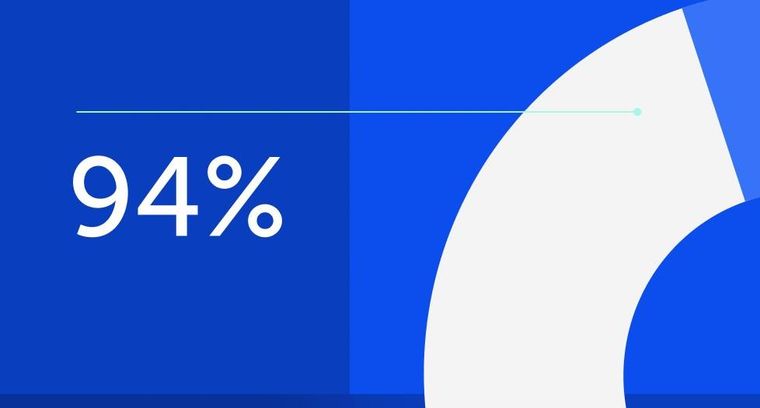
94% of researchers rate our articles as excellent or good
Learn more about the work of our research integrity team to safeguard the quality of each article we publish.
Find out more
ORIGINAL RESEARCH article
Front. Ecol. Evol., 06 October 2021
Sec. Coevolution
Volume 9 - 2021 | https://doi.org/10.3389/fevo.2021.709876
This article is part of the Research TopicAnthropogenic Stressors and Animal-Plant Interactions: Implications for Pollination and Seed DispersalView all 8 articles
Understanding the mechanisms by which non-native plants can attract pollinators in their new geographical zones is important because such species infiltrate native communities and can disrupt native ecological interactions. Despite the large number of studies assessing how invasive plants impact plant–pollinator interactions, the specific comparison of pollination interactions between native and non-native plant pairs has received much less attention. Here we focused on four coexisting co-flowering pairs of common native and non-native species, both with abundant flowers but different floral traits, and asked: (1) to what extent native and non-native plants share pollinator species, and whether the non-native plants attract a different set of pollinators, (2) whether the most shared pollinators are the most frequent floral visitors and the most generalized in their interactions, and (3) how much of the variation in the diversity and frequency of pollinator species between native and non-native plant species can be explained by floral trait dissimilarity and flower abundance. Direct pollinator observations revealed that the plant pairs shared a low fraction (0–33%) of insect species, i.e., non-native plants tended to acquire a different set of pollinators than their native counterparts. The most shared pollinators in each plant pair were the most common but not the most generalized species, and non-native species attracted both generalized and specialized pollinators. Corolla length at opening and flower abundance showed to be important in determining the differences in flower visitation rate between natives and non-natives. Our findings support the general pattern that non-native species have no barriers at the pollination stage to integrate into native communities and that they may attract a different assemblage of pollinators relative to those that visit native plants with which they coexist.
Among the different interactions that flowering non-native plants establish with other organisms in their new areas, those that involve their pollinators are expected to play an important role in plant reproduction and hence invasion success (Stout and Tiedeken, 2017). This is especially the case of most angiosperms that depend on, or benefit from, animal pollination to set fruits (Klein et al., 2007; Ollerton et al., 2011). Moreover, most of the top invasive plants in the world, at least the woody ones, are biotically pollinated (Traveset and Richardson, 2014). A recent review also shows that the large majority of non-native plants receive suitable pollination service from resident pollinators, whereas other species can minimize their reliance on pollinators by increasing their selfing levels (Montero-Castaño and Traveset, 2020).
An increasing body of literature has emerged in the last two decades assessing how invasive plants infiltrate native plant communities and impact plant–pollinator interactions in them (Aizen and Morales, 2020). Once in a new area, invasive plants attract pollinators that were previously monopolized by the natives (Thijs et al., 2012). Such pollinator “usurpation” can imply lower visitation rates to native flowers which can lead to decreases in seed set (Brown et al., 2002; Moragues and Traveset, 2005; Larson et al., 2006; Lopezaraiza-Mikel et al., 2007; Matsumoto et al., 2010; Thijs et al., 2012) and long-term changes in population size and species richness (SR) in the invaded habitats (Emer et al., 2015). By contrast, invasive plants can have a facilitative effect by enhancing pollinator abundance, diversity, and pollination success in native plants (Memmott and Waser, 2002; Feldman et al., 2004; Moragues and Traveset, 2005; Larson et al., 2006; Tepedino et al., 2008). Alternatively, neutral effects of invasive plants on native pollination interactions have been also documented (Aigner, 2004; Moragues and Traveset, 2005; Thijs et al., 2012). Different meta-analyses have indeed shown that the impact of plant invaders on native plant–pollinator interactions is context-dependent, varying among species, local populations, environmental conditions, study designs, and other local-scale effects (Morales and Traveset, 2009; Emer et al., 2015; Charlebois and Sargent, 2017). Despite the large number of studies, however, the comparison of pollination interactions between pairs of co-flowering common species that coexist in the same habitat with abundant flowers but with different floral traits has still received little attention (Brown et al., 2002; Morales and Traveset, 2009; Ha et al., 2020).
