- 1Department Agrobiological Resources, Faculty of Agriculture, Meijo University, Nagoya, Japan
- 2Faculty of Agriculture, Kindai University, Nara, Japan
- 3Center for Ecological Research, Kyoto University, Otsu, Japan
We evaluated the effectiveness of using a blend of volatiles that attract Cotesia vestalis, a specialist parasitoid wasp of diamondback moth (DBM) larvae, to control DBM larvae on cabbage plants under open field conditions. We set three dispensers of the synthetic C. vestalis attractant together with one sugary-food feeder in a cabbage plot (10 m × 1 m; the treated plot) on one side of a pesticide-free open agricultural field (approximately 20 m × 20 m) from June to September in 2010 and July to August in 2011. On the other side of the field, we created a control cabbage plot of the same size in which neither dispensers nor a feeder was set. The incidences of DBM larvae and C. vestalis cocoons in the control and treated plots were compared. In 2010, the incidence of DBM larvae in the treated plot was significantly lower than that in the control plot. Poisson regression analyses in 2010 showed that the rate of increase in the number of C. vestalis cocoons along with an increase in the number of DBM larvae in the treated plot was significantly higher than that in the control plot. In 2011, the incidence in both the treated and control plots remained low (five larvae per plant or less) with no significant difference between the plots. Poisson regression analyses in 2011 showed that the number of C. vestalis cocoons in the treated plot was significantly higher than that in the control plot, irrespective of the number of DBM larvae. This 2-year field study suggested that the dispensers recruited native C. vestalis from the surrounding environment to the treated plot, and the dispensers controlled the number of DBM larvae in 2010 when the density of DBM larvae exceeded the economic injury levels for the cabbage crop. We also compared the incidences of other arthropods in the control and treated plots. The incidences of Pieris rapae larvae and Plusiinae spp. were not affected by the treatments. The number of aphids in the treated and control plots was inconsistent between the 2 years. Based on these 2-year results, the possible use of C. vestalis attractants in open agricultural fields is discussed.
Introduction
Plants infested by herbivorous arthropods emit herbivory-induced plant volatiles (HIPVs); one of the ecological functions of HIPVs is to attract carnivorous natural enemies (i.e., parasitoid insects and predatory arthropods) of herbivorous arthropods (Takabayashi and Dicke, 1996; Arimura et al., 2009; McCormick et al., 2012; Takabayashi and Shiojiri, 2019). The attraction of natural enemies with HIPVs could be one of the induced indirect defence strategies of plants against herbivores when the emission reduces damage from herbivores. Interestingly, there are cases where plants attract the specific carnivorous natural enemies of currently infesting herbivorous arthropods by emitting herbivore species-specific blends of HIPVs (Takabayashi and Dicke, 1996; Arimura et al., 2009; McCormick et al., 2012; Takabayashi and Shiojiri, 2019).
Diamondback moth (DBM) (Plutella xylostella) larva is one of the most destructive pests of cruciferous crops worldwide, and it has developed resistance to many insecticides (Talekar and Shelton, 1993; Furlong et al., 2013). The annual cost for managing it is estimated to be USD 4–5 billion (Furlong et al., 2013). Cotesia vestalis, a solitary specialist parasitoid wasp of DBM larvae, can be effective in the control of DBM larvae (Talekar and Yang, 1993; Abe et al., 2007; Furlong et al., 2013). C. vestalis prefers DBM larvae-infested crucifer plants (cabbage or Japanese radish) to artificially damaged plants or plants infested by non-host larvae (cabbage white butterfly larvae, Pieris rapae) (Shiojiri et al., 2000), suggesting the above-mentioned specific responses to herbivore species-specific HIPVs. We also identified a C. vestalis-attractive blend of volatiles comprising (Z)-3-hexenyl acetate, α-pinene, sabinene, and n-heptanal from DBM larvae-infested cabbage plants, and the attractiveness of this synthetic blend to C. vestalis was confirmed under laboratory, greenhouse, and field conditions (Shiojiri et al., 2010; Uefune et al., 2012, 2020; Ohara et al., 2017).
The use of HIPVs for pest control has attracted interest (reviews, Khan et al., 2008; Kapran, 2012; Pappas et al., 2017; Turlings and Erb, 2018). Previous studies have used HIPVs commonly released by a wide range of infested plants to attract unspecified natural enemies (e.g., James, 2003a,b, 2005; for review Kapran, 2012). For example, James (2003a) found that (Z)-3-hexenyl acetate, one of the green leaf volatiles, attracted predatory mirids and anthocorids, whereas methyl salicylate attracted geocorids and hoverflies in hop yards. Artificial recruitment of specific natural enemies into a target agricultural field from the surrounding environment by utilising herbivore-species-specific HIPVs is another way to control target pest arthropods. We recently reported that setting dispensers of a synthetic C. vestalis-attractive blend of volatiles, together with sugary-food feeders for C. vestalis, resulted in a significant reduction in the monthly incidence of DBM in commercial greenhouses, with a significantly higher number of C. vestalis recorded when the number of DBM adults increased (Uefune et al., 2020).
It remains to be answered whether C. vestalis-attractant can be used not only in greenhouses but also in open agricultural fields for DBM control. Here, as the first step to answer this question, we conducted field experiments using pesticide-free cabbage plots in Kyoto in 2010 and 2011. The possible use of HIPVs that attract specific natural enemies in open agricultural fields is discussed.
Materials and Methods
Dispensers of Cotesia vestalis Attractants
Four HIPVs, (Z)-3-hexenyl acetate (>97%: Wako Pure Chemical Industries, Ltd., Osaka, Japan), α-pinene (a mixture of both isomers, >97%: Tokyo Kasei Kogyo Co. Ltd., Tokyo, Japan), sabinene (>70%: RC Treatt & Co. Ltd., Suffolk, United Kingdom), and n-heptanal, (>95%: Tokyo Kasei Kogyo Co. Ltd., Tokyo, Japan) were mixed in a mass ratio of 1.8:1.3:2.0:3.0 based on gas chromatographic analysis of emissions from DBM-infested cabbage plants (Shiojiri et al., 2010). The mixture was dissolved in triethyl citrate (TEC, >99%: Pfizer, New York, United States) to facilitate a low volatilisation rate.
