- 1Programa de Doctorado en Ciencias Biomédicas, Universidad Nacional Autónoma de México, Ciudad de México, Mexico
- 2Departamento de Ecología y Recursos Naturales, Facultad de Ciencias, Universidad Nacional Autónoma de México, Ciudad de México, Mexico
The evolution of matrotrophy (post-fertilization maternal provisioning to developing embryos) has been explained through several hypotheses. Trexler and DeAngelis proposed in 2003 a theoretical model that defines the ecological conditions under which matrotrophy would be favored over lecithotrophy (pre-fertilization maternal provisioning). According to this model, matrotrophy offers a selective advantage in environments with abundant and constantly available food, whereas environments with limited and fluctuating food resources should instead promote a lecithotrophic mode of maternal provisioning. This model also proposes that matrotrophy entails the consequence of leaner reproductive females and in turn shorter lifespans. In this study, we examined the Trexler-DeAngelis model using data from 45 populations of five viviparous species from the fish genus Poeciliopsis (family Poeciliidae). We used the matrotrophy index (MI) as a measure of post-fertilization maternal provisioning, and the index of stomach fullness and individual body condition (BC) as proxies for food availability. We also estimated the magnitude of fluctuations in food availability by calculating the temporal variances of these two proxies. Neither abundant nor constantly available food were associated with greater degrees of matrotrophy, which fails to support the predictions of the Trexler-DeAngelis model with respect to the ecological drivers of increased post-fertilization provisioning to embryos. Nonetheless, in all five species we observed that females with greater degrees of matrotrophy had poorer BC compared to females that provided less nutrients to embryos after fertilization. This finding is consistent with one of the expected consequences of advanced matrotrophy according to the Trexler-DeAngelis model, namely, a detriment to the nutritional status of females. Our study provides compelling evidence that gestating females experience a trade-off between post-fertilization provisioning to embryos and self-maintenance, revealing in turn that matrotrophy is a costly reproductive strategy.
Introduction
Viviparity is a reproductive strategy in which the embryo develops inside a specialized structure or cavity within the female after fertilization (Blackburn, 1999). This reproductive strategy can be found in mammals, sharks, anurans, salamanders, snakes, lizards, and fishes (Shine and Bull, 1979; Guillette and Jones, 1985; Greven and Guex, 1994; Castro, 2009; Renfree et al., 2013; Wake, 2015). In addition, viviparity also occurs in numerous invertebrate taxa, including several species from the following classes: Arachnida, Chromadorea, Digenea, Demospongiae, Insecta, and Stenolaemata, among others (Hagan, 1931; Meier et al., 1999; Ostrovsky et al., 2009, 2016). Females of viviparous species provide nutrients to their developing embryos by means of two different mechanisms. The first mechanism is lecithotrophy, in which the mother transfers all nutrients to her embryos before fertilization in the form of yolk and does not transfer additional resources during their development (Blackburn, 1992). The second mechanism is matrotrophy, which unlike lecithotrophy, involves the continuous provisioning of nutrients from the mother to the developing embryos after fertilization, usually through specialized structures (e.g., placentas; Wourms, 1981; Lombardi and Wourms, 1985; Blackburn, 1992; Marsh-Matthews, 2011; Kwan et al., 2015; Olivera-Tlahuel et al., 2019).
Theoretical models about the evolution of matrotrophy from a lecithotrophic strategy suggest that food availability is an ecological factor that could promote changes in the way that mothers provide nutrients to their developing offspring. Trexler and DeAngelis (2003, 2010) proposed a model that suggests that the amount of food available in the environment may favor the evolution of maternal adaptations such as matrotrophy. They suggested that one of the benefits of matrotrophy is that females may be able to produce a greater number of embryos than lecithotrophic females. The reason for this expected difference in fecundity is that lecithotrophic eggs are larger and more energetically costly than matrotrophic eggs because they contain all the necessary nutrients to complete embryo development. In contrast, matrotrophic eggs are notably smaller and imply a lower energy demand at the onset of reproduction, which should allow females to initiate gestation with a larger brood. However, this benefit of higher fecundity for matrotrophic females would only be possible if pregnant females have constant access to enough food to simultaneously meet their own energetic demands (i.e., self-maintenance) and to provide nutrients to their embryos all throughout development. Therefore, matrotrophy should be favored in environments with constant and high food availability. Lecithotrophy, on the other hand, should be favored in environments where resource availability is low or highly variable, because lecithotrophic females do not need a constant surplus of nutrients to support embryo development, but instead can store nutrients in the form of yolk during periods of relatively high food availability (Trexler and DeAngelis, 2003, 2010).
Viviparous fishes of the family Poeciliidae exhibit wide variation in the amount of nutrients that females can transfer to their developing embryos before and after fertilization. Some species are strictly lecithotrophic (e.g., Gambusia hubbsi and Gambusia. alvarezi), whereas other species exhibit extensive matrotrophy (e.g., Poecilia parae and Poeciliopsis prolifica), with several species showing varying degrees of the relative amounts of pre- and post-fertilization provisioning (Reznick et al., 2002; Pires et al., 2007, 2010; Pollux et al., 2009; Pollux and Reznick, 2011; Torres-Mejia, 2011; Olivera-Tlahuel et al., 2015). In addition, some studies have demonstrated that the degree of matrotrophy (i.e., the amount of post-fertilization maternal provisioning) may also vary among different populations of the same species (Reznick et al., 2007; Turcotte et al., 2008; Pires et al., 2010; Molina-Moctezuma et al., 2020). Due to the large variation in the way poeciliid females provide resources to their embryos, some studies have attempted to understand the potential benefits of matrotrophy over lecithotrophy (Trexler and DeAngelis, 2003, 2010; Pollux et al., 2009, 2014; Saleh-Subaie and Zúñiga-Vega, 2019).
Several studies have previously tested the Trexler-DeAngelis model under laboratory (Marsh-Matthews and Deaton, 2006; Pires et al., 2007; Banet and Reznick, 2008; Banet et al., 2010; Pollux and Reznick, 2011; Itonaga et al., 2012; Van Dyke et al., 2014; Molina-Moctezuma et al., 2020) and natural conditions (Schrader and Travis, 2008, 2012; Riesch et al., 2013; Molina-Moctezuma et al., 2020) obtaining mixed results. Interestingly, most of these studies have been conducted on poeciliid fishes and only three studies have evaluated this model with non-fish taxa, specifically using lizards as model systems (Cadby et al., 2011; Itonaga et al., 2012; Van Dyke et al., 2014). Some authors suggest that the Trexler-DeAngelis model is at least partially supported according to the relatively high levels of matrotrophy and the tendency to decrease the size of oocytes observed in sites with high food availability in both natural and experimental conditions (Pires et al., 2007; Schrader and Travis, 2008, 2012; Pollux and Reznick, 2011; Itonaga et al., 2012; Riesch et al., 2013; Van Dyke et al., 2014; Molina-Moctezuma et al., 2020). Nonetheless, other authors suggest that the model is not supported because they did not observe a significant decrease in oocyte size in matrotrophic females, nor did they find differences in the level of matrotrophy in experiments where the amount of food was constant or variable (Marsh-Matthews and Deaton, 2006; Banet et al., 2010; Pollux and Reznick, 2011; Riesch et al., 2013).
Since no conclusive results have been found, neither under experimental or natural conditions, it is important to study the generality of this phenomenon to determine if a higher degree of matrotrophy (i.e., greater amount of post-fertilization provisioning) indeed occurs with greater quantity and stability of food. Therefore, the main objective of this study is to evaluate the Trexler-DeAngelis model (Trexler and DeAngelis, 2003, 2010). In particular, we address the specific conditions that would lead to increased matrotrophy by comparing natural populations of five species of the genus Poeciliopsis. We predict that females from populations where the amount of available food resources is relatively high and constant, will show higher levels of matrotrophy. In contrast, we expect less post-fertilization maternal provisioning in populations that inhabit sites where there is evidence that the amount of food is lower or fluctuating. We used two proxies for food availability: an index of stomach fullness (IF) and an estimate of body condition (BC). High IF and/or BC values indicate that females inhabit an environment with high food availability, whereas low IF and/or BC values indicate that females inhabit an environment with low food availability. In addition, we estimated the variance among months of both IF and BC to quantify the degree of temporal variability in food availability. In environments where food resources are constantly available (i.e., populations with small temporal variances in IF and/or BC), we expect to find higher degrees of matrotrophy, whereas in environments where food availability fluctuates (i.e., populations with large temporal variances in IF and/or BC), we expect to find females exhibiting less post-fertilization provisioning. Our study represents an empirical test of this important theoretical model that attempts to explain how different strategies for embryo nourishment have evolved in viviparous organisms.
Materials and Methods
Study Species and Datasets
We focused on five species of the genus Poeciliopsis (family Poeciliidae) with relatively wide geographic distributions, which allowed us to examine interpopulation differences in food availability and how such differences affect the patterns of embryo nourishment. All five species exhibit superfetation, which is the ability of females to simultaneously bear two or more groups of embryos at different developmental stages (Scrimshaw, 1944), but differ in the degree of matrotrophy (i.e., lecithotrophy, matrotrophy, and incipient matrotrophy) (Table 1). The number of populations differed among species, from 6 to 11, for a total of 45 populations. The number of females per population varied from 14 to 141 (Table 1). Geographic coordinates of all populations can be found in Supplementary Table 1. For Poeciliopsis gracilis, Poeciliopsis infans, and Poeciliopsis turrubarensis we combined data from published studies (Zúñiga-Vega et al., 2007; Frías-Alvarez et al., 2014; Frías-Álvarez and Zúñiga-Vega, 2016), and from our own dissections of pregnant females, which we obtained from the National Collection of Fishes (Instituto de Biología, Universidad Nacional Autónoma de México). For the remaining two species (Poeciliopsis baenschi and P. prolifica), all data was obtained by dissecting preserved females, also from the National Collection of Fishes (Table 1). All specimens were preserved in ethanol (70%) until they were dissected.
