- 1Department of Biology, College of Staten Island, Staten Island, NY, United States
- 2Graduate Program in Biology, Graduate Center, City University of New York, New York, NY, United States
- 3Instituto de Ciencias Marinas y Pesquerías, Universidad Veracruzana, Veracruz, Mexico
- 4Department of Biological Science, California State University Fullerton, Fullerton, CA, United States
Elegant terns Thalasseus elegans breed in a very limited area of the northern Gulf of California and the Pacific coast of southern California, with up to 95% (mean 78%, 1991–2014, Perez et al., 2020) of the population nesting on Isla Rasa in the northern Gulf of California. On Isla Rasa, the primary nesting colony, elegant terns suffered predation by rodents which raised the possibility of population extinction, with a substantial proportion of the world population nesting on this single island. Because of this threat, rodents were successfully removed from Isla Rasa in 1995. The removal of rodents from Isla Rasa led to a near immediate increase in the population of elegant terns. That increase was associated with a changing pattern in dispersal by the terns, including extraordinary movements to the Gulf of Mexico, the Atlantic coast of the United States north to Massachusetts, and, remarkably, to western Europe. A few elegant terns successfully bred at these European localities during 2009 to the present. In this paper we use this exceptional example of long-distance dispersal to illustrate how rapid population growth during ∼ 1995 to present can lead to successful colonization of remote sites through repeated instances of vagrancy. We tested four Hypotheses that together support the idea that the growing population of elegant terns has produced increasing numbers of young, and these young have spread, through the mechanism of vagrancy, to the Pacific Northwest, the east coast of the United States, and western Europe. Our Hypotheses are: (1) The nesting population of elegant terns within their core nesting range has increased since removal of rodents from Isla Rasa; (2) Occurrence of vagrant elegant terns in the Pacific Northwest is driven by population growth within the core breeding range. (3) Occurrence of vagrant elegant terns at the east coast of the United States is driven by population growth within the core breeding range. (4) Occurrence and colonization of western Europe by elegant terns is driven by nesting population size within the core breeding range. Corollaries of these Hypotheses are, (i) that there is a time lag in occurrence of vagrants at each of these areas, based on increasing distance from the core breeding range and (ii) the number of vagrants in any given year is also related to sea surface temperature (SST), as expressed by Oceanic Niño Index, a proxy for food resource levels. Generally we found strong statistical support for each of these Hypotheses; an exception was for the occurrence of elegant terns in the Pacific Northwest, which initially occurred following El Niño events (low food supply) and profound breeding failure, but later corresponding to cold water years with high breeding success. We use elegant terns, exceptional for the highly restricted breeding range and sustained population growth over 25 years, to illustrate how growing populations may colonize very distant habitats through repeated instances of vagrancy.
Introduction
Populations of birds, especially but not exclusively migratory ones, are individually variable in their choice of migratory orientation, despite having a mean orientation (Gwinner and Wiltschko, 1978; Phillips, 2000), and distances dispersed by birds typically follow a leptokurtic probability distribution, so that a small but substantial fraction travel much farther than the mean distance dispersed (Moore and Dolbeer, 1989; van den Bosch et al., 1992; Veit and Lewis, 1996; Veit, 1997; Lewis et al., 2016). These characteristics are generally true of movement by birds, regardless of whether the movement consists of “migration,” “dispersal,” or vagrancy or some combination of these. Therefore, what is commonly referred to as “vagrancy” (e.g., Howell et al., 2014) may not require the positing of any additional causal factor beyond broad variability in inherent migratory orientation, combined with a strongly leptokurtic distribution of dispersal distances (Phillips, 2000).
Vagrant birds have previously been thought to be navigationally defective or blown by storms [summary in Newton (2008)], and therefore “biologically unimportant” because they are thought to have been “lost” from the population, despite Grinnell’s (1922) assertion that “[vagrancy] is the regular thing, to be expected. There is nothing “accidental” about [vagrancy]; the process is part of the ordinary evolutionary program.” We support Grinnell’s assertion. Using the very detailed data from the world population of elegant terns, we show that vagrancy, in fact, can result in colonization of extremely distant locations, thousands of km outside of the “normal” range. Demonstrating the process of colonization by vagrancy is extremely difficult to do, even with the advent and improvement of satellite tracking technology, due to enormous samples required. We are able to show this process of colonization by elegant terns because of the rather conspicuous nature of elegant terns themselves, and the enormous interest among both amateur and professional ornithologists that continually document changes in abundance and distribution throughout the world. Thus, studies of colonization by birds are likely to illuminate a similar process for other, less easily studied, organisms. Vagrancy occurs mainly, but not exclusively, during the time before an organism first breeds (Baker, 1978; Ronce, 2007). For passerines studied by Veit (1997, 2000) and Zawadzki et al. (2019) this amounts to the first year or two of life. But seabirds often do not breed until they are much older (up to ∼ 10 years in albatrosses, for example), leaving open the possibility for dispersal over a longer time, perhaps 3 years for elegant terns (Burness et al., 2020).
The concept of what does and what does not constitute “vagrancy” suffers from multiple definitions as well as a scarcity of quantitative analysis. To alleviate confusion, we use the following terminology: “Dispersal” we define as outward movement from a point source. As such, dispersal does not imply subsequent breeding, as it does in population genetics. Vagrancy is the movement by individuals substantially outside the boundaries of current breeding, wintering or migratory ranges of populations. This movement often but not always occurs during regular migration seasons, and can be either directional or seemingly random (Phillips, 2000). It is a continuing process, as these boundaries of course change as the population grows. We do not see compelling evidence that individual vagrants are acting differently from non-vagrants; rather, vagrants form the outermost fringe of a population of individuals dispersing in a way consistent with a correlated random walk (van den Bosch et al., 1992; DeSante and George, 1994; Bartoń et al., 2009). However, since several genes in a variety of organisms have been linked to dispersal behavior (Toonen and Pawlik, 2001; Matthysen, 2012), it is quite possible that these exists a yet undiscovered genetic component to vagrant movements in birds. We have no data to test that idea. Irruptions are instances of pronounced vagrancy occurring episodically. Migration is annually repeated dispersal, usually including spring movement toward a breeding location and fall movement toward a wintering location. Dispersal can be divided into three stages: “departure,” “vagrant,” and “settling” (Ronce, 2007). The movement itself is important and of interest regardless of whether each individual act of movement results in colonization of new geographical territory. Dispersal, migration and vagrancy may be part of a larger, nuanced, highly variable process which describes how birds and other organisms move about the landscape (e.g., Clobert et al., 2012). What we show using the example of elegant terns is that long distance dispersal sometimes does result in colonization of very distant locations, and this lends support for a hypothesis that vagrancy can be selected for and is not an aberration attributable to navigationally incompetent birds (DeSante, 1983; Howell et al., 2014). Further, one can avoid the unhelpful argument about how far an individual need move to be considered a vagrant (as opposed to a migrant) by considering that all individuals within the “vagrant” stage of dispersal, as described above, are vagrants.
Vagrancy may be a positive response; if environmental conditions are very good (high resource abundance), lots of young are produced and these young then disperse, especially if those young have acquired substantial energy reserves (“strong” individuals; Barbraud et al., 2003; Clobert et al., 2009; Kisdi et al., 2012). On the other hand, vagrancy could also be a negative response, an act of desperation to escape deteriorating conditions, though this seems to occur less often (“weak” individuals; Kisdi et al., 2012). Irruptions of boreal finches or raptors, for example, may represent a combined response to high food abundance followed by scarcity as the large number of young produced then consume the resources and need to disperse in search of more food (Newton, 2008). These ideas are similar to those for “nomadic” organisms (Teitelbaum and Mueller, 2019), although far more species of birds, including supposedly non-migratory ones, regularly generate long-distance vagrants than would be considered “nomadic” (Zawadzki et al., 2019). Seabird populations clearly suffer negative impacts of El Niño Southern Oscillation (ENSO) episodes (Schreiber, 2002), in combination with reduced food supply due to local overfishing and sometimes shift breeding locations in response (Velarde et al., 2015).
It is not possible to distinguish between “migrant” and “vagrant” individuals because vagrants are just the extremes of dispersal (DeSante and George, 1994) and we feel there is no meaningful difference between individual birds that are “migrating,” “dispersing” or “acting as a vagrant.” Instead, the process we are studying is one that results in birds spreading outward from a source using a variety of different directional orientations. Those with the most consistent orientations are often referred to as “migrating,” while all individuals are “dispersing.” The ones that travel farthest are vagrants. This pattern is supported by numerous graphics and quantifications of distances dispersed (e.g., Nathan et al., 2012).
Elegant terns, Thalasseus elegans, provide an especially data-rich example of how the process of vagrancy or long-distance dispersal can lead to colonization, despite the relative low likelihood that any one individual vagrant accomplishes such colonization. Seabirds differ from other birds in their requirement for predator-free islands on which to breed; clearly, this has implications for the probability of successful colonization following longer distance dispersal, and the choices made during a process of intelligent dispersal (Bartoń et al., 2009), or exploratory behavior (Baker, 1978; Liebl and Martin, 2012; Santoro et al., 2013; Fayet et al., 2016).
The core breeding population of elegant terns resides on islands in the northern Gulf of California and on the Pacific Coast of California between San Diego and Los Angeles (Velarde et al., 2015; Burness et al., 2020). Their nesting population size and distribution has shifted considerably since their description during the 1850s, due to the combined effects of introduced rodents, harvesting of eggs for food, and fluctuations of schooling fish populations that form their principal prey (Velarde et al., 2015). Currently, a large proportion of the population nests on Isla Rasa in the Midriff Island Region of the Gulf of California, though an increasing number of these birds abandon Isla Rasa and shift to colonies in coastal California, especially when their food supply is lowered during El Niño years, in combination with overfishing of their prey base (Velarde et al., 2015). Despite numerous El Niño episodes over the past 70 years, the elegant tern population continues to increase (Figure 1), mainly as a consequence of the removal of black rats (Rattus rattus) and house mice (Mus musculus) from Isla Rasa in 1995 (Velarde et al., 2015).
