- 1Institute of Evolutionary Ecology and Conservation Genomics, Ulm University, Ulm, Germany
- 2Department of Biosciences, Paris Lodron University Salzburg, Salzburg, Austria
- 3Department of Evolution and Ecology, University of California, Davis, Davis, CA, United States
- 4Division of Structural and Functional Botany, University of Vienna, Vienna, Austria
- 5Departamento de Botânica, Universidade Federal de Pernambuco, Recife, Brazil
- 6Departamento de Química Fundamental, Universidade Federal de Pernambuco, Recife, Brazil
- 7Institute of Organic Chemistry, University of Regensburg, Regensburg, Germany
- 8Laboratório de Ecologia Ciências Biológicas, Universidade de Pernambuco, Petrolina, Brazil
Male euglossine bees exhibit unique adaptations for the acquisition and accumulation of chemical compounds from “perfume flowers” and other sources. During courtship display, male bees expose perfume mixtures, presumably to convey species-specific recognition and/or mate choice signals to females. Because olfaction regulates both signal production (in males) and signal detection (in females) in this communication system, strong selective pressures are expected to act on the olfactory system, which could lead to sensory specialization in favor of an increased sensitivity to specific chemical compounds. The floral scents of euglossine-pollinated plants are hypothesized to have evolved in response to the preexisting sensory biases of their male euglossine bee pollinators. However, this has never been investigated at the peripheral olfactory circuitry of distinct pollinating genera. Here, we present a comparative analysis using electroantennography (EAG) of males across the phylogeny of 29 euglossine bee species, among them Euglossa and Eulaema species. First, we tested whether antennal responses differ among different euglossine genera, subgenera and species. Secondly, we conducted a comparative phylogenetic analysis to investigate the macroevolutionary patterns of antennal responses across the euglossine bee phylogeny. We found that antennal response profiles are very unique on the species level and differ on the subgenus and the genus level. The differences can be explained by chemical compounds typically found in the floral scent bouquets of perfume flowers and specific compounds of species either pollinated by Euglossa (e.g., ipsdienol) or Eulaema bees (e.g., (−)-(E)-carvone epoxide). Also, we detected a phylogenetic signal in mean antennal responses and found that especially at the species level of our simulation the overall antennal responses exhibit greater disparity relative to a null model of pure Brownian-motion across the phylogeny. Altogether, our results suggest that (1) euglossine bee species exhibit species-specific antennal responses that differ among euglossine genera and subgenera, (2) antennal responses diverge early after speciation events, and (3) scent composition of perfume flowers evolved in response to pollinator-mediated selection imposed by preexisting sensory biases in euglossine bees.
Introduction
For most insects, just like for the majority of animals across phyla, the ability to detect a diversity of airborne molecules in their environment is critically important for survival (Hildebrand and Shepherd, 1997; Hansson and Stensmyr, 2011). Olfaction plays a pivotal role in the detection of food, hosts, predators, and kin (Olsson and Hansson, 2013), as well as in the attraction, location and identification of potential mates (Birch and Haynes, 1982; Cardé and Baker, 1984; Roelofs, 1984; Ayasse et al., 2001). The importance of olfaction in insects is apparent by looking at the elaborate antennal structures that exist in a diversity of shapes (Hansson and Stensmyr, 2011). Insect antennae are covered with different types of olfactory sensilla (Schneider and Steinbrecht, 1968), which contain the sensitive dendrites of the olfactory sensory neurons (Zacharuk, 1980; Couto et al., 2005). Olfactory stimulation occurs when odor molecules enter through pores or slits on the antenna surface (Steinbrecht, 1997) and are directed by odorant binding proteins (OBPs) that bind together with the volatile to olfactory receptors (ORs) situated in the membrane of these dendrites (Hallem and Carlson, 2006; Robertson and Wanner, 2006). These olfactory receptors vary in the type of molecules that activate them, their chemical tuning spectrum and the molecular receptive ranges (Hallem and Carlson, 2006; Getahun et al., 2013). Therefore, the olfactory periphery plays an important role in compound discrimination and represents the first step of specificity in olfactory sensitivity (Shields and Hildebrand, 2001; Hallem and Carlson, 2006; Brand et al., 2015) prior higher-level neural processing in the insects brain (see e.g., Renou, 2014).
Specificity in olfactory signals can be achieved either through complex molecules that are rare in nature (Chow and Wang, 1981; Ayasse et al., 2003; Schäffler et al., 2015) or by specific blends of relatively simple and ubiquitous compounds (Knudsen et al., 2006; Ayasse et al., 2011; Ayasse and Dötterl, 2014). While most insects synthesize such specific olfactory signals (e.g., pheromones) de novo or modify precursors found in their diet (Roelofs, 1984), male euglossine bees (Apidae, Euglossini) are known to harvest volatile compounds directly from flowers (Vogel, 1966; Dodson et al., 1969) as well as from non-floral sources (e.g., rotting plant material, bark, leaves and feces; Whitten et al., 1993). A set of morphological, biochemical and behavioral adaptations thereby enable the location, collection and storage of volatile compounds (Eltz et al., 2005b) forming complex species-specific blends that are stored in tibial organs on the hindlegs and exposed by male euglossine bees during courtship in the forest understory (Eltz et al., 2005a,b). The blends are presumedly used to communicate species affiliation (Eltz et al., 2006; Zimmermann et al., 2006) and/or to demonstrate genetic fitness to conspecific females (Zimmermann et al., 2009b). However, the precise function of perfume blends in mediating mating decision by females awaits experimental support.
The perfume collection behavior of male euglossine bees has the unique feature that the olfactory system is involved in determining both signal production (i.e., the collection of volatile compounds) and signal detection (e.g., during mating) by female bees. Therefore, a strong selection pressure is expected to act on the olfactory system which could lead to sensory specialization in favor of an increased sensitivity to specific single volatiles or volatile blends in different species of euglossine bees (Eltz et al., 2006). In addition to the higher-level neural processing that takes place in the insect brain (see e.g., Renou, 2014), olfactory specialization can be achieved through changes in the peripheral sensory system, for example, by the presence/absence and abundance of specific types of ORs or by divergent chemical tuning of individual ORs (Hallem and Carlson, 2006; Renou, 2014). So far, more than 40 different chemical compounds are known to attract male euglossine bees (Williams and Whitten, 1983; Ramírez et al., 2002; Roubik and Hanson, 2004). Although there is a broad overlap in the range of compounds collected by different species, subgenera or genera of euglossine bees (Ackerman, 1983; Pearson and Dressler, 1985), several studies support a scenario of high species-specific preferences (Ackerman, 1989) as illustrated by the species-specific chemical blends stored in the hind-legs (Eltz et al., 2003, 2005a; Zimmermann et al., 2006; Weber et al., 2016).
This behavior evolved at least 38 million years ago (Engel, 1999; Ramírez et al., 2011) and various neotropical plants, mainly orchids, have adapted to attract male euglossine bees as pollinators by offering volatile compounds as floral reward (i.e., perfume-rewarding plants; Vogel, 1966; Dressler, 1982; Williams and Whitten, 1983; Ramírez et al., 2002). The mutualistic system between euglossine males and perfume-rewarding flowers involves diverse bee genera, which differ considerably in size/morphology, olfactory preferences and behavior (Dressler, 1982; Ramírez et al., 2002). Some of the plants pollinated by male euglossine bees attract many distinct species from all genera, irrespective of their body size (e.g., Anthurium spp. and Spathiphyllum spp.; Montalvo and Ackerman, 1986; Hentrich et al., 2010). However, mutualistic interactions can also be very specific if pollinator size is essential to ensure successful pollinarium removal and subsequent deposition. This is often the case in perfume-producing orchids (e.g., Dodson, 1962, 1978; Dressler, 1968; Meeuse and Morris, 1984).
The orchid genus Catasetum is mainly pollinated by species of Euglossa and Eulaema, but for a few species also pollination by Eufriesea (Hills et al., 1972; Peruquetti et al., 1999; Milet-Pinheiro et al., 2018) and Exaerete (Cancino and Damon, 2007) is reported. Species that are pollinated by Euglossa are usually visited by two or more congeneric pollinator species, but rarely by species of Eulaema, and vice versa (Frankie et al., 1983; Whitten et al., 1986, 1988). Chemical analysis of floral scents emitted by Catasetum orchids suggest that they differ among pollinator genera and subgenera (i.e., Eufriesea, Euglossa or Eulaema; Milet-Pinheiro and Gerlach, 2017; Brandt et al., 2019) but are also highly specific on the species level. Based on these findings, together with the fact that perfume as floral reward has evolved after perfume-gathering behavior, it has been hypothesized that preexisting sensory biases of each euglossine genus and the resulting behavioral preferences for distinct compounds among euglossine bees shaped the evolution of floral scent of perfume-rewarding plants (Ramírez et al., 2011). Experimental evidence for the possible influence of sensory biases on the evolution of floral scents of perfume-rewarding plants from the pollinator perspective, however, is missing. In the present study, we used electroantennography (EAG) to investigate, in a comparative approach, whether bees of the distinct genera Eufriesea, Euglossa, Eulaema, and Exaerete respond differently to chemical compounds that are most representative in the floral perfumes of euglossinophilous plants, particularly in the genus Catasetum (Milet-Pinheiro and Gerlach, 2017). We expect the antennal response profiles of euglossine species to differ among distinct genera thereby reflecting differences in the olfactory periphery of euglossine species that could have influenced the evolution of the floral scents in perfume flowers. Moreover, we conducted a comparative phylogenetic analysis to test whether antennal responses can be explained by bee phylogeny.
Materials and Methods
Tested Bee Species
In total, we tested the antennal sensitivity in males of all 29 euglossine bee species we were able to attract in the field, 19 occurring in Costa Rica and 10 in NE-Brazil, among them three species of Eufriesea (N = 12 individuals), 16 species of Euglossa (N = 154), eight species of Eulaema (N = 80), and two species of Exaerete (N = 16; Figure 1). The tested species of Eulaema belong to the subgenus Apeulaema and Eulaema s. st. (Nemésio, 2009; Melo, 2014; Table 1), whereas those of Euglossa belong to the subgenera Euglossa s. st., Glossura and Glossurella (Nemésio, 2009; Ramírez et al., 2010b; Table 1).