Dispensers were constructed by saturating each of five cellulose blocks (22 mm × 35 mm × 2.8 mm) (Hioki Co Ltd. Tokyo, Japan) with 1.7 g of TEC. These blocks were aligned in a row and wrapped in micropore polyethylene film (30 mm × 40 mm × 100 μm) (Mitsui Plastic Trade Co., Tokyo, Japan) using a thermal sealing device to ensure a low volatilisation rate (for details, see Kugimiya et al., 2017). Based on the results of a previous field study, we used a dispenser with 425 mg attractant (85 mg per block) for the open field experiments (for details, see Uefune et al., 2020).
Kugimiya et al. (2017) reported that the attractiveness of one cellulose block covered with film with the same amount (85 mg) of the attractant, as in this study, and that with 0.85 mg attractant were not significantly different under laboratory conditions. Further, the block with 85 mg attractant emitted more than 10% of the attractant after 42 days. The ratios of the blend showed some changes according to duration. However, these slight changes will not affect the attraction, since Uefune et al. (2013a) reported that not only the real blend (used in this study) but also an artificially made blend (1:1:1:1 blend: similar to the blend after 42 days) attracted C. vestalis. Thus, in this study, the dispensers were expected to be attractive during the experimental period.
Field Experimental Conditions
Field experiments were conducted in an open agricultural field owned by a farmer in a rural area of the northern part of Kyoto City, Japan. We set two plots (10 m × 1 m each) at opposite ends of the field (northwest and southeast). In each plot, we planted 50 cabbage seedlings (Brassica oleracea var. capitata ‘Shikidori’) on 12 June 2010 and 19 June 2011. Sweet potato plants were grown by the owner of the fields in the area between the two plots (17 m × 17 m). No pesticides were applied during the experimental period. We switched the control plot with the treated plot in the second year to exclude the effect of space.
One plot (the northwest plot in 2010 and the southeast plot in 2011) was assigned to be the treated plot in which the dispensers and feeder were set. On the planting day, we set three dispensers, each of which was attached to an iron pole 2 m above the ground. Three poles were set every 3 m along the plot, and one sugary-food feeder (5 cm diameter × 7 cm tall yellow glass bottle filled with honey; for details, see Shimoda et al., 2014) was set 50 cm above the ground on the centre pole. Another plot on the opposite side was assigned to be the control plot, in which neither dispensers nor feeders were set. The experimental days were as follows: 25 June, 2 July, 17 July, 22 July, 4 August, 26 August, 3 September, and 10 September 2010, and 8 July, 15 July, 9 August, 18 August, and 31 August 2011. We attached a new dispenser to each pole and renewed the feeder on 2 July, 22 July, and 26 August 2010, and on 15 July and 18 August 2011.
We observed 10 randomly chosen cabbage plants and counted the number of DBM larvae and cocoons of C. vestalis in the treated and control plots. DBMs and C. vestalis cocoons are commonly found on wild cruciferous weeds, particularly on yellowcress plants (Rorippa indica) in the northern part of Kyoto (Abe et al., 2020). In the treated and control plots, we also recorded aphids, Pieris rapae larvae, and Plusiinae spp. larvae as pest insects of cabbage crops; the caterpillars are non-hosts of C. vestalis. We also observed parasitised aphids (mummies) and clusters of cocoons of C. glomerata, a parasitoid wasp of P. rapae larvae. The numbers of these arthropods were also recorded. The observed arthropods, except for aphids, were identified based on their morphology. The aphid species observed in the plots were not identified.
Statistics
Comparisons Between Treated and Untreated Plots
We analysed the effects of the treatment, day, their interaction on the number of DBM larvae, C. vestalis cocoons, and other insect pests and their natural enemies in the treated and control plots. To do so, we used the least square mixed model after Box-Cox transformation in JMP version 14.2.0 (SAS Institute, 2018). When a data set had 0 values, 0.5 was added to all values in the data set before the Box-Cox transformation. The individual cabbage plant was a random effect in all models.
The Relationship Between DBM Larvae and Cotesia vestalis Cocoons in the Treated and Control Plots
To analyse the relationship between DBM larvae and C. vestalis in the treated and control plots, we focussed on the number of C. vestalis relative to the increased number of DBM. To do this, we used Poisson regression analysis in JMP version 14.2.0 (SAS Institute, 2018).
Results
Number of DBM Larvae and Cotesia vestalis Cocoons
Regarding the number of DBM larvae, the effect of day in both years was significant (P < 0.0001). In 2010, the effects of treatment and interaction between the treatment and day were significant (treatment: P = 0.0450, and interaction: P = 0.0011) (Figure 1A), indicating that the treatment suppressed the number of DBM larvae and that this effect varied on individual days. In 2011, the effect of treatment was not significant (P = 0.8053), while that of the interaction between treatment and day was significant (P = 0.0024) (Figure 1B), indicating that the treatment did not suppress the number of DBM larvae.
