- 1UMR 7207, CNRS, Département Origines and Evolution, Centre de Recherche en Paléontologie, Muséum National d’Histoire Naturelle, Sorbonne Université, Paris, France
- 2UMR 7179, CNRS, MECADEV, Département Adaptations du Vivant, Muséum National d’Histoire Naturelle, Sorbonne Université, Paris, France
The evolution of the appendages during the fin-to-limb transition has been extensively studied, yet the majority of studies focused on the skeleton and the fossil record. Whereas the evolution of the anatomy of the appendicular musculature has been studied, the changes in the muscular architecture during the fin-to-limb transition remain largely unstudied, yet may provide important new insights. The fin-to-limb transition is associated with the appearance of a new mode of locomotion and the associated shift from pectoral to pelvic dominance. Here, we propose ways to investigate this question and review data on muscle mass and muscle architecture of the pectoral and pelvic muscles in extant vertebrates. We explore whether changes in appendage type are associated with changes in the muscular architecture and the relative investment in different muscle groups. These preliminary data show a general increase in the muscle mass of the appendages relative to the body mass during the fin-to-limb transition. The locomotor shift suggested to occur during the fin-to-limb transition appears supported by our preliminary data since in “fish” the pectoral fins are heavier than the pelvic fins, whereas in tetrapods, the forelimb muscles are less developed than the hind limb muscles. Finally, a shift in the investment in different muscle groups with an increase of the contribution of the superficial groups in tetrapods compared to “fish” appears to take place. Our study highlights the potential of investigating quantitative features of the locomotor muscles, yet also demonstrates the lack of quantitative data allowing to test these ideas.
Introduction
The water-to-land transition of vertebrates is associated with a number of morphological transformations of the body (Brazeau and Ahlberg, 2006; Daeschler et al., 2006), including the transformation from fins into limbs (Coates et al., 2002; Ahlberg et al., 2005; Cole et al., 2011; Pierce et al., 2012). The evolution of the appendages in sarcopterygians has been extensively studied, but the majority of the studies to date have focused on the skeleton as it is well documented in the fossil record (Andrews and Westoll, 1970; Coates et al., 2002; Boisvert, 2005; Daeschler et al., 2006; Shubin et al., 2006; Boisvert et al., 2008; Pierce et al., 2012). Previous studies on the development (Shubin and Alberch, 1986; Joss and Longhurst, 2001; Cole et al., 2011; Boisvert et al., 2013), the genetics (Coates, 1995; Johanson et al., 2007; Zhang et al., 2010; Nakamura et al., 2016), and the musculature (Boisvert et al., 2013; Diogo et al., 2016; Molnar et al., 2018, 2020) of fins and limbs have shed light on the evolution of the tetrapod limb. However, the changes in the investment in different muscle groups as well as the architecture (i.e., muscle mass, fiber length, pennation angle, and cross-section area) of the appendicular muscles during the fin-to-limb transition remain virtually unstudied. However, as the fin-to-limb transition is marked by the appearance of a new mode of locomotion (Ahlberg et al., 2005; Cole et al., 2011; Pierce et al., 2012), changes in muscle architecture as well as the differential investment in different functional groups can be expected. Soft-tissue preservation is typically very incomplete in fossils and consequently it is not possible to directly study the evolution of the appendicular muscles during the fin-to-limb transition. Although some exceptionally preserved stem-tetrapods like Ossinodus pueri have allowed a reconstruction of the forelimb muscles (Bishop, 2014), quantitative data like muscle masses cannot be extrapolated from the fossils.
Osteichthyans are divided in actinopterygians and sarcopterygians, two clades partly defined on the anatomy of the pectoral and pelvic appendages (Janvier, 1996). Actinopterygians have poly-basal articulated paired fins, with dermal fin rays that insert at the level of the pectoral and pelvic girdles by means of several radial elements connected to three basal cartilages and covered by small muscles located within the body wall. Sarcopterygians have mono-basal articulated paired appendages with an endoskeletal metapterygial axis formed by the alignment of several endoskeletal elements between the pectoral and pelvic girdles and the fin rays/digits. This metapterygial axis is covered by large muscles located at least partly outside of the body wall. The metapterygial axis is connected to the girdles by a single proximal element (Rosen et al., 1981; Janvier, 1996), but see Mansuit et al. (2021) for the coelacanth Latimeria where two proximal elements are connected to the pelvic girdle. Living sarcopterygians include the coelacanth, lungfish and tetrapods. In actinopterygians locomotion is mainly driven by body undulation generated by the axial musculature and the use of the pectoral fins. In contrast, in tetrapods, there is a dominance of the pelvic appendages in generating propulsion (Coates et al., 2002; Boisvert, 2005; Cole et al., 2011; Boisvert et al., 2013). The evolution from “front-to-rear-wheel drive” has been studied in early tetrapodomorph fossils (Carroll et al., 2005; Clack, 2012), yet has rarely been explored using living analogs. Quantitative information such as the muscle mass, fiber length, or muscle cross-section area is, however, crucial to be able interpret the ecological and locomotor changes that may have occurred during the fin-to-limb transition.