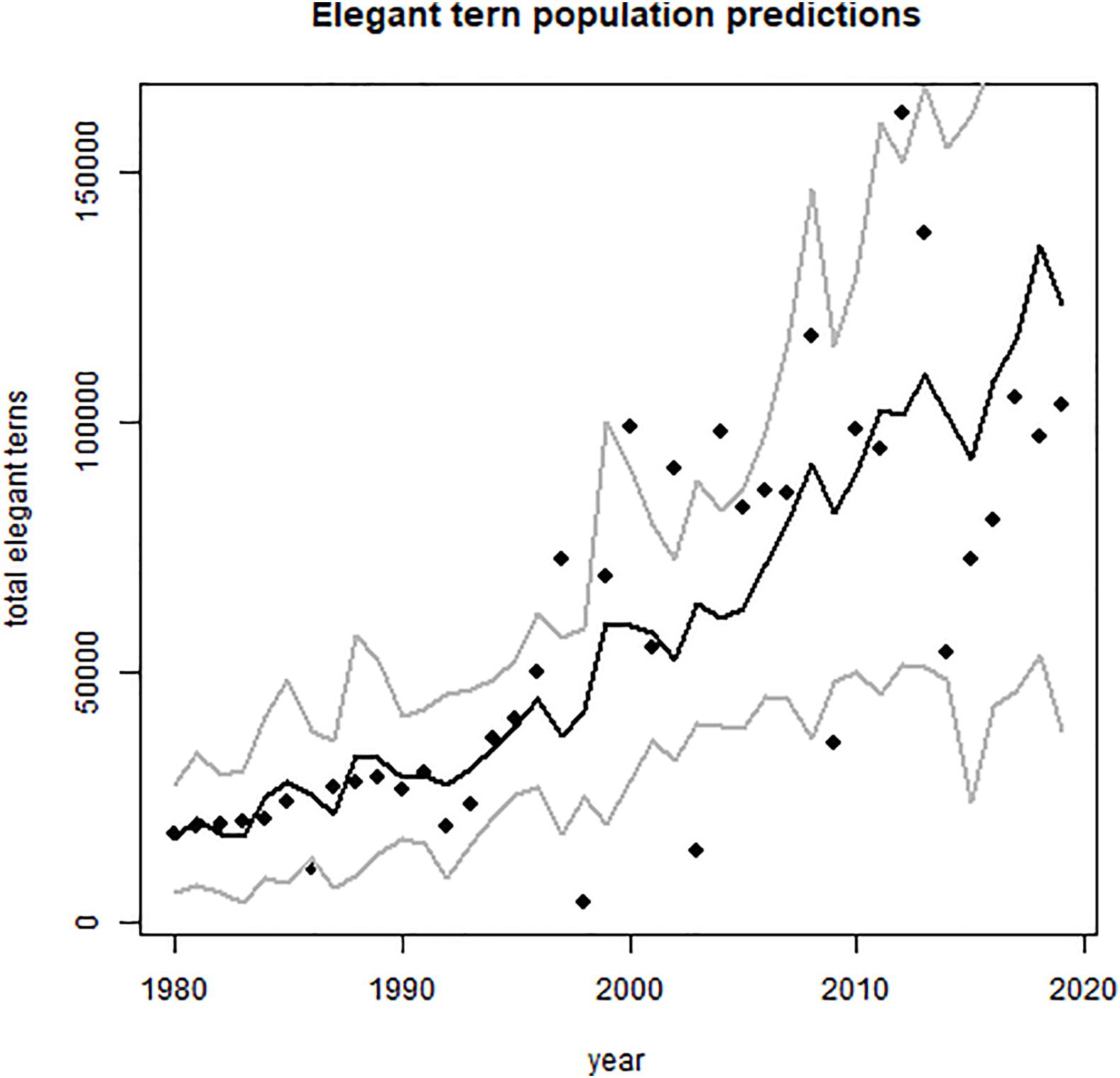
Figure 1. Growth of core area population of elegant terns, summed over Isla Rasa plus Southern California colonies 1980–2019 [1980 to 2014 taken from Velarde et al. (2015), 2015 to 2019 from M. Horn and Velarde (unpubl.)]. Statistical support in Table 2. Predicted values and confidence limits shown.
Thus there is an interplay between two factors – removal of rats on Isla Rasa and fluctuations in food availability caused by El Niño and fisheries - that influence population growth by elegant terns. The primary theme of this paper is that long-term population growth 1995–2018 has driven increased vagrancy through production of increased numbers of young that are then available to disperse. At the same time, there are shorter-term fluctuations in food availability that also influence the number of young produced, and, indirectly, the amount of population growth in that year. Because of this interplay, we include oceanic fluctuations (“Oceanic Niño index,” ONI) within our models.
Following the breeding season, some elegant terns travel northward to forage in the California Current Ecosystem, north to British Columbia. Elegant terns winter along the coasts of Central and South America from Nayarit, Mexico to southern Chile, with most between Ecuador and northern Chile (Howell and Webb, 1995; Burness et al., 2020). This basic pattern has become obscured over recent years, in that maximum numbers of birds moving north do not necessarily occur during El Niño years, and indeed some of the largest numbers have appeared during productive breeding seasons during La Niña rather than El Niño conditions (Velarde et al., 2015).
Very long-distance dispersals of elegant terns have been documented since the late 1960s. Vagrant elegant terns were first detected at Johnston Atoll in the central Pacific Ocean in 1969 (Amerson and Shelton, 1976) and in France in 1974 (Stoddart and Batty, 2019). Remarkably, given the ∼9000 km distance separating the Gulf of California from western Europe, elegant terns have repeatedly prospected Sandwich Tern Thalasseus sandvicensis colonies in France and Spain beginning in the 1980s, and have since successfully nested (Dies et al., 2019; Stoddart and Batty, 2019). Most instances of vagrancy are not followed by breeding, and indeed this is one of the primary reasons why the “biological importance” of vagrancy has been dismissed in the past (Davis and Watson, 2018).
Our basic thesis is that during years when large numbers of young are produced, those young spread outward over a broad range of distances as predicted by models using a correlated random walk, in which the degree of correlation among successive steps correlates positively with distance between patches of suitable habitat (Bartoń et al., 2009). Particularly, in these analyses we posit: (1) The nesting population of elegant terns within their core nesting range has increased since removal of rodents from Isla Rasa; (2) Occurrence of vagrant elegant terns in the Pacific Northwest is driven by population growth within the core breeding range. (3) Occurrence of vagrant elegant terns at the east coast of the United States is driven by population growth within the core breeding range. (4) Occurrence and colonization of western Europe by elegant terns is driven by population size within the core breeding range. Corollaries of these Hypotheses are (i) that there is a time lag in occurrence of vagrants at each of these areas, based on increasing distance from the core breeding range and (ii) the number of vagrants in any given year is also related to sea surface temperature (SST), as expressed by Oceanic Niño Index, a proxy for food resource levels.
Materials and Methods
Data
We have detailed, annual data on total number of nesting pairs for the entire population of elegant terns. We interpret significant population growth over time as evidence of reproductive success. Since we know where all nesting colonies of elegant terns are located, bias of this interpretation due to immigration and emigration is minimal, compared to that for other species. We used data on numbers of nesting pairs of elegant terns from Velarde et al. (2015), and M.H. Horn (unpubl. data). Hereafter, we designate as the “core area” the world breeding population (except for the new, small colonies in western Europe, see below) of elegant terns, which are on Isla Rasa in the northern Gulf of California (up to 95% of pairs), plus the rest on three colonies in the Southern California, United States area.
We gathered data on occurrence of vagrants in Washington and Oregon, United States and on the Gulf of Mexico and Atlantic coasts of the United States from regional reports the journal North American Birds https://www.aba.org/north-american-birds/and eBird1. Elegant terns, like other terns, form large roosts, often near mouths of rivers and estuaries where schooling small pelagic fish are abundant. These roosts occur at predictable locations, such as the mouths of the Chetco and Rogue River mouths in Oregon, and the Columbia River mouth at the border between Oregon and Washington. These areas are censused by birders on at least a weekly basis throughout the year, and maximum counts are consistently tallied in the publications Oregon Birds, Newsletter of the Washington Ornithological Society and North American Birds (Table 1). These data provide a reliable and consistent estimate of the total number of elegant terns appearing in the Pacific Northwest each year.
We also gathered data on occurrence of vagrants and breeding by Elegant Terns in Europe from Dies et al. (2019) and Stoddart and Batty (2019). There has been quite a bit of scrutiny of recent appearances of individual birds in Europe, which fit the elegant tern phenotype. Multi-locus barcoding on three elegant terns in France and Spain (Dufour et al., 2017) ruled out the possibility that these 3 terns are hybrids (of Sandwich and Elegant Tern); they are pure elegant terns. The Irish Rare Birds Committee has accepted 5 occurrences of elegant tern in recent years (1982–2005) (Parkin and Knox, 2010) as a Category A species, (Species Recorded in a Natural State since 1 January 1950). More recently, the British Ornithologists’ Union Records Committee (BOURC, 2018) also added elegant tern to the British List as a Category A species.
We characterized the strength of El Niño years based on the Oceanic Niño Index (ONI)2.
Analyses
We used the statistical program R (R Core Team, 2020) for all statistical computations and graphics. Given the non-linear nature of the relationships we sought, we used General Additive Models to model number of vagrants and population size of elegant terns. To test H1, we first modeled the numbers of elegant terns in the core area over time (including terns from both Isla Rasa and Southern California, Velarde et al., 2015), as a function of year and El Niño. We considered the possibility that the time series of elegant tern population sizes is autocorrelated; we conducted a partial autocorrelation analysis to test for this. The partial autocorrelation gave a significant result at lag 1, and insignificant results at higher lags, so we used a General Additive Mixed Model (Zuur et al., 2009; Knape, 2016) to incorporate the autocorrelation structure into the model (command gamm, in R package mgcv, correlation = corAR1, family: negative binomial). We compared these two models using Akaike’s Information Criterion (AIC), and retained the model with the lowest AIC.
