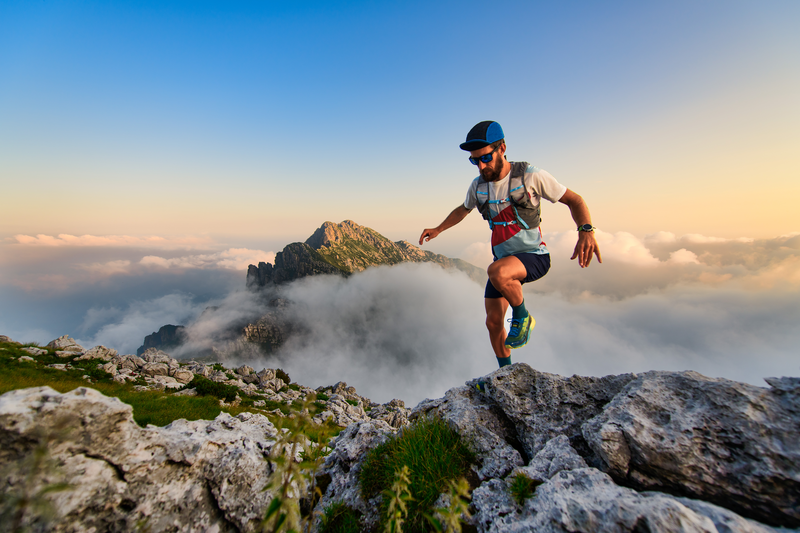
94% of researchers rate our articles as excellent or good
Learn more about the work of our research integrity team to safeguard the quality of each article we publish.
Find out more
ORIGINAL RESEARCH article
Front. Ecol. Evol. , 29 June 2021
Sec. Behavioral and Evolutionary Ecology
Volume 9 - 2021 | https://doi.org/10.3389/fevo.2021.699917
This article is part of the Research Topic Ecophysiological Adaptations Associated with Animal Migration View all 15 articles
Arctic migration presents unique challenges to circadian physiology. In addition to the metabolic cost of maintaining a relatively high body temperature (Tb) above ambient temperature, migratory birds are also exposed to rapidly changing light conditions as they transition between light-dark cycles and a 24-hour polar day. A previous study suggested that Arctic-migratory barnacle geese (Branta leucopsis) may utilise adaptive heterothermy (i.e., a controlled decrease in core Tb) during and around the autumn migratory period in order to minimise the metabolic cost of migration, but the impact of seasonally changing daylight conditions on other parameters of the circadian profile of Tb in these geese remained obscure. Here, we provide a detailed comparative analysis on the circadian rhythm of Tb and its seasonal development in free-living barnacle geese from three study populations that differ in their migratory behaviour and in the environments they occupy. We recorded abdominal Tb in non-migratory geese from a temperate breeding colony in Netherlands and in migratory geese from a colony in the Russian low Arctic, and analysed these data together with previously published Tb data on geese from a migratory colony in the high Arctic of Svalbard. We found that the circadian Tb profile in the barnacle goose was well aligned with the daily and seasonally changing daylight conditions. In the migratory populations, a fast re-entrainment of the rhythm and its phase was observed when zeitgeber conditions changed during migratory movements. The circadian rhythmicity of Tb was lost once the geese encountered permanent daylight at their northern staging and breeding sites. Circadian Tb rhythmicity was re-established when the period of permanent daylight ended, at rates corresponding to rates of seasonal changes in daylength in the high and low Arctic. Although our data corroborated findings of a decrease in daily mean Tb before autumn migration in both migratory populations in this study, the pre-migratory decrease in Tb was less drastic than previously reported. Moreover, in contrast to previous study, the decrease in Tb stopped at the onset of migration. Overall, our data reveal no evidence that heterothermy in the barnacle goose is functionally linked to migration.
Endotherms, such as birds and mammals, actively regulate their body temperature (Tb), enabling them to perform their activities largely independent of temporal and spatial variation in ambient temperature. As a result, endotherms generally inhabit broader regions and more diverse habitats than ectotherms (Gaston, 2003). In birds, a vivid expression of the independence from environmental constraints that can be gained from thermal independence is long-distance migration over large environmental gradients, such as between Arctic breeding grounds and southern wintering sites. Animal migration has evolved in many species as an adaptation that enables them to exploit spatially distant seasonal peaks of resource abundance and avoid resource depression (Alerstam et al., 2003; Holt and Fryxell, 2011).
When travelling to Arctic breeding grounds, fast-moving animals such as birds experience rapid changes in light conditions along the route, and finally arrive into a 24-h polar day. These rapid changes in light conditions present a unique challenge in the context of circadian physiology (Gwinner and Brandstätter, 2001). Circadian rhythms refer to the coordinated, endogenously driven, 24-h patterns of physiology and behaviour, such as activity and Tb, that improve the adaptability of an organism by allowing it to anticipate predictable changes in the environment (Dunlap et al., 2004). The daily cycle of light and darkness, due to the rotation of the earth around its axis, is one of the most predicable changes in the environment. Accordingly, light is the most salient zeitgeber, or entrainer, of circadian rhythms for most surface-dwelling organisms, including birds (Golombek and Rosenstein, 2010; Cassone, 2014). When an organism with a circadian rhythm is exposed to a change in the timing of a zeitgeber, like light, there is a temporary mismatch between the internal state of the animal, and the environment in which it is performing. Over time, the internal rhythm adjusts to this disruption, and eventually alignment is restored. However, cognitive performance may be less than optimal during realignment (Tapp and Natelson, 1989). Temporal mismatches between behaviour and environment can lead to a higher risk of being predated (DeCoursey et al., 2000), or alter metabolic homeostasis (Fatima and Rana, 2020). As such, maintaining a degree of plasticity in circadian timekeeping is likely advantageous for Arctic-migrating species (Helm et al., 2017; Åkesson et al., 2021).
Performing migratory journeys requires both time and energy. To fuel their migratory flights, birds deposit and replenish body stores (mainly fat and protein) by foraging intensively during the pre-migratory period and at stopover sites (Bairlein, 2003; Drent et al., 2007; Eichhorn et al., 2012). The rate of fuelling is a key factor for the overall speed of migration, thereby affecting the prospects for an individual’s fitness (Alerstam and Lindström, 1990; Gómez et al., 2017). Because the maintenance of a high and relatively stable Tb is energetically costly for endotherms when facing ambient conditions outside their thermoneutral zone, a potential mechanism that may positively influence the net (re)fuelling rate is a less robust defence of Tb against the cold, thus saving the energy that would be expended on heat production (Geiser and Brigham, 2012). Such adaptive heterothermy, which refers to the abandonment of homeothermy in favour of reducing metabolic costs and improving fuel economy, has been suggested for migrating bats and hummingbirds using torpor (Hiebert, 1993; McGuire et al., 2014). But shallower heterothermy was observed also in a songbird (i.e., blackcap Sylvia atricapilla) during nocturnal rest at a migratory stopover (Wojciechowski and Pinshow, 2009), and in the barnacle goose Branta leucopsis during autumn migration from Svalbard to wintering grounds in Scotland (Butler and Woakes, 2001).
The latter example is intriguing, because Tb decreased during both the resting phase and the active phase of the diel cycle. Together, those decreases caused a progressive decline in mean abdominal temperature of more than 4°C from few days before until approximately 20 days after the start of the autumn migration. Thus, heterothermy persisted throughout and for several days after the end of migration (Butler and Woakes, 2001). The energy savings that result from this heterothermic response may significantly influence the economy of fat stores during and after migration. Such pronounced heterothermy is remarkable for such a large bird especially during a period when exercise is such an important aspect of daily life (McKechnie and Lovegrove, 2002; Eichhorn et al., 2011). For example, in non-migratory greylag geese Anser anser, seasonal differences in mean Tb throughout the entire annual cycle (lowest in winter and peaking in summer) were only 1°C (Wascher et al., 2018).
