to divide the incubation period into two phases: early and late incubation. This way we could find the period before hatching when great tits have reached their maximum incubation effort (Haftorn, 1981). Subsequently, treatment effects were compared for early and late incubation separately within year. For our results, we considered significant comparisons (P < 0.05) and trends (P < 0.10).
Fitness Data
For each incubation parameter, we calculated per box the mean of the incubation days included in the analyses described above. We then tested if incubation behavior was correlated with incubation length in days and fitness, measured as number of hatchlings. For a subset of boxes from 2016, we also tested for effects on chick weight 2 days after hatching and on nest variation in chick weight at day two (i.e., standard deviation of chick weight in grams per nest). For the chick weight analysis, we had 12 nest boxes for the red light treatment and 9 boxes for each of the other light treatments.
For each fitness measure, we fit a linear mixed model (LMM) with year specific light treatment levels, and 1 day time-incubation effort parameter (off-bout frequency) and one night time-incubation effort parameter (mean night nest temperature) included as fixed effects as we expect ALAN to affect day and night behavior via different routes (Spoelstra et al., 2015). To avoid multicollinearity, we did not include the other incubation effort parameters (mean day nest temperature, variation in night nest temperature, and off-bout duration). For the analysis of number of hatchlings and hatch weight, incubation length was also included as fixed effect. The same centered covariates were included as in the analysis of incubation behavior (see above). Field site was included as a random factor. For models with light treatment as a significant explainer, we fit the same model with all fixed effects except light treatment, and then tested for the effect of light treatment on the residuals of these models. These models were more informative of the actual light treatment effects on fitness, as treatment effects are not only tested for the average value of the covariates, but the dependent variable is corrected for the covariates before testing. All post-hoc treatment comparisons were performed within breeding season year.
Results
Mean Day and Night Nest Temperature
Artificial light at night only affected nest temperature in 2017 (Figure 2, Supplementary Figure 6, and Supplementary Tables 1, 2), and the effects depended on light color, incubation day, incubation day squared, and year (Supplementary Figure 7). The fitted models explained a large proportion of the variance in nest temperature (day mean conditional R squared (R2c) = 0.79, night mean R2c = 0.67). Our broken stick analysis indicated that birds reached their maximum nest temperature 8 days before hatching of the eggs (day mean break point = −8.39 and night mean break point = −7.70), which we then used to split the incubation period in early and late phases. During the day, birds in the white treatment had a significantly lower mean nest temperature toward the end of incubation compared to birds breeding in the green treatment [estimate = −5.04°C, t(77) = −4.48, P < 0.001], with a similar trend compared to the red treatment [estimate = −3.47°C, t(77) = −3.00, P = 0.06] but not compared to the dark [estimate = −2.57°C, t(77) = −2.19, P = 0.34]. Mean day and mean night nest temperature were highly correlated [r(1,030) = 0.76, P < 0.001], and we found the same effects of light treatment on mean night nest temperatures in 2017 (Supplementary Figure 6 and Supplementary Table 2). We found no effects of light at night on variation in night nest temperature [F(7) = 0.67, P = 0.70, Supplementary Table 3].
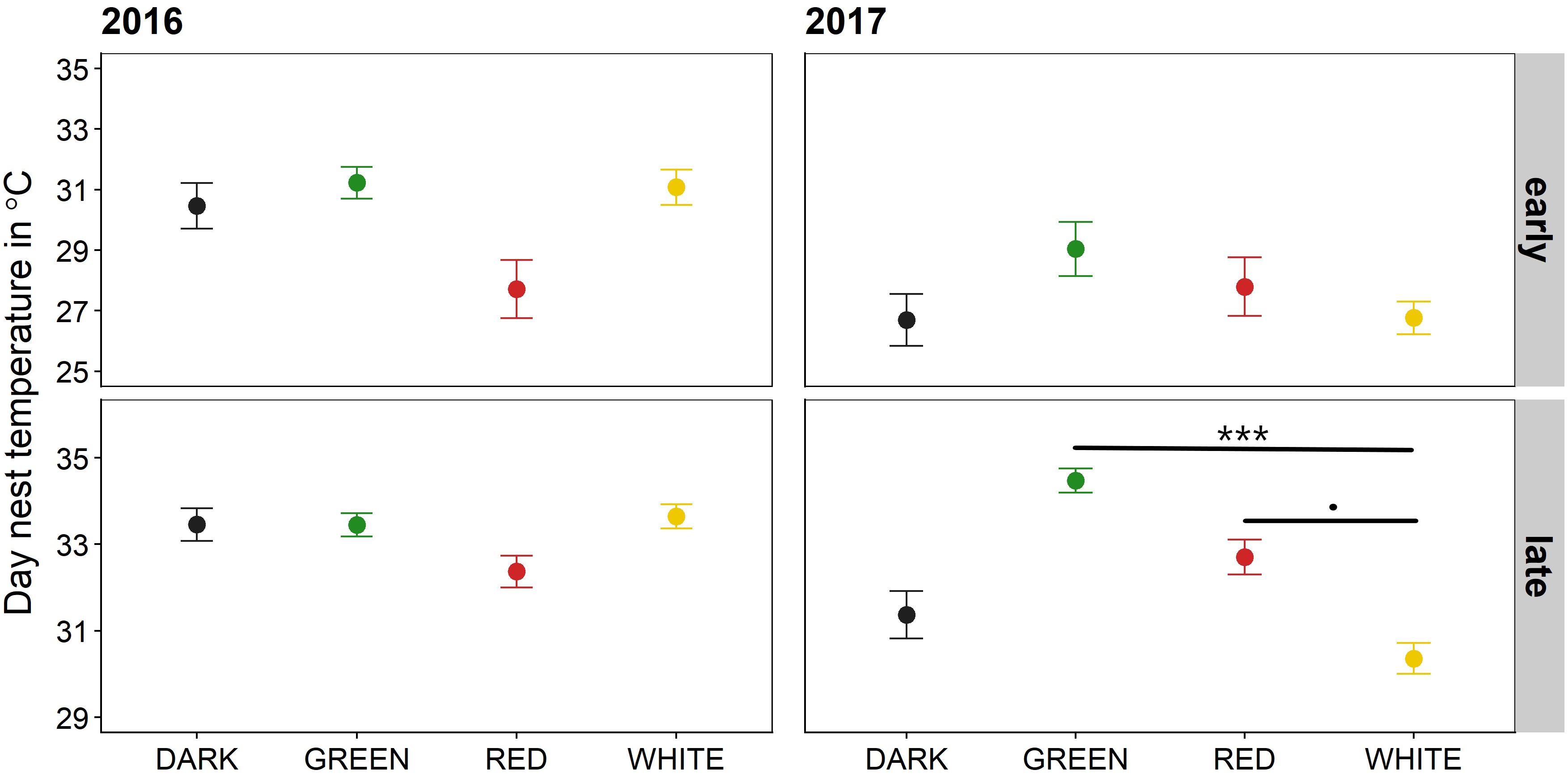
Figure 2. Average day nest temperature in early and late incubation. Raw nest temperature means +-SE are depicted for each artificial light treatment (2016: dark and green N = 14, red N = 17, white N = 16; 2017: dark N = 11, green N = 10, red N = 8, white N = 13 nest boxes). Treatments only differed from each other in the breeding season of 2017 late in incubation (bottom right panel): the average day nest temperature was significantly lower in in the white treatment compared to the green treatment (estimate = −5.04°C, t.ratio = −4.48, P < 0.001), with a trend in the same direction compared to the red treatment (estimate = −3.47°C, t.ratio = −3.00, P = 0.06). The same results were found for mean night nest temperatures (Supplementary Figure 6 and Supplementary Table 2). ***P < 0.001, ∘P < 0.10.
