- 1Lhasa Plateau Ecosystem Research Station, Key Laboratory of Ecosystem Network Observation and Modeling, Institute of Geographic Sciences and Natural Resources Research, Chinese Academy of Sciences, Beijing, China
- 2College of Resources and Environment, University of Chinese Academy of Sciences, Beijing, China
- 3School of Sciences, Tibet University, Lhasa, China
- 4Institute of Alpine Economics and Botany, Yunnan Academy of Agricultural Sciences, Lijiang, China
Grazing is a crucial anthropogenic disturbance on grasslands. However, it is unknown how livestock grazing affects the relationship between biodiversity and productivity of alpine grasslands in Tibet. We carried out a grazing-manipulated experiment from 2016 to 2019 with grazing intensity levels of null (control, grazing exclusion, C.K.), moderate grazing [1.65 standardized sheep unit (SSU) per hectare, M.G.], and heavy grazing (2.47 SSU per hectare, H.G.) on a typical alpine grassland in the Lhasa River Basin, central Tibet. We measured aboveground biomass (AGB), species assembly (alpha and beta diversity indices), and soil nutrients’ availability. The results showed that grazing differently affected plant community in different treatments. Notably, the total dissimilarity value between C.K. and H.G. is 0.334. Grazing decreased the Shannon–Wiener index, increased the Berger–Parker index from 2016 to 2018 significantly, and decreased AGB and total soil nitrogen (STN) significantly. Our results also showed that the grazing affected the relationship between AGB and diversity indices and soil nutrients, including soil organic carbon (SOC) and total soil phosphorus (STP). Specifically, AGB decreased with increasing SOC and STP in all treatments, and heavy grazing changed the positive relationships between AGB, STP, and Shannon–Wiener index to negative correlations significantly compared with grazing exclusion. There was a significant negative correlation between Berger–Parker and Shannon–Wiener indices under each treatment. The general linear models showed that H.G. altered the relationship between diversity and productivity of grassland in central Tibet, and AGB and Shannon–Wiener index positively correlated in C.K. but negatively correlated in H.G. Our study suggests that H.G. caused a negative relationship between plant diversity and productivity. Therefore, sustainable grazing management calls for a need of better understanding the relationship between biodiversity and productivity of alpine grassland in central Tibet.
Introduction
Livestock grazing is a crucial anthropogenic disturbance on grasslands (Wu et al., 2009), reshaping plant community assembly and affecting ecosystem functions (Cingolani et al., 2005). Grazing reduces plant species diversity in dry forests (Schulz et al., 2019) but increases it in grazed areas (Gonzalez-Hernandez et al., 2020). According to the intermediate disturbance hypothesis (Mcnaughton, 1983), the plant community productivity and diversity were the highest at light or moderate grazing intensity. However, over-grazing may reduce species richness (Cardinale et al., 2012). Moreover, grazing can affect plant community structure by changing species composition (Lin et al., 2010), where graminoid and herb species can be inhibited (Rada et al., 2014). Grazing has little influence on nestedness but can increase species turnover (Adler et al., 2001). Furthermore, heavy grazing can result in high species replacement (Sanaei et al., 2018). However, how different grazing intensities affect plant community assembly is still unclear.
Aboveground biomass (AGB) is an important indicator of the quantitative characteristics of ecosystem functions to an anthropogenic disturbance (Ma et al., 2010). Many studies have demonstrated that grazing significantly affected AGB and primary productivity at different scales (Wu, 2013; Su et al., 2017; Liu et al., 2020). Notably, heavy grazing seriously declined grassland productivity and plant coverage in grasslands and intensified land desertification (Wu et al., 2009). However, the effect of grazing intensities on alpine grasslands is still under debates. Therefore, a better understanding of the impact of grazing disturbance on AGB could help us to enhance ecosystem stability when facing external disturbances (Rusina et al., 2013). It should examine the balance between ecosystem function and ecosystem service for rational rangeland management (Dias et al., 2017).
The biodiversity–productivity relationship of grassland is a controversial topic in the field of ecosystem function and stability research (Axmanova et al., 2013; Liang et al., 2016; Wu et al., 2019a). The relationship is complex and is affected by many factors that are monotonous linear (positively or negatively) or have no relation (Almufti et al., 1977; Mcnaughton, 1983; Gough et al., 1994; Gillman and Wright, 2006; Hector and Bagchi, 2007; Cardinale et al., 2012; Zhang R. et al., 2018). A positive linear relationship between biodiversity and productivity has been found in the Eurasian Steppe (Bai et al., 2007) and a weak positive relation in the Qinghai–Tibetan Plateau (Fayiah et al., 2019). Zhang et al. (2011) found that the relationship can be changed with special research scales in the temperate steppe of Inner Mongolia. Wang et al. (2013) demonstrated no significant correlation between plant species richness and community productivity in the Tibetan semi-arid regions where available water is limited. How plant diversity regulates vegetation productivity is receiving wide attention to Erfanzadeh et al. (2015) and Grace et al. (2016). However, many studies rarely examine the impact of environmental factors and ways of utilization of the relationship shapes (White et al., 2019). Therefore, it is necessary to examine the mechanisms behind the different biodiversity–productivity relationships (Gross and Cardinale, 2007).
The Qinghai–Tibetan Plateau (QTP) is characterized by high altitude and high fluctuation in temperature, intense radiation, and fragile ecosystems (Gao et al., 2010; Liu et al., 2020). It covers more than 20% of China’s grassland (Cao et al., 2004). Alpine grasslands were an essential resource of pastoral production activities (Yi et al., 2019) affected by many biotic and abiotic factors (He et al., 2005; Zhu et al., 2016). At large scales, alpine grasslands on this plateau are thought to be sensitive to climate change (Wu et al., 2019b). At a regional or local scale, they are disturbed by livestock grazing activities (Bai et al., 2007; Milchunas et al., 2012; Erfanzadeh et al., 2015). The relationship between plant diversity and productivity is influenced by environmental variables differently at various spatial scales in Tibetan grasslands (Ma et al., 2010). Xiong et al. (2014) found that grazing exclusion improved forage yield and has negative influences on species diversity in alpine meadows. Wu et al. (2014b) discovered that AGB increased with precipitation in growing season and decreased with growing season temperature in alpine pastures across the northern Tibetan Plateau. They even found a unimodal pattern between AGB and species richness within steppes but no significant relationship neither in meadows nor desert steppes (Wu et al., 2014a). Previous studies focused on the impact of grazing or fencing on the productivity–species diversity relationship in the northern part of the Qinghai–Tibetan Plateau. Besides, rare efforts were done to explore how grazing intensity affects the alpine grasslands in central Tibet. Therefore, it is necessary to consider how different grazing intensities shape the relationship between plant diversity and productivity of grasslands in central Tibet.
Specifically, in the current study, we aim to (1) explore how the diversity–productivity relationship is driven by grazing management, (2) examine whether the relationship between plant diversity and productivity is monotonous linear (positively or negatively) or not related, and finally, and (3) inspect whether the relationship has been altered by heavy grazing. We examined the differences in plant community diversity, AGB, and soil nutrient content between three grazing intensity levels. Finally, we analyzed the relationship between plant diversity and productivity under the different grazing intensities.
Materials and Methods
Study Area and Experimental Design
The research station locates in the Linzhou County (29°45′∼30°08′ N, 90°51′∼91°28′ E, 3,900 m.a.s.l.), approximately 60 km away from Lhasa, the capital of the Tibet Autonomous Region, China (Figure 1). Table 1 shows community productivity, plant species richness, and soil nutrients. At this station, annual precipitation ranges from 343 to 466 mm, over 70% of which falls in the summer monsoon season, hereafter named the plant-growing season. Mean air temperature during the plant-growing season ranges from 10.6 to 12.6°C (Chen et al., 2014). The vegetation is alpine steppe dominated by Pennisetum centrasiaticum, Tripogon bromoides, Kobresia pygmaea, and Carex atrofusca. However, degradation indicator species are Stipa capillacea, Astragalus strictus, Euphorbia kansui, and Ligularia rumicifolia. The soil is poor with an average depth of about 20–30 cm.
The grassland at this station was fenced and excluded from livestock grazing since May 2011. In 2016, we built 15 plots of 10 m × 10 m within the fenced area, grouped randomly into three different treatments. According to the grassland carrying capacity in the Lhasa River Basin (Xiang et al., 2019), the grazing intensity level was manipulated at null (control, grazing exclusion, C.K.), moderate grazing [1.65 standardized sheep unit (SSU) per hectare, M.G.], and heavy grazing (2.47 SSU per hectare, H.G.). Nine fixed quadrats of 0.5 m × 0.5 m, at least 0.5 m away from the fence lines, were located in each plot. The grazing was carried out once per month, between July 15th and September 17th, from 2016 to 2019. Grazing duration is 8 h/day each time, with two female healthy sheep (native sheep) for M.G. and four female sheep for H.G., respectively.
Data Collection and Calculation
Plant composition was surveyed before the grazing experiment each year. Plant species height was measured and averaged as the community height. Species coverage was visually estimated and summed for the community coverage. The numbers of species within each quadrat and plot was used as plant species richness (n) at the quadrat and plot levels, respectively. A quadrate near each fixed quadrat was used to evaluate AGB. All plants in each quadrat were harvested, kept in an envelope, and weighed after being oven-dried at 65°C for 48 h. After harvesting AGB, three soil samples were collected from each plot. The soil samples were air-dried in a dark room. Finally, the contents of soil organic carbon (SOC), total soil nitrogen (STN), and total soil phosphorus (STP) were measured for further analysis. A given species’ frequency (Fri) was relative to the sum of all species that appeared in nine quadrats at each plot. Similarly, we calculated relative species coverage (Cri) and height (Hri) (Wu et al., 2019b). Thus, the Shannon–Wiener diversity index (H′) was calculated based on the relative species dominance (Pi), which is the mean of relative species coverage (Cri), frequency (Fri), and height (Hri) (see Eqs. 1–3). We also calculated the Berger–Parker index (B′), which is the ratio of the max number of dominant species (nmax) to all species numbers (N) (Morris et al., 2014), Eq. 4.
