- 1Department of Entomology, The Pennsylvania State University, University Park, PA, United States
- 2Jacob Blaustein Institutes for Desert Research, Mitrani Department of Desert Ecology, Ben-Gurion University of the Negev, Midreshet Ben-Gurion, Israel
- 3Department of Ecology and Evolutionary Biology, University of Kansas, Lawrence, KS, United States
Social animals are expected to experience a positive effect of conspecific number or density on fitness (an Allee effect) because of the benefits of group living. However, social animals also often disperse to live either solitarily or in small groups, so to understand why social animals leave their groups it is necessary to understand how group size affects both average fitness and the expected fitness outcomes of individuals. We examined the relationships between group size and fitness in the colonial spider Cyrtophora citricola using long-term observations of colony demographics. We censused colonies, recording the number of juveniles, large females, and egg sacs, approximately every 2 months for 2 years. We also recorded the substrates supporting colony webs, including plant species and size, and the azimuth the colony occupied on the plant. Colonies in all regions showed cyclical patterns of growth and decline; however, regions were not synchronized, and seasonal effects differed between years. Colonies with fewer individuals at the initial observation were less likely to survive over the course of observations, and extinction rates were also influenced by an interaction between region and plant substrate. Small colonies were more likely to be extinct by the next census, but if they survived, they were more likely to have high growth rates compared to larger colonies. Despite the potential for high growth rates, high extinction rates depressed the average fitness of small colonies so that population growth rates peaked at intermediate colony sizes. Variance in egg sac production also peaked at intermediate colony sizes, suggesting that competitive interactions may increase the uneven distribution of resources in larger groups. Even if average fitness is high, if spiders can anticipate poor outcomes in large colonies, they may disperse to live solitarily or in smaller, less competitive groups.
Introduction
Societies only evolve when the benefits of living in groups outweigh the substantial costs, such as increased competition and parasite transmission (Alexander, 1974; Krause and Ruxton, 2002). The ubiquity of social organization, from slime molds to humans (Samuelson, 2005; Bonner, 2009), indicates that these benefits, both active (e.g., cooperative prey capture) and passive (e.g., predator dilution), readily accrue in a variety of ecological contexts. However, social organisms face a problem. No environment will remain suitable forever, so all organisms must disperse to colonize new habitats (Méndez et al., 2014). For social organisms, this may necessitate individuals separating from groups and the advantages they provide. Some societies have solved this problem by colony fission and group dispersal (e.g., honey bees; Winston, 1987), but for many others, particularly those with limited control over dispersal (e.g., ballooning spiders), dispersal is a solitary endeavor (Tschinkel and Howard, 1983; Schneider et al., 2001; Chapple, 2003; Schoepf and Schradin, 2012). To understand how these societies grow, decline, and colonize new sites, where they may survive at necessarily low populations, we must understand how group size influences individual fitness, fitness variation, and the probability of group extinction.
An Allee effect is a positive effect of conspecific number or density on fitness or a component of fitness (Stephens et al., 1999). Although expected in any obligate sexual organism (due to a need to find mates), Allee effects are thought to be particularly strong in social species (Angulo et al., 2018), where solitary individuals or small groups may fail to reap the benefits of group living. But if leaving the group entails fitness costs, why then do some individuals of social species disperse to live solitarily or at sub-optimal group sizes? One potential reason is that reproduction within groups is often distributed unevenly (i.e., there is high reproductive skew). Reproductive skew theory predicts when subordinates (individuals expected to have relatively low reproduction in the group) should stay in the group or attempt solitary breeding (Reeve and Shen, 2013). The higher the constraints on solitary breeding, the more likely a subordinate is to stay in the group. But even if the average fitness of the group is high, if the expected payoff for the subordinate is below what could be achieved alone, the subordinate should leave (Reeve and Shen, 2006). In social species that frequently disperse to live alone or colonize new habitats with low populations, we expect two patterns: (1) Allee effects will increase average individual fitness with increasing group size (at least up to a point), and (2) reproductive skew will be higher in larger groups, as antagonistic interactions between dominant and subordinate individuals increase with increasing density. If groups provide both higher average fitness and egalitarian fitness allocation, there would be little reason to disperse and lose fitness advantages. This is particularly true in non-kin societies with reduced risk of inbreeding and kin competition, both of which can promote dispersal (Bowler and Benton, 2005).
We tested these hypotheses in a colonial tent-web spider, Cyrtophora citricola, using long-term monitoring of natural colony demographics. Like other colonial spider species, C. citricola spiders preferentially live in aggregations of webs built, maintained, and defended by individual spiders (Uetz and Hieber, 1997; Mestre and Lubin, 2011; Yip et al., 2017). There is little cooperative prey capture or brood care, but conspecifics are tolerated on support threads that connect neighboring webs (Uetz and Hieber, 1997). Despite little active cooperation, spiders may derive several benefits from grouping. In C. citricola, prey capture efficiency increases with colony size (Rypstra, 1979, but see Leborgne et al., 1998). While not conclusively demonstrated in C. citricola, other colonial spider species benefit from early warning of predators (Uetz and Hieber, 1997), protection from predators via the “selfish herd” (Rayor and Uetz, 1993), and reduced prey capture variance (Caraco et al., 1995; Uetz, 1996). Although some studies have examined the relationship between colony size and fitness in colonial spider species (e.g., Uetz and Hieber, 1997) and others have observed colonies over the course of several months (e.g., Rayor and Uetz, 1993; Grinsted et al., 2019), no study has documented long-term colony growth and decline, so it remains unclear how proposed costs and benefits of group living play out for the dynamics of group size.
Cyrtophora citricola colonies can comprise up to several thousand individuals, but individuals are also found singly (Mestre and Lubin, 2011). Colonies in a semi-natural environment grew by a combination of natal philopatry and immigration (Yip et al., 2019), and genetic analyses indicated considerable variation in genetic structure of natural colonies (Johannesen et al., 2012). In nature, spiders probably encounter a combination of kin and non-kin, and juvenile dispersal—particularly within a limited range—is common (Johannesen et al., 2012; Yip et al., 2019). Thus, C. citricola spiders can choose to stay in established colonies or disperse to live in smaller groups and potentially colonize new areas. Previous work on this species found that dispersal behavior is negatively density dependent, suggesting that Allee effects influence dispersal decisions (Ventura et al., 2017). In addition to local dispersal, C. citricola has successfully colonized multiple locations in North and South America and Caribbean islands from its native Asian, African, and circum-Mediterranean range (Chuang and Leppanen, 2018). Despite potentially strong Allee effects, its history of invasion success suggests that C. citricola spiders can have high fitness at small population sizes.
We tested whether high reproductive skew in larger colonies might explain dispersal to smaller groups or solitary living by censusing natural colonies repeatedly over two years. If Allee effects are driving colony dynamics and spider fitness, we predicted that colony growth and average reproduction would peak at large or intermediate colony sizes. We also predicted that variation in reproduction should increase with colony size, as increased interaction between spiders of differing competitive ability results in greater reproductive skew. If individuals can anticipate poor outcomes, high reproductive skew in colonies with high average fitness may explain dispersal to live solitarily or in smaller, less competitive groups. To understand how C. citricola invades new environments, we also measured Allee effects at the population level. Because there is little interaction between sessile colonies at a site, we predicted that Allee effects at the group level may not carry over to the population, i.e., small populations may grow as well as larger populations. We also investigated factors that influence the rate of colony extinction and recolonization. Larger colonies may be resistant to extinction, and recolonization rates provide a measure of dispersal to suitable substrates. To take into account local conditions, we assessed how seasonality and substrate features may affect group size and colony dynamics.
Materials and Methods
Cyrtophora citricola is a colonial araneid that builds a horizontal orb web, with support threads above and below that may connect to the substrate or other neighboring webs. Unlike most other araneids, the web of C. citricola lacks sticky glue droplets. Under laboratory conditions, females mature 150–200 days after hatching, while males mature after only 60–80 days (Yip and Lubin, 2016). After maturing, females can live another 300 days in the laboratory. The much smaller males (2–2.5 mm body length, compared to 8 mm for females) are usually eaten by females after their first copulation (Yip et al., 2016), but if they do not mate, they can live over 200 days after maturity (Yip and Lubin, 2016). Juveniles are tolerated in support threads of their mother’s web, but mothers do not provision offspring or discriminate against unrelated juveniles (Blanke, 1972; Yip et al., 2019). To our knowledge, data reported here are the first to describe the seasonal life cycle of C. citricola.
We selected seven sites across southern Israel, along an east to west gradient (Figure 1A and Table 1). We divided sites into three regions: West Negev (Shuva, Bessor and the central Negev sites of Beer Sheva and Retamim), East Negev (Nahal Gov and Mishor Yamin), and the Arava Valley. At each site, we flagged C. citricola colonies and solitary individuals for repeated observations (total colony n = 126). Censuses began in either November or December, 2012 and were repeated approximately every two months for two years (see Supplementary Table 1 for all census dates).
