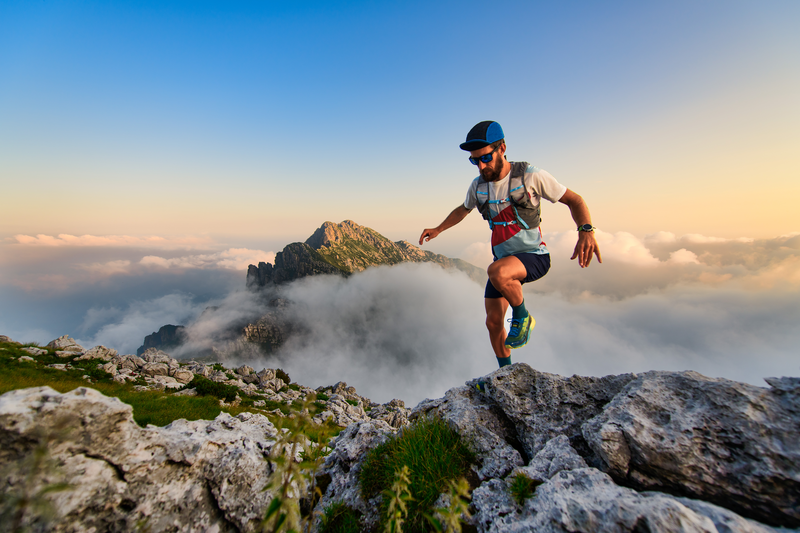
95% of researchers rate our articles as excellent or good
Learn more about the work of our research integrity team to safeguard the quality of each article we publish.
Find out more
ORIGINAL RESEARCH article
Front. Ecol. Evol. , 27 July 2021
Sec. Conservation and Restoration Ecology
Volume 9 - 2021 | https://doi.org/10.3389/fevo.2021.699649
This article is part of the Research Topic The Decline of Wild Bees: Causes and Consequences View all 8 articles
Bee pollinators are key components of terrestrial ecosystems. Evidence is mounting that bees are globally in decline, and species with a higher degree of specialization are the most vulnerable to local extinction. However, ecological features that could explain bee specialization remain poorly tested, especially in tropical species. Here, we aim to determine the most specialized bee species and their associated ecological traits in tropical plant–bee interaction networks, answering three questions: (1) Which bees in the interaction networks are specialists? (2) Is body size related to their role as specialists in interaction networks? (3) Are there phylogenetic relationships between the bee species identified as specialists? We used fifteen quantitative plant–bee interaction networks from different Brazilian biomes covering 1,702 interactions (386 bee and 717 plant species). We used the normalized degree (standardized number of partners) as a metric to determine trophic specialization of bee species. Body size was estimated by measuring intertegular distance (ITD), i.e., the distance between the bases of the wings on the thorax. Evolutionary distinctiveness (ED) was used to quantify species uniqueness, i.e., the singularity of species in the phylogenetic tree. Relationships between dietary specialism, ITD and ED were assessed using generalized linear models. We detected 34 specialist bee species (9% of total species), distributed in 13 genera, and four families. ITD and ED were important variables explaining the specialization of tropical bee species. Specialists were larger and less phylogenetically distinct than expected by chance. Based on a large data set covering some of the main tropical biomes, our results suggest that loss of specialist bees from Brazilian plant–bee networks could have deleterious consequences for native plant species preferentially pollinated by large-bodied bees. Moreover, by affecting more evolutionarily distinct species, i.e., those with fewer extant relatives, the loss of specialist bees will likely affect few clades but can result on considerable loss of evolutionary history and phylogenetic diversity in the Brazilian bee communities. The results are important for decision-making concerning conservation measures for these species and may also encourage the development of sustainable management techniques for bees.
Pollination between bees and plants is one of the key mutualistic interactions structuring ecosystems (Potts et al., 2016). This interaction is necessary for the reproduction of most plants, allowing fruit and seed formation in natural areas (Laura et al., 2013), agricultural lands (Klein et al., 2007), and areas under recovery (Montoya et al., 2012). Thus, pollinators are essential for the maintenance of natural communities and agricultural productivity (Potts et al., 2010, 2016; Vanbergen et al., 2014). However, several studies have indicated that the abundance and diversity of bees are declining and that some species are clearly at risk of extinction (Gallai et al., 2009; Garibaldi et al., 2009; Lever et al., 2014). In particular, specialist species (i.e., those that interact with a smaller number of partners) seem to be the most vulnerable to habitat loss (Bommarco et al., 2010; Aizen et al., 2012; Ferreira et al., 2020). Bees are considered the main taxon of pollinators, so understanding bee pollination networks and bee specialism patterns may provide effective support to conservation and restoration programs (Hallett et al., 2013).
Specialization is broadly defined as the ability of organisms to exploit a more restricted set of resources than that of related lineages (Day et al., 2016). Implicit in this concept is the idea that the subset of resources exploited is used more effectively and sometimes exclusively by specialists than by generalists (Larsson, 2005; Armbruster, 2017). Bees are usually polylectic, i.e., they collect floral resources from a great diversity of plant species (Michener, 1979), exhibiting generalist behavior (Ebeling et al., 2011). Bee specialization on a single food resource is rare (e.g., González-Varo et al., 2016) and usually, specialist species depend on generalist partners (see below; Bascompte et al., 2003). Nevertheless, oligolecty may function for reducing competition and foraging costs (Armbruster, 2017), and specialist bees can be key pollinators, i.e., network connectors (hubs) (Olesen et al., 2007; Romero et al., 2020) and crop pollinators, such as the case of Peponapis Robertson, 1902 that preferentially visit and pollinate cucurbit plants (Guzman et al., 2019; see also Cane, 2021). Therefore, the replacement of specialist species by generalist ones may have negative consequences on community stability and resilience (Peralta et al., 2020), as well as ecosystem functioning (Clavel et al., 2011). Moreover, visiting bees do not always act as pollinators (Popic et al., 2013), and specific methods are needed to determine the effectiveness of pollination (e.g., Dafni, 1993).
The theory of complex networks has been used to describe the structure of interspecific mutualistic interactions and the role of species within communities (Bascompte et al., 2003). Some patterns emerge from the analysis of these networks, such as nestedness, a pattern in which the interactions are asymmetric, i.e., specialist and generalist species tend to interact with the generalist ones, characterizing a core of species (Bascompte et al., 2006; Bascompte and Jordano, 2007; Aizen et al., 2012). Thus, the analysis of interaction networks is useful to characterize the level of specialization among species composing the network (Montoya et al., 2006; Weiner et al., 2014). However, such network-based approaches to determining ecological specialization are strongly influenced by sampling methods, and failure to use standard survey methods can produce inaccurate assessments of plant–bee interactions (Vázquez and Simberloff, 2002; Vázquez and Aizen, 2003; Dorado et al., 2011). For example, in tropical regions, mainly in tropical forests with high canopies, bee sampling is usually restricted to flowers in the lower strata of vegetation (Ferreira et al., 2020).
Initially, the generalist and specialist role of species was related to their abundance (Krishna et al., 2008) but, later evidence has also shown that morphological traits are important in determining the interaction patterns of these matrices (Chamberlain et al., 2010; Gibson et al., 2012). It has been suggested that similarity in resource use is higher among species with similar body size (Cortopassi-Laurino et al., 2003; Nogueira-Ferreira and Augusto, 2007; Rabelo et al., 2014). For instance, in temperate forests, smaller bees can be more specialized foragers than larger bees (Smith et al., 2019). The authors suggested that large bees fly greater distances and thus, encounter and exploit a wider range of plant species than bee taxa with more limited dispersal abilities (Smith et al., 2019). Some controversy may be found, however, when considering bumblebees from the northern hemisphere. A previous study indicated that the foraging behavior of some large European species (genus Bombus Latreille, 1802) is generalist, as these species did not show fidelity in their interactions with the studied grassland plants (they can collect pollen from up to three different plant species during a foraging trip, thus having a more generalist role) (Leonhardt and Blüthgen, 2012). Another study indicated high fidelity of interactions between bumblebees and a single plant species in undisturbed forest patches of North America (Villalobos et al., 2019). Hence, further studies are required to clarify the relationship between pollinator body size and diet specialization, especially in tropical regions where still exists an important knowledge gap.