For our analyses in this paper, we took advantage of naturally occurring variations in the migratory ecology of free-living barnacle geese. Specifically, we recorded abdominal Tb in non-migratory geese from a temperate breeding colony in the Netherlands, and in migratory geese from a colony in the Russian low Arctic. To re-examine previous findings of seasonal heterothermy reported for this species (Butler and Woakes, 2001), we also included in our analysis previously published Tb data from migratory geese breeding in the high Arctic of Svalbard (Portugal et al., 2019). Geese from the Russian and Dutch study colonies share common wintering grounds stretching along the mainland coast of the North Sea and southwestern Baltic. However, the geese from the Dutch colony generally stay in the Netherlands year-round. Barnacle geese from the Russian colony migrate from their wintering grounds via potential stopovers in the Baltic, and further a cross the boreal belt to reach Arctic staging sites in the White Sea (e.g., Dvina river delta and Kanin Peninsula). Approximately 2–3 weeks later, they reach breeding sites further northeast along the coast of the Barents Sea, including our study site at Tobseda (Eichhorn, 2005; Eichhorn et al., 2006). Traditionally, the time between mass departure from the wintering grounds (mid-April) and arrival at Arctic staging sites with continuous daylight conditions (after mid-May) is approximately 1 month, because of prolonged staging in the Baltic. However, since the 1990s, an increasing number of barnacle geese heading for the Russian Arctic have delayed their departure from wintering grounds until well into May. Individuals that follow this new strategy do not arrive later in the Arctic, but shorten, or entirely skip, staging in the Baltic, thereby experiencing changes in daylight conditions more rapidly as they move to the Arctic (Eichhorn et al., 2009; Jonker et al., 2010). By providing a detailed comparative analysis on the circadian and seasonally patterns of Tb in these three populations, we attempt to shed more light on chronobiological aspects in this Arctic migratory bird as well as on the question of whether heterothermy and migration might be functionally linked.
We studied migratory barnacle geese breeding in the Russian low Arctic and non-migratory geese breeding in the Netherlands. Additionally, we retrieved published data from migratory barnacle geese breeding in high Arctic Svalbard. The latter consist of data on abdominal body temperature (Tb) and heart rate of geese studied in a colony at Ny-Ålesund (78°55′N, 11°56′E) between summer 1999 (implantation of recording devices) and summer 2000 (retrieval). The original Tb and heart rate data were recorded continuously at a sampling rate of 5 s in that study, however, the data we accessed had 15-min averages, available from five individuals (Portugal et al., 2019). Following Portugal et al. (2012), periods of migratory flight were determined from heart rate. For data and further details we refer to Portugal et al. (2019) and references therein. Svalbard barnacle geese spend the winter in the Solway Firth, Scotland (United Kingdom). They leave their winter grounds from mid-April onward for a prolonged staging period along the Norwegian coast (mainly in the Helgeland district) before they travel further to reach Svalbard in the second half of May (Black et al., 2007; Shariati-Najafabadi et al., 2016). The geese leave the Svalbard archipelago again toward the end of September, often after a stopover on Bjørnøya (Bear Island), and fly again along the Norwegian coast but, in contrast to spring, make only brief stops and arrive back in their winter grounds at the end of September or early October (Butler et al., 1998). In Russia, we studied barnacle geese in a colony at Kolokolkova Bay near the abandoned settlement of Tobseda (68°35′N, 52°20′E), situated along the coast of the Barents Sea. Barnacle geese from a non-migratory population were studied in a colony in the Delta area in the southwest of the Netherlands (51°40′N, 4°14′E). More details on these study sites and populations can be found in Van der Jeugd et al. (2003, 2009) and references therein. For a map showing the routes of barnacle geese tracked to their breeding grounds in Svalbard and Russia see Shariati-Najafabadi et al. (2016).
In our Russian and Dutch study sites, geese were captured during their post-breeding wing moult in summer 2018, by driving the flightless geese into a catching pen. We selected adult female geese, which were identified by cloacal examination (sex) and the absence of any (not yet moulted) juvenile feathers. Hence the birds were most likely mature birds in their third calendar year or older (Cramp and Simmons, 1977). Additionally, we selected one immature female goose (CHL0289) that was born and ringed in the Netherlands (NL) in 2017. Selected geese (21 in Russia [RUS], 15 in NL) were instrumented with a solar-powered tracking device (36 × 70 × 15 mm, 24 g) placed on the back by a harness (16 g) recording GPS location and 3D accelerometry (GsmRadioTag, Milsar Technologies S.R.L.), and an implantable data logger (46 × 15 mm, 19 g) recording Tb and heart rate (DST centi-HRT, Star-Oddi). Tb was measured at ±0.2°C accuracy, and all loggers were calibrated by the manufacturer at 41 setpoints within the range of 5 to 45°C. The geese were also banded with individually inscribed darvic leg-rings to allow recognition in the field. Total instrument mass equated to 3% of average body mass. The sterilised data logger was implanted into the abdominal cavity via mid-line incision in skin and body wall while the bird was under isoflurane gas anaesthesia. Using silk suture, the logger was fixed at one end to the cartilage of the xiphoid process, and at the other end to the body wall. Dissolvable suture was used to close the muscle layers and skin. After surgery, the birds were monitored for at least 4 h while they were kept in an outdoor pen, before they were released back to the wild together with others in a small flock.
The data loggers were programmed to record Tb continuously every 10 min, except during the following periods when the interval was reduced to 5 min: from 20 September to 22 October 2018 (both NL and RUS geese) and again from 7 April to 23 June 2019 (NL geese) or 27 April to 13 July 2019 (RUS geese). Attempts to recapture the geese for retrieval of loggers were made in the Russian study site during wing-moult in summer 2019, and in the Dutch study site during summer 2019 and 2020. Unfortunately, most of the GPS trackers stopped working at an early phase of the study, which hindered the detection and recapture of study subjects, particularly at the Russian site. Recaptured focal geese were euthanised with an overdose of pentobarbital (Euthesate) injected intravenously, and all instruments were removed. Some data loggers were also sent in by hunters, who shot geese during their regular hunting activities, thereby retrieving loggers from one RUS goose and three NL geese. In total we gathered Tb data of various recording length from three RUS geese and 11 NL geese, of which three (RUS) and eight (NL) recordings were included in the present analysis (other NL geese had too short recordings as they were shot by hunters within few weeks of implantation).
Daily ambient mean, minimum and maximum temperature measured at meteorological stations in Ny Ålesund (breeding site of geese studied in Svalbard), Eskdalemuir (20 km from the Caerlaverock nature reserve in Scotland, the principal wintering site for Svalbard barnacle geese), Naryan-Mar (103 km from the Russian study colony), and Wilhelminadorp (33 km from the Dutch study colony) were retrieved from the European Climate Assessment and Dataset at http://www.ecad.eu (Klein Tank et al., 2002). For the computation of sunlight phases we used the R-package “suncalc” (Thieurmel and Elmarhraoui, 2019). Civil twilight refers to sun angles between 0° (i.e., sunset or sunrise) and −6°, and nautical twilight to sun angles between −6° and −12°.
Periodicity in the Tb data was determined using Lomb-Scargle periodogram analysis as implemented in the “lomb” package in RStudio (R version 4.0.4 for Windows) (Ruf, 1999; RStudio Team., 2020; R Core Team., 2021). Five-day blocks of data were scanned at an oversampling rate of 200 to detect significant (p < 0.001) periodicity between 6 to 30 h in duration. Because the normalised power (PN) is sensitive to sampling rate of the Tb time series, data were re-sampled at 30 min interval to ensure consistency where comparisons were made between study populations that were sampled at a different time interval. Otherwise, the native sampling intervals were used. Periodogram analyses confirmed that, when rhythmic, the Tb data typically contained significant periods at 24 h and occasionally at 12 h (Supplementary Figure 1). Accordingly, we used the “cosinor” (Sachs, 2014) and “card” (Shah, 2020) packages in RStudio (R version 4.0.4 for Windows) to fit multicomponent cosinor models to the daily Tb data, comprising of a 24 h period and a 12 h harmonic. The multicomponent model is described by the following formula:
Here, M refers to the midline estimation statistic of rhythm (MESOR), A1 and A2 are variables defining amplitude, φ1 and φ2 are variables describing phase, and t is time in hours. To describe the circadian profile, the MESOR, peak, trough, and global amplitude, defined as half of the distance between the peak and the trough of an oscillation, were extracted from the model. Since the data were sampled at equidistant intervals and an integer number of cycles were analysed, the MESOR is equal to the arithmetic mean (Refinetti et al., 2007). In addition to the PN, we also used the coefficient of determination (R2) multiplied by 100 as a measure of the relative robustness of the circadian rhythm, herein referred to as percent rhythm (Refinetti et al., 2007).