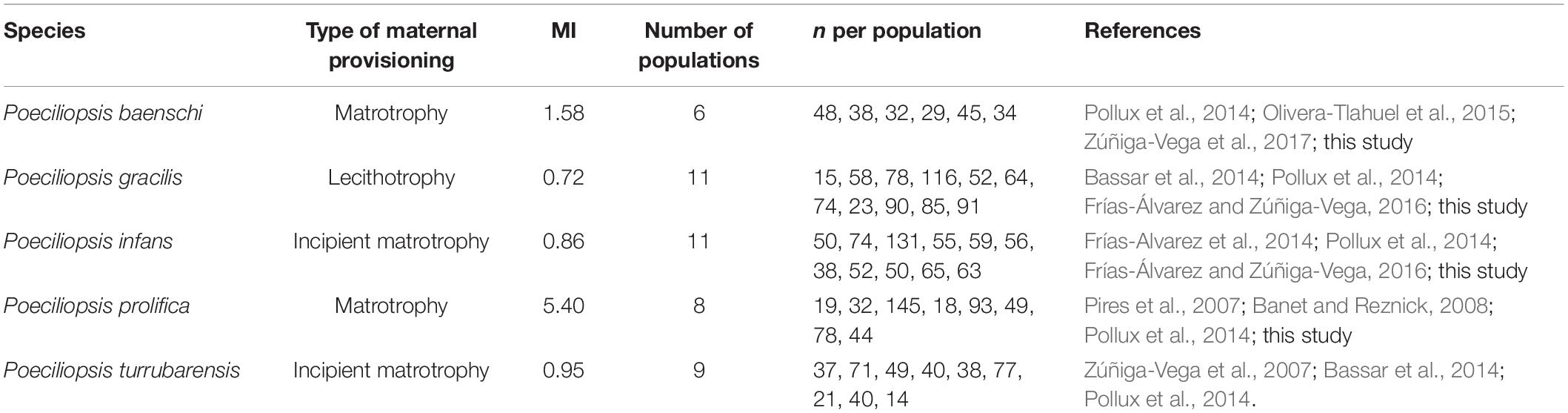
Table 1. List of the five study species from the fish genus Poeciliopsis with information on the type of maternal provisioning to developing embryos (matrotrophy, incipient matrotrophy, or lecithotrophy), matrotrophy index (MI), number of populations per species, and sample size (n = number of reproductive females) per population.
Laboratory Measurements
To test our hypothesis, we calculated the matrotrophy index (MI), and two proxies for food availability: the index of fullness (IF) and individual BC. To quantify these variables we measured the standard length (SL) of females before dissection with a digital caliper (±0.1 mm), from the tip of the mouth to the base of the caudal fin at the level of the lateral line. We then dissected females to quantify brood size (number of embryos in the same developmental stage) and superfetation (number of simultaneous broods). We used the classification of embryonic stages proposed by Haynes (1995) to identify the developmental stage of each brood. Individual embryo mass was quantified by drying an entire brood for 48 h at 55°C, weighing it with a precision scale (±0.05 mg), and dividing brood dry mass by the number of embryos in the brood. Upon dissection, the entire digestive tract (stomach and intestines) was removed from each female. We quantified the somatic dry mass of each female (excluding digestive and reproductive tracts) as well as the dry mass of the digestive tract (including all its content) following the same drying and weighing protocol that we implemented for embryos.
Matrotrophy Index
The MI is a standard measure of post-fertilization maternal provisioning, and is calculated as the dry mass of the offspring at birth divided by the dry mass of the egg at fertilization (Reznick et al., 2002; Marsh-Matthews, 2011). MI values <0.75 indicate that females provide all nutrients before fertilization in the form of yolk. MI values between 0.75 and 1 indicate that females provide most nutrients before fertilization and small amounts of nutrients during embryo development (i.e., incipient matrotrophy). MI values >1 indicate that a substantial amount of nutrients are provided after fertilization. MI values >5 indicate extensive matrotrophy (Marsh-Matthews, 2011).
We calculated the MI in two different ways. First, we calculated an MI separately for each population by using data from all females to estimate the dry mass at birth and the dry mass of the egg at fertilization (Reznick et al., 2002; Marsh-Matthews, 2011; Supplementary Table 2). For this purpose, we fitted a linear regression to our data on individual embryo mass (response variable, log-transformed) and developmental stage (predictor variable), separately for each population. From this regression, we used the predicted dry mass at stage 4 [recently fertilized eggs according to Haynes (1995)] as well as the predicted dry mass at stage 11 (last stage of development and, thus, an estimate of mass at birth) to calculate the MI. In this regression, each female was represented by a single data point (i.e., the average dry mass of individual embryos in a particular developmental stage). Because all species in this study exhibit superfetation, we randomly chose one brood from each female to quantify individual embryo mass (as per Frías-Alvarez et al., 2014; Gorini-Pacheco et al., 2018). In this way, we ensured that each female was represented only once in our linear regressions to avoid the problem of non-independence in the data.
Second, we calculated an matrotrophy index per individual female (MIind) by selecting females that had simultaneous broods in developmental stages 4 (recently fertilized) and 11 (close to be born). We calculated an MIind for each of these females by dividing the average dry mass of her embryos at stage 11 by the average dry mass of her embryos at stage 4. In total, 51 females across all species had broods in stages 4 and 11 simultaneously. Our MIind values represent how embryo mass changes throughout development within individual females and, hence, are individual estimates of the amount of post-fertilization provisioning. In contrast, our MI values per population are an average representation of the degree of matrotrophy across all the females that inhabit a population.
Index of Fullness and Body Condition as Proxies for Food Availability
To estimate food availability, we used the index of stomach fullness (IF), which was calculated for each female as the dry mass of her digestive tract (including all its content) divided by her somatic dry mass, and multiplying the result by 100 (Hyslop, 1980). The IF is an indirect measure of the amount of available food and, more specifically, it is a measure of how much females ate at a certain time. It gives us a proxy for food availability at the time when we collected the females, and maybe for a few days before sampling. High IF values indicate that females were in an environment with high food availability, whereas low IF values indicate that females were in an environment with low food availability. Once we obtained the IF for each female, we calculated an average IF (IFA) for each population (Supplementary Table 2). Given our hypothesis, we expected that in environments where food is abundant (i.e., populations with high IFA), females will have higher degrees of matrotrophy, whereas in environments where food is less abundant (i.e., populations with low IFA), we expected to find less matrotrophic females.
In addition, to quantify the magnitude of fluctuations in food availability, we calculated for each population the variance of the IF among months (VarIFmonths; Supplementary Table 2). Given that the amount of available food can vary over time, in those cases in which we had several collection dates (i.e., conducted in different months) from the same population (Supplementary Table 3), we calculated an IFA per month and then the variance among these monthly IFA values. Populations with large values of VarIFmonths are those with pronounced temporal fluctuations (among months or between seasons) in food availability. According to the Trexler-DeAngelis model (Trexler and DeAngelis, 2003, 2010), we expected a negative relationship between MI and VarIFmonths. That is, matrotrophy should be favored in populations where food availability is relatively constant, whereas lecithotrophy should be favored in environments with substantial variability in the access to food resources. Our data from populations with several collection dates revealed that, in some of these populations, both the MI and the index of stomach fullness varied substantially among months (Supplementary Figures 1, 2).
In most of the populations that were sampled in different occasions, the collection dates occurred within a period of 2 years (Supplementary Table 3). Given that the lifespan of poeciliid fishes varies between 2 and 4 years (Coad, 2017; Young et al., 2017), our multiple samples provide a reliable representation of the temporal fluctuations in food availability that a single generation of females experienced. Therefore, this is the appropriate temporal scheme to test the prediction that females facing changing conditions, in terms of food supply, over the course of their lifespan would increase their pre-fertilization investment (a less matrotrophic strategy).
We also calculated BC as another proxy for food availability. We used the scaled mass index as an estimate of individual BC (Peig and Green, 2009). This index is a more reliable indicator of the relative size of energy reserves compared to other indices of BC (Peig and Green, 2010). We calculated the scaled mass index, which we denote here as BC because it is our measure of BC, for each female as follows:
where Mi and SLi are the somatic dry mass and SL of female i, respectively, SL0 is the average SL of the corresponding species, b is the slope from a linear regression of female dry mass on SL (both variables log-transformed), and r is the correlation coefficient between log-transformed female dry mass and SL. Given that female mass and SL differed substantially among species, we calculated the scaled mass index separately for each species, but pooling data from different populations. This index (BCi) is an estimate of the dry mass that each female i would have at a fixed body length, which in our case is the average SL of each species (SL0). Thus, smaller values of BC indicate poorer nutritional status and less energetic reserves, whereas larger values of BC indicate better nutritional status and greater energetic reserves (Peig and Green, 2009, 2010).
We then calculated an average of this scaled mass index separately for each population [average body condition (BCA)] (Supplementary Table 2). BC is an estimate of food availability on a long time scale (weeks before capturing the females) because it reflects if females were able to gain somatic mass as a result of abundant food intake during the past weeks or, alternatively, if they lost mass as a consequence of food scarcity. According to our hypothesis, females will have good BC in environments with plenty of available food and, therefore, high degrees of matrotrophy. In contrast, poor BC indicates that females have experienced reduced food availability and, thus, they should exhibit lower degrees of matrotrophy.
Estimates of BC may be affected by morphological differences among females. For instance, heavier females may also have deep bodies and extended abdomens whereas leaner females may have thinner and elongated bodies. Given that body shape may be affected by ecological factors other than food availability, such as predation risk and water velocity (Zúñiga-Vega et al., 2007; Langerhans, 2009; Langerhans and Makowicz, 2009), an association between morphology and BC could imply that the observed differences among populations in average BC may have been driven by these other factors. This in turn would imply that our estimates of BC do not reflect food availability but indicate instead interpopulation differences in predation intensity or water velocity. We discarded a confounding effect of body shape on our estimates of BC by means of a morphological analysis based on geometric morphometric techniques (Supplementary Material: Methods). This analysis was conducted on four of our five study species because no digital photographs were available for one species (P. turrubarensis). The correlations between the scaled mass index and the first two axes of shape variation were rather weak in all four species (all correlation coefficients ranged between −0.20 and 0.31; Supplementary Table 4). Thus, in these Poeciliopsis species, the observed intraspecific variation in BC cannot be explained by morphological differences among females.
Similar to what we did for the IF, for each population we quantified temporal fluctuations in food availability by means of the variance of body condition among months (VarBCmonths; Supplementary Table 2). This temporal variance was calculated only for those populations that were sampled in different months (Supplementary Table 3). First, we calculated an average BC (BCA) per month and then the variance among these monthly BCA values. We expected a negative relationship between MI and VarBCmonths. Consistent with the observed temporal variation in both the MI and the IF, BC also varied substantially among months within some of the populations that were sampled in different occasions (Supplementary Figure 3).
Quantifications of stomach contents and BC indices have been used previously as indicators of food availability in the surrounding environment. In particular, studies on other fish species have demonstrated that both the index of stomach fullness and individual BC correlate positively with the abundance of important food sources, such as nutrient-rich prey (Simpkins and Hubert, 2000; Pothoven et al., 2001; Rikardsen et al., 2006). These previous observations give support to the use of IF and BC as reliable proxies for food availability.