In addition to body size, specialist behavior may be related to the degree of evolutionary relationship between species because interspecific interactions are largely structured by the degree of phenotypic complementarity between mutualistic partners (e.g., long-tongued bees visit flowers with deep corolla tubes) (Vázquez et al., 2009). As related species tend to have similar characteristics due to shared ancestry (Rezende et al., 2007a), they often occupy similar positions in terms of their interactions, both with regard to the number of partners with which they interact and to the functional attributes of their partner species (Hutchinson et al., 2017). On the other hand, it is likely that competition between similar individuals is greater, and the speciation process could take place by selecting different characteristics between species, allowing them to differentiate their niches (Johnson and Hubbell, 1975). Thus, closer species could also be potentially different in terms of trophic interactions.
The present study analyzes specialization in interaction networks between plants and bees in tropical biomes, aiming to determine: (1) Which bees in the interaction networks are specialists? (2) Is the body size of bees related to their role as specialists in interaction networks? (3) Are there phylogenetic relationships between the bee species identified as specialists? Answering those questions can help understanding the ecosystem functioning in the present (but also predict future global change responses), providing support to decision-make processes concerning pollinator’s conservation and sustainable management, and identifying pathways for future research on specialist pollinator taxa in tropical ecosystems.
Studies conducted in Brazil on interaction networks between bees and plants were compiled previously (Giannini et al., 2015). All studies followed standardized sampling procedures suggested by Sakagami et al. (1967), in which the researcher observes each plant in a pre-established transect for a fixed time (3–5 min) and collects all the bees observed using an entomological net, repeating this procedure monthly for at least one year. The interaction networks were represented as a set of nodes (species) connected through links, with each link representing an observed interaction (Bascompte and Jordano, 2007). We included surveys that determined the number of bees sampled per plant to construct fifteen quantitative networks. These networks belong to some of the most important Brazilian biomes (Figure 1). The nomenclature and systematic identification of the bees followed that of Moure’s Bee Catalog1, and plant identification was in accordance with the Missouri Botanical Garden’s Tropicos information system2.
Figure 1. Location of the interaction networks studied. The numbers correspond to the identifiers (ID) in Table 1.
To answer the first question and identify the specialist bees in the interaction networks, the following network metrics were used: (1) Degree, which describes the number of interactions of a given species with different species; thus, species with lower degree values are considered specialists because they interact with few species (Thompson, 2005; Vázquez et al., 2005; Krishna et al., 2008). (2) Strength, which is the relative frequency of interaction of a bee species with a plant species; it is represented as the number of interactions between a bee species and a plant species divided by the number of visits by all bee species to that same plant. Thus, specialist bees have lower interaction strength values (Vázquez et al., 2007; Dormann, 2011; Schleuning et al., 2011; Mello et al., 2016). (3) Closeness, which is based on the number of steps on the shortest path (in terms of interactions) that link a particular species to all other species in the network; a low closeness value for a species means that it is distant from most other species in the network, indicating specialization in interactions (González et al., 2010). (4) Betweenness, which measures the extent to which a particular species is located within the shortest paths between two other species, acting as a central connector in the network (Newman, 2003); species with lower betweenness values are also considered more specialized (Guimarães et al., 2011). To test whether the four metrics were correlated, a Pearson’s correlation test was performed. The results obtained for each metric derived from each network were scaled ranging from 0 to 1 (using x′ = x – min x/max x – minx x) to reduce a possible effect of the number of interactions observed. This procedure better reflects the role of each species in the network than the raw data, avoiding any trend in the analysis of the process (Legendre and Legendre, 2012). The four metrics presented were calculated using the bipartite package (Dormann, 2019) in R (R Core Team, 2021). We selected the 5% most specialized bees in each network, aiming to determine the smallest set of species in each network, especially to help in guiding further studies and conservation programs.
To answer our second question (does body size determine bee species’ roles as specialists in interaction networks?), we used a database containing intertegular distance (ITD) measurements of Brazilian bee species (Borges et al., 2020), which was complemented in the current study (46% of ITD measures). ITD is correlated with the body size of bee species (Cane, 1987; Figure 2), and was used as a predictor variable for the previously mentioned specialization metrics of the interaction networks.
Figure 2. The intertegular distance (ITD) is the distance between the bases of the wings on the bee thorax (Megachile orba Schrottky, 1913; Photo: Fernanda Trancoso).
To answer the third question (are there phylogenetic relationships between bee species identified as specialists?), a phylogeny was constructed containing all bee genera with available data recorded for Brazil, according to the TimeTree database (timetree.org, access on February 15, 2019). Species recorded in this study were inserted within the phylomatic function (Webb and Donoghue, 2005) from the software PHYLOCOM, version 4.2 (Webb et al., 2008). For tree calibration by the branch length adjustment (“bladj”) algorithm from PHYLOCOM, we used median time estimates for internal nodes, as given by the TimeTree database. The Evolutionary distinctiveness (ED) metric was used to quantify singularity of species in the phylogenetic tree (Faith, 1992; Fournier et al., 2017), being suggested to point out priority species for conservation (Veron et al., 2017). ED was computed for all species registered in this study by “evol.distinct” function using fair portions (Isaac et al., 2007) from the R package “picante” (Kembel, 2020). Additionally, phylogenetic signal, i.e., the tendency that closely related bee species resemble each other in terms of functionality, was computed as Pagel’s λ and Bloomberg’s K for the ITD using the “phylosig” function from the “phytools” R package (Revell, 2021). In both indices, the null expectation (high phylogenetic signal) assumes Brownian motion models of evolution (random walk). Pagel’s λ measures to which degree the shared history of taxa has driven trait distribution at the tips of the phylogeny (Pagel, 1997, 1999). By definition, a lambda value of 1 results from a trait distribution as expected from Brownian motion (high phylogenetic signal), and lambda values of 0 indicate that there is no phylogenetic signal. Bloomberg’s K (Blomberg et al., 2003) ranges between 0 and infinity, with K = 1 indicating Brownian motion evolution. Larger K indicates stronger phylogenetic signal. “phylosig” computes p-values to test if observed lambda and K value differ significantly from 0, i.e., if there is a phylogenetic signal.
To test effects of body size (ITD) and ED on bee species’ specialization (normalized degree), we constructed generalized linear mixed models (GLMMs), which are considered the best tool to analyze non-normal data involving random effects (Bolker et al., 2009). We used beta models in the R package glmmTMB (Gałecki and Burzykowski, 2013), because the data were scaled between 0 and 1, as mentioned above. Predictor variables included ITD and ED, and random variables included network and bee species, since the location where the networks were sampled can potentially affect the relationship to be tested, and some species may be more represented than others in the networks, which could increase the variability of the metrics. Candidate models were ranked by Akaike information criterion (AIC) values using the “dredge” function in the R package “MuMIn” (Barton, 2020), and selected models were those models <2 delta AIC of the top model.
The database organized in the present study included 15 interaction networks belonging to three Brazilian biomes (Atlantic Forests, Cerrado and Caatinga; Table 1 and Figure 1), containing 1,702 interactions between bees and plants (Figure 3). Data showed 814 specimens of bees distributed in 4 families, 118 genera, 386 species (Supplementary Material), and 869 specimens of plants distributed in 100 families, 336 genera, and 717 species.
Figure 3. The plant–bee interaction network compiled from all the data analyzed from 15 interaction networks surveyed in three different Brazilian Biomes. All non-specialist bee species are represented in black, all plants in green and all specialist bees and their links are in red. Network plot was generated with Circos (Krzywinski et al., 2009).
Correlation analysis of the four network metrics showed a strong correlation between degree and the other metrics (r > 0.70, p < 0.0001) and an intermediate correlation between the other three (strength, closeness, and betweenness) (r < 0.53, p < 0.0001). Thus, degree was used as our measure of specialism among bee taxa in the following analyses.
Specialist bees (5% of each network with the lowest degree) selected in the 15 networks totaled 34 species distributed in 13 genera and four families (Table 2). This corresponds to 8.7% of the total bee species recorded, and to 10.9% of the total bee genera in the database. The genus Centris (Fabricius, 1804) had the highest number of specialist bee species (10 species), followed by Augochloropsis (Cockerell, 1897) (6 species) and Augochlora (Smith, 1853) (5 species). The genera Anthodioctes (Holmberg, 1903), Bombus and Caenohalictus (Cameron, 1903) had two species each. Acanthopus (Klug, 1807), Agapostemon (Guérin-Méneville, 1844), Alepidosceles (Moure, 1947), Ariphanarthra (Moure, 1951), Augochlorella (Sandhouse, 1937), Callonychium (Brèthes, 1922), and Ceratina (Latreille, 1802) were represented with one species each.
Table 2. Specialist bee species selected on 15 Brazilian plant–bee networks (representing 5% of each network) and their degree.
The ITD was obtained for 85% of the analyzed bee species. A phylogenetic tree with all the genera analyzed in this study, including their branch length, can be found in Figure 4.