Vagrancy
We tested the hypotheses (H2, H3, and H4) that nesting population size in California and Baja California (the core area) significantly predicts the number of vagrants in three different non-core regions: the Pacific Northwest, the east coast of the United States, and Europe. We conducted GAM models using number of vagrants as the response (H2, H3, and H4, respectively, for the Pacific Northwest, United States east coast and Europe, and the smoothed terms of core area population size and El Niño. We further tested for significant autocorrelation in the response (Pacific coast vagrant numbers, East coast vagrant numbers, European vagrant numbers). If we found significant autocorrelation, we additionally calculated GAMMs using smoothed year and El Niño terms as predictors. For each given region (Pacific Northwest, East Coast United States, Europe) we used AIC to choose the best model of vagrant numbers.
Results
The core area population of elegant terns (sum of Isla Rasa and coast of California birds) increased, somewhat intermittently, from 1980 to the present (Figure 1 and Table 1), with highest numbers of nesting pairs in 2012 and 2013 (Velarde et al., 2015). Fluctuations in Oceanic Niño Index (ONI) are shown in Figure 2, with particularly strong El Niño and La Niña years marked.
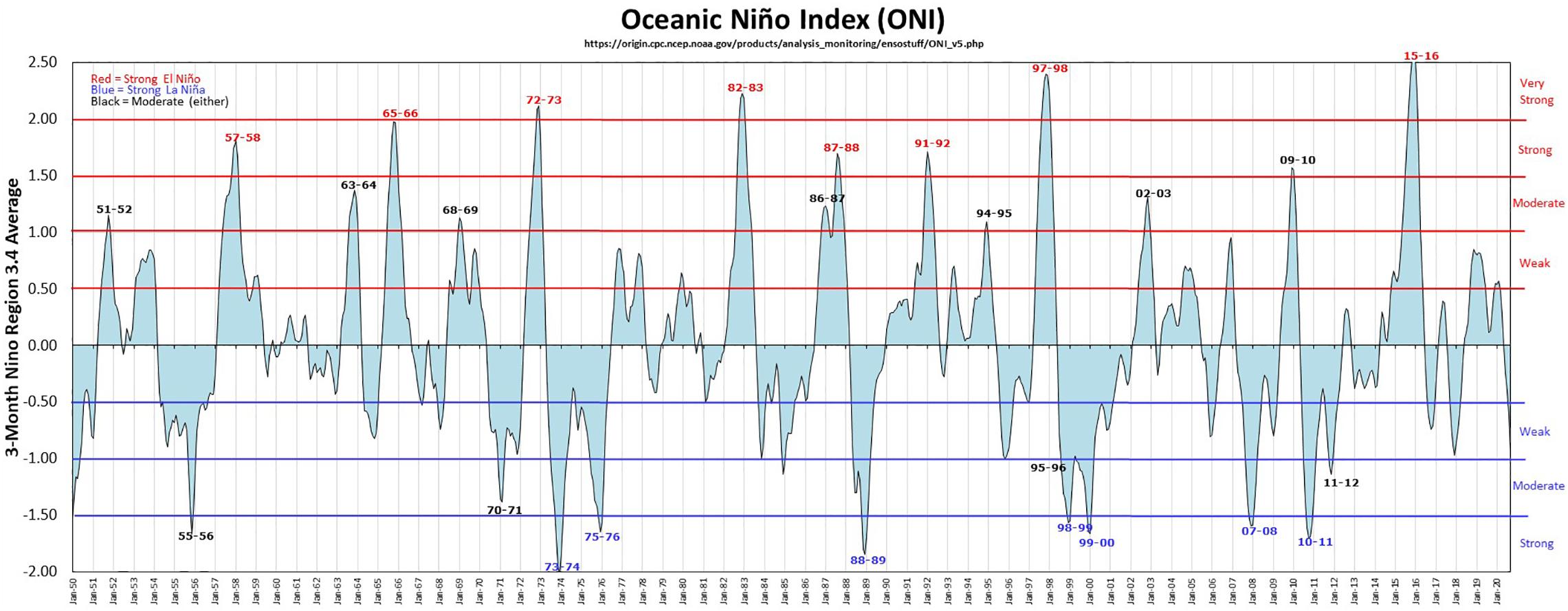
Figure 2. Oceanic Niño Index (ONI) for the North Pacific Ocean 1950—2020. The 2009–2010 El Nino was stronger in the Gulf of California than in the California Current and reduced food availability around Isla Rasa. From https://ggweather.com/enso/oni.htm. Accessed 17 November 2020; used with permission.
Models of the California and Baja California Core Population Size (H1)
We first did a partial autocorrelation test of the core area population size (R command pacf). The correlation coefficient (0.50) was significant at lag 1, but not significant thereafter, indicating that population size in the core area has an autocorrelated structure at lag 1. With this information, we formed a GAMM incorporating an autocorrelation structure (Table 2).
The best model had significant Year and El Niño effects, and incorporated an autoregressive (lag 1) structure [AR(1)]. This model explains 61% of the variability in core area population size.
Vagrants to the Pacific Northwest (H2)
Year and core area population are strongly correlated (r = 0.78, p = 3.9 × 10–9); thus, we only included core area population size (and not year) in our models of vagrant numbers. As well, a partial autocorrelation function for vagrant numbers in this region was significant at lag 1, but not significant at higher lags. Thus, we calculated a GAMM for this region, incorporating an autoregressive (1) structure. Model results for the following analyses are listed in Table 3.
The increase in the core area nesting population corresponded to an increase in abundance during late summer and fall in Oregon and Washington (Figure 3). This northward movement into the Pacific Northwest, increased during the early 1980s (Figure 3), especially after the 1982–1983, 1991–1992, and 1997–1998 El Niño episodes (Figures 2, 3). However, the largest numbers of elegant terns in the Pacific Northwest appeared during the years 2012–2014, which were cold water years (Figure 3).
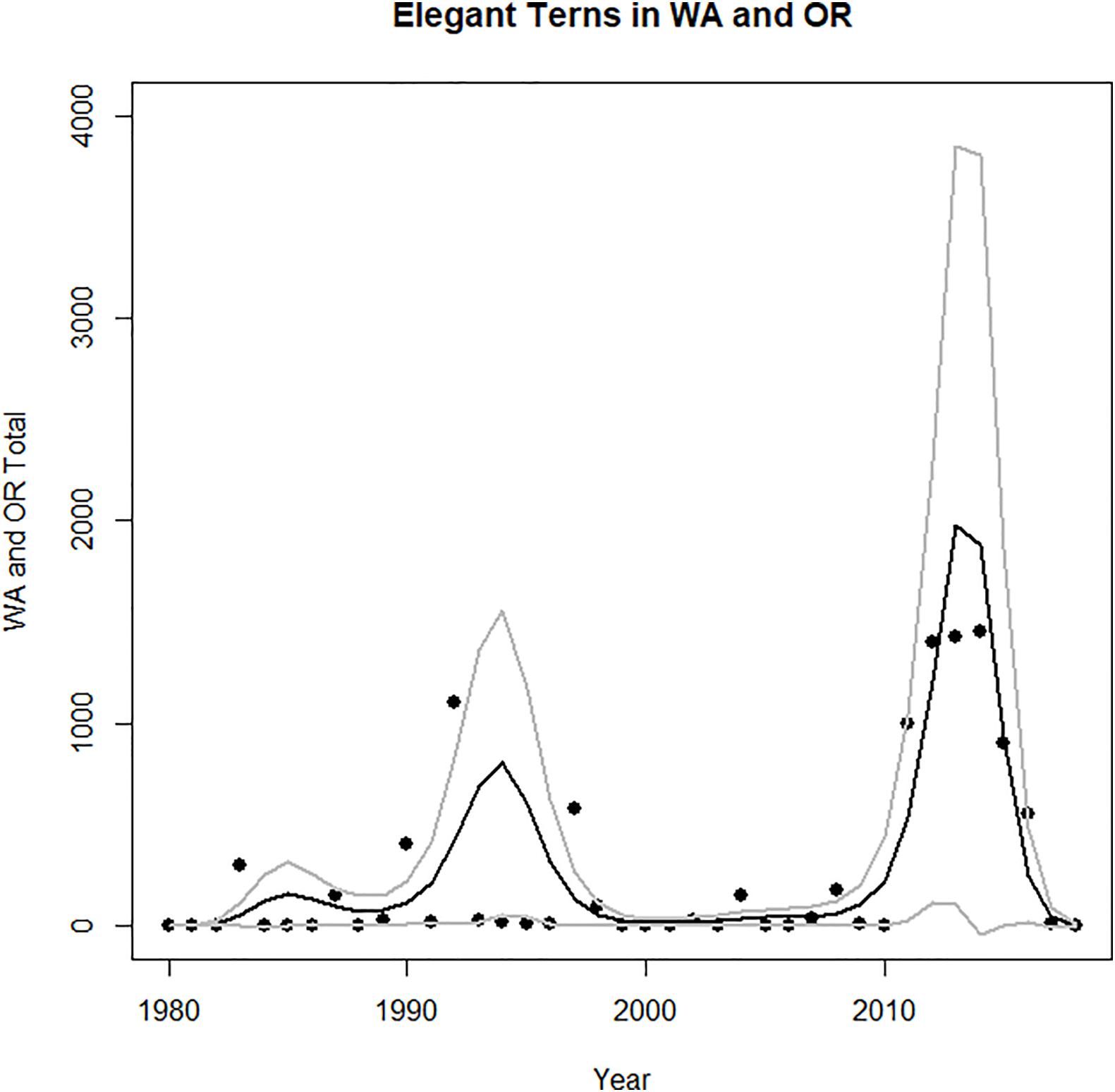
Figure 3. Time series of abundance of vagrant elegant terns in Washington and Oregon during July–October. Fit of model improved with addition of data on Oceanic Niño Index (Table 1). Predicted values and confidence limits shown.
Given these observations, it is not surprising that the best model for vagrant numbers to the Pacific Northwest is that including both core area population size and El Niño, and incorporating an autoregressive structure (with an AIC of 169, Table 3). In this model, both core area population size and El Niño are significant predictors of Pacific Northwest vagrants. This model has a deviance explained value of more than 63.4%, following the logic in Gilman et al. (2012). Although there is not an agreed method for calculating deviance explained for a GAMM (Gilman et al., 2012), since the AIC value for that model is much below the equivalent GAM, the deviance explained for the GAMM must be larger than that for the GAM (Gilman et al., 2012).
