- 1School of Geography and Remote Sensing, Guangzhou University, Guangzhou, China
- 2Department of Land, Environment, Agriculture and Forestry TESAF, University of Padova, Padua, Italy
- 3Rural Non-point Source Pollution Comprehensive Management Technology Center of Guangdong Province, Guangzhou, China
- 4Department of Ecology, College of Natural Resources and Environment, South China Agricultural University, Guangzhou, China
- 5Department of Agronomy, Animals, Food, Natural Resources and Environment DAFNAE, University of Padova, Padua, Italy
Sustained nitrogen (N) deposition has a wide-ranging impact on terrestrial ecosystems. However, still little attention has been paid to responses of urban soil fauna to the increasing N deposition. To clarify such effects on the soil properties and soil fauna in typical urban lawns (featuring Cynodon dactylon vegetation), a control experiment was conducted for 1 year, in which NH4NO3 was added as the external N source with four treatments of N addition: N0 (i.e., only water), N1 (50 kg N ha–1 yr–1), N2 (100 kg N ha–1 yr–1), N3 (150 kg N ha–1 yr–1). Results showed that N additions influence soil faunal communities in the urban lawns soil. The relative abundance of Oribatida increased with the N treatment level, partially replacing the more sensitive Collembola. Significant differences in the Shannon-Wiener and Margalef indices (p < 0.01) supported this statement. Although higher doses of N addition showed adverse effects on soil fauna communities, low N inputs increased the soil fauna diversity and richness, especially at 5–10 cm depth. A threshold effect appears to exist: low N addition (<25 kg N ha–1) did not negatively affect pedofauna structure and composition, while in the range from these values up to 50 kg N ha–1, the composition of the soil fauna underwent major changes which were confirmed by the decline of biodiversity indices. These changes are accompanied by the decreased pH values with increasing N inputs.
Introduction
Humans have modified more than 50% of Earth’s land surface (Hooke et al., 2012). The global nitrogen cycle has been dramatically perturbed by human activities (Decina et al., 2017), and the excessive nitrogen (N) deposition caused by fossil fuel emissions from industry and transportation has become a global ecological problem (Vitousek et al., 1997; Galloway et al., 2008; Bobbink et al., 2010). Environmental effects of N deposition on vegetation, soil, and water have been described at a global scale (Vitousek et al., 1997; Sutton et al., 2014), as well as at those of single continent or nation (Liu et al., 2011; Stevens et al., 2011). Existing reports predict that N deposition levels are not expected to decrease in the next decades and will probably further increase in Asian regions along with population growth and economic development (Kanakidou et al., 2016). Featuring one of the highest loads of nitrogen deposition, China has recorded rapidly increasing values, especially in areas with high-intensity human activities (Liu et al., 2013; Jia et al., 2014).
With the fast-growing urbanization all around the world, urban ecosystems are the most intense areas of human activity and the urbanization process has led to a rapid transformation of man-inhabited habitats with a profound impact on biodiversity (McKinney, 2006; Seto et al., 2012). Nitrogen emissions are the main source of nitrogen deposition, and urban areas are the ones where the phenomenon shows its maximum rates (Templer et al., 2015). The increased deposition in the urban areas was likely due to the volatilization and redeposition of N applied as mineral fertilizer or manure (Fahey et al., 1999; Bettez and Groffman, 2013). In terrestrial ecosystems, especially in nutrient-poor, N-limited ones, or those with weak acid-buffering capability, increasing N deposition leads to soil cations leaching, which causes soil acidification (Simkin et al., 2016). Therefore, N deposition heavily affects urban abiotic and biotic soil properties (Shi et al., 2016). Urban soils undertake a series of functions and services in the urban ecosystem, such as support (soil nutrient cycling or habitat space), regulation (climate and water balance), carrying or cultural services (Rawlins et al., 2015; Tresch et al., 2018), which are essential for an ecologically livable city (Elmqvist et al., 2015). Therefore, urban green spaces, amidst a concrete-dominated landscape, are becoming important refuges for native biodiversity in urban and suburban areas (Goddard et al., 2010). Improving the conditions for the biodiversity-hosting service in cities has been pointed out as a major contribution to the quality of life for city dwellers (Tzoulas et al., 2007; Standish et al., 2013).
The soil fauna community, the “engineer of the soil ecosystem,” along with the microbial counterpart, is endowed with remarkable values in terms of abundance and biodiversity and is an integral and crucial part of the soil ecosystem (Heneghan and Bolger, 1996; Wardle et al., 2004; Xu et al., 2006; Payne et al., 2012). The living soil biota represent the highest proportion of the planet’s species and are the main drivers of the ecosystem functions (Ochoa-Hueso et al., 2014), which have drawn increasing attention in environmental biodiversity studies (Fu et al., 2009). Some prior studies described changes in soil fauna both in aquatic and terrestrial systems as a result of increased N deposition (Berg and Verhoef, 1998; Xu et al., 2009). In N-limited ecosystems, soil fauna communities are affected by N deposition. Effects of increased nitrogen fallout on soil fauna could be determined by increased available N in soils, whose dominant forms are ammonium and nitrate N (Heneghan and Bolger, 1996; Ochoa-Hueso et al., 2011). Changes in soil fauna communities could also be operated through soil acidification and eutrophication (van Straalen and Verhoef, 1997; Xu et al., 2009; Stevens et al., 2014). Moreover, in comparison to other sites, the urban area has multiple burdens of pollution. Metals and in particular heavy metals content in urban soils can contribute to toxicity effects to biota (Shifav, 2018). However, studies investigating the impacts of increased nitrogen deposition on soil faunal community structure and composition are still scarce and virtually non-existing in urban ecosystems. It is known that in agricultural soils the communities of collembola are composed by few species, well adapted to these intensely exploited environments. Instead, the collembolans of the city soils show more varied and richer in species, with levels closer to those found in forests (Santorufo et al., 2015). The network that binds together the soil organisms and those living outside the soil is very complex (Zanella et al., 2018). This study is in the wake of those works which attempt to circumscribe at least the most fundamental aspects of the soil functioning. In particular, the soil organisms are related to available nitrogen and soil pH at different profile depths.
In essence, nitrogen deposition has not yet received due attention to clarify its effects on urban ecosystem functions and processes. In the absence of a thorough understanding of the causal mechanisms behind its effects on urban soil fauna species and their diversity, we cannot plan an optimized conservation or restoration measures. Although research in urban lawns has demonstrated that these landscapes have relatively high rates of N cycling and retention compared to unmanaged ecosystems (Groffman et al., 2009; Martinez et al., 2014; Trammell et al., 2016), the N deposition effects on soil fauna in these particular habitats had not been thoroughly explored yet. While observational surveys have been gathered, randomized trials with the possibility of manipulating the variables had not yet been carried out in these important habitats. Hence, to identify the risks caused by increasing N pollution to soil fauna and its diversity in urban ecosystems, we set up a simulated atmospheric nitrogen deposition-controlled experiment. The lawns, planted with Bermuda grass (Cynodon dactylon), which is one of the most popularly used gramineous plants in recreational greens, were selected as the research target. The working hypothesis of the present investigation was that, similar to what occurs in other ecosystems, nitrogen deposition can have a dose-dependent significant impact on soil fauna in urban lawns. The questions tested were the following: (1) Will the composition and diversity of soil fauna change in urban meadow soils subjected to incremental and repeated nitrogen supplementation in comparison to the control background? (2) at which soil depth and with which temporal dynamics would the possible effects mostly occur? (3) Are some pedofaunal taxonomical groups more sensitive than others to the nitrogen deposition stress? (4) In case of negative impact, which is the safety threshold of supplementation below which no significant changes in the diversity and/or abundance of soil arthropods would be detectable? (5) Are nitrogen compounds themselves a direct cause of the possible arthropod diversity disorders or are there other consequential factors enacting on the sensitive biota?