Although the relative mass of the limb muscles in tetrapods seems at first sight greater than that of the paired fins in “fishes”, this has never been investigated quantitatively. Here, we survey the literature to extract quantitative data on the muscle architecture of pectoral and pelvic appendages in extant vertebrates to investigate the hypothesis that a switch to a hindlimb-driven locomotion, as suggested by the skeletal anatomy of the appendages of tetrapodomorphs, is associated with changes in the limb muscles. We further explored whether changes in appendage type (fins or limbs) are associated with changes in muscle architecture and the investment in different muscle groups of the fore- and hind limbs. As quantitative data are scant, our preliminary analyses should be seen as a proof of concept and will hopefully stimulate researchers to investigate limb muscles more systematically and in a quantitative manner.
Materials and Methods
For this study we used data on muscle mass or architecture (e.g., cross-sectional area of a muscle) from published studies on the appendages of actinopterygians and sarcopterygians. The functional role of muscles can only be assessed by quantifying their size as well as their architecture. Architectural variables encompass muscle fiber length, fiber orientation and pennation, and the derived muscle cross-sectional areas which are a good proxy of the force-generating capacity of a muscle. Data for actinopterygians were taken from Thorsen and Westneat (2005), Dickson and Pierce (2019), and Crawford et al. (2020). Unfortunately, there was no study that compared the muscle architecture of the pectoral and pelvic fins in the same species; therefore, data were compiled across different studies. The data on the muscle architecture of the pectoral and pelvic fins of the extant coelacanth Latimeria chalumnae, as a model of a sarcopterygian fish, were taken from Huby et al. (2021). We used here data on Latimeria because it is the only sarcopterygian fish with data on the muscular architecture of its appendages, even if it is not the closest living relative to Tetrapoda. Concerning tetrapods, we excluded all birds from our dataset since they have highly derived forelimbs specialized for flight, but we covered a diversity of tetrapods that use quadrupedal locomotion. We included the data on the alligator Alligator mississippiensis (Allen et al., 2010), varanid lizards (Dick and Clemente, 2016; Cieri et al., 2020), and several mammals (Payne et al., 2005a; Ercoli et al., 2013, 2015; Moore et al., 2013; Rupert et al., 2015; Warburton et al., 2015; Olson et al., 2016; Martin et al., 2019). The publications on the coelacanth (Huby et al., 2021), the alligator (Allen et al., 2010), the varanids (Dick and Clemente, 2016; Cieri et al., 2020), the short-nosed bandicoot Isoodon (Warburton et al., 2015; Martin et al., 2019) and the grison Galictis (Ercoli et al., 2013, 2015) include both the pectoral and pelvic appendages. The data for the American badger, Taxidea taxus (Moore et al., 2013), the nine-banded armadillo, Dasypus novemcinctus (Olson et al., 2016), and the marmot Marmota monax (Rupert et al., 2015) pertain only to the forelimb, whereas the data for the horse Equus caballus (Payne et al., 2005a) pertain only to the hind limb.
The muscles were classified in homologous groups, using the homologies between fin and limb muscles proposed by Diogo et al. (2016) and Molnar et al. (2018, 2020). Four intrinsic muscle groups are considered here: the abductor superficialis, abductor profundus, adductor superficialis and adductor profundus groups (Figure 1). Concerning the pelvic limb of tetrapods, the caudofemoralis muscle is not considered in this study, despite being an important muscle for the retraction of the limb. As it originates from the axial musculature of the body it was considered not to be a limb muscle sensu stricto. Homologies between the muscles of the pectoral and pelvic appendages in each species included in this study are shown in Supplementary Tables 1, 2.