Off-Bout Frequency and Duration
Artificial light at night only affected mean off-bout frequency in 2017 (Figure 3 and Supplementary Table 4): birds in the white light treatment took significantly fewer off-bouts compared to the green treatment [estimate = −2.12, z(Inf) = −2.90, P = 0.04], with a similar trend compared to the red treatment [estimate = −2, z(Inf) = −2.59, P = 0.08], but not compared to the birds in the dark [estimate = −0.27, z(Inf) = −0.38, P = 1.00]. Although off-bout frequency increased over the course of incubation (P < 0.001), the slope was not modulated by light treatment (interaction terms P > 0.05 dropped from final model, model fit R2c delta = 0.40).
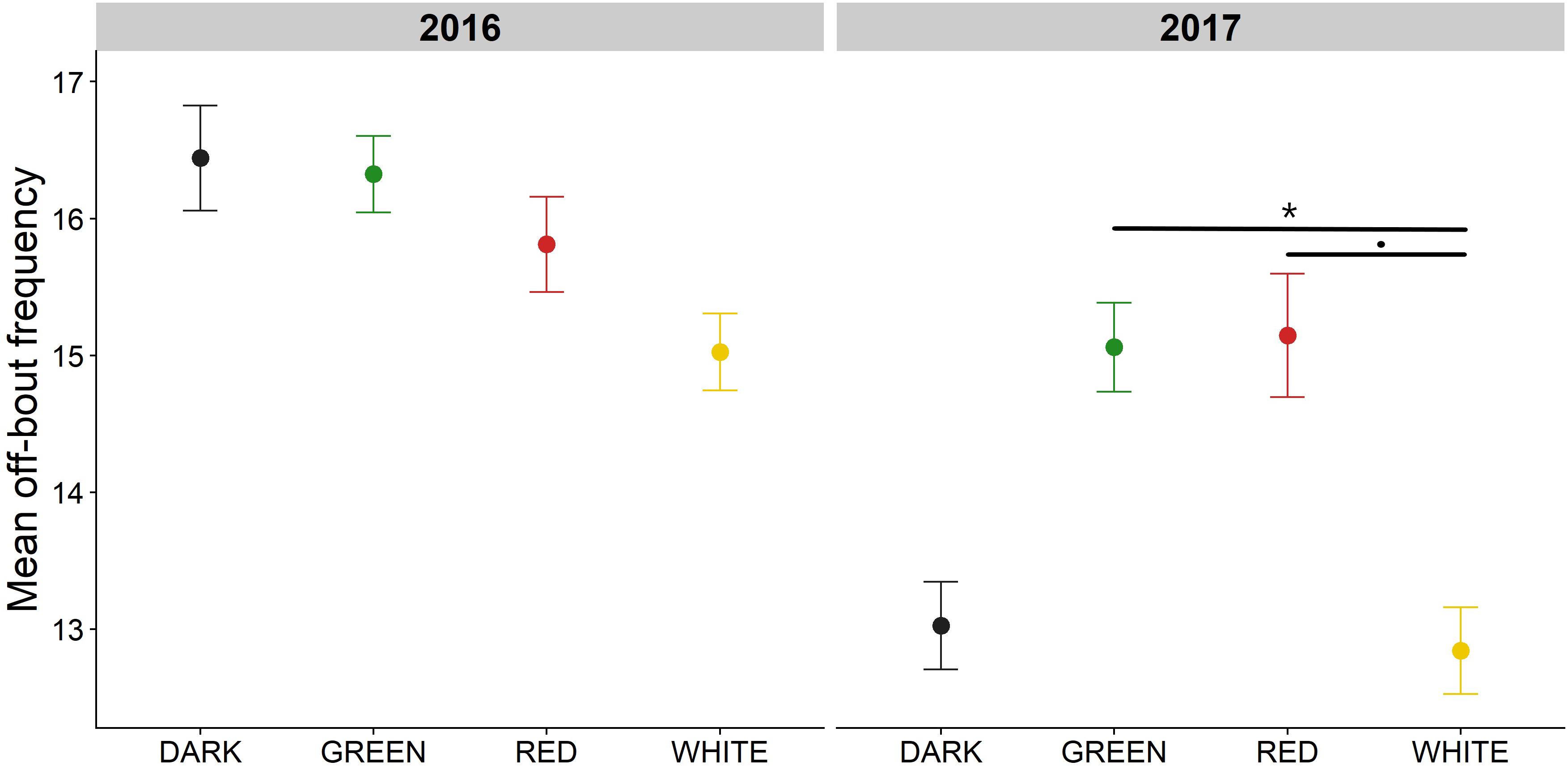
Figure 3. Mean off-bout frequency (number of off-bouts per incubation day). Raw means +-SE are depicted for each artificial light treatment in two breeding seasons. Treatments only differed from each other in the breeding season of 2017 (right panel): females in the white treatment took significantly fewer off-bouts compared to the green treatment (estimate = −2.1, z.ratio = −2.90, P = 0.04), with a trend in the same direction compared to the red treatment (estimate = −2, z.ratio = −2.59, P = 0.08). The effect was independent of incubation period. *P < 0.05, ∘P < 0.10.