Statistical Analyses
We implemented different sets of linear models using the R statistical software v. 3.4.2 (R Core Team, 2019). We used the Akaike information criterion adjusted for small sample sizes (AICc; Akaike, 1973) to select the best model (i.e., the model with smallest AICc). However, models that differ by less than seven units of the AICc with respect to the best model (ΔAICc < 7) also have some support in the data (Burnham and Anderson, 2002; Burnham et al., 2011). To deal with model uncertainty, we calculated Akaike weights (wi), which are measures of the relative support for each model i in the data (Burnham and Anderson, 2002). Then, we compared the support of two models relative to each other using evidence ratios, which are calculated as the quotient of their respective Akaike weights (w1/w2). These evidence ratios indicate how much greater the evidence is in favor of model 1 compared to that of model 2. We considered that strong evidence in support of the Trexler-DeAngelis model would come from two complementary lines of evidence. First, the best-fitting model must include one of our predictors of interest (IF, IFA, VarIFmonths, BC, BCA, or VarBCmonths). Second, this best-fitting model must have at least four times stronger support than models that do not include this predictor (i.e., an evidence ratio ≥4). In those cases where two or more supported models (models within ΔAICc < 7) had smaller evidence ratios (because such supported models had relatively large and similar Akaike weights), we chose the model that contained fewer parameters (the simplest model), since adding the additional parameters did not substantially improve model fit.
In addition, based on the Akaike weights, we calculated model-averaged regression coefficients, as instructed by Burnham and Anderson (2002), for those predictor variables with evident statistical effects. These model-averaged slopes account for model uncertainty and are more robust than those derived from any single model (Johnson and Omland, 2004). We used the R package “MuMIn” to implement all these procedures for model selection and multi-model inference (Bartoń, 2019).
For the first model set we used the MI per population (log-transformed) as response variable and IFA as the main explanatory variable. We also considered the factor “species” to take into account differences among species in the degree of matrotrophy. We included additive and interactive effects of IFA and species, as well as an intercept-only model, for a total of five competing models (Table 2). In this first model set, we had 45 data points, one for each of our study populations.
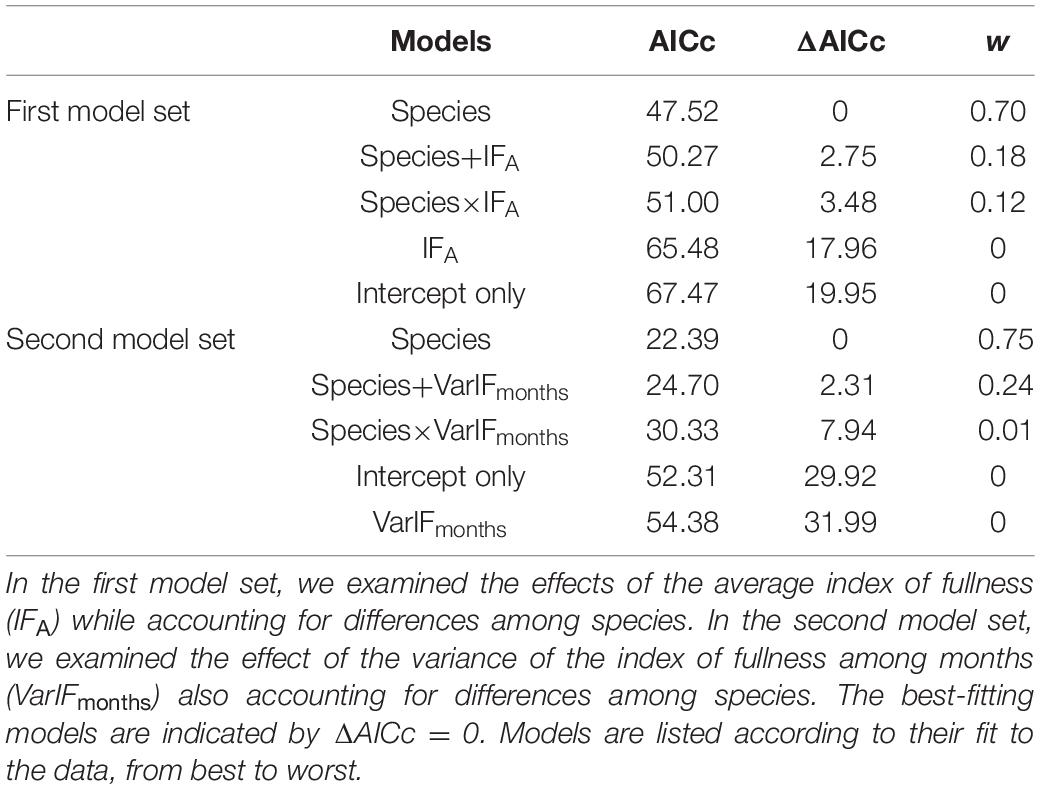
Table 2. Values of the Akaike information criterion adjusted for small sample sizes (AICc), differences in AICc with respect to the best-fitting model (ΔAICc), and Akaike weights (w) for competing models that examined variation in the MI per population.
In our second model set we also used MI per population (log-transformed) as response variable, but in this case, we analyzed the effects of temporal fluctuations in the IF by using VarIFmonths as explanatory variable. We also considered differences among species, additive and interactive effects of VarIFmonths and species, and an intercept-only model, for a total of five competing models (Table 2). Given that not all our study populations were visited in different months (Supplementary Table 3), we had a reduced number of data points (28) for this model set. In fact, all populations of P. baenschi and P. turrubarensis lacked data from different months and, thus, these two species were excluded from this model set.
The third model set was implemented using the MIind female (log-transformed) as response variable. The main explanatory variable was the IF calculated for each female. In addition, we considered differences among species, additive and interactive effects of IF and species, and an intercept-only model, for a total of five competing models (Table 3). In this case, we expected a positive relationship between MIind and the IF. Unlike the previous two model sets, which were conducted at the population level, this third model set was conducted at the individual level with 51 data points, one for each female with an estimate of MIind.
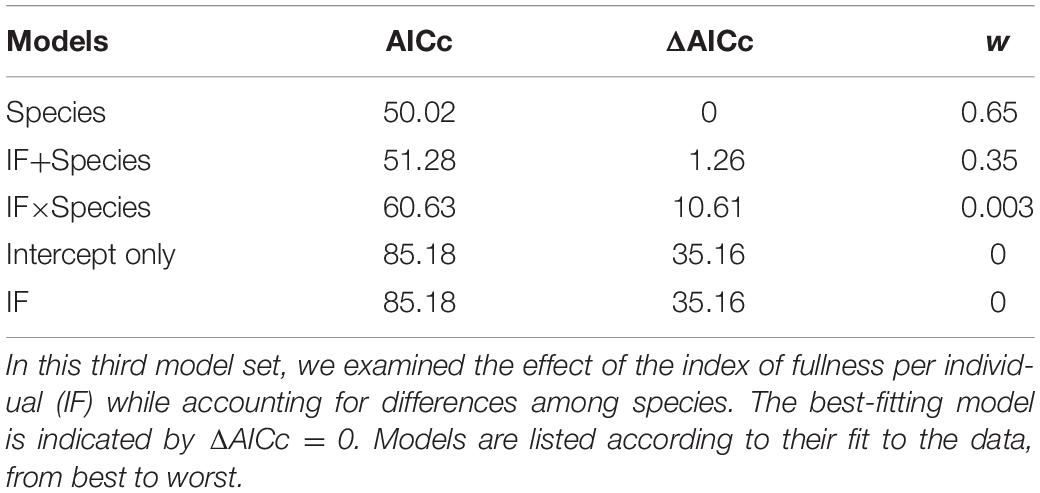
Table 3. Values of the Akaike information criterion adjusted for small sample sizes (AICc), differences in AICc with respect to the best-fitting model (ΔAICc), and Akaike weights (w) for competing models that examined variation in the matrotrophy index per individual female (MIind).
Additionally, we implemented all three model sets, but using BC as proxy for food availability, instead of the IF. This means that the response variable was the same (MI per population in the first and second model sets, and MIind in the third model set), but we used BCA as the main predictor of interest in the first model set (Table 4), VarBCmonths in the second (Table 4), and BC per individual female in the third (Table 5). We also implemented all other models that we described above (i.e., differences among species, additive and interactive effects, and an intercept-only model).
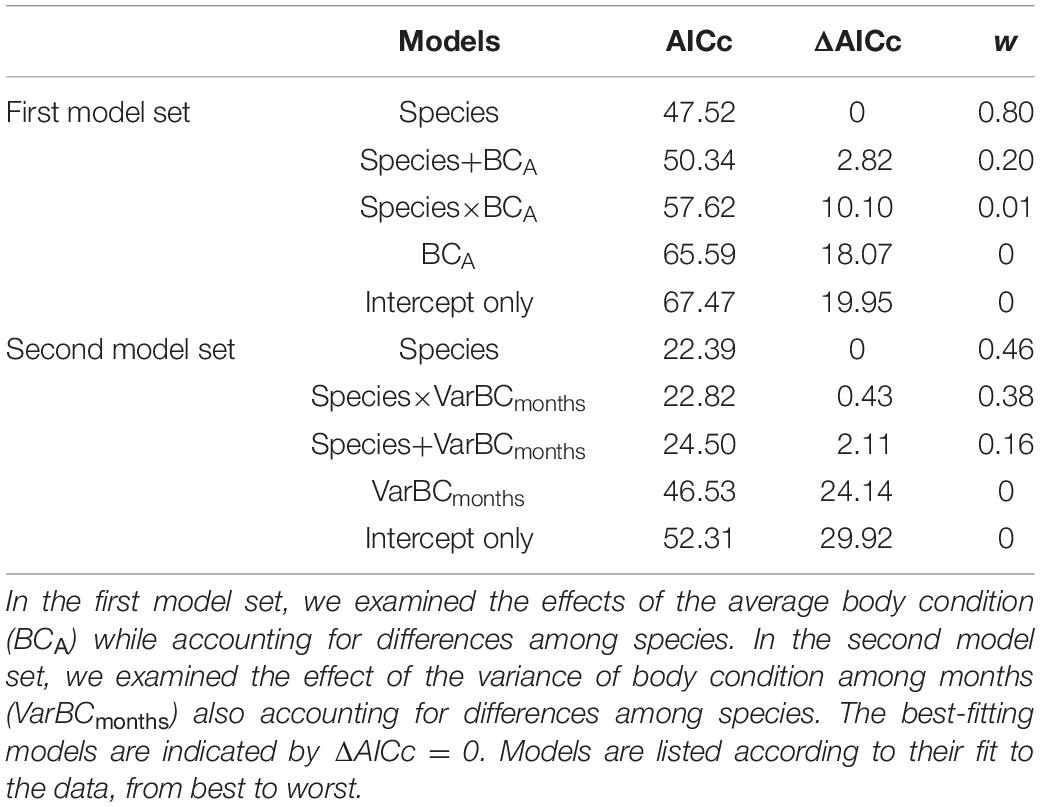
Table 4. Values of the Akaike information criterion adjusted for small sample sizes (AICc), differences in AICc with respect to the best-fitting model (ΔAICc), and Akaike weights (w) for competing models that examined variation in the MI per population.