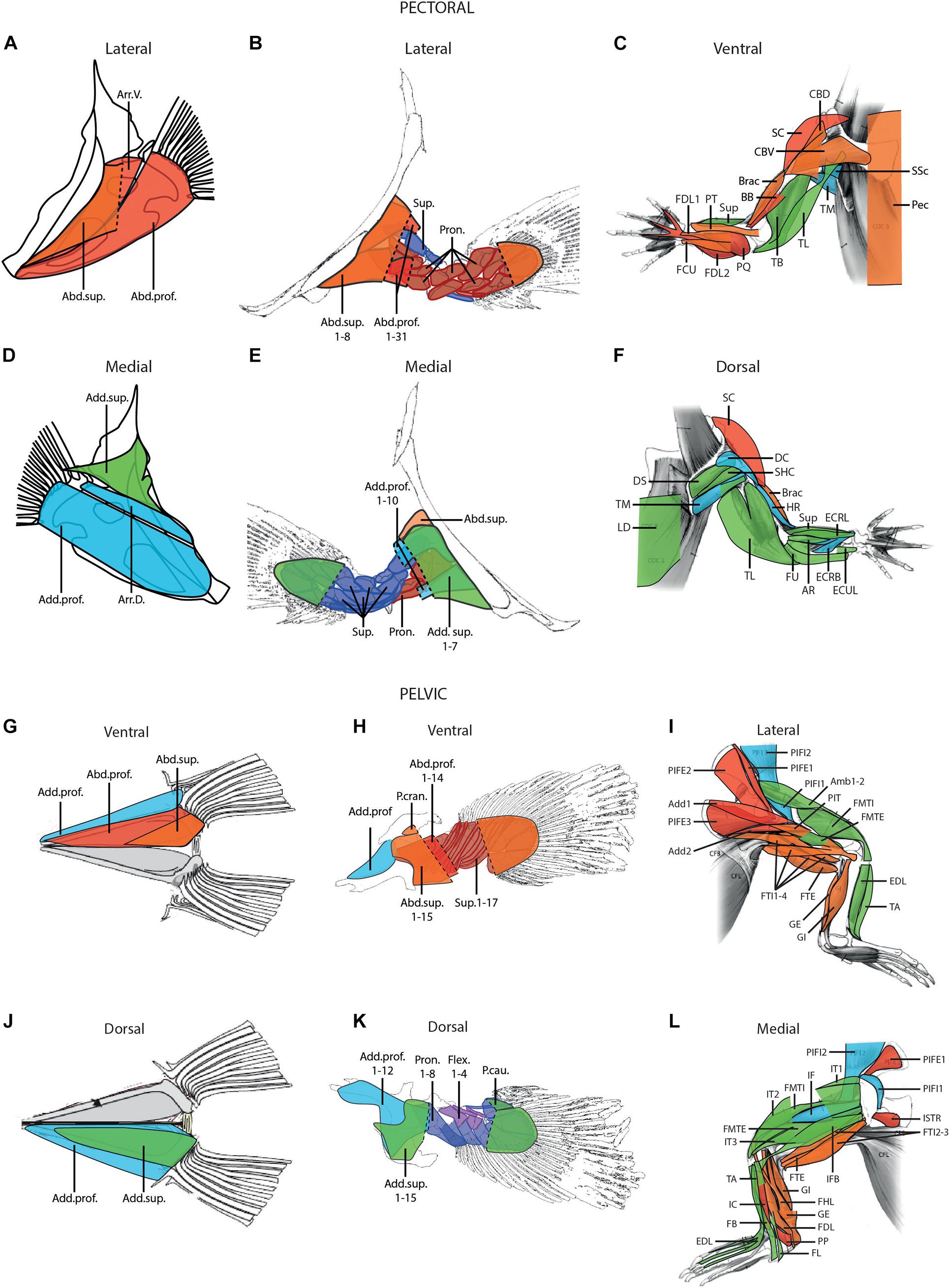
Figure 1. Hypotheses of homology of the muscles of the pectoral (A–F) and pelvic (G–L) appendages in fish (A,D,G,J), the coelacanth, Latimeria (B,E,H,K), and the alligator (C,F,I,L); modified from Thorsen and Westneat (2005), Allen et al. (2010), and Huby et al. (2021). Blue, adductor profundus group; green, adductor superficialis group; orange, abductor superficialis group; red, abductor profundus group. Note that for the coelacanth Latimeria (B,E,H,K) the profundus groups are separated in two different layers. Abd.prof., abductor profundus; Abd.sup., abductor superficialis; Add.prof., adductor profundus; Add.sup., adductor superficialis; Add, adductor; Amb, ambiens; Arr.D., arrector dorsalis; Arr.V., arrector ventralis; AR, abductor radialis; BB, biceps brachii; Brac, brachialis; CBD, coracobrachialis dorsalis; CBV, coracobrachialis ventralis; DC, deltoideus clavicularis; DS, deltoideus scapularis; ECRB, extensor carpi radialis brevis; ECRL, extensor carpi radialis longus; ECUL, extensor carpi ulnaris longus; EDL, extensor digitorum longus; FB, fibularis brevis; FCU, flexor carpi ulnaris; FDL, flexor digitorum longus; FHL, flexor hallucis longus; FL, fibularis longus; FMTE, femorotibialis externus; FMTI, femorotibialis internus; FTE, flexor tibialis externus; FTI, flexor tibialis internus; FU, flexor ulnaris; GE, gastrocnemius externus; GI, gastrocnemius internus; HR, humeroradialis; IC, interosseus cruris; IF, iliofemoralis; IFB, iliofibularis; ISTR, ischiotrochantericus; IT, iliotibialis; LD, latissimus dorsi; Pec, pectoralis; PIFE, puboischiofemoralis externus; PIFI, puboischiofemoralis internus; PIT, puboischiotibialis; PP, pronator profundus; PQ, pronator quadratus; Pron., pronator; PT, pronator teres; SC, supracoracoideus; SHC, scapulohumeralis caudalis; SSc, subscapularis; Sup, supinator; TA, tibialis anterior; TB, triceps brevis; TL, triceps longus; TM, teres major.