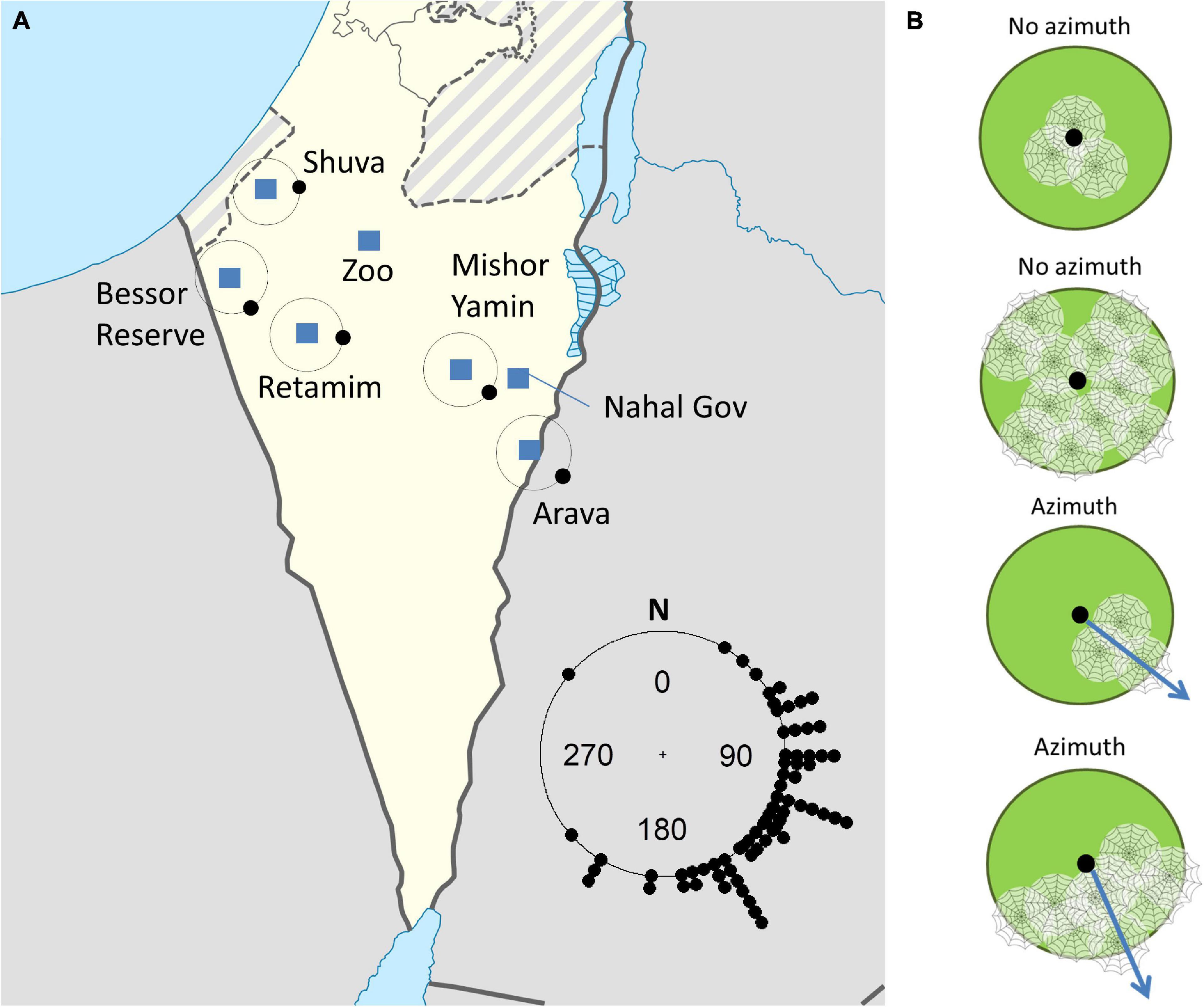
Figure 1. (A) A map indicating the location of census sites (squares) and the average azimuth of colonies at each site (indicated by the black dot on the circle). No colony had a distinct azimuth at the Beer Sheva Zoo (Zoo), and Nahal Gov only had two colonies with distinct azimuths (average 160 degrees off north). The inset circle shows the azimuth of all colonies. (B) How azimuth was measured: the green circles represent substrates with the black dot indicating the substrate’s center. Colonies (web icons) in the center or occupying the entire substrate had no azimuth. For large colonies that spanned a range of degrees, we took the azimuth (blue arrows) from the middle of the span.
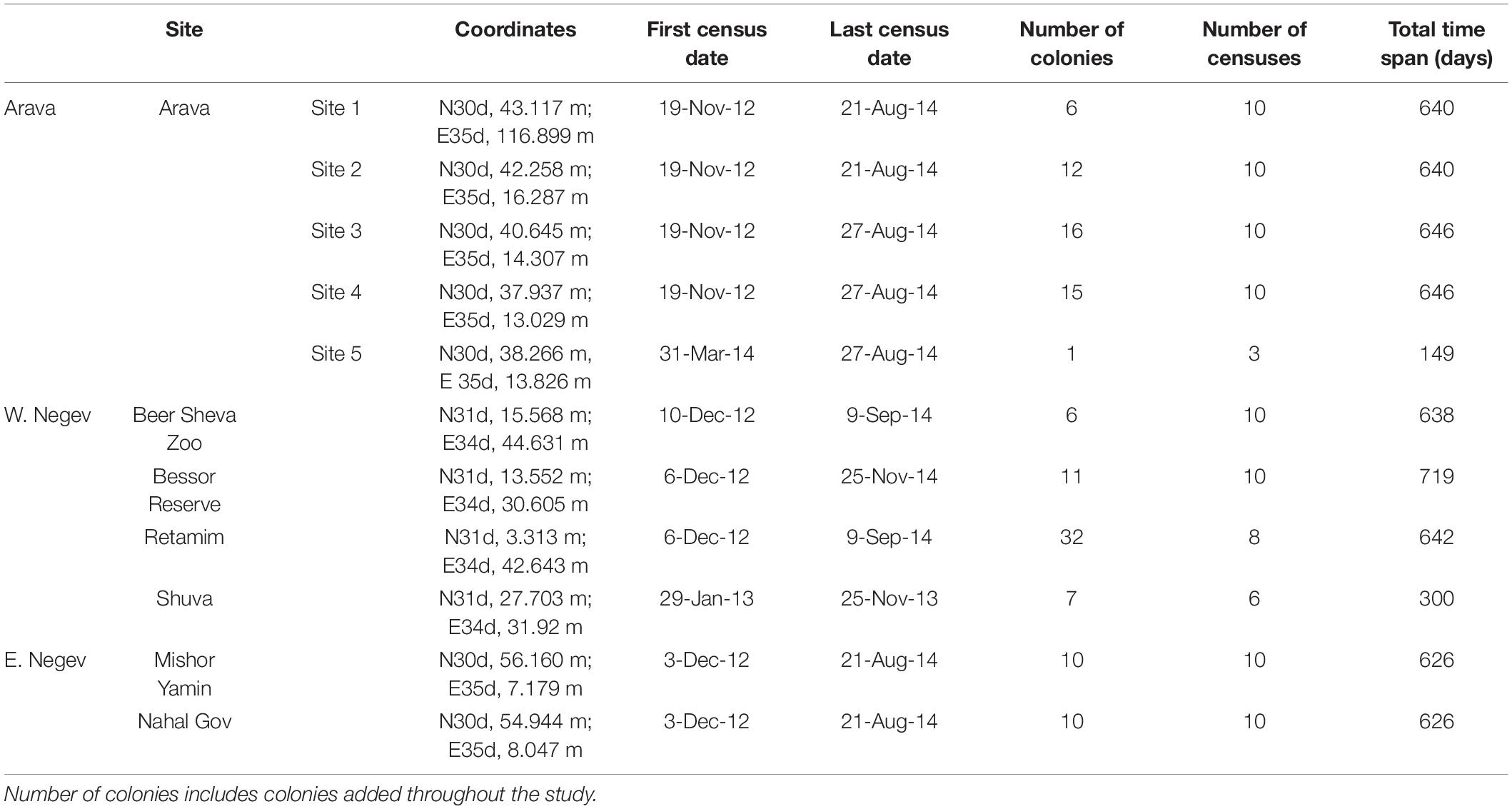
Table 1. Census locations, total observation times, and number of colonies observed by site and region.
At each census, we counted the number of spiders in every flagged colony and added new colonies if they appeared within the census area. Note that “colony size” throughout refers to the number of spiders in the colony, whereas “web volume” indicates the physical dimensions of the colony. For simplicity, we also use “colony” to refer to both groups of spiders and singletons (a group size of one). We counted adult females guarding egg sacs, large females that were either subadults (last pre-adult instar) or adults that did not have egg sacs, and juveniles or males. Because males are so small, they are easily mistaken for juveniles from a distance, so males and juveniles could not be reliably distinguished (Yip and Lubin, 2016). However, based on colonies we could observe closely, the vast majority of small spiders were juveniles. We recorded the number of egg sacs guarded by females. We also recorded unguarded egg sacs, but these appeared old and empty and were not included in our analyses. For small groups, we counted every individual. For large colonies (estimated population sizes >150) or for portions of colonies that we could not get close enough to census, we censused accessible portions of the colony using a 0.125 m3 frame (0.5 m × 0.5 m × 0.5 m) and counted all individuals in the framed volume. We sampled areas with similar web density to uncensused portions of the colony at 3–6 locations depending on the size of the colony, with frames spaced evenly along the colony. We then used tape measures and extendable poles (for elevated areas) to estimate the rectangular volume of uncensused web. We estimated the total number of spiders in the colony by multiplying the total volume of web by the spider density in the sampled areas.
In addition to spiders and their egg sacs, for the first census of every colony we recorded the colony substrate (usually plant genus), the size of the substrate (usually plant crown area), and the azimuth, or compass bearing of the colony’s position around the center of the substrate (Figure 1B). We categorized substrates as Vachellia (V. tortilis, V. raddiana, or V gerrardii), “cactus,” of which Pitaya (Stenocereus spp.) was most common, clementine trees, or “shrub” (most other plant genera).
Analyses
All analyses were conducted using R version 4.0.3. We compared colony azimuth among sites using circular ANOVA followed by pairwise comparisons and tested whether orientations differed from random using Rao’s spacing test of uniformity (using package “circular”).