We used the daily acrophase, defined as the timing of the daily maximum, to infer the timing of the Tb rhythm and thereby examine entrainment of the circadian rhythm. To focus on the timing of the circadian (i.e., 24 h) rhythm and avoid the confounding effects of the 12 h harmonic, we extracted the acrophase from the following single component cosinor, instead of the multicomponent cosinor model:
As above, M refers to the MESOR, A is a variable defining the amplitude, φ is a variable describing phase, and t is time in hours. Supplementary Figure 2 shows examples of the multicomponent and single-component models. Linear mixed effects analysis of the relationship between acrophase and stage of migration were performed using R and the “lme4” package (Bates et al., 2015) with stage of migration entered as a fixed effect, and individual ID as random effect. Visual inspection of residual plots revealed no heteroscedasticity or deviations from normality. The focus of our present analyses was on the circadian and seasonal Tb pattern related to different migration strategies and breeding environments. We deliberately excluded the breeding period itself to avoid additional interference from incubation rhythms.
Two-way mixed ANOVA (as implemented in IBM SPSS, Version 25.0) was used to evaluate differences in the daily mean Tb among the three goose populations (between-subjects factor) across three points in time/state around autumn migration (within-subjects factor), which allowed also to test for an interaction, i.e., to evaluate if any changes in Tb over time differed among populations. Data were log-transformed before analysis to improve distributional properties (normality, variance). Normality was assumed based on Shapiro-Wilk tests (all tests yielded P > 0.05). Homogeneity of variances was assumed based on Levene’s test using the median, because of skews or uncertainty in distribution (all tests yielded P > 0.05). Homogeneity of covariances was assumed based on Box’s M-test (yielding P > 0.001); a lower P-value is justified because of the high sensitivity of this test (Tabachnick et al., 2019). Sphericity was assumed based on Mauchly’s test of sphericity (yielding P > 0.05). Sample size in the data set was low, but multivariate analyses of repeated measures ANOVAs seem robust even when performed with sample sizes as low as three as long as data are normally distributed (Oberfeld and Franke, 2013). Based on a significant interaction in the mixed ANOVA, we followed up by running tests for single (main) effects (i.e., one-way models testing the effect of one factor at each level of the other factor). If a single main effect was significant, multiple pairwise comparisons were run to determine which groups were different. A Sidak correction was applied in multiple (pairwise) comparisons. Effects are reported as significant at P < 0.05.
Variation of Tb in the barnacle goose was well aligned to the daily and seasonal daylight conditions (i.e., light-dark cycle of the day) in areas and seasons with a day-night cycle (Figure 1, Supplementary Figure 3). Tb rose sharply around dawn and declined sharply around dusk, resulting in a robust and prominent circadian (24 h) rhythmicity with a mean acrophase of 13.6 ± 2.2 h (mean ± SD) when geese were in their wintering grounds (between November and March). Strong and rapid changes in the acrophase occurred during migratory movements and when the birds experienced continuous daylight conditions (Figure 2). The Tb rhythm and its acrophase was quickly adjusted to phase shifts in diel light conditions resulting from longitudinal movements, which was most apparent during the autumn migration when the birds experienced diel light-dark cycles throughout (Figures 1, 2; Supplementary Figure 3).
Figure 1. Examples of diel (vertical axis, in hours) and seasonal body temperature (Tb, in °C) patterns in individual barnacle geese (ID given on the right of each plot) from the Arctic-migratory populations (A: Svalbard, B: Russia) and the non-migratory population (D: Netherlands). Panel C refers to an immature individual (CHL0289; born in NL in 2017) that flew in spring 2019 to Estonia. Vertical bars at the start and end of the Tb plots indicate the period of daylight, including civil twilight (white) and night (black) in the wintering and breeding areas (SCO, Scotland; SVA, Svalbard; NL, Netherlands; RUS, Russia; EST, Estonia). Time of day refers to GMT in all panels. Migratory movements are indicated by triangles; if the triangles are connected by dashed lines, then migratory activity was recorded throughout this period. See also Supplementary Figure 3 for further examples.
Figure 2. Daily acrophase of individual Tb rhythms over spring and autumn in barnacle geese from the Svalbard (SVA) and Russian (RUS) populations (individual ID is given on top of each plot). Included is also goose CHL0289 from the Netherlands population (NL) that migrated to Estonia (EST) in the Baltic in spring. The migration period was determined from heart rate (SVA) or GPS locations (RUS, NL), and the 14 days immediately preceding and following migration are plotted as the pre-and post-migratory periods. Data from days where the migratory stage of the bird could not be inferred from either heart rate or GPS are plotted in grey. Open circles in the panel for CHL0269 refer to a period when the bird was located at a staging site (Kanin Peninsula) southwest of the breeding site. Model fits from linear mixed effects analysis of acrophase during each stage of migration are shown as horizontal lines.
The rhythmicity of Tb was lost in the migratory geese once they encountered continuous daylight conditions at their northern staging and breeding sites (Figure 3). After the period of continuous daylight had ended, rhythmicity resumed (Supplementary Figure 1), however, at a different pace in the high and low Arctic (Figure 4). In Svalbard, continuous daylight lasts longer throughout the season and, accordingly, the geese remained arrhythmic for longer. Virtually with the first day of sunset and the return of civil twilight on 28 August, circadian rhythmicity resumed. In contrast, at the Russian site, the first sunset was on 21 July, but circadian rhythmicity of Tb was resumed only after approximately 20 August, when the geese experienced already several hours of civil and nautical twilight. Geese in Netherlands generally showed stronger Tb rhythmicity than geese in the Arctic summer, although rhythmicity increased also in the Dutch geese toward the end of the temperate summer.
Figure 3. Course of circadian rhythmicity of Tb in barnacle geese during spring, encompassing the time before, during, and after migration to their Arctic breeding grounds in Svalbard (SVA) and Russia (RUS). Plotted are percent rhythm (mean + SD over individuals, blue symbols) calculated from daily cosinor analysis, and normalized power (PN, mean + SD over individuals, orange symbols) at a period length of 24 h calculated from Lomb-Scargle periodogram analyses on 5-day intervals (both left axis).
Figure 4. Course of local photoperiod and circadian rhythmicity of Tb in barnacle geese at the end of summer while staying in Svalbard (SVA), in Russia (RUS), or in the Netherlands (NL). Plotted are percent rhythm (mean + SD over individuals, blue symbols) calculated from daily cosinor analysis, and normalized power (PN, mean + SD over individuals, orange symbols) at a period length of 24 h calculated from Lomb-Scargle periodogram analyses on 5-day intervals (both left axis). The amount of time the sun was at certain angles below horizon (right axis) is shown by shades of grey representing categories of, respectively, civil twilight, nautical twilight, and darker.
A visual inspection of the individual traces shown in Figure 5 reveals that geese from all three populations exhibited a gradual decline in the daily mean of Tb throughout early autumn. In the non-migratory geese, the decline came to a halt in the 2nd week of October. In the migratory geese, the decline stopped with the onset of migration. Most geese departed when ambient temperatures fell to or below 0°C (i.e., when frost first occurred). Tb increased again during migration, albeit to a lower level than had been observed in summer. The increase happened most rapidly in Svalbard geese, which completed the migratory journey within a short period. Once the geese arrived at their wintering grounds or pre-wintering staging sites, the Tb remained relatively stable.