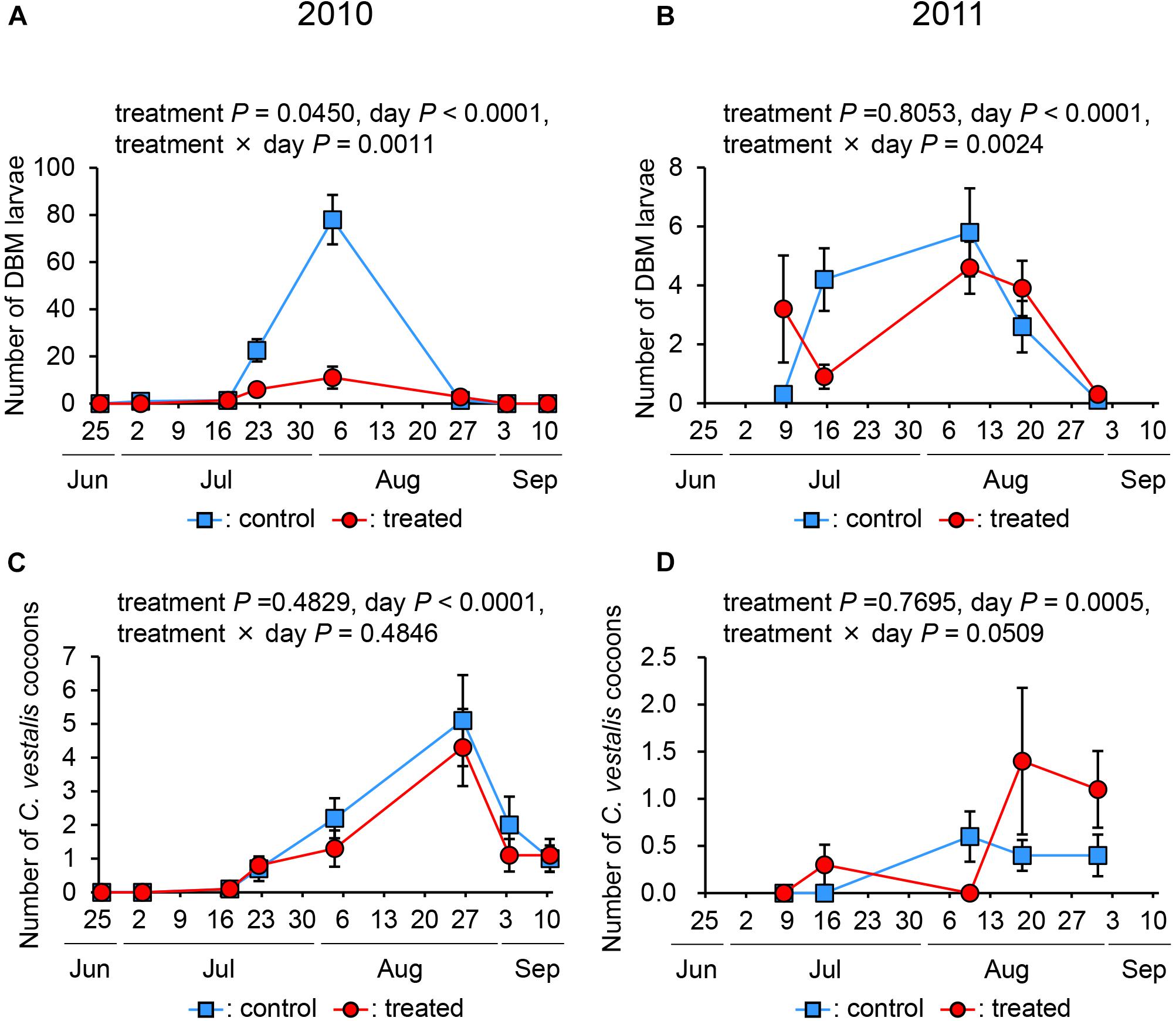
Figure 1. The number of diamondback moth larvae (A,B) and Cotesia vestalis cocoons (C,D) in treated and control plots in 2010 and 2011.
Regarding the number of C. vestalis, the effect of day in both years was significant (in 2010, P < 0.0001; in 2011, P = 0.0005). The treatment and interaction between the treatment and day in both years were not significant (in 2010, treatment: P = 0.4829, and interaction: P = 0.4846; in 2011, treatment: P = 0.7695, and interaction: P = 0.0509), indicating that the number of C. vestalis in the treated plot was not significantly different from that in the control plot in both years (Figures 1C,D).
The Relationship Between the Number of DBM Larvae and Cotesia vestalis Cocoons
In 2010, Poisson regression analyses showed that the factors of DBM larvae number and interaction (treatment × DBM larvae number) had a significant effect on the number of C. vestalis cocoons, while the factor treatment had no effect (treatment: P = 0.1816, day: P < 0.0001, interaction: P = 0.0060) (Figure 2A). In contrast, in 2011 the treatment had a significant effect on the number of C. vestalis cocoons, while the number of DBM and the day × treatment interaction had no significant effect (treatment: P = 0.0457, day: P = 0.1459, interaction: P = 0.5580) (Figure 2B). As interaction (2010) or treatment (2011) was significant, it is concluded that the treatment results in a significantly higher number of C. vestalis in the treated plot than in the control plot in both years.
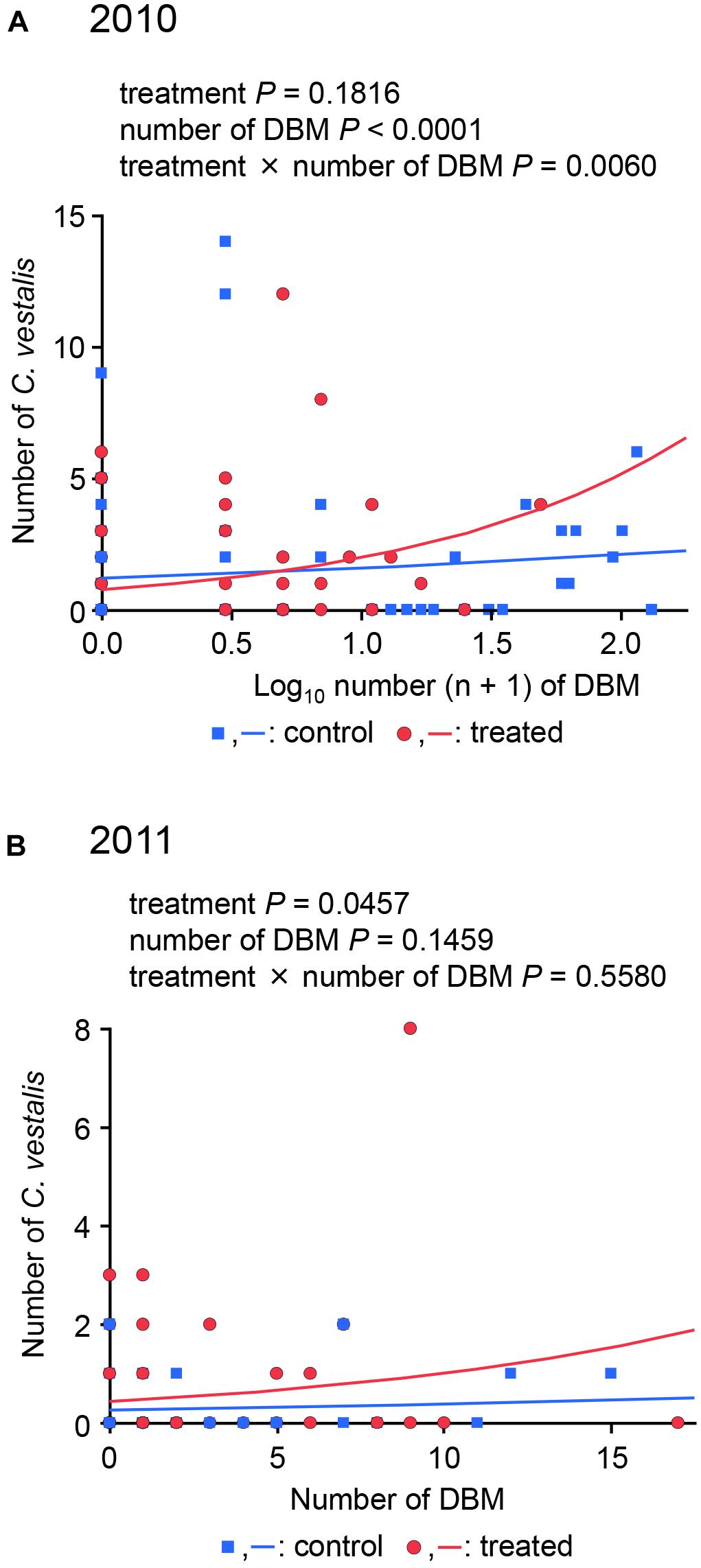
Figure 2. Poisson regression analyses of the number of diamondback moth larvae and Cotesia vestalis cocoons in 2010 (A) and 2011 (B).