The beta (β) diversity refers to the dissimilarity of species composition among different communities or species replacement rate along the environmental gradients. In this study, the β diversity index was calculated at plot level among treatments according to the approach of Baselga (2010). The binary presence or absence matrices for plant species across 15 plots were used for a measured dissimilarity index. Species turnover was expressed as Simpson dissimilarity (βsim, Eq. 5). Species nestedness was expressed as a nestedness-resultant fraction of Sørensen dissimilarity (βsne, Eq. 6). Species overall beta diversity was expressed as Sørensen dissimilarity (βsor, Eqs. 7–8), where a is the number of species shared in two plots, b is the number of species that only occurred at the species-poorest plot, and c is the number of species only appearing on the species-richest plot (Leprieur et al., 2012; Tonn et al., 2019).
Statistical Analyses
The data analysis was conducted with R statistic software version 4.0.3 for Windows. First, beta diversity indices (βsim, βsne, and βsor) were calculated by using the “betapart” package. The differences in plant diversity (Shannon–Wiener index and Berger–Parker index), soil nutrients, and AGB between treatments were examined with the Kruskal–Wallis and tested by rank, which is a non-parametric alternative to one-way analysis of variance (ANOVA) when the assumptions of homogeneity of variance and normality are not met (Wu et al., 2019b). The correlation coefficient matrix was calculated and tested the significance by “corrplot” package within each of the three treatments. Finally, we established general linear models (GLM) to analyze the relationships between soil nutrients, plant diversity indices, and community AGB at different grazing intensities.
Results
Comparisons of Community Assembly
Community dissimilarities was dominated by species turnover, with a relatively small contribution of the nestedness component (Figure 2). In 2016, the dissimilarity value of species turnover of C.K. to H.G. is 0.164, which is lower than C.K. to M.G. (0.209) and M.G. to H.G. (0.188). However, the dissimilarity value of species turnover of C.K. to H.G. gradually increased from 2017 to 2019 and reached the highest value (that is 0.219) in 2019 (Figure 2A). The total dissimilarity value of C.K. to H.G. is similar to the species turnover of C.K. to H.G., and the largest total dissimilarity value is 0.334 in 2019 (Figure 2C). For nestedness dissimilarity (Figure 2B), changing trends of C.K. to H.G., C.K. to M.G., and M.G. to H.G. were consistent from 2016 to 2019, and the largest value of nestedness dissimilarity are 0.145 and 0.160 in 2018 and 0.123 in 2017, respectively.
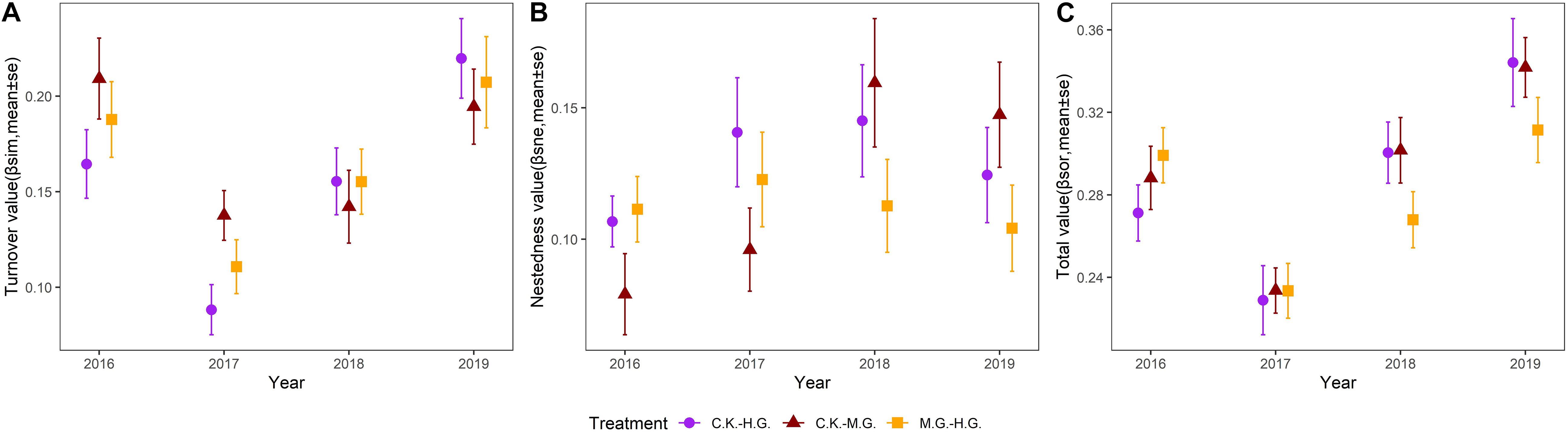
Figure 2. Pairwise dissimilarity in vegetation composition between grazing treatments from 2016 to 2019: grazing exclusion (C.K.), moderate grazing (M.G.), and heavy grazing (H.G.). Dissimilarity component includes species turnover (A), nestedness (B), and total dissimilarity (C).
The Shannon–Wiener index differed significantly between grazing treatments (C.K., M.G., and H.G., P < 0.05, Figure 3A), except for between C.K. and M.G. in 2017 and 2019 and between M.G. and H.G. in 2018. There was no significant difference in the Berger–Parker index (P > 0.05) between treatments in 2016 (Figure 3B). However, the Berger–Parker index was significantly different between C.K. and H.G. for 2017–2019 and between M.G. and H.G. in 2017 and 2019 (Figure 3B).
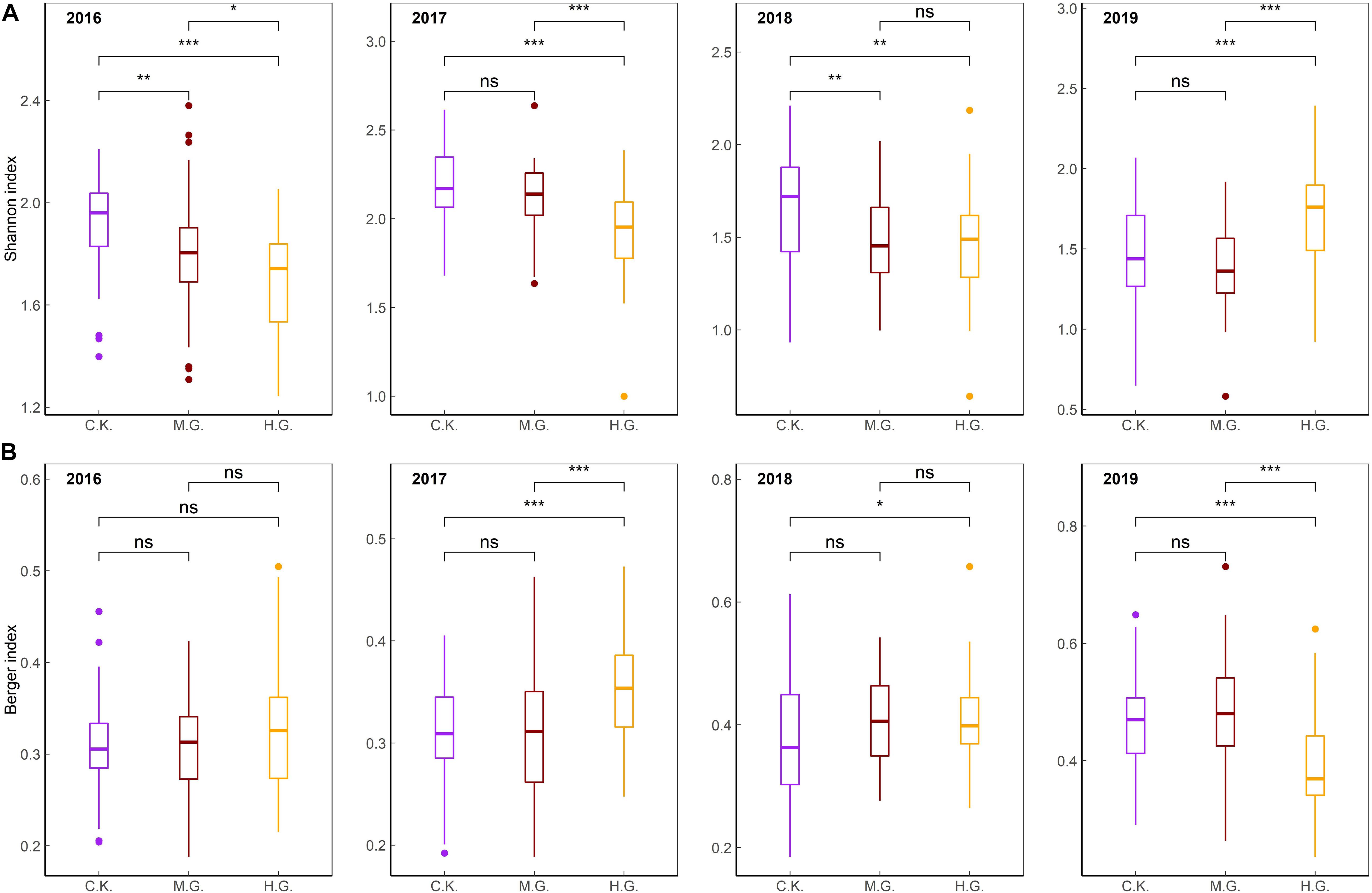
Figure 3. Comparisons of the Shannon–Wiener index (A) and Berger–Parker index (B) between grazing treatments. The differences were at the ***0.001, **0.01, and *0.05 levels. The three treatments include grazing exclusion (C.K.), moderate grazing (M.G.), and heavy grazing (H.G.).