Figure 5. Daily Tb (left axis) of barnacle geese before, during, and after their autumn migration from Svalbard (SVA) to the United Kingdom, from Russia (RUS) to (pre-) wintering grounds in the SW Baltic and Wadden Sea, and of non-migratory individuals in the Netherlands (NL). Shown is the MESOR Tb as modelled from a multi-component cosinor analysis, which equated to daily mean Tb in these data. Migratory movements are indicated by triangles matching in colour with the individual Tb series; if the triangles are connected by dashed lines, then migratory activity was recorded throughout this period. The timing of movements was derived from individual heart rate (SVA) or GPS tracking (RUS); in one case (open triangles) it was estimated from obvious phase shifts observed in Tb data (Figure 1). The grey line and the borders of the grey shaded area surrounding it refer to daily mean, maximum, and minimum ambient temperature (right axis) as measured in the breeding area before most individuals departed; for Svalbard geese (with a condensed migratory period) ambient temperature is also shown in their Scottish wintering area.
Any between-individual comparison of absolute Tb values is hampered by the nature of individual measurement variation that cannot be accounted for (e.g., differences in the exact position of the temperature probe). However, within-individual variation throughout autumn appeared higher in geese from Svalbard than in geese from Russia or Netherlands (Figure 5). To summarise and formally test for Tb patterns observed around autumn migration, we selected the day with the lowest daily mean Tb recorded during 21 September to 21 October (thereby effectively aligning the individual time series to a common “onset of migration” in migratory geese) and compared that time to a premigration summer mean Tb recorded during 10 to 20 August (when both migratory populations were still arrhythmic) and to a postmigration mean Tb recorded during 23 October to 15 November. The intra-individual decline in the daily mean Tb from summer to autumn amounted to, on average, 1.4°C in geese from Svalbard, and 1.0°C in geese from Russia and Netherlands (Table 1, Supplementary Figure 4). While there was an overall significant change in Tb across the three points in time / state (two-way mixed ANOVA F2,26 = 200.60, P < 0.001), this change differed among populations (time × population interaction, F4,26 = 6.83, P = 0.001). Contrasts revealed that the interaction was significant when “autumn low Tb” and “summer mean Tb” (F2,13 = 10.41, P = 0.002) were compared, but not when “autumn low Tb” and “postmigration mean Tb” (F2,13 = 3.61, P = 0.057) were compared. In other words, geese in Svalbard exhibited a stronger autumn decline in Tb than did geese in Russia or Netherlands (Supplementary Figure 4). The overall main effect of population was significant (F2,13 = 5.20, P = 0.022) due to generally lower Tb values in Svalbard geese than in geese from our study. One-way models and post-hoc tests indicated that, for each population, Tb differed in all comparisons among the three points in time / state (P ≤ 0.014) except for the comparison of mean Tb in summer and after migration in Russian geese (P = 0.064). The daily mean Tb in the Russian geese did not differ from that in Netherlands geese at any of the three points in time / state (P ≥ 0.968).
Table 1. Daily mean body temperature (Tb, in °C) in individual barnacle geese from Arctic-migratory populations in Svalbard (SVA) and Russia (RUS), and from a non-migratory population in Netherlands (NL) at three time periods / states around autumn migration.
The decline in mean (MESOR) Tb was attributable mainly to a deeper trough of Tb during the resting phase of the diel rhythm, and much less to a change in the peak Tb, resulting in an increase in the amplitude of the rhythm (Supplementary Figures 5, 6). However, in the Arctic, the peak Tb also showed a slight decline leading up to the onset of migration, followed by a more noticeable rebound thereafter.
We investigated the Tb patterns of barnacle geese from three study populations, which varied in their migratory strategy and also in the environments they occupied, most notably in solar light regime. Typical for a (largely) diurnally active species, Tb levels were higher during the light phase, and lower during the dark phase, of the diel period. The geese showed a robust circadian Tb rhythm, reflected by increased percent rhythm and normalised power corresponding to a 24-h periodogram, whenever a dark-light cycle was present. It is likely that daylight functions as the dominant zeitgeber that entrains their endogenous circadian clock. A fast re-entrainment of the Tb rhythm, shown by shifts in acrophase, which indicates the timing of the rhythm, was observed when zeitgeber conditions changed during migratory movements. Circadian rhythmicity disappeared under permanent light of the polar day, while the geese maintained a relatively high Tb during that time. There was a modest decrease in the daily mean Tb throughout autumn, which was more pronounced in Svalbard geese than in geese from Russia or Netherlands. However, the observed Tb changes during the pre-migratory period were of small magnitude compared to a previous study that was conducted on barnacle geese from the Svalbard colony. Moreover, and in contrast to the previous study, Tb increased again as soon as the migratory geese left their Arctic (post-) breeding grounds and returned to their wintering areas. Below we discuss the implications of our findings in more detail.
The seasonal change in day length (photoperiod) increases with latitude. It has been hypothesized that, for diurnal animals, the available daylight can be a limiting resource that may have favoured the evolution of latitudinal migration. Latitudinal migrants can benefit from a relatively longer photoperiod at higher latitudes, allowing them to prolong their daily activity and foraging time (Sockman and Hurlbert, 2020). In line with this hypothesis are observations of a strong positive relationship between daylight availability and activity in various species of latitudinal migrants (Pokrovsky et al., 2021), however, in those studies, no distinction was made between foraging and non-foraging activities. In the present study we can infer activity patterns of barnacle geese from their Tb patterns (see Supplementary Figure 7 for an illustration of this relationship). The geese prolonged their daily activity in response to longer daylength until they became active around the clock under continuous daylight. That prolonged activity in this species is mainly devoted to foraging is supported by the finding that the daily gain in body mass increased with the latitude of the staging site (and thus daylength) during the spring migration (Eichhorn, 2008). Intensive foraging continues upon arrival at Arctic (pre-)breeding grounds where the stores needed for clutch production and incubation are replenished (Hübner, 2006; Eichhorn et al., 2010).
After breeding, the migratory geese need to build up body stores for the autumn migration. It is perhaps for this reason that the Russian geese in the low Arctic remained largely arrhythmic, despite experiencing again light-dark cycles, during the first half of August (Figure 4). Geese are known to undertake additional nocturnal foraging during low light conditions, such as during twilight and moonlit nights, if they need extra intake to balance their energy budget (Lameris et al., 2021). Presumably, the Russian and Svalbard geese have similar energetic requirements when they are preparing for autumn migration (of a similar distance). However, at times when the geese in Svalbard can still benefit from the longer polar days, the geese in low Arctic Russia may need to extend their foraging activity into the dark phase of the day. The adaptive value for the geese of staying active around the clock, even in the presence of the usual external zeitgeber, is presumably to balance energy requirements in the context of migration. In contrast, as soon as the high Arctic Svalbard geese experienced the first days with sunset in late August, they aligned their primary resting phase to the dark phase of the day, which resulted in robust rhythmicity (Figure 4, Supplementary Figure 1). These different responses could illustrate the plasticity in individual timekeeping and / or in the behavioural and physiological output of timekeeping systems, and emphasize the functioning of the circadian system in an ecological context (Helm et al., 2017).
A malleable circadian pacemaking system, which can be quickly adjusted to changing zeitgeber conditions, is of advantage also during migratory movements. The free-ranging geese studied here showed a rapid re-entrainment of rhythm and its acrophase. Åkesson et al. (2021) demonstrated a fast re-entrainment of circadian rhythms in two songbird species by experimentally changing the photoperiod and simultaneously measuring the pattern of migratory restlessness and feeding.
It is thought that the hormone melatonin plays a key role for the malleability of the circadian pacemaker. A degree of malleability is important for maintaining entrainment when zeitgeber conditions change quickly, as they do during migration or when the diel amplitude of a zeitgeber is low, as it is at high latitudes. Accordingly, a reduced, but still rhythmic, amplitude of melatonin in the plasma has been reported during the polar day and during migration in birds (Gwinner and Brandstätter, 2001; Silverin et al., 2009). The observation of increased melatonin during a migratory stopover implies an entrainable clock during migration, and the stabilisation of circadian rhythms to a new photoperiod during staging periods (Fusani and Gwinner, 2004). Fast re-entrainment of the circadian clock has also been reported for an Arctic resident, the arctic ground squirrel Urocitellus parryii, which entrained to new light cycles over 1–2 days (Williams et al., 2017) as opposed to up to a week in laboratory mice (Jagannath et al., 2013). However, as opposed to melatonin in birds, the flexibility of the clocks in mammals is likely linked to vasopressin in the SCN (Williams et al., 2017).