Vagrants to the United States East Coast (H3)
This region also showed significant autocorrelated numbers of vagrants, with an autocorrelation of almost 0.60 at a lag of 1 year, and no significant autocorrelation for lags greater than 1 year. The increase in the observations of vagrant elegant terns beyond the Pacific Ocean, to both eastern North America (Figure 4) and Europe (Figure 5) began with individual birds in 1985–86 (perhaps a consequence of strong 1982–1983 El Niño), and then increasing numbers of individuals in about 1999, and continues to the present (Figure 4). A decline of records on the United States East Coast during 2004–2010 may reflect the weak El Niño of 2002–2003 and consequent lowered reproduction.
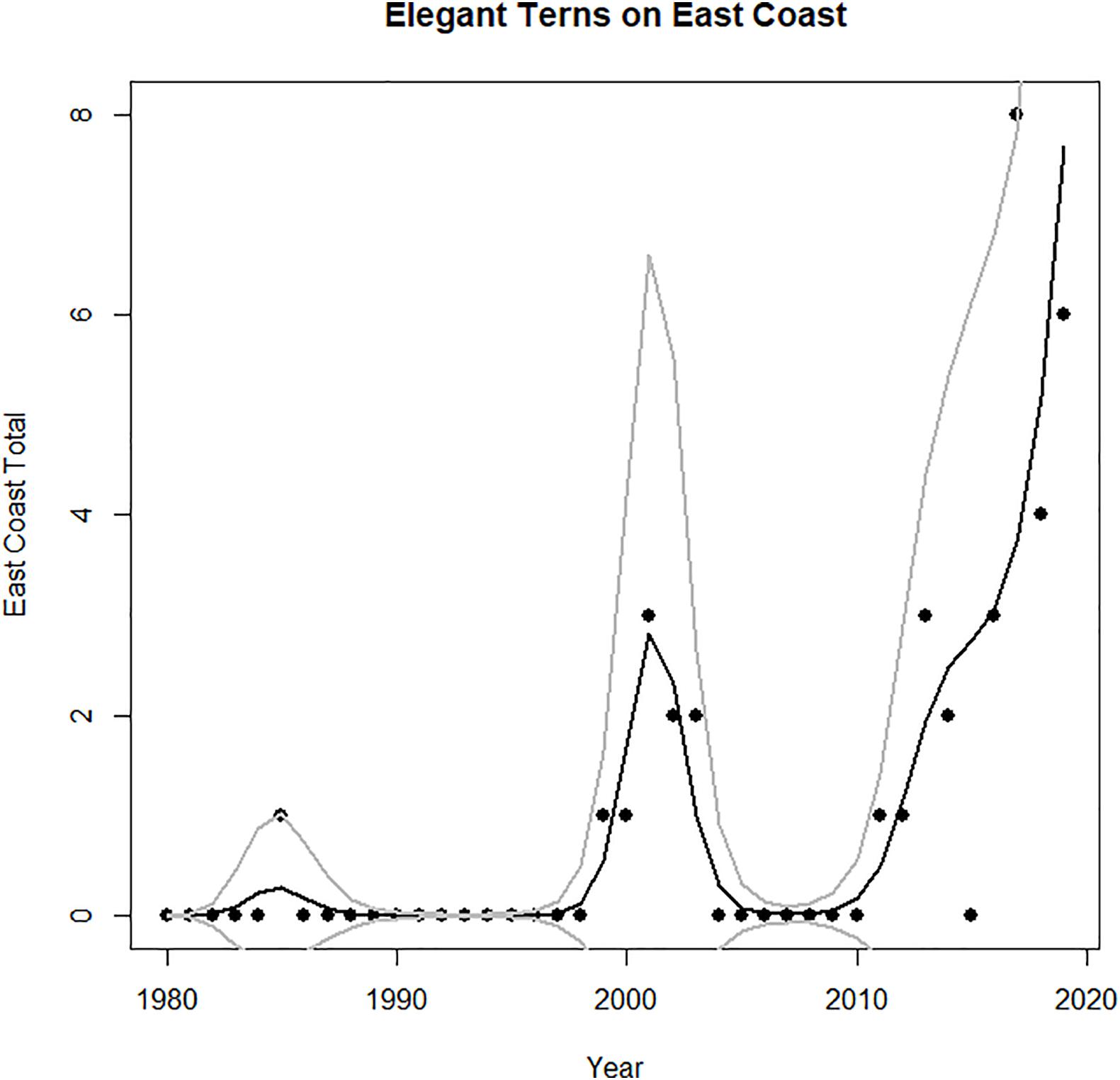
Figure 4. Time series of abundance of vagrant elegant terns to east coast of North America (Massachusetts to Florida). Predicted values and confidence intervals shown.
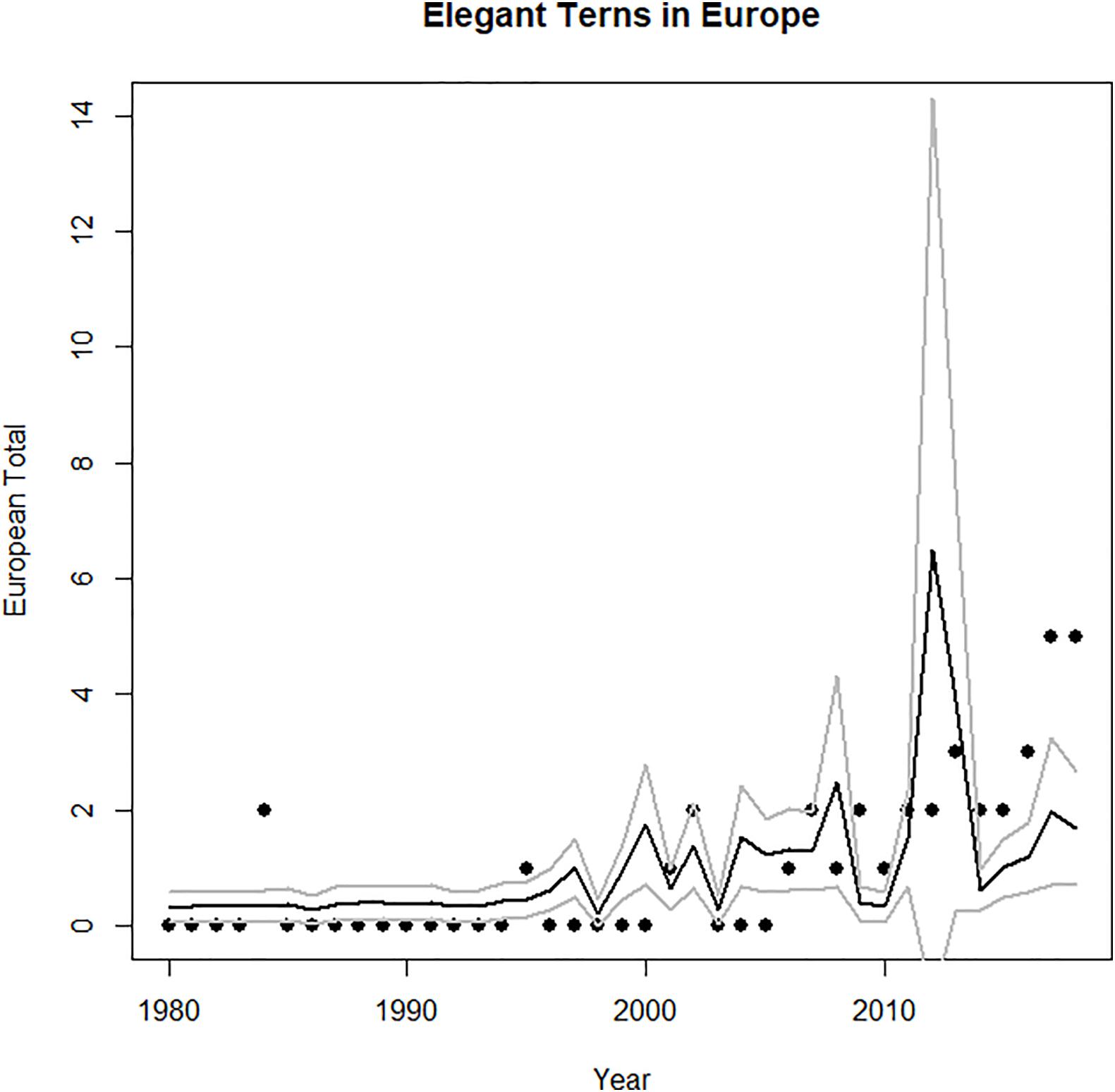
Figure 5. Time series of abundance of vagrant elegant terns in Europe. Predicted values and confidence intervals shown for GAM (Table 1) on each graph.
Models of East Coast vagrants show that the GAM (no autoregressive structure) is the better model (AIC value of 99 compared to AIC of 167 for the GAMM, Table 3). In both cases the core area population term is a significant predictor (but El Niño is not). The GAM then would have a higher deviance explained (33.3%) compared to that of the GAMM (Gilman et al., 2012; Table 3).
Vagrants to Europe (H4)
The European vagrants were significantly autocorrelated at a lag of 1 year (an autocorrelation coefficient of more than 0.60), and not significantly autocorrelated at higher lags. However, the best model for vagrants to Europe (Figure 5) is the GAM (not the GAMM, which incorporates the autocorrelation structure). Both the GAM and the GAMM have a significant and positive core area population size term. The GAM has a deviance explained of 40%. In both cases, the core area population size is a significant predictor (but El Niño is not).
Taken together, these results show us that the total core area population predicts the spread of birds northward to the Pacific Northwest (Figure 6 and Table 3), the spread to the United States East Coast (Figure 7 and Table 3) and the spread to Europe (Figure 8 and Table 3). The El Niño data does help to increase predictive capacity of the models, but in itself was a significant predictor only for vagrants to the Pacific Northwest.