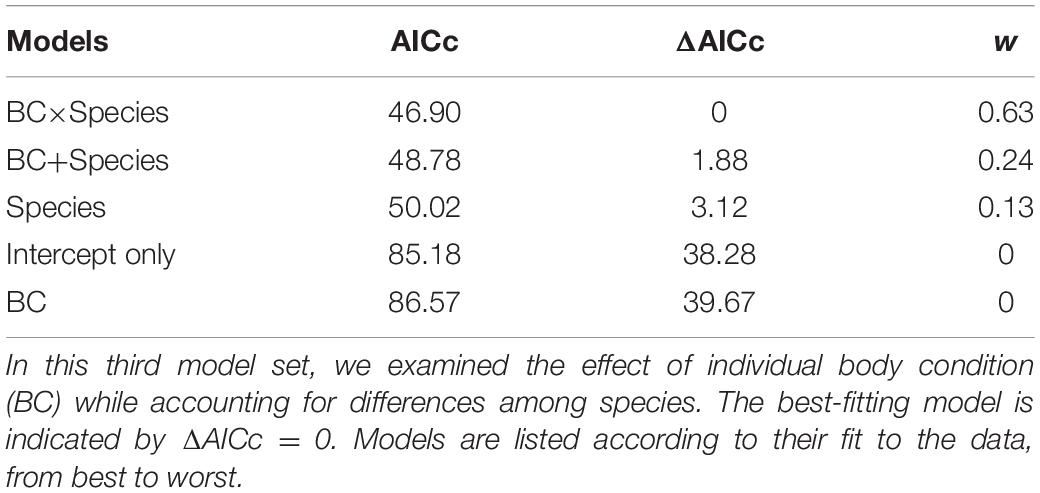
Table 5. Values of the Akaike information criterion adjusted for small sample sizes (AICc), differences in AICc with respect to the best-fitting model (ΔAICc), and Akaike weights (w) for competing models that examined variation in the MIind female.
Results
Matrotrophy and the Index of Fullness
In the first model set, using IF as proxy for food availability, we found no evident effect of IFA on the MI per population (Figure 1A). The top model indicated that MI per population only differed among species (Table 2). Two other models were within ΔAICc < 7 and both included the effect of IFA in addition to differences among species (additive effect in the second model and interactive effect in the third model). However, these additional effects of IFA did not improve model fit compared to the simpler model that only included differences among species (Table 2). Similarly, in the second model set, we found no evidence of an effect of VarIFmonths on the MI per population (Figure 1B). Also in this case, the top model indicated differences among species and adding the effect of VarIFmonths did not substantially improve model fit (Table 2). The highest degrees of matrotrophy occurred in four populations of P. prolifica, and the lowest (indicating a lecithotrophic mode of maternal provisioning) in one population of P. gracilis (Figure 1).
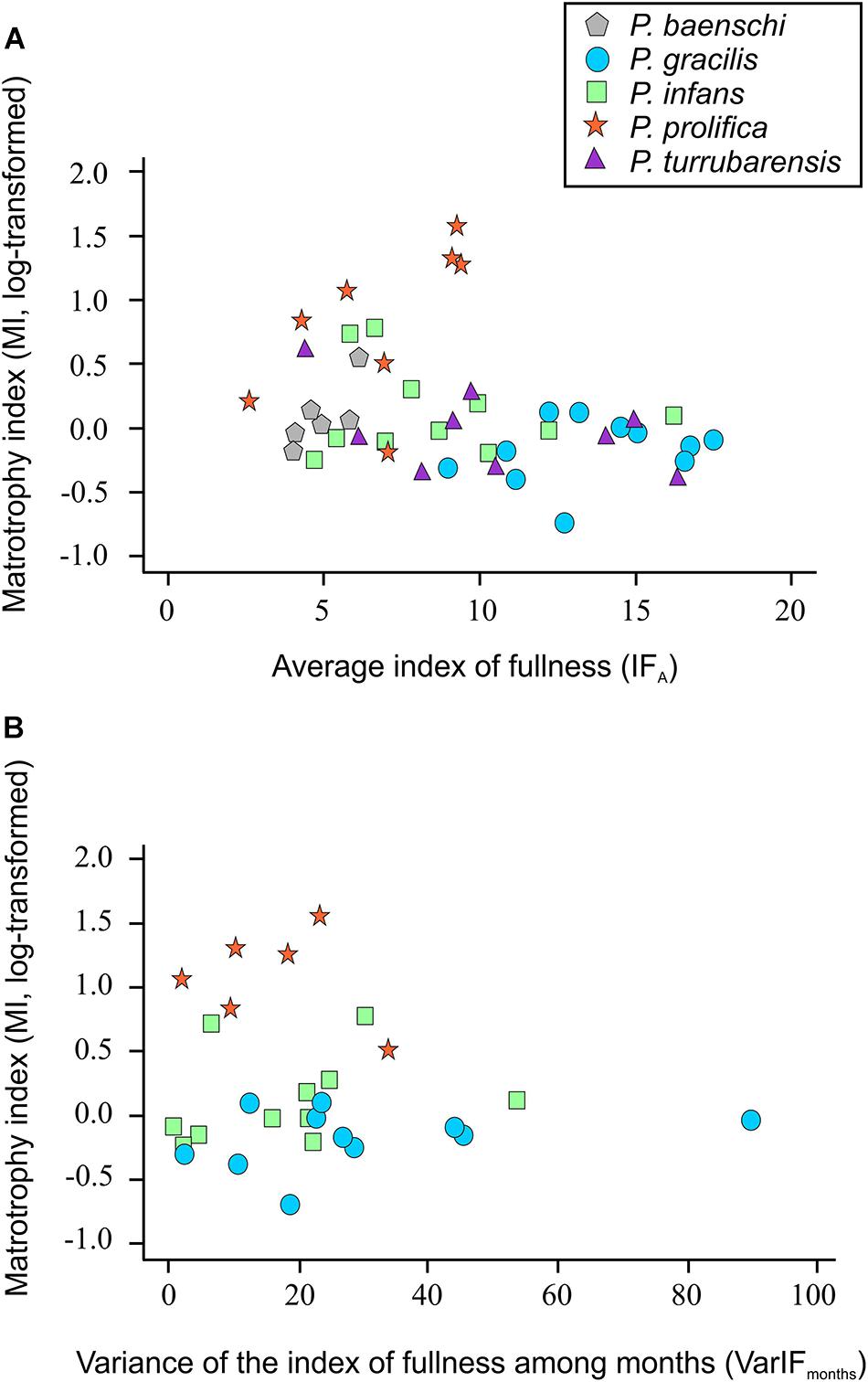
Figure 1. (A) Lack of association between the matrotrophy index per population (MI) and average index of fullness (IFA) for 45 populations of five fish species from the genus Poeciliopsis. (B) Lack of association between the MI per population and the variance of the index of fullness among months (VarIFmonths) for 28 populations of three fish species from the genus Poeciliopsis.
We obtained a similar result when we analyzed the potential effect of the individual IF on MIind in our third model set. The model with the best fit only included differences among species in the MIind (Table 3). The second model was within seven AICc units from the best-fitting model and included an additive effect of IF and species. Hence, evidence of an effect of IF on MIind was weak because adding IF did not substantially improve model fit with respect to the simpler model that only included differences among species (Table 3). Most females of P. prolifica had higher degrees of matrotrophy compared to females from all other species, whereas three females of P. gracilis had the lowest values of the MIind (Figure 2).
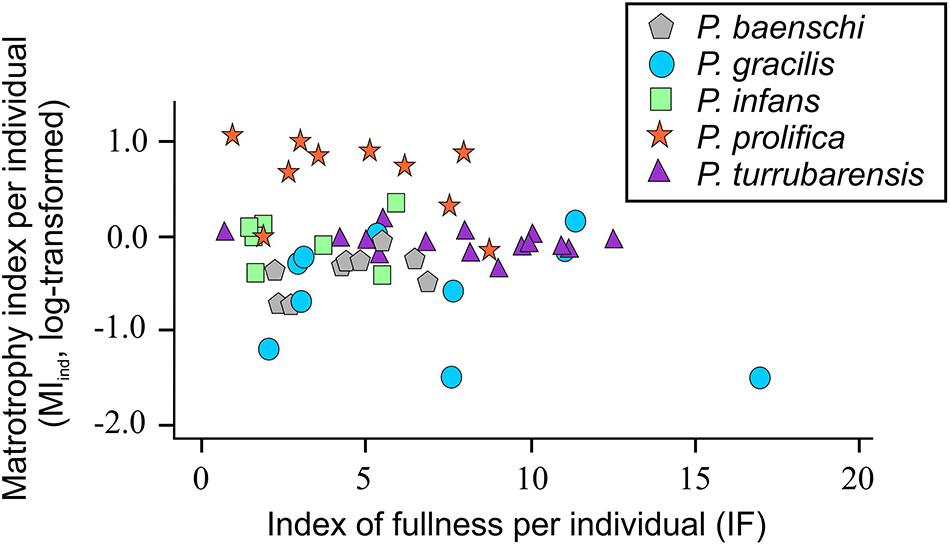
Figure 2. Lack of association between the matrotrophy index per individual (MIind) and the individual index of fullness (IF) for 51 females of five fish species from the genus Poeciliopsis.
Matrotrophy and Body Condition
In our first model set, using BC as proxy for food availability, BCA did not affect the MI per population (Figure 3). Again in this case, the best-fitting model indicated differences among species in the MI (Table 4). The model that ranked second was within ΔAICc < 7 and included the additive effect of species and BCA. However, adding BCA did not improve model fit compared to the model that only included differences among species. Similarly, in our second model set, the top model indicated that MI only differs among species (Table 4). Two other models had support (i.e., ΔAICc < 7) and both included the effect of VarBCmonths (interacting with species in the second model and as an additive effect in the third model). Hence, adding VarBCmonths did not substantially improve model fit, indicating a weak effect of this predictor on the MI per population (Figure 4). In P. prolifica, MI apparently decreased as VarBCmonths increased (Figure 4C). However, this negative association was caused by a single population with large temporal variance and quite small MI. For this reason, the model including the interaction between VarBCmonths and species did not outperform the simpler model that only included differences among species (Table 4).