A lack, or at least a strong attenuation, of circadian rhythmicity during the polar day is a common phenomenon among Arctic residents, particularly among non-burrowing herbivores such as reindeer Rangifer tarandus (Van Oort et al., 2007; Arnold et al., 2018), muskox Ovibos moschatus (Van Beest et al., 2020) and Svalbard ptarmigan Lagopus muta hyperborea (Appenroth et al., 2021), although they all show ultradian rhythmicity reflecting cycles of foraging and digestion. Irrespective of the exact mechanisms underlying the seasonal absence of overt circadian rhythmicity (see Bloch et al., 2013 for a discussion of putative mechanisms) the functional significance of around the clock activity in these Arctic herbivores seems mainly related to an increase in foraging activity, which facilitates balancing the energetic costs of reproduction and fattening during the short summer. We expect the same holds for migratory geese that visit the Arctic during summer and benefit not only from abundant food resources, but also the possibility of exploiting them throughout a 24-h foraging day. The combination allows for higher growth rates in Arctic barnacle geese compared to their temperate conspecifics; but, at the same time, the Arctic migrants are forced into running their annual cycle at a higher pace of life (Eichhorn et al., 2019). Additional motivations for geese, besides foraging, to stay active around the clock during the polar day likely include predator evasion and competition for nesting sites. In other species, such as Arctic breeding shorebirds, the competition for mates is another strong motivation, which can lead to “reproductive sleeplessness” during the pre-incubation period (Lesku et al., 2012; Steiger et al., 2013). In geese, however, pair formation takes place outside the breeding grounds, and pairs stay together usually throughout life.
Not all Arctic residents or visitors abolish diel rhythmicity during summer (see Williams et al., 2015 for a review). For certain species and circumstances, it is adaptive to maintain a circadian rhythm. It helps arctic ground squirrels, for example, to reduce the energy required for thermoregulation by aligning their above-ground activity rhythm to the generally warmest period of the day, while they use their burrow as a thermal refuge during the colder period (Williams et al., 2012). During the polar day, diel cycles of light intensity and ambient temperature can still be substantial, leading to daily cycles in the availability of arthropod prey, which may be the proximate reason for persistent circadian rhythmicity in insectivorous songbirds and shorebirds (Steiger et al., 2013). Environmental cues such as light intensity or colour, ambient temperature, or food availability, could act as alternative (low amplitude) zeitgebers in the absence of light-dark cycles (Stephan, 2002; Ashley et al., 2013). However, it remains unclear if these rhythms are indeed the output of an endogenous clock or are mediated by masking agents, i.e., a direct response to environmental changes (Ashley et al., 2013; Williams et al., 2015).
As outlined above, activity around the clock under continuous daylight has its obvious benefits, although, one wonders about potential negative consequences of prolonged sleep restriction or fragmentation. A recent study has shown that even low light level sources, like full moon or city sky glow, may be enough to suppress sleep in barnacle geese (Van Hasselt et al., 2021). Sleep restriction is known to compromise performance, health and survival. Indeed, “migratory sleeplessness” in white-crowned sparrows Zonotrichia leucophrys gambelii was associated with impaired execution of certain cognitive tasks (Jones et al., 2010). In contrast, “reproductive sleeplessness” shown by pectoral sandpipers during the Arctic breeding season appeared not to impair their performance or survival; in fact, males that slept the least sired the most offspring (Lesku et al., 2012). Under continuous daylight, melatonin secretion is reduced, which is expected to suppress immune function (Weil et al., 2015), and sleep deprivation may further weaken the immune system (Irwin and Opp, 2017). But in a pathogen poor environment like the Arctic, even a compromised immune defence might be sufficient (Piersma, 1997). Finally, sleep restriction in the Arctic geese and other birds for that matter may be less severe as it seems from the absence of diel rhythms. They still take resting bouts, which are also needed for food processing, and their ability for unihemispheric sleep enables birds to maintain vigilance against potential threats in their surroundings while still gaining some sleep (Rattenborg et al., 2000).
Like in our present study, Butler and Woakes (2001) aligned their data to the day when minimum abdominal Tb was reached in autumn by each of the ten Svalbard barnacle geese that they studied, and recorded, on average, a daily (24 h) mean value of 35.9°C, which is 4.8°C lower than the mean of 40.7°C recorded at the end of August. In another three non-migratory (captive-bred) geese held in captivity in Birmingham, they recorded a Tb reduction during autumn of only 1.8°C. These differences led the authors to suggest that the expression of heterothermy and migration (fuelling) might be functionally linked. In present study, however, we found that the average Tb reduction was only 1.0°C, and that reduction did not differ between Arctic-migratory geese from Russia and non-migratory geese from a Dutch colony (Table 1, Figure 5, and Supplementary Figure 4). Seasonal differences in mean Tb of 1°C were reported also for non-migratory greylag geese (Wascher et al., 2018). Although we see somewhat larger Tb reductions of 1.4°C (on average, and up to 1.6°C in one individual) in another study on Svalbard barnacle geese, the pronounced heterothermy as reported by Butler and Woakes (2001) does not seem to represent the norm for barnacle geese from Svalbard or other (migratory) populations.
Even though all data sets discussed here concern measurements of Tb inside the abdomen, measurements at multiple body locations in another bird, the king penguin Aptenodytes patagonicus, demonstrate that substantial Tb gradients can occur even within the abdominal cavity, and that Tb fluctuations are expected to increase with distance from the deep body core in the thorax with its constantly active organs, such as the heart and liver (Eichhorn et al., 2011). We positioned temperature probes in the Russian and Dutch barnacle geese in the upper abdomen, approximately three centimetres away from the liver. This may explain the generally higher Tb values in our data as compared to data from Svalbard geese in Portugal et al. (2019), where the Tb probe possibly was positioned further down in the abdomen. However, the position of a temperature probe cannot explain the notable differences between the two studies on barnacle geese from Svalbard. The data from Butler and Woakes (2001) show generally higher Tb values before the strong autumnal decline than all other data sets. Possibly extreme annual differences in weather (including e.g., snow or ice cover that influence access to food) and / or body condition may have played a role in the different levels of heterothermy exhibited by geese from Svalbard in the different studies. Most migratory geese that we examined in the present study left their breeding grounds when ambient temperatures fell to approximately 0°C (Figure 5), which is in line with the hypothesis that the onset of frost is a major trigger for the timing of the migratory departure in autumn in geese (Xu and Si, 2019).
The Butler and Woakes (2001) data are exceptional also in other ways. They showed large Tb reductions during both the resting phase and the active phase of the diel cycle. In contrast, our analyses show that the decline in the daily (24 h) mean Tb was far more the result of a decline during the resting phase, whereas the peak Tb during the active phase remained rather high and unchanged (Supplementary Figure 5). Perhaps the most remarkable finding in that earlier study on Svalbard geese was that the Tb decline continued throughout, and until several days after, migration, which was evidently not the case in the geese that we examined, in neither Russia or Svalbard. Migration was clearly associated with a rebound in Tb, which was particularly evident when we compared individuals that departed on different dates (Figure 5, Supplementary Figure 5). A continuous decline in Tb throughout migratory movements seems difficult to reconcile with an expected increase in heat production due to intensive exercise. We also assume that it would be advantageous for geese to forage intensively during daylight hours when they are replenishing body stores during staging and after migration. Moreover, although a lower Tb might save energy, it can impair the ability to fly and increase vulnerability to predation (Carr and Lima, 2013). Therefore, it seems adaptive to restrict more drastic Tb reductions to the resting phase and when geese are safe from predators. The dark period of the day offers geese protection from diurnal predators (e.g., white-tailed eagle Haliaeetus albicilla), and by retreating to open water or islands, the geese find additional safety from (nocturnal) mammalian predators (e.g., arctic fox Vulpes lagopus, red fox V. vulpes).