Number of Aphids and Aphid Mummies
Regarding the number of aphids, the day was significant in both years (P < 0.0001). In 2010, treatment was significant (P = 0.0038), while the interaction between treatment and day was not (P = 0.2264) (Figure 3A), indicating that the treatment results in the suppression of the number of aphids. In 2011, the effects of treatment and interaction between day and treatment were both significant (treatment, P < 0.0001; interaction, P = 0.0009) (Figure 3B). However, in 2011, the number of aphids in the treated plot was higher than that in the control plot.
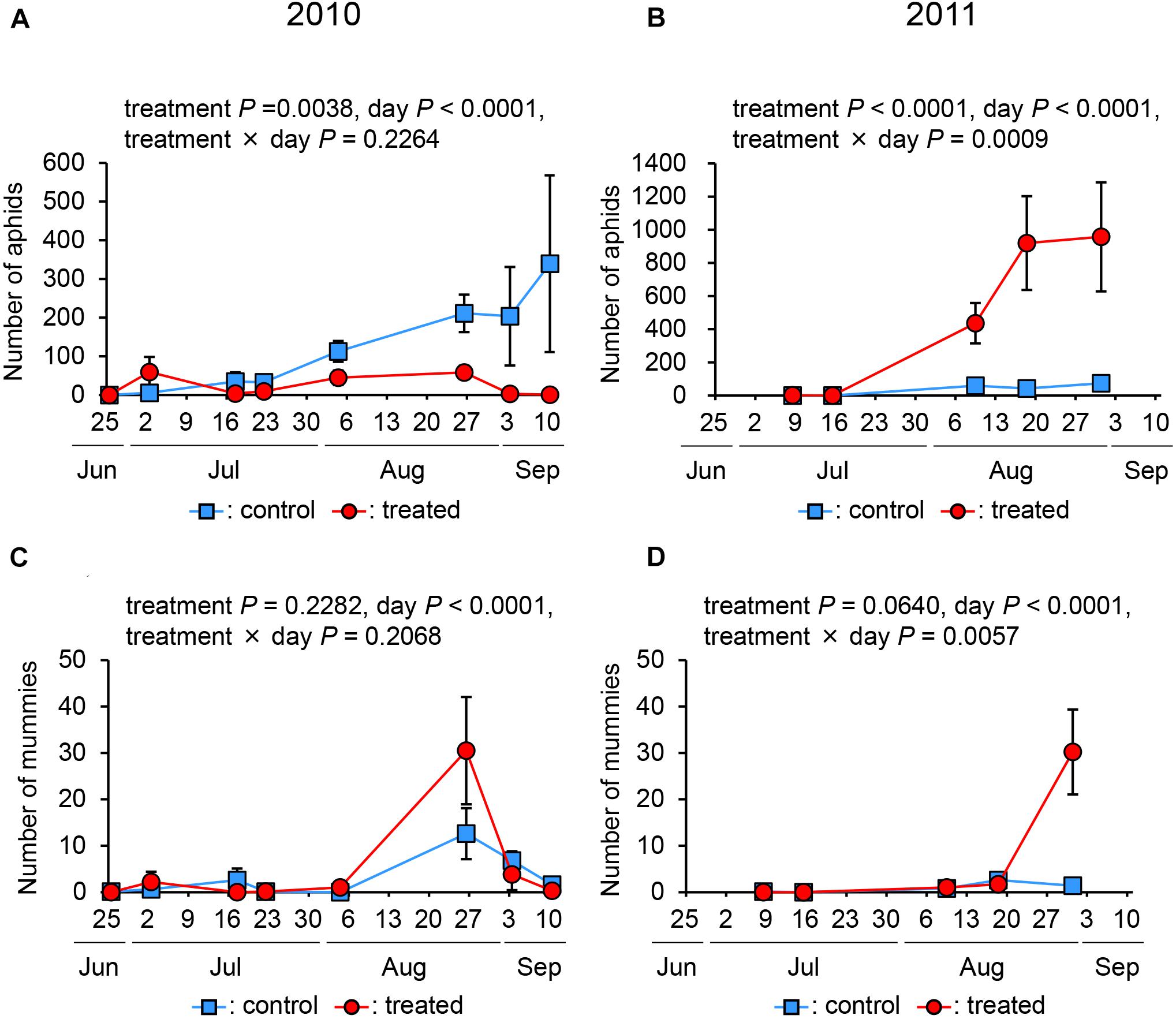
Figure 3. The number of aphids (A,B) and parasitised aphids (mummies) (C,D) in treated and control plots in 2010 and 2011.
Regarding the number of mummies, the day was significant in both years (P < 0.0001). In 2010, the effect of treatment and day × treatment interaction was not significant (treatment: P = 0.2282, and interaction: P = 0.2068), indicating that the treatment did not affect the number of mummies. In 2011, the effect of the treatment was not significant (P < 0.0640), and that of the interaction was significant (P = 0.0057) (Figures 3C,D). This indicates that the number of mummies in the treated plot was higher than that in the control plot.