Comparisons of AGB and Soil Nutrients
With the increase of grazing intensity, AGB and STN decreased significantly (Figures 4A,C, P < 0.05), whereas STP under H.G. were lower than C.K. significantly (Figure 4B, P < 0.05), and SOC under M.G. were higher than C.K. and H.G. significantly (Figure 4D, P < 0.05).
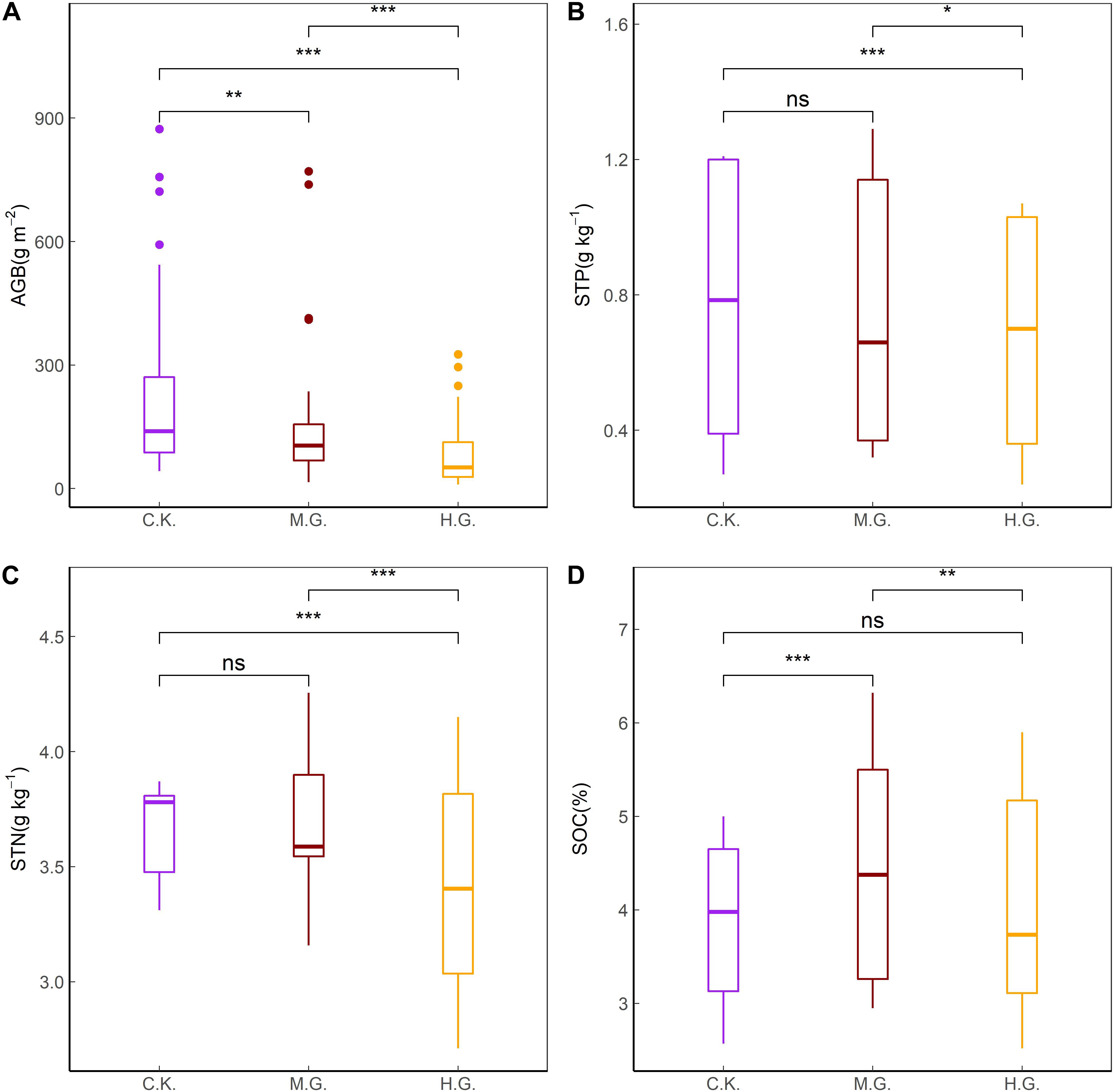
Figure 4. Comparisons of AGB (A), STP (B), STN (C), and SOC (D) between grazing treatments. The differences were at the ***0.001, **0.01, and *0.05 levels. The treatments include grazing exclusion (C.K.), moderate grazing (M.G.), and heavy grazing (H.G.).
Aboveground biomass and soil nitrogen decreased dramatically with increasing grazing intensity (Figures 4A,C, P < 0.05). STP under H.G. was lower than that under C.K. and M.G. (Figure 4B, P < 0.05). However, there was no significant difference in STP between M.G. and C.K. (Figure 4B, P > 0.05). SOC under M.G. was higher than that under C.K. and H.G. (Figure 4D, P < 0.05). There was no significant difference in SOC between H.G. and C.K. (Figure 4B, P > 0.05).
The Relationship Among Plant Diversity, AGB, and Soil Nutrients
There is a significantly negative correlation between Berger–Parker and Shannon–Wiener indices in all treatments (Figure 5). The relationships between AGB, STP, and Shannon–Wiener index changed from positive to negative correlations significantly under C.K. and H.G. There is no significant relationship between STN, plant diversity, and aboveground biomass among the three treatments.
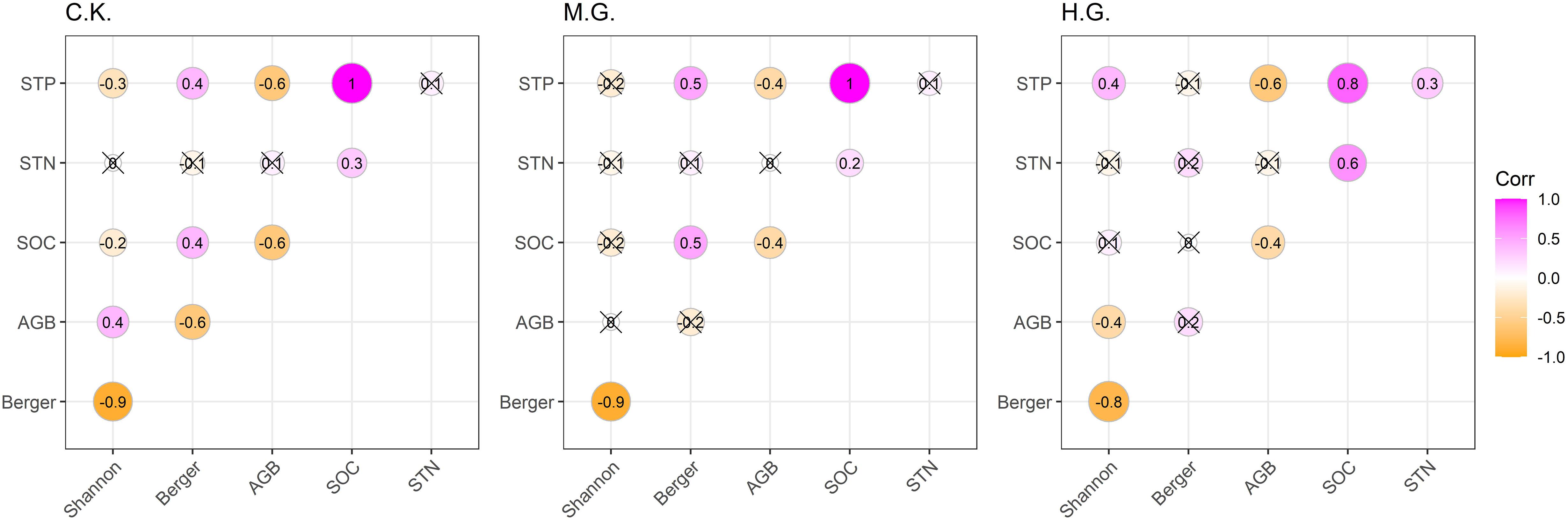
Figure 5. Correlation matrix of soil nutrients, AGB, and biodiversity indices for each of the three grazing treatments. The three treatments include grazing exclusion (C.K.), moderate grazing (M.G.), and heavy grazing (H.G.). The “×” symbol means that the correlation coefficient does not reach the significance level, P > 0.05.
Bivariate regressions showed similar patterns of AGB under the three treatments, which decreased with the increasing SOC and STP (Figures 6A,C). Grazing altered the slopes of AGB varying along with SOC and STP compared to C.K., namely, the slopes of AGB along with SOC and STP were steeper with increasing grazing intensities. The proportions of the total variance AGB explained by SOC and STP were 36 and 39% under C.K., 16 and 35% under M.G., 17 and 18% under H.G., respectively.
