- 1Evolutionary Studies Institute and School of Geosciences, University of the Witwatersrand, Johannesburg, South Africa
- 2Karoo Palaeontology, Iziko South African Museum, Cape Town, South Africa
Despite their significance for paleobiological interpretations, bite marks have been rarely reported in non-mammalian therapsids (NMT). Here we describe, for the first time, the occurrence of a tooth embedded in the snout of a gorgonopsian. The tooth is surrounded by a bony callus, which demonstrates that the animal was still alive after the attack and healed. The identity of the attacker is unknown. Two hypotheses are discussed to account for this healed bite: failed predation (most likely by a biarmosuchian, therocephalian, or another gorgonopsian) and intraspecific social biting. Though predation cannot be ruled out, it has been hypothesized that gorgonopsians used their saber-like teeth for social signaling, which suggests that social biting may be the most likely scenario. The practice of social biting has long been hypothesized in NMT, but this is the first fossilized evidence of the behavior to be described.
Introduction
The paleopathological study of extinct species is the gateway to many, otherwise inaccessible, paleobiological traits such as intraspecific behavior (Farke et al., 2009; Peterson and Vittore, 2012; Peterson et al., 2013), diet and food acquisition (DePalma et al., 2013), etiology and healing capabilities (Wolff et al., 2009; Kato et al., 2020), soft tissue reconstruction (Rega et al., 2012), thermophysiology (Benoit et al., 2015), interspecific interactions (Aureliano et al., 2021), biting force (Erickson et al., 1996; Gignac et al., 2010), and even key transitional events in the evolutionary history of vertebrates such as the onset of arboreality in hominins and terrestriality in early tetrapods (Bishop et al., 2015; Kappelman et al., 2016).
Published paleopathology literature on non-mammalian therapsids (NMT) is surprisingly scarce (Jinnah and Rubidge, 2007; Fröbisch and Reisz, 2008; Budziszewska-Karwowska et al., 2010; Fordyce et al., 2012; Vega and Maisch, 2014; Benoit et al., 2015; Whitney et al., 2017, 2019; Kato et al., 2020) despite the demonstrated species richness, abundance, and extensive spatial distribution of these animals in the fossil record (Ivakhnenko, 2008; Nicolas and Rubidge, 2010; Angielczyk and Kammerer, 2018). In contrast, osteopathologies are well documented in dinosaurs, crocodilians, mosasaurs, non-therapsid pelycosaurs, etc. (Buffetaut, 1983; Boucot, 1990; Rothschild and Martin, 1993; Mackness and Sutton, 2000; Tanke and Rothschild, 2002; Katsura, 2004; Lingham-Soliar, 2004; Reisz and Tsuji, 2006; Boucot and Poinar, 2011; Zammit and Kear, 2011; Rega et al., 2012; Bakker et al., 2015; Bastiaans et al., 2020), and the existence of paleopathologies in contemporaneous species is attested (Fernandez et al., 2013). Bite marks, in particular, have been documented in countless fossil mammals (e.g., Scott and Jepsen, 1936; Tanke and Currie, 1998; Chimento et al., 2019), pelycosaurs (Reisz and Tsuji, 2006; Bakker et al., 2015), and outside synapsids in, e.g., dinosaurs, crocodilians, mosasaurs, ichthyosaurs (Buffetaut, 1983; Boucot, 1990; Rothschild and Martin, 1993; Tanke and Currie, 1998; Mackness and Sutton, 2000; Tanke and Rothschild, 2002; Avilla et al., 2004; Katsura, 2004; Lingham-Soliar, 2004; Zammit and Kear, 2011; Bastiaans et al., 2020), but remain rarely reported in NMT. So far, bite marks have been described on the surface of the bones of two dicynodonts (Budziszewska-Karwowska et al., 2010; Fordyce et al., 2012) and one titanosuchid dinocephalian (Shelton et al., 2019). These bites have been attributed to a gorgonopsian (Fordyce et al., 2012), an anteosaur (Shelton et al., 2019), and unknown carnivores (Budziszewska-Karwowska et al., 2010). To find a tooth still embedded in bone is even rarer and extremely significant for paleobiological interpretations (Buffetaut et al., 2004; Bell and Currie, 2010; DePalma et al., 2013; Drumheller et al., 2014; Konishi and Brinkman, 2016), but such an occurrence has never been reported in NMT.
Here we report, for the first time, the preservation of a tooth found embedded in a callus within the bone of the snout of a gorgonopsian from South Africa, and discuss its paleobiological implications.
Materials and Methods
The embedded tooth was found in specimen SAM-PK-11490 (Figure 1). The specimen is an isolated and mostly unprepared snout of a gorgonopsian found on farm Mynhardskraal (Beaufort West District) by Dr. Lieuwe D. Boonstra in 1940. Biostratigraphically, it is from the Tapinocephalus Assemblage Zone of the Beaufort Group of the Karoo Supergroup (South Africa), which correlates with the Guadalupian epoch of the Permian period (Day and Rubidge, 2020). The snout was attributed to Arctognathus cf. curvimola by Sigogneau (1970) but was then re-identified as Gorgonopsia indet. by Kammerer et al. (2015). We maintain the identification of this specimen as Gorgonopsia indet. given: (i) that the sample preserves a fragment of the snout only, (ii) the unprepared state of the specimen, and (iii) the currently unclear number of postcanine teeth [four according to Kammerer et al. (2015), but five according to Sigogneau (1970), although ongoing preparation of the specimen appears to support that four is the correct number, see Figure 1], this last character being important for taxonomic identification. The presence of an embedded tooth in this specimen was not reported during these previous taxonomic assessments.