Off-bout duration decreased over the course of incubation and was significantly affected by ALAN with the effects depending on light color and incubation day (Figure 4, interaction terms P < 0.001, model fit R2c = 0.85, Supplementary Table 5). A broken stick analysis indicated that birds reached their minimum mean off-bout duration 9 days before hatching of the eggs (break point = 9.43). When comparing treatments in early and late incubation, we found that 2016 birds breeding in the green light took significantly shorter off-bouts compared to the red treatment in early incubation [estimate = −6.52 min, t(77) = −3.42, P = 0.02]. However, these differences disappeared toward the end of incubation. This is in contrast to 2017, where birds in the green light took significantly shorter off-bouts compared to birds in the white light only late in the incubation period [estimate = −2.63 min, t(77) = −3.65, P = 0.009]. Mean off-bout frequency correlated non-linearly to off-bout duration [Supplementary Figure 8, Generalized Additive Model (GAM) effective degrees of freedom (edf) = 7.85, F(9) = 387.6, P < 0.001]: birds that took very few off-bouts, tended to take very long off-bouts, while birds that took more off-bouts only stayed away from the nest for a short time. Mean off-bout frequency was also non-linearly correlated to mean absolute temperature [Supplementary Figure 9, GAM edf = 4.87, F(9) = 90.53, P < 0.001], with an increase in off-bout frequency relating to an increase in mean day nest temperature, but with diminishing returns.
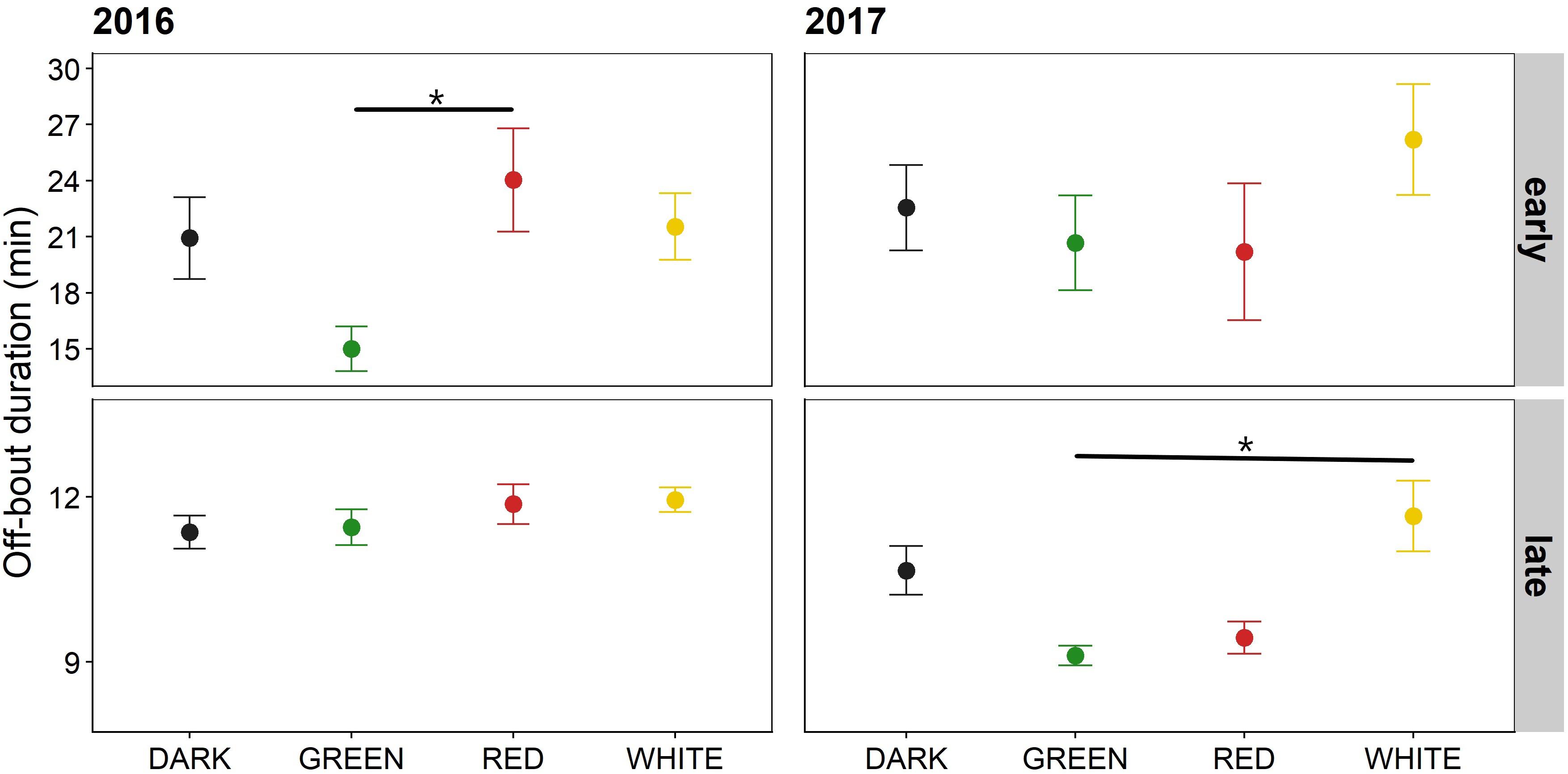
Figure 4. Mean off-bout duration in early and late incubation. Raw means for off-bout duration in minutes ± SE are depicted for each treatment. In the breeding season of 2016, females in the green light treatment had significantly shorter off-bouts compared to the red treatment (top left panel; estimate = −6.52 min, t.ratio = −3.43, P = 0.02). While this effect disappeared in late incubation in 2016, it became more pronounced in 2017 (bottom right panel). Specifically, in late incubation females had significantly shorter off-bouts in the green treatment compared to the white treatment (estimate = −2.63 min, t.ratio = −3.65, P = 0.009). *P < 0.05.