We first investigated the evolution of the muscle architecture of the pectoral and pelvic appendages, using the measurements obtained from the literature provided in Table 1. Only the data that allow the comparison between the pectoral and pelvic appendages are used here, i.e., for the coelacanth, the alligator, the varanid lizard and the short-nosed bandicoot. Although the data on the grison, Galictis, correspond to fore- and hind limbs, it is not possible to compare the two appendages since the muscle mass in the publications are given only in a relative proportion to the total muscle mass of the corresponding limb, and not to the total body mass (Ercoli et al., 2013, 2015).
We also explored the evolution of the distribution of the muscle groups in the appendages, using the measurements provided in Table 1. Since we focus on the distribution of the muscle groups inside an appendage, we can here also use the data from species for which only one appendage is available. We used data presented relative to body mass or relative to the overall appendicular muscle mass (Galictis, Ercoli et al., 2013, 2015) as well as the data on the physiological cross-sectional area (PCSA) of the fin muscles in fish (Cryptopsaras, Antennarius, and Carrasius; Dickson and Pierce, 2019; Crawford et al., 2020). The data are, however, not complete enough to statistically test the observed trends. Unfortunately, quantitative data on complete limbs are rare and data for both front and hind limbs of the same species are even rarer. Yet, such data are needed to statistically test hypotheses pertaining to the fin-to-limb transition.
Results
Evolution of the Muscle Architecture of the Pectoral and Pelvic Appendages
In the sarcopterygian fish Latimeria, the muscle mass of the pectoral limb is greater than that of the pelvic limb (0.43 vs. 0.30% of total body mass). In tetrapods, the muscle mass of the pectoral limb is smaller than that of the pelvic limb (Table 1). Moreover, it appears that in tetrapods, the muscle mass of the limbs is proportionally greater than in fish-like animals. Indeed, in actinopterygians and Latimeria, each appendage has a mass relative to the body mass inferior to 1% (Table 1), whereas in tetrapods, the mass, relative to body mass, is greater (>1.7%; up to 8.5% in the short-nosed bandicoot).
Evolution of the Architecture of the Muscle Groups
In actinopterygians, both for the pectoral and pelvic fins, the muscle groups that contribute the most to the overall fin muscle mass are the deep groups (abductor/adductor profundus) (Table 1). Similarly, in the sarcopterygian fish Latimeria, the muscle groups that contribute the most to the total muscle mass are the deep muscles (abductor/adductor profundus groups) (Table 1), irrespective of the limb. However, in tetrapods, the muscle groups that contribute most to the mass of the appendages are the superficial groups (abductor/adductor superficialis), both for the fore- and hind limbs. In this data set only the horse Equus caballus stands out since the adductor profundus group is heavier than the adductor superficialis group.
Discussion
Shift From Pectoral to Pelvic Appendage Dominance
The fin-to-limb transition has been proposed to be characterized by a shift from the pectoral to pelvic dominance causing changes in its size and proportions (Coates et al., 2002; Boisvert, 2005; Don et al., 2013). Indeed, in actinopterygians and sarcopterygian fishes the pectoral fin is greater than the pelvic fin and the pelvic girdle is small and “free” from the axial skeleton. The muscular data from the different studies included in our meta-analysis tend to confirm this hypothesis. Indeed, for the sarcopterygian fish Latimeria, the pectoral fin has a greater muscle mass than the pelvic fin, whereas in tetrapods, the hind limb has a greater mass than the forelimb. The fins are also proportionally smaller than the limbs of tetrapods since the summed muscle mass of each fin represents less than 1% of the total body mass of the organism (Table 1). In tetrapods, the relative size of each limb is greater, reaching up to nearly 6% of the total body mass. According to Andrews and Westoll (1970), the size difference between fins and limbs is probably due to their different function. Indeed, in fish locomotion and propulsion is mainly produced by lateral undulation of the body and the use of the caudal fin (Bainbridge, 1963; Lighthill, 1971; Webb, 1982; Lauder, 2000; George and Westneat, 2019). The paired fins are mainly used for different types of maneuvers (Webb, 1982; Wilga and Lauder, 1999; Lauder, 2000; Drucker and Lauder, 2003; Standen, 2008). During terrestrial locomotion in tetrapods the limbs need to support the body against gravity in addition to providing propulsion. In support of this idea, it has been demonstrated that in a more terrestrial living environment the endoskeleton of the pectoral fin of Polypterus becomes longer and more robust due to the increased use of the fins for locomotion and in the lifting the anterior part of the body above the substrate (Standen et al., 2014; Du and Standen, 2020). The terrestrial environment and a walking-type locomotion thus appear to require greater forces to move the appendages, compared to swimming (Du and Standen, 2017). Interestingly, in benthic anglerfish that walk on the substrate the pectoral fins are also stronger and more robust (Dickson and Pierce, 2019). Moreover, the muscles of the pectoral fin are heavier and stronger compared to body size, compared to the pelagic anglerfishes that swim. Dickson and Pierce (2019) showed, for example, that the benthic anglerfish Antennarius has four times stronger fin muscles than the pelagic anglerfish Carrassius. This stronger fin is associated with a larger muscle volume and mass. The larger and stronger fins or limbs are considered as essential to support the larger pushing forces against the substrate, necessary for locomotion (Du and Standen, 2017). Thus, larger muscles allow to produce the greater forces needed to support the body and to move on the substrate by walking both in benthic and terrestrial environments. It is, however, necessary to be cautious with these generalizations. Indeed, most of the data sets are incomplete and only focus on one appendage, thus complicating the interpretations of the results. Furthermore, even if there are numerous studies that focus on the muscle architecture of the limbs in mammals, data for other tetrapods are scarce, especially for lissamphibians. Data on the pelvic fins of fishes are also particularly scant. A larger sample of fish and tetrapods is clearly needed to be able to generalize these results, and may allow to test many of the trends observed here.