Number of Pieris rapae Larvae and Cotesia glomerata
Regarding the number of P. rapae larvae, in both years, the effects of treatment were not significant (in 2010, P = 0.0678; in 2011, P = 0.1943), while those of day and day × treatment interaction were significant (in 2010, day: P < 0.0001, interaction: P < 0.0001; in 2011, day: P < 0.0001, and interaction: P < 0.0068) (Figures 4A,B).
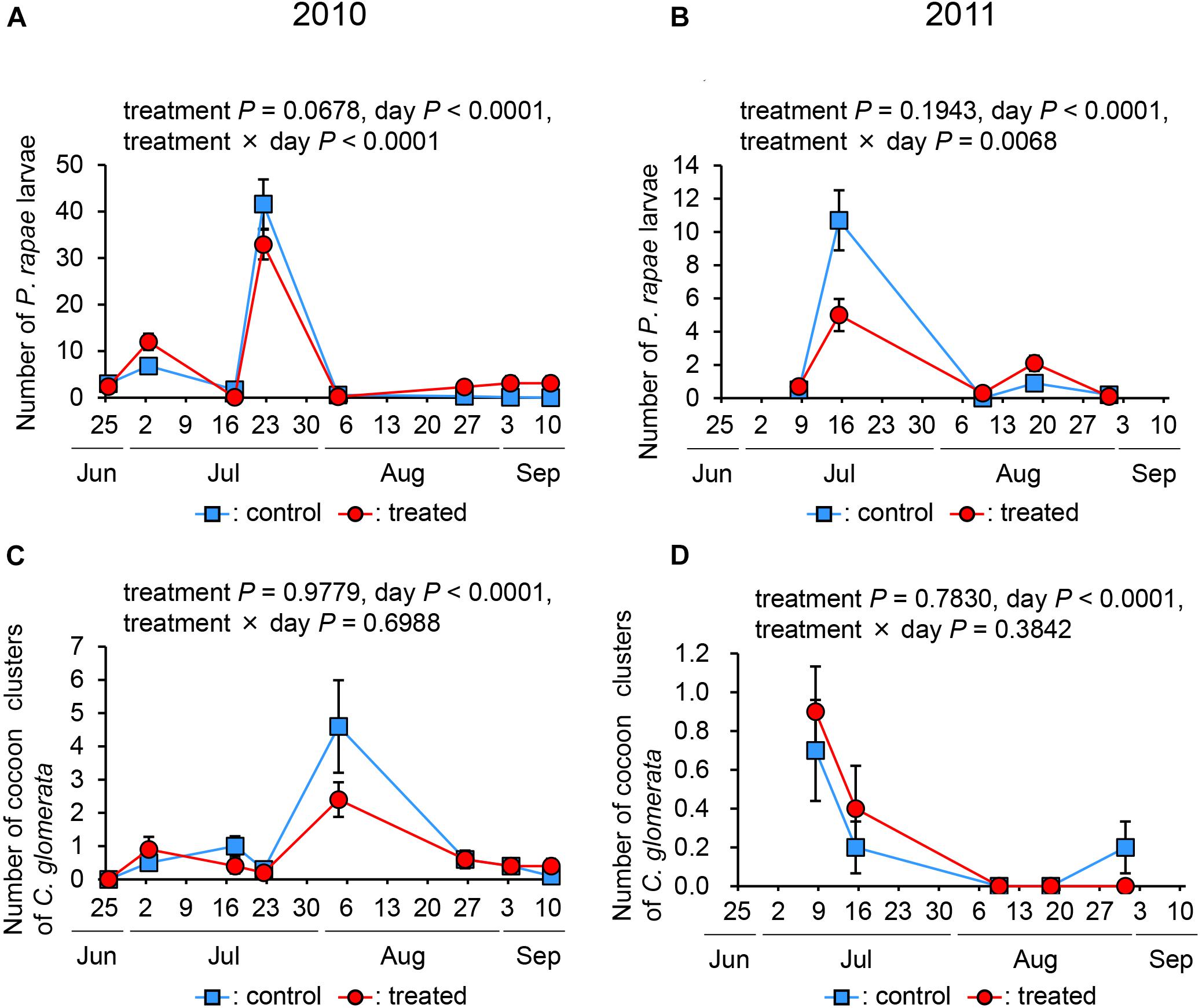
Figure 4. The number of Pieris rapae larvae (A,B) and Cotesia glomerata clusters of cocoons (C,D) in treated and control plots in 2010 and 2011.
Regarding the number of C. glomerata, the effects of treatment and day × treatment interaction in both years were not significant (in 2010, treatment: P = 0.9779, and interaction: P = 0.6988; in 2011, treatment: P = 07830, and interaction: P = 0.3842), while that of day was significant (P < 0.0001) (Figures 4C,D). These data indicate that the treatment did not affect the number of P. rapae larvae and C. glomerata.
Number of Plusiinae spp. Larvae
In both years, the effect of treatment was not significant (in 2010, P = 0.4056; in 2011, P = 0.0962), while that of day was significant (P < 0.0001 in both years). The effect of interaction in 2010 was significant (P = 0.0170), while that in 2011 was not (P = 0.1441) (Figure 5). We did not observe any natural enemies of Plusiinae spp. larvae. These data indicate that the treatment did not affect the number of Plusiinae spp. larvae.
Discussion
We set one yellow-coloured sugary-food feeder (Shimoda et al., 2014) in the treated plot in case there were insufficient sugary food sources (i.e., flowers) for adult C. vestalis in the surrounding environments during the experiments. In both years, however, sugary food sources in the surroundings were abundant throughout the experimental periods, and C. vestalis females visiting either the treated or control plots were, therefore, likely to be satiated. As satiated C. vestalis adults are not attracted to yellow colour (Kugimiya et al., 2010; Mitsunaga et al., 2012; Uefune et al., 2013b), it is unlikely that the presence or absence of the yellow-coloured feeder affected the number of C. vestalis recorded.
The number of DBM larvae in the control plot in 2011 was consistently low (approximately five larvae per plant), while in the control plot in 2010, an outbreak of DBM larvae was observed. It should be noted that insecticides were not used in either year. The difference in the number of DBM larvae in the control plots in 2010 and 2011 revealed the following two aspects of the treatment.