Materials and Methods
Study Site and Experimental Design
This study was conducted in a green space experimental field at Guangzhou University, Guangzhou, China (113°38′E, 23°04′N, Figure 1). The climate of the area is a typical subtropical monsoon, with warm and wet summer, and short and dry winter. The annual average temperature is 21.5°C and the average relative humidity is 77%. The hottest month is July, with a mean monthly temperature of 28.7°C. The coldest month is January, and the monthly average temperature is 9∼16°C. The rainy season is from April to September, and the annual rainfall is 1727.22 mm. The nitrogen deposition inputs in the four districts (Baiyun, Tianhe, Luogang, and Conghua) of Guangzhou, with average N deposition levels of 43.3, 41.2, 35.2, and 30.1 kg N ha–1 yr–1, respectively (Huang et al., 2012).
The experiment started in May 2016 and continued until April 2017. A randomized block design was used with four treatments and five replicate plots of each treatment. Twenty 2 m × 2 m plots were arranged in a 4 × 5 matrix. 50 g of C. dactylon seeds were evenly sown in each plot. The distance between any two adjacent plots was 0.5 m. NH4NO3 was added in a water solution as external N source once a month, and the final N input corresponded to N0 (i.e., only water), N1 (50 kg N ha–1 yr–1), N2 (100 kg N ha–1 yr–1), N3 (150 kg N ha–1 yr–1). Total annual N applications for the three N addition treatments were used according to the study of Xu et al. (2006). The input values of N correspond, respectively, to those of low, medium and high annual average N fertilization for common crops as wheat, barley and rice (van Grinsven et al., 2012). It needs to be remarked that fertilization was progressively reiterated and that the above correspondence to agricultural dosages is intended as the one reached at the end of the year. Up to 6 months fertilization remained therefore below average agricultural levels even in the case of the highest dosage. The whole experimental field and each sample plot were fenced off to prevent disturbance.
Soil Sampling and Analysis
At 3rd, 6th, 12th month after the experiment started, in each replicate plot, three soil cores from a 0 to 5 cm and three from a 5 to 10 cm depth, were sampled and extracted on a Tullgren funnel for 48 h. Ethanol 70% was used as conservation fluid and the soil fauna obtained was analyzed using a Leica S8 APO microscope (Leica, Germany) to reach taxonomical identification. Individuals of Oribatida, Prostigmata, and Mesostigmata were identified to order level, while others were identified to family or genus level according to Yin (1998).
For soil chemical properties 3 soil subsamples from the 0 to 5 cm and 5 to 10 cm depth from each plot were taken and mixed thoroughly into a composite sample. The samples were air-dried for several days and stored at room temperature in the dark until analysis. Soil pH (water: soil = 2.5:1) was determined using a pH meter. Nitrate N (NO3––N) and ammonium N (NH4+–N) were extracted from 10 g of soil using 2 M KCl and determined using a San++ Continuous Flow Analyzer (Skalar, Breda, Netherlands). Soil total carbon (TC) was determined by the dichromate digestion method, and total nitrogen (TN) was measured by the Kjeldahl method. For determining total phosphorus (TP) concentrations, soil samples were digested in perchloric acid-sulfuric acid (HClO4-HSO4) and sodium hydroxide (NaOH) mixtures, respectively. Total phosphorus (TP) was determined using colorimetry in an atomic absorption spectrophotometer (TAS-986, PGENERAL, Beijing, China). Soil chemical properties before the beginning of the trial (means of four replicates ± standard deviation) were the following: pH: 8.28 ± 0.06; ammonium N (mg kg–1): 1.54 ± 0.10; nitrate N (mg kg–1): 3.37 ± 0.03; total N (g kg–1): 0.61 ± 0.06; total C (g kg–1): 13.41 ± 0.96; total P (g kg–1): 0.25 ± 0.01 (Mo et al., 2018).
Statistical Analysis
To evaluate the differences in soil fauna abundance and diversity, we employed multiple indices, as studies have determined that single ones may miss some of the aspects of the possible biodiversity change (Bellard et al., 2012). The community abundance (individual density) and taxa richness (number of taxa per sample) in each sample were calculated (Gonçalves et al., 2020). Community parameters analyzed were the following: diversity using Density-Group (DG) and Shannon–Wiener (H′) indices, richness and evenness using Pielou (J) and Margalef (D) indices (Shannon, 1948; Margalef, 1968; Pielou, 1969; Liao et al., 1997).
We employed Multivariate analysis of variance (MANOVA for repeated measures) to test the significance of differences of the measured indices in the soil layers among treatments over time. The matrix was square-root transformed before being subjected to MANOVA. Chi-square tests were performed for the major soil fauna taxa. The principal component analysis method (PCA) was used to analyze how the major soil fauna taxa and diversity indices were influenced by N addition after 12 months, a statistical analysis of 9 factors was conducted. These factors included community abundance, taxa richness, Density-Group (DG), Shannon–Wiener (H′), Pielou (J), and Margalef (D) indices, dominant groups (the number of individuals represented over 10% of the total sample), which were sorted as follows: Oribatida, Collembola, and Mesostigmata.
We used R software (function “MANOVA,” package “BruceR”) to perform the MANOVA for repeated measures. RDA was conducted to analyze the relationships between soil fauna communities and soil chemical properties using the “rda” fuction in the “vegan” package. IBM SPSS Statistics (version 25.0) was used to perform the Chi square test and the PCA. We created the figures with R software (package “ggplot2”).
Results
Community Composition of Soil Fauna in Different Nitrogen Treatments
A total of 3820 individuals of soil fauna, distributed in 16 Classes or Orders or Suborders, encompassing 48 taxa, identified either at Order or Class or Suborder or Family or Genus level, were detected (Supplementary Table 1). The dominant faunal group was that of the Suborder Oribatida, followed by Order Collembola and Order Mesostigmata. These groups contain, respectively, 46.24, 26.48, and 12.82% of the fauna individuals found in the soil.
When categorizing the dataset according to the sampling date (Figure 2), the structure of the soil fauna at order level did not change particularly. After 6 months of N addition, however, the proportion of Oribatida in the plots with N2 and N3 treatment began to increase, while the proportion of Collembola decreased, and the other groups did not change significantly. But at twelfth month, the proportion of Collembola under N2 and N3 treatment significantly increased. Chi-square test statistic results showed that Oribatida (X2 = 37.08, p < 0.001) and Collembola (X2 = 191.18, p < 0.001) differed significantly across the different N treatment.