Fitness Effects
Length of the incubation period was not affected by light at night [F(7) = 0.78, P = 0.60] nor was it related to mean night nest temperature [F(1) = 1.37, P = 0.24], but it was related to off-bout frequency [F(1) = 5.47, P = 0.02, Supplementary Tables 6, 7). Incubation length and off-bout frequency were negatively correlated (Figure 5, estimate = −0.19 days, SE = 0.12, Supplementary Table 6): eggs from birds that took on average more off-bouts hatched after a shorter period of incubation. Most of the variance in length of the incubation period, however, remained unexplained (model fit R2c = 0.31).
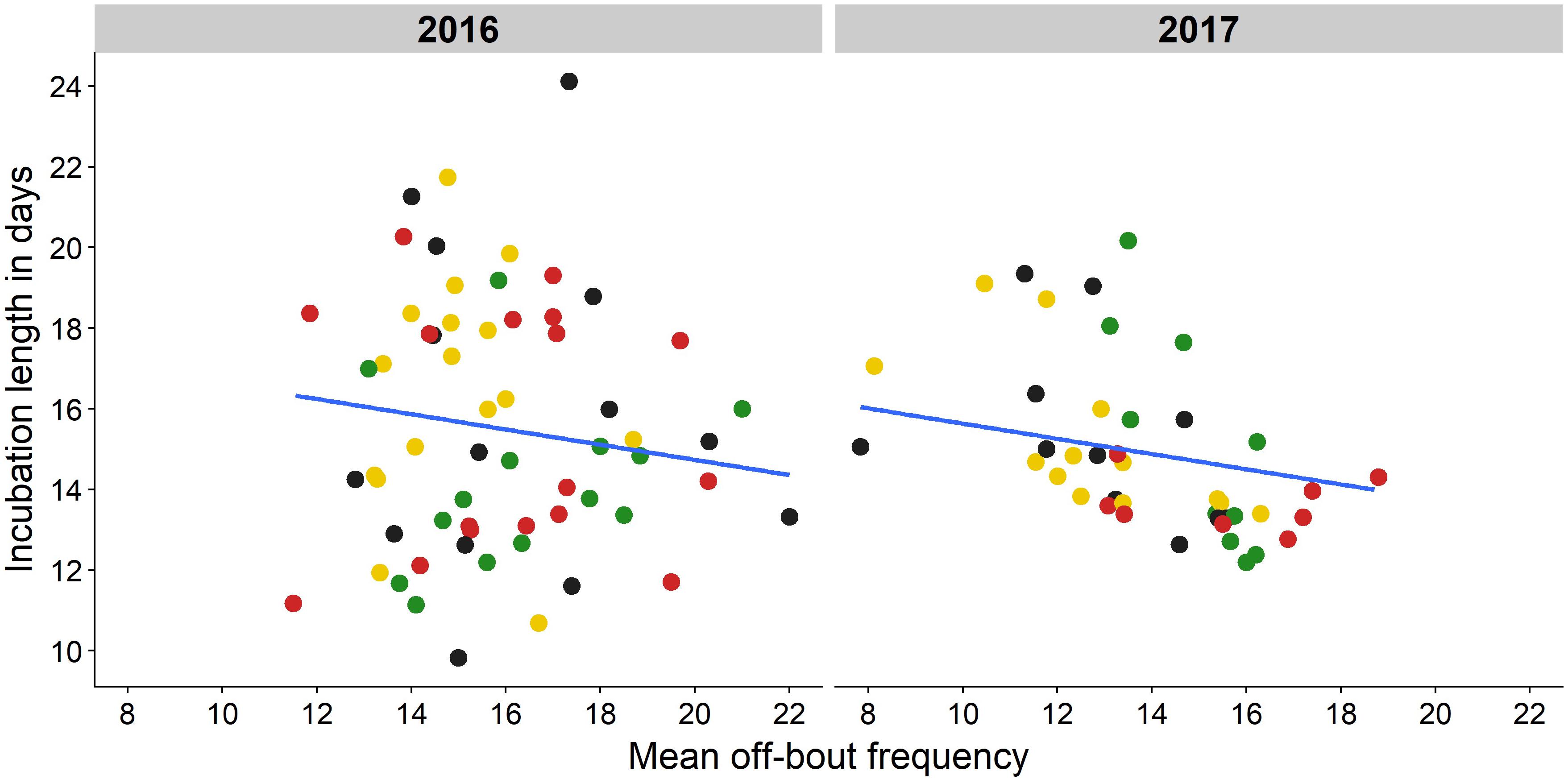
Figure 5. Relation between off-bout frequency and incubation length in days. Mean off-bout frequency during the incubation period is shown for each monitored nest in relation to incubation length in days (green: green light, red: red light, yellow: white light, black: no light). The line shows the significant negative relationship as predicted by a linear mixed model (estimate = −0.19 days, SE = 0.12, F = 5.47, P = 0.02), with a shorter incubation length when females took on average more off-bouts on incubation days. Artificial light treatment did not affect incubation length (P = 0.60) nor was it affected by average night nest temperature (estimate = −0.12 days, SE = 0.09, F = 1.37, P = 0.24, Supplementary Tables 6, 7).
We did not find any effects of incubation effort on number of hatchlings (Figure 6, mean night temp: P = 0.51, off-bout frequency: P = 0.60, model fit R2c = 0.59, Supplementary Tables 8–10), on chick hatching weight (mean night temp: P = 0.92, off-bout frequency: P = 0.86, incubation length: P = 0.71, model fit R2c = 0.10, Supplementary Table 11), nor on variation in chick hatching weight (mean night temp: P = 0.63, off-bout frequency: P = 0.61, incubation length: P = 0.13, model fit R2c = 0.45, Supplementary Table 12). Light at night did not affect chick hatching weight [F(3) = 0.84, P = 0.48), while we did find treatment effects on variation in chick hatching weight [F(3) = 3.08, P = 0.04, Supplementary Tables 12, 13): in 2016, nests from green light transects showed significantly less within-nest variation in chick hatching weight compared to nests from red light transects [estimate = −0.29, t(35) = −2.99, P = 0.02, Supplementary Table 14].