To analyze the effect of season on colony demographics, we categorized seasons as winter (December–February), spring (March–May), summer (June–August), and fall (September–November). We standardized colony size for each colony by subtracting the mean colony size from each observation and dividing by the standard deviation, so that a value of zero indicated the long-term average size of a particular colony. Positive values indicated the colony was larger than its long-term average, and negative values indicated that it was smaller. Standardization prevented changes in the demographics of large colonies from swamping the effects of smaller colonies. We then examined how standardized numbers of individuals varied by season, year, region, and age class of spider (females with eggs, large females, and juveniles plus males) using linear mixed models with colony ID as a random effect to account for repeated measures over time (“lmer” function in the package “lme4”). We took P-values from likelihood ratio tests by comparing the full model to the model without the fixed effect of interest.
We used right-censored data and Cox proportional hazard models to analyze colony extinction and recolonization (“coxph” function in the “survival” package). We tested whether risk of extinction correlated with colony size at the first observation, substrate size and type, region, and the number of degrees off the preferred azimuth for the site. Note that we divided our sites a priori into three regions (Table 1); where region had significant effects, we also looked at the effect of site to see if particular sites were driving these patterns. We used similar models to examine time to recolonization for colonies that had gone extinct, where the time to the event was measured from disappearance of spiders to reappearance at a flagged colony. We tested whether chances of recolonization correlated to substrate size and type, previous colony size (as a proxy for web remnants), and region. For continuous variables and categorical variables with only two levels, we used z-tests to calculate P-values. For categorical variables with more than two levels, we used likelihood ratio tests by comparing the full model to the model without the effect of interest.
We examined how colony size correlated with colony growth in two ways. We examined the average number of egg sacs per female (both females guarding egg sacs and large females without egg sacs) by the number of females in the colony using linear mixed models. We included colony ID as a random effect (both intercept and slope with respect to colony size) to account for repeated observations over time, and we included season, year, and their interaction as covariates to account for seasonality (see section “Results”). The average number of sacs per female and total number of females were natural log transformed to normalize residuals. We also examined the percent change in total number of spiders from one census to the next. As with the egg sac model, we included colony ID as a random effect, included year, season, and their interaction as covariates, and natural log transformed data to normalize residuals. We used a similar model to test for population-level Allee effects, but instead of examining changes in colony size, we analyzed how the total population of a site correlated with the change in site population size between censuses.
Finally, to examine how colony size correlated with variation in reproduction, we calculated the coefficient of variation (CV) of egg sac production among females within each colony for each census date. Singletons were sufficiently rare that many dates had only one singleton with eggs, so to measure the variation in reproduction for singletons, we calculated the CV for all singletons at each site across dates. We then used linear mixed models to test for correlations between CV and either the number of large females in the colony (females with and without eggs) and only females that were guarding egg sacs. Colony ID was a random effect with both intercept and slope with respect to the number of females in the colony, and year and season were included as covariates.
Results
Substrate Size, Type, and Azimuth
We found colonies on a variety of trees, shrubs and cacti. In undisturbed sites, C. citricola colonies occupied Vachellia spp. trees and shrubs of Hammada spp. Less frequently, colonies occupied shrubs of the genera Salsola, Prosopis, Anabisis, Ochradenus, Retama, Haloxylan, Atriplex, Atractylis, and Zygophyllum (in order of decreasing frequency; see Supplementary Table 2). In agricultural and disturbed areas, colonies were found on planted Pitaya (Stenocereus spp.) and other cacti, Vachellia gerrardii, clementine trees, and Juniperus sp. bushes. While colonies occasionally spanned more than one plant, these plants were always very close together. Colony webs did not span wide spaces between substrates, resulting in a correlation between substrate size and colony size (linear model: df = 123, t = 6.8, P < 0.0001, R2 = 0.27; Figure 2). Colony sizes varied from singletons to an estimated 28,400 spiders occupying a Vachellia tortilis tree with a crown area of 222 m2 and an estimated total web volume of 205 m3. The overall average azimuth of colonies was southeast (119° clockwise off north), and this preference was significant (Rao’s spacing test: P < 0.001; Figure 1A). Average azimuth differed among sites (circular ANOVA F5,69 = 4.5, P = 0.001), with pairwise comparisons indicating that Retamim and Shuva had colonies oriented more toward the east compared to other sites (Figure 1A).
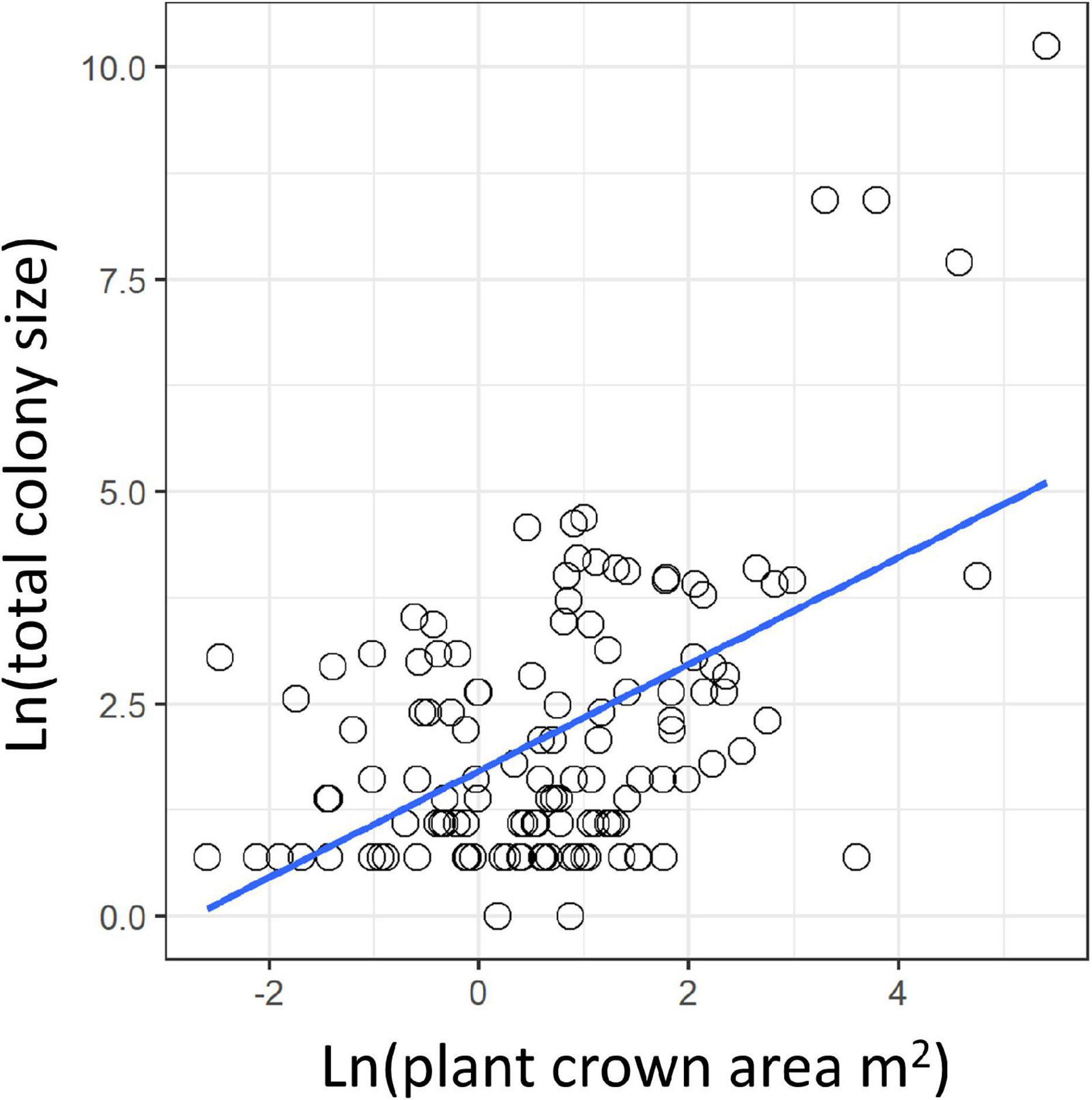
Figure 2. The correlation between substrate size (plant crown area) and the total number of spiders in the colony.
Seasonality
There was a significant interaction between season, year, region and spider age class (Linear mixed model: χ2 = 43, df = 8, P < 0.0001). Colonies were large at the start of observations at the end of 2012 (Figure 3), then declined in late winter and early spring of 2013. Colonies increased in size again in the spring and summer of 2014, except in the West Negev. The West Negev decline reflects the sharp population decline at the Bessor Reserve site, possibly due to pesticide application in adjacent citrus groves. Reproductive females peaked in late spring and summer, with the West Negev peaking earlier than the East Negev and Arava regions. These peaks in egg sac production were followed by increases in juvenile populations 2–3 months later (Figure 3).