Figure 4. Phylogenetic tree using all the bee genera analyzed from 15 interaction networks surveyed in three different Brazilian Biomes.
The best model of degree among Brazilian bee species included the predictor variables ITD and ED, with species and network held as random effects (Table 3). The resulting graphical representation of the mixed generalized linear model showed that the greater the specialization, the larger was ITD (Figure 5A). The result also shows that the greater the specialization, the lower was ED (Figure 5B). Thus, according to our data, specialist bee species are larger and less phylogenetically distinct than expected by chance. Moreover, a significant phylogenetic signal of ITD was found (Pagel’s λ [p-value] = 0.9999 [<0.001]; Bloomberg’s K [p-value] = 1.4069 [0.001]).
Table 3. Model selection results based on Akaike information criterion (AIC) for beta GLMMs of bee species’ specialization, as measured using degree (D).
Figure 5. Graphical representation of the degree (number of partners) in relation to the (A) ITD and (B) evolutionary distinctiveness (ED) of the selected model (Model 1 – see Table 3). A lower degree value indicates higher specialization. Shaded areas represent 95% confidence intervals.
Using 15 interaction networks sampled across a range of Neotropical biomes, we identified 34 bee species that had the lowest number of interaction partners, and thus can be considered the most specialized. Nearly one third of them belong to the oil-collecting bee genus (Centris). Results also showed that more specialized bees (i.e., low degree) tend to have larger body size and lower ED than more generalist species. Also, we found a phylogenetic signal for body size (ITD). Our study is the first with such quantitative assessment of specialization for Neotropical bees, and based on more than 1,700 plant–bee interactions.
The highly specialized mutualistic relationships between the solitary bees of the genus Centris and families of plants whose flowers produce oil are well known (Machado, 2004; Mello et al., 2013; Rabelo et al., 2014; Pacheco-Filho et al., 2015). The main sources of floral oils with recorded interactions with these bees belong to the following botanical families: Calceolariaceae, Iridaceae, Krameriaceae, Malpighiaceae, Orchidaceae, and Plantaginaceae (Giannini et al., 2013; Martins et al., 2015). The species Centris burgdorfi (Friese, 1900) was previously indicated as a specialist on a local scale (Sabino et al., 2019). Species of some subgenera of Centris (especially Paracentris and Wagenknechtia) are notably specialists (Giannini et al., 2013), and two species from the list obtained here belong to Paracentris [C. burgdorfi Friese (1900) and Centris klugii Friese (1899)]. For other species cited as specialists, Augochlorella ephyra (Schrottky, 1910) was classified as rare in interactions with flowers of the plant Ludwigia sericea (Cambess.) H. Hara (Onagraceae) in South Brazil (Gonçalves and Buschini, 2017). One species of the genus Caenohalictus was cited as a specialist in an interaction network study conducted in South Brazil (Diniz and Buschini, 2015), although the species quoted was different from the two species recorded in the present study. Bees in the genus Alepidosceles (including A. imitatrix Schrottky, 1909) are all components of an oligolectic clade (Michener, 2007), therefore demonstrating the strength of the methods applied here to determine specialist bees from Neotropical interaction networks. The bee Acanthopus excellens (Schrottky, 1902) is a cleptoparasite of Centris, subgenus Ptilotopus, although none of the Centris in this subgenus is specialist in our study. Acanthopus visits on flowers are likely an unusual event, as they are not required to provide food for their offspring. For the other species of bees identified as specialists, there are no published studies on their mutualistic interactions patterns, demonstrating the important knowledge gap. Available data are limited to the degree of rarity, record and frequency of occurrence (Schlindwein, 1998; Imperatriz-Fonseca et al., 2011; Boff et al., 2013; Mouga et al., 2015; Gostinski et al., 2016). It is also noteworthy that most of the specialist species emphasized here exhibit non-eusocial behavior, for which there are little data available in the literature and/or an expressive taxonomic impediment, especially for species in the Halictidae family (16 out of the 34 specialist species belong to this family).
The results of the present study also showed that bee specialization is positively related to body size, i.e., the larger the bee, the higher the specialization. Although these results contradict two previous studies (Leonhardt and Blüthgen, 2012; Smith et al., 2019), it is also in accordance to the finds of one study in undisturbed forests of North America (Villalobos et al., 2019). The study of Leonhardt and Blüthgen (2012) compared Apis mellifera L. and two species of Bombus [B. pascuorum (Scopoli, 1763) and B. terrestres (Linnaeus, 1758)]. Although Bombus species have large body sizes (Borges et al., 2020), this variable was not specifically evaluated. The study of Smith et al. (2019), conducted on forests in the mid-Atlantic United States, analyzed a much smaller number of bee species (56 species). This reinforces the importance of the results obtained in the present study, since they address several tropical and subtropical climate sites in Brazil, and a large number of species. Warmer climates might support the evolution of specialist behavior among pollinators, given the high resource supply and the costs for resources competition (Classen et al., 2020), which may explain the opposite relations between broad scale studies from temperate and tropical climates (see also Orr et al., 2021).
Body size directly influences foraging capacity and resource exploitation in bees (Rabelo et al., 2015) because larger bees have a larger flight range (Greenleaf et al., 2007). Our result may indicate that larger bee species, presenting a greater flight capacity, can most likely preferentially exploit the most rewarding food sources and thus, be more prone to specialize in these sources. Larger body size bees can maximize exploitation of a sparsely distributed food plant, becoming more efficient at resource collection on a single species or collection of closely related plant species than bee species with more generalized diets, thus gaining a fitness advantage. But this is only possible in large species that can overcome energetic costs of such a strategy. In fact, preferences based in nutritional benefits have already been shown. The amount of amino acids in pollen seems to influences floral preference in A. mellifera (Cook et al., 2003); similarly, the ratio between pollen proteins and lipids affects the preference of bumblebees (Vaudo et al., 2016). The discrimination capacity presented by bees seems to have a genetic basis, i.e., certain genes have an effect on the preference of pollinators and may therefore have played an important role in floral evolution (Schemske and Bradshaw, 1999). As mentioned above, there are few studies available on bees, but a previous study involving ants and their interactive plants showed an equally positive relationship between species body size and specialization in trophic interaction networks (Chamberlain and Holland, 2009).
The interactions between plants and bees may exhibit phylogenetic components that direct the preferences of a group of bees toward a particular group of plants (Pacheco-Filho et al., 2015). In fact, specialism seems to be an ancestral trait in bees, since the earliest branches of their phylogenetic tree include specialists (Danforth et al., 2006). Some bees developed convergent traits specialized in the exploitation of floral resources, forming functional groups (Fenster et al., 2004), especially in highly specialized interactions. One example is orchid bees (Euglossini), which through coevolutionary processes exhibit specialized morphological and behavioral modifications for the collection of aromatic compounds from orchids flowers (Ramírez et al., 2010, 2011). Body size, as showed by our results, presented a strong phylogenetic signal indicating that closely related species tend to resemble each other considering this trait. This was already showed for insects (Chown and Gaston, 2010; Pallarés et al., 2019), as well for vertebrates (Smith et al., 2004; Diniz-Filho et al., 2007). However, complex interactions between ecological function, environment, natural history and phylogenetic evolution likely play a joint role in body size (Smith et al., 2004), and how significant is specifically the phylogenetic signal in its variation remains unclear (Chown and Gaston, 2010).
As mentioned earlier, highly specialized mutualistic interactions are rare in nature (Hoeksema and Bruna, 2000), and our study showed that species with more specialized behavior have lower ED in the bee phylogeny. Together with the concentration of most specialist within few genera from mainly two families, this may indicate that, depending on how these species respond to environmental changes (climatic, land use, for example), the loss of evolutionary history and phylogenetic diversity within certain clades would be much higher than if there was a more heterogeneous distribution of specialist species in the phylogeny. Rezende et al. (2007b) tested whether simulated coextinctions in plant–animal mutualistic interaction networks involved phylogenetically related species more frequently than expected at random. The authors obtained evidence that phylogeny is partially responsible for the propensity of species to interact in more than one-third of the analyzed networks and that phylogenetically related species tend to interact with a similar set of species and tend to have similar roles in interaction networks.