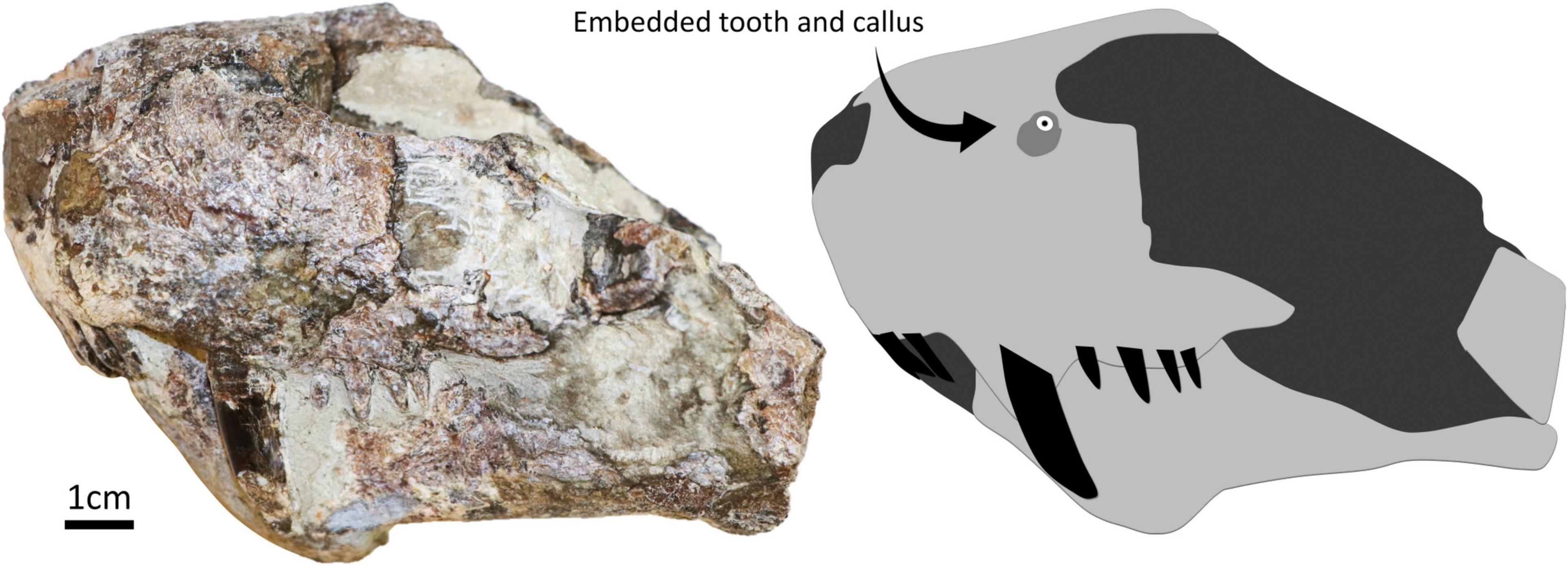
Figure 1. Specimen SAM-PK-11490 in left lateral with a simplified diagram showing the position of the embedded tooth and surrounding callus.
Institutional Abbreviations
MCA, Museo “Carlos Ameghino,” Mercedes, Buenos Aires province, Argentina; SAM, Iziko South African Museum, Cape Town, South Africa; SDSM, South Dakota School of Mines and Technology, Museum of Geology, Rapid City, SD, United States.
Description
The embedded tooth is located on the dorsal half of the left side of the snout, above the maxillary canine and approximately at the same height as the naris (Figure 2). The visible cross-section of the tooth is circular except for the presence of a carina (but this feature may be exaggerated due to the displacement of a portion of the tooth as it is heavily fractured). The tooth section measures 2.1 mm across, and the pulp cavity is exposed. The embedded tooth is surrounded by a porous, raised, and dorso-ventrally oblong callus of bone (Figure 2), which conforms to the cicatricial osseous tissue described in most tetrapods (Boucot, 1990; Rothschild and Martin, 1993; Tanke and Rothschild, 2002; Bishop et al., 2015) including gorgonopsians and other NMT (Fordyce et al., 2012; Kato et al., 2020). The callus measures 6.5 mm long and 8.5 mm wide. Its surface is porous, but no drainage canal for pus is visible. This feature is thus interpreted as a callus resulting from the healing of the bone around the tooth after it broke during a bite and remained embedded in the bone. Noticeably, the callus is not limited to the immediate surrounding of the tooth but is expanded laterally, which indicates that the bone in this area was damaged too (Figure 2). This suggests that the axis of the tooth row that inflicted the damage was oriented obliquely, almost perpendicular to the snout (Figure 3), so that the rest of the tooth row produced more superficial bone damages next to the embedded tooth. This is consistent with the position and orientation of the carina on the embedded tooth (assuming that the carina was pointing distally as in most carnivorous species). No exostosis indicative of bone infection is visible.
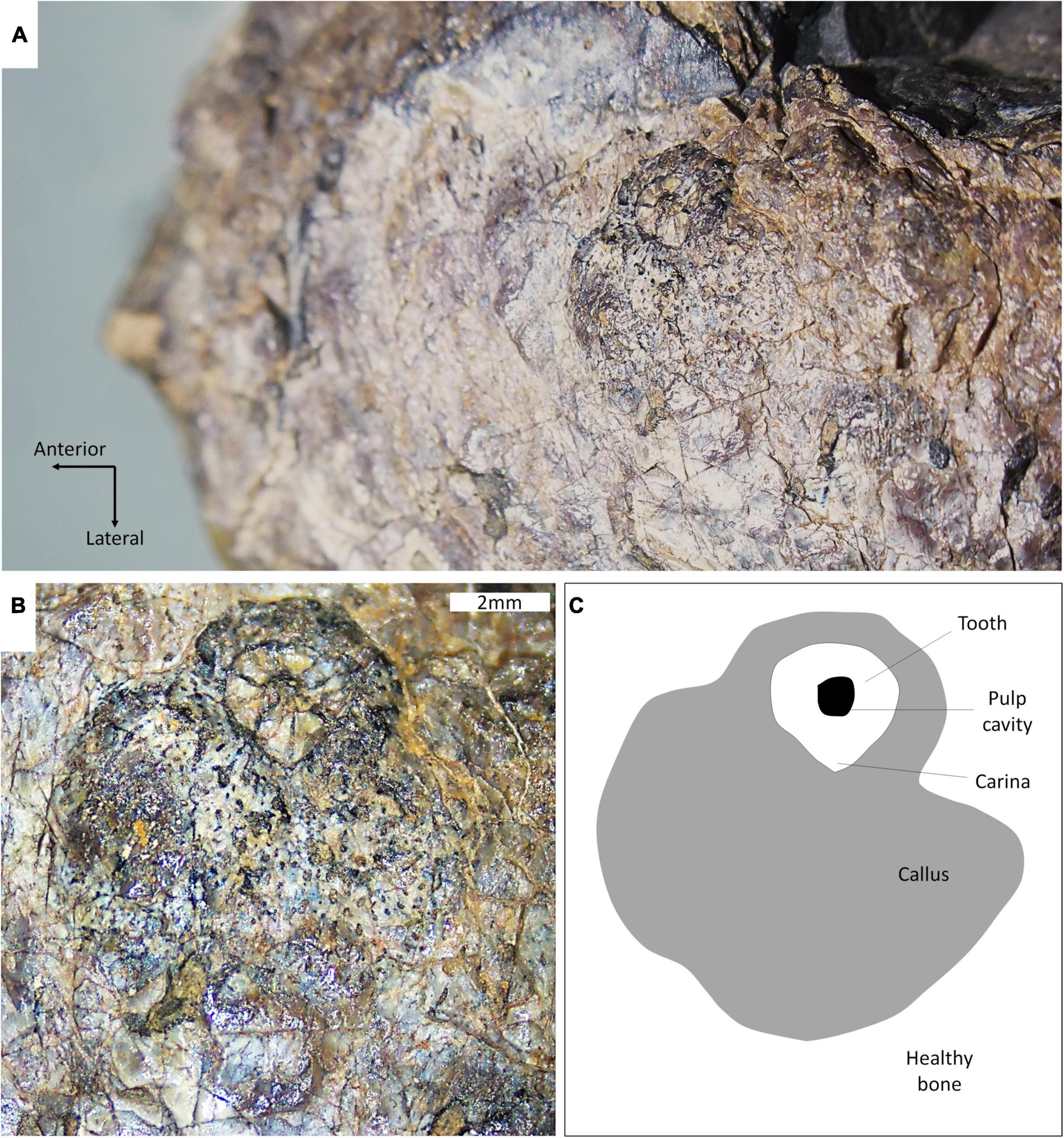
Figure 2. Close-up views of the embedded tooth and callus of SAM-PK-11490. (A) Dorsolateral view of the tooth and callus; (B) lateral view of the tooth and callus; and (C) interpretive drawing of (B).