Autumn is characterized by both a shortening of the daylight period and a decrease in ambient temperature. Studies that have investigated the effect of ambient temperature on heterothermy have found only rather small effects [reviewed in Hohtola (2012)]. Although it is difficult to assess the potential effect of ambient temperature in present study, the course of ambient temperature fluctuated substantially and was often not in line with the gradual decline in Tb (Figure 5). A gradually lower rest-phase Tb contributed to an increase in the amplitude of the diel rhythm (Supplementary Figures 5, 6) and to a reduction in the daily mean Tb during autumn. In addition, the nocturnal resting phase became progressively longer during autumn, which also contributed to a reduction in the (24 h) mean Tb. The change in photoperiod, and hence length of the nocturnal resting phase, progresses faster at higher latitudes. This might also be the main driver for the relatively steeper decline in mean Tb in geese from Svalbard as compared to geese in Russia or Netherlands (Table 1, Figure 5, and Supplementary Figure 4). In other words, the daily rate of Tb decline through autumn might be slower at lower latitude just because the daily rate of change in photoperiod is slower. Indeed, once these two variables were evaluated in direct relationship, the population differences in the rate of decline disappeared (Supplementary Figure 8). Altogether, our examinations revealed no evidence that heterothermy in the barnacle goose is particularly linked to migration. Future studies are encouraged to investigate how common, and under what circumstances, the remarkable Tb reductions reported by Butler and Woakes (2001) may occur.
The original contributions presented in the study are included in the article/Supplementary Material, further inquiries can be directed to the corresponding author.
The animal research protocol was approved by the Animal Welfare Committees of the Royal Netherlands Academy of Arts and Sciences (license AVD8010020173788) and the St. Petersburg State University (decision nr. 131-03-2 from 3 April 2018).
GE conceived the study and led the writing of the manuscript. GE, MB, and HJ collected the data. GG and GE analysed the data, and discussed the interpretation and presentation of the results together with SM and MB. AM performed the surgical implantations. MW provided crucial equipment. All authors contributed to drafts and gave final approval for publication.
GE, MB, and HJ received funding from the Polar Programme of Netherlands Organisation for Scientific Research (grant ALWPP.2016.030). Additional funding to support the costs of conducting fieldwork in Russia and Netherlands came from the KNAW Ecology Fund and the Van der Hucht De Beukelaar Foundation, awarded to MB.
The authors declare that the research was conducted in the absence of any commercial or financial relationships that could be construed as a potential conflict of interest.
The handling editor declared a past co-authorship with one of the authors HJ.
We are grateful to a large flock of fieldworkers that helped with the catching and handling of geese. Family de Leeuw and “Staatsbosbeheer” are thanked for permission to access their land. Jetty Baars from the hospital Gelderse Vallei cared for the sterilization of implanted data loggers, and Daniel Zuniga is thanked for his help with surgery during logger implantation trials. Steven Portugal kindly provided some clarifications on the published data set on Svalbard barnacle geese. We thank the two reviewers for their valuable comments on an earlier version of this manuscript.
The Supplementary Material for this article can be found online at: https://www.frontiersin.org/articles/10.3389/fevo.2021.699917/full#supplementary-material
Åkesson, S., Ilieva, M., and Bianco, G. (2021). Flexibility and control of circadian activity, migratory restlessness and fuelling in two songbird migrants. Front. Ecol. Evol. 9:666176. doi: 10.3389/fevo.2021.666176
Alerstam, T., Hedenström, A., and Åkesson, S. (2003). Long-distance migration: evolution and determinants. Oikos 103, 247–260. doi: 10.1034/j.1600-0706.2003.12559.x
Alerstam, T., and Lindström, Å (1990). “Optimal bird migration: the relative importance of time, energy and safety,” in Bird Migration: Physiology and Ecophysiology, ed. E. Gwinner (Berlin, Heidelberg: Springer), 331–351. doi: 10.1007/978-3-642-74542-3_22
Appenroth, D., Nord, A., Hazlerigg, D. G., and Wagner, G. C. (2021). Body temperature and activity rhythms under different photoperiods in high arctic Svalbard ptarmigan (Lagopus muta hyperborea). Front. Physiol. 12:633866. doi: 10.3389/fphys.2021.633866
Arnold, W., Ruf, T., Loe, L. E., Irvine, R. J., Ropstad, E., Veiberg, V., et al. (2018). Circadian rhythmicity persists through the Polar night and midnight sun in Svalbard reindeer. Sci. Rep. 8:14466. doi: 10.1038/s41598-018-32778-4
Ashley, N. T., Schwabl, I., Goymann, W., and Buck, C. L. (2013). Keeping time under the midnight sun: behavioral and plasma melatonin profiles of free-living Lapland longspurs (Calcarius lapponicus) during the arctic summer. J. Exp. Zool. A Ecol. Genet. Physiol. 319, 10–22. doi: 10.1002/jez.1768
Bairlein, F. (2003). “Nutritional strategies in migratory birds,” in Avian Migration, eds P. Berthold, E. Gwinner, and E. Sonnenschein (Berlin, Heidelberg: Springer), 321–332. doi: 10.1007/978-3-662-05957-9_22
Bates, D., Mächler, M., Bolker, B., and Walker, S. (2015). Fitting linear mixed-effects models using lme4. J. Stat. Softw. 67, 1–48. doi: 10.18637/jss.v067.i01
Bloch, G., Barnes, B. M., Gerkema, M. P., and Helm, B. (2013). Animal activity around the clock with no overt circadian rhythms: patterns, mechanisms and adaptive value. Proc. R. Soc. B 280:20130019. doi: 10.1098/rspb.2013.0019
Butler, P. J., and Woakes, A. J. (2001). Seasonal hypothermia in a large migrating bird: saving energy for fat deposition? J. Exp. Biol. 204, 1361–1367. doi: 10.1242/jeb.204.7.1361
Butler, P. J., Woakes, A. J., and Bishop, C. M. (1998). Behaviour and physiology of Svalbard barnacle geese Branta leucopsis during their autumn migration. J. Avian Biol. 29, 536–545. doi: 10.2307/3677173
Carr, J. M., and Lima, S. L. (2013). Nocturnal hypothermia impairs flight ability in birds: a cost of being cool. Proc. R. Soc. B 280:20131846. doi: 10.1098/rspb.2013.1846
Cassone, V. M. (2014). Avian circadian organization: A chorus of clocks. Front. Neuroendocrinol. 35, 76–88. doi: 10.1016/j.yfrne.2013.10.002
Cramp, S., and Simmons, K. E. L. (1977). The Birds of the Western Palaearctic. Oxford: Oxford University Press.
DeCoursey, P. J., Walker, J. K., and Smith, S. A. (2000). A circadian pacemaker in free-living chipmunks: essential for survival? J. Comp. Physiol. A 186, 169–180. doi: 10.1007/s003590050017
Drent, R. H., Eichhorn, G., Flagstad, A., Van der Graaf, A. J., Litvin, K. E., and Stahl, J. (2007). Migratory connectivity in Arctic geese: spring stopovers are the weak links in meeting targets for breeding. J. Ornithol. 148, S501–S514. doi: 10.1007/s10336-007-0223-4
Dunlap, J. C., Loros, J. J., and DeCoursey, P. J. (2004). Chronobiology: Biological Timekeeping. Sunderland, MA: Sinauer Associates.
Eichhorn, G. (2005). “Northward bound: fat for flight. How barnacle geese budget their spring travels,” in Seeking Nature’s Limits, eds R. H. Drent, J. M. Tinbergen, J. P. Bakker, and T. Piersma (Utrecht: KNNV Publishing), 102–113.
Eichhorn, G. (2008). Travels in a Changing World: Flexibility and Constraints in Migration and Breeding of the Barnacle Goose. PhD dissertation. Groningen: University of Groningen.