Our results also showed that none of the 34 specialist bee species highlighted here exhibit eusocial behavior. Social species (some degree of sociality, i.e., from cooperative working to eusociality) represent 10–15% of all extant bees and are usually regarded as generalists (Michener, 2007), however, this may not be true for all species. Worldwide, few social species have been studied (Smith et al., 2019), being bumblebees and honeybees the most represented taxa. Solitary species represent 85% of extant bees (Michener, 2007) (they are about 75% of the species in our study), but despite being the majority (Wcislo and Cane, 1996), the knowledge on solitary species biology is even more scarce. Generalist trends within eusocial bees have been hypothesized as a response to the (1) high number of individuals in the nest (high demand for resources in the nest and high abundances in the field), (2) eusocial colonies are active throughout the year (especially in tropical zones), and (3) labor division supports a higher diversity of interactions (independent search combined to recruitment foraging behavior) (Lichtenberg et al., 2010; Maia et al., 2019). From the 13 genera of specialist bees identified in the present study, one is a cleptoparasitic (Acanthopus), one is a representative of an oligolectic and solitary clade (Alepidosceles), four are solitary (Anthodioctes, Ariphanarthra, Centris, and Ceratina) and seven present some degree of sociality, from the formation of aggregated nesting sites (Caenohalictus and Callonychium), communal behavior (Agapostemon and Augochlora), variable social behavior (Augochlorella and Augochloropsis), and the primitively eusocial bumblebees (genus Bombus) (Janjic and Packer, 2003; Alves dos Santos, 2004; Coelho, 2004; Michener, 2007; Gonçalves, 2016; Gonçalves, 2019). Although most specialist species are solitary, some are also social, as found in undisturbed forests of north America (Villalobos et al., 2019); thus, both social and solitary bees are prone to adopt specialist behavior (Classen et al., 2020).
Specialism apparently has multiple independent evolutionary origins, being more common on Colletidae, Andrenidae, Megachilidae, and non-social Apinae, but most genera within these families contain also generalist species (Wcislo and Cane, 1996). Multiple drivers are likely related to trophic specialism on both social and solitary bees, such as, a larger or smaller suit of foraging behaviors (Portman et al., 2019), the plasticity to respond to floral resource coverage (Petanidou et al., 2008; Fründ et al., 2010; Baude et al., 2016; Kelly and Elle, 2021), seasonal or phenological change of nutritional value on available floral resources (Seitz et al., 2020), resource competition (Johnson and Hubbell, 1975; Sáez et al., 2017) specially related to nectar-robbing processes (Irwin et al., 2010), and also, can be based on the biology of individual-level interactions and not only on species-level (Brosi, 2016; Smith et al., 2019). Since bees are a megadiverse group (Orr et al., 2021), further studies are still necessary for understanding the roles of sociality and specialization on foraging behavior and species evolution.
As previously stated, it is necessary to consider that methodological survey biases may interfere with the results obtained here, especially because high canopy forests cannot be surveyed using the standard methodology analyzed. There remains a big gap regarding studies of interaction networks with standardized methods in tropical regions, as well as studies on phylogenetic and functional traits, which makes comparisons even more difficult. However, the present work is an important contribution considering current empirical data in tropical regions, and aims to help pave the way for a deeper understanding of the mechanisms driving specialization patterns in tropical environments.
Future studies should prioritize new surveys of plant–bee interaction in places not yet sampled and test other interaction network metrics to identify specialist species (e.g., Blüthgen et al., 2006; Kaiser-Bunbury and Blüthgen, 2015; Vargas et al., 2017). They also should deepen the ecological knowledge on bee species here identified as specialists to help devise conservation programs. A possible investigation is to determine if specialism is related to bee phenology and flight activity period. Additionally, it would be important to determine the geographical distribution of specialist species to protect their natural habitats, ensuring future habitat availability. Studies that involve conservation genetics for maintenance of the genetic viability of natural populations of specialist species are also urgently required.
Based on a data set covering some of the main tropical biomes, we demonstrate that interaction network metrics are useful for identifying the bees that have specialized interactions with plants and that these species have a larger body size and lower ED. These species deserve attention because they act as effective pollinators and are more vulnerable to environmental change. Our results can serve as support to decision-making and the implementation of conservation measures for these species, and may also encourage future studies. Given increasing human impacts on tropical ecosystems, evidence-based approaches to conserving specialist bees are urgently needed to safeguard their populations and functions in tropical plant–pollinator communities.
The original contributions presented in the study are included in the article/Supplementary Material, further inquiries can be directed to the corresponding author.
TG and RR: conceptualization. RR, MG, MA, RB, and TG: data curation. RR, AC, MG, and MA: formal analysis. TG: funding acquisition. RR and TG: writing – original draft. All authors reviewed and edited the manuscript.
The authors declare that the research was conducted in the absence of any commercial or financial relationships that could be construed as a potential conflict of interest.
All claims expressed in this article are solely those of the authors and do not necessarily represent those of their affiliated organizations, or those of the publisher, the editors and the reviewers. Any product that may be evaluated in this article, or claim that may be made by its manufacturer, is not guaranteed or endorsed by the publisher.
We thank the National Council for Scientific and Technological Development (Conselho Nacional de Desenvolvimento Científico e Tecnológico – CNPq) for the scholarship (RR and RB – process number 373092/2020-2); the Instituto Tecnologico Vale for the support and infrastructure; the São Paulo Research Support Foundation (Fundação de Amparo à Pesquisa do Estado de São Paulo – FAPESP 2004/15801-0) and the coordinators Vera Lucia Imperatriz-Fonseca and Astrid M. P. Kleinert for access to the data. We also thank Raphael Ligeiro and Willian Sabino for their suggestions on earlier versions of this study. We thank Fernando A. Silveira, Kirsten L. F. Haseyama, Alessandro Lima, and José Eustáquio Santos Junior from the entomological collection of Universidade Federal de Minas Gerais; Orlando Tobias from the Museu Paraense Emilio Goeldi; Isabel Alves dos Santos and Sheina Koffler from the Coleção Entomológica Paulo Nogueira Neto of the Universidade de São Paulo for allowing access to the bee specimens.
The Supplementary Material for this article can be found online at: https://www.frontiersin.org/articles/10.3389/fevo.2021.699649/full#supplementary-material
Aguiar, C. M. L. (1995). Abundância, diversidade e fenologia de abelhas (Hymenoptera - Apoidea) da caatinga (São João do Cariri, PB) e suas interações com a flora apícola. MSc thesis. João Pessoa, Brasil: Universidade Federal da Paraíba.
Aguiar, C. M. L. (2003). Utilização de recursos florais por abelhas (Hymenoptera: Apoidea) em uma área de Caatinga (Itatim, Bahia, Brasil). Revista Brasileira de Zool. 20, 457–467. doi: 10.1590/s0101-81752003000300015
Aguiar, C. M. L., and Zanella, F. C. V. (2005). Estrutura da comunidade de abelhas (Hymenoptera: Apoidea: Apiformis) de uma área na margem do domínio da Caatinga (Itatim, BA). Neotropical Entomol. 34, 15–24. doi: 10.1590/s1519-566x2005000100003
Aizen, M. A., Sabatino, M., and Tylianakis, J. M. (2012). Specialization and rarity predict nonrandom loss of interactions from mutualist networks. Science 335, 1486–1489. doi: 10.1126/science.1215320
Alves dos Santos, I. (2004). Biologia de nidificação de Anthodioctes megachiloides Holmberg (Anthidiini, Megachilidae, Apoidea). Revista Brasileira de Zool. 21, 739–744. doi: 10.1590/s0101-81752004000400002
Andena, S. R., Bego, L. R., and Mechi, M. R. (2005). As comunidades de abelhas (Hymenoptera, Apoidea) de uma área de cerrado (Corumbataí, SP) e suas visitas às flores. Revista Brasileira Zoociências 7, 55–91.
Armbruster, W. S. (2017). The specialization continuum in pollination systems: diversity of concepts and implications for ecology, evolution and conservation. Funct. Ecol. 31, 88–100. doi: 10.1111/1365-2435.12783
Barton, K. (2020). MuMIn: multi-model inference, R package. Available Online at: https://r-forge.r-project.org/projects/mumin/.