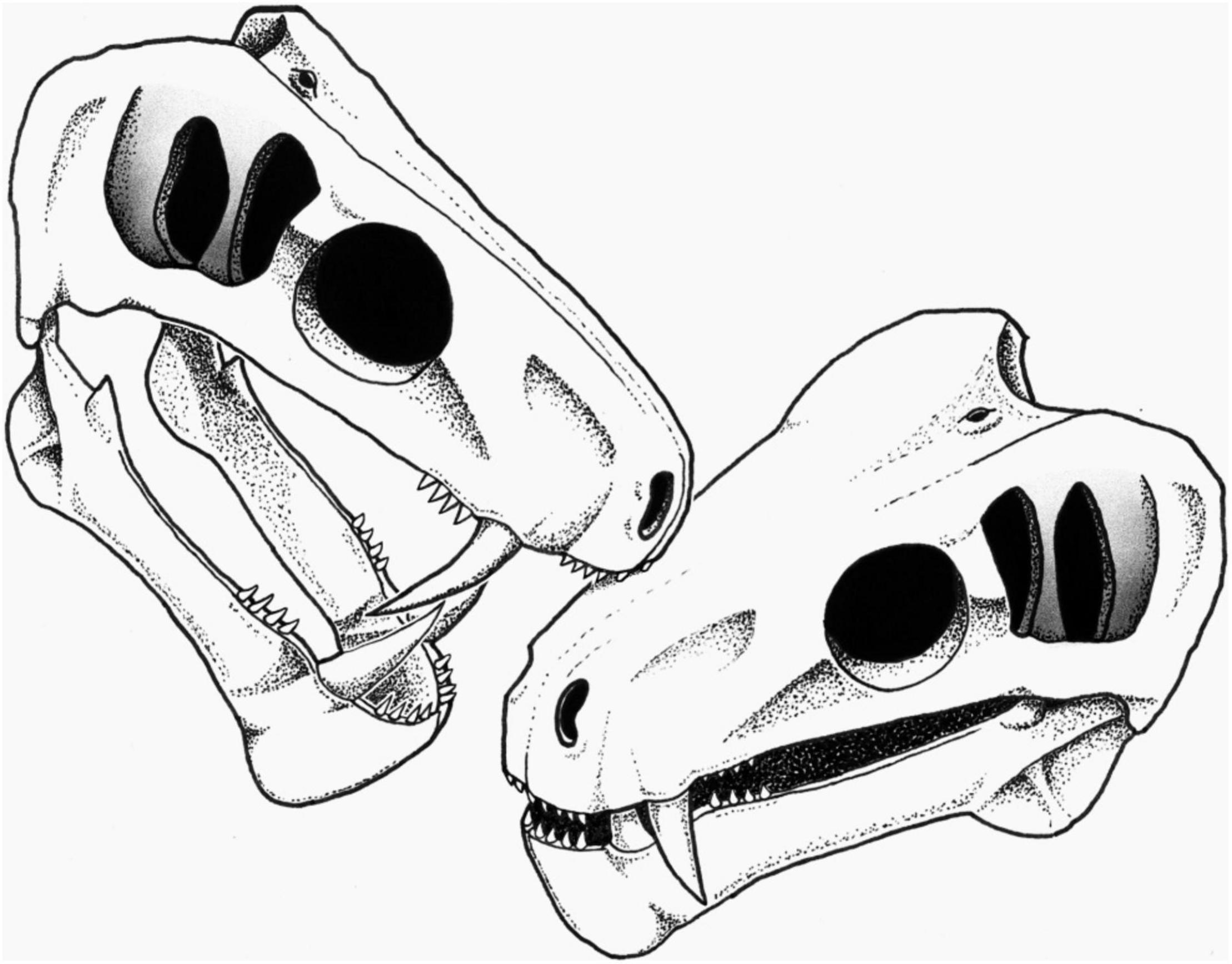
Figure 3. Reconstruction of the biting action by a conspecific leading to the embedded tooth on the snout of SAM-PK-11490 (see section “Discussion”). Artist: S. Vrard.
Discussion
The presence of a callus around the embedded tooth clearly distinguishes SAM-PK-11490 from cases of post-mortem bites (e.g., Bell and Currie, 2010; Fordyce et al., 2012) as it unmistakably indicates that healing of the periosteum took place after the tooth broke-off. The raised rim around the tooth provides direct evidence for the growth of a callus in response to the penetration of the tooth (Figure 2). The vascularized surface and slightly elevated aspect of the callus observed in SAM-PK-11490 conforms well with healed bite marks documented elsewhere in other tetrapods (Scott and Jepsen, 1936; Lingham-Soliar, 2004; Zammit and Kear, 2011; DePalma et al., 2013; Chimento et al., 2019). This strongly supports that the gorgonopsian was alive when it was bitten and survived the bite. This is extremely significant as only post-mortem damage (Budziszewska-Karwowska et al., 2010; Fordyce et al., 2012), bone infection caused by a bite (Shelton et al., 2019), or bone diseases unrelated to bites (Vega and Maisch, 2014; Kato et al., 2020) have been described in NMT to date.
The presence of a callus suggests that the gorgonopsian was bitten 2–9 weeks before it died based on mammalian healing capabilities (Lovell, 1997; Lingham-Soliar, 2004). The absence of a drainage channel for pus or any other trace of infection suggests that an infection resulting from the bite was likely not the cause of death.