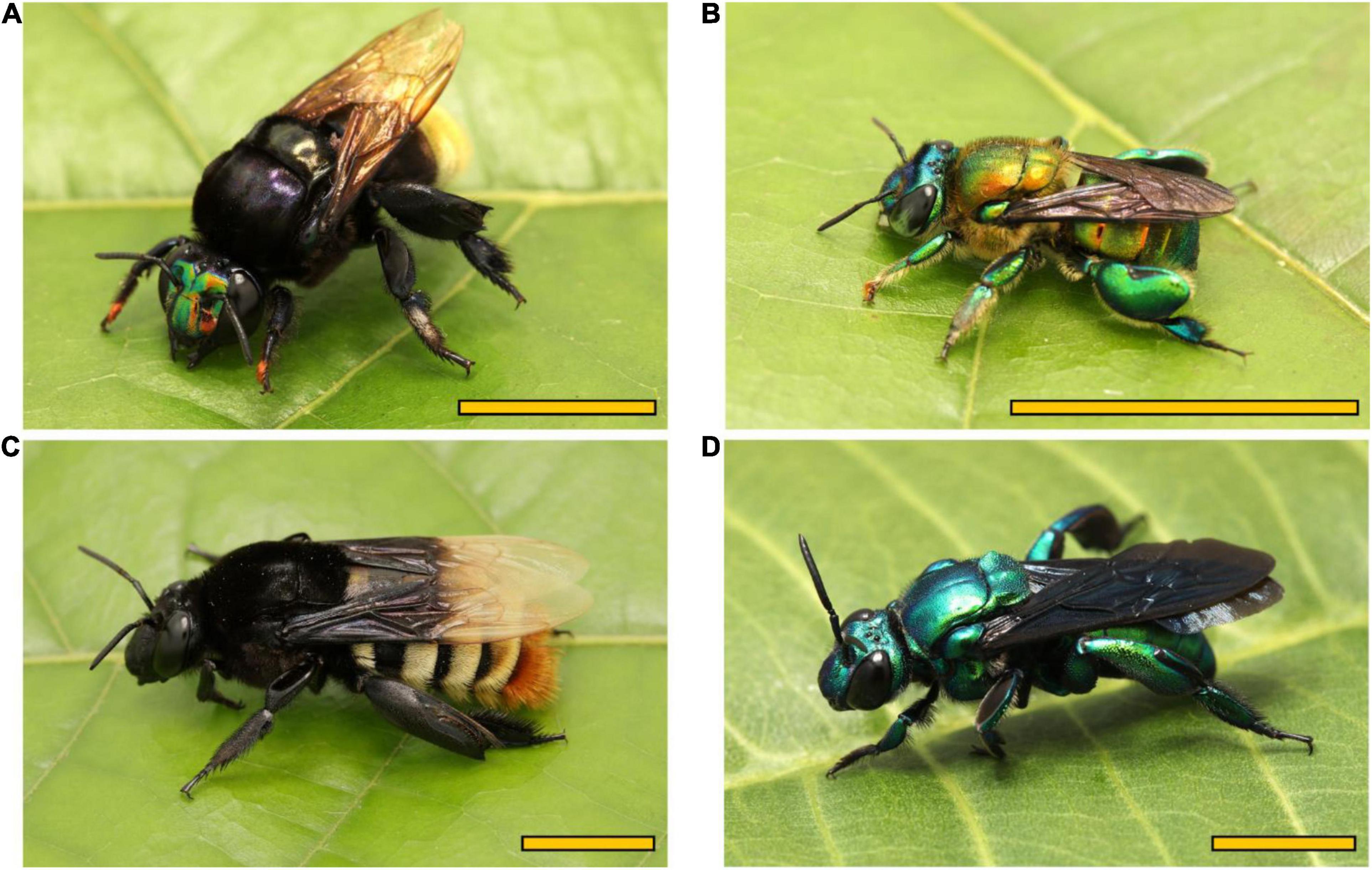
Figure 1. Overview of the four tested euglossine genera (A) Eufriesea, (B) Euglossa, (C) Eulaema, and (D) Exaerete. Scale bar: 1 cm. Photos by Paulo Milet-Pinheiro.
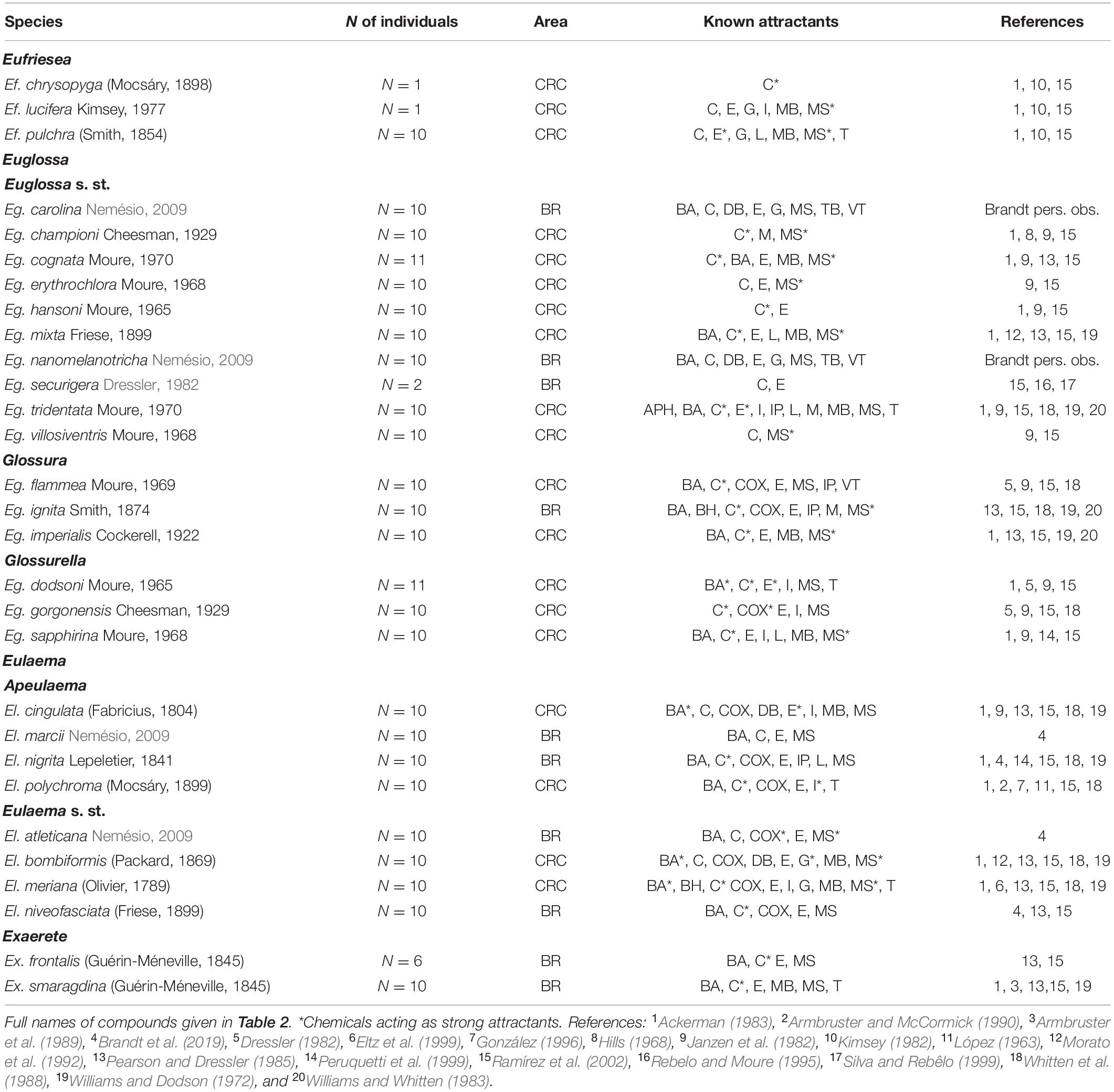
Table 1. Tested euglossine species of Brazil (BR) and Costa Rica (CRC) belonging to the genera Eufriesea, Euglossa (subgenera: Euglossa s. st., Glossura, and Glossurella), Eulaema (subgenera: Apeulaema and Eulaema s. st.), and Exaerete and known chemical compounds used in this study attracting male bees of these species.
In Costa Rica bees were collected at the surroundings of Piedras Blancas National Park (320 m a.s.l; 8°41′37.6″N 83°12′51.7″W) and the Tropical Field Station La Gamba (76 m a.s.l; 8°42′03.6″N 83°12′05.7″W). Sampling of bees in Costa Rica was authorized by the Ministerio de Ambiente y Energía Sistema Nacional de Áreas de Conservacíon (permit numbers SINAC-ACOSTA-PI-PC-001-19 and SINAC-ACOSTA-PI-PC-002-19). In Brazil, bees were either collected at the surroundings of the “Mata do Curado” (10 m a.s.l; 8°02′30.5″S, 34°57′54.1″W), municipality of Recife (Pernambuco), or at the surroundings of the farm “Agua Fria” (600 m a.s.l; 8°11′19.0″S, 35°28′13.6″W), located in the municipality of Chã-Grande (Pernambuco). Sampling of bees in Brazil was authorized by the Instituto Chico Mendes de Conservação da Biodiversidade (ICMBio) of the Ministério Brasileiro do Meio Ambiente (permit number 53545–1).
Bees were collect using entomological nets at scent baits (Gruber et al., 2008), i.e., filter papers (10 × 10 cm) impregnated with 100 μL of the following pure synthetic compounds: eucalyptol (99%; Merck), benzyl acetate (≥99%; Merck), eugenol (≥98%; Merck), methyl salicylate (≥99%; Merck), skatole (98%; Merck), veratrole (99%; Sigma-Aldrich). After analyses (see below), bees were mounted with entomological pins and deposited either at the collection of the Tropical Field Station La Gamba (Costa Rica) or at the UFPE (Brazil).
Electroantennographic Measurements (EAGs)
The physiological measurements were performed either at the facilities of the Tropical Field Station La Gamba or the Departamento de Química Fundamental (DQF) of the Universidade Federal of Pernambuco (UFPE). For the measurements, we used micro-scissors (Castroviejo, Fine Science tools; 69121 Heidelberg, Germany) to excise one antenna of each tested bee at the scape. Using a stereomicroscope (Stemi 2000-CS, ZEISS, Oberkochen, Germany) and a razor blade, the excised antenna was cut at the tip (last segment of flagellum) and at the base (first segment of flagellum). The antenna was mounted between two glass capillaries filled with insect Ringer solution (1 L demineralized water containing 5 g of NaCl, 0.42 g of KCl and 0.19 g of CaCl), which were connected to gold-electrodes. The electrode connected with the base of the antenna was grounded, while the electrode connected to the tip transmitted changes of the potential within the antenna to a signal acquisition controller (IDAC-2 Signal acquisition controller; Syntech, Hilversum, Netherlands). The preparation was placed in front of a glass tube, through which a constant humidified airflow (25 mL/s) was blown.
We tested the antennal sensitivity of the different species to compounds that are typically found in perfume-rewarding plants pollinated by different genera of euglossine bees. Based on a data set on floral scent chemistry of 60 euglossinophilous species (Milet-Pinheiro and Gerlach, 2017; Milet-Pinheiro, unpublished) we prepared testing solutions for 23 compounds (Table 2) in a concentration of 10 μL/mL using n-hexane as the solvent (Table 2). Testing solutions were applied to each antennal preparation in a randomized order using the Android App “Who’s Next?!” (v.0.8.0; Martin Philippi 2017) starting and ending with the negative control n-hexane. To avoid decreased antennal responses as a result of prolonged or repetitive stimulation (Strausfeld and Kaissling, 1986), we allowed a resting phase of 60 s between stimuli. For each stimulus, we added 5 μL of testing solution onto a v-shaped strip of filter paper (ca. 0.5 × 1 cm) located inside a Pasteur pipette (15 cm, VWR International, Darmstadt, Germany). After the solvent was allowed to evaporate for 1 min, the Pasteur pipettes were connected to a stimulus controller (CS-05; Syntech, Hilversum, Netherlands) that delivered an air-puff to the antenna for 0.3 s with a pulse flow of 25 ml/s. Antennal responses were analyzed by Syntech EAG software (EAG Pro, v. 2.2; Hilversum, Netherlands). Responses to n-hexane were used to normalize the data (using the option provided the software), and thus, to correct for a change in antennal sensitivity during measurements.
For the statistical analyses we used a different standardization of antennal responses to compare the different species and genera. The strongest antennal response of each tested bee individual was set as 100%, and the responses to all other stimuli were expressed as percentages in relation to this reference. To test for differences in these multivariate standardized antennal responses to the compounds (excluding the negative control) among genera, subgenera and species of euglossine bees, we used a multivariate three-level nested PERMANOVA analysis [factors: genus, subgenus (nested in genus), and species (nested in subgenus)] with subsequent pair-wise comparisons based on fourth-root transformed Bray Curtis similarities. Further, we used non-metric multidimensional scaling (nMDS; Clarke and Gorley, 2006), based on the Bray Curtis similarities, to graphically depict variation in antennal responses among genera, subgenera and species (species-means were used for analyses), and SIMPER was used to determine the compounds to which the genera responded most differently. We performed PERMDISP (factor: genus or subgenus) to test for differences in variability (dispersion) among antennal responses. Results of these analyses provided information about the variation of antennal responses per se and indicated potential influences of dispersion on the PERMANOVA results (see Anderson et al., 2008).