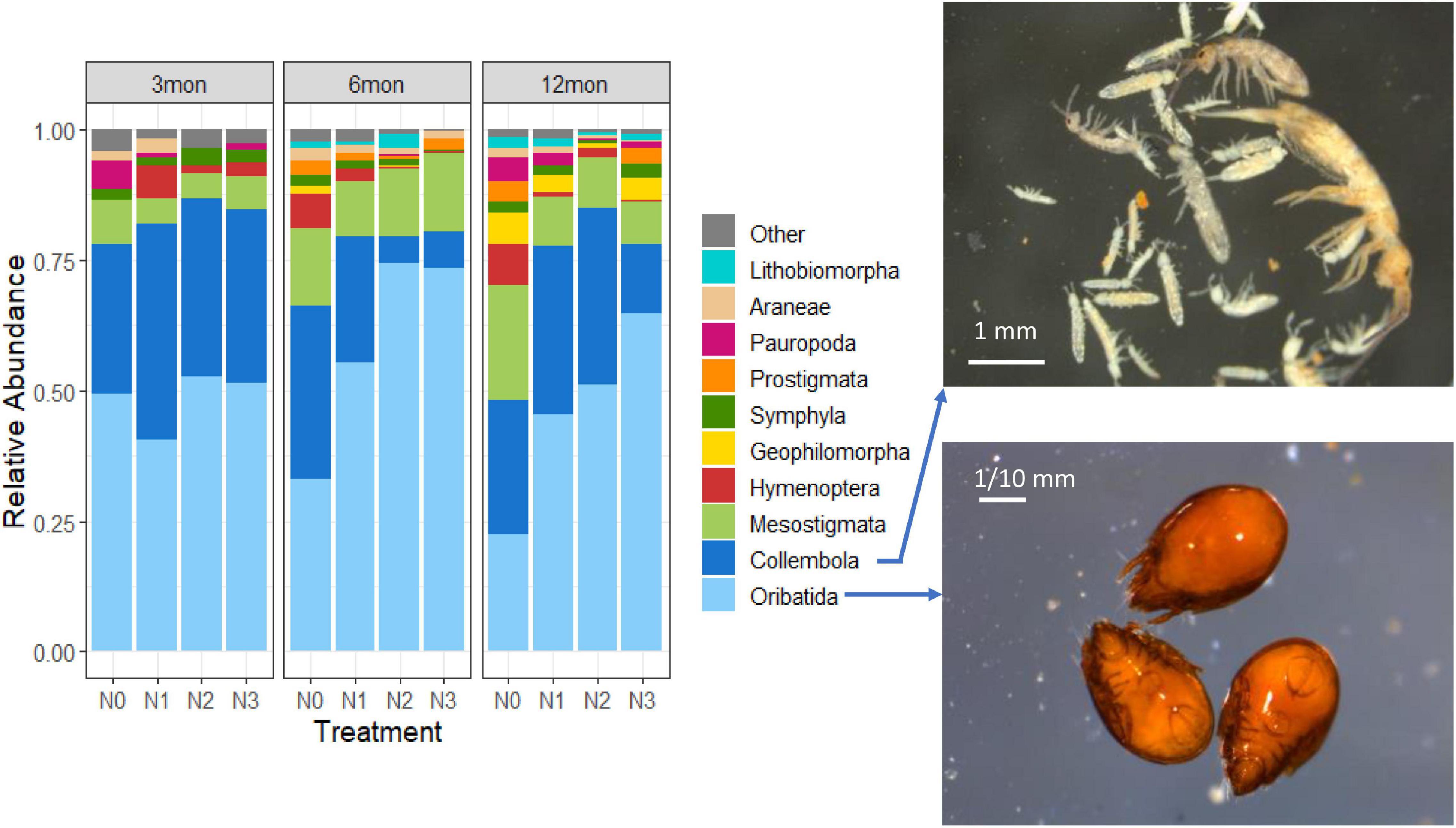
Figure 2. Left: Community composition (0–10 cm) of pedofauna populations associated with the increasing N doses added to the soil in relation to the duration of the treatment. The groups accounting for ≥ 1% are shown with explicit nomenclature, while those < 1% are integrated into “Other.” Right: Examples of Collembola (top panel) and Oribatida (bottom panel) individuals.
Response of Soil Fauna Abundance and Biodiversity Indices to N Treatment
To detect the potential effects of N treatments, we performed a MANOVA on the community abundance and taxa richness, and Density-Group, Shannon-Winner, Margalef and Pielou diversity indices (Table 1). The N addition treatment had significant effects on the taxa richness, Shannon-Winner index and Margalef index (p < 0.01) and Density-Group index (p < 0.05). Treatment duration had significant effects on the community abundance, taxa richness (p < 0.001), and Density-Group index and Shannon-Winner index (p < 0.05). The soil layer depth showed significant effects on the community abundance, taxa richness and Pielou index (p < 0.001), and Density-Group index (p < 0.05). Furthermore, The N addition treatment and soil layer depth showed a significant interaction influence on the taxa richness and Margalef index (p < 0.05). Treatment duration and soil layer depth also had a significant interaction effect on Density-Group index (p < 0.05).
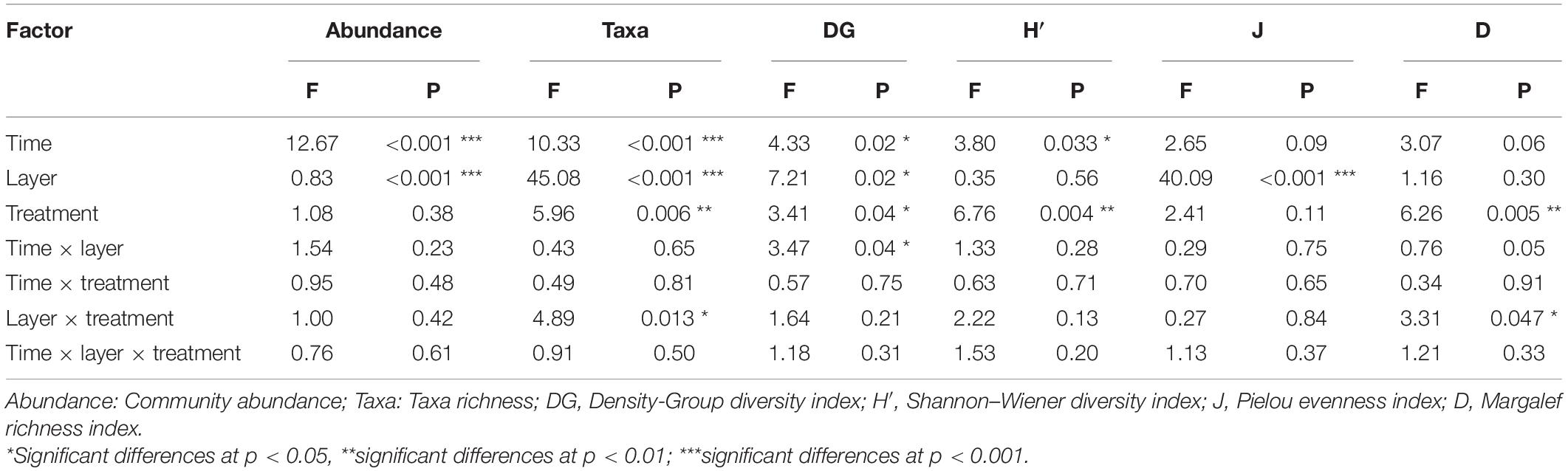
Table 1. Main and interaction effects of the N addition period (Time), soil layer depths (Layer) and N addition treatments (Treatment) on community abundance, taxa, and diversity indices (MANOVA results are showed).
As evidenced by multiple biodiversity indices in both soil layers, the N2 and N3 treatments decreased the diversity and taxa richness of soil fauna in comparison to the control (N0) (Figure 3). When compared at equal treatment duration, the differences among the treatments in 0–5 cm soil layer appeared at sixth month for taxa richness, Density-Group index, Shannon-Wiener index and Margalef index, but at 5–10 cm soil layer, the differences were detected only at twelfth month (Figure 3). The Pielou evenness index instead appeared insensitive to the increase of N doses, and was higher in deep layer (5–10 cm) than that in the surface one (0–5 cm) (Figure 3E).