In living animals, bites can be inflicted either during an act of predation or for social signaling (Poole, 1985; Tanke and Currie, 1998). Therefore, the two hypotheses that may account for the circumstances that led to the condition in SAM-PK-11490 are attempted predation or social signaling. Regarding the predation hypothesis, specimen SAM-PK-11490 is a small to medium sized gorgonopsian. The snout is only 54 mm wide at the canines, making it similar in size to the contemporaneous Eriphostoma microdon, which is generally recognized as a small gorgonopsian (Kammerer et al., 2015). A wide variety of possible predators large enough to attack such a species populated the Tapinocephalus Assemblage Zone fauna of the South African Karoo, including large biarmosuchians, therocephalians (scylacosaurid and lycosuchids), anteosaurs, and rhinesuchid amphibians (Day and Rubidge, 2020). Attempted cannibalism or attack by another gorgonopsian are not excluded. A rhinesuchid attacker can be excluded as the embedded tooth does not display the typical labyrinthodont pattern of plicidentine (Fortuny and Steyer, 2019) encountered in the sectioned teeth of these amphibians (Figure 2). The tooth being carinated, the bite of a prey (such as an anomodont) defending itself appears unlikely. Biarmosuchians, gorgonopsians, and therocephalians would be more likely compared to anteosaurs as the latter would have had large teeth and a crushing bite (Kammerer, 2011), not compatible with the small size of the embedded tooth and light damage observed in SAM-PK-11490. The cross-section of the anterior-most incisors is generally circular in biarmosuchians, gorgonopsians, and therocephalians (Sigogneau, 1970; Mendrez, 1975), which suggests the embedded tooth is either a lateral incisor, a canine, or a postcanine tooth. The very small size of the tooth would exclude a canine, although it could be the very tip of a canine; but this too is unlikely, given that the pulp cavity is visible (Figure 2). The lateral incisors and postcanine teeth can bear serrations on their posterior face and appear carinated in cross-section in biarmosuchians, gorgonopsians, and therocephalians (Sigogneau, 1970; Mendrez, 1975; Rubidge and Kitching, 2003; Abdala et al., 2008). It is thus likely that the embedded tooth is a lateral incisor or postcanine that belongs to a biarmosuchian, gorgonopsian, or a therocephalian (scylacosaurid or lycosuchid).
The second hypothesis is intraspecific social biting. Social biting occurs in many modern species (reptiles and mammals, particularly carnivores) to assert dominance, stimulate copulation and ovulation, compete for mates, territory and breeding rights, or simply as juvenile play biting (Poole, 1985; Tanke and Currie, 1998; Cabrera and Stankowich, 2018; Chimento et al., 2019; although, play biting can be safely excluded as this behavior is limited to juvenile individuals and the dimensions of SAM-PK-11490 corresponds to an adult-sized middle Permian gorgonopsian).
Social biting has been suggested in many extinct species, including theropod dinosaurs, aquatic reptiles, and saber-toothed cats (Scott and Jepsen, 1936; Buffetaut, 1983; Tanke and Currie, 1998; Avilla et al., 2004; Katsura, 2004; Everhart, 2008; Peterson et al., 2009; Wolff et al., 2009; Bell and Currie, 2010; Zammit and Kear, 2011; Chimento et al., 2019), and has also long been hypothesized in NMT (Geist, 1972; Barghusen, 1975; Benoit et al., 2016, 2021), but evidence was lacking. As stated above, the embedded tooth is likely a lateral incisor or postcanine of another carnivorous NMT. Its diameter is consistent with the size of the incisors and postcanines of specimen SAM-PK-11490, making a conspecific individual a possible candidate as the attacker (Figure 3).
The possibility that the embedded tooth belongs to a biarmosuchian, a therocephalian, or another species of gorgonopsian cannot be ruled out; however, intraspecific social biting is here preferred over predation to account for the condition in SAM-PK-11490 for three reasons. Firstly, the position of the embedded tooth is consistent with face biting. Face biting is a particular kind of social biting during which modern amphibians (e.g., Ceratophrys), reptiles (e.g., Varanus), and mammals (e.g., Panthera) bite each others’ head during more or less ritualized intraspecific combat to assert dominance and secure mates, territory, or breeding rights (Tanke and Currie, 1998; Chimento et al., 2019). Secondly, the non-lethality of the bite is an expected outcome of social biting (Geist, 1966). Lethal intraspecific combat would reduce population size and attract predators and scavengers (Geist, 1966; Lorenz, 1966; Cabrera and Stankowich, 2018), and as such, social biting is meant to signal dominance and power, or stimulate breeding rather than to injure or kill a member of the same species. Accordingly, social biting often targets the dorsal part of the neck or the snout because injuries there would neither be lethal nor disabling (Poole, 1985; Tanke and Currie, 1998; Bell and Currie, 2010; Zammit and Kear, 2011). In SAM-PK-11490, the embedded tooth is located on the maxilla, in an area of the snout generally targeted during intraspecific face-biting [e.g., in crocodilians, Peterson et al. (2009)] as this region is not susceptible to intense bleeding and has no vital organs. In contrast, a predatory action (interspecific or cannibalism) is expected to be lethal if successful, which was not the case with SAM-PK-11490. Thirdly, gorgonopsians are noticeable for their saber-like canines (Sigogneau, 1970) and one of the functions of such enlarged canines in modern species is social signaling, including sexual display and intraspecific combat (Poole, 1985; Miller, 2011; Cabrera and Stankowich, 2018). Evidence of social biting has been found in saber-toothed cats, such as specimens MCA 2046 of Smilodon populator and SDSM 348 of Nimravus brachyops, which bear distinctive healed bite marks made by their conspecifics’ enlarged canines (Scott and Jepsen, 1936; Chimento et al., 2019). The almost ubiquitous presence of enlarged canines among NMT strongly supports that social signaling using teeth (including social biting) was an important driver of their evolution (Geist, 1972; Benoit et al., 2016). Noticeably, the rare NMT with reduced or no canines (e.g., tapinocephalids, endothiodontids, cistecephalids, or bauriid therocephalians) generally have bosses, horns, or enlarged incisors to compensate (Nasterlack et al., 2012; Abdala et al., 2014; Benoit et al., 2016, 2021).