Absolute antennal responses were used to test, separately for each species and floral scent compound, whether responses were stronger than to the negative control, n-hexane. Therefore, we performed two-factorial PERMANOVA analyses [factors: bee individual and compound] with subsequent pair-wise comparisons (adjusted via Bonferroni correction) based on univariate (using single compounds) Euclidean distance matrices.
The PERMANOVA analyses were ran using the software PRIMER 6 (version 6.1.15; PRIMER-E Ltd., 2012) in combination with the add-on PERMANOVA + (version 1.0.5; PRIMER-E Ltd., 2012). We used (1) sums of squares type III (partial), (2) fixed effects sum to zero for mixed terms, (3) a permutation of residuals under a reduced model, and (4) 9,999 permutations for all analyses. The level of significance was defined at α ≤ 0.05.
Phylogenetic Analyses
In order to investigate the evolutionary patterns of antennal responses across the euglossine bee phylogeny, we used the species-level phylogenetic tree estimated by Ramírez et al. (2010b). Briefly, the species-level phylogeny was built using ∼ 4.0 kb of nuclear (EF1-a, ArgK, and Pol-II) and mitochondrial (CO1) DNA available for 26 of our 29 tested euglossine species (no data available for El. atleticana, El. Marcii, and El. niveofasciata). Phylogenetic tree searches and fossil calibrated molecular clock analyses were estimated as described in Ramírez et al. (2010b).
Comparative phylogenetic analyses were conducted in RStudio v.1.4.1103 (implemented R v.4.0.3) using the R packages “phytools” v.0.7-70 (Revell, 2012) and “geiger” v.2.0.7 (Pennell et al., 2014). For all phylogenetic analyses we used a Bray Curtis similarity matrix based on standardized mean antennal responses (in percent, see above). We computed a phylogenetic signal for continuous traits on multivariate antennal responses of tested euglossine species using Blomberg’s K-statistic test (Blomberg et al., 2003) based on 1,000 randomizations (“phylosig” function). Blomberg’s K measures phylogenetic signal by quantifying the amount of observed trait variance relative to trait variance expected under a Brownian motion model (simulating evolution conditions similar to genetic drift; Kamilar and Cooper, 2013).
We also examined the phylogenetic patterns of antennal responses across species when stimulated with individual compounds. To this end, we fitted and compared two different models of trait evolution. First, we fitted a single-rate multivariate Brownian Motion (BM) model that corresponds to a random walk process, in which the probability of divergence in antennal responses increases uniformly over time. Second, we fitted a single-optimum Orenstein-Uhlenbeck (OU) model, in which the variance in antennal responses decreased over time as trait values converge around a global phenotypic optimum. The OU model has a global evolutionary rate parameter (σ2), a global phenotypic optimum parameter (θ), and a global strength of selection (α) parameter. Parameter estimates and the associated likelihood values for continuous character evolution in univariate datasets (i.e., responses to a specific compound) were calculated using the “fitContinuous” function, which we compared using the corrected Akaike information criterion (AICc). Lower AICc values (AICc ≤ 10) thereby indicate better evidence for a given model. We estimated models without (AICc) and with standard errors (AICc_SE).
Additionally, we calculated disparity through time (DTT) plots (“dtt” function) to investigate how antennal responses occupy trait space throughout the evolutionary history of the lineages included in our study. To do this, we compared the observed DTT trajectory across the phylogeny relative to antennal responses simulated via a pure Brownian motion model of trait evolution (random-walk model; see also Harmon et al., 2003). We assessed the difference between the observed disparities and the simulated disparities using the morphological diversity index (MDI) statistics after Harmon et al. (2003), a measure of the area between the mean observed and simulated DTT. Significance of MDI expectation was assessed according to the 95% confidence interval of 100 simulations with a level of significance defined at α ≤ 0.05.
For graphical representation of the combined data, we plotted a phylogenetic tree with a heatmap reflecting the standardized mean antennal responses (in percent, see above; “phylo.heatmap” function).
Results
Electroantennographic Measurements (EAGs)
The statistical analyses comparing the antennal response profiles of tested bees revealed a significant difference among euglossine genera (PERMANOVA: Pseudo-F3,233 = 8.31, P < 0.001; Figure 2). Pair-wise comparisons showed that antennal responses of the two genera Euglossa and Eulaema differed significantly from each other and also from the other genera (P < 0.05 each). The only genera that did not significantly differ were Eufriesea and Exaerete (P = 0.19). Also, the dispersion of antennal response profiles differed among the genera (PERMDISP: F3,258 = 9.33, P < 0.001; Figure 2). The responses of Euglossa were most diverse, followed by Eulaema, Eufriesea and finally Exaerete. Thus, the dispersion is related with the number of species sampled per genus. The SIMPER analysis revealed that antennal responses to the chemical compounds, methyl o-anisate, α-copaene, eugenol separated Eufriesea and Exaerete bees from the other two genera, while the responses to β-ionone, ipsdienol, methyl salicylate and (−)-(E)-carvone epoxide seem to be mostly responsible for the dissimilarity between Euglossa and Eulaema bees (Figure 2).
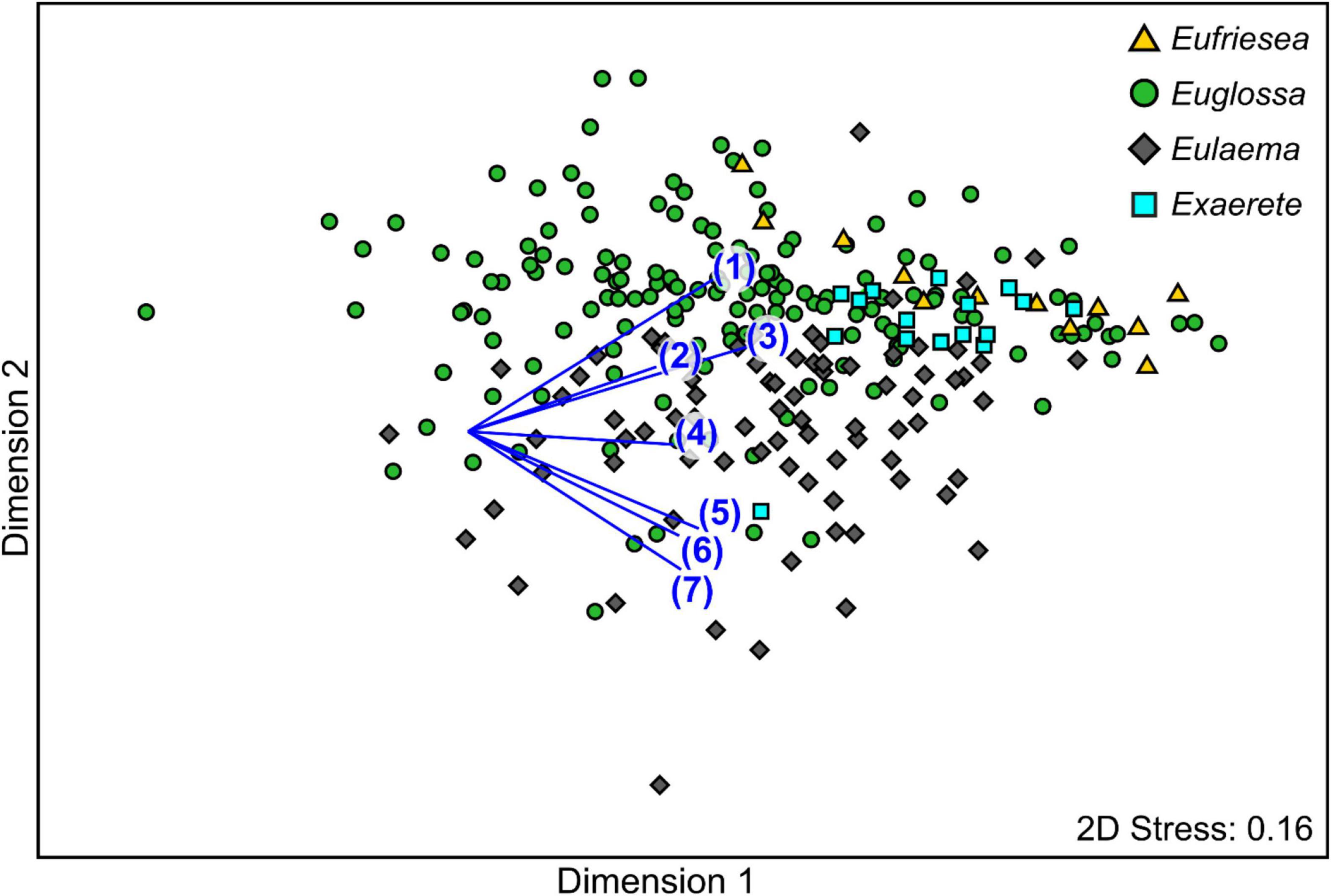
Figure 2. Non-metric multidimensional scaling (nMDS) representation of antennal responses of different euglossine genera to 23 compounds, based on a Bray Curtis similarity matrix (standardized responses in percent). The single dots represent single bee individuals. Vectors represent the Pearson correlations for compounds most responsible for the dissimilarity in antennal response profiles between genera as indicated in a SIMPER analysis: (1) methyl o-anisate, (2) α-copaene, (3) eugenol, (4) β-ionone (5) ipsdienol, (6) methyl salicylate, (7) (−)-(E)-carvone epoxide.
There was also a significant difference between antennal responses when comparing species within the subgenera (nested in genus) (PERMANOVA: Pseudo-F3,233: 8.87, P < 0.001). Pair-wise comparisons within Euglossa showed that antennal responses of Euglossa s. str. species, Glossurella species and Glossura species differed from each other (P < 0.01 each). Antennal responses to the chemical compounds α-humulene, α-copaene, terpinen-4-ol, α-pinene, (−)-(E)-carvone epoxide and ipsdienol were mainly responsible for the differences among all three subgenera (Figure 3A). Within the genus Eulaema antennal responses differed significantly among the two subgenera Eulaema s. str. and Apeulaema (P < 0.001). The responses to α-phellandrene, eugenol, ipsdienol, eucalyptol and (−)-(E)-carvone epoxide explained most of the response differences between these two subgenera (Figure 3B).