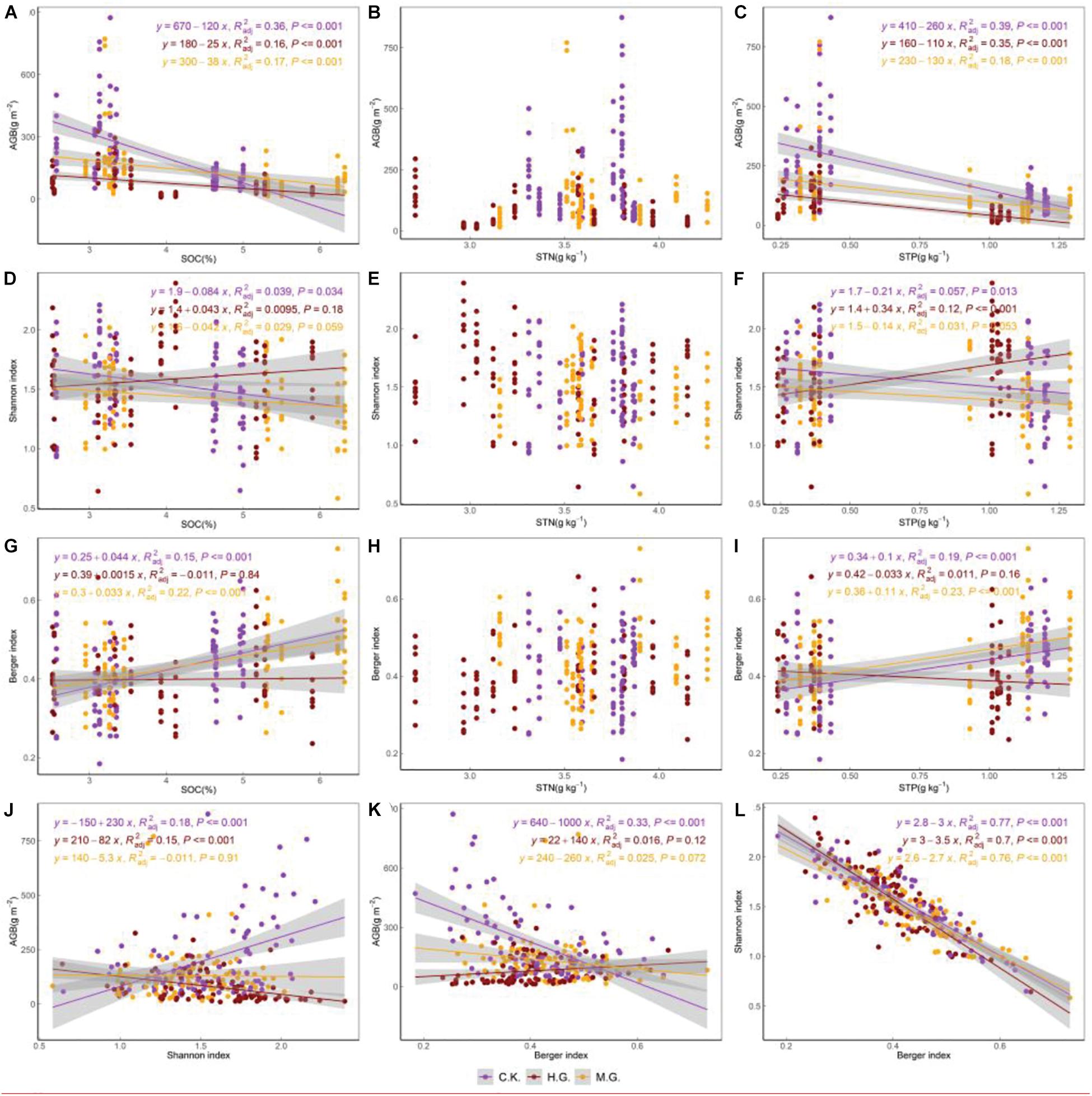
Figure 6. (A–L) The changing pattern of biodiversity indices, soil nutrients, and aboveground biomass at three treatments. The three treatments include grazing exclusion (C.K.), moderate grazing (M.G.), and heavy grazing (H.G.). The color purple represents C.K., the color orange represents M.G., and the color dark red represents H.G., respectively.
As Figures 6D,F shows, H.G. affected the patterns of the Shannon–Wiener index along with SOC and STP, from increasing under C.K. (k = −0.3, R2 = 0.039 and k = −0.25, R2 = 0.057) to decreasing under H.G. (k = 0.15, R2 = 0.0095 and k = 0.41, R2 = 0.12). Similarly, grazing also changed the pattern of the Berger–Parker index varying along with STP, from increasing under C.K. (k = 0.45, R2 = 0.19) to decreasing under H.G. (k = −0.14, R2 = 0.011, Figure 6I).
Grazing altered the linear relationship between AGB and Shannon–Wiener index, from positive (k = 0.53, R2 = 0.18, P ≤ 0.001) under C.K. to negative (k = −0.19, R2 = 0.15, P ≤ 0.001) under H.G. (Figure 6J). The relationship between AGB and Berger–Parker index was altered from being negative linear (k = −0.68, R2 = 0.33, P < 0.05) under C.K. to no relation under H.G. (Figure 6K, P > 0.05). Meanwhile, the Shannon–Wiener index decreased with the Berger–Parker index under three treatments (Figure 6L, P < 0.001). However, there is no correlation between STN and AGB (Figure 6B), Shannon-Wiener index (Figure 6E), Berger-Parker index (Figure 6H).
Discussion
Grasslands in central Tibet are intensively affected by anthropogenic disturbances (grazing, mowing, and construction) and climate change (such as precipitation, temperature, wind soil, and nutrient) (Zhu et al., 2016). Previous studies were concentrated on the effects of exclusion grazing on grasslands in north Tibet (Spooner et al., 2002; Xiong et al., 2014; Tai et al., 2021). However, it is rarely reported how fencing vs. grazing influences the grassland ecosystem in central Tibet. In the current study, we first demonstrated that grazing alerted alpha and beta diversity compared to grazing exclusion. Next, we detected that grazing activity decreased community AGB, and heavy grazing decreased soil total nitrogen and soil organic carbon. Then, we found that heavy grazing changed the positive relationships between AGB, STP, and Shannon–Wiener index to negative ones compared with grazing exclusion. A negative correlation was also found between Berger–Parker and Shannon–Wiener indices under each treatment. Finally, the GLM showed that heavy grazing altered diversity and productivity in central Tibet grassland. All potential mechanisms and causes are discussed below.
Effects of Grazing Intensity on Plant Community Assembly
Community dissimilarities were dependent on species composition and turnover (Figure 2A), strongly correlated with precipitation under C.K. Herbaceous communities exhibited high rates of temporal species turnover (Bar-Massada and Hadar, 2017), which can be explained by the modern co-existence theory (Chesson, 2000). On a given land, species turnover may be correlated with the different land management. Our results are similar to other scientists (Sanaei et al., 2018) who found that grazing intensity affected vegetation composition by enhancing species replacement. Peper et al. (2011) suggested that grazing should be considered in species composition shifts along ecological gradients. In this study, livestock frequently foraged for family Gramineae and Cyperaceae plants. Sheep grazing behavior can limit the growth of dominant species and increase habitat heterogeneity and species niches (Barot and Gignoux, 2004; Olden and Halme, 2016; Fischer et al., 2019). However, some other species cannot exist and may be lost due to sheep trampling (Su et al., 2005).
Grazing significantly influenced the diversity indices of alpine steppes in central Tibet. Specifically, grazing decreased the Shannon–Wiener index and increased the Berger–Parker index significantly compared to C.K. in 2016 to 2018 (Figure 3). The diversity and dominance of the community have changed greatly under H.G. It indicated that grazing altered the stability of the plant community. These findings were in line with findings that the Shannon–Wiener index under ungrazed sites was greater, but the Simpson dominance index was smaller under over-grazing sites in alpine Kobresia meadows in Tibet (Wei et al., 2008). However, with increasing grazing intensity, vegetation coverage decreased dramatically but had no effects on species richness, Shannon–Wiener index, and Pielou evenness index in Gangcha County, Qinghai Province (Chu et al., 2019). In the southern rangelands of Kenya, heavy grazing decreased species diversity, richness, and evenness (Jawuoro et al., 2017). Dingaan et al. (2016) found no dramatic difference in species richness between L.G. and H.G. sites in Kimberley. Therefore, due to different study areas and research objects, grazing effects on diversity are significantly different.
Effects of Grazing Intensity on Soil Nutrients and AGB
Grazing leads to a reduction in productivity. Our results showed that AGB decreased significantly with the increase of grazing intensity (Figure 4A). The livestock can directly reduce the AGB by eating a large amount of edible herbage (such as Gramineae, etc.). Removing the plant AGB will directly limit the plant growth and reproduction, and this process will redistribute productivities of photosynthesis (Hardman-Mountford et al., 2013). However, these results were inconsistent with other scientists. Olofsson et al. (2001) thought heavy grazing enhanced primary productivity of summer ranges, while moderate grazing decreased primary productivity in northern Norway. These results conceived that grazing activities are the main factors causing changes in grassland productivity, but others believed that grazing disturbance had a greater indirect effect on AGB via plant diversity (Zhang R. et al., 2018). Therefore, it is necessary to detect whether grazing directly affects plant productivity in a future study.