Distribution of the Muscle Groups
The distribution of the muscle groups along the appendages, based on the homologous relations described by Diogo et al. (2016) (Supplementary Tables 1, 2), is different between “fishes” and tetrapods. The abductor and adductor superficialis muscle groups are heavier than the deep muscle groups in tetrapods, both for the pectoral and pelvic appendages. In fish, the distribution of the muscle groups is different since the deep muscle groups are more developed. Thus, during the fin-to-limb transition there was a shift in the distribution of the muscle groups with a reduction of the deep muscles in tetrapods and the development of the superficial muscle groups in term of mass and strength. The heavier adductor profundus compared to adductor superficialis in the hind limb of Equus caballus represents an unusual condition among tetrapods. It is possible that this distribution of the muscle groups in the hind limb of the horse is linked with the adaptation for cursoriality and the skeletal anatomy of the limb. However, it would be necessary to look at the distribution of the muscle groups inside the forelimb of the horse to validate this hypothesis, yet the data in the literature are incomplete concerning this limb. The extrinsic muscle anatomy of this limb and the distal muscle anatomy were studied in two different studies (Brown et al., 2003; Payne et al., 2005b), and the compilation of the data does not allow to obtain a full overview of the fore limb muscle anatomy. Except for the horse which presents functional features of the fore- and hind limbs associated with a highly specialized cursorial locomotion, it appears that the increase in size and development of the muscles of the superficial groups may be linked to the adaptations of the limbs to terrestrial locomotion. However, the change in muscles distribution might not only be linked to this “new” mode of locomotion. Indeed, in walking anglerfishes, the distribution of the muscle groups is similar to that of swimming fishes with deep muscles that are stronger than the superficial muscles. It is thus likely that other constraints are involved in the changes in relative muscle development observed in tetrapods. Specifically, terrestrial tetrapods need to support their body mass whereas in anglerfish buoyancy helps to support body mass. It is consequently essential to complete the dataset with fish that “walk” in a terrestrial environment to test the idea that buoyancy has an impact on the distribution of the muscles in “walking” fishes.
Whereas in terrestrial tetrapods the change in muscle distribution seems directly linked to walking and the support of the body against gravity, specialized locomotor modes (e.g., digging, running, flying, or swimming) may cause animals to deviate from this general pattern. Adding species with specialized locomotor behaviors could help reconstruct the muscles in early tetrapods with different life styles.
Conclusion
This preliminary study presents promising results on the evolution of the muscular anatomy of the appendages during the fin-to-limb transition and shows that these types of data may help to better understand this major evolutionary transition. The general increase in the muscle mass of the appendages relative to body size in tetrapods is in line with observations on the skeletal elements in the fossil record. More strongly developed muscles are often associated with an increase in the force developed by the muscles directly linked to the function of the appendages. Indeed, in fishes, propulsion is mainly produced by the axial muscles involved in the lateral undulation of the body and the caudal fin, whereas in terrestrial tetrapods the limbs support the body and produce most of the thrust for the propulsion. The proposed shift from “front-” to “rear-wheel drive,” suggested by the changes in skeletal anatomy during the evolutionary history of tetrapodomorphs, is also corroborated by the muscle distribution data available in the literature. Indeed, in fish the pectoral fins are heavier than the pelvic fins, whereas in terrestrial tetrapods the forelimbs are smaller and lighter than the hind limbs relative to body size. Finally, this study highlights a shift in the distribution of the muscle groups during the fin-to-limb transition with an increase of the contribution of the superficial muscle groups in tetrapods compared to “fish.” However, as quantitative data in the literature are often fragmentary and incomplete, it is crucial to add quantitative data from dissections to be able to formally test these hypotheses.
Data Availability Statement
The original contributions presented in the study are included in the article/Supplementary Material, further inquiries can be directed to the corresponding author.
Author Contributions
RM and AH designed the study and interpreted the results. RM wrote the manuscript. AH revised the manuscript. Both authors contributed to the article and approved the submitted version.
Funding
This work was supported by a grant from Agence Nationale de la Recherche in the LabEx ANR-10-LABX-0003-BCDiv, program “Investissements d’avenir” no. ANR-11-IDEX-0004-02.