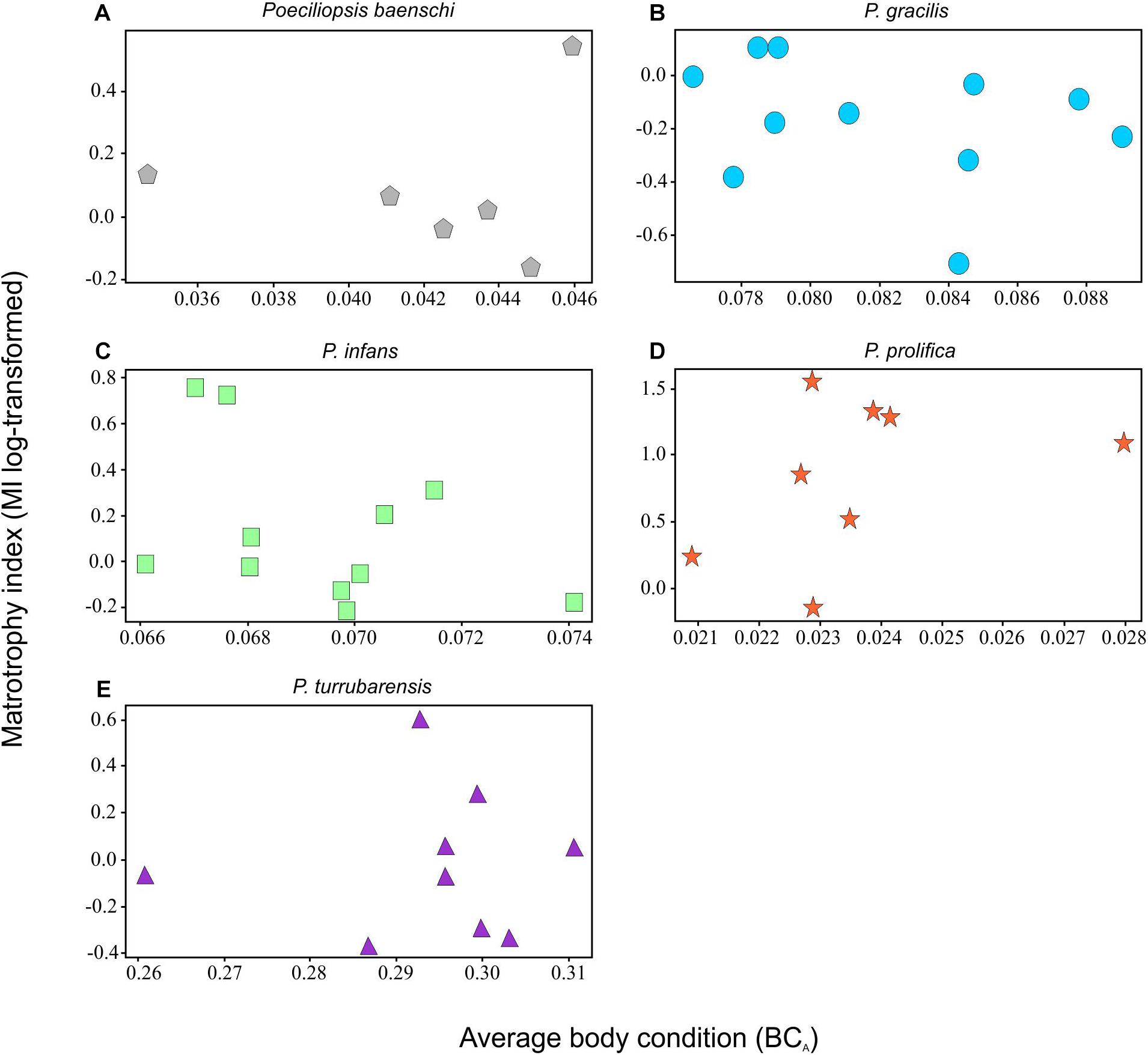
Figure 3. Lack of association between the MI per population and average body condition (BCA) for 45 populations of five fish species from the genus Poeciliopsis. Each species is shown in a different panel because of substantial differences among species in the values of body condition (BC). Each symbol represents a different species as per (Figures 1, 2).
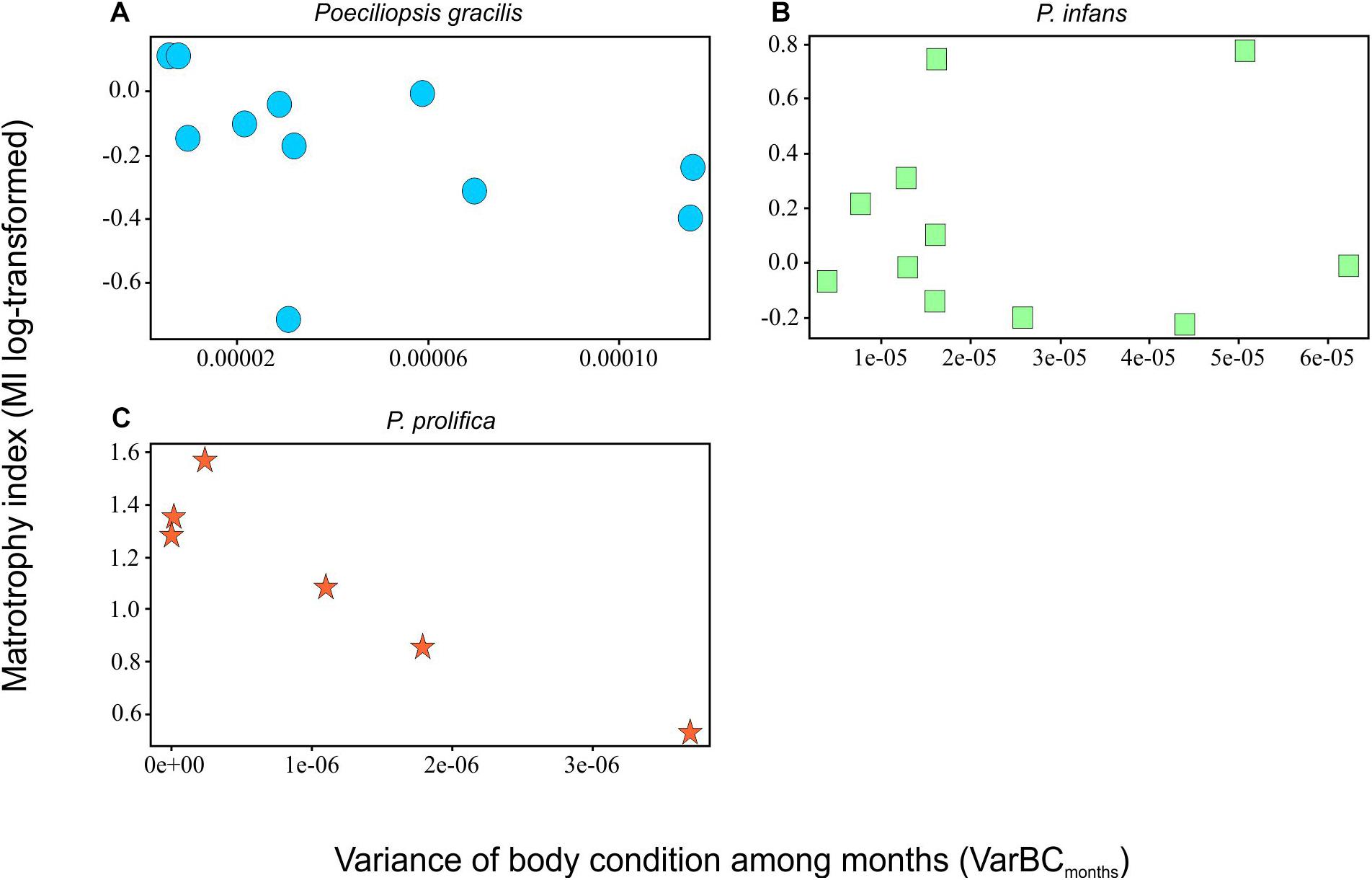
Figure 4. Lack of association between the MI per population and the variance of body condition among months (VarBCmonths) for 28 populations of three fish species from the genus Poeciliopsis. Each species is shown in a different panel because of substantial differences among species in the values of BC. Each symbol represents a different species as per (Figures 1, 2).
Finally, in the third model set in which we used BC as proxy for food availability, the model that included an interactive effect of individual BC and species on MIind ranked first (Table 5). The model that included an additive effect of these two predictors ranked second. The top model had 2.6 times stronger support than the second model (evidence ratio: 0.63/0.24 = 2.63). The third model only included differences among species with no effect of BC. However, the top model had almost five times stronger support than this third model (evidence ratio: 0.63/0.13 = 4.85) (Table 5), which in this case represents compelling evidence of a combined effect of both BC and species on MIind. Contrary to our prediction, model-averaged regression coefficients revealed a negative relationship between MIind and individual BC for females of all five species (Figure 5). This negative relationship was weaker (i.e., a less steep negative slope) for females of P. prolifica (Figure 5D).
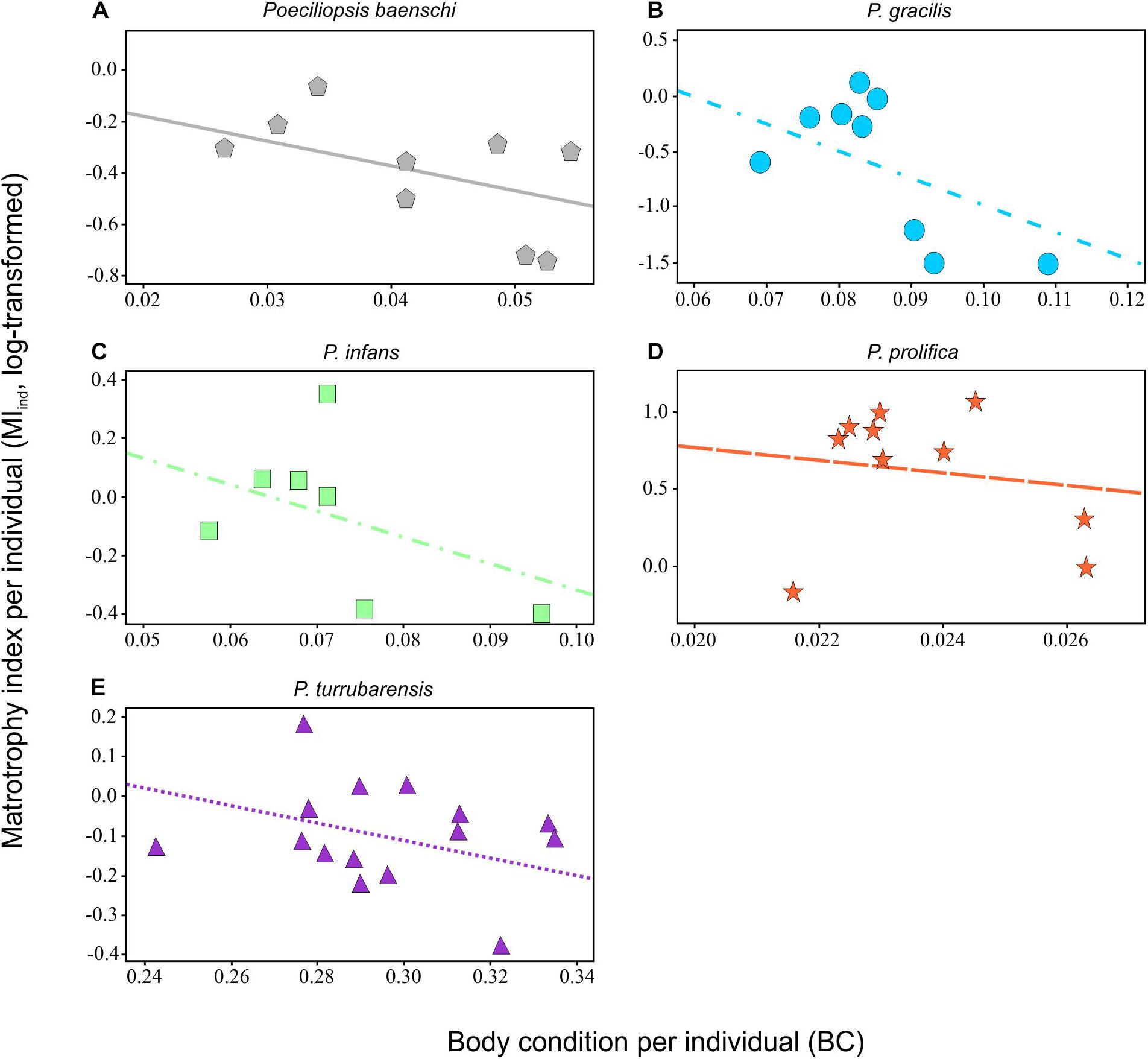
Figure 5. Negative association between the MIind and individual BC for 51 females of five fish species from the genus Poeciliopsis. Each species is shown in a different panel because of substantial differences among species in the values of BC. Fitted lines represent model-averaged regression coefficients. Each symbol represents a different species as per (Figures 1, 2).