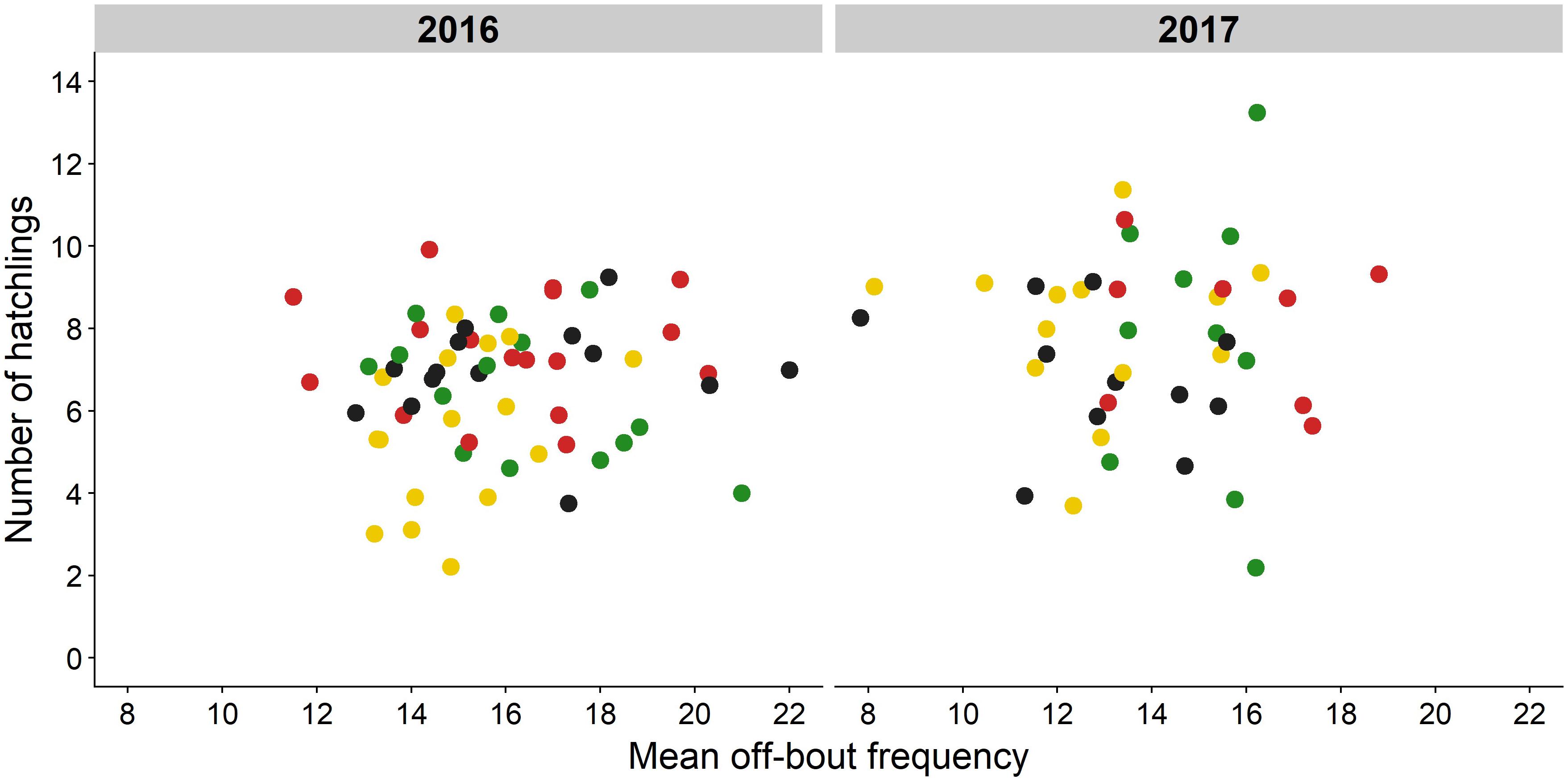
Figure 6. No relation between off-bout frequency and number of hatchlings. Mean off-bout frequency during the incubation period is shown for each monitored nest in relation to number of hatchlings (green: green light, red: red light, yellow: white light, black: no light). Number of hatchlings could not be explained by off-bout frequency, average night nest temperature, or incubation length (P > 0.05, Supplementary Table 8).
In 2016, birds in white light had significantly less hatchlings compared to the red light treatment [estimate = −1.51, t(95) = −3.52, P = 0.03], with a similar trend compared to the dark [t(95) = −1.39, P = 0.10, Supplementary Table 10]. However, this was only the case when considering the subset of nests for which we measured incubation behavior, since the effect disappeared when including all other nests for which we had hatchling data. An overview figure with the mean fitness measures per treatment can be found in (Supplementary Figure 10).
Discussion
Incubation largely determines the early developmental trajectory in birds (DuRant et al., 2013), but until now no studies have investigated how ALAN affects this key parental behavior. We investigated if ALAN of different spectra affects incubation behavior, and consequently how incubation behavior relates to fitness in two consecutive years. As expected, we found that white light affected incubation behavior of birds, but only when compared to birds in the green light in one of the two breeding seasons: these birds took fewer off-bouts, and late in incubation they took longer off-bouts and had lower nest temperatures. In our study, birds that took fewer off-bouts had a longer incubation length, but we did not find a relationship between incubation behavior and hatchling weight or hatchling number.
The observed lower incubation temperatures and longer off-bout durations in the white light treatment group in 2017 could indicate that these females had increased energy demands. This could be due to the effects of ALAN on body condition, as light pollution can affect immune responses in birds (Kernbach et al., 2018) and spectra combining long- and short-wavelengths can increase night-time activity and corticosterone levels in captive birds (Alaasam et al., 2018). Similarly, free-living birds nesting in white and close to red light have higher corticosterone levels (Ouyang et al., 2015). Another possibility is that male feeding is affected by white ALAN, for example because of sleep deprivation (Ouyang et al., 2017; Aulsebrook et al., 2020). Female blue tits that are fed more by males tend to have shorter off-bouts (Bambini et al., 2019). In our study, males subjected to white light might have fed females less leading to the observed longer off-bouts in this treatment group.