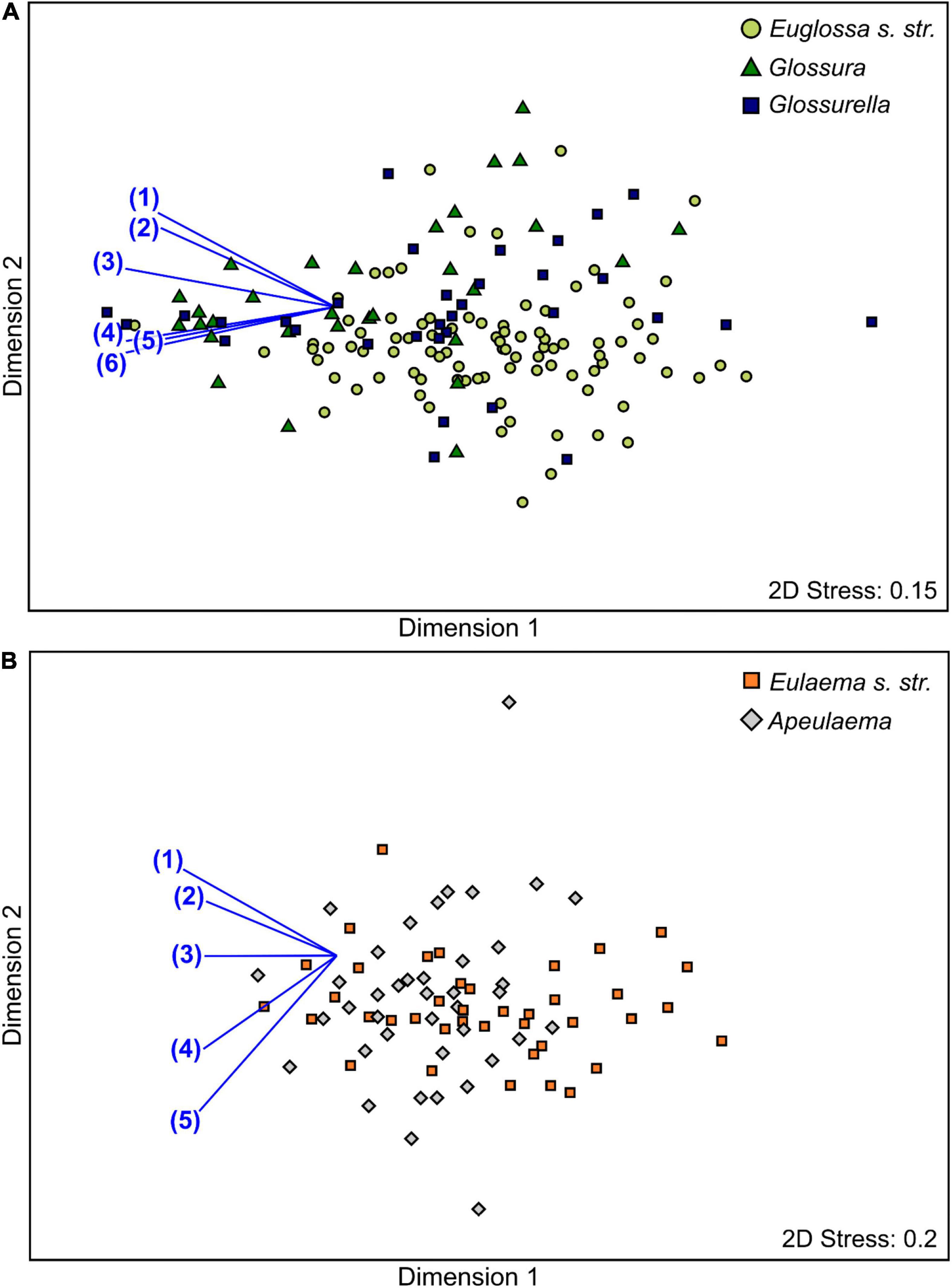
Figure 3. Non-metric multidimensional scaling (nMDS) representation of antennal responses of different euglossine genera to 23 compounds, based on a Bray Curtis similarity matrix (standardized responses in percent). The single dots represent single bee individuals. Vectors represent the Pearson correlations for compounds most responsible for the dissimilarity in antennal response profiles between genera as indicated in a SIMPER analysis. (A) Subgenera within Euglossa (i.e., Euglossa s. str., Glossurella, and Glossura); (1) α-humulene, (2) α-copaene, (3) terpinen-4-ol, (4) α-pinene, (5) (−)-(E)-carvone epoxide, (6) ipsdienol. (B) Subgenera within Eulaema (i.e., Eulaema s. str. and Apeulaema); (1) α-phellandrene, (2) eugenol, (3) ipsdienol, (4) eucalyptol, (5) (−)-(E)-carvone epoxide.
We also found a significant difference in antennal responses when comparing distinct species (nested in subgenera) among each other (PERMANOVA: Pseudo-F22,233: 3.03, P < 0.001; Figure 4).
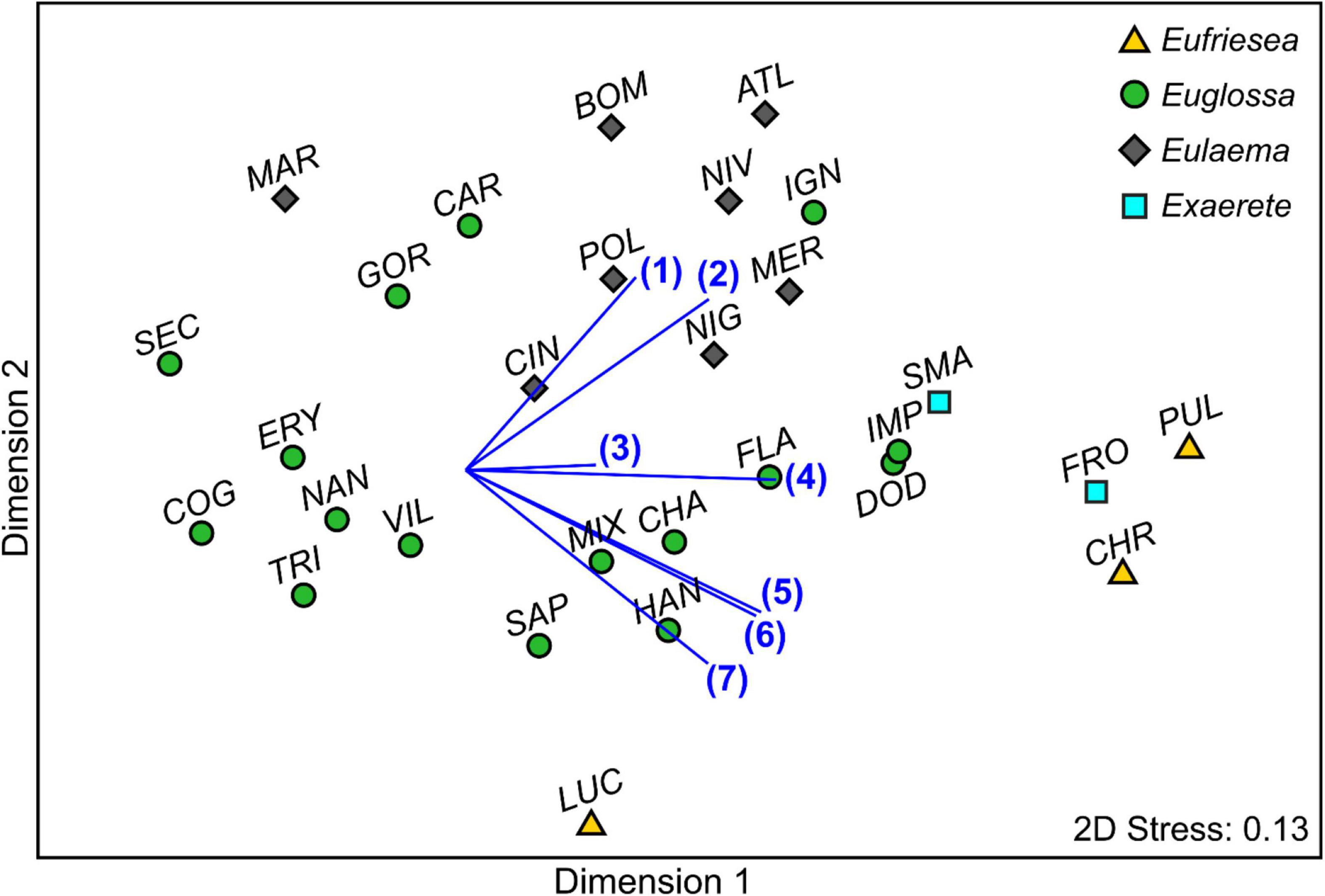
Figure 4. Non-metric multidimensional scaling (nMDS) representation of mean antennal responses of different euglossine bees to 23 compounds, based on a Bray Curtis similarity matrix (standardized responses in percent). Vectors represent the Pearson correlations for compounds most responsible for the dissimilarity in antennal response profiles between species as indicated in a SIMPER analysis: (1) (−)-(E)-carvone epoxide, (2) ipsdienol, (3) β-ionone, (4) α-pinene, (5) α-humulene, (6) α-copaene, (7) methyl o-anisate. Eg. carolina (CAR), Eg. championi (CHA), Eg. cognata (COG), Eg. dodsoni (DOD), Eg. erythrochlora (ERY), Eg. flammea (FLA), Eg. gorgonensis (GOR), Eg. hansoni (HAN), Eg. ignita (IGN), Eg. imperialis (IMP), Eg. mixta (MIX), Eg. nanomelanotricha (NAN), Eg. securigera (SEC), Eg. sapphirina (SAP), Eg. tridentata (TRI), Eg. villosiventris (VIL), Ef. chrysopyga (CRY), Ef. lucifera (LUC), Ef. pulchra (PUL), El. atleticana (ATL), El. bombiformis (BOM), El. cingulata (CIN), El. marcii (MAR), El. meriana (MER), El. nigrita (NIG), El. niveofasciata (NIV), El. polychroma (POL), Ex. frontalis (FRO), and Ex. smaragdina (SMA).
The comparisons of absolute EAG responses to n-hexane and each chemical compound at the tested concentration of 10–2 revealed significant differences in all tested species (P < 0.01 each; Supplementary Figure 2). Generally, all tested species, irrespective of genus, showed strong antennal responses to benzyl acetate, 1,4-dimetoxy-benzene and veratrole. In addition, benzyl alcohol, eugenol, linalool, methyl benzoate and methyl salicylate elicited strong responses in most species. Weak antennal responses were found to the compounds α-copaene, α-humulene, methyl o-anisate, α-pinene, and 1,2,4-trimethoxy benzene and cannot be perceived by all tested bee species.
Phylogenetic Analyses
The Blomberg’s K test revealed a significant level of phylogenetic signal in antennal response profiles of euglossine bees (n = 624, K = 0.68, P < 0.01), indicating that close relatives are more similar in their antennal response profiles than random pairs of species. These findings were supported by the calculated parameter estimates and the likelihood for continuous character evolution in a BM model (sigSq < 0.001, log-likelihood = 116.56, AICc < 1) as well as by the OU model (sigSq < 0.001, log-likelihood = 116.69, AICc < 1). Optimal antennal responses in all tested euglossine species were suggested for the chemical compounds benzyl alcohol (sigSq < 0.01, log-likelihood = −1.46, AICc ≤ 10; BM and OU model), 1,4-dimetoxybenzene (sigSq < 0.01, log-likelihood = −0.63, AICc ≤ 10; OU model), eugenol (sigSq < 0.01, log-likelihood = −2.22, AICc < 10; BM model), linalool (sigSq < 0.01, log-likelihood = −1.6, AICc ≤ 10; BM model), methyl benzoate (sigSq < 0.01, log-likelihood = 1.91, AICc < 5; BM and OU model), methyl salicylate (sigSq < 0.01, log-likelihood = −0.99, AICc < 10; OU model) and veratrole (sigSq < 0.01, log-likelihood = 1.85, AICc < 1; BM model). These compounds offer best evidence to the given models and are also reflected by the strong antennal responses of euglossine species shown in the heatmap of Figure 5.