Discussion
Matrotrophy Negatively Affects Body Condition and Is Apparently Not Influenced by Food Availability
In this study, we examined the Trexler-DeAngelis model of maternal provisioning to developing embryos (Trexler and DeAngelis, 2003, 2010). According to this model, higher levels of matrotrophy are only possible if food is relatively abundant and constantly available. To test this prediction, we used the index of stomach fullness and BC as proxies for the amount of available food in the environment. Based on these two variables, we also quantified the degree of temporal variability in food availability. We found that females with higher levels of matrotrophy had poorer BC compared to females that provided less nutrients to embryos after fertilization. This negative association was evident in females of all five species and is opposite to our prediction of greater matrotrophy in females that exhibit better BC, presumably as a result of constant access to abundant food resources. In contrast, our main finding implies that providing relatively large amounts of nutrients to embryos after fertilization has a negative consequence on the nutritional status of the females. Possibly, females experience a trade-off between active provisioning of embryos during gestation and allocation of energy and nutrients to somatic tissues, suggesting that matrotrophy is a costly physiological process. Severe demands for energy and nutrients are well-known consequences of large investments in reproduction (Koskela et al., 1998; Jonsson et al., 2002; Velando and Alonso-Alvarez, 2003).
According to the Trexler-DeAngelis model, one of the main benefits of matrotrophy is a potentially higher fecundity, because lecithotrophy implies that eggs must be full of yolk before fertilization and thus represent a large energetic investment since the onset of gestation. In contrast, matrotrophic eggs are considerably smaller and less energetically costly, which could allow females to initiate gestation with a higher number of these smaller eggs. If resources are abundant enough to provide embryos with the necessary nutrients all throughout gestation, then matrotrophy would be favored over lecithotrophy (Trexler and DeAngelis, 2003, 2010). Here, we have shown that neither the amount of food that is present in the digestive tract of females nor their nutritional status reflected in their BC are positively associated with the degree of matrotrophy, which in turn suggests that high food availability is not the main driver of advanced degrees of matrotrophy. In contrast, we detected in all five species that females that provided more nutrients to embryos after fertilization were in poorer BC compared to females that relied more on pre-fertilization provisioning. This observed pattern represents compelling evidence that, if advanced matrotrophy allows females to gestate more embryos, such increased fecundity comes at the cost of a reduced investment in self-maintenance.
Our work with 45 populations of five species of poeciliid fishes did not support the expectation of increased matrotrophy in sites with abundant food. Furthermore, sites where food availability fluctuates over time (i.e., sites with large temporal variances in the index of stomach fullness and in BC) did not promote reduced levels of matrotrophy. According to the Trexler-DeAngelis model, if food-limiting conditions are likely to arise, then females must rely more on pre-fertilization provisioning because they can store as much surplus energy as possible in the form of yolk whenever food becomes available (during periods when food intake exceeds metabolic demands) (Trexler and DeAngelis, 2003, 2010). Our data did not support this prediction either. Interestingly, however, the original model specifies that the total cost per brood may be greater for matrotrophic females compared to lecithotrophic females, because the former initially invest less per egg (because eggs are smaller) which allows them to initiate gestation with larger broods. If the size of offspring at birth is the same between matrotrophic and lecithotrophic females, then producing a larger number of similar-sized young will result in an overall higher reproductive cost for females with advanced matrotrophy. As a consequence, Trexler and DeAngelis (2003, 2010) predicted leaner bodies and shorter lifespans in females that allocate more resources to developing embryos after fertilization. Our findings revealed poor BC in females with advanced matrotrophy and are thus consistent with this particular prediction of the model that is related to the consequences of matrotrophy. In conclusion, we failed to provide evidence in support of the expected ecological causes of this reproductive mode (high and constant food availability), but we have demonstrated a critical consequence of advanced matrotrophy that appears to be pervasive because it occurred in five different species.
The Costs of Matrotrophy Decrease as Yolk Dependency Decreases
Our findings, along with previous evidence, suggest that the severity of the physiological costs imposed by greater post-fertilization provisioning depends on the degree to which females rely on yolk reserves for embryo nutrition. A previous study in the congeneric Poeciliopsis retropinna found that the amount of post-fertilization provisioning was positively associated with maternal body fat and unrelated to maternal lean mass (Hagmayer et al., 2018). This finding is somehow contrary to what we observed in this study, because our estimates of BC included both lipids and lean tissue (i.e., we did not extract lipids from the females). Thus, our observed reduction in BC associated with higher degrees of matrotrophy likely entailed reductions in both body fat and lean mass. The reasons why in P. retropinna a higher investment in embryo development after fertilization does not entail a reduction in maternal body fat or in lean mass, whereas in the five congeneric species that we studied advanced matrotrophy clearly resulted in poorer BC, remain unknown. A tentative explanation may involve the remarkably high degree of matrotrophy of P. retropinna, in which embryos increase in mass more than 100-fold during development (MI = 117; Reznick et al., 2002). Such an extensive degree of matrotrophy could substantially reduce the costs derived from reproduction because mature ova are notably small with no nutrients before fertilization and, hence, overall investment in reproduction is low during a significant proportion of pregnancy. All our study species exhibit considerably lower degrees of matrotrophy, from an MI = 0.72 in P. gracilis (which in fact indicates strict lecithotrophy) to an MI = 5.40 in P. prolifica (Table 1). Therefore, energetic demands derived from a higher investment in embryos since the onset of gestation (in our study species mature ova already contain some nutrient-rich yolk before fertilization) are likely higher in our five study species than in P. retropinna.
The weaker negative relationship between degree of matrotrophy and BC that we observed in P. prolifica confirms a lower physiological cost of actively providing embryos with nutrients during gestation for species that depend less on yolk reserves. Unlike P. prolifica, which exhibits a substantial amount of post-fertilization provisioning (MI = 5.40) and, hence, smaller amounts of yolk in mature ova, the other four species that we studied have a greater dependency on the yolk that is deposited into the eggs before fertilization for embryo nourishment (their MI values range between 0.72 and 1.58; Table 1; Reznick et al., 2002; Olivera-Tlahuel et al., 2015). Females of these four species lack specialized placentas that facilitate the transfer of nutrients from the mother to developing embryos and, instead, they have relatively simple maternal follicles that have the primary functions of gas exchange and osmoregulation (Kwan et al., 2015; Olivera-Tlahuel et al., 2019). Certainly, the observed variation in the degree of matrotrophy among individual females (Figure 2) as well as among populations (Figure 1) of these four species indicates that females are able to increase the amount of nutrients that are actively transferred to developing embryos after fertilization. Apparently, doing so without the specialized anatomical structures that are present in placental species such as Poeciliopsis prolifica and Poeciliopsis turneri (i.e., abundant microvilli and capillaries, thicker maternal follicles, numerous enlarged vesicles; Kwan et al., 2015; Olivera-Tlahuel et al., 2019) entails a greater energetic expenditure for gestating females of non-placental and yolk-dependent species that causes a detrimental effect on their somatic tissues and lipid reserves.
Caveats and Limitations
Even though previous studies have demonstrated that the index of stomach fullness and estimates of BC are accurate indicators of the amount and quality of food that is available in the surrounding environment (Simpkins and Hubert, 2000; Pothoven et al., 2001; Rikardsen et al., 2006), we recognize the potential limitations of using these proxies for food availability. These limitations may explain, at least partially, the lack of association between food availability and degree of matrotrophy that we observed in all five species. First, stomachs of females could be quite full, but most of their content may be of low nutritional value. For instance, females may have consumed abundant sediment or shells of mollusks that provide little to no energy (Langerhans et al., 2021). If this were the case, stomach fullness may not be correlated with the actual availability of nutritious food items.
Second, food availability, as well as stomach fullness and BC, may vary substantially over relatively short timescales (e.g., between weeks) and, hence, our collections of females may have occurred during an uncommon episode of low or high food abundance that does not reflect the general pattern of food availability in our study sites. Therefore, the lack of association between food availability and matrotrophy that we observed in all our focal species may have been caused by a mismatch between food availability at the time when our collections took place (e.g., low food availability during the past couple of days) and the observed degree of matrotrophy that was likely driven by the general long-term pattern of food availability of each population (e.g., high food availability during most of the past months).
Third, both stomach fullness and BC may be affected by factors other than food availability, such as temperature (which has a direct influence on digestive efficiency in ectotherms; Harlow et al., 1976), prevalence of pathogens (sick females may have poor BC even if food is abundant in the surrounding environment; Ageze and Menzir, 2018), and rates of activity (e.g., fish may maintain low foraging rates in the presence of abundant predators; Botham et al., 2006). Hence, females from populations where food is abundant may have less full stomachs or poor BC if these ecological conditions are suboptimal (low temperatures or abundant pathogens and predators). In these cases, neither stomach fullness nor BC would be reliable indicators of food availability. Therefore, the lack of associations between degree of matrotrophy and our proxies for food availability must be interpreted with caution, since future examination of additional measures of food availability, such as in situ abundances of algae and aquatic invertebrates (Quintans et al., 2009; Carbajal-Becerra et al., 2020), may provide supporting evidence for this prediction of the Trexler-DeAngelis model.