According to the Darwin’s naturalization hypothesis (DNH), non-native plants should find higher competition for the same resource in the presence of close relatives in the native flora (Darwin, 1859). Darwin’s idea was that closely related species are more likely to have similar ecological niches due to common ancestry, and therefore would be competing for the same resources. By contrast, “unrelated” species would be relatively free from competition, hence they could more easily enter the new environment. Community-level studies have shown that non-native plants easily integrate into native pollination webs by being visited by resident pollinators (Memmott and Waser, 2002; Traveset et al., 2015; Valdovinos, 2019). This could be expected as non-native species use a wide range of pollinator taxa including generalized species of different functional groups, such as eusocial and solitary bees, flies, other Diptera and Coleoptera (Wilson et al., 2004; Matsuyama et al., 2009; Gómez et al., 2015), but also specialist species (i.e., visit one or very few plant species; Stouffer et al., 2014).
Whether one group of pollinators will be more likely to integrate a particular plant invader than another will depend on different pollinator and plant traits (Parra-Tabla et al., 2019; Montero-Castaño and Traveset, 2020). In the case of plants, flower abundance, shape, color, symmetry, and corolla size (width and length) are among the most influential in the flowering plant–pollinator interactions (Gibson et al., 2012; Junker et al., 2013), acting as “cues” used by flower visitors to discriminate between the different reward sources available (Reverté et al., 2016). In a review study, Morales and Traveset (2009) found that the effect of non-native species on pollination visitation of native plants was more likely to be negative if the native and non-native plants have similar flower symmetry or color, whereas the effect was not significant when species differed in such traits. Co-flowering plant species with dissimilar floral traits have been found to usually attract different pollinator assemblages and thus are not likely to reciprocally facilitate pollination (Reverté et al., 2016; Albor et al., 2020; Ha et al., 2020). Other studies, however, have shown either floral trait similarity to be associated with pollination facilitation (Bergamo et al., 2020) or that pollinator sharing was not related with the floral similarity between native and non-native species (Muñoz and Cavieres, 2019). Therefore, a consensus regarding the role of floral traits on pollinator interactions between native and non-native plant species remains elusive.
In this study, we focused on four different coexisting co-flowering pairs of one native and one non-native species that have morphologically distinct flowers. The two species of each pair are unrelated and very common in the study sites and their flowers are the most abundant in the community. First, we aimed to determine to what extent the coexisting pairs share pollinators, and if specific floral traits (e.g., flower abundance, corolla shape, symmetry, and size) can explain the difference in diversity and frequency of the pollinators. Secondly, we wanted to assess which are the most frequently shared pollinator species and functional groups among the different pairs. Our specific hypotheses were:
1. Non-native species attract a new assemblage of pollinators, including pollinators of different functional groups, and share only a small proportion of pollinator species with their native counterparts, in accordance with the DNH.
2. The shared pollinators by each plant pair are the most frequent and/or the most generalized species.
3. Differences in pollinator SR, the number of shared pollinator species and visitation rates between native and non-native plant species can be explained by floral trait dissimilarity and flower abundance.
The study was carried out at three sites on Mallorca (Balearic Islands, Western Mediterranean Sea), two at the south of the island (Torrent de Sa Riera: 39°35′12.6″N, 2°38′17.6″E; Platja de Ca’n Pere Antoni: 39°33′45.7″N, 2°39′43.5″E), and the other at the western part (Torrent d’Esporles: 39°39′56.5″N, 2°34′46.8″E). Torrent de Sa Riera is an intermittent stream predominately occupied by the native Withania somnifera (L.) Dunal and non-native species such as Nicotiana glauca Graham and Physalis peruviana L. Ca’n Pere Antoni is a sandy beach mostly dominated by species dispersed along its shore such as the native Glaucium flavum Crantz and the non-native Mesembryanthemum crystallinum L. Lastly, Torrent d’Esporles is also a temporary stream with abundant species such as the annual native Convolvulus arvensis L. and Cyperus involucratus Rottb and the non-natives Tropaeolum majus L. and Arundo donax L.
At each study site, the selected co-flowering plant pair, of one native and one non-native species, was based on the species spatial co-occurrence and high flower abundance (Figure 1 and Table 1). Because pollinator activity and flowering characteristics (e.g., flower opening and receptivity) usually fluctuate during daytime and nighttime, we ensured that the chosen flowers of both species within a pair were open and receptive simultaneously, particularly during the direct observations.
Figure 1. Images of the flowers of all species pairs studied on Mallorca (pictures on the left represent native species whereas their corresponding partners are shown at right). (1) Convolvulus arvensis L. (Convolvulaceae) and (2) Tropaeolum majus L. (Tropaeolaceae) in Torrent d’Esporles are pair 1; (3) Glaucium flavum Crantz (Papaveraceae) and (4) Mesembryanthemum crystallinum L. (Aizoaceae) in Ca’n Pere Antoni are pair 2; (5) Hyoscyamus albus L. and (6) Nicotiana glauca Graham; and (7) Withania somnifera L. and (8) Physalis peruviana L. (Solanaceae) in Torrent de Sa Riera are pair 3 and 4, respectively.
Table 1. Qualitative and quantitative (mean and SD) values of floral traits and number of open flowers of each studied plant species.