Grazing may affect soil nutrient parameters (i.e., organic matters, soil total and available nitrogen, and soil total and available phosphorus). Our results showed that grazing had dramatically adverse effects on soil nutrients. Specifically, heavy grazing significantly influenced STN and STP comparing to grazing exclusion, but moderate grazing significantly increased both nutrients. Our results agree with Frank et al. (1995), Steffens et al. (2008), Talore et al. (2016), and Dai et al. (2021). Moreover, Wu et al. (2010) had reported that grazing could influence soil carbon pool and nitrogen sequestration and storage in the Qinghai–Tibetan Plateau. Dong et al. (2012) also found that high grazing intensity decreased soil organic carbon and total nitrogen than low grazing intensity did. A meta-analysis concluded that grazing significantly decreased soil organic carbon, total soil nitrogen, and total soil phosphorus (Liu et al., 2021).
Furthermore, overgrazing significantly and directly increased the exposure of soil surface and water erosion storage in grassland ecosystems (Wang et al., 2011). This may be attributed to trampling by heavy grazing that is much greater than other treatments on alpine grassland. Trampling can increase the exposure of topsoil and wind erosion and reduce SOM (Wang et al., 2011). Additionally, topsoil exposure made soil temperatures down, which can accelerate the rate of soil organic matter decay and mineralization, thus declining soil organic matter levels. Soil parameter changes are closely associated with vegetation deterioration or over-grazing (Dong et al., 2012). Soil is the physical support of vegetation growth and the main source of plant nutrients, so they often independently interplay with each other (Li et al., 2014). Su et al. (2005) found that continuous grazing dramatically decreased vegetation coverage, plant litters, soil organic carbon, and soil nitrogen contents. Thus, the vegetation and soil influences restrict each other and coordinate development as a unified interaction network. Therefore, grazing by livestock directly affects vegetation and soil characteristics (Gao et al., 2009), but the soil characteristic change will react on the vegetation (Olofsson et al., 2001), thus forming a complicated vegetation–soil network.
Effects of Grazing Intensity on the Biodiversity–Productivity Relationship
In grazing ecosystems, soil management profoundly impacts ecosystem stability (Tscharntke et al., 2005). Soil properties are important for formations of vegetation productivity, species richness, and community assembly (Dingaan et al., 2016). Particularly, soil organic carbon, nitrogen, and phosphorus are highly essential for plant growth and productivity, which are affected by physical, chemical, and biological factors in multiple ways (Yuan and Jiang, 2021). In our study, the SOC and STP significantly negatively correlate with AGB in all treatments (Figure 5). It maybe that forage growth and compensatory growth absorb and utilize more soil nutrients (i.e., SOC and STP), which caused more AGB and less soil nutrient content (Yuan et al., 2015), as Fay et al. (2015) also proved that plant diversity and productivity are closely related to soil nutrients. Another reason is that livestock ingested plant aboveground biomass (such as plant litter and green leaves), causing decreasing SOC content (Yan et al., 2020) and increasing AGB (Yang et al., 2019). Furthermore, our GLM results also showed that AGB decreased significantly with the increase of the values of SOC and STP. More interesting, grazing dramatically altered the slopes of AGB along with SOC and STP compared with grazing exclusion (Figures 6A,C). The possible reasons are that the C.K. had higher vegetation coverage (62.67 ± 1.17%, Table 1), more litter, and moister topsoil; hence, litter decomposition rate and content of SOC were higher in C.K. than M.G. and H.G. (Dimitrakopoulos, 2010). Meanwhile, litter may hinder plant growth and decrease aboveground biomass (He et al., 2012), as intensive grazing has affected and changed soil nutrients and further ignited to changing of ecosystem functions (Li et al., 2018). Previous studies manifested that grazing caused decreasing of AGB and plant coverage, increasing of soil water evaporation and erosion, and decreasing SOC and STN consequently (Rickart et al., 2013; Lu et al., 2017; Su et al., 2017; Zhang M. et al., 2018). Therefore, plant AGB and soil nutrient availability were directly or indirectly affected by livestock.
Grazing exhibited no significant influence on the Shannon–Wiener index and SOC and STN (Figure 5). The finding seems to be similar to Wang et al. (2017), who demonstrated that grazing intensity had no effects on plant composition and soil properties across Tibet. Moreover, the pattern of the Shannon–Wiener index declined with SOC dramatically in grazing exclusion, while the pattern of the Shannon–Wiener index increased with SOC without significance in heavy grazing (Figure 6D). However, the pattern of the Berger–Parker index increased with SOC significantly in grazing exclusion (Figure 6G), which was opposite with the pattern of the Shannon–Wiener index with SOC in grazing exclusion. The relation varies in the study may be due to the highly significant negative correlation of the Shannon–Wiener index with the Berger–Parker index at all treatments (Figures 5, 6L). Our results correspond to previous studies (Prober and Wiehl, 2012; Wang et al., 2017). One possible reason is that grazing had changed the species competitively. Specifically, heavy grazing reduced the competitive exclusion among plants. Another possible reason is that heavy grazing had relatively higher urine and dung contents and more trampling (Mipam et al., 2021).
Heavy grazing altered the relationship between diversity and productivity. Specifically, the Shannon–Wiener index–AGB relationships tended to be positive on grazing exclusion, but a negative relationship was detected on heavy grazing (Figure 6J). These outcomes seem to be similar to other studies, namely, the patterns of diversity and biomass display a positive or negative linear trend across different grassland ecosystems of the Qinghai–Tibetan Plateau (Zhang et al., 2011; Milchunas et al., 2012). These variations in the study may be due to unavailable water, temperature, precipitation, and soil nutrient limitation on a different spatial scale for natural grassland (Zobel and Paertel, 2008; Li et al., 2019) and maybe driven by sample effects (Wolda, 1981), density-dependent factors, plant biomass, livestock grazing intensity, or other potential factors on a local scale (He et al., 2005; Ma et al., 2010). Grazing can significantly influence plant diversity and productivity patterns of plant communities (Guo and Berry, 1998). The major differences between the positive linear relationship at grazing exclusion and negative linear relationship at heavy grazing we obtained in this study could be connected with livestock grazing activities (Rillig et al., 2019). The results are similar to other researchers, who found that the patterns of biodiversity–productivity were overall linear models in alpine grassland but changed with temporal–spatial scales (Du et al., 2003). Li et al. (2019) found that a plant community with relatively few individuals of each species and concurrently with more species could be accompanied by bigger plant biomass, so the species richness and Shannon–Wiener index have a positive linear pattern with both above- and below-ground biomass. Comparing to our study, with lack of grazing livestock activities, each species in a plant community could fully use natural resources (e.g., light, available water, and soil nutrients) for growth (Zuo et al., 2012). However, when grazing at grassland, the livestock could alter the stability of plant community and plant species’ competitiveness or even lead to plant species’ niche changing (Pavlu et al., 2021). One possible reason is that the response rate of AGB and species diversity on grazing is different in plant community. In other words, heavy grazing decreased AGB remarkably (Figure 4A) but gradually changed plant diversity (Figures 2, 3). It indicated that the community stability of diversity and productivity exhibits hysteresis concerning grazing (Cipriotti et al., 2019). Another possible reason is that grazing altered the plant species’ competitive exclusion within communities (Huston, 1979; DeMalach et al., 2016) and plant species’ synchrony. Plant competition and synchrony may be different in communities (Sanaei et al., 2018). Grazing activities released the plant competition for light and niche breadth (Borer et al., 2014). In our study, heavy grazing decreased AGB significantly (Figure 4A), resulting in decreasing competition among plant species and increasing remnant niche breadth of other species; thus, the poorly competitive species may colonize into the communities. Therefore, heavy grazing can influence plant diversity–productivity relationship at small scales through plant competition and colonization (Fayiah et al., 2019). So, it is critical to reveal the effects of species losses on ecosystem functioning (Mulder et al., 2004).
Conclusion
This study suggested that heavy grazing lead to high species replacement and greatly changed the species diversity in plant community. Intensified grazing decreased plant productivity and SOC. Furthermore, a positive relationship between the Shannon–Wiener diversity and AGB was found under the condition of grazing exclusion, but a negative relationship was detected on heavy grazing. In conclusion, heavy grazing altered plant community features, soil nutrients, and the patterns of biodiversity–productivity relationship. However, this study focused on alpine grassland in central Tibet. Therefore, it is critically necessary to set a reasonable grazing intensity based on alpine grassland carrying capacity at different areas in Tibet.
Data Availability Statement
The raw data supporting the conclusions of this article will be made available by the authors, without undue reservation.
Author Contributions
MX: concepts, ideas, experimental design, data collection, and analysis. JXW: experimental design, interpretation of the results, and editing. JJW: data collection and analysis. YG: experimental design and data collection. DL: ideas, experimental design, interpretation of the results, and editing. YP: editing and part of data collection. XZ: interpretation of the results. All authors have reviewed and approved the final manuscript.
Funding
This work was funded by the National Key Research and Development Program of China (Grant No. 2016YFC0502004) and the Second Tibetan Plateau Scientific Expedition and Research (STEP) Program (Grant No. 2019QZKK1002).
Conflict of Interest
The authors declare that the research was conducted in the absence of any commercial or financial relationships that could be construed as a potential conflict of interest.
Acknowledgments
The authors thank Ciren, Xiaoqing Zhang, Gusangqunzong, Huaiming Jin, and Zhennan Li for assistance in experimenting and Ben Niu, Zhipeng Wang, and Yu Zhang for data analysis and comments on earlier versions of the manuscript.