It may be argued that the embedded tooth cannot belong to a gorgonopsian because their bite force was too weak to puncture bone. To achieve a bone-crushing bite on long bones, it is generally accepted that a carnivorous species would have to develop a bite force of at least 1,000–3000 N (Tanner et al., 2008; Bell and Currie, 2010; Snively et al., 2015), whereas the estimated bite force of Eriphostoma and Gorgonops (the two taxa that most closely resemble SAM-PK-11490) is only 25 N and 116 N, respectively (Lautenschlager et al., 2020). Even the largest gorgonopsians, such as Rubidgea atrox, would have had an estimated bite force of 715 N, insufficient for long bone-crushing (Lautenschlager et al., 2020). However, a bone-crushing bite is not a necessary condition to puncture bone, especially the rather thin bone of the snout, as demonstrated by the Komodo dragons (Varanus komodoensis), which can leave distinct bite marks on bones (D’Amore and Blumensehine, 2009) despite their weak bite force of less than 20 N (Moreno et al., 2008). Additionally, a distinctly large and deep puncture was found on the femur of an Oudenodon specimen (SAM-PK-K06446) and was attributed to the gorgonopsian Aelurognathus based on a broken tooth found close to the bone (Fordyce et al., 2012). According to Lautenschlager et al. (2020), the bite force of Aelurognathus was also very weak (61 N), but in the case of SAM-PK-K06446, it appears that the bite force of Aelurognathus was arguably strong enough to puncture the thigh bone of this large dicynodont. This shows that even a small gorgonopsian like SAM-PK-11490 could have been capable of puncturing bone and that its inability to crush bone does not disqualify social biting to account for the embedded tooth in its snout.
Conclusion
Social biting, and more particularly face biting, was advocated by (Geist, 1972; Barghusen, 1975; Benoit et al., 2016, 2021) to account for the evolution of oversized canines in NMT, but the absence of bite marks in the abundant fossil record of these taxa was puzzling. This is the first time a healed bite mark is documented in NMT, and its position on the snout, in line with other evidence, suggests that it was made during a face biting contest or some other type of social biting behavior (though, attempted predation by a therocephalian, biarmosuchian, or another gorgonopsian cannot be completely ruled out). Evidence of possible social behavior in NMT already supported parental care/brooding in cynodonts and the dicynodont Diictodon (Jasinoski and Abdala, 2017; Hoffman and Rowe, 2018; Smith et al., 2021 but see Sues, 2018 and Benoit, 2019 for different interpretations), gregariousness in dicynodonts (Fiorelli et al., 2013; Viglietti et al., 2013; Francischini et al., 2018), and head butting mostly in tapinocephalid dinocephalians (Barghusen, 1975; Benoit et al., 2017, 2021). In the synapsid lineage, these behaviors are traditionally considered as complex and more typical of mammals because they imply some level of social intelligence and usually correlate well with the presence of a large brain (Pérez-Barbería and Gordon, 2005; Sues, 2018; Benoit et al., 2021). The current work suggests social biting may be added to this list of advanced behaviors already present in NMT. The discovery of such significant evidence of ancient behavior on a specimen that was collected some 80 years ago and studied for at least five published and unpublished works (Sigogneau, 1970; Sigogneau-Russell, 1989; Gebauer, 2007; Kammerer, 2015; Kammerer et al., 2015) suggests that bite marks have been overlooked in NMT. This may account for the apparent paucity of recorded bite marks and other paleopathologies in NMT despite their rich fossil record. The systematic search for bite marks in the extensive collections of Permo-Triassic specimens will likely unravel a hitherto unknown diversity of bite marks and their associated behavior.
Data Availability Statement
The original contributions presented in the study are included in the article/supplementary material; further inquiries can be directed to the corresponding author/s.
Author Contributions
JB designed the study. JB and CB provided and analyzed the data. CB made the microscope photographs. JB drafted the manuscript. CB and LN provided critical comments. All authors gave final approval for publication.
Funding
This study received financial support from the DST-NRF Centre of Excellence in Palaeosciences.
Conflict of Interest
The authors declare that the research was conducted in the absence of any commercial or financial relationships that could be construed as a potential conflict of interest.
Acknowledgments
The authors thank Zaituna Skosan for providing access to the Karoo collections of the Iziko Museum of Natural History, Charlton Dube for preparing the specimen, and the paleoartist Sophie Vrard.
References
Abdala, F., Jashashvili, T., Rubidge, B. S., and van den Heever, J. (2014). “New material of Microgomphodon oligocynus (Eutherapsida, Therocephalia) and the taxonomy of Southern African Bauriidae,” in Early Evolutionary History of the Synapsida Vertebrate Paleobiology and Paleoanthropology, eds C. F. Kammerer, K. D. Angielczyk, and J. Fröbisch (Dordrecht: Springer), 209–231. doi: 10.1007/978-94-007-6841-3_13
Abdala, F., Rubidge, B. S., and Heever, J. V. D. (2008). The oldest Therocephalians (Therapsida, Eutheriodontia) and the early diversification of Therapsida. Palaeontology 51, 1011–1024. doi: 10.1111/j.1475-4983.2008.00784.x
Angielczyk, K. D., and Kammerer, C. F. (2018). “5. Non-mammalian synapsids: the deep roots of the mammalian family tree,” in Mammalian Evolution, Diversity and Systematics, eds F. Zachos and R. Asher (Berlin: De Gruyter), 117–198. doi: 10.1515/9783110341553-005
Aureliano, T., Nascimento, C. S. I., Fernandes, M. A., Ricardi-Branco, F., and Ghilardi, A. M. (2021). Blood parasites and acute osteomyelitis in a non-avian dinosaur (Sauropoda, Titanosauria) from the Upper Cretaceous Adamantina formation, Bauru Basin, Southeast Brazil. Cretac. Res. 118:104672. doi: 10.1016/j.cretres.2020.104672
Avilla, L. S., Fernandes, R., and Ramos, D. F. B. (2004). Bite marks on a crocodylomorph from the upper cretaceous of Brazil: evidence of social behavior? J. Vertebr. Paleontol. 24, 971–973.
Bakker, R. T., Flis, C. J., George, C. D., Cook, L. A., Bell, T. H., and Zoehfeld, K. W. (2015). “Dimetrodon and the earliest apex predators: the Craddock Bone Bed and George Ranch facies show that aquatic prey, not herbivores, were key food sources,” in Proceedings of the 75th Anniversary Annual Meeting of the Society of Vertebrate Paleontology, (Dallas, TX: Society of Vertebrate Paleontology), 83.