Convolvulus arvensis (Convolvulaceae), native to the Mediterranean Basin, is a climbing perennial plant with solitary flowers on long peduncles. It grows in a wide range of conditions from full sun to full shade, on all soils, particularly on warm, dry soils, and is drought tolerant (Culhavi and Manea, 2011). Its flowering stalk occasionally branches and can produce 1–3 flowers. T. majus (Tropaeolaceae), native to the Andes Mountains in South America, is a fast growing climbing annual plant. It has single flowers and can form extensive carpet-like growths in sunny torrent banks and beds (Garzón and Wrolstad, 2009).
Glaucium flavum (Papaveraceae) is a perennial herb, native to the Mediterranean Basin that occurs along its shores and the coasts of Western Europe. Its large flowers have a central tuft of orange stamens and four silky long petals that drop as the long-curved seedpods develop (Thanos et al., 1989). Native to South Africa, M. crystallinum (Aizoaceae) is an annual or biennial succulent herb with trailing and branched stem. Its flowers are in cymes, with petals longer than the calyx.
Hyoscyamus albus (Solanaceae) is an herb, primary found in coastal areas in southern Europe and in the Near East, that grows to a height of about 40 or 50 cm. When blooming, it develops a long inflorescence with all flowers attached to the stem. N. glauca (Solanaceae), native to northwestern Argentina and Bolivia, is an evergreen perennial soft-wooded shrub or small tree up to 6 m in height with many branches. It produces flowers that are found in branched clusters (Ollerton et al., 2012).
Withania somnifera (Solanaceae) is a perennial shrub native to a wide range, spreading from the Mediterranean region through tropical regions of Africa to South Africa and also from the Macaronesian region to the Arabia and Middle East region (Gaurav et al., 2015). Flowers emerge in compact groups from the leaf axils in the shape of a greenish bell. P. peruviana (Solanaceae) is an herbaceous, semi-shrub, upright, and perennial plant from the subtropical zones of the Andes (South America). It is usually 0.6–0.9 m tall, although in some cases it can grow up to 1.8 m. Its hermaphrodite flowers are formed singly in the axils of the leaves on pedicels 1–2 cm long (Cedeño and Montenegro, 2004).
All eight species flower between April and September and are insect-pollinated, although C. arvensis (pair 1) (Prokop and Neupauerova, 2014), H. albus (Fettig and Hufbauer, 2017) and N. glauca (pair 3) (Issaly et al., 2020), and W. somnifera (pair 4) (Kaul et al., 2005) can also be self-pollinated. For the eight plant species, seven floral morphology traits were measured on three fresh flowers from five individuals by the same observer (M. Abdallah). Four morphological traits were measured with a digital caliper: corolla length and diameter at opening, corolla total length and corolla bell diameter at nectary level. Following codes by Castroviejo (1986), floral shape was classified as: (1) tubular, (2) funnel, and (3) bell, whereas floral symmetry was either (1) radial or (2) bilateral (Table 1).
Direct observations of insect flower visitation were done in 2018, from April 6th to June 25th. Each plant species was censused on an average of 10 h in total, during an average of 4 days, between 10:00 am to 8:00 pm. Between 10 and 30 individuals per species were observed during 25 min periods from a 1 m distance. In each census, we recorded the insect species (or morphospecies) visiting the flowers, the number of legitimate visits (i.e., times that the visitor entered the corolla and contacted the reproductive organs), the number of flowers contacted by each visitor, the number of flowers observed during the census, and the total number of open flowers in the individual plant as a proxy of flower abundance. All insects performing legitimate visits were considered as pollinators regardless of their effectiveness. After finishing each census, flowers were closely inspected to confirm the insect species or to collect them for further identification. Identifications were performed at the Mediterranean Institute for Advanced Studies (IMEDEA), using the pollinator reference collection available at this institution. All pollinators were grouped into the following functional groups: Coleoptera, Diptera, Formicidae, Hemiptera, Hymenoptera, and Lepidoptera.
For each plant species and census day, we estimated pollinator species richness (SR) as the total number of species visiting the flowers, insect visitation rate (IVR) as the number of legitimate visits to the plant by any insect, regardless the number of flowers contacted, and flower visitation rate (FVR) as the number of flowers contacted by each insect species, standardized by the number of flowers observed in each census and by total flower abundance of the plant species.
The observed pollinator species were categorized as either specialized or generalized based on the number of flowering plant species they visited according to published information and on our own data from Mallorcan pollinators. To do this, we searched for records on flower visitation from previous pollination studies in Mallorca island and by using the search engine Google Scholar with the following keywords: (“pollinator species” OR “pollinator genus”) AND (“pollinat∗” OR “flower visit∗” OR “nectarivor∗”). Arbitrarily, we considered a pollinator as specialized if it visited less than five plant species (even if these did not belong to the same family) and as a generalized if it visited at least five different plant species from different families.
For each pair, we obtained the number of shared and non-shared pollinator species with its native counterpart. Contingency tables were used to compare the number of functional groups between natives and non-native plants with the function “chisq.test” from the R package “car.” To test whether the pollinators shared between native and non-natives within each pair are the most frequent and/or the most generalized species, a generalized linear model (GzLMs) with binomial distribution was performed using shared/non-shared species as response variable, and IVR, and generalized/specialized species as independent variables. Finally, a set of generalized linear mixed models (GLMMs) were built for each of the response variable (SR, IVR, and FVR) to test if variation in SR (Poisson distribution) and visitation rate (either IVR or FVR; Gamma distribution) can be explained by differences in floral traits, flower abundance and origin status of the species (native vs. non-native). For this, we included shape, symmetry, corolla size, flower abundance and status, and their interactions, as independent variables, and “pair” as a random variable, using the “glmer” function from the package “lme4” in R version 3.6.3. Because the study species differ greatly in color regardless of their status (Figure 1), and because floral spectral readings by insects were not available, we decided not to include this variable in the statistical analyses.