Bascompte, J., and Jordano, P. (2007). Plant-animal mutualistic networks: the architecture of biodiversity. Ann. Rev. Ecol. Evol. Syst. 38, 567–593. doi: 10.1146/annurev.ecolsys.38.091206.095818
Bascompte, J., Jordano, P., Melián, C. J., and Olesen, J. M. (2003). The nested assembly of plant–animal mutualistic networks. Proc. Natl. Acad. Sci. 100, 9383–9387. doi: 10.1073/pnas.1633576100
Bascompte, J., Jordano, P., and Olesen, J. M. (2006). Asymmetric coevolutionary networks facilitate biodiversity maintenance. Science 312, 431–433. doi: 10.1126/science.1123412
Baude, M., Kunin, W. E., Boatman, N. D., Conyers, S., Davies, N., Gillespie, M. A., et al. (2016). Historical nectar assessment reveals the fall and rise of floral resources in Britain. Nature 530, 85–88. doi: 10.1038/nature16532
Blomberg, S. P., Garland, T., and Ives, A. R. (2003). Testing for phylogenetic signal in comparative data: behavorial traits more labile. Evolution 5, 717–745. doi: 10.1554/0014-3820(2003)057[0717:tfpsic]2.0.co;2
Blüthgen, N., Menzel, F., and Blüthgen, N. (2006). Measuring specialization in species interaction networks. BMC Ecol. 6:9. doi: 10.1186/1472-6785-6-9
Boff, S., Araujo, A. C., and Pott, A. (2013). Bees (Hymenoptera: Apoidea) and flowers in natural forest patches of southern Pantanal. Biota Neotropica 13, 46–56. doi: 10.1590/s1676-06032013000400005
Bolker, B. M., Brooks, M. E., Clark, C. J., Geange, S. W., Poulsen, J. R., Stevens, M. H. H., et al. (2009). Generalized linear mixed models: a practical guide for ecology and evolution. Trends Ecol. Evol. 24, 127–135. doi: 10.1016/j.tree.2008.10.008
Bommarco, R., Biesmeijer, J. C., Meyer, B., Potts, S. G., Poyry, J., Roberts, S. P. M., et al. (2010). Dispersal capacity and diet breadth modify the response of wild bees to habitat loss. Proc. R. Soc. 277, 2075–2082. doi: 10.1098/rspb.2009.2221
Borges, R. C., Padovani, K., Imperatriz-Fonseca, V. L., and Giannini, T. C. (2020). A dataset of multi-functional ecological traits of Brazilian bees. Sci. Data 7, 120.
Brosi, B. J. (2016). Pollinator specialization: From the individual to the community. New Phytol. 210, 1190–1194. doi: 10.1111/nph.13951
Cane, J. (1987). Estimation of bee size using intertegular span (Apoidea). J. Kansas Entomol. Soc. 60, 145–147.
Cane, J. H. (2021). A brief review of monolecty in bees and benefits of a broadened definition. Apidologie 52, 17–22. doi: 10.1007/s13592-020-00785-y
Carvalho, C. A. L. (1993). Abelhas (Hymenoptera, Apoidea) no município Cruz das Almas - Bahia: levantamento, identificação e material coletado em plantas de importância econômica. MSc thesis. Salvador, Bahia: Universidade Federal da Bahia.
Carvalho, C. A. L. (1999). Diversidade de abelhas (Hymenoptera, Apoidea) e plantas visitadas no município de Castro Alves – BA. PhD thesis. Piracicaba, São Paulo: Universidade de São Paulo.
Chamberlain, S. A., and Holland, J. N. (2009). Body size predicts degree in ant–plant mutualistic networks. Funct. Ecolol. 23, 196–202. doi: 10.1111/j.1365-2435.2008.01472.x
Chamberlain, S. A., Kilpatrick, J. R., and Holland, J. N. (2010). Do extrafloral nectar resources, species abundances, and body sizes contribute to the structure of ant–plant mutualistic networks? Oecologia 164, 741–750. doi: 10.1007/s00442-010-1673-6
Chown, S. L., and Gaston, K. J. (2010). Body size variation in insects: a macroecological perspective. Biol. Rev. 85, 139–169. doi: 10.1111/j.1469-185x.2009.00097.x
Classen, A., Connal, D. E., Andreas, H., Peters, M. K., Peters, R. S., Ssymank, A., et al. (2020). Specialization of plant–pollinator interactions increase with temperature at Mt. Kilimanjaro. Ecol. Evol. 10, 2182–2195. doi: 10.1002/ece3.6056
Clavel, J., Julliard, R., and Devictor, V. (2011). Worldwide decline of specialist species, toward a global functional homogenization? Front. Ecol. Environ. 9, 222–228. doi: 10.1890/080216
Coelho, B. W. T. (2004). A review of the bee genus Augochlorella (Hymenoptera: Halictidae: Augochlorini). Syst. Entomol. 29, 282–323. doi: 10.1111/j.0307-6970.2004.00243.x
Cook, S. M., Awmack, C. S., Murray, D. A., and Williams, I. H. (2003). Are honey bees’ foraging preferences affected by pollen amino acid composition? Ecol. Entomol. 28, 622–627. doi: 10.1046/j.1365-2311.2003.00548.x
Cortopassi-Laurino, M., Knoll, F. R., and Imperatriz-Fonseca, V. L. (2003). “Nicho trófico e abundância de Bombus morio e Bombus atratus em diferentes biomas brasileiros,” in Apoidea Neotropica: Homenagem aos 90 anos de Jesus Santiago Moure, eds G. A. R. Melo and I. Alves-dos-Santos (Criciúma: Editora UNESC), 285–295.
Danforth, B. N., Sipes, S., Fang, J., and Brady, S. G. (2006). The history of early bee diversification based on five genes plus morphology. PNAS 103, 15118–15123. doi: 10.1073/pnas.0604033103
D’Avila, M. (2006). Insetos visitantes florais em áreas de cerradão e cerrado sensu stricto no Estado de São Paulo. PhD thesis. Piracicaba, São Paulo: Universidade de São Paulo.
Day, E. H., Hua, X., and Bromham, L. (2016). Is specialization an evolutionary dead end? Testing for differences in speciation, extinction and trait transition rates across diverse phylogenies of specialists and generalists. Evol. Biol. 29, 1257–1267. doi: 10.1111/jeb.12867
Diniz, M. E. R., and Buschini, M. L. T. (2015). Pollen analysis and interaction networks of floral visitor bees of Eugenia uniflora L. (Myrtaceae), in Atlantic Forest areas in southern Brazil. Arthropod-Plant Interac. 9, 623–632. doi: 10.1007/s11829-015-9400-1
Diniz-Filho, J. A. F., Bini, L. M., Rodríguez, M. A., Rangel, T. F. L. V. B., and Hawkins, B. A. (2007). Seeing the forest for the trees: partitioning ecological and phylogenetic components of Bergmann’s rule in European Carnivora. Ecography 30, 598–608. doi: 10.1111/j.0906-7590.2007.04988.x
Dorado, J., Vázquez, D. P., Stevani, E. L., and Chacoff, N. P. (2011). Rareness and specialization in plant–pollinator networks. Ecology 92, 19–25. doi: 10.1890/10-0794.1
Dormann, C. F. (2011). How to be a specialist? Quantifying specialisation in pollination networks. Network Biol. 1, 1–20.
Dormann, C. F. (2019). Using bipartite to describe and plot two-mode networks in R. Germany: University of Freiburg.
Ebeling, A., Klein, A. M., and Tscharntke, T. (2011). Plant–flower visitor interaction webs: temporal stability and pollinator specialization increases along an experimental plant diversity gradient. Basic Appl. Ecol. 12, 300–309. doi: 10.1016/j.baae.2011.04.005
Faith, D. P. (1992). Conservation evaluation and phylogenetic diversity. Biol. Conserv. 61, 1–10. doi: 10.1016/0006-3207(92)91201-3
Faria, G. M. (1994). A flora e a fauna apícola de um ecossistema de campo rupestre, Serra do Cipó – MG, Brasil: Composição, fenologia e suas interações. PhD thesis. São Paulo: Universidade de São Paulo.
Faria-Mucci, G. M., Melo, M. A., and Campos, L. A. O. (2003). “A fauna de abelhas (Hymenoptera, Apoidea) e plantas utilizadas como fonte de recursos florais, em um ecossistema de campos rupestres em Lavras Novas, Minas Gerais, Brasil,” in Apoidea Neotropica: homenagem aos 90 anos de Jesus de Santiago Moure, eds G. A. R. Melo and I. Alves-dos-Santos (Criciúma: Editora Unesc), 241–256.
Fenster, C. B., Armbruster, W. S., Wilson, P., Dudash, M. R., and Thomson, J. D. (2004). Pollination syndromes and floral specialization. Ann. Rev. Ecol. Evol. Syst. 35, 375–403.
Ferreira, P. A., Boscolo, D., Lopes, L. E., Carvalheiro, L. G., Biesmeijer, J. C., Rocha, P. L. B., et al. (2020). Forest and connectivity loss simplify tropical pollination networks. Oecologia 192, 577–590. doi: 10.1007/s00442-019-04579-7
Fournier, B., Mouly, A., Moretti, M., and Gillet, F. (2017). Contrasting processes drive alpha and beta taxonomic, functional and phylogenetic diversity of orthopteran communities in grasslands. Agric. Ecosyst. Environ. 242, 43–52. doi: 10.1016/j.agee.2017.03.021
Fründ, J., Linsenmair, K. E., and Blüthgen, N. (2010). Pollinator diversity and specialization in relation to flower diversity. Oikos 119, 1581–1590. doi: 10.1111/j.1600-0706.2010.18450.x
Gałecki, A., and Burzykowski, T. (2013). Linear mixed-effects models using R: A step-by-step approach. Berlin: Springer Science & Business Media, 557.