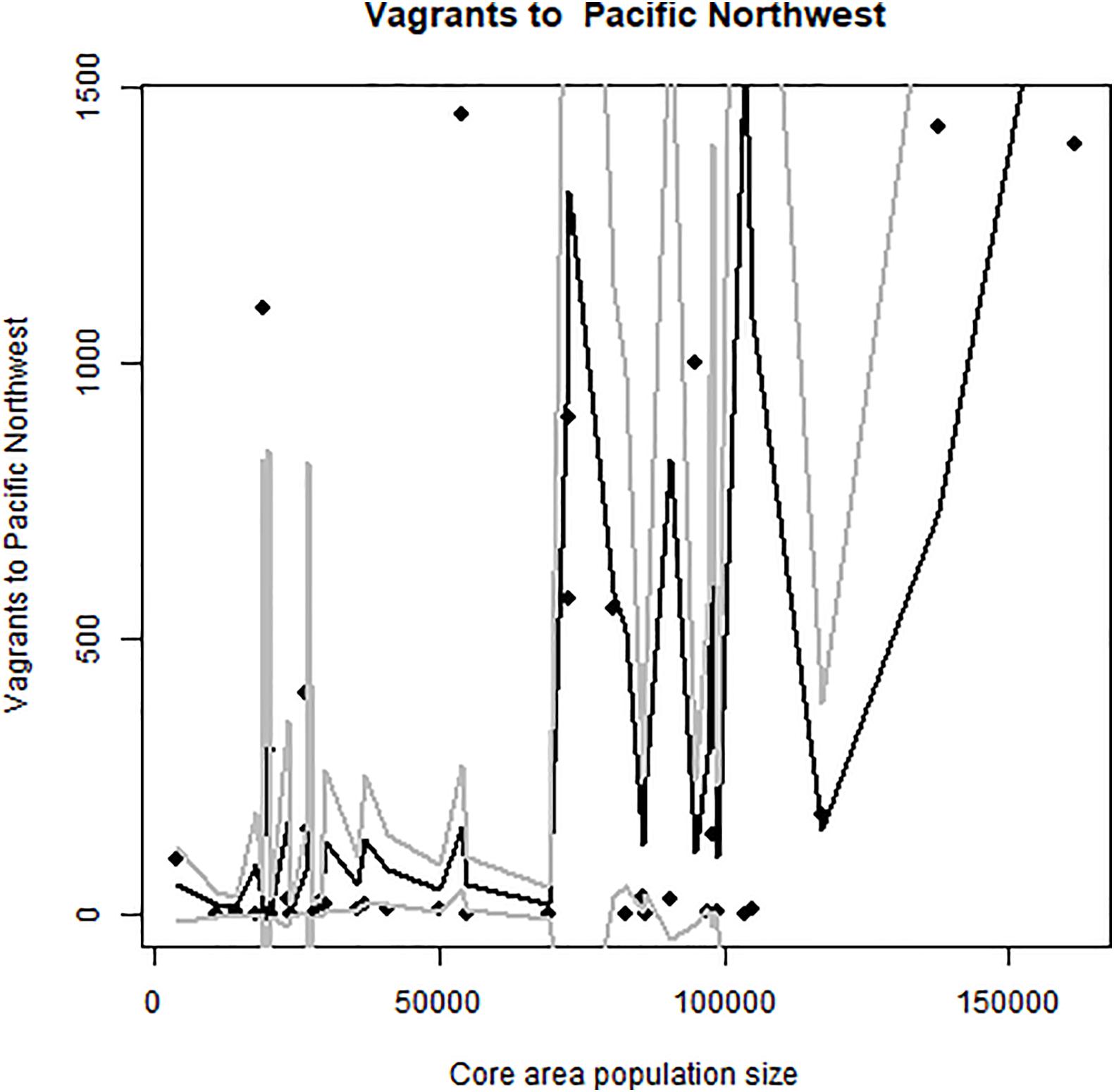
Figure 6. Occurrence of vagrant elegant terns in Washington and Oregon, relative to abundance at Pacific breeding colonies. Predicted values and confidence intervals shown.
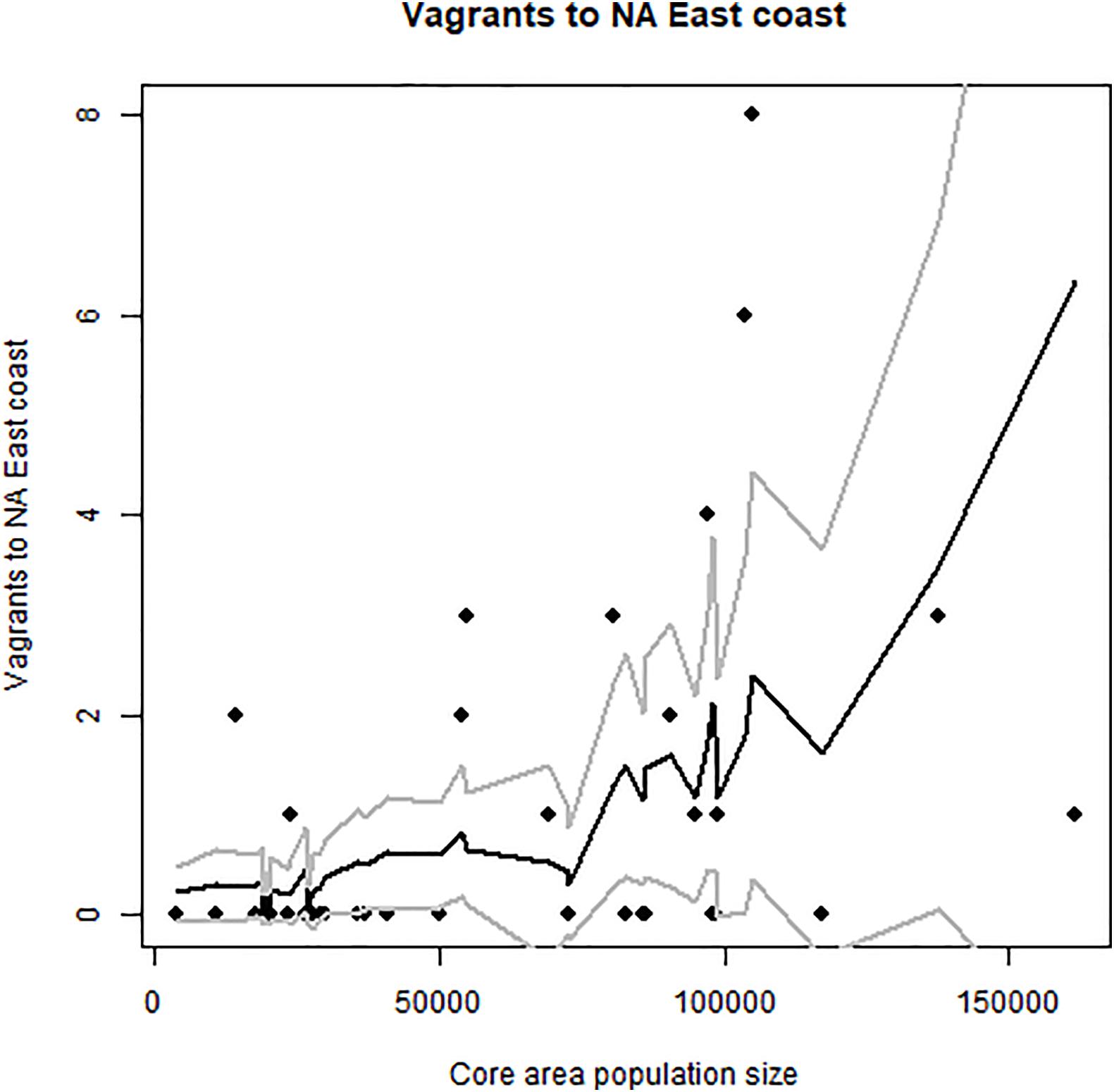
Figure 7. Occurrence of vagrant elegant terns on United States east coast vs. Pacific nesting population size (number of pairs) in the same year. Peak numbers on east coast in 2017–2019. There was a strong El Niño in 2015–16 and a weak one in 2019, but 2017 and 2018 were La Niña (cold water) years. Predicted values and confidence intervals shown.