Eichhorn, G., Afanasyev, V., Drent, R. H., and Van der Jeugd, H. P. (2006). Spring stopover routines in Russian barnacle geese Branta leucopsis tracked by resightings and geolocation. Ardea 94, 667–678.
Eichhorn, G., Drent, R. H., Stahl, J., Leito, A., and Alerstam, T. (2009). Skipping the Baltic: the emergence of a dichotomy of alternative spring migration strategies in Russian barnacle geese. J. Anim. Ecol. 78, 63–72. doi: 10.1111/j.1365-2656.2008.01485.x
Eichhorn, G., Enstipp, M. R., Georges, J.-Y., Hasselquist, D., and Nolet, B. A. (2019). Resting metabolic rate in migratory and non-migratory geese following range expansion: go south, go low. Oikos 128, 1424–1434. doi: 10.1111/oik.06468
Eichhorn, G., Groscolas, R., Le Glaunec, G., Parisel, C., Arnold, L., Medina, P., et al. (2011). Heterothermy in growing king penguins. Nat. Commun. 2:435. doi: 10.1038/ncomms1436
Eichhorn, G., Meijer, H., Oosterbeek, K., and Klaassen, M. (2012). Does agricultural food provide a good alternative to a natural diet for body store deposition in geese? Ecosphere 3:art35. doi: 10.1890/es11-00316.1
Eichhorn, G., Van der Jeugd, H. P., Meijer, H. A. J., and Drent, R. H. (2010). Fuelling incubation: differential use of body stores in arctic- and temperate-breeding barnacle geese (Branta leucopsis). Auk 127, 162–172. doi: 10.1525/auk.2009.09057
Fatima, N., and Rana, S. (2020). Metabolic implications of circadian disruption. Pflugers Arch. 472, 513–526. doi: 10.1007/s00424-020-02381-6
Fusani, L., and Gwinner, E. (2004). Simulation of migratory flight and stopover affects night levels of melatonin in a nocturnal migrant. Proc. R. Soc. B 271, 205–211. doi: 10.1098/rspb.2003.2561
Gaston, K. J. (2003). The Structure and Dynamics of Geographic Ranges. Oxford: Oxford University Press.
Geiser, F., and Brigham, R. M. (2012). “The other functions of torpor,” in Living in a Seasonal World: Thermoregulatory and Metabolic Adaptations, eds T. Ruf, C. Bieber, W. Arnold, and E. Millesi (Berlin, Heidelberg: Springer), 109–121. doi: 10.1007/978-3-642-28678-0_10
Golombek, D. A., and Rosenstein, R. E. (2010). Physiology of circadian entrainment. Physiol. Rev. 90, 1063–1102. doi: 10.1152/physrev.00009.2009
Gómez, C., Bayly, N. J., Norris, D. R., Mackenzie, S. A., Rosenberg, K. V., Taylor, P. D., et al. (2017). Fuel loads acquired at a stopover site influence the pace of intercontinental migration in a boreal songbird. Sci. Rep. 7:3405. doi: 10.1038/s41598-017-03503-4
Gwinner, E., and Brandstätter, R. (2001). Complex bird clocks. Philos. Trans. R. Soc. B 356, 1801–1810. doi: 10.1098/rstb.2001.0959
Helm, B., Visser, M. E., Schwartz, W., Kronfeld-Schor, N., Gerkema, M., Piersma, T., et al. (2017). Two sides of a coin: ecological and chronobiological perspectives of timing in the wild. Philos. Trans. R. Soc. B 372:20160246. doi: 10.1098/rstb.2016.0246
Hiebert, S. (1993). Seasonal changes in body mass and use of torpor in a migratory hummingbird. Auk 110, 787–797. doi: 10.2307/4088634
Hohtola, E. (2012). “Thermoregulatory adaptations to starvation in birds,” in Comparative Physiology of Fasting, Starvation, and Food Limitation, ed. M. D. McCue (Berlin, Heidelberg: Springer), 155–170. doi: 10.1007/978-3-642-29056-5_10
Holt, R. D., and Fryxell, J. M. (2011). “Theoretical reflections on the evolution of migration,” in Animal Migration: A Synthesis, eds E. J. Milner-Gulland, J. M. Fryxell, and A. R. E. Sinclair (Oxford: Oxford University Press), 17–31. doi: 10.1093/acprof:oso/9780199568994.003.0003
Hübner, C. E. (2006). The importance of pre-breeding areas for the arctic barnacle goose Branta leucopsis. Ardea 94, 701–713.
Irwin, M. R., and Opp, M. R. (2017). Sleep health: reciprocal regulation of sleep and innate immunity. Neuropsychopharmacology 42, 129–155. doi: 10.1038/npp.2016.148
Jagannath, A., Butler, R., Godinho, S. I. H., Couch, Y., Brown, L. A., Vasudevan, S. R., et al. (2013). The CRTC1-SIK1 pathway regulates entrainment of the circadian clock. Cell 154, 1100–1111. doi: 10.1016/j.cell.2013.08.004
Jones, S. G., Paletz, E. M., Obermeyer, W. H., Hannan, C. T., and Benca, R. M. (2010). Seasonal influences on sleep and executive function in the migratory white-crowned Sparrow (Zonotrichia leucophrys gambelii). BMC Neurosci. 11:87. doi: 10.1186/1471-2202-11-87
Jonker, R. M., Eichhorn, G., Van Langevelde, F., and Bauer, S. (2010). Predation danger can explain changes in timing of migration: the case of the barnacle goose. PLoS One 5:e11369. doi: 10.1371/journal.pone.0011369
Klein Tank, A. M. G., Wijngaard, J. B., Können, G. P., Böhm, R., Demarée, G., Gocheva, A., et al. (2002). Daily dataset of 20th-century surface air temperature and precipitation series for the european climate assessment. Int. J. Climatol. 22, 1441–1453. doi: 10.1002/joc.773
Lameris, T. K., Dokter, A. M., Van der Jeugd, H. P., Bouten, W., Koster, J., Sand, S. H. H., et al. (2021). Nocturnal foraging lifts time constraints in winter for migratory geese but hardly speeds up fueling. Behav. Ecol. 2021:araa152. doi: 10.1093/beheco/araa152
Lesku, J. A., Rattenborg, N. C., Valcu, M., Vyssotski, A. L., Kuhn, S., Kuemmeth, F., et al. (2012). Adaptive sleep loss in polygynous pectoral sandpipers. Science 337, 1654–1658. doi: 10.1126/science.1220939
McGuire, L. P., Jonasson, K. A., and Guglielmo, C. G. (2014). Bats on a budget: torpor-assisted migration saves time and energy. PLoS One 9:e115724. doi: 10.1371/journal.pone.0115724
McKechnie, A. E., and Lovegrove, B. G. (2002). Avian facultative hypothermic responses: a review. Condor 104, 705–724. doi: 10.1650/0010-5422(2002)104[0705:afhrar]2.0.co;2
Oberfeld, D., and Franke, T. (2013). Evaluating the robustness of repeated measures analyses: The case of small sample sizes and nonnormal data. Behav. Res. Methods 45, 792–812. doi: 10.3758/s13428-012-0281-2
Piersma, T. (1997). Do global patterns of habitat use and migration strategics co-evolve with relative investments in immunocompetence due to spatial variation in parasite pressure? Oikos 80, 623–631. doi: 10.2307/3546640
Pokrovsky, I., Kölzsch, A., Sherub, S., Fiedler, W., Glazov, P., Kulikova, O., et al. (2021). Longer days enable higher diurnal activity for migratory birds. J. Anim. Ecol. 2021:13484.
Portugal, S. J., Green, J. A., White, C. R., Guillemette, M., and Butler, P. J. (2012). Wild geese do not increase flight behaviour prior to migration. Biol. Lett. 8, 469–472. doi: 10.1098/rsbl.2011.0975
Portugal, S. J., White, C. R., Frappell, P. B., Green, J. A., and Butler, P. J. (2019). Impacts of “supermoon” events on the physiology of a wild bird. Ecol. Evol. 9, 7974–7984. doi: 10.1002/ece3.5311
R Core Team. (2021). R: a language and environment for statistical computing. Vienna, Austria: R Foundation for Statistical Computing.