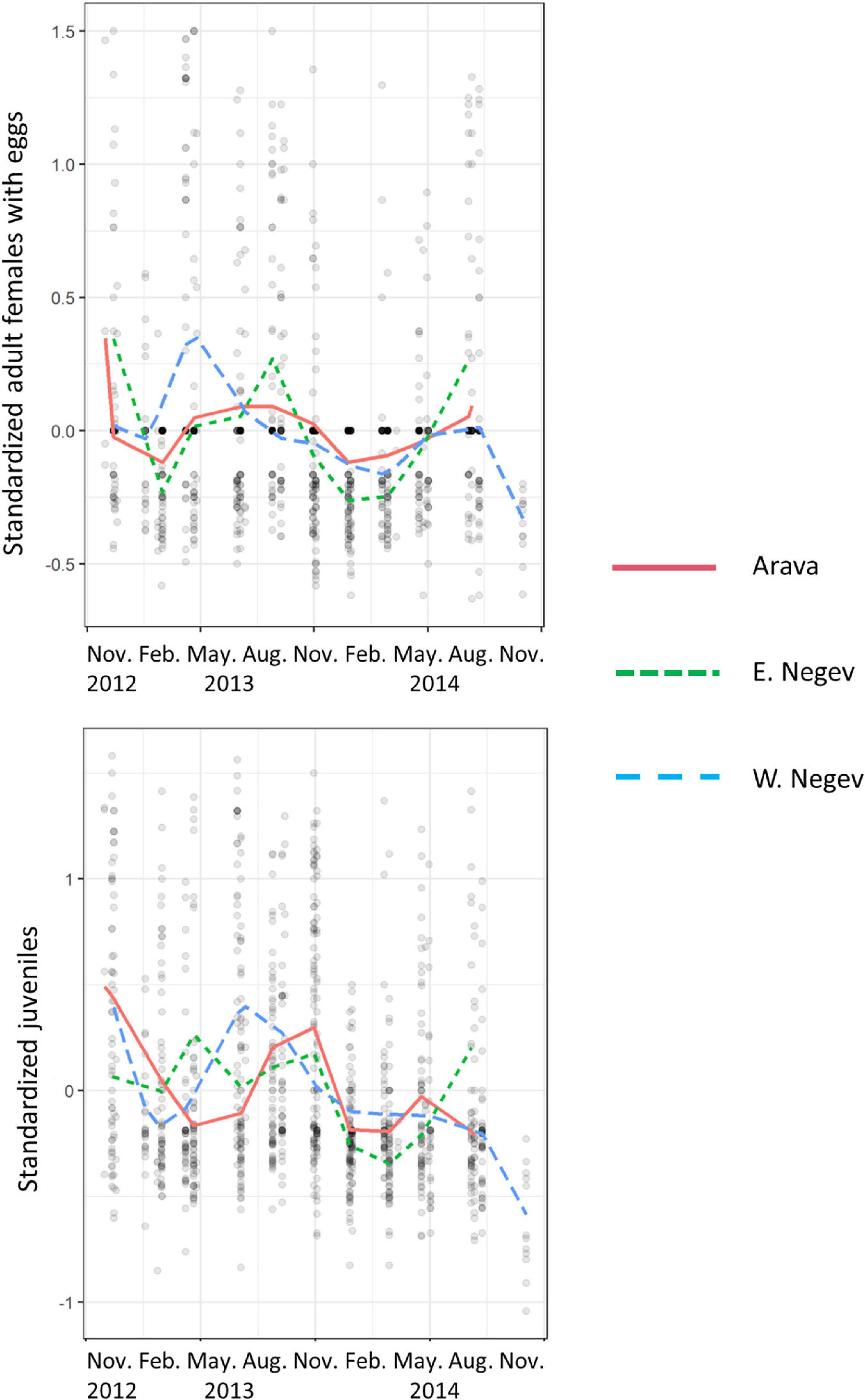
Figure 3. Seasonal fluctuations in standardized colony size, separated by region and spider age class (adult females with eggs and juveniles). Curves are uniform splines of 8 knots.
Extinction and Recolonization
Ninety-five colonies (78%) went extinct during the observations, and we observed 66 recolonizations of flagged colonies that had gone extinct. Including these recolonizations, a total of 134 colonies went extinct and 40 survived to the end of the observation period. Even colonies that did not go extinct often experienced a population collapse, with a >90% reduction in population. Out of 174 colonies (including recolonizations), a total of 150 (86%) collapsed or went extinct. When considered alone, both deviation from the preferred azimuth and smaller substrates correlated positively with increased extinction risk (azimuth deviation Cox P.H.: z = 2.9, P = 0.004; substrate size Cox P.H.: z = 2.1, P = 0.03). However, the center of larger colonies tended to be closer to the preferred azimuth of the site, and substrate size also correlated with colony size (Figure 2) so that these effects disappeared when initial colony size was added to the model. Considered together, initial colony size, region, and substrate type all influenced time to extinction. Larger colonies had a much lower risk of extinction (Cox P.H.: χ2 = 25.2, df = 1, P < 0.0001; Figure 4A). Substrate type interacted with region (Cox P.H.: χ2 = 20.5, df = 1, P < 0.0001; Figure 4B), which indicated that while colonies on shrubs performed similarly across regions, colonies on Vachellia trees survived better in the Arava than in the West Negev (Figure 4B). This was because colonies in a row of planted V. gerrardii nearly all went extinct at the Retamim site, while most colonies on V. tortilis or V. raddiana in the Arava region survived.
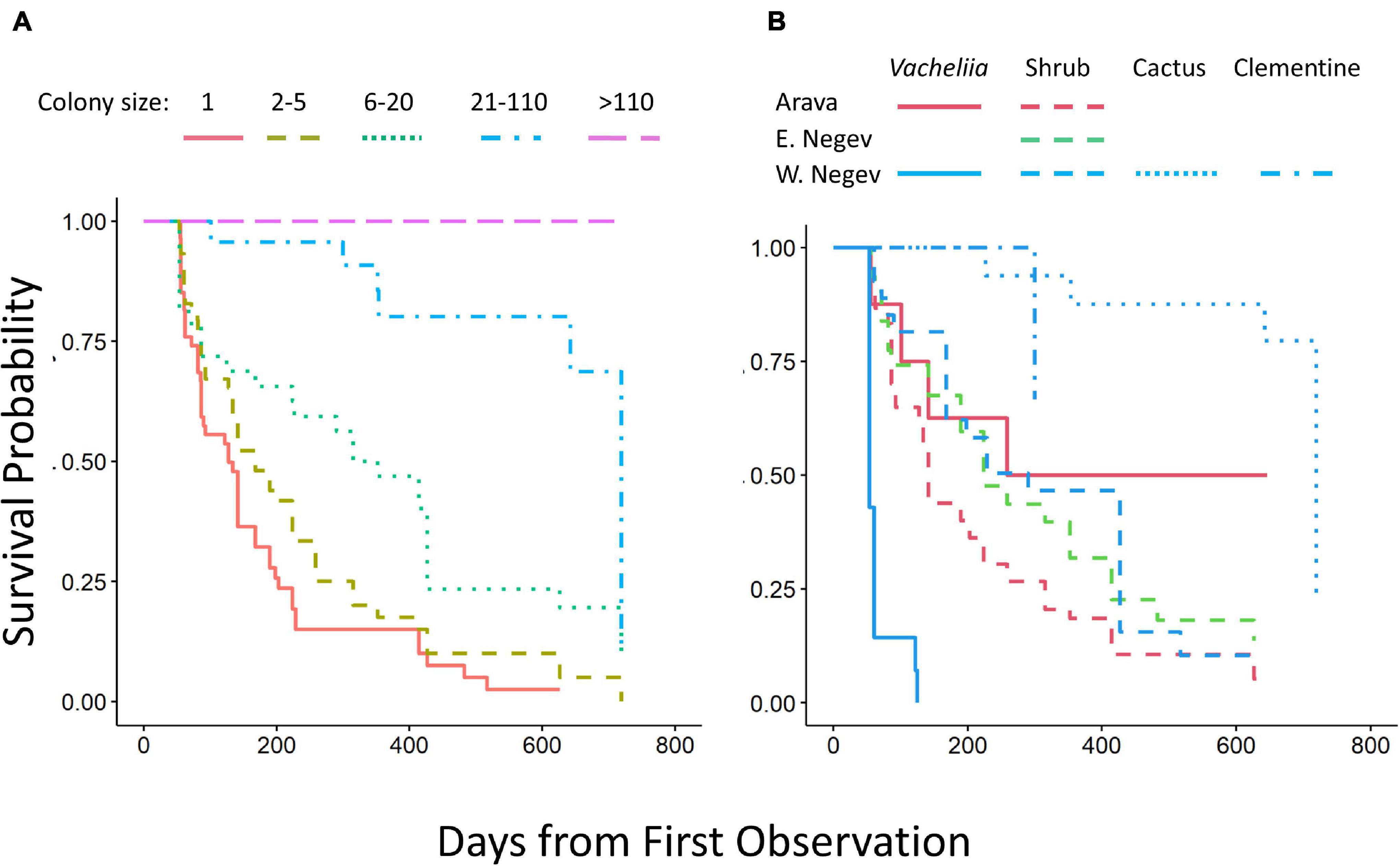
Figure 4. Kaplan-Meier survival curves for C. citricola colonies by initial colony size (A) and by substrate and region (B).
The rate of recolonization was not affected by substrate size nor by previous colony size (Cox P.H.: substrate size z = −0.07, P = 0.95; previous colony size z = 1.02, P = 0.31). When considered by itself, substrate type influenced recolonization rate, with colonies on shrubs being recolonized sooner than those on Vachellia (Cox P.H.: χ2 = 10.1, df = 2, P = 0.006). However, this effect disappeared when considered alongside region (Cox P.H.: χ2 = 2.3, df = 2, P = 0.32). Colonies that had gone extinct were more likely to be recolonized in the Arava and East Negev, compared to the West Negev (Cox P.H.: χ2 = 17.4, df = 2, P = 0.0002; Figure 5A). This effect was largely driven by the Retamim site, where recolonizations were rare (Figure 5B).