In 2010, we observed a high number of DBM larvae in the control plot, whereas in the treated plot, the number was consistently low, with a significant difference between the two plots. In the Poisson regression analyses, the effect of DBM number and the interaction of DBM with treatment (treatment × DBM number) were significant. This means that in the treated plot, the increase in the number of C. vestalis cocoons, along with an increase in the number of DBM larvae in the treated plot, was significantly higher compared to the control plot. C. vestalis females are attracted to the attractant under field conditions, and this attraction increases the incidence of parasitism by C. vestalis (Uefune et al., 2012). Thus, it is likely that more C. vestalis females are attracted from the surrounding area to the treated plot than to the control plot in a DBM-larvae density-dependent manner. This was also supported by the fact that the incidences of C. vestalis cocoons were the same between the control and treated plots, even though the incidence of DBM larvae was significantly different between the two plots. Taken together, in 2010, it is indicated that the attraction of C. vestalis to the dispensers in the treated plot contributed to the significant reduction in DBM larvae infestation in the plot.
In 2011, the number of DBM in both the control and treated plots was consistently low, with the effect of treatment not being significant. This finding is consistent with a previous report, which revealed the economic injury level of DBM larvae to be 5.0 per plant in cabbage production in Japan (Morishita, 1988). In the Poisson regression analysis, only the treatment factor was significant. This means that the number of C. vestalis cocoons in the treated plot was significantly higher than that in the control plot, irrespective of the number of DBM larvae. The results in 2011 indicate that a significantly higher number of C. vestalis is attracted to the treated plot by the dispensers. The results further indicate that when the densities of DBM larvae are around the economic injury level, the presence of the dispensers in the plot are unlikely to sufficiently reduce the number of DBM larvae to eliminate the risk of economic damage.
In both years, we also observed other arthropods such as aphids, mummies of aphids, P. rapae larvae, cocoons of C. glomerata, and Plusiinae spp. larvae. The incidence of aphids was not consistent in the 2 years. The incidences of other arthropods were not affected by the treatments. These results indicate that the treatment did not affect the presence of these herbivores and their respective natural enemies. Interestingly, segregation was observed between herbivore species in 2 years. In 2010, an increase in Plusiinae spp. larvae was observed; afterward, an increase in the number of the other herbivore species was observed for P. rapae larvae, DBM larvae, and aphids, in that order. In 2011, similar segregation was observed except in Plusiinae spp. larvae, the small number of which was constantly noticed during the observation period.
The attractant used in this study did not affect the oviposition behaviour and performance (pupation rate and pupal weight) of DBM adults/larvae when we set the dispenser next to two crucifer crops: mizuna plants, Brassica rapa var. laciniifolia (Uefune et al., 2017) and komatsuna plants, Brassica rapa var. perviridis (M. Uefune, unpublished data). However, in Cruciferae, Arabidopsis plants that have been exposed to either (E)-2-hexenal, (Z)-3-hexenal, (Z)-3-hexenol, n-octanol, or allo-ocimene induce several genes related to defence against herbivores and pathogens (Kishimoto et al., 2005). Yamauchi et al. (2018) reported that (Z)-3-hexenal, (Z)-3-hexenol, and (Z)-3-hexenyl acetate triggered the expression of defence-related genes in Arabidopsis. Green leaf volatiles and/or volatile terpenoids have also been shown to induce defence responses in maize, lima bean, citrus, tomato, poplar, and wild tobacco (Nicotiana attenuate) (Arimura et al., 2000; Gomi et al., 2003; Choh et al., 2004; Engelberth et al., 2004; Farag et al., 2005; Paschold et al., 2006; Frost et al., 2008; Sugimoto et al., 2014). Thus, the possibility that setting dispensers in the field affected the physiology of cruciferous crops remained.
This 2-year field study suggests that the dispensers recruited native C. vestalis from the surrounding environment to the treated plot. We also found that the dispensers controlled the number of DBM larvae in 2010 when the density of DBM larvae exceeded the economic injury levels for the cabbage crop. Although this study shows the possibility of the use the attractants for DBM control under open field conditions, additional research on the use of the attractant is needed to propose a general use to control DBM in cases where the surrounding landscape structure harbours both DBMs and C. vestalis (source), considering the size of cabbage fields (sink). Since DBM is one of the pests of cabbage and other cruciferous crops worldwide, applying the attractant in other areas is our future goal.
Data Availability Statement
The datasets for this study are included in the article/Supplementary Material.
Author Contributions
MU designed the experiments, conducted the experiments, analysed the data, and wrote the manuscript. KY conducted the experiments and analysed the data. MY conducted the experiments. JT analysed the data and wrote the manuscript. All authors have approved the final manuscript for publication.
Funding
This study was supported by grants for scientific research (A) [grant number 18H03952] from the Ministry of Education, Culture, Sports, Science and Technology and by Science and Engineering Entrepreneurship Development Program for Vigorous Researchers (SEED-V) from Japan Science and Technology Agency.
Conflict of Interest
The authors declare that the research was conducted in the absence of any commercial or financial relationships that could be construed as a potential conflict of interest.
Publisher’s Note
All claims expressed in this article are solely those of the authors and do not necessarily represent those of their affiliated organizations, or those of the publisher, the editors and the reviewers. Any product that may be evaluated in this article, or claim that may be made by its manufacturer, is not guaranteed or endorsed by the publisher.
Acknowledgments
We thank Kunihiro Tokumaru for finding the experimental field used in this study and Susumu Ueno for offering us his fields to use for the experiments. We also thank Editage (www.editage.com) for English language editing.