Rattenborg, N. C., Amlaner, C. J., and Lima, S. L. (2000). Behavioral, neurophysiological and evolutionary perspectives on unihemispheric sleep. Neurosci. Biobehav. Rev. 24, 817–842. doi: 10.1016/S0149-7634(00)00039-7
Refinetti, R., Cornélissen, G., and Halberg, F. (2007). Procedures for numerical analysis of circadian rhythms. Biol. Rhythm. Res. 38, 275–325. doi: 10.1080/09291010600903692
Ruf, T. (1999). The lomb-scargle periodogram in biological rhythm research: analysis of incomplete and unequally spaced time-series. Biol. Rhythm. Res. 30, 178–201. doi: 10.1076/brhm.30.2.178.1422
Sachs, M. (2014). Cosinor: tools for estimating and predicting the cosinor model. R package version 1.1. Available Online at: https://CRAN.R-project.org/package=cosinor.
Shah, A. S. (2020). Card: cardiovascular and autonomic research design. R package version 0.1.0. Available Online at: https://CRAN.R-project.org/package=card.
Shariati-Najafabadi, M., Darvishzadeh, R., Skidmore, A. K., Kölzsch, A., Exo, K. M., Nolet, B. A., et al. (2016). Environmental parameters linked to the last migratory stage of barnacle geese en route to their breeding sites. Anim. Behav. 118, 81–95. doi: 10.1016/j.anbehav.2016.05.018
Silverin, B., Gwinner, E., Van’t Hof, T. J., Schwabl, I., Fusani, L., Hau, M., et al. (2009). Persistent diel melatonin rhythmicity during the Arctic summer in free-living willow warblers. Horm. Behav. 56, 163–168. doi: 10.1016/j.yhbeh.2009.04.002
Sockman, K. W., and Hurlbert, A. H. (2020). How the effects of latitude on daylight availability may have influenced the evolution of migration and photoperiodism. Funct. Ecol. 34, 1752–1766. doi: 10.1111/1365-2435.13578
Steiger, S. S., Valcu, M., Spoelstra, K., Helm, B., Wikelski, M., and Kempenaers, B. (2013). When the sun never sets: diverse activity rhythms under continuous daylight in free-living arctic-breeding birds. Proc. R. Soc. B 280:20131016. doi: 10.1098/rspb.2013.1016
Stephan, F. K. (2002). The “other” circadian system: Food as a zeitgeber. J. Biol. Rhythms 17, 284–292. doi: 10.1177/074873040201700402
Tabachnick, B. G., Fidell, L. S., and Ullman, J. B. (2019). Using Multivariate Statistics. Boston: Pearson.
Tapp, W. N., and Natelson, B. H. (1989). Circadian rhythms and patterns of performance before and after simulated jet lag. Am. J. Physiol. 257, R796–R803. doi: 10.1152/ajpregu.1989.257.4.R796
Thieurmel, B., and Elmarhraoui, A. (2019). Package ‘suncalc’: Compute sun position, sunlight phases, moon position and lunar phase.
Van Beest, F. M., Beumer, L. T., Chimienti, M., Desforges, J.-P., Huffeldt, N. P., Pedersen, S. H., et al. (2020). Environmental conditions alter behavioural organization and rhythmicity of a large Arctic ruminant across the annual cycle. R. Soc. Open Sci. 7:201614. doi: 10.1098/rsos.201614
Van der Jeugd, H. P., Eichhorn, G., Litvin, K. E., Stahl, J., Larsson, K., Van der Graaf, A. J., et al. (2009). Keeping up with early springs: rapid range expansion in an avian herbivore incurs a mismatch between reproductive timing and food supply. Glob. Change Biol. 15, 1057–1071. doi: 10.1111/j.1365-2486.2008.01804.x
Van der Jeugd, H. P., Gurtovaya, E., Eichhorn, G., Litvin, K. Y., Mineev, O. Y., and Van Eerden, M. R. (2003). Breeding barnacle geese in Kolokolkova Bay, Russia: number of breeding pairs, reproductive success and morphology. Polar Biol. 26, 700–706. doi: 10.1007/s00300-003-0535-7
Van Hasselt, S. J., Hut, R. A., Allocca, G., Vyssotski, A. L., Piersma, T., Rattenborg, N. C., et al. (2021). Cloud cover amplifies the sleep-suppressing effect of artificial light at night in geese. Environ. Pollut. 273:116444. doi: 10.1016/j.envpol.2021.116444
Van Oort, B. E. H., Tyler, N. J. C., Gerkema, M. P., Folkow, L., and Stokkan, K.-A. (2007). Where clocks are redundant: weak circadian mechanisms in reindeer living under polar photic conditions. Naturwissenschaften 94, 183–194. doi: 10.1007/s00114-006-0174-2
Wascher, C. A. F., Kotrschal, K., and Arnold, W. (2018). Free-living greylag geese adjust their heart rates and body core temperatures to season and reproductive context. Sci. Rep. 8:2142. doi: 10.1038/s41598-018-20655-z
Weil, Z. M., Borniger, J. C., Cisse, Y. M., Abi Salloum, B. A., and Nelson, R. J. (2015). Neuroendocrine control of photoperiodic changes in immune function. Front. Neuroendocrinol. 37, 108–118. doi: 10.1016/j.yfrne.2014.10.001
Williams, C. T., Barnes, B. M., and Buck, C. L. (2012). Daily body temperature rhythms persist under the midnight sun but are absent during hibernation in free-living arctic ground squirrels. Biol. Lett. 8, 31–34. doi: 10.1098/rsbl.2011.0435
Williams, C. T., Barnes, B. M., and Buck, C. L. (2015). Persistence, entrainment, and function of circadian rhythms in Polar vertebrates. Physiology 30, 86–96. doi: 10.1152/physiol.00045.2014
Williams, C. T., Barnes, B. M., Yan, L., and Buck, C. L. (2017). Entraining to the polar day: circadian rhythms in arctic ground squirrels. J. Exp. Biol. 220, 3095–3102. doi: 10.1242/jeb.159889
Wojciechowski, M. S., and Pinshow, B. (2009). Heterothermy in small, migrating passerine birds during stopover: use of hypothermia at rest accelerates fuel accumulation. J. Exp. Biol. 212, 3068–3075. doi: 10.1242/jeb.033001
Keywords: activity, arctic, arrhythmicity, body temperature, circadian rhythms, herbivore, heterothermy, migration
Citation: Eichhorn G, Boom MP, van der Jeugd HP, Mulder A, Wikelski M, Maloney SK and Goh GH (2021) Circadian and Seasonal Patterns of Body Temperature in Arctic Migratory and Temperate Non-migratory Geese. Front. Ecol. Evol. 9:699917. doi: 10.3389/fevo.2021.699917
Received: 24 April 2021; Accepted: 07 June 2021;
Published: 29 June 2021.
Edited by:
Ivan Maggini, University of Veterinary Medicine Vienna, AustriaReviewed by:
Claudia A. F. Wascher, Anglia Ruskin University, United KingdomCopyright © 2021 Eichhorn, Boom, van der Jeugd, Mulder, Wikelski, Maloney and Goh. This is an open-access article distributed under the terms of the Creative Commons Attribution License (CC BY). The use, distribution or reproduction in other forums is permitted, provided the original author(s) and the copyright owner(s) are credited and that the original publication in this journal is cited, in accordance with accepted academic practice. No use, distribution or reproduction is permitted which does not comply with these terms.
*Correspondence: Götz Eichhorn, ZWljaGdvZXR6QHdlYi5kZQ==
Disclaimer: All claims expressed in this article are solely those of the authors and do not necessarily represent those of their affiliated organizations, or those of the publisher, the editors and the reviewers. Any product that may be evaluated in this article or claim that may be made by its manufacturer is not guaranteed or endorsed by the publisher.
Research integrity at Frontiers
Learn more about the work of our research integrity team to safeguard the quality of each article we publish.