Conflict of Interest
The authors declare that the research was conducted in the absence of any commercial or financial relationships that could be construed as a potential conflict of interest.
Publisher’s Note
All claims expressed in this article are solely those of the authors and do not necessarily represent those of their affiliated organizations, or those of the publisher, the editors and the reviewers. Any product that may be evaluated in this article, or claim that may be made by its manufacturer, is not guaranteed or endorsed by the publisher.
Acknowledgments
We thank Gaël Clément and Marc Herbin from the MNHN for their advice that improved our work. We are grateful to Catherine Boisvert and Julia Molnar for their invitation to contribute to this research collection. We also thank reviewers for constructive comments that improved this manuscript.
Supplementary Material
The Supplementary Material for this article can be found online at: https://www.frontiersin.org/articles/10.3389/fevo.2021.702576/full#supplementary-material
Supplementary Table 1 | Hypotheses of homology between pectoral appendicular muscles of fishes and tetrapods used in this manuscript, based on Diogo et al. (2016).
Supplementary Table 2 | Hypotheses of homology between pelvic appendicular muscles of fishes and tetrapods used in this manuscript, based on Diogo et al. (2016).
References
Ahlberg, P. E., Clack, J. A., and Blom, H. (2005). The axial skeleton of the Devonian tetrapod Ichthyostega. Nature 437, 137–140. doi: 10.1038/nature03893
Allen, V. R., Elsey, R. M., Jones, N., Wright, J., and Hutchinson, J. R. (2010). Functional specialization and ontogenetic scaling of limb anatomy in Alligator mississippiensis. J. Anat. 216, 423–445. doi: 10.1111/j.1469-7580.2009.01202.x
Andrews, S. M., and Westoll, T. S. (1970). The postcranial skeleton of Eusthenopteron foordi Whiteaves. Earth Environ. Sci. Trans. R. Soc. Edinburgh 68, 207–329.
Bainbridge, R. (1963). Caudal fin and body movement in the Propulsion of some Fish. J. Exp. Biol. 40, 23–56.
Bishop, P. J. (2014). The humerus of Ossinodus pueri, a stem tetrapod from the Carboniferous of Gondwana, and the early evolution of the tetrapod forelimb. Alcheringa 38, 209–238. doi: 10.1080/03115518.2014.861320
Boisvert, C. A. (2005). The pelvic fin and girdle of Panderichthys and the origin of tetrapod locomotion. Nature 438, 1145–1147. doi: 10.1038/nature04119
Boisvert, C. A., Joss, J. M., and Ahlberg, P. E. (2013). Comparative pelvic development of the axolotl (Ambystoma mexicanum) and the Australian lungfish (Neoceratodus forsteri): conservation and innovation across the fish-tetrapod transition. EvoDevo 4, 1–19. doi: 10.1186/2041-9139-4-3
Boisvert, C. A., Mark-Kurik, E., and Ahlberg, P. E. (2008). The pectoral fin of Panderichthys and the origin of digits. Nature 456, 636–638. doi: 10.1038/nature07339
Brazeau, M. D., and Ahlberg, P. E. (2006). Tetrapod-like middle ear architecture in a Devonian fish. Nature 439, 318–321. doi: 10.1038/nature04196
Brown, N. A. T., Kawcak, C. E., McIlwraith, C. W., and Pandy, M. G. (2003). Architectural properties of distal forelimb muscles in horses, Equus caballus. J. Morphol. 258, 106–114. doi: 10.1002/jmor.10113
Carroll, R. L., Irwin, J., and Green, D. M. (2005). Thermal physiology and the origin of terrestriality in vertebrates. Zool. J. Linn. Soc. 143, 345–358. doi: 10.1111/j.1096-3642.2005.00151.x
Cieri, R. L., Dick, T. J. M., and Clemente, C. J. (2020). Monitoring muscle over three orders of magnitude: widespread positive allometry among locomotor and body support musculature in the pectoral girdle of varanid lizards (Varanidae). J. Anat. 237, 1114–1135. doi: 10.1111/joa.13273
Clack, J. A. (2012). Gaining Ground: The Origin and Evolution of Tetrapods. Second Edition. Bloomington: Indiana University Press.
Coates, M. I., Jeffery, J. E., and Ruta, M. (2002). Fins to limbs: what the fossils say. Evol. Dev. 4, 390–401.