References
Adler, P. B., Raff, D. A., and Lauenroth, W. K. (2001). The effect of grazing on the spatial heterogeneity of vegetation. Oecologia 128, 465–479. doi: 10.1007/s004420100737
Almufti, M. M., Sydes, C. L., Furness, S. B., Grime, J. P., and Band, S. R. (1977). Quantitative-analysis of shoot phenology and dominance in herbaceous vegetation. J. Ecol. 65, 759–791. doi: 10.2307/2259378
Axmanova, I., Chytry, M., Danihelka, J., Lustyk, P., Koci, M., Kubesova, S., et al. (2013). Plant species richness-productivity relationships in a low-productive boreal region. Plant Ecol. 214, 207–219. doi: 10.1007/s11258-012-0160-z
Bai, Y., Wu, J., Pan, Q., Huang, J., Wang, Q., Li, F., et al. (2007). Positive linear relationship between productivity and diversity: evidence from the Eurasian Steppe. J. Appl. Ecol. 44, 1023–1034. doi: 10.1111/j.1365-2664.2007.01351.x
Bar-Massada, A., and Hadar, L. (2017). Grazing and temporal turnover in herbaceous communities in a Mediterranean landscape. J. Veg. Sci. 28, 270–280. doi: 10.1111/jvs.12489
Barot, S., and Gignoux, J. (2004). Mechanisms promoting plant coexistence: can all the proposed processes be reconciled? Oikos 106, 185–192. doi: 10.1111/j.0030-1299.2004.13038.x
Baselga, A. (2010). Partitioning the turnover and nestedness components of beta diversity. Glob. Ecol. Biogeogr. 19, 134–143. doi: 10.1111/j.1466-8238.2009.00490.x
Borer, E. T., Seabloom, E. W., Gruner, D. S., Harpole, W. S., Hillebrand, H., Lind, E. M., et al. (2014). Herbivores and nutrients control grassland plant diversity via light limitation. Nature 508, 517–520. doi: 10.1038/nature13144
Cao, G., Tang, Y., Mo, W., Wang, Y., Li, Y., and Zhao, X. (2004). Grazing intensity alters soil respiration in an alpine meadow on the Tibetan plateau. Soil Biol. Biochem. 36, 237–243. doi: 10.1016/j.soilbio.2003.09.010
Cardinale, B. J., Duffy, J. E., Gonzalez, A., Hooper, D. U., Perrings, C., Venail, P., et al. (2012). Biodiversity loss and its impact on humanity. Nature 486, 59–67. doi: 10.1038/nature11148
Chen, B. X., Zhang, X. Z., Tao, J., Wu, J. S., Wang, J. S., Shi, P. L., et al. (2014). The impact of climate change and anthropogenic activities on alpine grassland over the Qinghai-Tibet Plateau. Agric. For. Meteorol. 189, 11–18. doi: 10.1016/j.agrformet.2014.01.002
Chesson, P. (2000). Mechanisms of maintenance of species diversity. Annu. Rev. Ecol. Syst. 31, 343–366. doi: 10.1146/annurev.ecolsys.31.1.343
Chu, H., Zhang, C., Dong, Q., Shang, Z., Degen, A. A., Yang, X., et al. (2019). The effect of grazing intensity and season on the soil seed bank and its relation with above-ground vegetation on the alpine steppe. Agric. Ecosyst. Environ. 285:106622. doi: 10.1016/j.agee.2019.106622
Cingolani, A. M., Noy-Meir, I., and Diaz, S. (2005). Grazing effects on rangeland diversity: a synthesis of contemporary models. Ecol. Appl. 15, 757–773. doi: 10.1890/03-5272
Cipriotti, P. A., Aguiar, M. R., Wiegand, T., and Paruelo, J. M. (2019). Combined effects of grazing management and climate on semi-arid steppes: hysteresis dynamics prevent recovery of degraded rangelands. J. Appl. Ecol. 56, 2155–2165. doi: 10.1111/1365-2664.13471
Dai, L., Fu, R., Guo, X., Du, Y., Lin, L., Zhang, F., et al. (2021). Long-term grazing exclusion greatly improve carbon and nitrogen store in an alpine meadow on the northern Qinghai-Tibet Plateau. CATENA 197:104955. doi: 10.1016/j.catena.2020.104955
DeMalach, N., Zaady, E., Weiner, J., and Kadmon, R. (2016). Size asymmetry of resource competition and the structure of plant communities. J. Ecol. 104, 899–910. doi: 10.1111/1365-2745.12557
Dias, R. A., Gianuca, A. T., Vizentin-Bugoni, J., Goncalves, M. S. S., Bencke, G. A., and Bastazini, V. A. G. (2017). Livestock disturbance in Brazilian grasslands influences avian species diversity via turnover. Biodivers. Conserv. 26, 2473–2490. doi: 10.1007/s10531-017-1370-4
Dimitrakopoulos, P. G. (2010). Influence of evenness on the litter-species richnes“ decomposition relationship in Mediterranean grasslands. J. Plant Ecol. 3, 71–78. doi: 10.1093/JPE/RTQ009
Dingaan, M. N. V., Walker, S., Tsubo, M., and Newby, T. (2016). Influence of grazing on plant diversity-productivity relationship in semi-arid grassland of South Africa. Appl. Ecol. Environ. Res. 14, 1–13. doi: 10.15666/aeer/1404_001013
Dong, Q. M., Zhao, X. Q., Wu, G. L., Shi, J. J., Wang, Y. L., and Sheng, L. (2012). Response of soil properties to yak grazing intensity in a Kobresia parva-meadow on the Qinghai–Tibetan Plateau, China. J. Soil Sci. Plant Nutr. 12, 535–546. doi: 10.4067/S0718-95162012005000024
Du, G., Qin, G., Li, Z., Liu, Z., and Dong, G. (2003). Relationship between species richness and productivity in an alpine meadow plant community. Acta Phytoecol. Sin. 27, 125–132.
Erfanzadeh, R., Omidipour, R., and Faramarzi, M. (2015). Variation of plant diversity components in different scales in relation to grazing and climatic conditions. Plant Ecol. Divers. 8, 537–545. doi: 10.1080/17550874.2015.1033774
Fay, P. A., Prober, S. M., Harpole, W. S., Knops, J. M. H., Bakker, J. D., Borer, E. T., et al. (2015). Grassland productivity limited by multiple nutrients. Nat. Plants 1:15080. doi: 10.1038/nplants.2015.80
Fayiah, M., Dong, S. K., Li, Y., Xu, Y. D., Gao, X. X., Li, S., et al. (2019). The relationships between plant diversity, plant cover, plant biomass and soil fertility vary with grassland type on Qinghai-Tibetan Plateau. Agric. Ecosyst. Environ. 286:106659. doi: 10.1016/j.agee.2019.106659
Fischer, F. M., Bonnet, O. J. F., Cezimbra, I. M., and Pillar, V. D. (2019). Long-term effects of grazing intensity on strategies and spatial components of functional diversity in subtropical grassland. Appl. Veg. Sci. 22, 39–47. doi: 10.1111/avsc.12402
Frank, A. B., Tanaka, D. L., Hofmann, L., and Follett, R. F. (1995). Soil carbon and nitrogen of northern great-plains grasslands as influenced by long-term grazing. J. Range Manag. 48, 470–474. doi: 10.2307/4002255
Gao, Q.-Z., Wan, Y.-F., Xu, H.-M., Li, Y., Jiangcun, W.-Z., and Borjigidai, A. (2010). Alpine grassland degradation index and its response to recent climate variability in Northern Tibet, China. Quat. Int. 226, 143–150. doi: 10.1016/j.quaint.2009.10.035
Gao, Y., Schumann, M., Chen, H., Wu, N., and Luo, P. (2009). Impacts of grazing intensity on soil carbon and nitrogen in an alpine meadow on the eastern Tibetan Plateau. J. Food Agric. Environ. 7, 749–754.
Gillman, L. N., and Wright, S. D. (2006). The influence of productivity on the species richness of plants: a critical assessment. Ecology 87, 1234–1243.
Gonzalez-Hernandez, M. P., Mouronte, V., Romero, R., Rigueiro-Rodriguez, A., and Mosquera-Losada, M. R. (2020). Plant diversity and botanical composition in an Atlantic heather-gorse dominated understory after horse grazing suspension: comparison of a continuous and rotational management. Glob. Ecol. Conserv. 23:e01134. doi: 10.1016/j.gecco.2020.e01134
Gough, L., Grace, J. B., and Taylor, K. L. (1994). The relationship between species richness and community biomass - the importance of environmental variables. Oikos 70, 271–279. doi: 10.2307/3545638
Grace, J. B., Anderson, T. M., Seabloom, E. W., Borer, E. T., Adler, P. B., Harpole, W. S., et al. (2016). Integrative modelling reveals mechanisms linking productivity and plant species richness. Nature 529, 390–393. doi: 10.1038/nature16524
Gross, K., and Cardinale, B. J. (2007). Does species richness drive community production or vice versa? Reconciling historical and contemporary paradigms in competitive communities. Am. Nat. 170, 207–220. doi: 10.1086/518950
Guo, Q. F., and Berry, W. L. (1998). Species richness and biomass: dissection of the hump-shaped relationships. Ecology 79, 2555–2559. doi: 10.2307/176844
Hardman-Mountford, N. J., Polimene, L., Hirata, T., Brewin, R. J. W., and Aiken, J. (2013). Impacts of light shading and nutrient enrichment geo-engineering approaches on the productivity of a stratified, oligotrophic ocean ecosystem. J. R. Soc. Interface 10:20130701. doi: 10.1098/rsif.2013.0701
He, F., Wang, K., Wan, L., Li, C., and Li, X. (2012). Influence of litter and standing litter on soil microenvironment and plant growth. Chin. J. Grassl. 34, 19–23.