Contrary to our expectations, we only find differences for birds breeding in white light compared to green light, and to some extent compared to red light, but not compared to the dark control. Whereas birds in the green light had on average high incubation temperatures and took many short off-bouts already from the start of the incubation period, birds in the dark control areas had more intermediate incubation parameters in 2017. It might be that areas illuminated with green artificial light have become high quality habitats, as food abundance seems to be higher in green and white light areas at least during the chick feeding phase (Welbers et al., 2017). This could mean that foraging is more successful and leads to shorter off-bouts. However, a potential increase in energy demands in the white light, as discussed above, might counteract the positive effects of higher food abundance for birds breeding in the white light areas.
At our field sites, previously dark forest has been experimentally illuminated since 2012. In this setting it is not possible to control for settlement differences, although previous work did not find differences in breeding density between light treatments nor settlement preference for a particular light color in surviving birds (de Jong et al., 2015). When given the choice in the lab, wild birds preferred to sleep under green light as opposed to white light or darkness (Ulgezen et al., 2019). Thus, the differences we found between birds breeding in the white light compared to the green light could also be due to individual differences with high quality individuals nesting more in green lit areas as opposed to white lit areas.
Although we find some negative effects of white ALAN on incubation, this was only observed in one of the two breeding seasons. Such year-to-year variation in the effects of ALAN has been observed before (de Jong et al., 2015; Dominoni et al., 2020) and highlights the need for long-term monitoring (Spoelstra et al., 2015). When we compare the two monitored breeding seasons, the season of 2017 started earlier and the weather conditions during the incubation period were much colder compared to 2016. Cold weather conditions are thought to increase the energy demands of incubating females (Schöll et al., 2020), which perhaps aggravated the subtle effects of white ALAN, causing females in the white treatment group to have suboptimal incubation in this year. However, we will need more than 2 years of data to test if this is really the case.
Earlier in the season, off-bouts can result in greater incubation temperature drops (Bentzen et al., 2010), and the onset of full incubation tends to start later (Haftorn, 1981), indicating that long off-bouts could have been more stressful for developing embryos in 2017. Nevertheless, incubation behavior was not related to hatchling number in either year or to hatchling weight in 2016. This is in contrast with previous studies, which found decreased hatching success with lower incubation temperatures (Nord and Nilsson, 2011) and decreased embryo mass (Olson et al., 2006). However, in these studies eggs were incubated experimentally in the laboratory, which may have resulted in greater temperature differences between groups than those we observe in our wild bird populations. Moreover, small alterations in incubation can already affect offspring phenotype (DuRant et al., 2013), such as metabolic rate (Nord and Nilsson, 2011) and immune response (DuRant et al., 2012), which we did not measure here.
In both years, off-bout frequency was related to incubation length, with a shorter incubation period when birds took many off-bouts. In our study, birds that took many off-bouts tended to take shorter off-bouts, which keeps incubation temperatures more stable (Cooper and Voss, 2013), and could reduce the incubation period (Olson et al., 2006). For parents, a longer incubation length is undesirable as it is related to reduced adult survival, immunity, and future reproductive success, as well as increased risk of predation (reviewed in DuRant et al., 2013). Although we did not find significant differences in incubation length between the light treatments, birds in the white light took fewer off-bouts that were longer in 2017 compared to birds breeding in the green light. Future studies should investigate if this can lead to reduced adult survival and future reproduction for birds breeding under white light.
Our study finds spectrum dependent effects of ALAN on avian incubation behavior. The effects we found were subtle, did not affect offspring fitness in terms of hatchling number and weight, and were only observed in a cold, early season. This highlights the need for long-term monitoring to further map the conditions under which light pollution is harmful to breeding birds, to be able to successfully mitigate such effects. The subtlety of the effects indicates that future studies should investigate not only direct fitness consequences such as chick survival, but also investigate offspring phenotype and offspring recruitment. The fact that we only observe effects in a cold but not a warmer spring could indicate that ALAN at best becomes harmful when more stressors are present, which is an important consideration in urban environments. Moreover, we find effects on incubation behavior of cavity breeders, which are only exposed to very low levels of ALAN. This suggests that the potential effects of ALAN on open-cup breeders might be much stronger, but this is currently an overlooked research area.
Data Availability Statement
The datasets and scripts used in the final analysis in this study can be found in Dryad (van Dis et al., 2021) at https://doi.org/10.5061/dryad.73n5tb2xq.
Ethics Statement
The animal study was reviewed and approved by the Animal Experimentation Committee KNAW, with the protocol number “NIOO 14.05 addendum2”.
Author Contributions
ND, KS, MV, and DD designed the study. ND and DD collected the data. ND and KS analyzed the data. ND wrote the manuscript. All authors read, commented on, and approved the final version of the manuscript.
Funding
This research was supported by the Dutch Technology Foundation STW, which was part of the Netherlands Organization for Scientific Research (NWO) and which was partly funded by the Ministry of Economic Affairs. The project was supported by the Philips and the Nederlandse Aardolie Maatschappij (NAM). Additional funding was obtained by an NWO Open Competition grant (“Aging in the light,” 260-25310) to DD and MV.
Conflict of Interest
The authors declare that the research was conducted in the absence of any commercial or financial relationships that could be construed as a potential conflict of interest.
Publisher’s Note
All claims expressed in this article are solely those of the authors and do not necessarily represent those of their affiliated organizations, or those of the publisher, the editors and the reviewers. Any product that may be evaluated in this article, or claim that may be made by its manufacturer, is not guaranteed or endorsed by the publisher.
Acknowledgments
We thank Dutch nature conservation organizations and terrain owners for allowing us to test the effects of experimental artificial lighting on their terrain: Staatsbosbeheer, Natuurmonumenten, the Dutch Ministry of Defence, Het Drentse Landschap, and the Municipality of Ede. We also thank Anouk Welbers, Anne Dijkzeul, Jamie Kalla, and Chiel Boom for their assistance in the field and Hugo Loning and Pablo Capilla-Lasheras for their help with scripting in R. We thank the two reviewers for their constructive comments to improve the manuscript.