Supplementary Material
The Supplementary Material for this article can be found online at: https://www.frontiersin.org/articles/10.3389/fevo.2021.702314/full#supplementary-material
References
Abe, J., Uefune, M., Yoneya, K., Shiojiri, K., and Takabayashi, J. (2020). Synchronous occurrences of the diamondback moth (Lepidoptera: Plutellidae) and its parasitoid wasp Cotesia vestalis (Hymenoptera: Braconidae) in greenhouses in a satoyama area. Environ. Entomol. 49, 10–14. doi: 10.1093/ee/nvz140
Abe, J., Urano, S., Nagasaka, K., and Takabayashi, J. (2007). Release ratio of Cotesia vestalis to diamondback moth larvae (Plutella xylostella) that suppresses the population growth of diamondback moth feeding Brassica leaf vegetables in a greenhouse. Bullet. Nat. Agr. Res. Centr. West. Region (in Japanese with English summary) 6, 125–132. doi: 10.24514/00001624
Arimura, G., Matsui, K., and Takabayashi, J. (2009). Chemical and molecular ecology of herbivore-induced plant volatiles: proximate factors and their ultimate functions. Plant Cell Physiol. 50, 911–923. doi: 10.1093/pcp/pcp030
Arimura, G., Ozawa, R., Shimoda, T., Nishioka, T., Boland, W., and Takabayashi, J. (2000). Herbivory-induced volatiles elicit defence genes in lima bean leaves. Nature 6795, 512–515. doi: 10.1038/35020072
Choh, Y., Shimoda, T., Ozawa, R., Dicke, M., and Takabayashi, J. (2004). Exposure of lima bean leaves to volatiles from herbivore-induced conspecific plants results in emission of carnivore attractants: active or passive process? J. Chem. Ecol. 30, 1797–1808.
Engelberth, J., Alborn, H. T., Schmelz, E. A., and Tumlinson, J. H. (2004). Airborne signals prime plants against insect herbivore attack. Proc. Natl. Acad. Sci. U.S.A 101, 1781–1785. doi: 10.1073/pnas.0308037100
Farag, M. A., Fokar, M., Abd, H., Zhang, H., Allen, R. D., and Pare ì, P. W. (2005). (Z)-3-Hexenol induces defense genes and downstream metabolites in maize. Planta 220, 900–909. doi: 10.1007/s00425-004-1404-5
Frost, C. J., Mescher, M. C., Dervinis, C., Davis, J. M., Carlson, J. E., and De Moraes, C. M. (2008). Priming defense genes and metabolites in hybrid poplar by the green leaf volatile cis-3-hexenyl acetate. New Phytol. 180, 722–733. doi: 10.1111/j.1469-8137.2008.02599.x
Furlong, M. J., Wright, D. J., and Dosdall, L. M. (2013). Diamondback moth ecology and management: problems, progress, and prospects. Ann. Rev. Entomol. 58, 517–541. doi: 10.1146/annurev-ento-120811-153605
Gomi, K., Yamasaki, Y., Yamamoto, H., and Akimitsu, K. (2003). Characterization of a hydroperoxide lyase gene and effect of C6-volatiles on expression of genes of the oxylipin metabolism in Citrus. J. Plant Physiol. 160, 1219–1231. doi: 10.1078/0176-1617-01177
James, D. G. (2003a). Synthetic herbivore-induced plant volatiles as field attractants for beneficial insects. Environ. Entomol. 32, 977–982. doi: 10.1603/0046-225x-32.5.977
James, D. G. (2003b). Field evaluation of herbivore-induced plant volatiles as attractants for beneficial insects: methyl salicylate and the green lacewing, Chrysopa nigricornis. J. Chem. Ecol. 29, 1601–1609.
James, D. G. (2005). Further field evaluation of synthetic herbivore-induced plant volatiles as attractants for beneficial insects. J. Chem. Ecol. 31, 481–495. doi: 10.1007/s10886-005-2020-y
Kapran, I. (2012). Attracting carnivorous arthropods with plant volatiles: the future of biocontrol or playing with fire? Biol. Cont. 60, 77–89. doi: 10.1016/j.biocontrol.2011.10.017
Khan, Z. R., James, D. G., Midega, C. A. O., and Pickett, J. A. (2008). Chemical ecology and conservation biological control. Biol. Control 45, 210–224. doi: 10.1016/j.biocontrol.2007.11.009
Kishimoto, K., Matsui, K., Ozawa, R., and Takabayashi, J. (2005). Volatile C6-aldehydes and allo-ocimene activate defense genes and induce resistance against Botrytis cinerea in Arabidopsis thaliana. Plant Cell Physiol. 46, 1093–1102. doi: 10.1093/pcp/pci122
Kugimiya, S., Uefune, M., Sano, K., and Takabayashi, J. (2017). A device to disperse synthetic herbivore-induced plant volatiles that attract natural enemies of herbivores in the field for pest control. Acta Hortic. 1169, 113–118. doi: 10.17660/ActaHortic.2017.1169.17
Kugimiya, S., Uefune, M., Shimoda, T., and Takabayashi, J. (2010). Orientation of the parasitic wasp, Cotesia vestalis (Haliday) (Hymenoptera: Braconidae), to visual and olfactory cues of field mustard flowers, Brassica rapae L. (Brassicaceae), to exploit food sources. Appl. Entomol. Zool. 45, 369–375. doi: 10.1303/aez.2010.369
McCormick, A. C., Unsicker, S. B., and Gershenzon, J. (2012). The specificity of herbivore-induced plant volatiles in attracting herbivore enemies. Trends Plant Sci. 17, 303–310. doi: 10.1016/j.tplants.2012.03.012
Mitsunaga, T., Shimoda, T., Mukawa, S., Kobori, Y., Goto, C., Suzuki, Y., et al. (2012). Color and height influence the effectiveness of an artificial feeding site for a larval endoparasitoid, Cotesia vestalis (Haliday) (Hymenoptera, Braconidae). Jpn. Agric. Res. Quart. 46, 161–166. doi: 10.6090/jarq.46.161
Morishita, M. (1988). Tolerable larval density of diamondback moth (Plutella xylostella L.) in cabbage. Proc. Kansai Plant Prot. (in Japanese with English summary) 40, 77–81. doi: 10.4165/kapps1958.40.0_77
Ohara, Y., Uchida, T., Kakibuchi, K., Uefune, M., and Takabayashi, J. (2017). Effects of an artificial blend of host-infested plant volatiles on plant attractiveness to specialist parasitic wasps. J. Appl. Entomol. 141, 231–234. doi: 10.1111/jen.12328
Pappas, M. L., Broekgaarden, C., Broufas, G. D., Kant, M. R., Messelink, G. J., Steppuhn, A., et al. (2017). Induced plant defences in biological control of arthropod pests: a double-edged sword. Pest Manage. Sci. 73, 1780–1788. doi: 10.1002/ps.4587