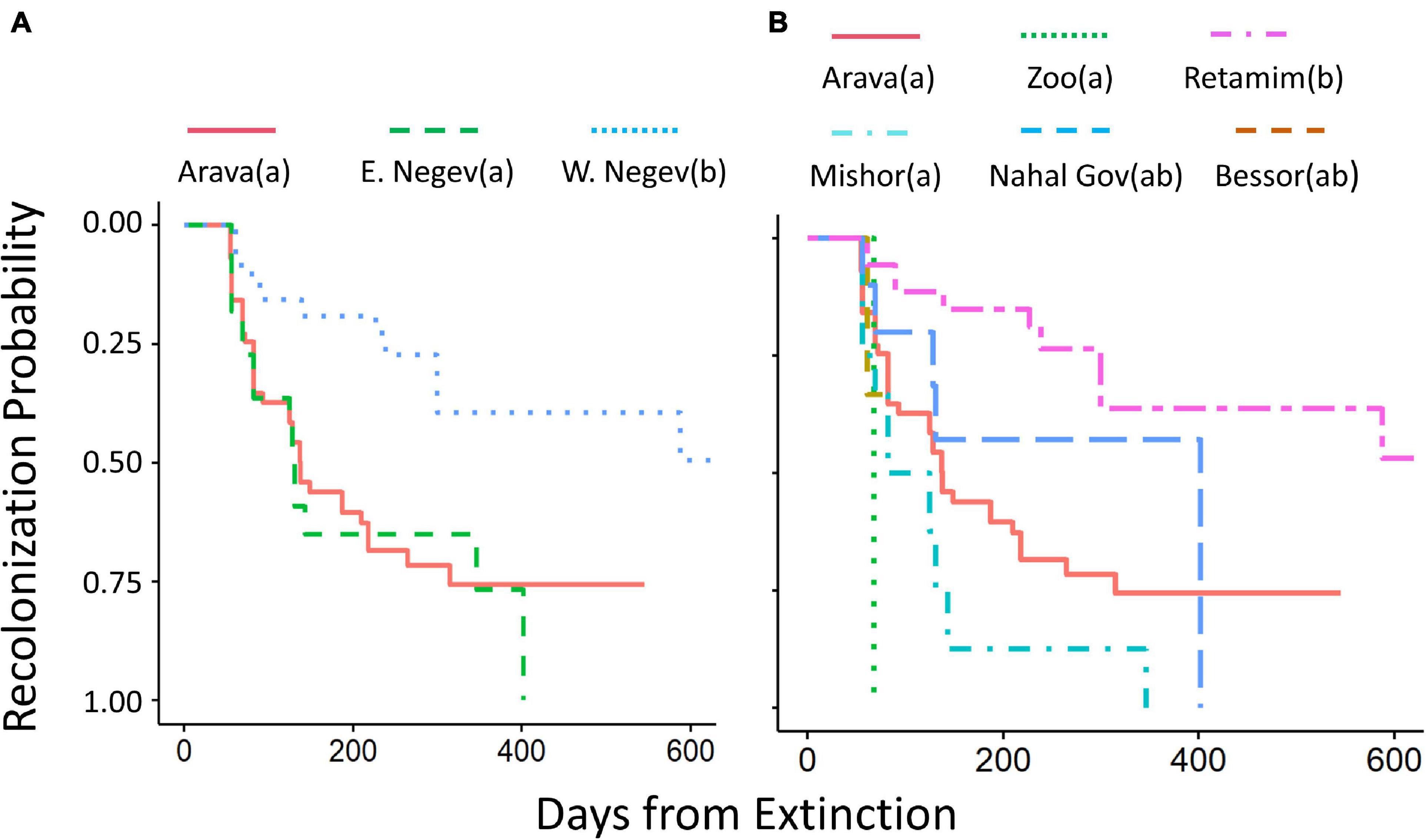
Figure 5. Cumulative recolonization probability by region (A) and site (B) versus the time elapsed (days) from extinction of the previous colony. Significant differences among curves are denoted by different letters in parentheses in the line legend. Pesticide application caused a population collapse at Shuva, and we ended observations at that site before any colonies went extinct.
Relationships Between Colony Size and Colony Growth
Colonies with fewer females were more likely to either have a high average number of egg sacs per female or no egg sacs at all (Figure 6). For only those colonies that produced at least one egg sac, sacs per female decreased with increasing numbers of females (Linear mixed model: χ2 = 33, df = 1, P < 0.0001; Figure 6B). When colonies that failed to reproduce are included, sacs per female increased with colony size (Linear mixed model: χ2 = 9.1, df = 1, P = 0.003), and there was a non-significant quadratic effect (Linear mixed model: quadratic term χ2 = 3.5, df = 1, P = 0.06; Figure 6A). Similarly, smaller colonies in terms of total number of spiders were more likely to either grow between censuses or go extinct (Figure 7A). A significant quadratic effect indicated an optimal intermediate group size of about 185 spiders (Linear mixed model: number of spiders χ2 = 14.6, df = 1, P = 0.0001; number of spiders squared χ2 = 6.1, df = 1, P = 0.013; Figure 7A). There was no interaction between colony size and study site, indicating that the humped relationship was consistent across sites (Linear mixed model: number of spiders∗site χ2 = 10.3, df = 10, P = 0.42; number of spiders squared∗site χ2 = 9.1, df = 10, P = 0.52). Over the two-year study, spider populations declined overall, so even at the optimal group size, the average colony decreased by 34% from its previous size at the next census. Most colonies that grew from one census to the next were small to medium sized (Figure 7A).
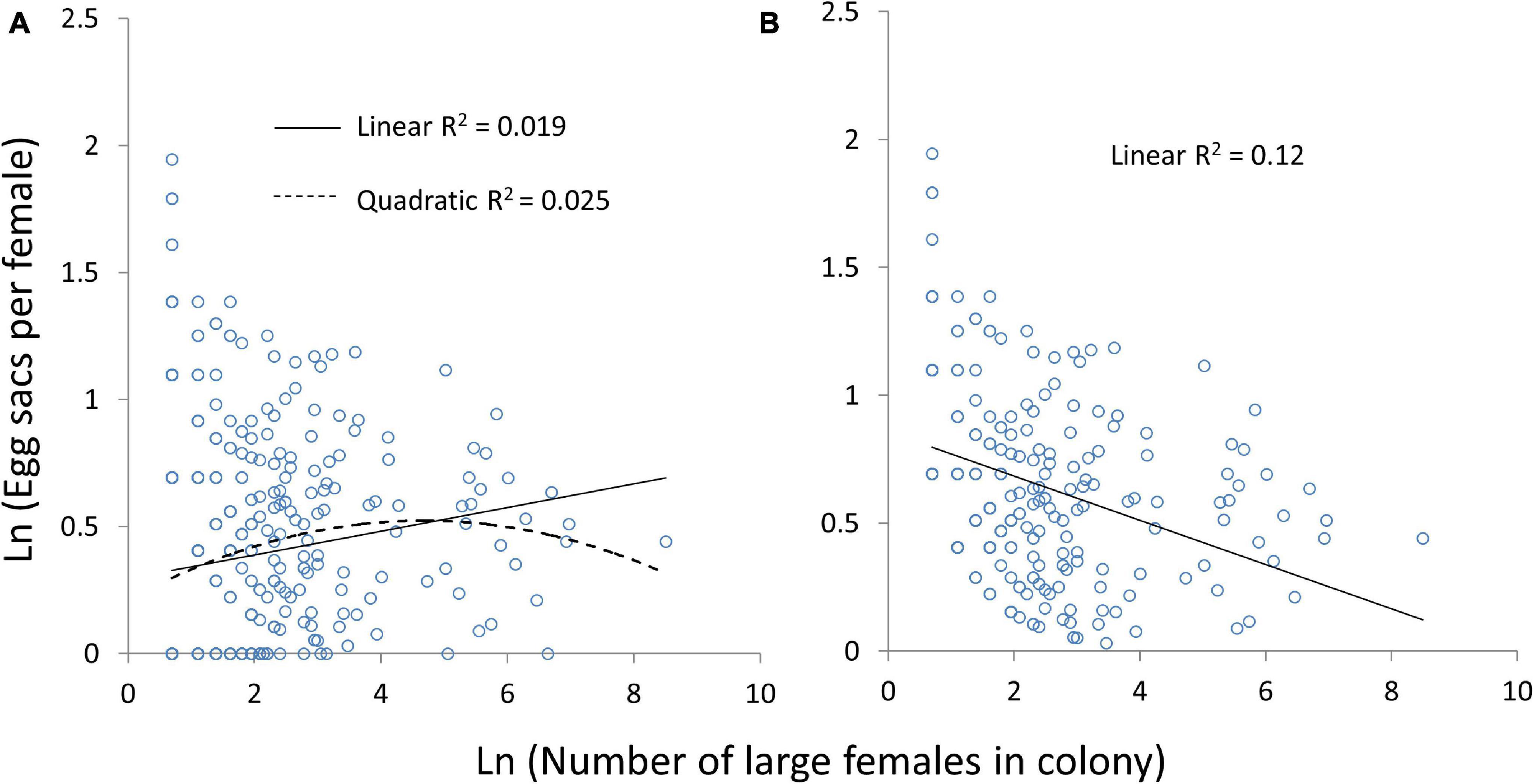
Figure 6. The correlation between the number of adult or subadult females in the colony and the number of egg sacs per female. All colonies are included in panel (A), while colonies that failed to reproduce at all are excluded in panel (B). Significant linear correlations are indicated by solid lines, while a non-significant quadratic effect is indicated by a dashed line.