Supplementary Material
The Supplementary Material for this article can be found online at: https://www.frontiersin.org/articles/10.3389/fevo.2021.728377/full#supplementary-material
References
Alaasam, V. J., Duncan, R., Casagrande, S., Davies, S., Sidher, A., Seymoure, B., et al. (2018). Light at night disrupts nocturnal rest and elevates glucocorticoids at cool color temperatures. J. Exp. Zool. Part A 329, 465–472. doi: 10.1002/jez.2168
Aulsebrook, A. E., Connelly, F., Johnsson, R. D., Jones, T. M., Mulder, R. A., Hall, M. L., et al. (2020). White and amber light at night disrupt sleep physiology in birds. Curr. Biol. 30, 3657–3663.e5. doi: 10.1016/j.cub.2020.06.085
Bambini, G., Schlicht, E., and Kempenaers, B. (2019). Patterns of female nest attendance and male feeding throughout the incubation period in Blue Tits Cyanistes caeruleus. IBIS 161, 50–65. doi: 10.1111/ibi.12614
Bates, D., Maechler, M., Bolker, B., and Walker, S. (2015). Fitting linear mixed-effects models using lme4. J. Stat. Softw. 67, 1–48. doi: 10.18637/jss.v067.i01
Bentzen, R. L., Powell, A. N., Phillips, L. M., and Suydam, R. S. (2010). Incubation behavior of king eiders on the coastal plain of northern Alaska. Polar Biol. 33, 1075–1082. doi: 10.1007/s00300-010-0787-y
Cooper, C. B., and Voss, M. A. (2013). Avian incubation patterns reflect temporal changes in developing clutches. PLoS One 8:e65521. doi: 10.1371/journal.pone.0065521
de Jong, M., Caro, S. P., Gienapp, P., Spoelstra, K., and Visser, M. E. (2017). Early birds by light at night: effects of light color and intensity on daily activity patterns in blue tits. J. Biol. Rhythms 32, 323–333. doi: 10.1177/0748730417719168
de Jong, M., Ouyang, J. Q., Da Silva, A., Grunsven, R. H. A., Van, Kempenaers, B., et al. (2015). Effects of nocturnal illumination on life-history decisions and fitness in two wild songbird species. Philos. Trans. R. Soc. Lond. B Biol. Sci. 370, 1–8. doi: 10.1098/rstb.2014.0128
De Kogel, C. H. (1997). Long-term effects of brood size manipulation on morphological development and sex-specific mortality of offspring. J. Anim. Ecol. 66:167. doi: 10.2307/6019
Dominoni, D. M., Kjellberg Jensen, J., Jong, M., Visser, M. E., and Spoelstra, K. (2020). Artificial light at night, in interaction with spring temperature, modulates timing of reproduction in a passerine bird. Ecol. Appl. 30, e2062. doi: 10.1002/eap.2062
Dunlap, J. C. (1999). Molecular bases for circadian clocks. Cell 96, 271–290. doi: 10.1016/S0092-8674(00)80566-8
DuRant, S. E., Hopkins, W. A., Hawley, D. M., and Hepp, G. R. (2012). Incubation temperature affects multiple measures of immunocompetence in young wood ducks (Aix Sponsa). Biol. Lett. 8, 108–111. doi: 10.1098/rsbl.2011.0735
DuRant, S. E., Hopkins, W. A., Hepp, G. R., and Walters, J. R. (2013). Ecological, evolutionary, and conservation implications of incubation temperature-dependent phenotypes in birds. Biol. Rev. 88, 499–509. doi: 10.1111/brv.12015
Falcón, J., Torriglia, A., Attia, D., Viénot, F., Gronfier, C., Behar-Cohen, F., et al. (2020). Exposure to artificial light at night and the consequences for flora, fauna, and ecosystems. Front. Neurosci. 14:602796. doi: 10.3389/fnins.2020.602796
Gaston, K. J., Visser, M. E., and Hölker, F. (2015). The biological impacts of artificial light at night: the research challenge. Philos. Trans. B 370:20140133. doi: 10.1098/rstb.2014.0133
Greeney, H. F. (2009). Unusual incubation rhythms the Spotted Barbtail, Premnoplex brunnescens. J. Ornithol. 150, 529–535. doi: 10.1007/s10336-009-0372-8
Grubisic, M., Haim, A., Bhusal, P., Dominoni, D. M., Gabriel, K. M. A., Jechow, A., et al. (2019). Light pollution, circadian photoreception, and melatonin in vertebrates. Sustainability 11:6400. doi: 10.3390/su11226400
Haftorn, S. (1981). Incubation during the egg-laying period in relation to clutch-size and other aspects of reproduction in the great tit parus major. Ornis Scand. 12, 169–185.
Hölker, F., Wolter, C., Perkin, E. K., and Tockner, K. (2010). Light pollution as a biodiversity threat. Trends Ecol. Evol. 25, 681–682. doi: 10.1016/j.tree.2010.09.007
Hunt, D. M., Carvalho, L. S., Cowing, J. A., and Davies, W. L. (2009). Evolution and spectral tuning of visual pigments in birds and mammals. Philos. Trans. R. Soc. B 364, 2941–2955. doi: 10.1098/rstb.2009.0044
Kernbach, M. E., Miller, J. M., Hall, R. J., Unnasch, T. R., Burkett-Cadena, N. D., and Martin, L. B. (2018). Light pollution increases west nile virus competence in a ubiquitous passerine reservoir species. BioRxiv [Preprint] doi: 10.1101/269209
Lenth, R. (2020). emmeans: Estimated Marginal Means, aka Least-Squares Means. R package version 1.5.1. Available online at: https://cran.r-project.org/package=emmeans (accessed October 23, 2020).
Nord, A., and Nilsson, J. -Å (2011). Incubation temperature affects growth and energy metabolism in blue tit nestlings. Am. Nat. 178, 639–651. doi: 10.1086/662172
Olson, C. R., Vleck, C. M., and Vleck, D. (2006). Periodic cooling of bird eggs reduces embryonic growth efficiency. Physiol. Biochem. Zool. 79, 927–936.