Gallai, N., Salles, J. M., Settele, J., and Vaissière, B. E. (2009). Economic valuation of the vulnerability of world agriculture confronted with pollinator decline. Ecol. Eco. 68, 810–821. doi: 10.1016/j.ecolecon.2008.06.014
Garibaldi, L. A., Aizen, M. A., Cunningham, S., and Klein, A. M. (2009). Pollinator shortage and global crop yield: looking at the whole spectrum of pollinator dependency. Commun. Integr. Biol. 2, 37–39. doi: 10.4161/cib.2.1.7425
Giannini, T. C., Garibaldi, L. A., Acosta, A. L., Silva, J. S., Maia, K. P., Saraiva, A. M., et al. (2015). Native and non-native supergeneralist bee species have different effects on plant-bee networks. PloS One 10:e0137198. doi: 10.1371/journal.pone.0137198
Giannini, T. C., Pinto, C. E., Acosta, A. L., Taniguchi, M., Saraiva, A. M., and Alves-dos-Santos, I. (2013). Interactions at large spatial scale: the case of Centris bees and floral oil producing plants in South America. Ecol. Model. 258, 74–81. doi: 10.1016/j.ecolmodel.2013.02.032
Gibson, M. R., Richardson, D. M., and Pauw, A. (2012). Can floral traits predict an invasive plant’s impact on native plant–pollinator communities? J. Ecol. 100, 1216–1223. doi: 10.1111/j.1365-2745.2012.02004.x
Gonçalves, L., and Buschini, M. L. T. (2017). Diversity of bees and their interaction networks with Ludwigia sericea (Cambessides) H. Hara and Ludwigia peruviana (L.) H. Hara (Onagraceae) flowers in a swamp area in the Brazilian Atlantic Foresteae) flowers in the Atlantic Forest Area in southern Brazil. Sociobiology 64, 57–68. doi: 10.13102/sociobiology.v64i1.1192
Gonçalves, R. B. (2016). A molecular and morphological phylogeny of the extant Augochlorini (Hymenoptera, Apoidea) with comments on implications for biogeography. Syst. Entomol. 41, 430–440. doi: 10.1111/syen.12166
Gonçalves, R. B. (2019). Phylogeny of the Augochlora clade with the description of four new species (Hymenoptera, Apoidea). Revista Brasileira de Entomol. 63, 91–100. doi: 10.1016/j.rbe.2018.12.003
González, A. M. M., Dalsgaard, B., and Olesen, J. M. (2010). Centrality measures and the importance of generalist species in pollination networks. Ecol. Complexity 7, 36–43. doi: 10.1016/j.ecocom.2009.03.008
González-Varo, J. P., Ortiz-Sánchez, F. J., and Vilà, M. (2016). Total bee dependence on one flower species despite available congeners of similar floral shape. PloS One 11:e0163122. doi: 10.1371/journal.pone.0163122
Gostinski, L. F., Carvalho, G. C. A., Rêgo, M. M. C., and Albuquerque, P. M. C. D. (2016). Species richness and activity pattern of bees (Hymenoptera, Apidae) in the restinga area of Lençóis Maranhenses National Park, Barreirinhas, Maranhão, Brazil. Revista Brasileira de Entomol. 60, 319–327. doi: 10.1016/j.rbe.2016.08.004
Greenleaf, S. S., Williams, N. M., Winfree, R., and Kremen, C. (2007). Bee foraging ranges and their relationship to body size. Oecologia 153, 589–596. doi: 10.1007/s00442-007-0752-9
Guimarães, P. R. Jr., Jordano, P., and Thompson, J. N. (2011). Evolution and coevolution in mutualistic networks. Ecol. Lett. 14, 877–885. doi: 10.1111/j.1461-0248.2011.01649.x
Guzman, A., Chase, M., and Kremen, C. (2019). On-Farm diversification in an agriculturally-dominated landscape positively influences specialist pollinators. Front. Sust. Food Syst. 3:87. doi: 10.3389/fsufs.2019.00087
Hallett, L. M., Diver, S., Eitzel, M. V., Olson, J. J., Ramage, B. S., Sardinas, H., et al. (2013). Do we practice what we preach? Goal setting for ecological restoration. Restor. Ecol. 21, 312–319. doi: 10.1111/rec.12007
Hoeksema, J. D., and Bruna, E. M. (2000). Pursuing the big questions about interspecific mutualism: a review of theoretical approaches. Oecologia 125, 321–330. doi: 10.1007/s004420000496
Hutchinson, M. C., Cagua, E. F., and Stouffer, D. B. (2017). Cophylogenetic signal is detectable in pollination interactions across ecological scales. Ecology 98, 2640–2652. doi: 10.1002/ecy.1955
Imperatriz-Fonseca, V. L., Alves-dos-Santos, I., de Souza Santos-Filho, P., Engels, W., Ramalho, M., Wilms, W., et al. (2011). Checklist das abelhas e plantas melitófilas no Estado de São Paulo, Brasil. Biota Neotropica 11, 1–25.
Irwin, R. E., Bronstein, J. L., Manson, J. S., and Richardson, L. (2010). Nectar robbing: ecological and evolutionary perspectives. Ann. Rev. Ecol. Evol. Syst. 41, 271–292. doi: 10.1146/annurev.ecolsys.110308.120330
Isaac, N. J. B., Turvey, S. T., Collen, B., Waterman, C., and Baillie, J. E. M. (2007). Mammals on the EDGE: conservation priorities based on threat and phylogeny. PLoS One 2:e296. doi: 10.1371/journal.pone.0000296
Janjic, J., and Packer, L. (2003). Phylogeny of the bee genus Agaspotemosn (Hymenopera: Halictdae). Syst. Entomol. 28, 101–123. doi: 10.1046/j.1365-3113.2003.00204.x
Johnson, L. K., and Hubbell, S. P. (1975). Contrasting foraging strategies and coexistence of two bee species on a single resource. Ecology 56, 1398–1406. doi: 10.2307/1934706
Kaiser-Bunbury, C. N., and Blüthgen, N. (2015). Integrating network ecology with applied conservation: a synthesis and guide to implementation. AoB PLANTS 7:lv076.
Kelly, T. T., and Elle, E. (2021). Investigating bee dietary preferences along a gradient of floral resources: how does resource use align with resource availability? Insect Sci. 28, 555–565. doi: 10.1111/1744-7917.12785
Kembel, S. W. (2020). Package ‘picante’. Available Online at https://cran.r-project.org/web/packages/picante/picante.pdf.
Klein, A. M., Vaissiere, B. E., Cane, J. H., Steffan-Dewenter, I., Cunningham, S. A., Kremen, C., et al. (2007). Importance of pollinators in changing landscapes for world crops. Proc. R. Soc. B Biol. Sci. 274, 303–313. doi: 10.1098/rspb.2006.3721
Krishna, A., Guimarães, P. R. Jr., Jordano, P., and Bascompte, J. (2008). A neutral-niche theory of nestedness in mutualistic networks. Oikos 117, 1609–1618. doi: 10.1111/j.1600-0706.2008.16540.x
Krzywinski, M. I., Schein, J. E., Birol, I., Connors, J., Gascoyne, R., Horsman, D., et al. (2009). Circos: An information aesthetic for comparative genomics. Genome Res. 19, 1639–1645. doi: 10.1101/gr.092759.109
Larsson, M. (2005). Higher pollinator effectiveness by specialist than generalist flower-visitors of unspecialized Knautia arvensis (Dipsacaceae). Oecologia 146, 394–403. doi: 10.1007/s00442-005-0217-y
Laura, A., Burkle, L. A., Marlin, J. C., and Knight, T. M. (2013). Plant-pollinator interactions over 120 Years: Loss of Species, Co-occurrence and function. Science 339, 1611–1615. doi: 10.1126/science.1232728
Leonhardt, S. D., and Blüthgen, N. (2012). The same, but different: pollen foraging in honeybee and bumblebee colonies. Apidologie 43, 449–464. doi: 10.1007/s13592-011-0112-y
Lever, J. J., van Nes, E. H., Scheffer, M., and Bascompte, J. (2014). The sudden collapse of pollinator communities. Ecol. Lett. 17, 350–359.