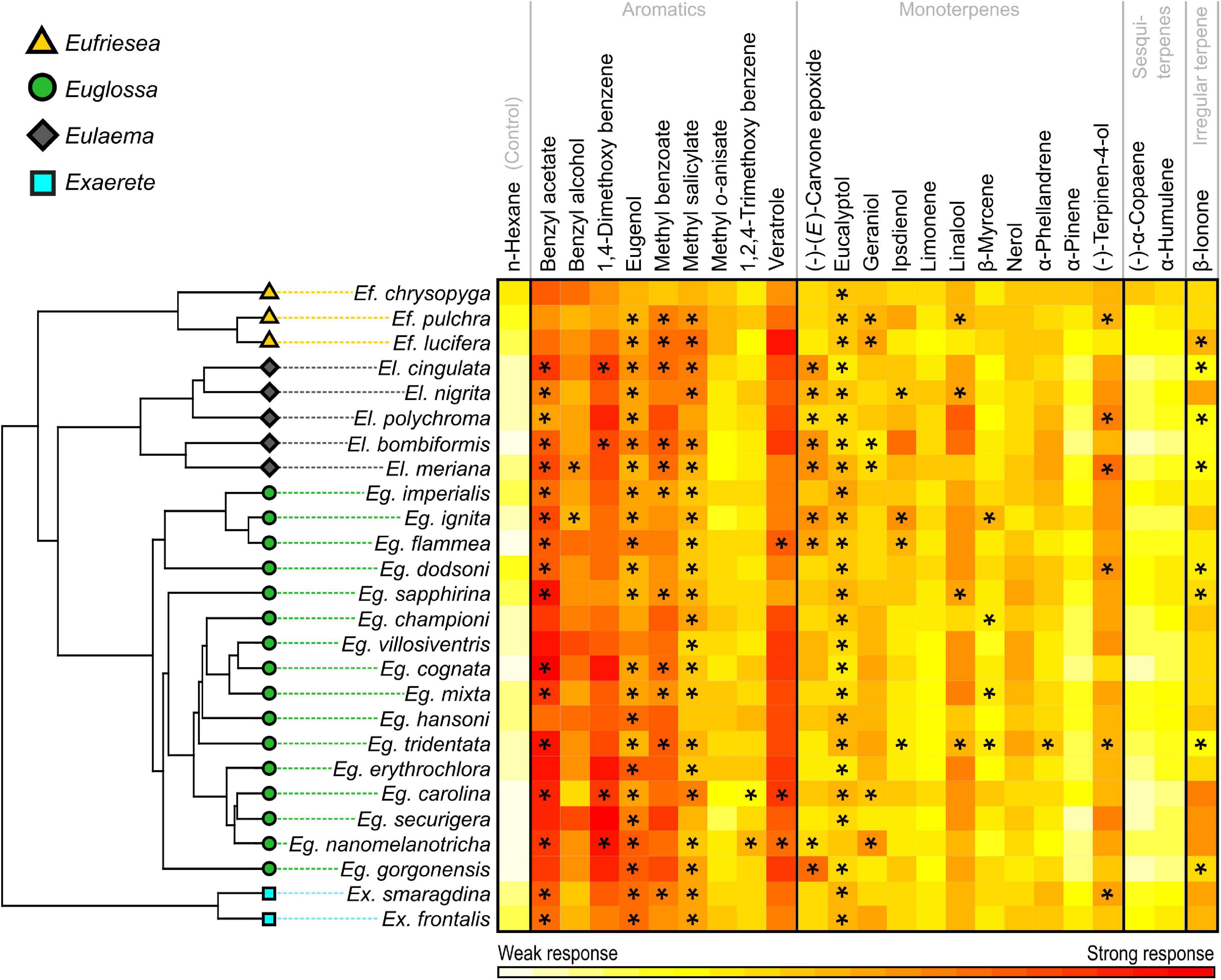
Figure 5. Phylogenetic relationships of euglossine bee species based on data available for 26 of our 29 tested euglossine species (Ramírez et al., 2010b) included in this study along with a heatmap of the standardized antennal response profiles (standardized responses in percent). Colors indicate relative values of antennal strength to different chemical compounds, ranging from weak (bright yellow) to strong (deep red) responses. *Chemicals known to act as attractants for species; see also Table 1.
Our analyses on the disparity of antennal response profiles through time show that the observed disparity in antennal responses was higher than expected under a neutral Brownian motion model of trait evolution (MDI: Average square = 0.24; Figure 6). In fact, we found that the relative disparity was most pronounced towards recent times (equivalent to the tips of the phylogeny in Figure 5).
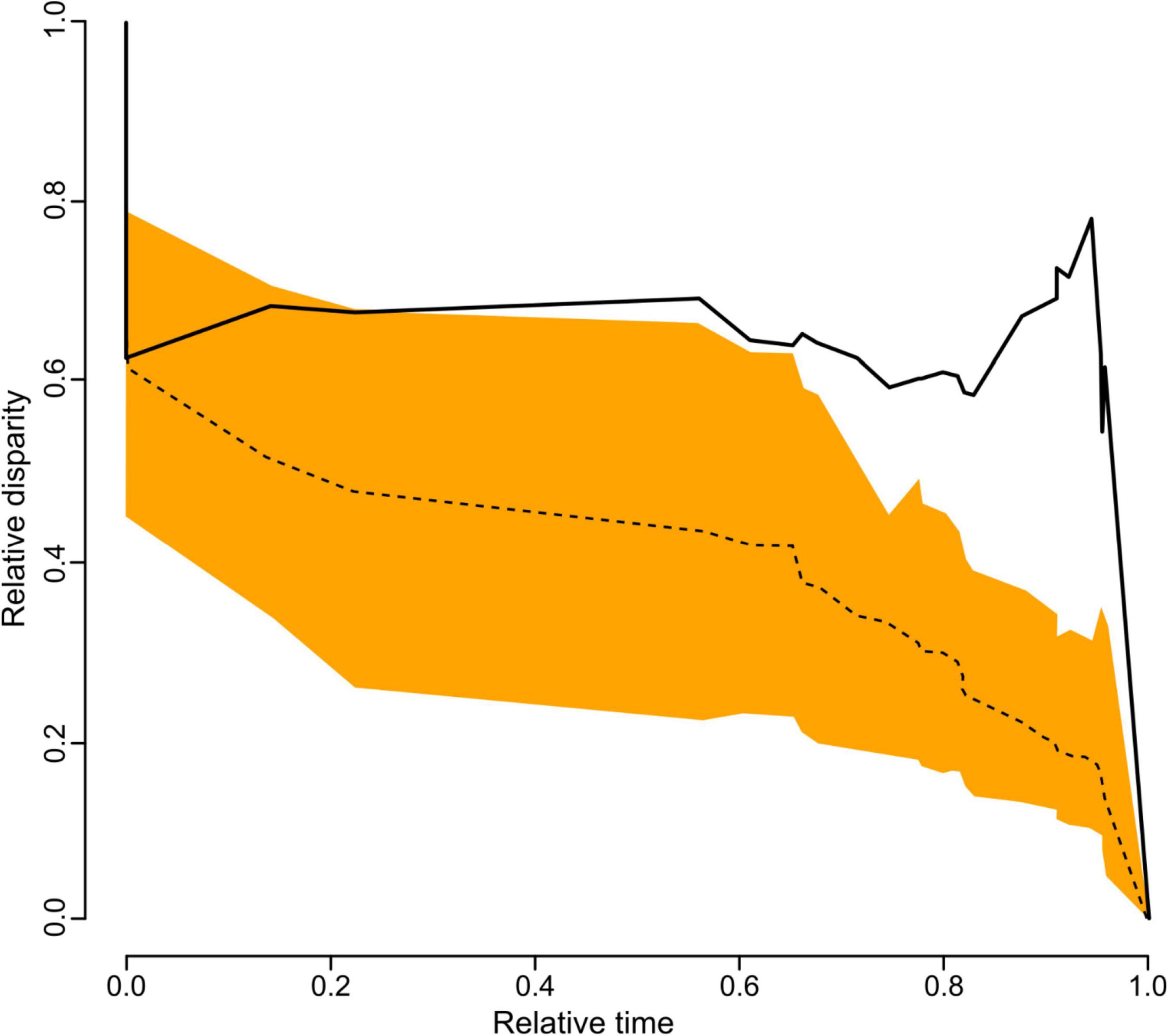
Figure 6. Disparity through time (DDT) based on 100 simulations of phenotypic evolution of antennal response profiles (standardized responses in percent) based on data available for 26 of our 29 tested euglossine species. The relative time ranges from the beginning of the simulated evolution (0.0) to recent times (1.0; equivalent to the tips of the phylogeny in Figure 5). The dashed line represents the mean change in disparity across 100 replicates of simulated diversification and trait evolution as expected under a Brownian motion model with a 95% confidence interval of DDT range (orange area). The solid black line represents the actual mean change in disparity as calculated across the trees.
Discussion
In support to our hypothesis, the data revealed that antennal responses differ among euglossine genera, subgenera and species. Antennal responses to the chemical compounds methyl o-anisate, α-copaene, eugenol, β-ionone, ipsdienol, methyl salicylate and (−)-(E)-carvone epoxide were most responsible for these differences. Our phylogenetic analyses revealed that antennal response profiles to some compounds exhibit a phylogenetic signal and the variation in responses across the phylogeny are congruent with a Brownian motion model of trait evolution. This was the case with the antennal responses to benzyl alcohol, 1,4-dimetoxy-benzene, eugenol, linalool, methyl benzoate, methyl salicylate and veratrole. Our data also demonstrates that throughout the evolutionary history of the species we tested, the overall disparity in response between species was greater than expected under a null model of Brownian evolution.
The observed variation among antennal response profiles of tested euglossine bee species and taxonomic groups suggest that the antennae of the different bee species possess distinct types of ORs for different chemical compounds or different amounts of specific ORs. However, previous studies have shown that the sensitivity of ORs can also be influenced by further processes, such as tuning via metabotropic auto-regulation (Getahun et al., 2013) or variability in molecular receptive ranges (Hallem and Carlson, 2006), demonstrating the complexity of the olfactory periphery that could be responsible for the different antennal responses among tested species. Neural processing in the insect brain could also influence the olfactory perception in euglossine bees (e.g., Renou, 2014). To investigate the antennal responses of euglossine bees to specific compounds on the neuronal level, several approaches can be taken, including assaying individual olfactory receptors or measuring neural activity of brain regions in vivo (see e.g., Renou, 2014). For example, methods like single sensillum recording (SSR), the empty neuron system (Brand et al., 2020), or calcium imaging of glomerular responses in the antennal lobe (Galizia and Vetter, 2004) could further contribute to the understanding of the complexity of olfactory tuning, processing and encoding in euglossine bees to chemical compounds that are used during courtship display and that several plants lineages, including orchids, have exploited for pollination services. In addition, sequences of the genome of all tested species could be used in further phylogenetic investigations to study the diversity of OR genes.
The results of our electroantennographic analyses revealed a clear difference among the antennal response profiles among euglossine bee genera (especially between Euglossa and Eulaema). Our study offers the first experimental evidence for the assumption that properties of the sensory equipment assort according to major taxonomic groups of euglossine bees. Bees of different genera respond differently to compounds, such as α-copaene, eugenol, ipsdienol, and (−)-(E)-carvone epoxide. In agreement to these patterns, the chemical composition of floral scents of perfume-rewarding orchids has been shown to differ among Euglossa- and Eulaema-pollinated species. Several chemical compounds which seem to be typically found in the floral scent bouquets of either Euglossa- (i.e., ipsdienol and myrcene; Milet-Pinheiro and Gerlach, 2017; Brandt et al., 2020) or Eulaema-pollinated species (e.g., α-pinene and (−)-(E)-carvone epoxide; Whitten et al., 1986; Milet-Pinheiro and Gerlach, 2017) coincide with the compounds most responsible for the separation of antennal response profiles among tested euglossine genera in our study. Altogether, these findings underline the finding of Ramírez et al. (2011) suggesting that distinct sensory biases between euglossine bee lineages have shaped the evolution of floral scents in perfume-rewarding plants. Under such scenario, floral scent bouquets evolve to target the compounds with strong sensory responses and behavioral attraction (Milet-Pinheiro and Gerlach, 2017) and lead to a genus specific attraction of pollinators. This is important because of the highly specialized pollination mechanisms exhibited by some perfume-producing orchids (see Dodson, 1962; Vogel, 1966; Romero and Carnevali, 2009) in which the morphological properties (i.e., the size) of euglossine bees, which typically differ among genera, ensure successful pollinarium transfer from male to female flowers (Dodson, 1962, 1978). For example, Catasetum species that are pollinated by Euglossa bees (8–18 mm in size; Dressler, 1982; Carvalho and Machado, 2002; Ramírez et al., 2002) are usually visited by two or more congeneric species, but rarely by species of Eulaema with a larger body size (20–35 mm in size; Dressler, 1982; Ramírez et al., 2002), and vice versa (Whitten et al., 1986, 1988; Ramírez et al., 2002). In fact, a similar pattern has also been reported in the orchid genus Gongora, which is also exclusively pollinated by euglossine bees (Hetherington-Rauth and Ramírez, 2015).