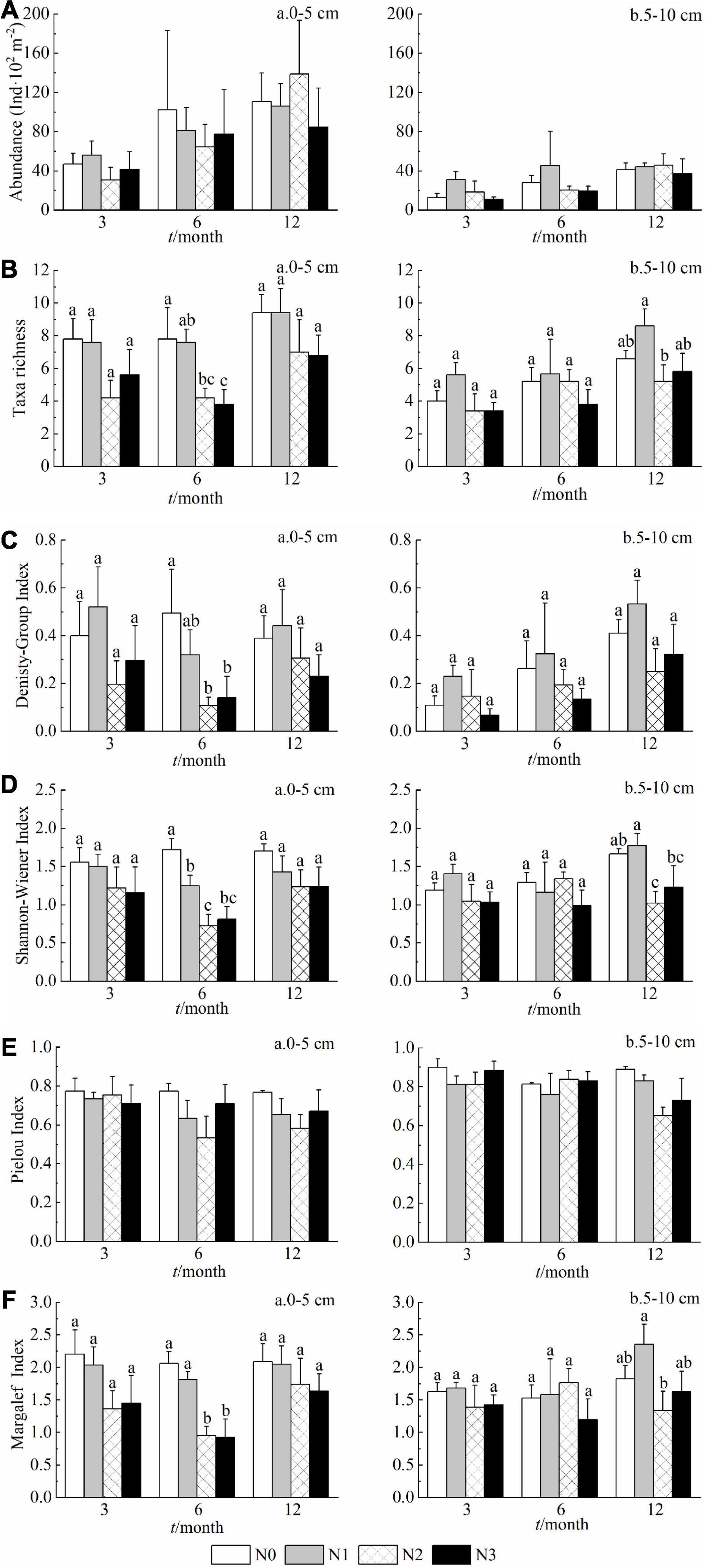
Figure 3. Effects of N addition on the community abundance (A), taxa richness (B), and diversity indices (C–F) across sampling dates in 0–5 cm and 5–10 cm layer (a and b, respectively). Data are means ± SE (n = 5). Results of post hoc tests among treatments are provided with lowercase letters only when the effects of the N treatment in the MANOVA are significant (p < 0.05) or marginally significant (0.05 < p < 0.1).
Relationships of Soil Fauna With Abiotic Factors
According to principal component analysis (PCA), the eigenvalues of the correlation matrix of the first two components were both greater than 1, reaching 3.839 and 3.011, respectively. The cumulative contribution rate reached the high value of 76.108% (Supplementary Table 2). The Shannon-Wiener and Margalef indices, and taxa richness, with the eigenvectors of 0.968 and 0.920 and 0.825, respectively, contributed to the first main component. The Pielou and Density-Group indices followed, with eigenvectors of 0.684 and 0.666, respectively. For the second major component, the community abundance (eigenvector of 0.924) had a main contribution, followed by Oribatida individuals (eigenvector of 0.768), the Density-Group index (eigenvector of 0.698), and Mesostigmata individuals (eigenvector of 0.650) (Supplementary Table 2). These results reflect the relative abundance dynamics of the different soil fauna groups. In Figure 2 the oscillations of Mesostigmata, Collambola and Oribata are very evident.
In the Redundancy analysis (RDA), it indicated that soil pH and NH4+-N were determining variables for the soil fauna’s main taxa and indices, followed by NO3–-N. Soil pH positively correlated to most soil fauna parameters, while NH4+-N showed a negative correlation to them. Increasing soil acidity corresponded to increasing soil fauna diversity indices (Figure 4).
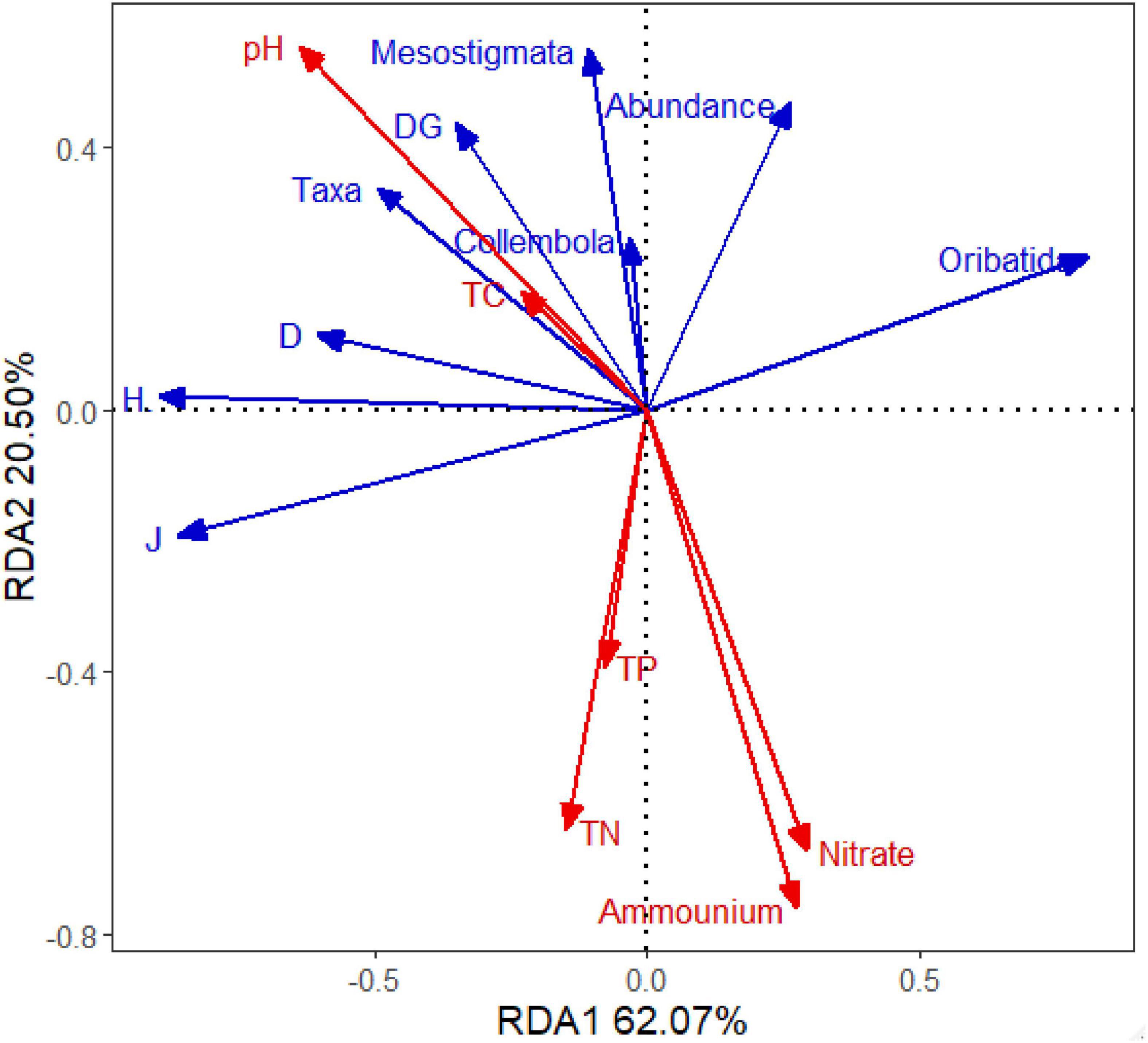
Figure 4. Results of redundancy analysis of main taxa and indices of soil fauna in association with soil chemical properties. Soil fauna data are labeled as: Abundance (community abundance); Taxa (Taxa richness); Oribatida individuals; Collembola individuals; Mesostigmata individuals; DG (Density-Group index); H′ (Shannon–Wiener index); J (Pielou index); D (Margalef index). Soil chemical properties are labeled as: Ammonium (ammonium nitrogen); Nitrate (nitrate nitrogen); TN (total nitrogen); TC (total carbon); TP (total phosphorus).