Lichtenberg, E. M., Imperatriz-Fonseca, V. L., and Nieh, J. C. (2010). Behavioral suites mediate group-level foraging dynamics in communities of tropical stingless bees. Insectes Sociaux 57, 105–113. doi: 10.1007/s00040-009-0055-8
Lima, M. F. C. (2004). Comunidade de abelhas, nidificação de abelhas solitárias em cavidades preexistentes (Hymenoptera: Apoidea) e interação abelha-planta na Reserva Biológica Guaribas, Mamanguape, Paraíba, Brasil. PhD thesis. João Pessoa, Paraíba: Universidade Federal da Paraíba.
Machado, I. C. (2004). Oil-collecting bees and related plants: a review of the studies in the last twenty years and case histories of plants occurring in NE Brazil. Solitary bees, conservation, rearing and management for pollination. Fortaleza, BR: Editora Universitária, 255–280.
Maia, K. P., Rasmussen, C., Olesen, J. M., and Guimarães, P. R. (2019). Does the sociality of pollinators shape the organisation of pollination networks? Oikos 128, 741–752. doi: 10.1111/oik.05387
Martins, A. C., Melo, G. A., and Renner, S. S. (2015). Gain and loss of specialization in two oil-bee lineages, Centris and Epicharis (Apidae). Evolution 69, 1835–1844. doi: 10.1111/evo.12689
Mateus, S. (1998). Abundância relativa, fenologia e visita às flores pelos Apoidea do cerrado da Estação Ecológica de Jataí - Luiz Antônio – SP. MSc thesis. Ribeirão Preto, São Paulo: Universidade de São Paulo.
Mello, M. A., Bezerra, E. L., and Machado, I. C. (2013). Functional roles of centridini oil bees and malpighiaceae oil flowers in biome-wide pollination networks. Biotropica 45, 45–53. doi: 10.1111/j.1744-7429.2012.00899.x
Mello, M. A., Muylaert, R. L., Pinheiro, R. B. P., and Ferreira, G. M. F. (2016). Guia para análise de redes ecológicas. Belo Horizonte: 112.
Montoya, D., Rogers, L., and Memmott, J. (2012). Emerging perspectives in the restoration of biodiversity-based ecosystem services. Trends Ecol. Evol. 27, 666–672. doi: 10.1016/j.tree.2012.07.004
Montoya, J. M., Pimm, S. L., and Solé, R. V. (2006). Ecological networks and their fragility. Nature 442:259. doi: 10.1038/nature04927
Mouga, D. M. D., Nogueira-Neto, P., Warkentin, M., Feretti, V., and Dec, E. (2015). Comunidade de abelhas (Hymenoptera, Apidae) e plantas associadas em área de mata atlântica em São Francisco do Sul, Santa Catarina, Brasil. Acta Biol. Catarinense 2, 12–31.
Nogueira-Ferreira, F. H., and Augusto, S. C. (2007). Amplitude de nicho e similaridade no uso de recursos florais por abelhas eussociais em uma área de cerrado. Biosci. J. 23, 45–51.
Olesen, J. M., Bascompte, J., Dupont, Y. L., and Jordano, P. (2007). The modularity of pollinator networks. Proc. Natl. Acad. Sci. USA. 104, 19891–19896.
Orr, M. C., Hughes, A. C., Chesters, D., Pickering, J., Zhu, C. D., and Ascher, J. S. (2021). Global patterns and drivers of bee distribution. Curr. Biol. 31, 451–458. doi: 10.1016/j.cub.2020.10.053
Pacheco-Filho, A. J. S., Verola, C. F., Verde, L. W. L., and Freitas, B. M. (2015). Bee-flower association in the Neotropics: implications to bee conservation and plant pollination. Apidologie 46, 530–541. doi: 10.1007/s13592-014-0344-8
Pagel, M. (1997). Inferring evolutionary processes from phylogenies. Zool. Scripta 26, 331–348. doi: 10.1111/j.1463-6409.1997.tb00423.x
Pagel, M. (1999). Inferring the historical patterns of biological evolution. Nature 401:877. doi: 10.1038/44766
Pallarés, S., Lai, M., Abellán, P., Ribera, I., and Sánchez-Fernández, D. (2019). An interspecific test of Bergmann’s rule reveals inconsistent body size patterns across several lineages of water beetles (Coleoptera: Dytiscidae). Ecol. Entomol. 44, 249–254. doi: 10.1111/een.12701
Pedro, S. E. M. (1992). Sobre as abelhas (Hymenoptera, Apoidea) em um ecossistema de cerrado (Cajuru, NE do Estado de São Paulo): composição, fenologia e visita às flores. MSc thesis. Ribeirão Preto, São Paulo: Universidade de São Paulo.
Peralta, G., Stouffer, D. B., Bringa, E. M., and Vázquez, D. P. (2020). No such thing as a free lunch: interaction costs and the structure and stability of mutualistic networks. Oikos 129, 503–511. doi: 10.1111/oik.06503
Petanidou, T., Kallimanis, A. S., Tzanopoulos, J., Sgardelis, S. P., and Pantis, J. D. (2008). Long-term observation of a pollination network: fluctuation in species and interactions, relative invariance of network structure and implications for estimates of specialization. Ecol. Lett. 11, 564–575. doi: 10.1111/j.1461-0248.2008.01170.x
Popic, T. J., Wardle, G. M., and Davila, E. C. (2013). Flower-visitor networks only partially predict the function of pollen transport by bees. Aus. Ecol. 38, 76–86. doi: 10.1111/j.1442-9993.2012.02377.x
Portman, Z. M., Orr, M. C., and Griswold, T. (2019). A review and updated classification of pollen gathering behavior in bees (Hymenoptera, Apoidea). J. Hymenoptera Res. 71, 171–208. doi: 10.3897/jhr.71.32671
Potts, S. G., Biesmeijer, J. C., Kremen, C., Neumann, P., Schweiger, O., and Kunin, W. E. (2010). Global pollinator declines: trends, impacts and drivers. Trends Ecol. Evol. 25, 345–353. doi: 10.1016/j.tree.2010.01.007
Potts, S. G., Imperatriz-Fonseca, V., Ngo, H. T., Aizen, M. A., Biesmeijer, J. C., Breeze, T. D., et al. (2016). Safeguarding pollinators and their values to human well-being. Nature 540, 220–229. doi: 10.1038/nature20588
R Core Team. (2021). R: A language and environment for statistical computing. Vienna, Austria: R Foundation for Statistical Computing.
Rabelo, L. S., Vilhena, A. M., Bastos, E. M., Aguiar, C. M., and Augusto, S. C. (2015). Oil-collecting bee–flower interaction network: do bee size and anther type influence the use of pollen sources? Apidologie 46, 465–477. doi: 10.1007/s13592-014-0336-8
Rabelo, L. S., Vilhena, A. M. G. F., Bastos, E. M. A. F., and Augusto, S. C. (2014). Differentiated use of pollen sources by two sympatric species of oil-collecting bees (Hymenoptera: Apidae). J. Nat. His. 48, 1595–1609. doi: 10.1080/00222933.2014.886342
Ramírez, S. R., Eltz, T., Fujiwara, M. K., Gerlach, G., Goldman-Huertas, B., Tsutsui, N. D., et al. (2011). Asynchronous diversification in a specialized plant-pollinator mutualism. Science 333, 1742–1746.
Ramírez, S. R., Roubik, D. W., Skov, C., and Pierce, N. E. (2010). Phylogeny, diversification patterns and historical biogeography of euglossine orchid bees (Hymenoptera: Apidae). Biol. J. Linnean Soc. 100, 552–572.
Rêgo, M. M. C. (1998). Abelhas silvestres (Hymenoptera, Apoidea) em um ecossistema de cerrado sl (Chapadinha – MA, Brasil): uma abordagem biocenótica. PhD thesis. Ribeirão Preto, São Paulo: Universidade de São Paulo.
Revell, L. J. (2021). Phylogenetic Tools for Comparative Biology (and Other Things). Available Online at https://github.com/liamrevell/phytools.