Barghusen, H. R. (1975). A review of fighting adaptations in dinocephalians (Reptilia, Therapsida). Paleobiology 1, 295–311. doi: 10.1017/S0094837300002542
Bastiaans, D., Kroll, J. J. F., Cornelissen, D., Jagt, J. W. M., and Schulp, A. S. (2020). Cranial palaeopathologies in a Late Cretaceous mosasaur from the Netherlands. Cretac. Res. 112:104425. doi: 10.1016/j.cretres.2020.104425
Bell, P. R., and Currie, P. J. (2010). A tyrannosaur jaw bitten by a confamilial: scavenging or fatal Agonism? Lethaia 43, 278–281. doi: 10.1111/j.1502-3931.2009.00195.x
Benoit, J. (2019). Parental care or opportunism in South African Triassic cynodonts? South Afr. J. Sci. 115, 1–2. doi: 10.17159/sajs.2019/5589
Benoit, J., Abdala, F., Van den Brandt, M. J., Manger, P. R., and Rubidge, B. S. (2015). Physiological implications of the abnormal absence of the parietal foramen in a late Permian cynodont (Therapsida). Sci. Nat. 102:69. doi: 10.1007/s00114-015-1321-4
Benoit, J., Kruger, A., Jirah, S., Fernandez, V., and Rubidge, B. (2021). Palaeoneurology and palaeobiology of the dinocephalian Anteosaurus magnificus. Acta Palaeontol. Pol. 66, 29–39. doi: 10.4202/app.00800.2020
Benoit, J., Manger, P. R., Fernandez, V., and Rubidge, B. S. (2016). Cranial bosses of Choerosaurus dejageri (Therapsida, Therocephalia): earliest evidence of cranial display structures in Eutheriodonts. PLoS One 11:e0161457. doi: 10.1371/journal.pone.0161457
Benoit, J., Manger, P. R., Norton, L., Fernandez, V., and Rubidge, B. S. (2017). Synchrotron scanning reveals the palaeoneurology of the head-butting Moschops capensis (Therapsida, Dinocephalia). PeerJ 5:e3496. doi: 10.7717/peerj.3496
Bishop, P. J., Walmsley, C. W., Phillips, M. J., Quayle, M. R., Boisvert, C. A., and McHenry, C. R. (2015). Oldest Pathology in a Tetrapod Bone Illuminates the Origin of Terrestrial Vertebrates. PLoS One 10:e0125723. doi: 10.1371/journal.pone.0125723
Boucot, A. J. (1990). Evolutionary Paleobiology of Behavior and Coevolution. New York, NY: Elsevier.
Budziszewska-Karwowska, E., Bujok, A., and Sadlok, G. (2010). Bite marks on an upper Triassic dicynodontoid tibia from Zawiercie, Krakow-Czestochowa upland, Southern Poland. PALAIOS 25, 415–421. doi: 10.2110/palo.2009.p09-136r
Buffetaut, E. (1983). Wounds on the jaw of an Eocene mesosuchian crocodilian as possible evidence for the antiquity of crocodilian intraspecific fighting behaviour. Paläontol. Z. 57, 143–145. doi: 10.1007/BF03031756
Buffetaut, E., Martill, D., and Escuillié, F. (2004). Pterosaurs as part of a spinosaur diet. Nature 430, 33–33. doi: 10.1038/430033a
Cabrera, D., and Stankowich, T. (2018). Stabbing Slinkers: tusk evolution among artiodactyls. J. Mamm. Evol. 27, 265–272. doi: 10.1007/s10914-018-9453-x
Chimento, N. R., Agnolin, F. L., Soibelzon, L., Ochoa, J. G., and Buide, V. (2019). Evidence of intraspecific agonistic interactions in Smilodon populator (Carnivora, Felidae). Compt. Rendus Palevol. 18, 449–454. doi: 10.1016/j.crpv.2019.02.006
D’Amore, D. C., and Blumensehine, R. J. (2009). Komodo monitor (Varanus komodoensis) feeding behavior and dental function reflected through tooth marks on bone surfaces, and the application to ziphodont paleobiology. Paleobiology 35, 525–552. doi: 10.1666/0094-8373-35.4.525
Day, M. O., and Rubidge, B. S. (2020). Biostratigraphy of the Tapinocephalus assemblage zone (Beaufort Group, Karoo Supergroup), South Africa. South Afr. J. Geol. 123, 149–164. doi: 10.25131/sajg.123.0012
DePalma, R. A., Burnham, D. A., Martin, L. D., Rothschild, B. M., and Larson, P. L. (2013). Physical evidence of predatory behavior in Tyrannosaurus rex. Proc. Natl. Acad. Sci. U.S.A. 110, 12560–12564. doi: 10.1073/pnas.1216534110
Drumheller, S. K., Stocker, M. R., and Nesbitt, S. J. (2014). Direct evidence of trophic interactions among apex predators in the Late Triassic of western North America. Naturwissenschaften 101, 975–987. doi: 10.1007/s00114-014-1238-3
Erickson, G. M., Kirk, S. D. V., Su, J., Levenston, M. E., Caler, W. E., and Carter, D. R. (1996). Bite-force estimation for Tyrannosaurus rex from tooth-marked bones. Nature 382, 706–708. doi: 10.1038/382706a0
Everhart, M. J. (2008). A bitten skull of Tylosaurus kansasensis (Squamata: Mosasauridae) and a review of mosasaur-on-mosasaur pathology in the fossil record. Trans. Kans. Acad. Sci. 111, 251–262. doi: 10.1660/0022-8443-111.3.251
Farke, A. A., Wolff, E. D. S., and Tanke, D. H. (2009). Evidence of Combat in Triceratops. PLoS One 4:e4252. doi: 10.1371/journal.pone.0004252
Fernandez, V., Abdala, F., Carlson, K. J., Cook, D. C., Rubidge, B. S., Yates, A., et al. (2013). Synchrotron reveals early triassic odd couple: injured amphibian and aestivating Therapsid share burrow. PLoS One 8:e64978. doi: 10.1371/journal.pone.0064978
Fiorelli, L. E., Ezcurra, M. D., Hechenleitner, E. M., Argañaraz, E., Taborda, J. R. A., Trotteyn, M. J., et al. (2013). The oldest known communal latrines provide evidence of gregarism in Triassic megaherbivores. Sci. Rep. 3, 1–7. doi: 10.1038/srep03348
Fordyce, N., Smith, R., and Chinsamy, A. (2012). Evidence of a therapsid scavenger in the Late Permian Karoo Basin, South Africa. South Afr. J. Sci. 108, 114–118.
Fortuny, J., and Steyer, J.-S. (2019). New insights into the evolution of temnospondyls. J. Iber. Geol. 45, 247–250. doi: 10.1007/s41513-019-00104-0
Francischini, H., Dentzien-Dias, P., Lucas, S. G., and Schultz, C. L. (2018). Tetrapod tracks in Permo-Triassic eolian beds of southern Brazil (Paraná Basin). PeerJ 6:e4764. doi: 10.7717/peerj.4764
Fröbisch, J., and Reisz, R. R. (2008). A new species of Emydops (Synapsida, Anomodontia) and a discussion of dental variability and pathology in dicynodonts. J. Vertebr. Paleontol. 28, 770–787.