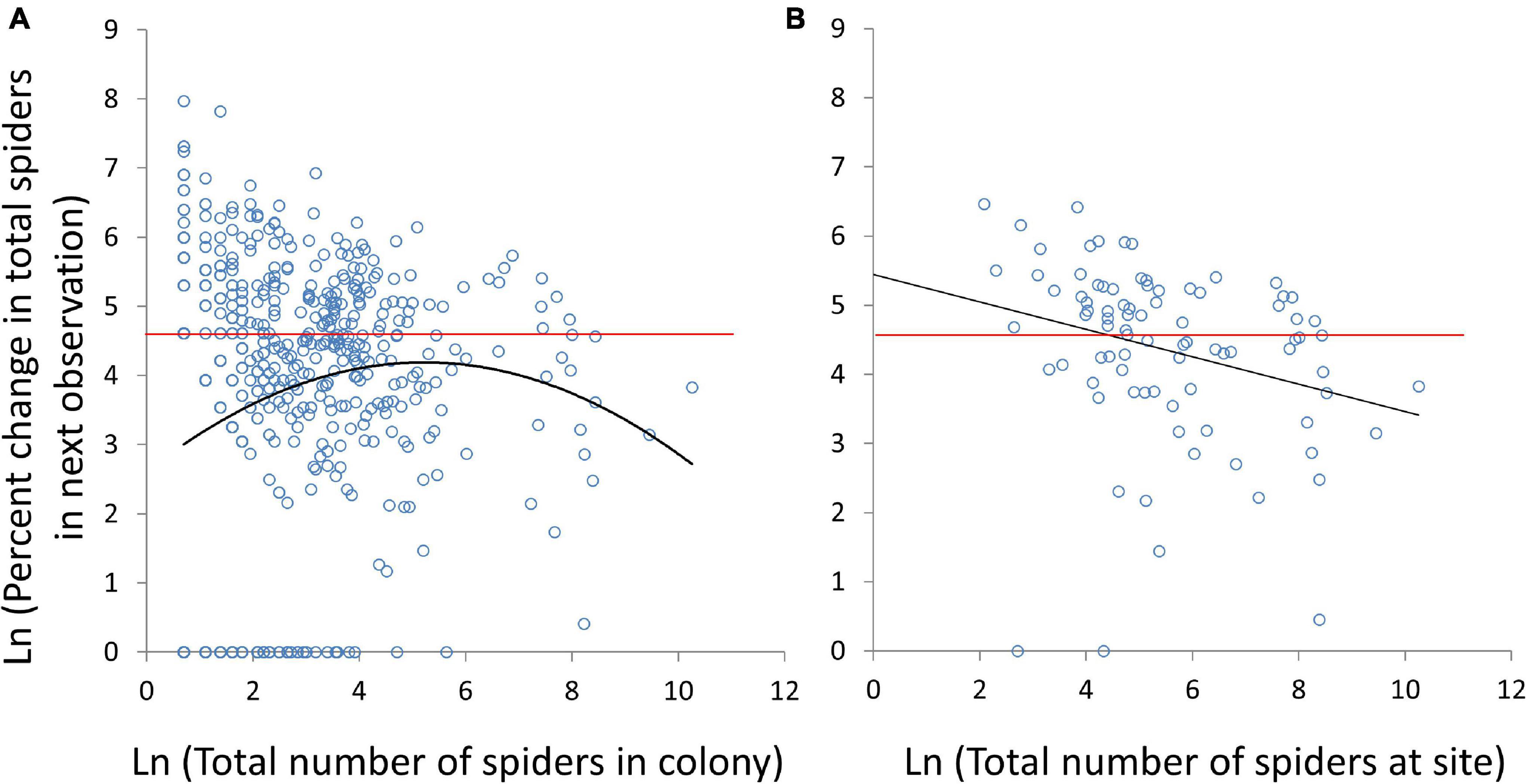
Figure 7. The relationship between numbers of spiders in the colony (A) or population (i.e., at a particular site) (B) and the percent change in number in the next census. Significant quadratic and linear effects are sown in solid black lines. No change in colony or population size (Loge 100% = 4.6) is shown with a red line.
Although we detected a positive relationship between the percent increase and colony size in the previous census (i.e., an Allee effect) at the colony level (Figure 7A), we did not detect a population-level Allee effect (Figure 7B). Instead, population growth in the next census monotonically decreased with population size (Linear mixed model: χ2 = 5.3, df = 1, P = 0.02).
Variation in egg sac production also peaked at intermediate colony sizes (Figure 8). The largest single chain of egg sacs (a single chain represents the reproductive output of a single female) was 9 sacs. Only two singletons produced chains of 5, and only one singleton produced a chain of 6. All chains larger than 6 were produced by females living in multi-female groups. The pattern was consistent regardless of whether we examined all large females, some of which may not have reached adulthood (Linear mixed model: number of spiders χ2 = 31.9, df = 1, P < 0.0001; number of spiders squared χ2 = 16.4, df = 1, P < 0.0001; Figure 8A), or only those females guarding egg sacs (Linear mixed model: number of spiders χ2 = 83.2, df = 1, P < 0.0001; number of spiders squared χ2 = 28.5, df = 1, P < 0.0001; Figure 8B).
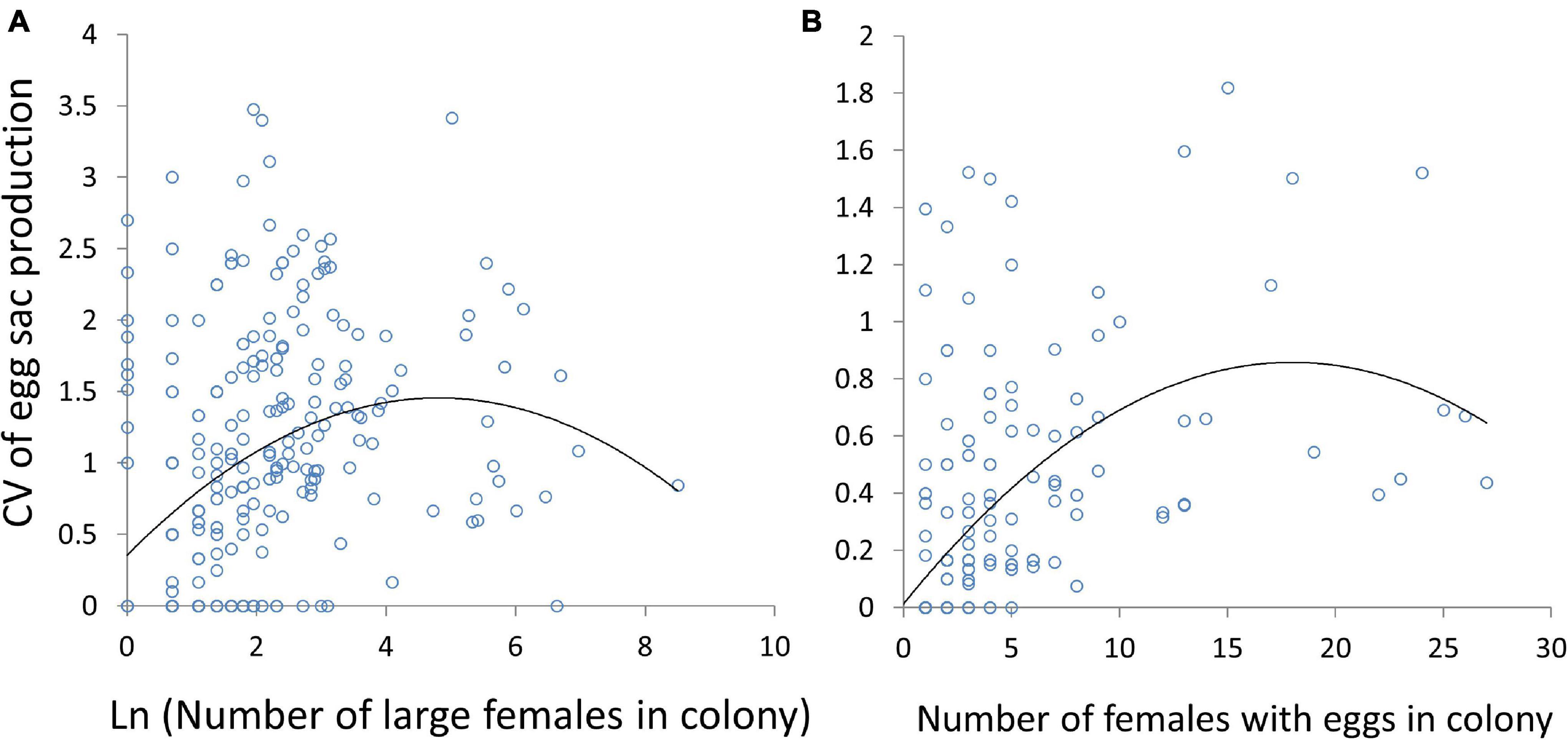
Figure 8. The relationship between the coefficient of variation (CV) of egg sac production within colonies and colony size, measured as the total number of large females in panel (A) and only females with egg sacs (B). CVs for singletons were derived from all singletons within a site. Significant quadratic effects are shown in solid black lines.
Discussion
We examined changes in colony demographics over two years in the colonial spider C. citricola to understand how colony size relates to fitness and population growth. Colony orientation largely corresponded to the lee side of the substrate, as winds come off the Mediterranean moving east and then head more toward the south in the Arava Valley (Shemer, 1986). The exception was the Bessor Reserve (Figure 1A), which is in a river valley that runs north to south. Reproduction declined in winter in all regions, but reproduction peaked earlier in western populations compared to the Eastern Negev and Arava Valley (Figures 1A, 3). In other colonial spider species, wetter habitats are linked to faster development (Fernández Campón, 2010), which may also be the case here. Although there were seasonal cycles of growth and decline, overall spider populations decreased over our observations, with frequent colony extinctions and population crashes. Large colony size could buffer against colony extinction, but otherwise extinction and recolonization probabilities were idiosyncratic to particular sites. Even though single spiders and small colonies could have high growth and reproduction rates, the high incidence of extinction and total reproductive failure depressed average growth and reproduction below that of larger colonies (Figures 6A, 7A).
Growth peaked at intermediate colony sizes, but the relationship between colony growth and spider fitness is not necessarily simple. Because C. citricola juveniles disperse (Johannesen et al., 2012; Yip et al., 2019), colony size decline could result from a combination of emigration out of the colony and mortality. Over the course of our observations, we added new colonies to censuses. Although some quite large colonies experienced severe population declines, we did not find very large numbers of new webs or colonies and only 66 recolonizations, suggesting that emigration alone cannot account for colony declines. Undoubtedly, some spiders dispersed outside the study area and could not be counted. However, dispersal is very costly, and net house data show that young spiders that disperse usually die before even building a web (Yip et al., 2019). Furthermore, the pattern of colony growth in relation to colony size (Figure 7A) resembled the pattern for egg sac production (Figure 6A), suggesting that colony decline is a consequence of low reproductive rates and therefore low individual fitness. Additionally, large colonies might attract more egg parasites (Uetz and Hieber, 1997), so that egg sac number might be a misleading measure of fitness; however, only 20 of 724 (2.8%) collected egg sacs showed signs of parasitism or predation (see Supplementary Table 3), suggesting that parasitism was not strong enough at these sites to either explain the decline in colony growth at the largest sizes or negate the increase in reproduction (egg sac counts) in mid- to large-sized colonies.