Finally, we must notice that our study is based on two important assumptions. (1) Populations differ in the average amount of food availability, which means that over the course of a relatively long period, a year for example, food availability is overall higher in a particular population whereas it is constantly lower in another. This assumption implies that our two proxies for food availability should reflect this same situation, namely, in the former population females must have fuller stomachs and better BC, whereas in the latter, females must have less full stomachs and poorer BC, regardless of the particular time of collection. If this assumption is true, then females experience the same regime of food availability during most of their reproductive lives (i.e., during most of their pregnancies), which in turn must promote the evolution of either reduced or advanced matrotrophy. (2) Fluctuations in food availability occur on relatively long timescales, such as among months or seasons. This means that a female inhabiting a population where food supply fluctuates over time, experiences some months of food restriction and other months of abundant food. Given that gestation lasts approximately 30 days in poeciliid fishes (Veggetti et al., 1993; Bisazza and Marin, 1995), some broods are produced under limiting conditions and others under abundant food, which in the long term must promote the evolution of a predominantly lecithotrophic strategy. If these two assumptions are incorrect and food availability fluctuates drastically on shorter timescales (among weeks or days), then our statistical approach to estimate the degree of post-fertilization provisioning (a linear regression that predicts how embryo mass changes from fertilization to birth) may be invalid because the mass of embryos at different developmental stages, which depends to a large extent on the amount of food that females consume, would also change drastically within months or even weeks (during a single pregnancy). This, in turn, would promote short-term temporal changes in the overall degree of matrotrophy (i.e., in the MI). Thus, in summary, the temporal scale at which food availability fluctuates may be a critical aspect that could determine whether evidence in support of the Trexler-DeAngelis model is found or not.
Future Directions
The studies that have tested the Trexler-DeAngelis model of maternal provisioning have yielded mixed results, which may be attributed to different methodologies. Some studies were based on laboratory experiments (Marsh-Matthews and Deaton, 2006; Pires et al., 2007; Banet and Reznick, 2008; Banet et al., 2010; Pollux and Reznick, 2011; Itonaga et al., 2012; Van Dyke et al., 2014; Molina-Moctezuma et al., 2020), whereas others were conducted under natural conditions (Schrader and Travis, 2008, 2012; Riesch et al., 2013; Molina-Moctezuma et al., 2020). In controlled experiments, the amount of food and fluctuations in its availability are the only variables at play. Such experimental studies have provided evidence that abundant and constantly available food are positively associated with the amount of post-fertilization maternal provisioning, which supports the Trexler-DeAngelis model (Van Dyke et al., 2014; Molina-Moctezuma et al., 2020). In contrast, when data are obtained under natural conditions, several ecological factors interact and may mask the potential effects of food availability. Some of these studies have not found evidence in support of the Trexler-DeAngelis model (Schrader and Travis, 2008; Riesch et al., 2013). In addition to high and constant food availability, other ecological conditions may select for increased matrotrophy, such as a high predation risk. Given that matrotrophic embryos are substantially smaller during a large proportion of pregnancy, the ovarian mass and volume remain relatively small in gestating females, which provides them with an advantage in terms of swimming performance and escape velocity. Consistent with this hypothesis, in two poeciliid species, P. retropinna and Phalloceros harpagos, females from high-predation environments exhibit greater degrees of matrotrophy compared to females from low-predation environments (Gorini-Pacheco et al., 2018; Hagmayer et al., 2020). The combined effects of predation intensity and food availability on the relative amounts of pre- and post-fertilization provisioning to developing embryos have not been investigated yet and deserve further examination.
The Trexler-DeAngelis model was originally proposed using poeciliid fishes as model systems (Trexler and DeAngelis, 2003, 2010). To date, most empirical tests of this model have been conducted also on species of this group of viviparous fishes (Pires et al., 2007; Schrader and Travis, 2008, 2012; Pollux and Reznick, 2011). The few notable exceptions are three studies conducted on lizards (Cadby et al., 2011; Itonaga et al., 2012; Van Dyke et al., 2014). This means that we are still far from understanding if the causes and consequences of advanced degrees of matrotrophy are the same across diverse phylogenetic groups. The search for generalizations about the influence of food availability on post-fertilization maternal provisioning requires additional studies in other viviparous taxa such as some cartilaginous fishes and numerous invertebrates, which also exhibit wide variation in the relative amounts of pre- and post-fertilization maternal investment (Hamlett et al., 2005; Ostrovsky et al., 2016; Carter and Soma, 2020). These other taxa would be excellent model systems to test the predictions of the Trexler-DeAngelis model.
Data Availability Statement
The original contributions presented in the study are included in the article/Supplementary Material, further inquiries can be directed to the corresponding author/s.
Ethics Statement
Ethical review and approval was not required for the animal study because we used only museum specimens.
Author Contributions
JZ-V conceived the idea for the study and designed the statistical analyses. NS-S dissected the museum specimens, compiled the data, conducted the analyses, prepared the figures, and wrote the first draft of the manuscript. GR-C compiled part of the data and provided the ideas in all stages. All authors have contributed to the preparation, research, writing of the manuscript, reviewed and edited the manuscript, and approved the final version.
Funding
This study was funded by the Consejo Nacional de Ciencia y Tecnología through a fellowship awarded to NS-S (number 596700).
Conflict of Interest
The authors declare that the research was conducted in the absence of any commercial or financial relationships that could be construed as a potential conflict of interest.
Publisher’s Note
All claims expressed in this article are solely those of the authors and do not necessarily represent those of their affiliated organizations, or those of the publisher, the editors and the reviewers. Any product that may be evaluated in this article, or claim that may be made by its manufacturer, is not guaranteed or endorsed by the publisher.
Acknowledgments
Israel Solano-Zavaleta, Pedro Mendoza-Hernández, and Mariana Hernández-Apolinar provided technical assistance. NS-S is a doctoral student from Programa de Doctorado en Ciencias Biomédicas, Universidad Nacional Autónoma de México (UNAM) and received fellowship 596700 from CONACYT. NS-S thanks her brothers for all their moral support, so she does it by saying: thank you “Oso-Republic.”
Supplementary Material
The Supplementary Material for this article can be found online at: https://www.frontiersin.org/articles/10.3389/fevo.2021.690430/full#supplementary-material
References
Ageze, N., and Menzir, A. (2018). Prevalence of Nematode (Contracaecum) and Cestode (Ligula intestinalis) parasites infection in two fish species at Lake Tana. J. Adv. Res. Pub. 2:1.
Akaike, H. (1973). “Information theory and an extension of the maximum likelihood principle,” in Second International Symposium on Information Theory, eds B. N. Petrov and F. Czáki (Budapest: Akademiai Kiadó), 267–281.
Banet, A. I., Au, A. G., and Reznick, D. N. (2010). Is mom in charge? Implications of resource provisioning on the evolution of the placenta. Evolution 64, 3172–3182. doi: 10.1111/j.1558-5646.2010.01059.x
Banet, A. I., and Reznick, D. N. (2008). Do placental species abort offspring? Testing an assumption of the Trexler-DeAngelis model. Funct. Ecol. 22, 323–331. doi: 10.1111/j.1365-2435.2007.01367.x
Bartoń, K. (2019). MuMIn: Multi-Model Inference. R Package Version 1.43.6. Available online at: https://CRAN.R-project.org/package=MuMIn (accessed April 15, 2020).
Bassar, R. D., Auer, S. K., and Reznick, D. N. (2014). Why do placentas evolve? A test of the life-history facilitation hypothesis in two clades in the genus Poeciliopsis representing two independent origins of placentas. Funct. Ecol. 28, 999–1010. doi: 10.1111/1365-2435.12233
Bisazza, A., and Marin, G. (1995). Sexual selection and sexual size dimorphism in the eastern mosquitofish Gambusia holbrooki (Pisces Poeciliidae). Ethol. Ecol. Evol. 7, 169–183. doi: 10.1080/08927014.1995.9522963
Blackburn, D. G. (1999). Viviparity and oviparity - evolution and strategies. Encycl. Reprod. 4, 994–1003.
Blackburn, D. G. (1992). Convergent evolution of viviparity, matrotrophy, and specializations for fetal nutrition in reptiles and other vertebrates. Am. Zool. 32, 313–321.
Botham, M. S., Kerfoot, C. J., Louca, V., and Krause, J. (2006). The effects of different predator species on antipredator behavior in the Trinidadian guppy, Poecilia reticulata. Naturwissenschaften 93, 431–439. doi: 10.1007/s00114-006-0131-0
Burnham, K. P., and Anderson, D. R. (2002). Model Selection and Multimodel Inference: A Practical Information-Theoretic Approach, 2nd Edn. New York, NY: Springer Science & Business Media.
Burnham, K. P., Anderson, D. R., and Huyvaert, K. P. (2011). AIC model selection and multimodel inference in behavioral ecology: some background, observations, and comparisons. Behav. Ecol. Sociobiol. 65, 23–35. doi: 10.1007/s00265-010-1029-6
Cadby, C. D., Jones, S. M., and Wapstra, E. (2011). Potentially adaptive effects of maternal nutrition during gestation on offspring phenotype of a viviparous reptile. J. Exp. Biol. 214, 4234–4239. doi: 10.1242/jeb.057349
Carbajal-Becerra, O., Olvera-Rodríguez, K. J., Mariscal de Souza, G., Durán-Rodríguez, O. Y., Ramírez-García, A., and Ramírez-Herrejón, J. P. (2020). Trophic strategies of the invasive Twospot livebearer (Pseudoxiphophorus bimaculatus, Teleostei: Poeciliidae) in a gradient of environmental quality in central Mexico. Neotrop. Ichthyol. 18:e190080. doi: 10.1590/1982-0224-2019-0080
Carter, A. M., and Soma, H. (2020). Viviparity in the longest-living vertebrate, the Greenland shark (Somniosus microcephalus). Placenta 97, 26–28. doi: 10.1016/j.placenta.2020.05.014
Castro, J. I. (2009). Observations on the reproductive cycles of some viviparous North American sharks. Aqua, Int. J. Ichthyol. 15, 205–222.