He, J., Wolfe, B., Kelly, S., Schmid, B., and Bazzaz, F. A. (2005). Density may alter diversity–productivity relationships in experimental plant communities. Basic Appl. Ecol. 6, 505–517. doi: 10.1016/j.baae.2005.04.002
Hector, A., and Bagchi, R. (2007). Biodiversity and ecosystem multifunctionality. Nature 448, 188–U186. doi: 10.1038/nature05947
Huston, M. (1979). General hypothesis of species-diversity. Am. Nat. 113, 81–101. doi: 10.1086/283366
Jawuoro, S. O., Koech, O. K., Karuku, G. N., and Mbau, J. S. (2017). Plant species composition and diversity depending on piospheres and seasonality in the southern rangelands of Kenya. Ecological Processes 6:16. doi: 10.1186/s13717-017-0083-7
Leprieur, F., Albouy, C., De Bortoli, J., Cowman, P. F., Bellwood, D. R., and Mouillot, D. (2012). Quantifying phylogenetic beta diversity: distinguishing between ‘true’ turnover of lineages and phylogenetic diversity gradients. PLoS One 7:e42760. doi: 10.1371/journal.pone.0042760
Li, G., Zhang, Z., Shi, L. L., Zhou, Y., Yang, M., Cao, J. X., et al. (2018). Effects of different grazing intensities on soil C, N, and P in an alpine meadow on the QinghaiTibetan Plateau, China. Int. J. Environ. Res. Public Health 15:2584. doi: 10.3390/ijerph15112584
Li, Y., Dong, S., Liu, S., Su, X., Wang, X., Zhang, Y., et al. (2019). Relationships between plant diversity and biomass production of alpine grasslands are dependent on the spatial scale and the dimension of biodiversity. Ecol. Eng. 127, 375–382. doi: 10.1016/j.ecoleng.2018.12.015
Li, Y., Dong, S., Liu, S., Wang, X., Wen, L., and Wu, Y. (2014). The interaction between poisonous plants and soil quality in response to grassland degradation in the alpine region of the Qinghai-Tibetan Plateau. Plant Ecol. 215, 809–819. doi: 10.1007/s11258-014-0333-z
Liang, J. J., Crowther, T. W., Picard, N., Wiser, S., Zhou, M., Alberti, G., et al. (2016). Positive biodiversity-productivity relationship predominant in global forests. Science 354:aaf8957. doi: 10.1126/science.aaf8957
Lin, Y., Hong, M., Han, G., Zhao, M., Bai, Y., and Chang, S. X. (2010). Grazing intensity affected spatial patterns of vegetation and soil fertility in a desert steppe. Agric. Ecosyst. Environ. 138, 282–292. doi: 10.1016/j.agee.2010.05.013
Liu, C. L., Li, W. L., Xu, J., Wei, W., Xue, P. F., and Yan, H. P. (2021). Response of soil nutrients and stoichiometry to grazing management in alpine grassland on the Qinghai-Tibet Plateau. Soil Tillage Res. 206:104822. doi: 10.1016/j.still.2020.104822
Liu, X., Ma, Z., Huang, X., and Li, L. (2020). How does grazing exclusion influence plant productivity and community structure in alpine grasslands of the Qinghai-Tibetan Plateau? Glob. Ecol. Conserv. 23:e01066. doi: 10.1016/j.gecco.2020.e01066
Lu, X., Kelsey, K., Yan, Y., Sun, J., Wang, X., Cheng, G., et al. (2017). Effects of grazing on ecosystem structure and function of alpine grasslands in Qinghai-Tibetan Plateau: a synthesis. Ecosphere 8:16. doi: 10.1002/ecs2.1656
Ma, W., He, J., Yang, Y., Wang, X., Liang, C., Anwar, M., et al. (2010). Environmental factors covary with plant diversity-productivity relationships among Chinese grassland sites. Glob. Ecol. Biogeogr. 19, 233–243. doi: 10.1111/j.1466-8238.2009.00508.x
Mcnaughton, S. J. (1983). Compensatory plant-growth as a response to herbivory. Oikos 40, 329–336. doi: 10.2307/3544305
Milchunas, D. G., Lauenroth, W. K., and Burke, I. C. (2012). Livestock grazing: animal and plant biodiversity of shortgrass steppe and the relationship to ecosystem function. Oikos 83, 65–74. doi: 10.2307/3546547
Mipam, T. D., Chen, S. Y., Liu, J. Q., Miehe, G., and Tian, L. M. (2021). Short-term yak-grazing alters plant-soil stoichiometric relations in an alpine meadow on the eastern Tibetan Plateau. Plant Soil 458, 125–137. doi: 10.1007/s11104-019-04401-6
Morris, E. K., Caruso, T., Buscot, F., Fischer, M., Hancock, C., Maier, T. S., et al. (2014). Choosing and using diversity indices: insights for ecological applications from the German Biodiversity Exploratories. Ecol. Evol. 4, 3514–3524. doi: 10.1002/ece3.1155
Mulder, C. P. H., Bazeley-White, E., Dimitrakopoulos, P. G., Hector, A., Scherer-Lorenzen, M., and Schmid, B. (2004). Species evenness and productivity in experimental plant communities. Oikos 107, 50–63. doi: 10.1111/j.0030-1299.2004.13110.x
Olden, A., and Halme, P. (2016). Grazers increase beta-diversity of vascular plants and bryophytes in wood-pastures. J. Veg. Sci. 27, 1084–1093. doi: 10.1111/jvs.12436
Olofsson, J., Kitti, H., Rautiainen, P., Stark, S., and Oksanen, L. (2001). Effects of summer grazing by reindeer on composition of vegetation, productivity and nitrogen cycling. Ecography 24, 13–24. doi: 10.1034/j.1600-0587.2001.240103.x
Pavlu, L., Pavlu, V. V., and Fraser, M. D. (2021). What is the effect of 19 years of restoration managements on soil and vegetation on formerly improved upland grassland? Sci. Total Environ. 755:142469. doi: 10.1016/j.scitotenv.2020.142469
Peper, J., Jansen, F., Pietzsch, D., and Manthey, M. (2011). Patterns of plant species turnover along grazing gradients. J. Veg. Sci. 22, 457–466. doi: 10.1111/j.1654-1103.2011.01260.x
Prober, S. M., and Wiehl, G. (2012). Relationships among soil fertility, native plant diversity and exotic plant abundance inform restoration of forb-rich eucalypt woodlands. Divers. Distrib. 18, 795–807. doi: 10.1111/j.1472-4642.2011.00872.x
Rada, S., Mazalová, M., Šipoš, J., and Kuras, T. (2014). Impacts of mowing, grazing and edge effect on orthoptera of submontane grasslands: perspectives for biodiversity protection. Pol. J. Ecol. 62, 123–138. doi: 10.3161/104.062.0112
Rickart, E. A., Bienek, K. G., and Rowe, R. J. (2013). Impact of livestock grazing on plant and small mammal communities in the Ruby Mountains, Northeastern Nevada. West. N. Am. Nat. 73, 505–515. doi: 10.3398/064.073.0403
Rillig, M. C., Ryo, M., Lehmann, A., Aguilar-Trigueros, C. A., Buchert, S., Wulf, A., et al. (2019). The role of multiple global change factors in driving soil functions and microbial biodiversity. Science 366, 886–890. doi: 10.1126/science.aay2832
Rusina, S., Puspure, I., and Gustina, L. (2013). Diversity patterns in transitional grassland areas in floodplain landscapes with different heterogeneity. Tuexenia 33, 347–369. doi: 10.1523/JNEUROSCI.1442-15.2015
Sanaei, A., Ali, A., and Chahouki, M. A. Z. (2018). The positive relationships between plant coverage, species richness, and aboveground biomass are ubiquitous across plant growth forms in semi-steppe rangelands. J. Environ. Manag. 205, 308–318. doi: 10.1016/j.jenvman.2017.09.079
Schulz, K., Guschal, M., Kowarik, I., de Almeida-Cortez, J. S., Sampaio, E. V. D. B., and Cierjacks, A. (2019). Grazing reduces plant species diversity of Caatinga dry forests in northeastern Brazil. Appl. Veg. Sci. 22, 348–359. doi: 10.1111/avsc.12434
Spooner, P., Lunt, I., and Robinson, W. (2002). Is fencing enough? The short-term effects of stock exclusion in remnant grassy woodlands in southern NSW. Ecol. Manag. Restor. 3, 117–126. doi: 10.1046/j.1442-8903.2002.00103.x
Steffens, M., Koelbl, A., Totsche, K. U., and Koegel-Knabner, I. (2008). Grazing effects on soil chemical and physical properties in a semiarid steppe of Inner Mongolia (PR China). Geoderma 143, 63–72. doi: 10.1016/j.geoderma.2007.09.004
Su, R., Cheng, J., Chen, D., Bai, Y., Jin, H., Chao, L., et al. (2017). Effects of grazing on spatiotemporal variations in community structure and ecosystem function on the grasslands of Inner Mongolia, China. Sci. Rep. 7:40. doi: 10.1038/s41598-017-00105-y
Su, Y., Li, Y., Cui, J., and Zhao, W. (2005). Influences of continuous grazing and livestock exclusion on soil properties in a degraded sandy grassland, Inner Mongolia, northern China. Catena 59, 267–278. doi: 10.1016/j.catena.2004.09.001
Tai, X. L., Epstein, H. E., and Li, B. (2021). Effects of grazing exclusion on spring and autumn pastures in arid regions of China: Insights from field surveys and landsat images. Agric. Ecosyst. Environ. 310:107302. doi: 10.1016/j.agee.2021.107302
Talore, D. G., Tesfamariam, E. H., Hassen, A., Du Toit, J. C. O., Klampp, K., and Jean-Francois, S. (2016). Long-term impacts of grazing intensity on soil carbon sequestration and selected soil properties in the arid Eastern Cape, South Africa. J. Sci. Food Agric. 96, 1945–1952. doi: 10.1002/jsfa.7302
Tonn, B., Densing, E. M., Gabler, J., and Isselstein, J. (2019). Grazing-induced patchiness, not grazing intensity, drives plant diversity in European low-input pastures. J. Appl. Ecol. 56, 1624–1636. doi: 10.1111/1365-2664.13416
Tscharntke, T., Klein, A. M., Kruess, A., Steffan-Dewenter, I., and Thies, C. (2005). Landscape perspectives on agricultural intensification and biodiversity–ecosystem service management. Ecol. Lett. 8, 857–874. doi: 10.1111/j.1461-0248.2005.00782.x
Wang, X., Yan, Y., and Cao, Y. (2011). Impact of historic grazing on steppe soils on the northern Tibetan Plateau. Plant Soil 354, 173–183. doi: 10.1007/s11104-011-1053-y
Wang, Y., Heberling, G., Gorzen, E., Miehe, G., Seeber, E., and Wesche, K. (2017). Combined effects of livestock grazing and abiotic environment on vegetation and soils of grasslands across Tibet. Appl. Veg. Sci. 20, 327–339. doi: 10.1111/avsc.12312
Wang, Z., Luo, T. X., Li, R. C., Tang, Y. H., and Du, M. Y. (2013). Causes for the unimodal pattern of biomass and productivity in alpine grasslands along a large altitudinal gradient in semi-arid regions. J. Veg. Sci. 24, 189–201. doi: 10.1111/j.1654-1103.2012.01442.x
Wei, X. H., Yang, P., Li, S., Dong, Y. X., and Zhang, C. L. (2008). Effects of over grazing on plant species diversity of alpine kobresia (Kobresia pygmaea) meadow in Tibet. Proc. China Assoc. Sci. Technol. 4, 190–197.