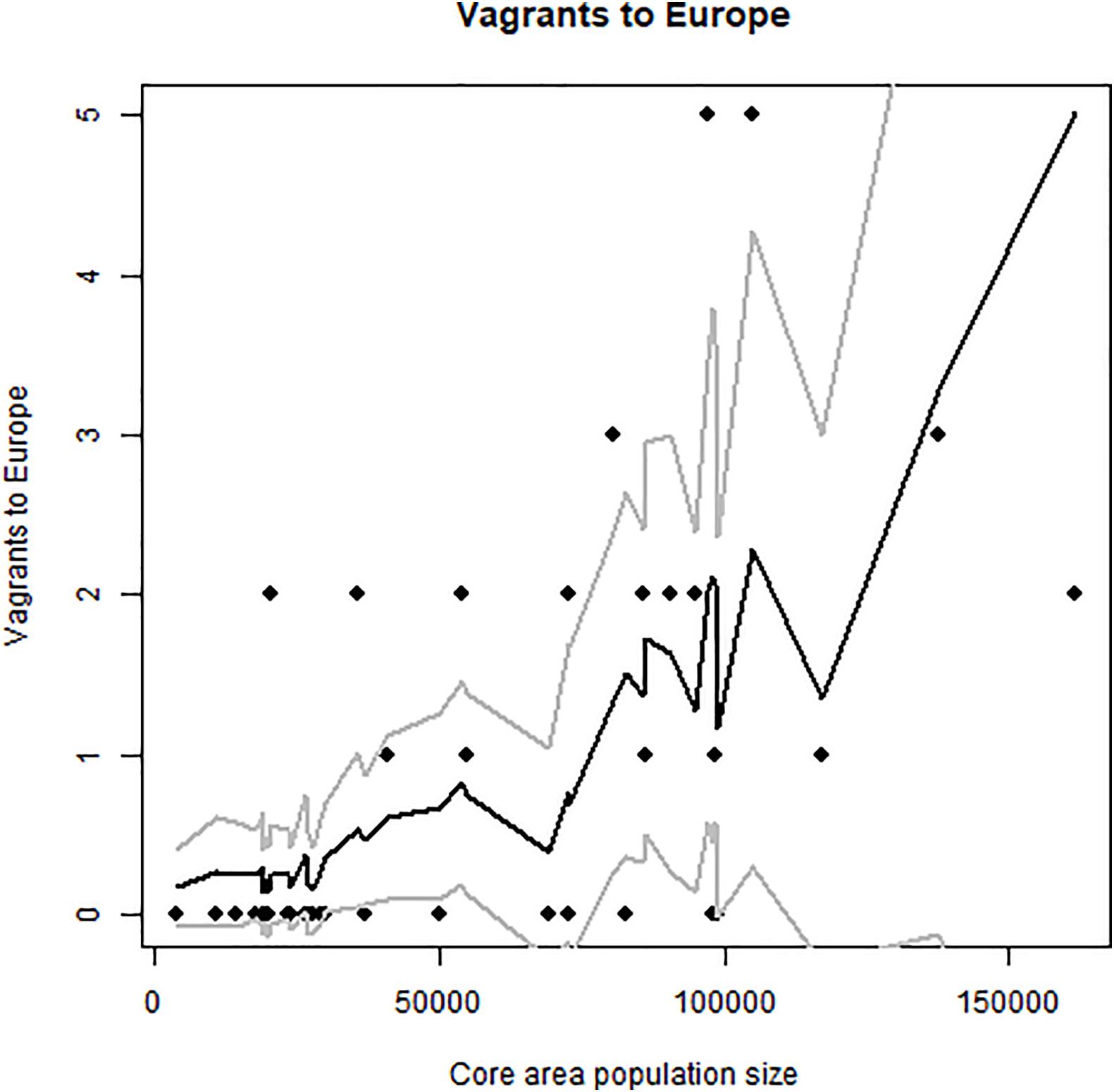
Figure 8. Occurrence of vagrant elegant terns in Europe (Spain and France; number of individual vagrants). Note increase beginning in about 2000. Note the coincidence of the increase in vagrancy to both Europe and Eastern North America in about the year 2000, following major growth of Pacific colonies after 1995. Predicted values and confidence intervals shown.
Discussion
Colonization of new habitats, as well as new geographical locations, is of increasing importance as changes in the earth’s climate alters existing habitats (DeSante and George, 1994; Johnson, 1994; Zawadzki et al., 2019). Whether a particular species is able to survive a changing climate must depend, at least in part, on the ability to find and colonize new locations. While this basic process has obviously occurred in the past, as all or most of the faunas of oceanic islands are ultimately the result of colonization (Lovette et al., 1999), we do not know very much about whether birds (or other organisms) can achieve this rate of colonization at the rate at which the earth’s climate is warming. Thus, the data-rich example provided by elegant terns can inform models of possible future colonization, where colonization depends on the coupled processes of population growth (Johnson, 1994; Veit, 2000; Veit et al., 2016; Zawadzki et al., 2019), exploratory behavior, “intelligent dispersal” (Bartoń et al., 2009) and “informed dispersal” (Reed et al., 1999; Clobert et al., 2009).
It is perhaps puzzling why elegant terns, who so clearly suffer from food shortages during major ENSO events (Velarde et al., 2015, 2019), have nevertheless managed to increase their population since before the major El Niño event of 1982–1983. Part of the explanation is certainly, first, the reduction of human disturbance since Isla Rasa was protected and, later, the removal of rats and mice from Isla Rasa in 1993–1995 (Velarde et al., 2015). Furthermore, the nesting sites in southern California are all located on state or federally protected lands. Another important factor for the continued increase in the species population size may be their ability to shift nesting sites when conditions in one area are adverse. Through genetic analysis and banding information Perez et al. (2020) have shown that individual elegant terns move freely between the Gulf of California and Southern California nesting sites, and this may be occurring in response to local conditions of food availability. Our model results (Tables 2, 3) show that population size in the core area was the primary driver of vagrancy to the east coast (H3) and Europe (H4), and El Niño was not influential, whereas both population size and El Niño were drivers for northward dispersal to the Pacific Northwest. Our interpretation is that during El Niño years, disrupted food supplies is enough to trigger relatively short distance vagrancy to the Pacific Northwest, but sustained population growth over 25 years is necessary to drive long -distance vagrancy to the United States east coast and Europe.
Vagrants to the Pacific Northwest
The pattern of occurrence of elegant terns in Oregon and Washington is not what one would expect if these episodes of northward dispersal were driven by breeding failure during ENSO. The first elegant terns recorded in either Oregon or Washington were observed during summer 1983, when 300 birds appeared following the major El Niño event of 1982–1983. Thereafter the association was less clear. The largest numbers occurred during the summers of 2011–2014 preceding the very strong 2015–2016 El Niño, as well as in 1992, itself a strong El Niño year, during summer 1997, which preceded the very strong 1997–1998 El Niño, and 1990, a non -El Niño year (Figure 2). The highest abundances of elegant terns in the Pacific Northwest during in the four summers of 2011–2014, which were La Niña years that followed a strong El Niño in 2009–2010. Thus, our H2 is supported after ∼ 2000, but not before then because elegant terns dispersed northward during and immediately following a crash of the food supply during El Niño events.
East Coast of United States
The impact of population growth in California upon the spread of elegant terns to the East Coast and Europe was more consistent than the impact of population growth on spread to the Pacific Northwest H3 and H4. The major increase in records of vagrants to the United States East Coast and to Europe coincided with the peak of maximum growth within the core area, which took place from about 2000–2013 (Figures 1, 6–8). Maximum numbers on the east coast occurred in 2017–2019, and abundance on the United States east coast was correlated with breeding population size (Figure 7). Virtually all records from the Pacific Northwest are during July–October, and most records on the Gulf and Atlantic Coasts of the United States are during fall and winter.
Vagrants to Europe
The first elegant tern appeared in Europe in 1974, perhaps associated with a very strong El Niño in 1972–73, a second one appeared in 1985, and the main increase began in about 2000, and peaked in 2017–2018 (Figure 5). They first attempted breeding in 2009, and produced an egg that failed to hatch, then began breeding successfully in 2012, and produced 7 “pure” and 2 hybrid (with Sandwich Tern) chicks through 2018 (Dies et al., 2019; Figure 9).
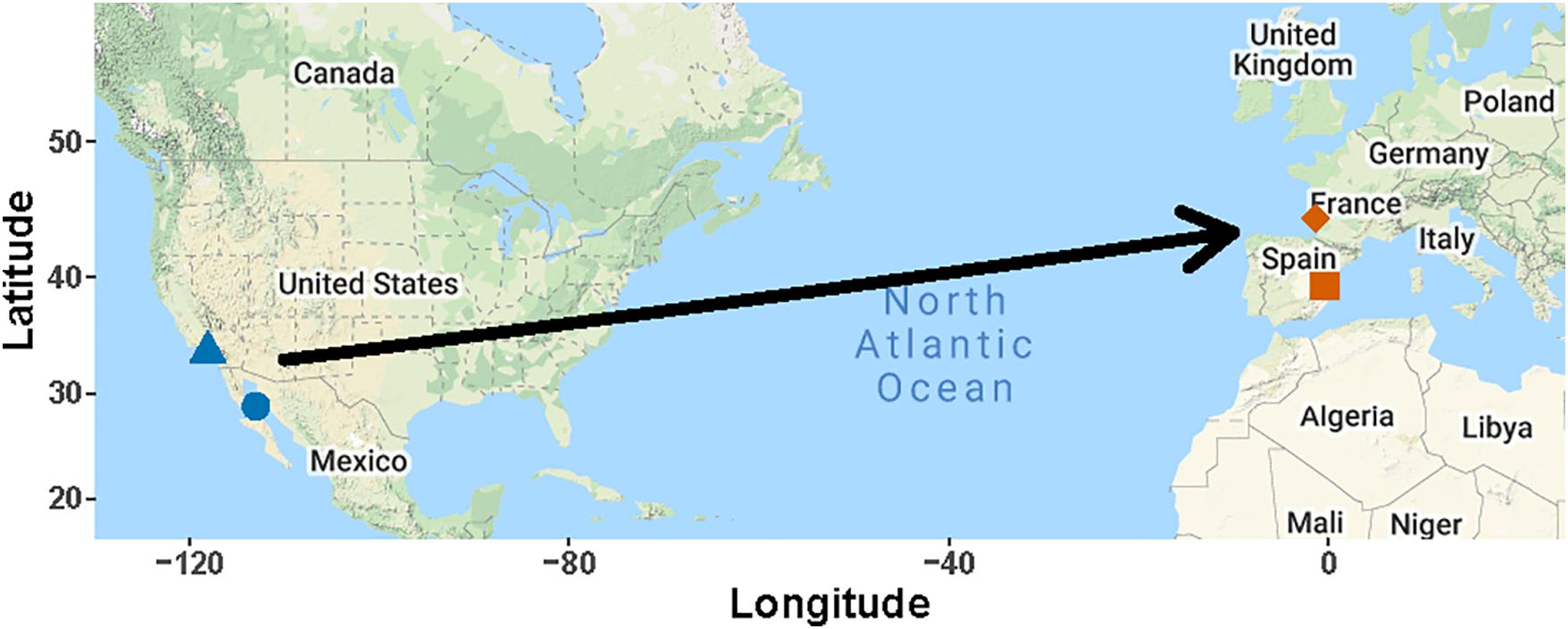
Figure 9. Colonization of Europe by elegant terns. The primary colony, Isla Rasa in the Gulf of California is shown by the blue circle, and the location of three colonies on the Pacific Coast of California is shown by the blue triangle. European breeding has occurred at L’Albufera de Valencia, Spain (red square) and Banc D’Arguin, Gironde, France (red diamond) (Dies et al., 2019; Stoddart and Batty, 2019).