Within the tested euglossine bee genera, we found that the antennal response profiles are also specific at the subgenera and species levels. This observation provides further evidence for the idea of sensory niche partitioning provided by Zimmermann et al. (2009a). In areas where many different euglossine species of the same genus occur sympatrically, species-specific attraction of pollinators to perfume-rewarding flowers is not only essential to ensure pollinator fidelity and avoid pollinator competition but also to ensure reproductive isolation among closely related orchid species. Appropriate mixtures of chemical compounds or the presence of specific major compounds in the floral scents enable the attraction of only few out of many different euglossine species (Dodson, 1970; Whitten et al., 1986). Together with further isolating mechanisms (see e.g., Hills et al., 1972; Williams and Whitten, 1983) the resulting highly specific attraction of pollinators in euglossinophilous plants can act as an effective reproductive barrier among otherwise interfertile plant species (Milet-Pinheiro and Gerlach, 2017). This is possible due to compound-specific differences in antennal perception even among closely related euglossine species, as we report here. For example, Eltz et al. (2008) showed how males of Eg. dilemma are strongly attracted to hydroxy-6-nona-1,3-dienyl-benzaldehyde (HNDB) and show strong antennal responses, while bees of the closely related and morphologically (Eltz et al., 2011) as well as ecologically (Villanueva-Gutierrez et al., 2013) similar species Eg. viridissima neither responds to this compound behaviorally nor electroantennographically. Brand et al. (2015, 2020) found that this divergence can be explained by a different selection among one single olfactory receptor gene (i.e., OR41), proving that (1) changes in the chemosensory gene family occur among closely related species and that (2) strong divergent selection acting on chemosensory receptor genes plays an important role in the evolution and diversification of the olfactory system in euglossine bees. The high species-specificity in antennal response profiles among species could be explained by the patterns of evolution of chemical sexual signaling. For example, the study of Cardé and Baker (1984) suggests that female preferences for a signal (receiver) impose strong stabilizing selection on male signal traits (sender), favoring the stability of the signal among populations and leading to a high species-specificity of chemical traits even across large geographic distances (Ord and Stamps, 2009). In this context, Zimmermann et al. (2006) and Ramírez et al. (2010a) revealed a qualitative consistency in perfume composition of tibial organs within euglossine bee species even when comparing populations from distant geographic regions that harbor different perfume sources.
The results of the Blomberg’s K test indicate the presence of a phylogenetic signal in the antennal response profiles of euglossine bee species. More specifically, there seems to be a tendency for species within a lineage to resemble each other more in their antennal responses than they resemble other lineages or random pairs of species, indicating that the diversification of the olfactory system of euglossine bee clades (genera) is phylogenetically conserved. Some chemical compounds (i.e., benzyl alcohol, 1,4-dimetoxy benzene, eugenol, linalool, methyl benzoate, methyl salicylate and veratrole) revealed an optimal level of antennal responses in the Brownian motion or Orenstein-Uhlenbeck model. A similar pattern was already described by Mitko et al. (2016) comparing the antennal responses of males belonging to 15 sympatric Euglossa species stimulated with compounds present in the hind tibiae. The results of this study suggest that sensory specialization has occurred within multiple lineages due to strong antennal responses for some chemicals that are present as major compounds in the perfume of the same species. Such a pattern is congruent with strong stabilizing selection acting to maintain antennal responses to specific compounds across the phylogeny (Hansen, 1997). The compounds affected by that pattern in our study have been frequently reported, not only within the floral scents of perfume-rewarding pollination systems (e.g., Montalvo and Ackerman, 1986; Gerlach and Schill, 1991; Hentrich et al., 2010), but in a variety of angiosperms worldwide (Knudsen et al., 2006). Therefore, we can assume that the selection on antennal response profiles of euglossine bee species could not only be driven by the association of perfume-rewarding plants but also by other aspects. For example, the compounds promoting an optimal level of antennal responses, as suggested by our phylogenetic analysis, could be important signal traits in the discrimination of sex partners by female orchid bees (see also Cardé and Baker, 1984) or in the search for nectar (see e.g., Borrell, 2005). Since perfume-rewarding flowers seem to contribute only little to the aromatic richness found in the tibial organs of male euglossine bees (Whitten et al., 1993; Ramírez et al., 2010a), we also cannot exclude, for example, the influence of non-floral perfume sources for male euglossine bees, such as rotting plant material, bark, leaves, and feces (Whitten et al., 1993).
At the same time, the results derived from the DDT plots indicate that closely related euglossine diverge more quickly on their antennal response profiles than expected under a Brownian motion model of neutral trait evolution, especially at the species level underlining the specificity of antennal responses on the species level of euglossine bees found in our electroantennographic analyses. Generally, these results resemble the patterns that have already been described on the macroevolution of perfume signaling in euglossine bees (i.e., perfumes collected in the tibial organs of males). For example, the study of Weber et al. (2016) revealed both high species-specificity and elevated rates of evolution in perfume signals found in extracts of the tibial organs of distinct Euglossa species and stated that perfume evolution may be tied to the high number of orchid bee species coexisting together in neotropical communities. Furthermore, they described a rapid divergence at speciation and character displacement (see also Zimmermann et al., 2009b). Because of the high diversity of antennal response profiles on the species level, we cannot totally exclude the possibility that there might exist co-evolutionary adaptations between perfume flowers and euglossine bees, especially during the latest stage of evolution. Earlier studies have, indeed, suspected a (rather loose) coevolution for perfume rewarding orchids and their euglossine pollinators (e.g., Kiester et al., 1984). However, the already mentioned findings that (1) floral scent of perfume flowers developed much later than the collection behavior in euglossine bees (Ramírez et al., 2011) and that (2) a great part of chemical compounds collected by euglossine bees to build their unique tibial blends derive from non-floral rather than floral sources (Whitten et al., 1993; Ramírez et al., 2010a), suggest that sexual selection/changes in mating ecology might influence the evolution of the olfactory equipment of distinct euglossine bee species rather than the floral sources or their availability.
Altogether, the results of our study offer an overview of antennal responses for many different euglossine bee species belonging to distinct genera. The differences in antennal responses between distinct euglossine genera and subgenera, as well as species-specific patterns, reinforce the findings for the floral scent compositions in different species of perfume-rewarding flowers and offer first experimental evidence for the hypothesis of pollinator-mediated selection of floral scents driven by preexisting sensory biases in euglossine bees (Ramírez et al., 2011). The findings of our phylogenetic analyses indicate that a diversification of the olfactory system between euglossine bee genera could be (at least partly) phylogenetically conserved. Moreover, our results are congruent with a scenario of stabilizing selection acting on antennal responses to individual compounds, in particular to chemical compounds commonly found in perfume-rewarding flowers. At the same time, closely related species within taxonomic groups can differ considerably in their olfactory system due to a rapid evolution and a high level of disparity (Brand et al., 2020). Further phylogenetic investigations, for example on chemosensory genes of euglossine species (similar to the work of Brand et al., 2015) in combination with electroantennographic comparisons could shed more light into the evolution of the sensory periphery of euglossine bees and, consequently, in the evolution of floral scents in perfume-rewarding flowers.
Data Availability Statement
The raw data supporting the conclusions of this article will be made available by the authors, without undue reservation.
Author Contributions
KB, SD, PM-P, and MA developed the experimental design and the idea. OR and DD synthetized the tested compound carvone epoxide. KB and PM-P conducted all experiments and collected the data. KB wrote the first draft of the manuscript and analyzed the results of the electroantennography. SR was mainly responsible for the phylogenetic analyses. All authors contributed to the revision of the manuscript.
Funding
This work was supported by the Deutsche Forschungsgemein schaft (AY 12/12-1), the Fundaçao de Apoio ao Desenvolvimento da Universidade Federal de Pernambuco (FADE-UFPE), the Deutscher Akademischer Austauschdienst (DAAD grant number 57210526 to KB), the “Ulrike Goldschmid-Grant” (grant to KB), the Coordenação de Aperfeiçoamento de Nível Superior (CAPES, Brazil – Finance Code 001 – grant to PM-P), the Fundação de Amparo à Ciência e Tecnologia do Estado de Pernambuco (grant number FACEPE/BCT-0288-2.05/17 to PM-P), and the Conselho Nacional de Desenvolvimento Científico e Tecnológico (grant number CNPq/PDJ-152077/2016-2 to PM-P).
Conflict of Interest
The authors declare that the research was conducted in the absence of any commercial or financial relationships that could be construed as a potential conflict of interest.
Publisher’s Note
All claims expressed in this article are solely those of the authors and do not necessarily represent those of their affiliated organizations, or those of the publisher, the editors and the reviewers. Any product that may be evaluated in this article, or claim that may be made by its manufacturer, is not guaranteed or endorsed by the publisher.
Acknowledgments
We thank the staff of the field station La Gamba in Costa Rica for providing research facilities, accommodation and personal care. We further thank Ulrike Goldschmid and all responsible people for awarding the private “Ulrike Goldschmid-Grant” in cooperation with the University of Vienna.
Supplementary Material
The Supplementary Material for this article can be found online at: https://www.frontiersin.org/articles/10.3389/fevo.2021.727471/full#supplementary-material
References
Ackerman, J. D. (1983). Diversity and seasonality of male euglossine bees (Hymenoptera: Apidae) in Central Panama. Ecology 64, 274–283. doi: 10.2307/1937075
Ackerman, J. D. (1989). Geographic and seasonal variation in fragrance choices and preferences of male euglossine bees. Biotropica 21, 340–347. doi: 10.2307/2388284
Anderson, M. J., Gorley, R. N., and Clarke, K. R. (2008). PERMANOVA + for PRIMER: Guide to Software and Statistical Methods. Plymouth: PRIMER-E.