Cole, N. J., Hall, T. E., Don, E. K., Berger, S., Boisvert, C. A., Neyt, C., et al. (2011). Development and evolution of the muscles of the pelvic fin. PLoS Biol. 9:e1001168. doi: 10.1371/journal.pbio.1001168
Crawford, C. H., Randall, Z. S., Hart, P. B., Page, L. M., Chakrabarty, P., Suvarnaraksha, A., et al. (2020). Skeletal and muscular pelvic morphology of hillstream loaches (Cypriniformes: Balitoridae). J. Morphol. 281, 1280–1295. doi: 10.1002/jmor.21247
Daeschler, E. B., Shubin, N. H., and Jenkins, F. A. (2006). A Devonian tetrapod-like fish and the evolution of the tetrapod body plan. Nature 440, 757–763. doi: 10.1038/nature04639
Dick, T. J. M., and Clemente, C. J. (2016). How to build your dragon: scaling of muscle architecture from the world’s smallest to the world’s largest monitor lizard. Front. Zool. 13:5. doi: 10.1186/s12983-016-0141-5
Dickson, B. V., and Pierce, S. E. (2019). How (and why) fins turn into limbs: insights from anglerfish. Earth Environ. Sci. Trans. R. Soc. Edinburgh 109, 87–103. doi: 10.1017/S1755691018000415
Diogo, R., Johnston, P., Molnar, J. L., and Esteve-Altava, B. (2016). Characteristic tetrapod musculoskeletal limb phenotype emerged more than 400 MYA in basal lobe-finned fishes. Sci. Rep. 6, 1–9. doi: 10.1038/srep37592
Don, E. K., Currie, P. D., and Cole, N. J. (2013). The evolutionary history of the development of the pelvic fin/hindlimb. J. Anat. 222, 114–133. doi: 10.1111/j.1469-7580.2012.01557.x
Drucker, E. G., and Lauder, G. V. (2003). Function of pectoral fins in rainbow trout: behavioral repertoire and hydrodynamic forces. J. Exp. Biol. 206, 813–826. doi: 10.1242/jeb.00139
Du, T. Y., and Standen, E. M. (2017). Phenotypic plasticity of muscle fiber type in the pectoral fins of Polypterus senegalus reared in a terrestrial environment. J. Exp. Biol. 220, 3406–3410. doi: 10.1242/jeb.162909
Du, T. Y., and Standen, E. M. (2020). Terrestrial acclimation and exercise lead to bone functional response in Polypterus pectoral fins. J. Exp. Biol. 223(Pt 11):jeb217554. doi: 10.1242/jeb.217554
Ercoli, M. D., Álvarez, A., Stefanini, M. I., Busker, F., and Morales, M. M. (2015). Muscular anatomy of the forelimbs of the lesser grison (Galictis cuja), and a functional and phylogenetic overview of mustelidae and other Caniformia. J. Mamm. Evol. 22, 57–91. doi: 10.1007/s10914-014-9257-6
Ercoli, M. D., Echarri, S., Busker, F., Álvarez, A., Morales, M. M., and Turazzini, G. F. (2013). The functional and phylogenetic implications of the myology of the lumbar region, tail, and hind limbs of the lesser grison (Galictis cuja). J. Mamm. Evol. 20, 309–336. doi: 10.1007/s10914-012-9219-9
George, A. B., and Westneat, M. W. (2019). Functional morphology of endurance swimming performance and gait transition strategies in balistoid fishes. J. Exp. Biol. 222:jeb194704.
Huby, A., Mansuit, R., Herbin, M., and Herrel, A. (2021). Revision of the muscular anatomy of the paired fins of the living coelacanth Latimeria chalumnae (Actinistia, Sarcopterygii). Biol. J. Linn. Soc. 2020:blab047. doi: 10.1093/biolinnean/blab047
Johanson, Z., Joss, J., Boisvert, C. A., Ericsson, R., Sutija, M., and Ahlberg, P. E. (2007). Fish fingers: digit homologues in sarcopterygian fish fins. J. Exp. Zool. Part B Mol. Dev. Evol. 308, 757–768. doi: 10.1002/jez.b.21197
Joss, J., and Longhurst, T. (2001). “Lungfish paired fins,” in Major Events in Early Vertebrate Evolution, ed. P. E. Ahlberg (London: Taylor and Francis), 370–376.
Lauder, G. V. (2000). Function of the caudal fin during locomotion in fishes: kinematics, flow visualization, and evolutionary patterns. Am. Zool. 40, 101–122.
Lighthill, M. J. (1971). Large-amplitude elongated-body theory of fish locomotion. Proc. R. Soc. London. Ser. B. Biol. Sci. 179, 125–138.