A total of 50 insect species were observed visiting the flowers of the eight flowering study plants. Nearly one third of them (32%; n = 16 spp.) were shared between native and non-native plants, whereas 40% visited only native species and 28% visited only the non-native ones. No differences were found in the number of functional groups (X2 = 6.34, df = 8, p = 0.609) visiting native vs. non-native species.
Only five (16%) of all insect species were shared by the two species of this plant pair. The non-native T. majus showed the highest values in SR, IVR, and FVR (Figure 2). Hymenoptera was the most important pollinator functional group for both species; namely Lasioglossum malachurum and Apis mellifera for C. arvensis and Polistes dominula and A. mellifera for T. majus.
Figure 2. Mean and SD of insect species richness SR (A), insect visitation rate IVR (B) and flower visitation rate FVR (C,D) to native (in gray) and non-native (in red) plant species studied. SR is the number of insect species visiting the flowers, IVR is the number of legitimate visits regardless the number of flowers contacted, and FVR is the number of flowers contacted by each insect species, standardized by the number of flowers observed in each census and by total flower abundance of the plant species.
This pair shared only 12% of the insect species. The native G. flavum showed the highest SR, IVR, and FVR (Figure 2). Sarcophaga carnaria (Diptera) was the most important visitor of the native plant (in both IVR and FVR), followed by one Coleoptera (Oedemera simplex) and one dipteran species (Ensina sonchi). The non-native M. crystallinum was mostly visited by two hymenopterans Lasioglossum punctatissimum and Nomioides squamigera and one coleopteran Tentyria schaumi.
The flowers of H. albus and N. glauca were visited by a different set of insect species, i.e., they did not share any visitors. The non-native N. glauca recorded the greatest values in SR, IVR, and FVR (Figure 2). The most frequent visitor of H. albus was Sphaerophoria sp. (Diptera), followed by a wasp (Braconidae sp.), whereas N. glauca was mainly visited by an alien ant (Crematogaster scutellaris) and the fly E. sonchi.
This pair shared six (33%) of the insect species visiting their flowers. The native plant W. somnifera recorded the maximum values of SR, IVR, and FVR (Figure 2). Spilostethus pandurus (Hemiptera) was the most important visitor for both plant species, followed by P. dominula (Hymenoptera) and Oxycarenus lavaterae (Hemiptera) for W. somnifera and P. peruviana, respectively.
Results of the GzLM showed that the shared pollinator species between natives and non-natives species were also the most frequent floral visitors (β = 25.78, z = 2.20, p = 0.028), but not the most generalized species (β = 0.50, z = 0.66, p = 0.505).
Variation in FVR can be explained by corolla length dissimilarity, flower abundance as well as status (native and non-native). Overall, non-native species, usually with larger corolla length at opening and more flowers, showed higher FVR than natives (β = 0.20, z = 0.03, p < 0.001; β = 0.02, z = 5.46, p < 0.001, respectively). SR and IVR significantly increased with corolla length at opening (β = 0.05, z = 2.76, p < 0.006; β = 0.06, z = 2.23, p = 0.026, respectively) but it was independent of plant species status (see model selection summary in Supplementary Table 1).
Although previous studies have shown small-scale effects of plant invaders on neighboring natives (Gibson et al., 2012; Goodell and Parker, 2017; Ramsey et al., 2019), we found that, overall, the shared proportion of pollinators between non-native species and their co-existing native counterparts fluctuated from 0 to 33%, suggesting little “interference” with the pollination success of the latter. This result is in accordance with the Darwin’s Naturalization Hypothesis, suggesting that “unrelated” flowering non-native plants are more likely to coexist with native ones if they do not compete for resources (i.e., if they share few pollinators). It is important to note that even when comparing pairs of species belonging to the same family (in our case, H. albus vs. N. glauca and W. somnifera vs. P. peruviana, all in the Solanaceae), the number of shared pollinator species is very low (0% in the first pair vs. 33%% in the latter). This is probably because of the wide variation found in flower traits and display in this family (Knapp et al., 2004; Knapp, 2010; Sarkinen et al., 2013). Species in the two pairs indeed differ much in flower traits, as seen in Table 1.
Our findings are also congruent with those of previous studies that showed that non-native plants can acquire a completely different set of pollinators, encompassing even different orders, than their native counterparts (Ollerton et al., 2012; Ackerman et al., 2014; Maruyama et al., 2016). One possible explanation of the different ways non-native species integrate into resident-pollinators networks is that the non-native species might find their specific pollinators or pollinators functional groups, due to the pollinator’s wide distribution ranges, or because these might have been introduced in the same area as the non-native plant species (Rodger et al., 2010). Indeed, a study conducted by Rodger et al. (2010) showed that the main pollinator of Lilium formosanum is the moth Agrius convolvuli, whose wide distribution area covers both the native (Taiwan) and the invaded (South Africa) distribution areas of this lily. In our study, it might also be possible that the “new assemblage” of pollinators do prefer the non-natives. To test this, however, data on the pollinator interactions of native species in sites without the non-native species would be needed.