Rezende, E. L., Jordano, P., and Bascompte, J. (2007a). Effects of phenotypic complementarity and phylogeny on the nested structure of mutualistic networks. Oikos 116, 1919–1929. doi: 10.1111/j.0030-1299.2007.16029.x
Rezende, E. L., Lavabre, J. E., Guimarães, P. R., Jordano, P., and Bascompte, J. (2007b). Non-random coextinctions in phylogenetically structured mutualistic networks. Nature 448:925. doi: 10.1038/nature05956
Romero, D., Ornosa, C., Vargas, P., and Olesen, J. M. (2020). Solitary bees (Hymenoptera, Apoidea) as connectors in pollination networks: the case of Rhodanthidium. Apidologie 51, 844–854. doi: 10.1007/s13592-020-00765-2
Sabino, W. O., Alves-dos-Santos, I., and da Silva, C. I. (2019). Versatility of the trophic niche of Centris (Paracentris) burgdorfi (Apidae, Centridini). Arthropod-Plant Interact. 13, 227–237. doi: 10.1007/s11829-018-9654-5
Sáez, A., Morales, C. L., Garibaldi, L. A., and Aizen, M. A. (2017). Invasive bumble bees reduce nectar availability for honey bees by robbing raspberry flower buds. Basic Appl. Ecol. 19, 26–35. doi: 10.1016/j.baae.2017.01.001
Sakagami, S., Laroca, S., and Moure, J. (1967). Wild bee biocenotics in São José dos Pinhais (PR), South Brazil, preliminary report. J. Faculty Sci. Hokkaido Univ. 16, 253–291.
Schemske, D. W., and Bradshaw, H. D. Jr. (1999). Pollinator preference and the evolution of floral traits in monkeyflowers (Mimulus). PNAS 96, 11910–11915. doi: 10.1073/pnas.96.21.11910
Schleuning, M., Blüthgen, N., Flörchinger, M., Braun, J., Schaefer, H. M., and Böhning-Gaese, K. (2011). Specialization and interaction strength in a tropical plant–frugivore network differ among forest strata. Ecology 92, 26–36. doi: 10.1890/09-1842.1
Schlindwein, C. (1998). Frequent oligolecty characterizing a diverse bee–plant community in a xerophytic bushland of subtropical Brazil. Stud. Neotropical Fauna Environ. 33, 46–59. doi: 10.1076/snfe.33.1.46.2168
Seitz, N., vanEngelsdorp, D., and Leonhardt, S. D. (2020). Are native and non-native pollinator friendly plants equally valuable for native wild bee communities? Ecol. Evol. 10, 12838–12850. doi: 10.1002/ece3.6826
Silva, M. C. M. (1998). Estrutura da comunidade de abelhas (Hymenoptera, Apoidea) de uma área de restinga (Praia de Intermares, Cabedelo, Paraíba, Nordeste do Brasil). MSc thesis. João Pessoa, Paraíba: Universidade Federal da Paraíba.
Silva, M. C. M., and Martins, C. F. (1999). Flora apícola e relações tróficas de abelhas (Hymenoptera, Apoidea) em uma área de restinga (Praia de Intermares, Cabedelo - PB, Brasil). Principia 7, 40–51.
Silveira, M. S. (2006). Fauna de abelhas (Hymenoptera, Apoidea, Apiformes) e recursos florais utilizados em áreas urbanas e no entorno da cidade de João Pessoa, PB. MSc thesis. João Pessoa, Paraíba: Universidade Federal da Paraíba.
Smith, C., Weinman, L., Gibbs, J., and Winfree, R. (2019). Specialist foragers in forest bee communities are small, social or emerge early. J. Anim. Ecol. 88, 1158–1167. doi: 10.1111/1365-2656.13003
Smith, F. A., Brown, J. H., Haskell, J. P., Lyons, S. K., Alroy, J., Charnov, E. L., et al. (2004). Similarity of mammalian body size across the taxonomic hierarchy and across space and time. Am. Nat. 163, 672–691. doi: 10.1086/382898
Thompson, J. N. (2005). The geographic mosaic of coevolution. Chicago: University of Chicago Press, 443.
Vanbergen, A. J., Woodcock, B. A., Gray, A., Grant, F., Telford, A., Lambdon, P., et al. (2014). Grazing alters insect visitation networks and plant mating systems. Funct. Ecol. 28, 178–189. doi: 10.1111/1365-2435.12191
Vargas, P., Liberal, I., Ornosa, C., and Gomez, J. M. (2017). Flower specialisation: the occluded corolla of snapdragons (Antirrhinum) exhibits two pollinator niches of large long-tongued bees. Plant Biol. 19, 787–797. doi: 10.1111/plb.12588
Vaudo, A. D., Patch, H. M., Mortensen, D. A., Tooker, J. F., and Grozinger, C. M. (2016). Macronutrient ratios in pollen shape bumble bee (Bombus impatiens) foraging strategies and floral preferences. PNAS 113, 4035–4042.
Vázquez, D. P., and Aizen, M. A. (2003). Null model analyses of specialization in plant-pollinator interactions. Ecology 84, 2493–2501. doi: 10.1890/02-0587
Vázquez, D. P., Chacoff, N. P., and Cagnolo, L. (2009). Evaluating multiple determinants of the structure of plant-animal mutualistic networks. Ecology 90, 2039–2046. doi: 10.1890/08-1837.1
Vázquez, D. P., Melián, C. J., Williams, N. M., Blüthgen, N., Krasnov, B. R., and Poulin, R. (2007). Species abundance and asymmetric interaction strength in ecological networks. Oikos 116, 1120–1127. doi: 10.1111/j.0030-1299.2007.15828.x
Vázquez, D. P., Morris, W. F., and Jordano, P. (2005). Interaction frequency as a surrogate for the total effect of animal mutualists on plants. Ecol. Lett. 8, 1088–1094. doi: 10.1111/j.1461-0248.2005.00810.x
Vázquez, D. P., and Simberloff, D. (2002). Ecological specialization and susceptibility to disturbance: Conjectures and refutations. Am. Nat. 159, 606–623. doi: 10.1086/339991
Veron, S., Davies, J., Cadotte, M. W., Clergeau, P., and Pavoine, S. (2017). Predicting loss of evolutionary history: Where are we? Biol. Rev. 92, 271–291. doi: 10.1111/brv.12228
Villalobos, S., Sevenello-Montagner, J. M., and Vamosi, J. C. (2019). Specialization in plant–pollinator networks: insights from local-scale interactions in Glenbow Ranch Provincial Park in Alberta, Canada. BMC Ecol. 19:34. doi: 10.1186/s12898-019-0250-z
Wcislo, W. T., and Cane, J. H. (1996). Floral resource utilization by solitary bees (Hymenoptera: Apoidea) and exploitation of their stored foods by natural enemies. Ann. Rev. Entomol. 41, 257–286. doi: 10.1146/annurev.en.41.010196.001353
Webb, C. O., Ackerly, D. D., and Kembel, S. W. (2008). Phylocom: software for the analysis of phylogenetic community structure and character evolution. Bioinformatics 24, 2098–2100. doi: 10.1093/bioinformatics/btn358
Webb, C. O., and Donoghue, M. J. (2005). Phylomatic: tree assembly for applied phylogenetics. Mol. Ecol. 5, 181–183.
Weiner, C. N., Werner, M., Linsenmair, K. E., and Blüthgen, N. (2014). Land-use impacts on plant–pollinator networks: interaction strength and specialization predict pollinator declines. Ecology 95, 466–474.
Keywords: pollinators, mutualism, trophic niche, response traits, phylogeny, biodiversity, conservation
Citation: Raiol RL, Gastauer M, Campbell AJ, Borges RC, Awade M and Giannini TC (2021) Specialist Bee Species Are Larger and Less Phylogenetically Distinct Than Generalists in Tropical Plant–Bee Interaction Networks. Front. Ecol. Evol. 9:699649. doi: 10.3389/fevo.2021.699649
Received: 23 April 2021; Accepted: 05 July 2021;
Published: 27 July 2021.
Edited by:
Maria Augusta Lima, Universidade Federal de Viçosa, BrazilReviewed by:
Pablo Vargas, Consejo Superior de Investigaciones Científicas (CSIC), SpainCopyright © 2021 Raiol, Gastauer, Campbell, Borges, Awade and Giannini. This is an open-access article distributed under the terms of the Creative Commons Attribution License (CC BY). The use, distribution or reproduction in other forums is permitted, provided the original author(s) and the copyright owner(s) are credited and that the original publication in this journal is cited, in accordance with accepted academic practice. No use, distribution or reproduction is permitted which does not comply with these terms.
*Correspondence: Tereza Cristina Giannini, dGVyZXphLmdpYW5uaW5pQGl0di5vcmc=
Disclaimer: All claims expressed in this article are solely those of the authors and do not necessarily represent those of their affiliated organizations, or those of the publisher, the editors and the reviewers. Any product that may be evaluated in this article or claim that may be made by its manufacturer is not guaranteed or endorsed by the publisher.
Research integrity at Frontiers
Learn more about the work of our research integrity team to safeguard the quality of each article we publish.