Gebauer, E. V. I. (2007). Phylogeny and Evolution of the Gorgonopsia with a Special Reference to the Skull and Skeleton of GPIT-RE-7113 (“Aelurognathus?” parringtoni). Available online at: http://nbn-resolving.de/urn:nbn:de:bsz:21-opus-29355 (accessed July 06, 2019).
Geist, V. (1966). The evolution of horn-like organs. Behaviour 27, 175–214. doi: 10.1163/156853966X00155
Geist, V. (1972). An ecological and behavioural explanation of mammalian characteristics and their implication to therapsid evolution. Z. Für Säugetierkd. Im Auftrage Dtsch. Ges. Für Säugetierkd. EV 37, 1–15.
Gignac, P. M., Makovicky, P. J., Erickson, G. M., and Walsh, R. P. (2010). A description of Deinonychus antirrhopus bite marks and estimates of bite force using tooth indentation simulations. J. Vertebr. Paleontol. 30, 1169–1177. doi: 10.1080/02724634.2010.483535
Hoffman, E. A., and Rowe, T. B. (2018). Jurassic stem-mammal perinates and the origin of mammalian reproduction and growth. Nature 561, 104–108. doi: 10.1038/s41586-018-0441-3
Ivakhnenko, M. F. (2008). Cranial morphology and evolution of permian Dinomorpha (Eotherapsida) of eastern Europe. Paleontol. J. 42, 859–995. doi: 10.1134/S0031030108090013
Jasinoski, S. C., and Abdala, F. (2017). Aggregations and parental care in the early triassic basal cynodonts Galesaurus planiceps and Thrinaxodon liorhinus. PeerJ 5:e2875. doi: 10.7717/peerj.2875
Jinnah, Z. A., and Rubidge, B. (2007). A double-tusked dicynodont and its biostratigraphic significance. South Afr. J. Sci. 103, 51–53.
Kammerer, C. F. (2011). Systematics of the Anteosauria (Therapsida: Dinocephalia). J. Syst. Palaeontol. 9, 261–304. doi: 10.1080/14772019.2010.492645
Kammerer, C. F. (2015). Cranial osteology of Arctognathus curvimola, a short-snouted gorgonopsian from the Late Permian of South Africa. Pap. Palaeontol. 1, 41–58. doi: 10.1002/spp2.1002
Kammerer, C. F., Smith, R. M. H., Day, M. O., and Rubidge, B. S. (2015). New information on the morphology and stratigraphic range of the mid-Permian gorgonopsian Eriphostoma microdon Broom, 1911. Pap. Palaeontol. 1, 201–221. doi: 10.1002/spp2.1012
Kappelman, J., Ketcham, R. A., Pearce, S., Todd, L., Akins, W., Colbert, M. W., et al. (2016). Perimortem fractures in Lucy suggest mortality from fall out of tall tree. Nature 537, 503–507. doi: 10.1038/nature19332
Kato, K. M., Rega, E. A., Sidor, C. A., and Huttenlocker, A. K. (2020). Investigation of a bone lesion in a gorgonopsian (Synapsida) from the Permian of Zambia and periosteal reactions in fossil non-mammalian tetrapods. Philos. Trans. R. Soc. B Biol. Sci. 375:20190144. doi: 10.1098/rstb.2019.0144
Katsura, Y. (2004). Paleopathology of Toyotamaphimeia machikanensis (Diapsida, Crocodylia) from the Middle pleistocene of Central Japan. Hist. Biol. 16, 93–97. doi: 10.1080/08912963400015041
Konishi, T., and Brinkman, D. (2016). Non-lethal face biting between mosasaurs (Squamata: Mosasauridae): The first unequivocal evidence from an exceptional skeleton of Mosasaurus sp. From southern Alberta, Canada. J. Verteb. Paleontol. Suppl. 36(6 Suppl.):168.
Lautenschlager, S., Figueirido, B., Cashmore, D. D., Bendel, E.-M., and Stubbs, T. L. (2020). Morphological convergence obscures functional diversity in sabre-toothed carnivores. Proc. R. Soc. B Biol. Sci. 287:20201818. doi: 10.1098/rspb.2020.1818
Lingham-Soliar, T. (2004). Palaeopathology and injury in the extinct mosasaurs (Lepidosauromorpha, Squamata) and implications for modern reptiles. Lethaia 37, 255–262. doi: 10.1080/00241160410006519
Mackness, B., and Sutton, R. (2000). Possible evidence for intraspecific aggression in a Pliocene crocodile from north Queensland. Alcheringa Austr. J. Palaeontol. 24, 55–62. doi: 10.1080/03115510008619523
Mendrez, C. (1975). Principales variations du palais chez les Thérocéphales Sud-Africans (Pristerosauria et Scaloposauria) au cours du Permien Supérieur et du Trias Inférieur. Problèm. Actuels Paléontol. Vertébrés Colloq. Int. Cent. Natl. Recherchée Sci. 218, 379–408.
Miller, E. H. (2011). Walrus ethology. I. The social role of tusks and applications of multidimensional scaling. Can. J. Zool. 53, 590–613. doi: 10.1139/z75-073
Moreno, K., Wroe, S., Clausen, P., McHenry, C., D’Amore, D. C., Rayfield, E. J., et al. (2008). Cranial performance in the Komodo dragon (Varanus komodoensis) as revealed by high-resolution 3-D finite element analysis. J. Anat. 212, 736–746. doi: 10.1111/j.1469-7580.2008.00899.x
Nasterlack, T., Canoville, A., and Chinsamy, A. (2012). New insights into the biology of the Permian genus Cistecephalus (Therapsida, Dicynodontia). J. Vertebr. Paleontol. 32, 1396–1410. doi: 10.1080/02724634.2012.697410
Nicolas, M., and Rubidge, B. S. (2010). Changes in Permo-Triassic terrestrial tetrapod ecological representation in the Beaufort Group (Karoo Supergroup) of South Africa. Lethaia 43, 45–59. doi: 10.1111/j.1502-3931.2009.00171.x
Pérez-Barbería, F. J., and Gordon, I. J. (2005). Gregariousness increases brain size in ungulates. Oecologia 145, 41–52. doi: 10.1007/s00442-005-0067-7
Peterson, J. E., Dischler, C., and Longrich, N. R. (2013). Distributions of cranial pathologies provide evidence for head-butting in dome-headed dinosaurs (Pachycephalosauridae). PLoS One 8:e68620. doi: 10.1371/journal.pone.0068620
Peterson, J. E., Henderson, M. D., Scherer, R. P., and Vittore, C. P. (2009). Face biting on a juvenile tyrannosaurid and behavioural implications. PALAIOS 24, 780–784. doi: 10.2110/palo.2009.p09-056r
Peterson, J. E., and Vittore, C. P. (2012). Cranial pathologies in a specimen of Pachycephalosaurus. PLoS One 7:e36227. doi: 10.1371/journal.pone.0036227
Poole, T. B. (1985). Social Behaviour in Mammals: Tertiary Level Biology. Boston, MA: Springer, doi: 10.1007/978-1-4613-2345-7
Rega, E. A., Noriega, K., Sumida, S. S., Huttenlocker, A., Lee, A., and Kennedy, B. (2012). Healed fractures in the neural spines of an associated skeleton of Dimetrodon: implications for dorsal sail morphology and function. Fieldiana Life Earth Sci. 5, 104–111. doi: 10.3158/2158-5520-5.1.104
Reisz, R. R., and Tsuji, L. A. (2006). An articulated skeleton of Varanops with bite marks: the oldest known evidence of scavenging among terrestrial vertebrates. J. Vertebr. Paleontol. 26, 1021–1023.