Overall, we found that the most shared pollinators in each plant pair are the most common but not necessarily the most generalized species. However, the non-native plants did not only interact with generalized but also with specialized species, which might result from the asymmetrical nature of plant–pollinator webs (Aizen and Morales, 2020). The fact that generalized pollinators often introduce non-native plants into pollination networks might simply be a result of their abundance and wide diets and not a preferential linking (Montero-Castaño and Traveset, 2020). However, by analyzing 25 invaded pollination networks, Stouffer et al. (2014) found that specialized pollinators are far more likely to integrate with non-native plants than would be expected by chance. Further studies on the role of specialized resident pollinators integrating non-native species are necessary, both from an ecological and evolutionary viewpoint.
Among the assessed floral traits, we discovered that corolla length at opening was important in explaining variation in the diversity and frequency of pollinators. Our findings revealed that non-native species with longer corollas at opening had higher FVR than native species. It is well established that the primary function of corollas is to attract pollinators and corolla size can influence such attraction (Ida and Totland, 2014; Gao et al., 2019). However, compared to the considerable attention that has been paid to specific floral traits such as floral color and corolla shape in explaining the differences in pollination attraction between native and non-native species, research on the effect of corolla size is strikingly scarce (Gómez et al., 2016; Gao et al., 2019). Further studies are thus needed to assess whether there is a general pattern in the effect of corolla size on pollinator attraction when comparing native and non-native species. It is also important to note that evaluating other traits (e.g., scent, nectar rewards, volatiles from different parts of the plant) at multiple stages of the pollination process, and across a larger number of species, is necessary to unveil the different mechanisms by which non-native plants can attract pollinators in their new geographical zones (Parra-Tabla and Arceo- Gómez, 2021).
Our non-native plants also produced more flowers and this resulted in higher insect visitation rates. Such finding is consistent with previous studies showing that non-native plants with more flowers/inflorescences tend to attract a wider array of pollinators and receive higher FVRs (Lopezaraiza-Mikel et al., 2007; Padrón et al., 2009; Kaiser-Bunbury et al., 2011). However, non-native plants might be more attractive to pollinators than natives not only for their flower display but also for their rewards. Indeed, some non-native plants can allocate more resources to both floral display and rewards (Bjerknes et al., 2007) as they are superior competitors for abiotic resources or are released from enemies in the invaded regions (Agrawal and Kotanen, 2003). Besides attracting legitimate pollinators, however, flower abundance also enhances the visits by animals that interact negatively with the pollination process, such as nectar robbers (Irwin et al., 2010). This is actually what we found with T. majus. Nectar robbers are important members of the community of floral visitors and have both neutral and negative effects on the visitation rates of pollinators (Irwin et al., 2010; Lobo et al., 2016).
The impact of plant invaders on native interactions varies among species and is influenced by different ecological attributes of plant species. In our study system, the non-native species attract an assemblage of pollinators which are by and large not shared with their native counterparts. This, however, does not preclude that those that are shared interfere with the pollination success of the natives, for instance by usurping floral rewards to natives and reducing fruit/seed set (not measured in this study) or by lowering the quality of pollination (through, e.g., allelopathy, stigma clogging, or hybridization) (Morales and Traveset, 2009). This highlights the importance of performing long-term studies mainly at the community level to understand under what circumstances non-native species are more likely to be successful and even outcompete native species, using both observational and experimental studies that evaluate pollination success.
Derived data supporting the findings of this study are available from the corresponding author (MA) on request.
MA and AT conceived the study. MA collected the data, identified the insects, prepared the figures, and led the writing with significant contributions from all authors. MA and SH-P prepared the datasets and performed the statistical analyses.
This study was framed within project GLC2017-88122-P financed to AT by the Spanish Ministry of Science, Innovation, and Universities.
The authors declare that the research was conducted in the absence of any commercial or financial relationships that could be construed as a potential conflict of interest.
All claims expressed in this article are solely those of the authors and do not necessarily represent those of their affiliated organizations, or those of the publisher, the editors and the reviewers. Any product that may be evaluated in this article, or claim that may be made by its manufacturer, is not guaranteed or endorsed by the publisher.
We are grateful to Miguel Ángel González, Anna Díaz Lorca, and Xavier Canyelles Ferrà for their help with insect identification.
The Supplementary Material for this article can be found online at: https://www.frontiersin.org/articles/10.3389/fevo.2021.709876/full#supplementary-material
Ackerman, J. D., Falcón, W., Molinari, J., Espino, I., and Cuevas, A. A. (2014). Biotic resistance and invasional meltdown: consequences of acquired interspecific interactions for an invasive orchid, Spathoglottis plicata in Puerto Rico. Biol. Invasions 16, 2435–2447. doi: 10.1007/s10530-014-0676-3
Agrawal, A. A., and Kotanen, P. M. (2003). Herbivores and the success of exotic plants: a phylogenetically controlled experiment. Ecol. Lett. 6, 712–715. doi: 10.1046/j.1461-0248.2003.00498.x
Aigner, P. A. (2004). Ecological and genetic effects on demographic processes: pollination, clonality and seed production in Dithyrea maritima. Biol. Conserv. 116, 27–34. doi: 10.1016/S0006-3207(03)00170-8
Aizen, M. A., and Morales, C. L. (2020). “Impacts of alien plants on plant-pollinator interactions,” in Plant Invasions: The Role of Biotic Interactions, eds A. Traveset and D. M. Richardson (Wallingford: CABI Invasives Series), 241–255.