Discussion
Evidence of a Nitrogen Threshold-Related Impact
Especially in the first 5 cm of soil, the abundance and taxa of soil fauna decreased with an increasing nitrogen addition, across the three sampling periods, in comparison to the control site. Most of the soil fauna indices tended to decrease in the first 5 cm of soil surface under increasing N additions (significantly for H′ and D). N input was therefore observed as a suppressing factor for soil fauna. The negative effects of N deposition on mesofauna communities had already been highlighted by numerous works (Xu et al., 2007; Ochoa-Hueso et al., 2014; Song et al., 2015; Liu et al., 2020).
Within the range of N inputs used in the tests, it was possible to individuate a threshold N-value and the effects that the use of higher doses can have on arthropods. Xu et al. (2007) reported that soil fauna increased in the lower nitrogen treatments and that there was a threshold around 100 kg N ha–2 yr–1 for N deposition in southern China. Then, adding rates of about 105 kg N ha–2 yr–1, Bai et al. (2010) proved that an ecosystem under this dosage in northern China would reach nitrogen saturation. The same type of response to increasing N deposition was also described for soil fauna in European regions. In central Spain, upon additions within ranges comparable to ambient N deposition, a critical N point was recorded between 20 and 50 kg N ha–2 yr–1 (Pinho et al., 2012; Ochoa-Hueso et al., 2014). These authors suggested 26 kg N ha–1 yr–1 as a critical point for N deposition in Mediterranean ecosystems.
We found that at low doses (<50 N kg ha–1) the number of groups increased (DG index) but that the abundance changed more in some groups than that in others, with lowering the Shannon index (H′). From Figure 2, it can be clearly seen that the animals’ groups did not undergo major changes after 3 months from the beginning of the treatments. After 6 months, the change was noticeable with the N1 treatment, but it appeared relatively much clearer under the N2 and N3 treatments (Figure 2). Yearly N doses are assessable, knowing the setup as follows by dividing by 12 and then multiplying by the number of elapsed months: N1 = (50/12) ∗ 6 = 25; N2 = (100/12) ∗ 6 = 50; and N3 = (150/12) ∗ 6 = 75 kg ha−1. An effect appeared at 25 kg N ha−1; fauna had already changed at 50 kg N ha−1 and continued to experience stress with higher doses of 75 kg N ha−1.
The other objective of this study was to observe the response of soil fauna in different soil layers under increasing N addition. Generally speaking, surface soil gets better resource conditions including supply of oxygen, water, and organic matter to arthropods than deeper soil which shows relatively harsher living conditions for these animals (Wei et al., 2017). Xu et al. (2009) found that the effects of N addition on arthropod density, group richness, and diversity indices were especially strong in the first 0–5 cm soil layer, and that the stratification of soil fauna tended to disappear after a long-term N treatment. Our results confirm these outcomes (Table 1 and Figures 3A,B). However, we noticed that diversity and richness indices under N1 were still higher than N0 after 12 months at both soil depths. Besides, at higher N addition, the groups of animals benefit from a low N fertilization at deeper depth between 5 and 10 cm (Table 1 and Figures 3C–F). A substantial proportion of the deposited N could be retained by the surface soil, which thereby would reduce the N input reaching the deeper soil layer (Providoli et al., 2006). The threshold in the nitrogen dose calculated above could therefore be considered as valid for the first 5 cm of soil layer and not for deeper layers.
The Effect of Nitrogen Addition on Soil Fauna Community Structure
Oribatida, Collembola, and Mesostigmata reacted more than other Arthropod groups to nitrogen inputs. With doses N2 and N3, Oribatida almost completely replaced Collembola after 6 months. However, after 12 months Collembola recovered to a partial balance with the N2 treatment and Oribatida replaced part of the Collembola niche with the N3 treatment. Notably, Oribatida individuals increased along with the N addition time (Supplementary Table 1).
It is well known that soil fauna taxa and diversity respond to soil acidification (Ponge and Chevalier, 2006; Treseder, 2008; Auclerc et al., 2012; Serna-Chavez et al., 2013; Wang et al., 2015), and that soil faunal communities altered by an increasing N deposition can also be influenced by a decreasing soil pH (Badiane Ndour et al., 2008; Rousk et al., 2010; Ochoa-Hueso et al., 2014). Thus, nitrogen can indirectly affect soil fauna communities by consequent soil acidification (Treseder, 2008; Serna-Chavez et al., 2013), but the effects of soil acidification and N addition on soil fauna are difficult to separate.
An increasing abundance of Collembola with N additions or with an increasing available resource was suggested by Ochoa-Hueso et al. (2014) and Sun et al. (2020), respectively. According to our RDA results, soil pH was positively correlated with Collembola and Mesostigmata individuals, but ammonium and nitrate impacted Collembola and Mesostigmata negatively.
On the other side, in our study the Acari Subclass did not respond to available N, and soil pH was not significantly correlated to Acari abundance. In fact, Acari may tend to be more tolerant to low pH and environmental changes (Makkonen et al., 2011; Bokhorst et al., 2012; Zanella et al., 2018). We suggest that alterations in soil pH due to continuous N addition could negatively and significantly impact Collembola populations. The consequent decrease in soil pH can affect Collembola abundance. On the contrary, Acari population can increase because they tolerate acidic conditions and take advantage of the reduced Collembola competition.
N addition caused changes in the soil chemistry such as pH and N availability, which was consistent with other relevant studies (Vourlitis and Fernandez, 2012). These trends were confirmed by ANOVA, which revealed a change in pH in soil samples at the end of the treatments even with N1 doses, a difference of NH4+ and NO3– starting with N2 doses, and a difference of TN with N3 doses (Supplementary Table 3). In the first 5 cm of soil layer, at the end of twelfth month of N inputs, soil samples began to show a different pH with N1 doses.
With this observation in mind and looking at Figure 2, we can state that the changes at the fauna level started as early as after 6 months and as low as under N1 doses. This suggests that fauna did not react directly to the N amount but to the soil pH, and that this change is supposedly caused by doses between 25 and 50 kg ha–1 of nitrogen, as calculated above. We can therefore infer that N deposition does not appear to affect soil fauna community composition directly, but rather indirectly by decreasing soil pH. A more comprehensive and dynamic approach is needed to couple soil pedological and biological processes and to combine current experimental and theoretical knowledge (Briones, 2018). Our reasoning on pH is also based on the fact that it can profoundly affect the bacterial communities which on a lower ecological level, it could in turn affect the soil faunal components. In this regard, our data can be compared with a parallel analysis that we carried out from the same experiment and setup, in which we analyzed soil bacterial communities by culture-independent 16S DNA metabarcoding (Mo et al., 2021), also finding a dramatic drop in microbial diversity and evenness in a dose and time-dependent fashion. Thus, the relevance of pH as a factor that could act in this sense, is supported by such prior analysis of the bacterial communities on the very same plots, in which we demonstrated the parallel effects on the bacterial trophic level, which is consistent with those displayed on arthropods described in the present study.