Our contention is that colonization of Europe transpired through repeated vagrancy to Europe by Elegant Terns originating in North America. Alternative theories would include misidentification of Cayenne terns (T. sandwichensis eurygnatha) in Europe as elegant terns, and origin of correctly identified elegant terns in Europe in so far undiscovered colonies in Africa or elsewhere outside of the Western Hemisphere. It is also possible that elegant terns could be breeding elsewhere on the eastern side of the Atlantic, perhaps West Africa, among the very large royal tern colonies there (Cabot and Nisbet, 2013). The identity of elegant terns in Europe has been confirmed on the basis of multilocus barcoding as elegant terns (Dufour et al., 2017). While there is no evidence of breeding by elegant terns in Africa, despite an increasing number of records from South Africa (eBird Basic Dataset, 2013) any breeding far outside their original range in the southern California region supports our main contention in this paper that colonization following long-distance vagrancy is a viable means by which birds may escape climate extinction. Furthermore, if occurrence by elegant terns on the east coast of North America was part of the dispersive process bringing elegant terns to Europe, we would expect a correlation between occurrence in both regions, and this is in fact true (Spearman’s rho = 0.54, p = 0.00035; Figure 10).
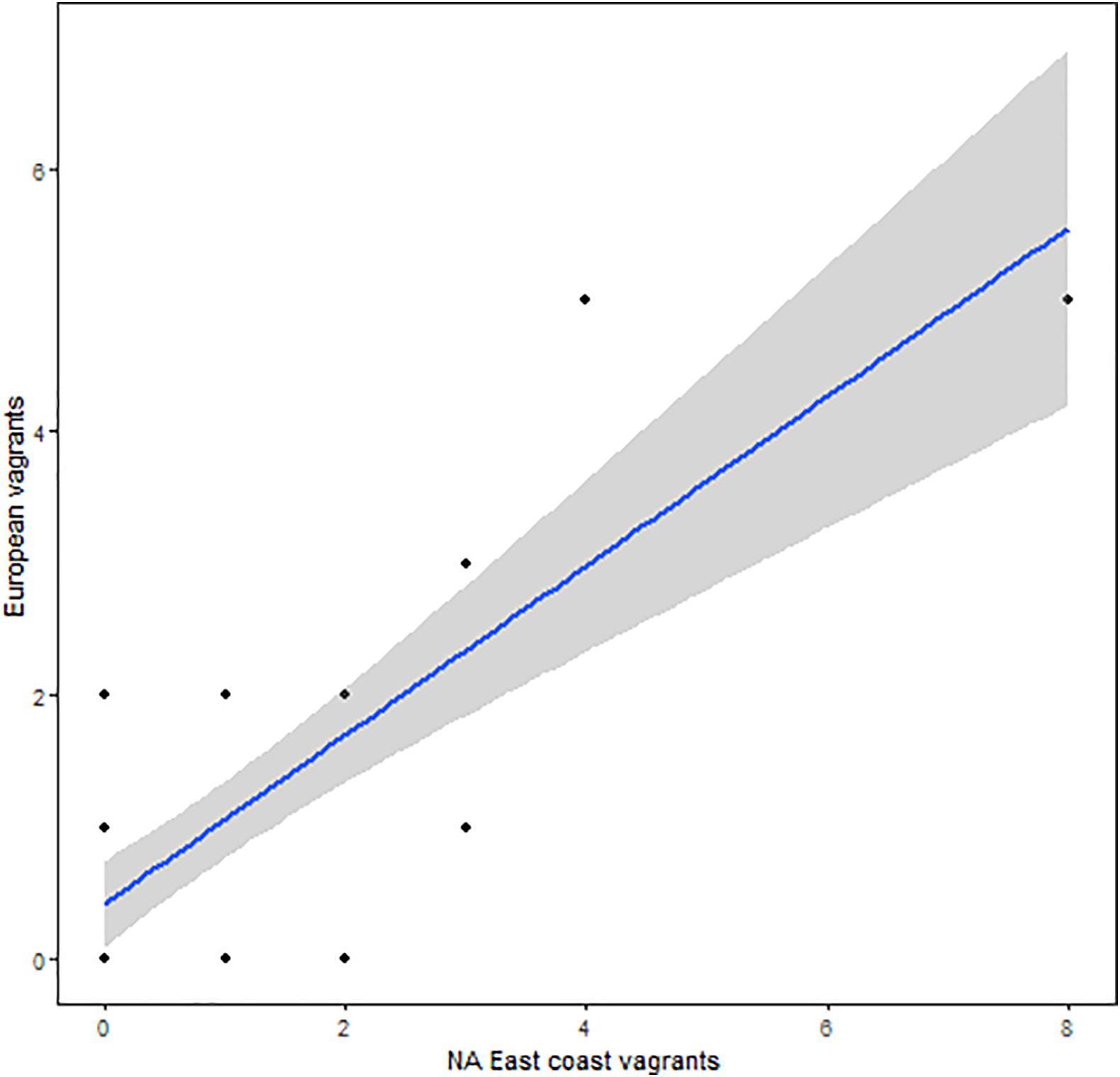
Figure 10. Relation between numbers of vagrant elegant terns on the east coast of North America and vagrants to Europe.
Elegant terns probably do not breed until their third summer (3 years old; Burness et al., 2020), so they could reasonably use their first three years of life to prospect for new colonies using “intelligent dispersal,” which is movement modeled using a correlated random walk (Bach et al., 2007; Bartoń et al., 2009). The data on elegant terns appearing on the Pacific coast during July–November are not segregated by age classes, but at first glance, adult-plumaged birds seem to dominate the age composition. An analysis of occurrences in British Columbia (Toochin and Haviland, 2016) lists 3 hatching year birds out of 30 total records, and our examination of photographs (Birdfellow, 2021; Cornell Laboratory of Ornithology, 20213) show that at least some hatching year birds appear there during July to October. Elegant terns that are one or two years old are very similar to full adults (Pyle, 2008) and may be overlooked as being of subadult or pre-breeding age among the larger numbers of full adults present. Further research should quantify the proportion of pre-breeding age elegant terns that accompany the larger number of adults moving along the North American west coast during fall to determine whether this age ratio varies with El Niño versus La Niña conditions. Such data would resolve the question of whether long distance dispersal or vagrancy is accomplished predominantly by young birds or adults.
We conclude that the driving factor behind increasing vagrancy northward and eastward by elegant terns, and their subsequent colonization of Europe (Figure 9), is the rapid increase of the core population in the Gulf of California and San Diego area. This rapid growth produced large numbers of young birds that subsequently dispersed; a few of the dispersing individuals found suitable habitat in Europe, 9000 km from their natal colony. We suggest the process we describe for elegant terns may be a way that species can persist during rapidly changing climate.
Data Availability Statement
The original contributions presented in the study are included in the article/supplementary material, further inquiries can be directed to the corresponding author/s.
Author Contributions
RV formulated the idea for the study and wrote most of the manuscript and jointly conducted statistical analyses with LM. EV and MH collected all data on nesting Elegant Terns from Isla Rasa, Mexico, and San Diego area, California, United States. LM helped with statistical analysis and writing the parts of the manuscript related to those findings. All authors contributed to the article and approved the submitted version.
Conflict of Interest
The authors declare that the research was conducted in the absence of any commercial or financial relationships that could be construed as a potential conflict of interest.
Publisher’s Note
All claims expressed in this article are solely those of the authors and do not necessarily represent those of their affiliated organizations, or those of the publisher, the editors and the reviewers. Any product that may be evaluated in this article, or claim that may be made by its manufacturer, is not guaranteed or endorsed by the publisher.
Acknowledgments
We thank Jan Null of CCM, San Jose State University, for permission to use the ONI graphic for Figure 3, Carlos Alvarez for helpful suggestions, Jonas Knape for providing R code of his models, and Samantha Monier for graphics. Ryan Merrill and Adrian Hinkle provided unpublished data on elegant terns in Washington and Oregon. We thank Jarrod Santora for many helpful suggestions, Ian Nisbet, Ruud van Halewijn, and David Schoch for discussion, and two reviewers for useful comments that enhanced the clarity of our presentation. RV and LM were supported by NSF DEB 2049303.
Footnotes
- ^ https://ebird.org/home
- ^ https://ggweather.com/enso/oni.htm
- ^ https://farm6.staticflickr.com/5585/15085202237_a14454c343_o.jpg
References
Amerson, A. B., and Shelton, P. C. (1976). The natural history of Johnson Atoll, central Pacific Ocean. Atoll Res. Bull. 192, 1–477. doi: 10.5479/si.00775630.192.1
Bach, L. A., Ripa, J., and Lundberg, P. (2007). On the evolution of conditional dispersal under environmental and demographic stochasticity. Evol. Ecol. Res. 9, 663–673.
Baker, R. R. (1978). The Evolutionary Ecology of Animal Migration. New York, NY: Holmes and Meier, 1012.
Barbraud, C., Johnson, A. R., and Bertault, G. (2003). Phenotypic correlates of post-fledging dispersal in a population of greater flamingos: the importance of body condition. J. Anim. Ecol. 72, 246–257.
Bartoń, K. A., Phillips, B. L., Morales, J. M., and Travis, J. M. (2009). The evolution of an ‘intelligent’ dispersal strategy: biased, correlated random walks in patchy landscapes. Oikos 118, 309–319.
Birdfellow (2021). WFO 2012 Trip (California and Oregon). Available online at: http://www.birdfellow.com/photos/thumbnails/620-wfo-2012-trip-california-and-oregon-?id=8179 (accessed November 19, 2021).
BOURC (2018). British Ornithologists’ Union Records Committee (BOURC): 49th report (October 2018). Ibis 160, 936–942. doi: 10.1111/ibi.12654
Burness, G. P., Lefevre, K. L., and Collins, C. T. (2020). “Elegant tern (Thalasseus elegans), version 1.0,” in Birds of the World, eds A. F. Poole and F. B. Gill (Ithaca, NY: Cornell Lab of Ornithology). doi: 10.2173/bow.eleter1.01
Clobert, J., Baguette, M., Benton, T. G., and Bullock, J. M. (2012). Dispersal Ecology and Evolution. Oxford: Oxford University Press.
Clobert, J., Le Galliard, J. F., Cote, J., Meylan, S., and Massot, M. (2009). Informed dispersal, heterogeneity in animal dispersal syndromes and the dynamics of spatially structured populations. Ecol. Lett. 12, 197–209.