White, H. J., Gaul, W., Sadykova, D., Leon-Sanchez, L., Caplat, P., Emmerson, M. C., et al. (2019). Land cover drives large scale productivity-diversity relationships in Irish vascular plants. PeerJ 7:e7035. doi: 10.7717/peerj.7035
Wolda, H. (1981). Similarity indexes, sample-size and diversity. Oecologia 50, 296–302. doi: 10.1007/bf00344966
Wu, G., Du, G., Liu, Z., and Thirgood, S. (2009). Effect of fencing and grazing on a Kobresia-dominated meadow in the Qinghai-Tibetan Plateau. Plant Soil 319, 115–126. doi: 10.1007/s11104-008-9854-3
Wu, G. L., Liu, Z. H., Zhang, L., Chen, J. M., and Hu, T. M. (2010). Long-term fencing improved soil properties and soil organic carbon storage in an alpine swamp meadow of western China. Plant Soil 332, 331–337. doi: 10.1007/s11104-010-0299-0
Wu, J., Li, M., Fiedler, S., Ma, W., Wang, X., Zhang, X., et al. (2019a). Impacts of grazing exclusion on productivity partitioning along regional plant diversity and climatic gradients in Tibetan alpine grasslands. J. Environ. Manag. 231, 635–645. doi: 10.1016/j.jenvman.2018.10.097
Wu, J., Shen, Z., and Zhang, X. (2014a). Precipitation and species composition primarily determine the diversity–productivity relationship of alpine grasslands on the Northern Tibetan Plateau. Alp. Bot. 124, 13–25. doi: 10.1007/s00035-014-0125-z
Wu, J., Song, M., Ma, W., Zhang, X., Shen, Z., Tarolli, P., et al. (2019b). Plant and soil’s delta N-15 are regulated by climate, soil nutrients, and species diversity in alpine grasslands on the northern Tibetan Plateau. Agric. Ecosyst. Environ. 281, 111–123. doi: 10.1016/j.agee.2019.05.011
Wu, J., Zhang, X., Shen, Z., Shi, P., Yu, C., and Chen, B. (2014b). Effects of livestock exclusion and climate change on aboveground biomass accumulation in alpine pastures across the Northern Tibetan Plateau. Chin. Sci. Bull. 59, 4332–4340. doi: 10.1007/s11434-014-0362-y
Wu, J. G. (2013). Landscape sustainability science: ecosystem services and human well-being in changing landscapes. Landsc. Ecol. 28, 999–1023. doi: 10.1007/s10980-013-9894-9
Xiang, M., Guo, Y., Gu, S., Zhang, X., Pan, Y., Wu, J., et al. (2019). Effects of grazing intensity on plant community and species diversity of temperate Steppe in Lhasa River Valley. Acta Agrestia Sin. 27, 668–674. doi: 10.11733/j.issn.1007-0435.2019.03.020
Xiong, D., Shi, P., Sun, Y., Wu, J., and Zhang, X. (2014). Effects of grazing exclusion on plant productivity and soil carbon, nitrogen storage in alpine meadows in northern Tibet, China. Chin. Geogr. Sci. 24, 488–498. doi: 10.1007/s11769-014-0697-y
Yan, L., Li, Y., Wang, L., Zhang, X., Wang, J., Wu, H., et al. (2020). Grazing significantly increases root shoot ratio but decreases soil organic carbon in Qinghai-Tibetan Plateau grasslands: a hierarchical meta-analysis. Land Degrad. Dev. 31, 2369–2378. doi: 10.1002/ldr.3606
Yang, C., Zhang, Y., Hou, F., Millner, J. P., Wang, Z., and Chang, S. (2019). Grazing activity increases decomposition of yak dung and litter in an alpine meadow on the Qinghai-Tibet plateau. Plant Soil 444, 239–250. doi: 10.1007/s11104-019-04272-x
Yi, S. H., Xiang, B., Meng, B. P., Wu, X. D., and Ding, Y. J. (2019). Modeling the carbon dynamics of alpine grassland in the Qinghai-Tibetan Plateau under scenarios of 1.5 and 2 degrees C global warming. Adv. Clim. Change Res. 10, 80–91. doi: 10.1016/j.accre.2019.06.001
Yuan, Z. Q., Epstein, H., and Li, F. M. (2015). Factors affecting the recovery of abandoned semi-arid fields after legume introduction on the Loess Plateau. Ecol. Eng. 79, 86–93. doi: 10.1016/j.ecoleng.2015.03.012
Yuan, Z.-Q., and Jiang, X. (2021). Vegetation and soil covariation, not grazing exclusion, control soil organic carbon and nitrogen in density fractions of alpine meadows in a Tibetan permafrost region. CATENA 196:104832. doi: 10.1016/j.catena.2020.104832
Zhang, M., Li, X., Wang, H., and Huang, Q. (2018). Comprehensive analysis of grazing intensity impacts soil organic carbon: a case study in typical steppe of Inner Mongolia, China. Appl. Soil Ecol. 129, 1–12. doi: 10.1016/j.apsoil.2018.03.008
Zhang, Q., Niu, J., Buyantuyev, A., Zhang, J., Ding, Y., and Dong, J. (2011). Productivity-species richness relationship changes from unimodal to positive linear with increasing spatial scale in the Inner Mongolia steppe. Ecol. Res. 26, 649–658. doi: 10.1007/s11284-011-0825-4
Zhang, R., Wang, Z., Han, G., Schellenberg, M. P., Wu, Q., and Gu, C. (2018). Grazing induced changes in plant diversity is a critical factor controlling grassland productivity in the Desert Steppe, Northern China. Agric. Ecosyst. Environ. 265, 73–83. doi: 10.1016/j.agee.2018.05.014
Zhu, K., Chiariello, N. R., Tobeck, T., Fukami, T., and Field, C. B. (2016). Nonlinear, interacting responses to climate limit grassland production under global change. Proc. Natl. Acad. Sci. 113:10589. doi: 10.1073/pnas.1606734113
Zobel, M., and Paertel, M. (2008). What determines the relationship between plant diversity and habitat productivity? Glob. Ecol. Biogeogr. 17, 679–684. doi: 10.1111/j.1466-8238.2008.00400.x
Keywords: aboveground biomass, alpine grasslands, central Tibet, plant diversity, productivity-diversity relationships, grazing
Citation: Xiang M, Wu J, Wu J, Guo Y, Lha D, Pan Y and Zhang X (2021) Heavy Grazing Altered the Biodiversity–Productivity Relationship of Alpine Grasslands in Lhasa River Valley, Tibet. Front. Ecol. Evol. 9:698707. doi: 10.3389/fevo.2021.698707
Received: 22 April 2021; Accepted: 22 June 2021;
Published: 22 July 2021.
Edited by:
Bayartungalag Batsaikhan, Mongolian Academy of Sciences, MongoliaReviewed by:
Xuyang Lu, Institute of Mountain Hazards and Environment, Chinese Academy of Sciences, ChinaZhanhuan Shang, Lanzhou University, China
Copyright © 2021 Xiang, Wu, Wu, Guo, Lha, Pan and Zhang. This is an open-access article distributed under the terms of the Creative Commons Attribution License (CC BY). The use, distribution or reproduction in other forums is permitted, provided the original author(s) and the copyright owner(s) are credited and that the original publication in this journal is cited, in accordance with accepted academic practice. No use, distribution or reproduction is permitted which does not comply with these terms.
*Correspondence: Duo Lha, lhaduo@hotmail.com; Xianzhou Zhang, Zhangxz@igsnrr.ac.cn