Albor, C., Arceo-Gómez, G., and Parra-Tabla, V. (2020). Integrating floral trait and flowering time distribution patterns help reveal a more dynamic nature of co-flowering community assembly processes. J. Ecol. 108, 2221–2231. doi: 10.1111/1365-2745.13486
Bergamo, P. J., Streher, N. S., Wolowski, M., and Sazima, M. (2020). Pollinator-mediated facilitation is associated to floral abundance, trait similarity and enhanced community-level fitness. J. Ecol. 108, 1334–1346. doi: 10.1111/1365-2745.13348
Bjerknes, A. L., Totland, Ø, Hegland, S. J., and Nielsen, A. (2007). Do alien plant invasions really affect pollination success in native plant species? Biol. Conserv. 138, 1–12. doi: 10.1016/j.biocon.2007.04.015
Brown, B. J., Mitchell, R. J., and Graham, S. A. (2002). Competition for pollination between an invasive species (purple loosestrife) and a native congener. Ecology 83, 2328–2336.
Castroviejo, S. (1986). Flora ibérica: Plantas Vasculares de la Península Ibérica e Islas Baleares. Madrid: CSIC-CSIC Press.
Cedeño, M., and Montenegro, D. (2004). Plan Exportador, Logístico y Comercialización de Uchuva al Mercado de Estados Unidos para FRUTEXPO SCI Ltda. Ph.D. thesis. Colombia: Pontificia Universidad Javeriana.
Charlebois, J. A., and Sargent, R. D. (2017). No consistent pollinator-mediated impacts of alien plants on natives. Ecol. Lett. 20, 1479–1490. doi: 10.1111/ele.12831
Culhavi, C. D., and Manea, D. (2011). Controlling Convolvulus arvensis L. in grain maize and winter wheat in Banat (Romania). Res. J. Agric. Sci. 43, 21–27.
Emer, C., Vaughan, I. P., Hiscock, S., and Memmott, J. (2015). The impact of the invasive alien plant, Impatiens glandulifera, on pollen transfer networks. PLoS One 10:e0143532. doi: 10.1371/journal.pone.0143532
Feldman, T. S., Morris, W. F., and Wilson, W. G. (2004). When can two plant species facilitate each other’s pollination? Oikos 105, 197–207. doi: 10.1111/j.0030-1299.2004.12845.x
Fettig, C., and Hufbauer, R. (2017). Reproductive strategy, performance, and population dynamics of the introduced weed black henbane (Hyoscyamus niger). Weed Sci. 6, 83–96. doi: 10.1614/WS-D-16-00034.1
Gao, Y., Wang, Ch, Song, B., and Du, F. (2019). Corolla retention after pollination facilitates the development of fertilized ovules in Fritillaria delavayi (Liliaceae). Sci. Rep. 9:729. doi: 10.1038/s41598-018-37358-0
Garzón, G. A., and Wrolstad, R. E. (2009). Major anthocyanins and antioxidant activity of Nasturtium flowers (Tropaeolum majus). Food Chem. 114, 44–49. doi: 10.1016/j.foodchem.2008.09.013
Gaurav, N., Kumar, A., Tyagi, M., Kumar, D., Chauhan, U. K., and Singh, A. P. (2015). Morphology of Withania Somnifera (Distribution, morphology, phytosociology of Withania somnifera L. Dunal). Int. J. Curr. Res. 1, 164–173.
Gibson, M. R., Richardson, D. M., and Pauw, A. (2012). Can floral traits predict an invasive plant’s impact on native plant–pollinator communities? J. Ecol. 100, 1216–1223. doi: 10.2307/23257543
Gómez, J. M., Perfectti, F., and Abdelaziz, M. (2015). Evolution of pollination niches in a generalist plant clade. New Phytol. 205, 440–453. doi: 10.1111/nph.13016
Gómez, J. M., Torices, R., Lorite, J., Klingenberg, C. P., and Perfectti, F. (2016). The role of pollinators in the evolution of corolla shape variation, disparity and integration in a highly diversified plant family with a conserved floral bauplan. Ann. Bot. 117, 889–904. doi: 10.1093/aob/mcv194
Goodell, K., and Parker, I. M. (2017). Invasion of a dominant floral resource: effects on the floral community and pollination of native plants. Ecology 98, 57–69. doi: 10.1002/ecy.1639
Ha, M. K., Schneider, S. A., and Adler, L. S. (2020). Facilitative pollinator sharing decreases with floral similarity in multiple systems. Oecologia 195, 273–286. doi: 10.1007/s00442-020-04770-1
Ida, T. Y., and Totland, O. (2014). Heating effect by perianth retention on developing achenes and implications for seed production in the alpine herb Ranunculus glacialis. Alp. Bot. 124, 37–47. doi: 10.1007/s00035-014-0129-8
Irwin, R. E., Bronstein, J. L., and Manson, J. S. (2010). Nectar robbing: ecological and evolutionary perspectives. Annu. Rev. Ecol. Evol. Syst. 41, 271–292. doi: 10.1146/annurev.ecolsys.110308.120330
Issaly, E. A., Sérsic, A. N., Pauw, A., Cocucci, A. A., Traveset, A., Benítez-Vieyra, S. M., et al. (2020). Reproductive ecology of the bird-pollinated Nicotiana glauca across native and introduced ranges with contrasting pollination environments. Biol. Invasions 22, 485–498. doi: 10.1007/s10530-019-02104-8
Junker, R. R., Blüthgen, N., Brehm, T., Binkenstein, J., Paulus, J., Schaefer, H. M., et al. (2013). Specialization on traits as basis for the niche-breadth of flower visitors and as structuring mechanism of ecological networks. Funct. Ecol. 27, 329–341. doi: 10.2307/23480830
Kaiser-Bunbury, C. N., Valentin, T., Mougal, J., Matatiken, D., and Ghazoul, J. (2011). The tolerance of island plant–pollinator networks to alien plants. J. Ecol. 99, 202–213. doi: 10.1111/j.1365-2745.2010.01732.x
Kaul, M. K., Kumar, A., and Sharma, A. (2005). Reproductive biology of Withania somnifera (L.) Dunal. Curr. Sci. 88, 1375–1377.