Mansuit, R., Clément, G., Herrel, A., Dutel, H., Tafforeau, P., Santin, M. D., et al. (2021). Development and growth of the pelvic fin in the extant coelacanth Latimeria chalumnae. Anat. Rec. 304, 541–558. doi: 10.1002/ar.24452
Martin, M. L., Warburton, N. M., Travouillon, K. J., and Fleming, P. A. (2019). Mechanical similarity across ontogeny of digging muscles in an Australian marsupial (Isoodon fusciventer). J. Morphol. 280, 423–435. doi: 10.1002/jmor.20954
Molnar, J. L., Diogo, R., Hutchinson, J. R., and Pierce, S. E. (2018). Reconstructing pectoral appendicular muscle anatomy in fossil fish and tetrapods over the fins-to-limbs transition. Biol. Rev. 93, 1077–1107. doi: 10.1111/brv.12386
Molnar, J. L., Diogo, R., Hutchinson, J. R., and Pierce, S. E. (2020). Evolution of hindlimb muscle anatomy across the tetrapod water-to-land transition, including comparisons with forelimb anatomy. Anat. Rec. 303, 218–234. doi: 10.1002/ar.23997
Moore, A. L., Budny, J. E., Russell, A. P., and Butcher, M. T. (2013). Architectural specialization of the intrinsic thoracic limb musculature of the American badger (Taxidea taxus). J. Morphol. 274, 35–48. doi: 10.1002/jmor.20074
Nakamura, T., Gehrke, A. R., Lemberg, J., Szymaszek, J., and Shubin, N. H. (2016). Digits and fin rays share common developmental histories. Nature 537, 225–228. doi: 10.1038/nature19322
Olson, R. A., Womble, M. D., Thomas, D. R., Glenn, Z. D., and Butcher, M. T. (2016). Functional morphology of the forelimb of the nine-banded armadillo (Dasypus novemcinctus): comparative perspectives on the myology of dasypodidae. J. Mamm. Evol. 23, 49–69. doi: 10.1007/s10914-015-9299-4
Payne, R. C., Hutchinson, J. R., Robilliard, J. J., Smith, N. C., and Wilson, A. M. (2005a). Functional specialisation of pelvic limb anatomy in horses (Equus caballus). J. Anat. 206, 557–574.
Payne, R. C., Veenman, P., and Wilson, A. M. (2005b). Erratum: the role of the extrinsic thoracic limb muscles in equine locomotion (Journal of Anatomy (2004)). J. Anat. 206, 193–204.
Pierce, S. E., Clack, J. A., and Hutchinson, J. R. (2012). Three-dimensional limb joint mobility in the early tetrapod Ichthyostega. Nature 486, 523–526. doi: 10.1038/nature11124
Rosen, D. E., Forey, P. L., Gardiner, B. G., and Patterson, C. (1981). Lungfishes, tetrapods, paleontology, and plesiomorphy. Bull. Am. Museum Nat. Hist. 167, 159–276.
Rupert, J. E., Rose, J. A., Organ, J. M., and Butcher, M. T. (2015). Forelimb muscle architecture and myosin isoform composition in the groundhog (Marmota monax). J. Exp. Biol. 218, 194–205. doi: 10.1242/jeb.107128
Shubin, N. H., and Alberch, P. (1986). A morphogenetic approach to the origin and basic organization of the tetrapod limb. Evol. Biol. 20, 319–387.
Shubin, N. H., Daeschler, E. B., and Jenkins, F. A. (2006). The pectoral fin of Tiktaalik roseae and the origin of the tetrapod limb. Nature 440, 764–771. doi: 10.1038/nature04637
Standen, E. M. (2008). Pelvic fin locomotor function in fishes: three-dimensional kinematics in rainbow trout (Oncorhynchus mykiss). J. Exp. Biol. 211, 2931–2942. doi: 10.1242/jeb.018572
Standen, E. M., Du, T. Y., and Larsson, H. C. E. (2014). Developmental plasticity and the origin of tetrapods. Nature 513, 54–58. doi: 10.1038/nature13708
Thorsen, D. H., and Westneat, M. W. (2005). Diversity of pectoral fin structure and function in fishes with labriform propulsion. J. Morphol. 263, 133–150. doi: 10.1002/jmor.10173
Warburton, N. M., Malric, A., Yakovleff, M., Leonard, V., and Cailleau, C. (2015). Hind limb myology of the southern brown bandicoot (Isoodon obesulus) and greater bilby (Macrotis lagotis) (Marsupialia: Peramelemorphia). Aust. J. Zool. 63, 147–162. doi: 10.1071/ZO14087
Webb, P. W. (1982). Locomotor patterns in the evolution of actinopterygian fishes. Am. Zool. 22, 329–342.
Wilga, C. D., and Lauder, G. V. (1999). Locomotion in sturgeon: function of the pectoral fins. J. Exp. Biol. 202, 2413–2432.
Keywords: appendages, muscle architecture, locomotor shift, homology, functional morphology
Citation: Mansuit R and Herrel A (2021) The Evolution of Appendicular Muscles During the Fin-to-Limb Transition: Possible Insights Through Studies of Soft Tissues, a Perspective. Front. Ecol. Evol. 9:702576. doi: 10.3389/fevo.2021.702576
Received: 29 April 2021; Accepted: 13 July 2021;
Published: 03 August 2021.
Edited by:
Ingmar Werneburg, University of Tübingen, GermanyReviewed by:
Virginia Abdala, IBN, CONICET-UNT, ArgentinaDavid Marjanović, Museum of Natural History Berlin (MfN), Germany
Copyright © 2021 Mansuit and Herrel. This is an open-access article distributed under the terms of the Creative Commons Attribution License (CC BY). The use, distribution or reproduction in other forums is permitted, provided the original author(s) and the copyright owner(s) are credited and that the original publication in this journal is cited, in accordance with accepted academic practice. No use, distribution or reproduction is permitted which does not comply with these terms.
*Correspondence: Rohan Mansuit, cm9oYW4ubWFuc3VpdEBtbmhuLmZy