Armbruster, W. S., and McCormick, K. D. (1990). Diel foraging patterns of male euglossine bees: ecological causes and evolutionary responses by plants. Biotropica 22, 160–171. doi: 10.2307/2388409
Armbruster, W. S., Keller, S., Matsuki, M., and Clausen, T. (1989). Pollination of Dalechampia magnoliifolia (Euphorbiaceae) by male euglossine bees. Am. J. Bot. 76, 1279–1285. doi: 10.1002/j.1537-2197.1989.tb15109.x
Ayasse, M., and Dötterl, S. (2014). The role of preadaptations or evolutionary novelties for the evolution of sexually deceptive orchids. New Phytol. 203, 710–712. doi: 10.1111/nph.12914
Ayasse, M., Paxton, R. J., and Tengö, J. (2001). Mating behavior and chemical communication in the order Hymenoptera. Annu. Rev. Entomol. 46, 31–78. doi: 10.1146/annurev.ento.46.1.31
Ayasse, M., Schiestl, F. P., Paulus, H. F., Ibarra, F., and Francke, W. (2003). Pollinator attraction in a sexually deceptive orchid by means of unconventional chemicals. Proc. R. Soc. Lond. B Biol. Sci. 270, 517–522. doi: 10.1098/rspb.2002.2271
Ayasse, M., Stökl, J., and Francke, W. (2011). Chemical ecology and pollinator-driven speciation in sexually deceptive orchids. Phytochemistry 72, 1667–1677. doi: 10.1016/j.phytochem.2011.03.023
Blomberg, S. P., Garland, T. Jr., and Ives, A. R. (2003). Testing for phylogenetic signal in comparative data: behavioral traits are more labile. Evolution 57, 717–745. doi: 10.1111/j.0014-3820.2003.tb00285.x
Borrell, B. J. (2005). Long tongues and loose niches: evolution of euglossine bees and their nectar flowers 1. Biotropica 37, 664–669. doi: 10.1111/j.1744-7429.2005.00084.x
Brand, P., Hinojosa-Díaz, I. A., Ayala, R., Daigle, M., Obiols, C. L. Y., Eltz, T., et al. (2020). The evolution of sexual signaling is linked to odorant receptor tuning in perfume-collecting orchid bees. Nat. Commun. 11:244. doi: 10.1038/s41467-019-14162-6
Brand, P., Ramírez, S. R., Leese, F., Quezada-Euan, J. J. G., Tollrian, R., and Eltz, T. (2015). Rapid evolution of chemosensory receptor genes in a pair of sibling species of orchid bees (Apidae: Euglossini). BMC Evol. Biol. 15:176. doi: 10.1186/s12862-015-0451-9
Brandt, K., Dötterl, S., Fuchs, R., Navarro, D. M. D. A. F., Machado, I. C. S., Dobler, D., et al. (2019). Subtle chemical variations with strong ecological significance: stereoselective responses of male orchid bees to stereoisomers of carvone epoxide. J. Chem. Ecol. 45, 464–473. doi: 10.1007/s10886-019-01072-6
Brandt, K., Machado, I. C., Navarro, D. M. D. A. F., Dötterl, S., Ayasse, M., and Milet-Pinheiro, P. (2020). Sexual dimorphism in floral scents of the neotropical orchid Catasetum arietinum and its possible ecological and evolutionary significance. AoB Plants 12:laa030. doi: 10.1093/aobpla/plaa030
Cancino, A. D. M., and Damon, A. (2007). Fragrance analysis of euglossine bee pollinated orchids from Soconusco, south-east Mexico. Plant Species Biol. 22, 129–134. doi: 10.1111/j.1442-1984.2007.00185.x
Cardé, R. T., and Baker, T. C. (1984). “Sexual communication with pheromones,” in Chemical Ecology of Insects, eds W. J. Bell and R. T. Cardé (Boston, MA: Springer), 355–383. doi: 10.1007/978-1-4899-3368-3_13
Carvalho, R., and Machado, I. (2002). Pollination of Catasetum macrocarpum (Orchidaceae) by Eulaema bombiformis (Euglossini). Lindleyana 17, 85–90.
Chow, Y., and Wang, S. (1981). Attraction responses of the American cockroach to synthetic periplanone-B. J. Chem. Ecol. 7, 265–272. doi: 10.1007/BF00995749
Clarke, K. R., and Gorley, R. N. (2006). PRIMER V6: User Manual / Tutorial. Plymouth: PRIMER-E, Ltd.
Couto, A., Alenius, M., and Dickson, B. J. (2005). Molecular, anatomical, and functional organization of the Drosophila olfactory system. Curr. Biol. 15, 1535–1547. doi: 10.1016/j.cub.2005.07.034
Dodson, C. (1970). “The Role of Chemical Attractants in Orchid Pollination,” in Biochemical Co-Evolution, ed. K. L. Chambers (Corvallis: Oregon State University Press), 83–107.
Dodson, C. H. (1962). Pollination and variation in the subtribe Catasetinae (Orchidaceae). Ann. Mo. Bot. Gard. 49, 35–56. doi: 10.2307/2394740
Dodson, C. H., Dressler, R. L., Hills, H. G., Adams, R. M., and Williams, N. H. (1969). Biologically active compounds in orchid fragrances. Science 164, 1243–1249. doi: 10.1126/science.164.3885.1243
Dressler, R. L. (1968). Pollination by euglossine bees. Evolution 22, 202–210. doi: 10.1111/j.1558-5646.1968.tb03463.x
Dressler, R. L. (1982). Biology of the orchid bees (Euglossini). Annu. Rev. Ecol. Syst. 13, 373–394. doi: 10.1146/annurev.es.13.110182.002105
Eltz, T., Ayasse, M., and Lunau, K. (2006). Species-specific antennal responses to tibial fragrances by male orchid bees. J. Chem. Ecol. 32, 71–79. doi: 10.1007/s10886-006-9352-0
Eltz, T., Fritzsch, F., Pech, J. R., Zimmermann, Y., Ramirez, S. R., Quezada-Euan, J. J. G., et al. (2011). Characterization of the orchid bee Euglossa viridissima (Apidae: Euglossini) and a novel cryptic sibling species, by morphological, chemical, and genetic characters. Zool. J. Linn. Soc. 163, 1064–1076. doi: 10.1111/j.1096-3642.2011.00740.x
Eltz, T., Roubik, D. W., and Whitten, M. W. (2003). Fragrances, male display and mating behaviour of Euglossa hemichlora: a flight cage experiment. Physiol. Entomol. 28, 251–260. doi: 10.1111/j.1365-3032.2003.00340.x
Eltz, T., Roubik, D. W., and Lunau, K. (2005a). Experience-dependent choices ensure species-specific fragrance accumulation in male orchid bees. Behav. Ecol. Sociobiol. 59:149. doi: 10.1007/s00265-005-0021-z
Eltz, T., Sager, A., and Lunau, K. (2005b). Juggling with volatiles: exposure of perfumes by displaying male orchid bees. J. Comp. Physiol. A 191, 575–581. doi: 10.1007/s00359-005-0603-2
Eltz, T., Whitten, W., Roubik, D., and Linsenmair, K. (1999). Fragrance collection, storage, and accumulation by individual male orchid bees. J. Chem. Ecol. 25, 157–176. doi: 10.1023/A:1020897302355
Eltz, T., Zimmermann, Y., Pfeiffer, C., Pech, J. R., Twele, R., Francke, W., et al. (2008). An olfactory shift is associated with male perfume differentiation and species divergence in orchid bees. Curr. Biol. 18, 1844–1848. doi: 10.1016/j.cub.2008.10.049
Engel, M. S. (1999). The First Fossil Euglossa and Phylogeny of Orchid Bees (Hymenoptera, Apidae, Euglossini). American Museum novitates; No. 3272. New York, NY: American Museum of Natural History.
Frankie, G., Haber, W., Opler, P., and Bawa, K. (1983). “Characterstics and organization of the large bee pollination system in the Costa Rican dry forest,” in Handbook of Experimental Pollination Biology, eds C. E. Jones and R. J. Little (Berkeley, CA: University of California), 411–447.
Galizia, C. G., and Vetter, R. S. (2004). “Optical methods for analyzing odor-evoked activity in the insect brain,” in Methods in Insect Sensory Neuroscience, ed. T. A. Christensen (Boca Raton, FL: CRC-Press), 349–392.
Garver, L., Van Eikeren, P., and Byrd, J. E. (1976). A facile synthesis of (+)-pinol from (−)-carvone. J. Org. Chem. 41, 2773–2774. doi: 10.1021/jo00878a030
Gerlach, G., and Schill, R. (1991). Composition of orchid scents attracting euglossine bees. Bot. Acta 104, 379–384. doi: 10.1111/j.1438-8677.1991.tb00245.x
Getahun, M. N., Olsson, S. B., Lavista-Llanos, S., Hansson, B. S., and Wicher, D. (2013). Insect odorant response sensitivity is tuned by metabotropically autoregulated olfactory receptors. PLoS One 8:e58889. doi: 10.1371/journal.pone.0058889
González, J. (1996). Fauna of the Henri Pittier national park: Euglossini (Hymenoptera: Apidae: Bombinae). Keys and preliminary list. Mem. Soc. Cien. Nat. Salle 56, 45–54.
Gruber, M. H., Morawetz, L., and Wiemers, M. (2008). Diversidad de Euglossini (Hymenoptera, Apidae) en bosques lluviosos de tierras bajas primarios y secundarios en el sudoeste de Costa Rica. Katal. Oberösterreichischen Landesmuseen Neue Ser. 80, 257–266.
Hallem, E. A., and Carlson, J. R. (2006). Coding of odors by a receptor repertoire. Cell 125, 143–160. doi: 10.1016/j.cell.2006.01.050
Hansen, T. F. (1997). Stabilizing selection and the comparative analysis of adaptation. Evolution 51, 1341–1351. doi: 10.1111/j.1558-5646.1997.tb01457.x
Hansson, B. S., and Stensmyr, M. C. (2011). Evolution of insect olfaction. Neuron 72, 698–711. doi: 10.1016/j.neuron.2011.11.003
Harmon, L. J., Schulte, J. A., Larson, A., and Losos, J. B. (2003). Tempo and mode of evolutionary radiation in iguanian lizards. Science 301, 961–964. doi: 10.1126/science.1084786
Hentrich, H., Kaiser, R., and Gottsberger, G. (2010). Floral biology and reproductive isolation by floral scent in three sympatric Aroid species in French Guyana. Plant Biol. 12, 587–596. doi: 10.1111/j.1438-8677.2009.00256.x
Hetherington-Rauth, M. C., and Ramírez, S. R. (2015). Evolutionary trends and specialization in the euglossine bee-pollinated orchid genus Gongora. Ann. Mo. Bot. Gard. 100, 271–299. doi: 10.3417/2014035
Hildebrand, J. G., and Shepherd, G. M. (1997). Mechanisms of olfactory discrimination: converging evidence for common principles across phyla. Annu. Rev. Neurosci. 20, 595–631. doi: 10.1146/annurev.neuro.20.1.595
Hills, G. (1968). Fragrance Analysis in Chemotaxonomy of the Genus Catasetum (Orchidaceae) Ph. D. Thesis. Coral Gables, FL: University of Miami.
Hills, H. G., Williams, N. H., and Dodson, C. H. (1972). Floral fragrances and isolating mechanisms in the genus Catasetum (Orchidaceae). Biotropica 4, 61–76. doi: 10.2307/2989728
Janzen, D. H., DeVries, P. J., Higgins, M. L., and Kimsey, L. S. (1982). Seasonal and site variation in Costa Rican euglossine bees at chemical baits in lowland deciduous and evergreen forests. Ecology 63, 66–74. doi: 10.2307/1937032
Kamilar, J. M., and Cooper, N. (2013). Phylogenetic signal in primate behaviour, ecology and life history. Philos. Trans. R. Soc. B 368:20120341. doi: 10.1098/rstb.2012.0341
Kiester, A. R., Lande, R., and Schemske, D. W. (1984). Models of coevolution and speciation in plants and their pollinators. Am. Nat. 124, 220–243.
Kimsey, L. S. (1982). Systematics of Bees of the Genus Eufriesea (Hymenoptera, Apidae). Berkeley, CA: University of California Press.