Cornell Laboratory of Ornithology (2021). Elegant Tern Identification. Available online at: https://www.allaboutbirds.org/guide/Elegant_Tern/id (accessed November 19, 2021).
Davis, R. A., and Watson, D. M. (2018). Vagrants as vanguards of range shifts in a dynamic world. Biol. Conserv. 224, 238–241. doi: 10.1016/j.biocon.2018.06.006
DeSante, D. (1983). Vagrants: when orientation or navigation goes wrong. Point Reyes Bird Observ. Newslett. 61, 12–16.
DeSante, D. F., and George, T. L. (1994). Population trends in the landbirds of western North America. Stud. Avian Biol. 15, 173–190.
Dies, J. I., Chard, M., and Abad, A. (2019). Elegant terns breeding at L’Albufera de Valencia, Spain. Br. Birds 112, 110–117.
Dufour, P., Pons, J. M., Collinson, J. M., Gernigon, J., Dies, J. I., Sourrouille, P., et al. (2017). Multilocus barcoding confirms the occurrence of elegant terns in Western Europe. J. Ornithol. 158, 351–361. doi: 10.1007/s10336-016-1380-0
Fayet, A. L., Freeman, R., Shoji, A., Boyle, D., Kirk, H. L., Dean, B. J., et al. (2016). Drivers and fitness consequences of dispersive migration in a pelagic seabird. Behav. Ecol. 27, 1061–1072. doi: 10.1093/beheco/arw013
Gilman, E., Chaloupka, M., Read, A., Dalzell, P., Holetschek, J., and Curtice, C. (2012). Hawaii longline tuna fishery temporal trends in standardized catch rates and length distributions and effects on pelagic and seamount ecosystems. Aquat. Conserv. Mar. Freshw. Ecosyst. 22, 446–488.
Gwinner, E., and Wiltschko, W. (1978). Endogenously controlled changes in migratory direction of the garden warbler, Sylvia borin. J. Comp. Physiol. 125, 267–273. doi: 10.1007/bf00656605
Howell, S. N. G., Lewington, I., and Russell, W. (2014). Rare Birds of North America. Princeton, NJ: Princeton University Press.
Howell, S. N. G., and Webb, S. (1995). A Guide to the Birds of Mexico and Northern Central America. Oxford: Oxford University Press.
Johnson, N. K. (1994). Pioneering and natural expansion of breeding distributions in western North American birds. Stud. Avian Biol. 15, 27–44.
Kisdi, E., Utz, M., and Gyllenberg, M. (2012). “Evolution of condition-dependent dispersal,” in Dispersal Ecology and Evolution, eds J. Clobert, M. Baguette, T. G. Benton, and J. M. Bullock (Oxford: Oxford University Press), 139–151. doi: 10.1093/acprof:oso/9780199608898.003.0011
Knape, J. (2016). Decomposing trends in Swedish bird populations using generalized additive mixed models. J. Appl. Ecol. 53, 1852–1861. doi: 10.1111/1365-2664.12720
Lewis, M. A., Petrovskii, S. V., and Potts, J. R. (2016). The Mathematics Behind Biological Invasions. Berlin: Springer.
Liebl, A. L., and Martin, L. B. (2012). Exploratory behaviour and stressor hyper-responsiveness facilitate range expansion of an introduced songbird. Proc. R. Soc. B 279, 4375–4381. doi: 10.1098/rspb.2012.1606
Lovette, I. J., Seutin, G., Ricklefs, R. E., and Bermingham, E. (1999). The assembly of an island fauna by natural invasion: sources and temporal patterns in the avian colonization of Barbados. Biol. Invasions 1, 33–41.
Matthysen, E. (2012). “Multicausality of dispersal: a review,” in Dispersal Ecology and Evolution, eds J. Clobert, M. Baguette, T. G. Benton, and J. M. Bullock (Oxford: Oxford University Press), 3–18. doi: 10.1093/acprof:oso/9780199608898.003.0001
Moore, W. S., and Dolbeer, R. A. (1989). The use of banding recovery data to estimate dispersal rates and gene flow in avian species: case studies in the red-winged blackbird and common grackle. Condor 91, 242–253. doi: 10.2307/1368301
Nathan, R., Klein, E. K., Robledo-Arnuncio, J. J., and Revilla, E. (2012). “Dispersal kernels,” in Dispersal Ecology and Evolution, eds J. Clobert, M. Baguette, T. G. Benton, and J. M. Bullock (Oxford: Oxford University Press), 187–210.
Parkin, D. T., and Knox, A. G. (2010). The Status of Birds in Britain and Ireland. London: Christopher Helm.
Perez, G. S., Goodenough, K. S., Horn, M., Patton, R. T., Ruiz, E. A., Velarde, E., et al. (2020). High connectivity among breeding populations of the elegant tern (Thalasseus elegans) in Mexico and Southern California revealed through population genomic analysis. Waterbirds 43, 17–27. doi: 10.1675/063.043.0102
Phillips, J. (2000). Autumn vagrancy: “reverse migration” and migratory orientation. Ringing Migr. 20, 35–38. doi: 10.1080/03078698.2000.9674226
Pyle, P. (2008). Identification Guide to North American Birds, Part II. Point Reyes Station, CA: Slate Creek Press.
R Core Team (2020). R: A Language and Environment for Statistical Computing. Vienna: R Foundation for Statistical Computing.
Reed, J. M., Boulinier, T., Danchin, E., and Oring, L. W. (1999). “Informed dispersal,” in Current Ornithology, eds V. Nolan, E. D. Ketterson, and C. F. Thompson (Boston, MA: Springer), 189–259. doi: 10.1007/978-1-4757-4901-4_5
Ronce, O. (2007). How does it feel to be like a rolling stone? Ten questions about dispersal evolution. Annu. Rev. Ecol. Evol. Syst. 38, 231–253. doi: 10.1146/annurev.ecolsys.38.091206.095611
Santoro, S., Green, A. J., and Figuerola, J. (2013). Environmental instability as a motor for dispersal: a case study from a growing population of glossy ibis. PLoS One 8:e82983. doi: 10.1371/journal.pone.0082983
Schreiber, E. A. (2002). “Climate and weather effects on seabirds,” in Biology of Marine Birds, eds E. A. Schreiber and J. Burger (Boca Raton, FL: CRC Press), 197–234.
Stoddart, A., and Batty, C. (2019). The elegant tern in Britain and Europe. Br. Birds 112, 99–109. doi: 10.1093/jhmas/jrq045
Teitelbaum, C. S., and Mueller, T. (2019). Beyond migration: causes and consequences of nomadic animal movements. Trends Ecol. Evol. 34, 569–581. doi: 10.1016/j.tree.2019.02.005
Toochin, R., and Haviland, L. (2016). Status and Occurrence of Elegant Tern (Thalasseus elegans) in British Columbia. Available online at: http://ibis.geog.ubc.ca/biodiversity/efauna/documents/BCRareBirdListVersionXZABC.pdf (accessed November 17, 2020).
Toonen, R. J., and Pawlik, J. R. (2001). Foundations of gregariousness: a dispersal polymorphism among the planktonic larvae of a marine invertebrate. Evolution 55, 2439–2454. doi: 10.1111/j.0014-3820.2001.tb00759.x
van den Bosch, F., Hengeveld, R., and Metz, J. A. J. (1992). Analyzing the velocity of animal range expansion. J. Biogeogr. 19, 135–150.
Veit, R. R. (1997). Long-distance dispersal and population growth of yellow-headed blackbirds (Xanthocephalus xanthocephalus). Ardea 85, 135–143.
Veit, R. R. (2000). Vagrants as the expanding fringe of a growing population. Auk 117, 242–246. doi: 10.1093/auk/117.1.242
Veit, R. R., and Lewis, M. A. (1996). Dispersal, population growth, and the Allee effect: dynamics of the house finch invasion of eastern North America. Am. Nat. 148, 255–274. doi: 10.1086/285924
Veit, R. R., Zawadzki, L. C., Manne, L. L., Cales, P., Fibikar, D., Curley, S., et al. (2016). Vagrancy and colonization of St. Thomas and St. John, U.S. Virgin Islands by Adelaide’s Warblers Setophaga adelaidae. J. Caribb. Ornithol. 29, 47–50.
Velarde, E., Anderson, D. W., and Ezcurra, E. (2019). Seabird clues to ecosystem health. Science 365, 116–117. doi: 10.1126/science.aaw9999
Velarde, E., Ezcurra, E., Horn, M. H., and Patton, R. T. (2015). Warm oceanographic anomalies and fishing pressure drive seabird nesting north. Sci. Adv. 1:e1400210. doi: 10.1126/sciadv.1400210
Zawadzki, L. C., Veit, R. R., and Manne, L. L. (2019). The influence of population growth and wind on vagrancy in a North American passerine. Ardea 107, 131–147. doi: 10.5253/arde.v107i2.a2
Keywords: elegant tern, vagrancy, exploratory behavior, intelligent dispersal, colonization
Citation: Veit RR, Velarde E, Horn MH and Manne LL (2021) Population Growth and Long-Distance Vagrancy Leads to Colonization of Europe by Elegant Terns Thalasseus elegans. Front. Ecol. Evol. 9:725614. doi: 10.3389/fevo.2021.725614
Received: 16 June 2021; Accepted: 27 October 2021;
Published: 30 November 2021.
Edited by:
Mauricio Lima, Pontificia Universidad Católica de Chile, ChileReviewed by:
Amy Whitehead, National Institute of Water and Atmospheric Research (NIWA), New ZealandJeffery Sullivan, Natural Systems Analysts, Inc., United States
Copyright © 2021 Veit, Velarde, Horn and Manne. This is an open-access article distributed under the terms of the Creative Commons Attribution License (CC BY). The use, distribution or reproduction in other forums is permitted, provided the original author(s) and the copyright owner(s) are credited and that the original publication in this journal is cited, in accordance with accepted academic practice. No use, distribution or reproduction is permitted which does not comply with these terms.
*Correspondence: Richard Reed Veit, cnJ2ZWl0MjNAZ21haWwuY29t