Ouyang, J. Q., de Jong, M., Hau, M., Visser, M. E., van Grunsven, R. H. A., and Spoelstra, K. (2015). Stressful colours: corticosterone concentrations in a free-living songbird vary with the spectral composition of experimental illumination. Biol. Lett. 11:20150517. doi: 10.1098/rsbl.2015.0517
Ouyang, J. Q., de Jong, M., van Grunsven, R. H. A., Matson, K. D., Haussmann, M. F., Meerlo, P., et al. (2017). Restless roosts: light pollution affects behavior, sleep, and physiology in a free-living songbird. Glob. Change Biol. 23, 4987–4994. doi: 10.1111/gcb.13756
Pinheiro, J., Bates, D., DebRoy, S., Sarkar, D., and Team, R. C. (2021). nlme: Linear and Nonlinear Mixed Effects Models. R Package Version 3.1-152. Available online at: from https://cran.r-project.org/package=nlme (accessed February 26, 2021).
Proudman, J. A., and Opel, H. (1981). Turkey prolactin: validation of a radioimmunoassay and measurement of changes associated with broodiness. Biol. Reprod. 25, 573–580.
R Core Team (2020). R: A Language And Environment For Statistical Computing. Available online at: https://www.r-project.org/ (accessed October 23, 2020).
Raap, T., Casasole, G., Costantini, D., AbdElgawad, H., Asard, H., Pinxten, R., et al. (2016a). Artificial light at night affects body mass but not oxidative status in free-living nestling songbirds: an experimental study. Sci. Rep. 6:35626. doi: 10.1038/srep35626
Raap, T., Casasole, G., Pinxten, R., and Eens, M. (2016b). Early life exposure to artificial light at night affects the physiological condition: an experimental study on the ecophysiology of free-living nestling songbirds. Environ. Pollut. 218, 909–914. doi: 10.1016/j.envpol.2016.08.024
Raap, T., Pinxten, R., and Eens, M. (2018). Cavities shield birds from effects of artificial light at night on sleep. J. Exp. Zool. Part A 329, 449–456. doi: 10.1002/jez.2174
Rani, S., and Kumar, V. (2000). Phasic response of the photoperiodic clock to wavelength and intensity of light in the redheaded bunting, Emberiza bruniceps. Physiol. Behav. 69, 277–283. doi: 10.1016/S0031-9384(99)00247-4
Saini, C., Hutton, P., Gao, S., Simpson, R. K., Giraudeau, M., Sepp, T., et al. (2019). Exposure to artificial light at night increases innate immune activity during development in a precocial bird. Comp. Biochem. Physiol. Part A 233, 84–88. doi: 10.1016/j.cbpa.2019.04.002
Saino, N., Romano, M., Ferrari, R. P., Martinelli, R., and Møller, A. P. (2005). Stressed mothers lay eggs with high corticosterone levels which produce low-quality offspring. J. Exp. Zool. Part A 303, 998–1006. doi: 10.1002/jez.a.224
Schöll, E. M., Aparisi, M. P., and Hille, S. M. (2020). Diurnal patterns of ambient temperature but not precipitation influence incubation behavior in Great Tits. J. Ornithol. 161, 529–538. doi: 10.1007/s10336-019-01737-9
Spoelstra, K., van Grunsven, R. H. A., Donners, M., Gienapp, P., Huigens, M. E., Slaterus, R., et al. (2015). Experimental illumination of natural habitat–an experimental set-up to assess the direct and indirect ecological consequences of artificial light of different spectral composition. Philos. Trans. R. Soc. B 370:20140129. doi: 10.1098/rstb.2014.0129
Titulaer, M., Spoelstra, K., Lange, C. Y. M. J. G., and Visser, M. E. (2012). Activity patterns during food provisioning are affected by artificial light in free living great tits (Parus major). PLoS One 7:e37377. doi: 10.1371/journal.pone.0037377
Ulgezen, Z. N., Käpylä, T., Meerlo, P., Spoelstra, K., Visser, M. E., and Dominoni, D. M. (2019). The preference and costs of sleeping under light at night in forest and urban great tits. Proc. R. Soc. B 286:20190872. doi: 10.1098/rspb.2019.0872
Van Balen, H. (1973). A comparative study of the breeding ecology of the great tit Parus major in different habitats. Ardea 61, 1–93.
van Dis, N. E., Spoelstra, K., Visser, M. E., and Dominoni, D. M. (2021). Color of artificial light at night affects incubation behavior in the great tit, Parus major. Dryad, Dataset doi: 10.5061/dryad.73n5tb2xq
van Oers, K., Kohn, G., Hinde, C., and Naguib, M. (2015). Parental food provisioning is related to nestling stress response in wild great tit nestlings: implications for the development of personality. Front. Zool. 12(Suppl 1):S10. doi: 10.1186/1742-9994-12-S1-S10
Keywords: light pollution, incubation, spectral composition, Parus major, fitness, ALAN
Citation: van Dis NE, Spoelstra K, Visser ME and Dominoni DM (2021) Color of Artificial Light at Night Affects Incubation Behavior in the Great Tit, Parus major. Front. Ecol. Evol. 9:728377. doi: 10.3389/fevo.2021.728377
Received: 21 June 2021; Accepted: 21 September 2021;
Published: 11 October 2021.
Edited by:
Ann Valerie Hedrick, University of California, Davis, United StatesReviewed by:
Thomas Wassmer, Siena Heights University, United StatesSydney Frances Hope, UMR 7372 Centre d’Études Biologiques de Chizé (CEBC), France
Copyright © 2021 van Dis, Spoelstra, Visser and Dominoni. This is an open-access article distributed under the terms of the Creative Commons Attribution License (CC BY). The use, distribution or reproduction in other forums is permitted, provided the original author(s) and the copyright owner(s) are credited and that the original publication in this journal is cited, in accordance with accepted academic practice. No use, distribution or reproduction is permitted which does not comply with these terms.
*Correspondence: Natalie E. van Dis, n.vandis@nioo.knaw.nl; orcid.org/0000-0002-9934-6751