Knudsen, J. T., Eriksson, R., Gershenzon, J., and Ståhl, B. (2006). Diversity and distribution of floral scent. Bot. Rev. 72:1. doi: 10.1663/0006-8101(2006)72[1:DADOFS]2.0.CO;2
López, D. F. (1963). Two attractants for Eulaema tropica L. J. Econ. Entomol. 56:540. doi: 10.1093/jee/56.4.540
Melo, G. A. (2014). Notes on the systematics of the orchid-bee genus Eulaema (Hymenoptera, Apidae). Rev. Bras. Entomol. 58, 235–240. doi: 10.1590/S0085-56262014000300003
Milet-Pinheiro, P., and Gerlach, G. (2017). Biology of the neotropical orchid genus Catasetum: a historical review on floral scent chemistry and pollinators. Perspect. Plant Ecol. Evol. Syst. 27, 23–34. doi: 10.1016/j.ppees.2017.05.004
Milet-Pinheiro, P., Silva, J. B. F., Navarro, D. M., Machado, I. C., and Gerlach, G. (2018). Notes on pollination ecology and floral scent chemistry of the rare neotropical orchid Catasetum galeritum R chb. f. Plant Species Biol. 33, 158–163. doi: 10.1111/1442-1984.12202
Mitko, L., Weber, M. G., Ramirez, S. R., Hedenström, E., Wcislo, W. T., and Eltz, T. (2016). Olfactory specialization for perfume collection in male orchid bees. J. Exp. Biol. 219, 1467–1475. doi: 10.1242/jeb.136754
Montalvo, A. M., and Ackerman, J. D. (1986). Relative pollinator effectiveness and evolution of floral traits in Spathiphyllum friedrichsthalii (Araceae). Am. J. Bot. 73, 1665–1676. doi: 10.1002/j.1537-2197.1986.tb09697.x
Morato, E., Campos, L. D. O., and Moure, J. (1992). Abelhas euglossini (Hymenoptera, Apidae) coletadas na Amazônia central. Rev. Bras. Entomol. 36, 767–771.
Nemesio, A. (2009). Orchid bees (Hymenoptera: Apidae) of the Brazilian Atlantic forest. Zootaxa 2041, 1–242. doi: 10.11646/zootaxa.2041.1.1
Olsson, S. B., and Hansson, B. S. (2013). “Electroantennogram and single sensillum recording in insect antennae,” in Pheromone Signaling, ed. K. Touhara (Berlin: Springer), 157–177. doi: 10.1007/978-1-62703-619-1_11
Ord, T. J., and Stamps, J. A. (2009). Species identity cues in animal communication. Am. Nat. 174, 585–593. doi: 10.1086/605372
Pearson, D. L., and Dressler, R. L. (1985). Two-year study of male orchid bee (Hymenoptera: Apidae: Euglossini) attraction to chemical baits in lowland south-eastern Peru. J. Trop. Ecol. 1, 37–54. doi: 10.1017/S0266467400000067
Pennell, M. W., Eastman, J. M., Slater, G. J., Brown, J. W., Uyeda, J. C., FitzJohn, R. G., et al. (2014). geiger v2. 0: an expanded suite of methods for fitting macroevolutionary models to phylogenetic trees. Bioinformatics 30, 2216–2218. doi: 10.1093/bioinformatics/btu181
Peruquetti, R. C., Campos, L. D. O., Coelho, C. D. P., Abrantes, C. V. M., and Lisboa, L. D. O. (1999). Abelhas Euglossini (Apidae) de áreas de Mata Atlântica: abundância, riqueza e aspectos biológicos. Rev. Bras. Zool. 16(Suppl. 2), 101–118. doi: 10.1590/S0101-81751999000600012
Ramírez, S. R., Eltz, T., Fritzsch, F., Pemberton, R., Pringle, E. G., and Tsutsui, N. D. (2010a). Intraspecific geographic variation of fragrances acquired by orchid bees in native and introduced populations. J. Chem. Ecol. 36, 873–884. doi: 10.1007/s10886-010-9821-3
Ramírez, S. R., Roubik, D. W., Skov, C., and Pierce, N. E. (2010b). Phylogeny, diversification patterns and historical biogeography of euglossine orchid bees (Hymenoptera: Apidae). Biol. J. Linn. Soc. 100, 552–572. doi: 10.1111/j.1095-8312.2010.01440.x
Ramírez, S. R., Eltz, T., Fujiwara, M. K., Gerlach, G., Goldman-Huertas, B., Tsutsui, N. D., et al. (2011). Asynchronous diversification in a specialized plant-pollinator mutualism. Science 333, 1742–1746. doi: 10.1126/science.1209175
Ramírez, S., Dressler, R. L., and Ospina, M. (2002). Abejas euglosinas (Hymenoptera: Apidae) de la región Neotropical: listado de especies con notas sobre su biología. Biota Colomb. 3, 7–118.
Rebelo, J. M. M., and Moure, J. S. (1995). The species of Euglossa Latreille from the northeast of São Paulo, Brazil (Apidae, Euglossinae). Rev. Bras. Zool. 12, 445–466. doi: 10.1590/S0101-81751995000300001
Renou, M. (2014). “Pheromones and general odor perception in insects,” in Neurobiology of Chemical Communication, 1 Edn, ed. C. Mucignat-Caretta (Boca Raton, MA: CRC Press). doi: 10.1201/b16511-3
Revell, L. J. (2012). phytools: an R package for phylogenetic comparative biology (and other things). Methods Ecol. Evol. 3, 217–223. doi: 10.1111/j.2041-210X.2011.00169.x
Robertson, H. M., and Wanner, K. W. (2006). The chemoreceptor superfamily in the honey bee, Apis mellifera: expansion of the odorant, but not gustatory, receptor family. Genome Res. 16, 1395–1403. doi: 10.1101/gr.5057506
Roelofs, W. L. (1984). “Electroantennogram assays: rapid and convenient screening procedures for pheromones,” in Techniques in Pheromone Research, eds H. E. Hummel and T. A. Miller (New York, NY: Springer), 131–159. doi: 10.1007/978-1-4612-5220-7_5
Romero, G. A., and Carnevali, G. (2009). “Catasetum,” in Genera Orchidearum, Epidendroidea – Part II, eds A. M. Pridgeon, P. J. Cribb, M. W. Chase, and F. N. Rasmussen (New York: Oxford University Press), 13–18.
Roubik, D. W., and Hanson, P. E. (2004). Abejas de Orquídeas de la América Tropical: Biología y Guía de Campo. Orchid Bees of Tropical America. Santo Domingo: Editorial Institutuo Nacional de Biodiversidad.
Schäffler, I., Steiner, K. E., Haid, M., van Berkel, S. S., Gerlach, G., Johnson, S. D., et al. (2015). Diacetin, a reliable cue and private communication channel in a specialized pollination system. Sci. Rep. 5:12779. doi: 10.1038/srep12779
Schneider, D., and Steinbrecht, R. A. (1968). Checklist of insect olfactory sensilla. Symp. Zool. Soc. Lond. 23, 279–297.
Shields, V., and Hildebrand, J. G. (2001). Responses of a population of antennal olfactory receptor cells in the female moth Manduca sexta to plant-associated volatile organic compounds. J. Comp. Physiol. A 186, 1135–1151. doi: 10.1007/s003590000165
Silva, F., and Rebêlo, J. M. M. (1999). Euglossine bees (Hymenoptera: Apidae) of buriticupu, amazonia of Maranhão, Brazil1. Acta Amaz. 29, 587–599. doi: 10.1590/1809-43921999294599
Steinbrecht, R. A. (1997). Pore structures in insect olfactory sensilla: a review of data and concepts. Int. J. Insect Morphol. Embryol. 26, 229–245. doi: 10.1016/S0020-7322(97)00024-X
Strausfeld, C. Z., and Kaissling, K.-E. (1986). Localized adaptation processes in olfactory sensilla of Saturniid moths. Chem. Senses 11, 499–512. doi: 10.1093/chemse/11.4.499
Takita, S., Yokoshima, S., and Fukuyama, T. (2011). A practical synthesis of (−)-kainic acid. Org. Lett. 13, 2068–2070. doi: 10.1021/ol200434a
Villanueva-Gutierrez, R., Quezada-Euan, J., and Eltz, T. (2013). Pollen diets of two sibling orchid bee species, Euglossa, in Yucatán, southern Mexico. Apidologie 44, 440–446. doi: 10.1007/s13592-013-0194-9
Vogel, S. (1966). Parfümsammelnde Bienen als Bestäuber von Orchidaceen und Gloxinia. Österr. Bot. Z. 113, 302–361. doi: 10.1007/BF01373435
Wang, Q., Huang, Q., Chen, B., Lu, J., Wang, H., She, X., et al. (2006). Total synthesis of (+)-machaeriol D with a key regio-and stereoselective SN2’ reaction. Angew. Chem. 118, 3733–3735. doi: 10.1002/ange.200600006
Weber, M. G., Mitko, L., Eltz, T., and Ramírez, S. R. (2016). Macroevolution of perfume signalling in orchid bees. Ecol. Lett. 19, 1314–1323. doi: 10.1111/ele.12667
Whitten, W. M., Hills, H. G., and Williams, N. H. (1988). Occurrence of ipsdienol in floral fragrances. Phytochemistry 27, 2759–2760. doi: 10.1016/0031-9422(88)80657-5
Whitten, W. M., Williams, N. H., Armbruster, W. S., Battiste, M. A., Strekowski, L., and Lindquist, N. (1986). Carvone oxide: an example of convergent evolution in euglossine pollinated plants. Syst. Bot. 11, 222–228. doi: 10.2307/2418960
Whitten, W. M., Young, A. M., and Stern, D. L. (1993). Nonfloral sources of chemicals that attract male euglossine bees (Apidae: Euglossini). J. Chem. Ecol. 19, 3017–3027. doi: 10.1007/BF00980599
Williams, N. H., and Dodson, C. H. (1972). Selective attraction of male euglossine bees to orchid floral fragrances and its importance in long distance pollen flow. Evolution 26, 84–95. doi: 10.1111/j.1558-5646.1972.tb00176.x
Williams, N. H., and Whitten, W. M. (1983). Orchid floral fragrances and male euglossine bees: methods and advances in the last sesquidecade. Biol. Bull. 164, 355–395. doi: 10.2307/1541248
Yasuda, A., Yamamoto, H., and Nozaki, H. (1979). A stereoselective 1, 3-transposition reaction of allylic alcohols. Bull. Chem. Soc. Jpn. 52, 1757–1759. doi: 10.1246/bcsj.52.1757
Zacharuk, R. Y. (1980). Ultrastructure and function of insect chemosensilla. Annu. Rev. Entomol. 25, 27–47. doi: 10.1146/annurev.en.25.010180.000331
Zimmermann, Y., Roubik, D. W., and Eltz, T. (2006). Species-specific attraction to pheromonal analogues in orchid bees. Behav. Ecol. Sociobiol. 60:833. doi: 10.1007/s00265-006-0227-8
Zimmermann, Y., Ramírez, S. R., and Eltz, T. (2009a). Chemical niche differentiation among sympatric species of orchid bees. Ecology 90, 2994–3008. doi: 10.1890/08-1858.1
Keywords: antennal responses, electroantennography (EAG), Eufriesea, Euglossa, Eulaema, Exaerete, euglossine bees, perfume flowers
Citation: Brandt K, Dötterl S, Ramírez SR, Etl F, Machado IC, Navarro DMdAF, Dobler D, Reiser O, Ayasse M and Milet-Pinheiro P (2021) Unraveling the Olfactory Biases of Male Euglossine Bees: Species-Specific Antennal Responses and Their Evolutionary Significance for Perfume Flowers. Front. Ecol. Evol. 9:727471. doi: 10.3389/fevo.2021.727471
Received: 18 June 2021; Accepted: 16 September 2021;
Published: 15 October 2021.
Edited by:
Johannes Spaethe, Julius Maximilian University of Würzburg, GermanyReviewed by:
David Roubik, Smithsonian Tropical Research Institute, PanamaJohannes Stökl, University of Bayreuth, Germany
Bjorn Bohman, Swedish University of Agricultural Sciences, Sweden
Copyright © 2021 Brandt, Dötterl, Ramírez, Etl, Machado, Navarro, Dobler, Reiser, Ayasse and Milet-Pinheiro. This is an open-access article distributed under the terms of the Creative Commons Attribution License (CC BY). The use, distribution or reproduction in other forums is permitted, provided the original author(s) and the copyright owner(s) are credited and that the original publication in this journal is cited, in accordance with accepted academic practice. No use, distribution or reproduction is permitted which does not comply with these terms.
*Correspondence: Katharina Brandt, a2F0amJyYW5kdEBnbWFpbC5jb20=