Klein, A. M., Vaissière, B. E., Cane, J. H., Steffan-Dewenter, I., Cunningham, S. A., Kremen, C., et al. (2007). Importance of pollinators in changing landscapes for world crops. Proc. R. Soc. B 274, 303–313. doi: 10.1098/rspb.2006.3721
Knapp, S. (2010). On ‘various contrivances’: pollination, phylogeny and flower form in the Solanaceae. Philos. Trans. R. Soc. Lond. B Biol. Sci. 365, 449–460. doi: 10.1098/rstb.2009.0236
Knapp, S., Bohs, L., Nee, M., and Spooner, D. M. (2004). Solanaceae—a model for linking genomics with biodiversity. Comp. Funct. Genom. 5, 285–291. doi: 10.1002/cfg.393
Larson, D. L., Royer, R. A., and Royer, M. R. (2006). Insect visitation and pollen deposition in an invaded prairie plant community. Biol. Conserv. 130, 148–159. doi: 10.1016/j.biocon.2005.12.009
Lobo, J. A., Ramos, D., and Barga, A. C. (2016). Visitation rate of pollinators and nectar robbers to the flowers and inflorescences of Tabebuia aurea (Bignoniaceae): effects of floral display size and habitat fragmentation. Bot. J. Linn. Soc. 181, 667–681. doi: 10.1111/boj.12435
Lopezaraiza-Mikel, M. E., Hayes, R. B., Whalley, M. R., and Memmott, J. (2007). The impact of an alien plant on a native plant–pollinator network: an experimental approach. Ecol. Lett. 10, 539–550. doi: 10.1111/j.1461-0248.2007.01055.x
Maruyama, P. K., Vizentin-Bugoni, J., Sonne, J., Martín González, A. M., Schleuning, M., Araujo, A. C., et al. (2016). The integration of alien plants in mutualistic plant-hummingbird networks across the Americas: the importance of species traits and insularity. Divers. Distrib. 22, 672–681. doi: 10.1111/ddi.12434
Matsumoto, T., Takakura, K. I., and Nishida, T. (2010). Alien pollen grains interfere with the reproductive success of native congener. Biol. Invasions 12, 1617–1626. doi: 10.1007/s10530-009-9574-5
Matsuyama, S., Osawa, N., and Sakimoto, M. (2009). Generalist pollinators in the dioecious shrub Rhus trichocarpa Miq. (Anacardiaceae) and their role in reproductive success. Plant Species Biol. 24, 215–224. doi: 10.1111/j.1442-1984.2009.00258.x
Memmott, J., and Waser, N. M. (2002). Integration of alien plants into a native flower–pollinator visitation web. Proc. R. Soc. B 269, 2395–2399. doi: 10.1098/rspb.2002.2174
Montero-Castaño, A., and Traveset, A. (2020). “Pollination interactions promoting plant invasions,” in Plant Invasions: The Role of Biotic Interactions, eds A. Traveset and D. M. Richardson (Wallingford: CABI Invasives Series), 67–89.