Conclusion
In this study, we observed that nitrogen addition treatments, simulating N deposition, induce changes in soil fauna community composition and diversity of urban green spaces. The negative effects of higher doses of N addition on soil fauna diversity were stronger in the first 0–5 cm of soil than in a deeper soil layer (5–10 cm). Although N addition showed negative effects on some soil fauna communities, Oribatida maintained a high abundance even in soils with high nitrogen input. We pointed out a clear threshold effect of N addition: low N input (<25 kg N ha–1) in the urban area had even some positive effect on all fauna communities. The low-dose N addition to some extent increased the soil fauna diversity and richness, especially in the lower (5–10 cm) soil layers. However, after a critical nitrogen amount of 50 kg N ha–1, mesofauna radically changed. With doses N2 and N3, the Oribatida almost completely replaced the Collembola populations after 6 months. Soil then underwent a partial resilient recovery and a new balance by the end of the experiment.
N addition decreased soil pH which, in turn, affected the mesofauna structure and biodiversity. A long-term monitoring of at least 12 months appears therefore necessary to explain urban soil fauna living strategy and to identify the mechanisms behind possible changes due to N deposition.
Data Availability Statement
The original contributions presented in the study are included in the article/Supplementary Material, further inquiries can be directed to the corresponding author/s.
Author Contributions
GX designed the experiments. LM, XC, BP, and GX contributed to the field samples and data collection. LM and XC contributed to the soil mesofauna taxa identification. BP, SY, and XC analyzed the soil property. LM, AZ, and AS conducted the statistical analysis. LM, AZ, AS, JZ, ZW, and SY wrote and revised the manuscript. All authors contributed to the article and approved the submitted version.
Funding
This work was supported by the National Natural Science Foundation of China (Grant Nos. 42071061, U1701236, and 41571247).
Conflict of Interest
The authors declare that the research was conducted in the absence of any commercial or financial relationships that could be construed as a potential conflict of interest.
Publisher’s Note
All claims expressed in this article are solely those of the authors and do not necessarily represent those of their affiliated organizations, or those of the publisher, the editors and the reviewers. Any product that may be evaluated in this article, or claim that may be made by its manufacturer, is not guaranteed or endorsed by the publisher.
Acknowledgments
We express our gratitude to Guangzhou University for the scholarship.
Supplementary Material
The Supplementary Material for this article can be found online at: https://www.frontiersin.org/articles/10.3389/fevo.2021.711774/full#supplementary-material
References
Auclerc, A., Nahmani, J., Aran, D., Baldy, V., Callot, H., Gers, C., et al. (2012). Changes in soil macroinvertebrate communities following liming of acidified forested catchments in the Vosges Mountains (North-eastern France). Ecol. Eng. 42, 260–269. doi: 10.1016/j.ecoleng.2012.02.024
Badiane Ndour, N. Y., Achouak, W., Christen, R., Heulin, T., Brauman, A., and Chotte, J. L. (2008). Characteristics of microbial habitats in a tropical soil subject to different fallow management. Appl. Soil Ecol. 38, 51–61. doi: 10.1016/j.apsoil.2007.09.001
Bai, Y., Wu, J., Clark, C. M., Naeemz, S., Pan, Q., Huang, J., et al. (2010). Tradeoffs and thresholds in the effects of nitrogen addition on biodiversity and ecosystem functioning: evidence from inner Mongolia Grasslands. Glob. Chang. Biol. 16, 358–372. doi: 10.1111/j.1365-2486.2009.01950.x
Bellard, C., Bertelsmeier, C., Leadley, P., Thuiller, W., and Courchamp, F. (2012). Impacts of climate change on the future of biodiversity. Ecol. Lett. 15, 365–377. doi: 10.1111/j.1461-0248.2011.01736.x
Berg, M. P., and Verhoef, H. A. (1998). Ecological characteristics of a nitrogen-saturated coniferous forest in The Netherlands. Biol. Fertil. Soils 26, 258–267. doi: 10.1007/978-94-011-2866-7
Bettez, N. D., and Groffman, P. M. (2013). Nitrogen deposition in and near an urban ecosystem. Environ. Sci. Technol. 47, 6047–6051. doi: 10.1021/es400664b
Bobbink, R., Hicks, K., Galloway, J., Spranger, T., Alkemade, R., Ashmore, M., et al. (2010). Global assessment of nitrogen deposition effects on terrestrial plant diversity: a synthesis. Ecol. Appl. 20, 30–59. doi: 10.1890/08-1140.1
Bokhorst, S., Phoenix, G. K., Bjerke, J. W., Callaghan, T. V., Huyer-Brugman, F., and Berg, M. P. (2012). Extreme winter warming events more negatively impact small rather than large soil fauna: shift in community composition explained by traits not taxa. Glob. Chang. Biol. 18, 1152–1162. doi: 10.1111/j.1365-2486.2011.02565.x
Briones, M. J. I. (2018). The serendipitous value of soil fauna in ecosystem functioning: the unexplained explained. Front. Environ. Sci. 6:149. doi: 10.3389/fenvs.2018.00149
Decina, S. M., Templer, P. H., Hutyra, L. R., Gately, C. K., and Rao, P. (2017). Variability, drivers, and effects of atmospheric nitrogen inputs across an urban area: emerging patterns among human activities, the atmosphere, and soils. Sci. Total Environ. 609, 1524–1534. doi: 10.1016/j.scitotenv.2017.07.166
Elmqvist, T., Setälä, H., Handel, S. N., van der Ploeg, S., Aronson, J., Blignaut, J. N., et al. (2015). Benefits of restoring ecosystem services in urban areas. Curr. Opin. Environ. Sustain. 14, 101–108. doi: 10.1016/j.cosust.2015.05.001
Fahey, T. J., Williams, C. J., Rooney-Varga, J. N., Cleveland, C. C., Postek, K. M., Smith, S. D., et al. (1999). Nitrogen deposition in and around an intensive agricultural district in central New York. J. Environ. Qual. 28, 1585–1600. doi: 10.2134/jeq1999.00472425002800050025x
Fu, S., Zou, X., and Coleman, D. (2009). Highlights and perspectives of soil biology and ecology research in China. Soil Biol. Biochem. 41, 868–876. doi: 10.1016/j.soilbio.2008.10.014
Galloway, J. N., Townsend, A. R., Erisman, J. W., Bekunda, M., Cai, Z., Freney, J. R., et al. (2008). Transformation of the nitrogen cycle: recent trends, questions, and potential solutions. Science 320, 889–892. doi: 10.1126/science.1136674
Goddard, M. A., Dougill, A. J., and Benton, T. G. (2010). Scaling up from gardens: biodiversity conservation in urban environments. Trends Ecol. Evol. 25, 90–98. doi: 10.1016/j.tree.2009.07.016
Gonçalves, F., Nunes, C., Carlos, C., López, Á, Oliveira, I., Crespí, A., et al. (2020). Do soil management practices affect the activity density, diversity, and stability of soil arthropods in vineyards? Agric. Ecosyst. Environ. 294:106863. doi: 10.1016/j.agee.2020.106863
Groffman, P. M., Williams, C. O., Pouyat, R. V., Band, L. E., and Yesilonis, I. D. (2009). Nitrate leaching and nitrous oxide flux in urban forests and grasslands. J. Environ. Qual. 38, 1848–1860. doi: 10.2134/jeq2008.0521
Heneghan, L., and Bolger, T. (1996). Effect of components of’acid rain’on the contribution of soil microarthropods to ecosystem function. J. Appl. Ecol. 33, 1329–1344. doi: 10.2307/2404774
Hooke, R. L. B., Martín-Duque, J. F., and Pedraza, J. (2012). Land transformation by humans: a review. GSA Today 22, 4–10. doi: 10.1130/GSAT151A.1
Huang, L., Zhu, W., Ren, H., Chen, H., and Wang, J. (2012). Impact of atmospheric nitrogen deposition on soil properties and herb-layer diversity in remnant forests along an urban-rural gradient in Guangzhou, Southern China. Plant Ecol. 213, 1187–1202. doi: 10.1007/s11258-012-0080-y
Jia, Y., Yu, G., He, N., Zhan, X., Fang, H., Sheng, W., et al. (2014). Spatial and decadal variations in inorganic nitrogen wet deposition in China induced by human activity. Sci. Rep. 4, 1–7. doi: 10.1038/srep03763
Kanakidou, M., Myriokefalitakis, S., Daskalakis, N., Fanourgakis, G., Nenes, A., Baker, A. R., et al. (2016). Past, present, and future atmospheric nitrogen deposition. J. Atmos. Sci. 73, 2039–2047. doi: 10.1175/JAS-D-15-0278.1
Liao, C. H., Li, J. X., and Huang, H. T. (1997). Soil animal community diversity in the forest of the southern subtropical region, China. Acta Ecol. Sin. 17, 549–555.