Mid-sized colonies (and perhaps larger colonies; see a quadratic effect Figure 6A) had a higher number of eggs sacs per female, as well as higher variance in egg sac production (Figure 8). Our methods provide snapshots in time, so variation in egg sac production might reflect either differences in reproductive output (and thus fitness) or differences in timing of development, with younger females having not yet reproduced to the same extent as older females. However, we repeatedly observed sites over time, and it is unlikely that mid-sized colonies would consistently have females further along in their reproductive lives than females in larger or smaller colonies. Thus, egg sac variation among colonies likely represents differences in reproductive output. Similarly, within colonies, variation in egg sac production might be due to either asynchronous development of individuals or to variation in reproductive capacity. In either case, increased variation in mid-sized colonies would result from increased variation in prey consumption. Reduced feeding slows development and reduces total reproductive output in C. citricola (Yip and Lubin, 2016), and because all spiders in a colony – and moreover an entire field site—experience similar climatic conditions, nutritional differences are the most likely source of increased variation in development over the underlying level of population asynchrony (i.e., spiders being born at different times).
Thus, it seems likely that average fitness does peak at intermediate colony sizes. This pattern is similar to that seen in cooperative social spiders, such as Anelosimus eximius and Stegodyphus dumicola. In these species, fitness increases with colony size due to very low survivorship of singletons and small groups (Avilés and Tufino, 1998; Bilde et al., 2007). Singletons and small groups could have high reproductive success, but this was offset by poor survivorship, and increased competition in the largest colonies resulted in a humped fitness curve (Avilés and Tufino, 1998; Bilde et al., 2007). Similar patterns were found in subsocial Anelosimus studiosus, where small groups of siblings captured more prey per capita than singletons or larger groups (Jones and Parker, 2000). It seems logical that fitness peaks at intermediate group sizes should be universal, as competition eventually overwhelms the benefits of group living (e.g., VanderWaal et al., 2009; Markham et al., 2015), yet surprisingly this is not always borne out by empirical data (Dornhaus et al., 2012; Rudolph et al., 2019). A review of colony size in social insects found that the relationship between group size and reproductive output could be positive, negative, or neutral, with no detectable intermediate peak (Dornhaus et al., 2012). Nevertheless, our data suggest that the general patterns of high fitness at intermediate groups sizes can apply to vastly different spider societies, exhibiting either highly cooperative behaviors (e.g., A. eximius, A. studiosus, and S. dumicola) or mostly passive benefits to group living in colonial species (e.g., C. citricola).
Reproductive variation increased with colony size and either decreased or leveled-off at the largest colony sizes, depending on whether larger females without egg sacs were included in the analysis (Figure 8). This supports our hypothesis that individuals that anticipate poor reproductive payoffs might leave groups, even if the group has a high average reproductive output. Previous work on C. citricola has demonstrated that conflict and dominance hierarchies are common within colonies. Larger spiders typically win interactions and force smaller spiders into less favorable web positions or prevent web construction altogether (Yip et al., 2017). Spiders may also attempt to usurp existing webs (Rypstra, 1979) or steal prey from smaller spiders (E.C.Y. pers. obs.). Because colony webs are constrained by their substrate (Figure 2), as colonies grow there may be fewer suitable sites for web construction. In larger colonies, subordinate spiders may have difficulty securing favorable web locations or avoiding aggression by larger neighbors. This conflict may then result in increased variation in prey consumption and weight (Ventura et al., 2017), which may then lead to variation in egg sac production (Yip and Lubin, 2016).
Despite the ability to freely disperse, join neighboring groups, or attempt to survive solitarily (Yip et al., 2019), many females in larger colonies either failed to reproduce or produced only one egg sac, while some of their neighbors produced many egg sacs. High variance in reproduction is also observed in cooperatively social spider species, but these species also form highly inbred demes so that average colony fitness likely also reflects individual inclusive fitness (Avilés, 1997). By contrast, C. citricola is outbred with colonies comprising mixtures of kin and non-kin (Johannesen et al., 2012; Yip et al., 2019). Why did C. citricola females with low reproduction stay in the colony? One possible answer is that spiders probably have limited information about their future reproductive prospects. Interestingly, when partial information has been incorporated into reproductive skew models, this has increased independent reproduction away from the groups (Kokko, 2003; Akçay et al., 2012), even when staying might have mutually benefited both dominants and subordinates (Akçay et al., 2012). Neither of these models predicted that limited information would increase grouping (Kokko, 2003; Akçay et al., 2012). These models incorporated uncertainty in the amount of reproduction allotted to the subordinate (Kokko, 2003) or prospects away from the group (Akçay et al., 2012). We hypothesize that C. citricola spiders might face a different kind of uncertainty. Cyrtophora citricola spiders appear to disperse as juveniles (or adult males), while subadult and adult females are largely sedentary (Johannesen et al., 2012; Yip et al., 2019). Dominance in C. citricola spiders, as in many species, is related to size, and spiders may face uncertainly about their final size and dominance (Yip et al., 2017). This seems particularly likely because reduced feeding decreases size at a given molt, but spiders can also increase the number molts to adulthood. Thus, juveniles with poor feeding rates might still obtain large adult sizes (Yip and Lubin, 2016). This uncertainty in dominance may be similar to pleometrosis in ants, where multiple queens found a colony, but only one survives to reap the benefits (Tschinkel and Howard, 1983; Teggers et al., 2021). Queens may be uncertain whether they will be the surviving monarch, and by the time they have reliable information about their chances of success (such as fecundity), it is too late to disperse and live solitarily (Teggers et al., 2021).
While we detected an Allee effect at the level of the colony, we did not detect any Allee affect at the population level. It seems paradoxical that Allee effects at the group level do not translate to the population, and some have suggested that the two levels should mirror each other (Courchamp et al., 2000). However, a recent model predicted a strong correlation between group and population Allee effects only if group size homogeneity was moderate to high (Angulo et al., 2018). Group sizes can be extremely heterogeneous in C. citricola populations, with large colonies surrounded by smaller groups and singletons (Supplementary Figure 1). The mismatch between colony and population Allee effects supports the model’s assumptions that larger groups can buffer Allee effects in smaller groups at the population level (Angulo et al., 2013, 2018).
Interestingly, we found a strong negative correlation between population size and growth in the next census (Figure 7B). In African wild dogs, there is a great deal of group size heterogeneity and groups largely avoid one another, so that there was no relationship between population size and growth rate (Angulo et al., 2013). Because C. citricola colonies are physically attached to their substrates, they do not interact directly, and so we predicted similar independence of population size and growth. The negative relationship could stem from large colonies with poor growth (Figure 7A) driving the dynamics of the overall population. This may explain why C. citricola has been a successful invader despite having higher fitness at intermediate group sizes. If a few founders can survive small colony size (which is not uncommon; see Figures 4A, 7A), the incipient population is likely to do well without large colonies of competing conspecifics.
Data Availability Statement
The raw data supporting the conclusions of this article will be made available by the authors, without undue reservation.
Author Contributions
EY and YL collected and analyzed the data. All authors conceived and designed the project, helped secure funding for the project, and contributed to writing the manuscript.
Funding
Funding was provided by the Jacob Blaustein Center for Scientific Cooperation and the Kreitman School for Advanced Graduate Studies to EY, and the U.S.-Israel Binational Science Foundation, grant No. 2010178 to YL and DS.
Conflict of Interest
The authors declare that the research was conducted in the absence of any commercial or financial relationships that could be construed as a potential conflict of interest.
Publisher’s Note
All claims expressed in this article are solely those of the authors and do not necessarily represent those of their affiliated organizations, or those of the publisher, the editors and the reviewers. Any product that may be evaluated in this article, or claim that may be made by its manufacturer, is not guaranteed or endorsed by the publisher.
Acknowledgments
We thank Iris Musli and Ishai Hoffman for help censusing colonies, and we further thank Iris Musli for dissecting egg sacs. We also thank Ingi Agnarsson, Matjaz Kuntner, and Hema Somanathan for their helpful comments.
Supplementary Material
The Supplementary Material for this article can be found online at: https://www.frontiersin.org/articles/10.3389/fevo.2021.725647/full#supplementary-material
References
Akçay, E., Meirowitz, A., Ramsay, K. W., and Levin, S. A. (2012). Evolution of cooperation and skew under imperfect information. Proc. Natl. Acad. Sci. U.S.A. 109, 14936–14941. doi: 10.1073/pnas.1212925109
Angulo, E., Luque, G. M., Gregory, S. D., Wenzel, J. W., Bessa-Gomes, C., Berec, L., et al. (2018). Review: Allee effects in social species. J. Anim. Ecol. 87, 47–58. doi: 10.1111/1365-2656.12759
Angulo, E., Rasmussen, G. S. A., Macdonald, D. W., and Courchamp, F. (2013). Do social groups prevent Allee effect related extinctions: The case of wild dogs. Front. Zool. 10:11. doi: 10.1186/1742-9994-10-11
Avilés, L. (1997). “Causes and consequences of cooperation and permanent-sociality in spiders,” in Social Behavior in Insects and Arachnids, eds J. C. Choe and B. J. Crespi (Cambridge: Cambridge University Press), 476–498. doi: 10.1017/cbo9780511721953.024
Avilés, L., and Tufino, P. (1998). Colony size and individual fitness in the social spider Anelosimus eximius. Am. Nat. 152, 403–418. doi: 10.1086/286178
Bilde, T., Coates, K. S., Birkhofer, K., Bird, T., Maklakov, A. A., Lubin, Y., et al. (2007). Survival benefits select for group living in a social spider despite reproductive costs. J. Evol. Biol. 20, 2412–2426. doi: 10.1111/j.1420-9101.2007.01407.x
Blanke, R. (1972). Untersuchungen zur okophysiologie und oketheologie von Cyrtophora citricola Forskal (Aranaea, Araneidae) in Andalusien. Forma Funct. 5, 125–206.