Paschold, A., Halitschke, R., and Baldwin, I. T. (2006). Using ‘mute’ plants to translate volatile signals. Plant J. 45, 275–291. doi: 10.1111/j.1365-313X.2005.02623.x
Shimoda, T., Mitsunaga, T., Uefune, M., Abe, J., Kugimiya, S., Nagasaka, K., et al. (2014). A food-supply device for maintaining Cotesia vestalis, a larval parasitoid of the diamondback moth Plutella xylostella, in greenhouses. BioControl 59, 681–688. doi: 10.1007/s10526-014-9611-x
Shiojiri, K., Ozawa, R., Kugimiya, S., Uefune, M., van Wijk, M., Sabelis, M., et al. (2010). Herbivore-specific, density-dependent induction of plant volatiles: honest or “Cry Wolf” signals? PLoS One 5:e12161. doi: 10.1371/journal.pone.0012161
Shiojiri, K., Takabayashi, J., Yano, S., and Takafuji, A. (2000). Flight response of parasitoid toward plant-herbivore complexes: a comparative study of two parasitoid-herbivore systems on cabbage plants. Appl. Entomol. Zool. 35, 87–92. doi: 10.1303/aez.2000.87
Sugimoto, K., Matsui, K., Iijima, Y., Akakabe, Y., Muramoto, S., Ozawa, R., et al. (2014). Intake and transformation to a glycoside of (Z)-3-hexenol from infested neighbors reveals a mode of plant odor reception and defense. Proc. Nat. Acad. Sci. U.S.A. 111, 7144–7149. doi: 10.1073/pnas.1320660111
Takabayashi, J., and Dicke, M. (1996). Plant-carnivore mutualism through herbivore-induced carnivore attractants. Trends Plant Sci. 1, 109–113. doi: 10.1016/S1360-1385(96)90004-7
Takabayashi, J., and Shiojiri, K. (2019). Multifunctionality of herbivory-induced plant volatiles in chemical information transmission during tritrophic interactions among plants, herbivorous arthropods, and arthropod predators. Curr. Opin. Insect Sci. 32, 110–117. doi: 10.1016/j.cois.2019.01.003
Talekar, N. S., and Shelton, A. M. (1993). Biology, ecology, and management of the diamondback moth. Ann. Rev. Entomol. 38, 275–301. doi: 10.1146/annurev.en.38.010193.001423
Talekar, N. S., and Yang, J. C. (1993). Influence of crucifer cropping system on the parasitism of Plutella xylostella (Lep., Yponomeutidae) by Cotesia plutelae (Hym., Braconidae) and Diadegma semiclausum (Hym., Ichneumonidae. Entomophaga 38, 541–550. doi: 10.1007/BF02373089
Turlings, T. C. J., and Erb, M. (2018). Tritrophic interactions mediated by herbivore-induced plant volatiles: mechanisms, ecological relevance, and application potential. Ann. Rev. Entomol. 63, 433–452. doi: 10.1146/annurev-ento-020117-043507
Uefune, M., Abe, J., Urano, S., Nagasaka, K., and Takabayashi, J. (2020). Targeting diamondback moth in greenhouses by attracting specific native parasitoids with herbivory-induced plant volatiles. Royal Soc. Open Sci. 7:201592. doi: 10.1098/rsos.201592
Uefune, M., Choh, Y., Abe, J., Shiojiri, K., Sano, K., and Takabayashi, J. (2012). Application of synthetic herbivore-induced plant volatiles causes increased parasitism of herbivores in the field. J. Appl. Entomol. 136, 561–567. doi: 10.1111/j.1439-0418.2011.01687.x
Uefune, M., Kugimiya, S., Ozawa, R., and Takabayashi, J. (2013a). Parasitic wasp females are attracted to blends of host-induced plant volatiles: do qualitative and quantitative differences in the blend matter? [version 2; peer review: 2 approved]. F1000Res. 2013:57. doi: 10.12688/f1000research.2-57.v2
Uefune, M., Kugimiya, S., Shimoda, T., and Takabayashi, J. (2013b). Starvation and herbivore-induced plant volatiles affect the color preferences of parasitic wasps. BioControl 58, 187–193. doi: 10.1007/s10526-012-9483-x
Uefune, M., Shiojiri, K., and Takabayashi, J. (2017). Oviposition of diamondback moth Plutella xylostella females is affected by herbivore-induced plant volatiles that attract the larval parasitoid Cotesia vestalis. Arthropod Plant Interact. 11, 235–239. doi: 10.1007/s11829-016-9484-2
Yamauchi, Y., Matsuda, A., Matsuura, N., Mizutani, M., and Sugimoto, Y. (2018). Transcriptome analysis of Arabidopsis thaliana treated with green leaf volatiles: possible role of green leaf volatiles as self-made damage-associated molecular patterns. J. Pestic. Sci. 43, 207–213. doi: 10.1584/jpestics.D18-020
Keywords: herbivory-induced plant volatiles, synthetic attractants, Plutella xylostella, diamondback moth, Cotesia vestalis, cabbage plants, field study
Citation: Uefune M, Yoneya K, Yamamoto M and Takabayashi J (2021) The Use of Synthetic Herbivory-Induced Plant Volatiles That Attract Specialist Parasitoid Wasps, Cotesia vestalis, for Controlling the Incidence of Diamondback Moth Larvae in Open Agricultural Fields. Front. Ecol. Evol. 9:702314. doi: 10.3389/fevo.2021.702314
Received: 29 April 2021; Accepted: 30 June 2021;
Published: 23 July 2021.
Edited by:
Stefano Colazza, University of Palermo, ItalyReviewed by:
Betty Benrey, Université de Neuchâtel, SwitzerlandMaria Carolina Blassioli Moraes, Brazilian Agricultural Research Corporation (EMBRAPA), Brazil
Copyright © 2021 Uefune, Yoneya, Yamamoto and Takabayashi. This is an open-access article distributed under the terms of the Creative Commons Attribution License (CC BY). The use, distribution or reproduction in other forums is permitted, provided the original author(s) and the copyright owner(s) are credited and that the original publication in this journal is cited, in accordance with accepted academic practice. No use, distribution or reproduction is permitted which does not comply with these terms.
*Correspondence: Masayoshi Uefune, bXVlZnVuZUBtZWlqby11LmFjLmpw; Junji Takabayashi, dGFrYWJheWFzaGkuanVuamkuNGFAa3lvdG8tdS5hYy5qcA==
†Present address: Masaki Yamamoto, Ministry of Agriculture, Forestry and Fisheries, Tokyo, Japan