Liu, T., Mao, P., Shi, L., Eisenhauer, N., Liu, S., Wang, X., et al. (2020). Forest canopy maintains the soil community composition under elevated nitrogen deposition. Soil Biol. Biochem. 143:107733. doi: 10.1016/j.soilbio.2020.107733
Liu, X., Duan, L., Mo, J., Du, E., Shen, J., Lu, X., et al. (2011). Nitrogen deposition and its ecological impact in China: an overview. Environ. Pollut. 159, 2251–2264. doi: 10.1016/j.envpol.2010.08.002
Liu, X., Zhang, Y., Han, W., Tang, A., Shen, J., Cui, Z., et al. (2013). Enhanced nitrogen deposition over China. Nature 494, 459–462. doi: 10.1038/nature11917
Makkonen, M., Berg, M. P., van Hal, J. R., Callaghan, T. V., Press, M. C., and Aerts, R. (2011). Traits explain the responses of a sub-arctic Collembola community to climate manipulation. Soil Biol. Biochem. 43, 377–384. doi: 10.1016/j.soilbio.2010.11.004
Margalef, R. (1968). Perspectives In Ecological Theory. Chicago, IL: The University of Chicago Press.
Martinez, N. G., Bettez, N. D., and Groffman, P. M. (2014). Sources of variation in home lawn soil nitrogen dynamics. J. Environ. Qual. 43, 2146–2151. doi: 10.2134/jeq2014.03.0103
McKinney, M. L. (2006). Urbanization as a major cause of biotic homogenization. Biol. Conserv. 127, 247–260. doi: 10.1016/j.biocon.2005.09.005
Mo, L., Peng, B., Wang, J., Huang, W., Chen, X., and Xu, G. (2018). Effects of nitrogen deposition on plants and soil in urban green space——a case study on Cynodon dactylon. Ecol. Environ. 27, 459–468.
Mo, L., Zanella, A., Chen, X., Peng, B., Lin, J., Su, J., et al. (2021). Effects of simulated nitrogen deposition on the bacterial community of urban green spaces. Appl. Sci. 11, 1–15. doi: 10.3390/app11030918
Ochoa-Hueso, R., Allen, E. B., Branquinho, C., Cruz, C., Dias, T., Fenn, M. E., et al. (2011). Nitrogen deposition effects on Mediterranean-type ecosystems: an ecological assessment. Environ. Pollut. 159, 2265–2279. doi: 10.1016/j.envpol.2010.12.019
Ochoa-Hueso, R., Rocha, I., Stevens, C. J., Manrique, E., and Luciañez, M. J. (2014). Simulated nitrogen deposition affects soil fauna from a semiarid Mediterranean ecosystem in central Spain. Biol. Fertil. Soils 50, 191–196. doi: 10.1007/s00374-013-0838-y
Payne, R. J., Thompson, A. M., Standen, V., Field, C. D., and Caporn, S. J. M. (2012). Impact of simulated nitrogen pollution on heathland microfauna, mesofauna and plants. Eur. J. Soil Biol. 49, 73–79. doi: 10.1016/j.ejsobi.2011.08.003
Pinho, P., Theobald, M. R., Dias, T., Tang, Y. S., Cruz, C., Martins-Loução, M. A., et al. (2012). Critical loads of nitrogen deposition and critical levels of atmospheric ammonia for semi-natural Mediterranean evergreen woodlands. Biogeosciences 9, 1205–1215. doi: 10.5194/bg-9-1205-2012
Ponge, J. F., and Chevalier, R. (2006). Humus Index as an indicator of forest stand and soil properties. For. Ecol. Manag. 233, 165–175. doi: 10.1016/j.foreco.2006.06.022
Providoli, I., Bugmann, H., Siegwolf, R., Buchmann, N., and Schleppi, P. (2006). Pathways and dynamics of 15NO3- and 15NH4+ applied in a mountain Picea abies forest and in a nearby meadow in central Switzerland. Soil Biol. Biochem. 38, 1645–1657. doi: 10.1016/j.soilbio.2005.11.019
Rawlins, B. G., Harris, J., Price, S., and Bartlett, M. (2015). A review of climate change impacts on urban soil functions with examples and policy insights from England, UK. Soil Use Manag. 31, 46–61. doi: 10.1111/sum.12079
Rousk, J., Bååth, E., Brookes, P. C., Lauber, C. L., Lozupone, C., Caporaso, J. G., et al. (2010). Soil bacterial and fungal communities across a pH gradient in an arable soil. ISME J. 4, 1340–1351. doi: 10.1038/ismej.2010.58
Santorufo, L., Cortet, J., Nahmani, J., Pernin, C., Salmon, S., Pernot, A., et al. (2015). Responses of functional and taxonomic collembolan community structure to site management in Mediterranean urban and surrounding areas. Eur. J. Soil Biol. 70, 46–57. doi: 10.1016/j.ejsobi.2015.07.003
Serna-Chavez, H. M., Fierer, N., and Van Bodegom, P. M. (2013). Global drivers and patterns of microbial abundance in soil. Glob. Ecol. Biogeogr. 22, 1162–1172. doi: 10.1111/geb.12070
Seto, K. C., Güneralp, B., and Hutyra, L. R. (2012). Global forecasts of urban expansion to 2030 and direct impacts on biodiversity and carbon pools. Proc. Natl. Acad. Sci. U.S.A. 109, 16083–16088. doi: 10.1073/pnas.1211658109
Shi, L., Zhang, H., Liu, T., Zhang, W., Shao, Y., Ha, D., et al. (2016). Consistent effects of canopy vs. understory nitrogen addition on the soil exchangeable cations and microbial community in two contrasting forests. Sci. Total Environ. 553, 349–357. doi: 10.1016/j.scitotenv.2016.02.100
Shifav, E. (2018). Review of heavy metals pollution in China in agricultural and urban soils. J. Health Pollut. 8:180607. doi: 10.5696/2156-9614-8.18.180607
Simkin, S. M., Allen, E. B., Bowman, W. D., Clark, C. M., Belnap, J., Brooksf, M. L., et al. (2016). Conditional vulnerability of plant diversity to Atmospheric nitrogen deposition across the United States. Proc. Natl. Acad. Sci. U.S.A. 113, 4086–4091. doi: 10.1073/pnas.1515241113
Song, M., Jing, S., Zhou, Y., Hui, Y., Zhu, L., Wang, F., et al. (2015). Dynamics of soil nematode communities in wheat fields under different nitrogen management in Northern China Plain. Eur. J. Soil Biol. 71, 13–20. doi: 10.1016/j.ejsobi.2015.09.002
Standish, R. J., Hobbs, R. J., and Miller, J. R. (2013). Improving city life: options for ecological restoration in urban landscapes and how these might influence interactions between people and nature. Landsc. Ecol. 28, 1213–1221. doi: 10.1007/s10980-012-9752-1
Stevens, C. J., Duprè, C., Dorland, E., Gaudnik, C., Gowing, D. J. G., Bleeker, A., et al. (2014). “Biodiversity of acid grasslands in the Atlantic regions of Europe: the impact of nitrogen deposition,” in Nitrogen Deposition, Critical Loads and Biodiversity, eds H. Sverdrup, K. E. Mason, L. J. Sheppard, M. A. Sutton, and R. Haeuber (Dordrecht: Springer), 243–250. doi: 10.1007/978-94-007-7939-6_26
Stevens, C. J., Manning, P., Van Den Berg, L. J. L., De Graaf, M. C. C., Wamelink, G. W. W., Boxman, A. W., et al. (2011). Ecosystem responses to reduced and oxidised nitrogen inputs in European terrestrial habitats. Environ. Pollut. 159, 665–676. doi: 10.1016/j.envpol.2010.12.008
Sun, X., Marian, F., Bluhm, C., Maraun, M., and Scheu, S. (2020). Response of Collembola to the addition of nutrients along an altitudinal gradient of tropical montane rainforests. Appl. Soil Ecol. 147:103382. doi: 10.1016/j.apsoil.2019.103382
Sutton, M. A., Mason, K. E., Sheppard, L. J., Sverdrup, H., Haeuber, R., and Hicks, W. K. (2014). Nitrogen Deposition, Critical Loads And Biodiversity. Dordrecht: Springer Science & Business Media.