Bonner, J. T. (2009). The Social Amoebae The Biology of Celluar Slime Molds. Princeton, NJ: Princeton University Press.
Bowler, D. E., and Benton, T. G. (2005). Causes and consequences of animal dispersal strategies: relating individual behaviour to spatial dynamics. Biol. Rev. Camb. Philos. Soc. 80, 205–225. doi: 10.1017/S1464793104006645
Caraco, T., Uetz, G. W., Gillespie, R. G., and Giraldeau, L. A. (1995). Resource consumption variance within and among individuals: on coloniality in spiders. Ecology 76, 196–205. doi: 10.2307/1940641
Chapple, D. G. (2003). Ecology, life-history, and behavior in the Australian scincid genus Egernia, with comments on the evolution of complex sociality in lizards. Herpetol. Monogr. 17, 145–180. doi: 10.1655/0733-1347(2003)017[0145:elabit]2.0.co;2
Chuang, A., and Leppanen, C. (2018). Location and web substrate records of Cyrtophora citricola (Araneae: Araneidae) in southern Spain, including Tenerife. Rev. Ibérica Aracnol. 33, 89–100.
Courchamp, F., Clutton-Brock, T., and Grenfell, B. (2000). Multipack dynamics and the Allee effect in the African wild dog, Lycaon pictus. Anim. Conserv. 3, 277–285. doi: 10.1017/S1367943000001001
Dornhaus, A., Powell, S., and Bengston, S. (2012). Group size and its effects on collective organization. Annu. Rev. Entomol. 57, 123–141. doi: 10.1146/annurev-ento-120710-100604
Fernández Campón, F. (2010). Cross-habitat variation in the phenology of a colonial spider: insights from a reciprocal transplant study. Naturwissenschaften 97, 279–289. doi: 10.1007/s00114-009-0640-8
Grinsted, L., Deutsch, E. K., Jimenez-Tenorio, M., and Lubin, Y. (2019). Evolutionary drivers of group foraging: a new framework for investigating variance in food intake and reproduction. Evolution 73, 2106–2121. doi: 10.1111/evo.13826
Johannesen, J., Wennmann, J. T., and Lubin, Y. (2012). Dispersal behaviour and colony structure in a colonial spider. Behav. Ecol. Sociobiol. 66, 1387–1398. doi: 10.1007/s00265-012-1394-4
Jones, T. C., and Parker, P. G. (2000). Costs and benefits of foraging associated with delayed dispersal in the spider Anelosimus studiosus (Araneae, Theridiidae). J. Arachnol. 28, 61–69. doi: 10.1636/0161-8202(2000)028[0061:cabofa]2.0.co;2
Kokko, H. (2003). Are reproductive skew models evolutionarily stable? Proc. R. Soc. B Biol. Sci. 270, 265–270. doi: 10.1098/rspb.2002.2238
Leborgne, R., Cantarella, T., and Pasquet, A. (1998). Colonial life versus solitary life in Cyrtophora citricola (Araneae, Araneidae). Insectes Soc. 45, 125–134. doi: 10.1007/s000400050074
Markham, A. C., Gesquiere, L. R., Alberts, S. C., and Altmann, J. (2015). Optimal group size in a highly social mammal. Proc. Natl. Acad. Sci. U.S.A. 112, 14882–14887. doi: 10.1073/pnas.1517794112
Méndez, V., Campos, D., and Bartumeus, F. (2014). Stochastic Foundations in Movement Ecology: Anomalous Diffusion, Front Propagation and Random Searches. Heidelberg: Springer-Verlag. Available online at: http://link.springer.com/10.1007/978-3-642-39010-4 (accesesed August 17, 2021).
Mestre, L., and Lubin, Y. (2011). Settling where the food is: prey abundance promotes colony formation and increases group size in a web-building spider. Anim. Behav. 81, 741–748. doi: 10.1016/j.anbehav.2011.01.002
Rayor, L. S., and Uetz, G. W. (1993). Ontogenetic shifts within the selfish herd: predation risk and foraging trade-offs change with age in colonial web-building spiders. Oecologia 95, 1–8. doi: 10.1007/BF00649499
Reeve, H. K., and Shen, S. F. (2006). A missing model in reproductive skew theory: the bordered tug-of-war. Proc. Natl. Acad. Sci. U.S.A. 103, 8430–8434. doi: 10.1073/pnas.0603005103
Reeve, H. K., and Shen, S. F. (2013). Unity and disunity in the search for a unified reproductive skew theory. Anim. Behav. 85, 1137–1144. doi: 10.1016/j.anbehav.2013.04.007
Rudolph, K., Fichtel, C., Schneider, D., Heistermann, M., Koch, F., Daniel, R., et al. (2019). One size fits all? Relationships among group size, health, and ecology indicate a lack of an optimal group size in a wild lemur population. Behav. Ecol. Sociobiol. 73:Art. 132. doi: 10.1007/s00265-019-2746-0
Rypstra, A. L. (1979). Foraging flocks of spiders. Behav. Ecol. Sociobiol. 5, 291–300. doi: 10.1007/BF00293677
Samuelson, L. (2005). Foundations of human sociality: a review essay. J. Econ. Lit. 43, 488–497. doi: 10.1257/0022051054661549
Schneider, J. M., Jörg, R., Lubin, Y., and Henschel, J. R. (2001). Dispersal of Stegodyphus dumicola (Araneae, Eresidae): They do balloon after all! J. Arachnol. 29, 114–116. doi: 10.1636/0161-8202(2001)029[0114:dosdae]2.0.co;2
Schoepf, I., and Schradin, C. (2012). Differences in social behaviour between group-living and solitary African striped mice, Rhabdomys pumilio. Anim. Behav. 84, 1159–1167. doi: 10.1016/j.anbehav.2012.08.019
Shemer, D. (1986). “The climate,” in Atlas of the Negev, eds E. Stern, Y. Gradus, A. Meir, S. Krakover, and H. Tsoar (Be’er Sheva: Ben-Gurion University of the Negev), 59–66.
Stephens, P. A., Sutherland, W. J., and Freckleton, R. P. (1999). What is the Allee effect? Oikos 87, 185–190. doi: 10.2307/3547011
Teggers, E. M., Deegener, F., and Libbrecht, R. (2021). Fecundity determines the outcome of founding queen associations in ants. Sci. Rep. 11:2986. doi: 10.1038/s41598-021-82559-9
Tschinkel, W. R., and Howard, D. F. (1983). Colony founding by pleometrosis in the fire ant, Solenopsis invicta. Behav. Ecol. Sociobiol. 12, 103–113. doi: 10.1007/BF00343200
Uetz, G. W. (1996). Risk sensitivity and the paradox of colonial web-building in spiders. Am. Zool. 36, 459–470. doi: 10.1093/icb/36.4.459
Uetz, G. W., and Hieber, C. S. (1997). “Colonial web-building spiders: balancing the costs and benefits of group-living,” in The Evolution of Social Behavior in Insects and Arachnids, eds J. C. Choe and B. J. Crespi (Cambridge: Cambridge University Press), 458–475. doi: 10.1017/cbo9780511721953.023
VanderWaal, K. L., Mosser, A., and Packer, C. (2009). Optimal group size, dispersal decisions and postdispersal relationships in female African lions. Anim. Behav. 77, 949–954. doi: 10.1016/j.anbehav.2008.12.028
Ventura, L., Smith, D. R., and Lubin, Y. (2017). Crowding leads to fitness benefits and reduced dispersal in a colonial spider. Behav. Ecol. 28, 1384–1392. doi: 10.1093/beheco/arx106
Yip, E. C., Berner-Aharon, N., Smith, D. R., and Lubin, Y. (2016). Coy males and seductive females in the sexually cannibalistic colonial spider, Cyrtophora citricola. PLoS One 11:e0155433. doi: 10.1371/journal.pone.0155433
Yip, E. C., Levy, T., and Lubin, Y. (2017). Bad neighbors: hunger and dominance drive spacing and position in an orb-weaving spider colony. Behav. Ecol. Sociobiol. 71:128. doi: 10.1007/s00265-017-2357-6
Yip, E. C., and Lubin, Y. (2016). Effects of diet restriction on life history in a sexually cannibalistic spider. Biol. J. Linn. Soc. 118, 410–420. doi: 10.1111/bij.12730
Keywords: Allee effect, colonization, extinction, reproductive skew, social, spider, demographics, population dynamics
Citation: Yip EC, Smith DR and Lubin Y (2021) Long-Term Colony Dynamics and Fitness in a Colonial Tent-Web Spider Cyrtophora citricola. Front. Ecol. Evol. 9:725647. doi: 10.3389/fevo.2021.725647
Received: 15 June 2021; Accepted: 09 August 2021;
Published: 31 August 2021.
Edited by:
Miriam H. Richards, Brock University, CanadaReviewed by:
Matjaz Kuntner, National Institute of Biology (NIB), SloveniaHema Somanathan, Indian Institute of Science Education and Research, India
Ingi Agnarsson, The University of Vermont, United States
Copyright © 2021 Yip, Smith and Lubin. This is an open-access article distributed under the terms of the Creative Commons Attribution License (CC BY). The use, distribution or reproduction in other forums is permitted, provided the original author(s) and the copyright owner(s) are credited and that the original publication in this journal is cited, in accordance with accepted academic practice. No use, distribution or reproduction is permitted which does not comply with these terms.
*Correspondence: Eric C. Yip, ecy7@cornell.edu