Moragues, E., and Traveset, A. (2005). Effect of Carpobrotus spp. on the pollination success of native plant species of the Balearic Islands. Biol. Conserv. 122, 611–619. doi: 10.1016/j.biocon.2004.09.015
Morales, C. L., and Traveset, A. (2009). A meta-analysis of impacts of alien vs native plants on pollinator visitation and reproductive success of co-flowering native plants. Ecol. Lett. 12, 716–728. doi: 10.1111/j.1461-0248.2009.01319.x
Muñoz, A. A., and Cavieres, L. A. (2019). Sharing of pollinators between the invasive Taraxacum officinale and co-flowering natives is not related to floral similarity in the high-Andes. Flora 261:151491. doi: 10.1016/j.flora.2019.151491
Ollerton, J., Watts, S., Connerty, S., Lock, J., Parker, L., Wilson, I., et al. (2012). Pollination ecology of the invasive tree tobacco Nicotiana glauca: comparisons across native and non-native ranges. J. Pollinat. Ecol. 9, 85–95. doi: 10.26786/1920-7603201212
Ollerton, J., Winfree, R., and Tarrant, S. P. (2011). How many flowering plants are pollinated by animals? Oikos 120, 32–326. doi: 10.1111/j.1600-0706.2010.18644.x
Padrón, B., Traveset, A., Bidenweg, T., Diaz, D., Nogales, M., and Olsen, J. M. (2009). Impact of alien plant invaders on pollination networks in two archipelagos. PLoS One 4:e6275. doi: 10.1371/journal.pone.0006275
Parra-Tabla, V., Angulo-Pérez, D., Albor, C., Campos-Navarette, M. J., Tun-Garrido, J., Sosenski, P., et al. (2019). The role of alien species on plant-floral visitor network structure in invaded communities. PLoS One 14:e0218227. doi: 10.1371/journal.pone.0218227
Parra-Tabla, V., and Arceo- Gómez, G. (2021). Impacts of plant invasions in native plant–pollinator networks. New Phytol. 230, 2117–2128. doi: 10.1111/nph.17339
Prokop, P., and Neupauerova, D. (2014). Flower closure in the field bindweed (Convolvulus arvensis): a field test of the pollination hypothesis. Tur. J. Bot. 38, 877–882. doi: 10.3906/bot-1310-57
Ramsey, A. J., Ballou, S. M., and Mandel, J. R. (2019). Altered pollination ecology of a native plant species in the presence of an attractive non-native plant species. Rhodora 121, 159–173. doi: 10.3119/18-11
Reverté, S., Retana, J., Gómez, J. M., and Bosch, J. (2016). Pollinators show flower colour preferences but flowers with similar colours do not attract similar pollinators. Ann. Bot. 118, 249–257. doi: 10.1093/aob/mcw103
Rodger, J. G., Van Kleunen, M., and Johnson, S. D. (2010). Does specialized pollination impede plant invasions? Int. J. Plant Sci. 171, 382–391. doi: 10.1086/651226
Sarkinen, T., Bohs, L., Olmstead, R. G., and Knapp, S. (2013). A phylogenetic framework for evolutionary study of the nightshades (Solanaceae): a dated 1000-tip tree. BMC Evol. Biol. 13:214. doi: 10.1186/1471-2148-13-214
Stouffer, D. B., Cirtwill, A. R., and Bascompte, J. (2014). How exotic plants integrate into pollination networks. J. Ecol. 102, 1442–1450. doi: 10.1111/1365-2745.12310
Stout, J. C., and Tiedeken, E. J. (2017). Direct interactions between invasive plants and native pollinators: evidence, impacts and approaches. Funct. Ecol. 31, 38–46.
Tepedino, V. J., Bradley, B. A., and Griswold, T. L. (2008). Might flowers of invasive plants increase native bee carrying capacity? Intimations from Capitol Reef National Park, Utah. Nat. Areas J. 28, 44–50.
Thanos, C. A., Georghiou, K., and Skarou, F. (1989). Glaucium flavum seed germination - an ecophysiological approach. Ann. Bot. 63, 121–130. doi: 10.1093/oxfordjournals.aob.a087714
Thijs, K., Brys, R., Verboven, H., and Hermy, M. (2012). The influence of an invasive plant species on the pollination success and reproductive output of three riparian plant species. Biol. Invasions 14, 355–365. doi: 10.1007/s10530-011-0067-y
Traveset, A., Chamorro, S., Olsen, J. M., and Heleno, R. (2015). Space, Time, and Aliens: charting the dynamic structure of Galápagos pollination networks. AoB PLANTS 7:plv068. doi: 10.1093/aobpla/plv068
Traveset, A., and Richardson, D. M. (2014). Mutualistic interactions and biological invasions. Annu. Rev. Ecol. Evol. Syst. 45, 89–113. doi: 10.1146/annurev-ecolsys-120213-091857
Valdovinos, F. S. (2019). Mutualistic networks: moving closer to a predictive theory. Ecol. Lett. 22, 1517–1534. doi: 10.1111/ele.13279
Keywords: Balearic Islands, Darwin’s naturalization hypothesis, plant invasions, plant–pollination interactions, floral traits, generalized pollinators, species richness, visitation rate
Citation: Abdallah M, Hervías-Parejo S and Traveset A (2021) Low Pollinator Sharing Between Coexisting Native and Non-native Plant Pairs: The Effect of Corolla Length and Flower Abundance. Front. Ecol. Evol. 9:709876. doi: 10.3389/fevo.2021.709876
Received: 14 May 2021; Accepted: 13 September 2021;
Published: 06 October 2021.
Edited by:
Eliana Cazetta, Universidade Estadual de Santa Cruz, BrazilReviewed by:
Jair E. Garcia, RMIT University, AustraliaCopyright © 2021 Abdallah, Hervías-Parejo and Traveset. This is an open-access article distributed under the terms of the Creative Commons Attribution License (CC BY). The use, distribution or reproduction in other forums is permitted, provided the original author(s) and the copyright owner(s) are credited and that the original publication in this journal is cited, in accordance with accepted academic practice. No use, distribution or reproduction is permitted which does not comply with these terms.
*Correspondence: Mohamad Abdallah, bWhtYWQuYWJkYWxsYWgxQGdtYWlsLmNvbQ==
Disclaimer: All claims expressed in this article are solely those of the authors and do not necessarily represent those of their affiliated organizations, or those of the publisher, the editors and the reviewers. Any product that may be evaluated in this article or claim that may be made by its manufacturer is not guaranteed or endorsed by the publisher.
Research integrity at Frontiers
Learn more about the work of our research integrity team to safeguard the quality of each article we publish.