Rothschild, B. M., and Martin, L. D. (1993). Paleopathology Disease in the Fossil Record. Boca Raton, FL: CRC-Press.
Rubidge, B. S., and Kitching, J. W. (2003). A new burnetiamorph (Therapsida: Biarmosuchia) from the Lower Beaufort Group of South Africa. Palaeontology 46, 199–210. doi: 10.1111/1475-4983.00294
Scott, W. B., and Jepsen, G. L. (1936). The Mammalian Fauna of the White River Oligocene: Part I. Insectivora and Carnivora. Trans. Am. Philos. Soc. 28, 1–153. doi: 10.2307/1005507
Shelton, C. D., Chinsamy, A., and Rothschild, B. M. (2019). Osteomyelitis in a 265-million-year-old titanosuchid (Dinocephalia, Therapsida). Hist. Biol. 31, 1093–1096. doi: 10.1080/08912963.2017.1419348
Sigogneau, D. (1970). Révision Systématique des Gorgonopsiens Sud-Africains: par D. Sigogneau. Paris: Editions du Centre national de la recherche scientifique.
Sigogneau-Russell, D. (1989). Theriodontia I: Phthinosuchia, Biarmosuchia, Eotitanosuchia, Gorgonopsia. New York, NY: Gustav Fischer Verlag.
Smith, R. M. H., Angielczyk, K. D., Benoit, J., and Fernandez, V. (2021). Neonate aggregation in the Permian dicynodont Diictodon (Therapsida, Anomodontia): evidence for a reproductive function for burrows? Palaeogeogr. Palaeoclimatol. Palaeoecol. 569:110311. doi: 10.1016/j.palaeo.2021.110311
Snively, E., Fahlke, J. M., and Welsh, R. C. (2015). Bone-breaking bite force of Basilosaurus isis (Mammalia, Cetacea) from the Late Eocene of Egypt estimated by finite element analysis. PLoS One 10:e0118380. doi: 10.1371/journal.pone.0118380
Sues, H.-D. (2018). Palaeontology: many babies or bigger brains? Curr. Biol. 28, R1254–R1256. doi: 10.1016/j.cub.2018.09.041
Tanke, D. H., and Currie, P. J. (1998). Head-biting behavior in theropod dinosaurs: paleopathological evidence. Gaia 15, 167–184.
Tanke, D. H., and Rothschild, B. M. (2002). DINOSORES: An Annotated Bibliography of Dinosaur Paleopathology and Related Topics—1838-2001: Bulletin 20. Albuquerque: New Mexico Museum of Natural History and Science.
Tanner, J. B., Dumont, E. R., Sakai, S. T., Lundrigan, B. L., and Holekamp, K. E. (2008). Of arcs and vaults: the biomechanics of bone-cracking in spotted hyenas (Crocuta crocuta): FE analysis of the spotted hyena skull. Biol. J. Linn. Soc. 95, 246–255. doi: 10.1111/j.1095-8312.2008.01052.x
Vega, C. S., and Maisch, M. W. (2014). “Pathological features in upper permian and middle triassic Dicynodonts (Synapsida, Therapsida),” in Early Evolutionary History of the Synapsida Vertebrate Paleobiology and Paleoanthropology, eds C. F. Kammerer, K. D. Angielczyk, and J. Fröbisch (Dordrecht: Springer), 151–161. doi: 10.1007/978-94-007-6841-3_9
Viglietti, P. A., Smith, R. M. H., and Compton, J. S. (2013). Origin and palaeoenvironmental significance of Lystrosaurus bonebeds in the earliest Triassic Karoo Basin, South Africa. Palaeogeogr. Palaeoclimatol. Palaeoecol. 392, 9–21. doi: 10.1016/j.palaeo.2013.08.015
Whitney, M. R., Mose, L., and Sidor, C. A. (2017). Odontoma in a 255-Million-Year-Old Mammalian Forebear. JAMA Oncol. 3:998. doi: 10.1001/jamaoncol.2016.5417
Whitney, M. R., Tse, Y. T., and Sidor, C. A. (2019). Histological evidence of trauma in tusks of southern African dicynodonts. Palaeont. Afr. 53, 75–80.
Wolff, E. D. S., Salisbury, S. W., Horner, J. R., and Varricchio, D. J. (2009). Common avian infection plagued the tyrant dinosaurs. PLoS One 4:e7288. doi: 10.1371/journal.pone.0007288
Keywords: Gorgonopsia, bite mark, social biting, callus, paleopathology, saber-toothed
Citation: Benoit J, Browning C and Norton LA (2021) The First Healed Bite Mark and Embedded Tooth in the Snout of a Middle Permian Gorgonopsian (Synapsida: Therapsida). Front. Ecol. Evol. 9:699298. doi: 10.3389/fevo.2021.699298
Received: 23 April 2021; Accepted: 31 May 2021;
Published: 21 June 2021.
Edited by:
Jörg Fröbisch, Museum of Natural History Berlin (MfN), GermanyReviewed by:
Aaron R. H. LeBlanc, King’s College London, United KingdomChristian Kammerer, North Carolina Museum of Natural Sciences, United States
Copyright © 2021 Benoit, Browning and Norton. This is an open-access article distributed under the terms of the Creative Commons Attribution License (CC BY). The use, distribution or reproduction in other forums is permitted, provided the original author(s) and the copyright owner(s) are credited and that the original publication in this journal is cited, in accordance with accepted academic practice. No use, distribution or reproduction is permitted which does not comply with these terms.
*Correspondence: Julien Benoit, SnVsaWVuLmJlbm9pdEB3aXRzLmFjLnph