Templer, P. H., Weathers, K. C., Lindsey, A., Lenoir, K., and Scott, L. (2015). Atmospheric inputs and nitrogen saturation status in and adjacent to Class I wilderness areas of the northeastern US. Oecologia 177, 5–15. doi: 10.1007/s00442-014-3121-5
Trammell, T. L. E., Pataki, D. E., Cavender-Bares, J., Groffman, P. M., Hall, S. J., Heffernan, J. B., et al. (2016). Plant nitrogen concentration and isotopic composition in residential lawns across seven US cities. Oecologia 181, 271–285. doi: 10.1007/s00442-016-3566-9
Tresch, S., Moretti, M., Le Bayon, R. C., Mäder, P., Zanetta, A., Frey, D., et al. (2018). A gardener’s influence on urban soil quality. Front. Environ. Sci. 6:25. doi: 10.3389/fenvs.2018.00025
Treseder, K. K. (2008). Nitrogen additions and microbial biomass: a meta-analysis of ecosystem studies. Ecol. Lett. 11, 1111–1120. doi: 10.1111/j.1461-0248.2008.01230.x
Tzoulas, K., Korpela, K., Venn, S., Yli-Pelkonen, V., Kaźmierczak, A., Niemela, J., et al. (2007). Promoting ecosystem and human health in urban areas using Green Infrastructure: a literature review. Landsc. Urban Plan 81, 167–178. doi: 10.1016/j.landurbplan.2007.02.001
van Grinsven, H. J. M., Ten Berge, H. F. M., Dalgaard, T., Fraters, B., Durand, P., Hart, A., et al. (2012). Management, regulation and environmental impacts of nitrogen fertilization in northwestern Europe under the Nitrates Directive; A benchmark study. Biogeosciences 9, 5143–5160. doi: 10.5194/bg-9-5143-2012
van Straalen, N. M., and Verhoef, H. A. (1997). The development of a bioindicator system for soil acidity based on arthropod pH preferences. J. Appl. Ecol. 34, 217–232. doi: 10.2307/2404860
Vitousek, P. M., Aber, J. D., Howarth, R. W., Likens, G. E., Matson, P. A., Schindler, D. W., et al. (1997). Human alteration of the global nitrogen cycle: sources and consequences. Ecol. Appl. 7, 737–750. doi: 10.2307/2269431
Vourlitis, G. L., and Fernandez, J. S. (2012). Changes in the soil, litter, and vegetation nitrogen and carbon concentrations of semiarid shrublands in response to chronic dry season nitrogen input. J. Arid. Environ. 82, 115–122. doi: 10.1016/j.jaridenv.2012.02.006
Wang, X., Zhao, J., Fu, S., Zhao, C., Wan, S., and Zhou, L. (2015). Soil nematode assemblages in an acid soil as affected by lime application. Nematology 17, 179–191. doi: 10.1163/15685411-00002860
Wardle, D. A., Bardgett, R. D., Klironomos, J. N., Setälä, H., Van Der Putten, W. H., and Wall, D. H. (2004). Ecological linkages between aboveground and belowground biota. Science 304, 1629–1633. doi: 10.1126/science.1094875
Wei, H., Liu, W., Zhang, J., and Qin, Z. (2017). Effects of simulated acid rain on soil fauna community composition and their ecological niches. Environ. Pollut. 220, 460–468. doi: 10.1016/j.envpol.2016.09.088
Xu, G. L., Mo, J. M., Fu, S. L., Per, G., Zhou, G. Y., and Xue, J. H. (2007). Response of soil fauna to simulated nitrogen deposition: a nursery experiment in subtropical China. J. Environ. Sci. 19, 603–609. doi: 10.1016/S1001-0742(07)60100-4
Xu, G. L., Mo, J. M., Zhou, G. Y., and Fu, S. L. (2006). Preliminary response of soil fauna to simulated n deposition in three typical subtropical forests. Pedosphere 16, 596–601. doi: 10.1016/s1002-0160(06)60093-3
Xu, G. L., Schleppi, P., Li, M. H., and Fu, S. L. (2009). Negative responses of Collembola in a forest soil (Alptal, Switzerland) under experimentally increased N deposition. Environ. Pollut. 157, 2030–2036. doi: 10.1016/j.envpol.2009.02.026
Yin, W. (1998). Pictorial Keys To Soil Animals Of China: Science Press Keys To Soil Animals Of China. Beijing: Science Press.
Keywords: N deposition, urban lawn, urban soil, soil fauna, soil biodiversity
Citation: Mo L, Xu G, Zhang J, Wu Z, Yu S, Chen X, Peng B, Squartini A and Zanella A (2021) Threshold Reaction of Soil Arthropods to Simulative Nitrogen Deposition in Urban Green Spaces. Front. Ecol. Evol. 9:711774. doi: 10.3389/fevo.2021.711774
Received: 19 May 2021; Accepted: 28 September 2021;
Published: 04 November 2021.
Edited by:
Franco Leandro Souza, Federal University of Mato Grosso do Sul, BrazilReviewed by:
Jérôme Cortet, Université Paul Valéry, Montpellier III, FranceRafael Dettogni Guariento, Federal University of Mato Grosso do Sul, Brazil
Copyright © 2021 Mo, Xu, Zhang, Wu, Yu, Chen, Peng, Squartini and Zanella. This is an open-access article distributed under the terms of the Creative Commons Attribution License (CC BY). The use, distribution or reproduction in other forums is permitted, provided the original author(s) and the copyright owner(s) are credited and that the original publication in this journal is cited, in accordance with accepted academic practice. No use, distribution or reproduction is permitted which does not comply with these terms.
*Correspondence: Guoliang Xu, xugl@gzhu.edu.cn; Jiaen Zhang, jeanzh@scau.edu.cn