Coad, B. W. (2017). Review of the livebearer fishes of Iran (Family Poeciliidae). Iran. J. Ichthyol. 4, 305–330. doi: 10.22034/iji.v4i4.232
Frías-Alvarez, P., Garcia, C. M., Vázquez-Vega, L. F., and Zúñiga-Vega, J. J. (2014). Spatial and temporal variation in superfoetation and related life history traits of two viviparous fishes: Poeciliopsis gracilis and P. infans. Naturwissenschaften 101, 1085–1098. doi: 10.1007/s00114-014-1247-2
Frías-Álvarez, P., and Zúñiga-Vega, J. J. (2016). Superfetation in live-bearing fishes is not always the result of a morphological constraint. Oecologia 181, 645–658. doi: 10.1007/s00442-015-3477-1
Gorini-Pacheco, B., Zandonà, E., and Mazzoni, R. (2018). Predation effects on matrotrophy, superfetation and other life history traits in Phalloceros harpagos. Ecol. Freshw. Fish. 27, 442–452. doi: 10.1111/eff.12359
Greven, H., and Guex, G.-D. (1994). Structural and physiological aspects of viviparity in Salamandra atra. Mertensiella 4, 161–208.
Guillette, L. J., and Jones, R. E. (1985). Ovarian, oviductal, and placental morphology of the reproductively bimodal lizard, Sceloporus aeneus. J. Morphol. 184, 85–98. doi: 10.1002/jmor.1051840109
Hagan, H. R. (1931). The embryogeny of the polyctenid, Hesperoctenes fumarius Westwood, with reference to viviparity in insects. J. Morphol. 51, 1–117. doi: 10.1002/jmor.1050510102
Hagmayer, A., Furness, A. I., Reznick, D. N., Dekker, M. L., and Pollux, B. J. A. (2020). Predation risk shapes the degree of placentation in natural populations of live-bearing fish. Ecol. Lett. 23, 831–840. doi: 10.1111/ele.13487
Hagmayer, A., Furness, A. I., Reznick, D. N., and Pollux, B. J. A. (2018). Maternal size and body condition predict the amount of post-fertilization maternal provisioning in matrotrophic fish. Ecol. Evol. 8, 12386–12396. doi: 10.1002/ece3.4542
Hamlett, W. C., Kormanik, G. A., Storrie, M. T., Stevens, B., and Walker, T. I. (2005). “Chondrichthyan parity, lecithotrophy and matrotrophy,” in Reproductive Biology and Phylogeny of Chondrichthyes: Sharks, Batoids and Chimaeras, ed. W. C. Hamlett (Enfield, NH: Science Publishers Inc), 395–434.
Harlow, H. J., Hillman, S. S., and Hoffman, M. (1976). The effect of temperature on digestive efficiency in the herbivorous lizard, Dipsosaurus dorsalis. J. Comp. Physiol. 111, 1–6.
Haynes, J. L. (1995). Standardized classification of Poeciliid development for life-history studies. Copeia 1995, 147–154.
Hyslop, E. J. (1980). Stomach contents analysis—a review of methods and their application. J. Fish Biol. 17, 411–429. doi: 10.1111/j.1095-8649.1980.tb02775.x
Itonaga, K., Jones, S. M., and Wapstra, E. (2012). Effects of maternal basking and food quantity during gestation provide evidence for the selective advantage of matrotrophy in a viviparous lizard. PLoS One 7:e41835. doi: 10.1371/journal.pone.0041835
Johnson, J. B., and Omland, K. S. (2004). Model selection in ecology and evolution. Trends Ecol. Evol. 19, 101–108. doi: 10.1016/j.tree.2003.10.013
Jonsson, P., Hartikainen, T., Koskela, E. S. A., and Mappes, T. (2002). Determinants of reproductive success in voles: space use in relation to food and litter size manipulation. Evol. Ecol. 16, 455–467. doi: 10.1023/A:1020854525220
Koskela, E., Jonsson, P., Hartikainen, T., and Mappes, T. (1998). Limitation of reproductive success by food availability and litter size in the bank vole, Clethrionomys glareolus. Proc. R. Soc. B Biol. Sci. 265, 1129–1134. doi: 10.1098/rspb.1998.0408
Kwan, L., Fris, M., Rodd, F. H., Rowe, L., Tuhela, L., and Panhuis, T. M. (2015). An examination of the variation in maternal placentae across the genus Poeciliopsis (Poeciliidae). J. Morphol. 276, 707–720. doi: 10.1002/jmor.20381
Langerhans, R. B. (2009). Trade-off between steady and unsteady swimming underlies predator-driven divergence in Gambusia affinis. J. Evol. Biol. 22, 1057–1075. doi: 10.1111/j.1420-9101.2009.01716.x
Langerhans, R. B., and Makowicz, A. M. (2009). Shared and unique features of morphological differentiation between predator regimes in Gambusia caymanensis. J. Evol. Biol. 22, 2231–2242. doi: 10.1111/j.1420-9101.2009.01839.x
Langerhans, R. B., Goins, T. R., Stemp, K. M., Riesch, R., Araújo, M. S., and Layman, C. A. (2021). Consuming costly prey: optimal foraging and the role of compensatory growth. Front. Ecol. Evol. 8:603387. doi: 10.3389/fevo.2020.603387
Lombardi, J., and Wourms, J. P. (1985). The trophotaenial placenta of a viviparous goodeid fish. III: Protein uptake by trophotaeniae, the embryonic component. J. Exp. Zool. 236, 165–179. doi: 10.1002/jez.1402360207
Marsh-Matthews, E. (2011). “Matrotrophy,” in Ecology and Evolution of Poeciliid Fishes, eds J. Evans, A. Pilastro, and I. Schlupp (Chicago, IL: The University of Chicago Press), 18–27.
Marsh-Matthews, E., and Deaton, R. (2006). Resources and offspring provisioning: a test of the Trexler-DeAngelis model for matrotrophy evolution. Ecology 87, 3014–3020. doi: 10.1890/0012-9658200687[3014:RAOPAT]2.0.CO;2
Meier, R., Kotrba, M., and Ferrar, P. (1999). Ovoviviparity and viviparity in the Diptera. Biol. Rev. 74, 199–258. doi: 10.1017/S0006323199005320
Molina-Moctezuma, A., Hernández-Rosas, A. L., and Zúñiga-Vega, J. J. (2020). Resource availability and its effects on mother to embryo nutrient transfer in two viviparous fish species. J. Exp. Zool. Part A Ecol. Integr. Physiol. 333, 181–193. doi: 10.1002/jez.2342
Olivera-Tlahuel, C., Moreno-Mendoza, N. A., Villagrán-Santa Cruz, M., and Zúñiga-Vega, J. J. (2019). Placental structures and their association with matrotrophy and superfetation in poeciliid fishes. Acta Zool. 100, 167–181. doi: 10.1111/azo.12244
Olivera-Tlahuel, C., Ossip-Klein, A. G., Espinosa-Pérez, H. S., and Zúñiga-Vega, J. J. (2015). Have superfetation and matrotrophy facilitated the evolution of larger offspring in poeciliid fishes? Biol. J. Linn. Soc. 116, 787–804. doi: 10.1111/bij.12662
Ostrovsky, A. N., Gordon, D. P., and Lidgard, S. (2009). Independent evolution of matrotrophy in the major classes of Bryozoa: transitions among reproductive patterns and their ecological background. Mar. Ecol. Prog. Ser. 378, 113–124. doi: 10.3354/meps07850
Ostrovsky, A. N., Lidgard, S., Gordon, D. P., Schwaha, T., Genikhovich, G., and Ereskovsky, A. V. (2016). Matrotrophy and placentation in invertebrates: a new paradigm. Biol. Rev. 91, 673–711. doi: 10.1111/brv.12189
Peig, J., and Green, A. J. (2009). New perspectives for estimating body condition from mass/length data: the scaled mass index as an alternative method. Oikos 118, 1883–1891. doi: 10.1111/j.1600-0706.2009.17643.x
Peig, J., and Green, A. J. (2010). The paradigm of body condition: a critical reappraisal of current methods based on mass and length. Funct. Ecol. 24, 1323–1332. doi: 10.1111/j.1365-2435.2010.01751.x
Pires, M. N., Arendt, J., and Reznick, D. N. (2010). The evolution of placentas and superfetation in the fish genus Poecilia (Cyprinodontiformes: Poeciliidae: subgenera Micropoecilia and Acanthophacelus). Biol. J. Linn. Soc. 99, 784–796. doi: 10.1111/j.1095-8312.2010.01391.x
Pires, M. N., McBride, K. E., and Reznick, D. N. (2007). Interpopulation variation in life-history traits of Poeciliopsis prolifica: implications for the study of placental evolution. J. Exp. Zool. Part A Ecol. Genet. Physiol. 307A, 113–125. doi: 10.1002/jez.a.356
Pollux, B. J. A., Meredith, R. W., Springer, M. S., Garland, T., and Reznick, D. N. (2014). The evolution of the placenta drives a shift in sexual selection in livebearing fish. Nature 513, 233–236. doi: 10.1038/nature13451
Pollux, B. J. A., Pires, M. N., Banet, A. I., and Reznick, D. N. (2009). Evolution of placentas in the fish family Poeciliidae: an empirical study of macroevolution. Annu. Rev. Ecol. Evol. Syst. 40, 271–289. doi: 10.1146/annurev.ecolsys.110308.120209
Pollux, B. J. A., and Reznick, D. N. (2011). Matrotrophy limits a female’s ability to adaptively adjust offspring size and fecundity in fluctuating environments. Funct. Ecol. 25, 747–756. doi: 10.1111/j.1365-2435.2011.01831.x
Pothoven, S. A., Nalepa, T. F., Schneeberger, P. J., and Brandt, S. B. (2001). Changes in diet and body condition of lake whitefish in southern Lake Michigan associated with changes in benthos. N. Am. J. Fish. Manag. 21, 876–883. doi: 10.1577/1548-86752001021<0876:CIDABC<2.0.CO;2
Quintans, F., Scasso, F., Loureiro, M., and Yafe, A. (2009). Diet of Cnesterodon decemmaculatus (Poeciliidae) and Jenynsia multidentata (Anablepidae) in a hypertrophic shallow lake of Uruguay. Iheringia 99, 99–105.
R Core Team (2019). R: A Language and Environment for Statistical Computing. Available online at: https://www.r-project.org/ (accessed June 2019).
Rikardsen, A. H., Amundsen, P. A., Knudsen, R., and Sandring, S. (2006). Seasonal marine feeding and body condition of sea trout (Salmo trutta) at its northern distribution. ICES J. Mar. Sci. 63, 466–475. doi: 10.1016/j.icesjms.2005.07.013
Renfree, M. B., Suzuki, S., and Kaneko-Ishino, T. (2013). The origin and evolution of genomic imprinting and viviparity in mammals. Philos. Trans. R. Soc. B Biol. Sci. 368:20120151. doi: 10.1098/rstb.2012.0151
Reznick, D. N., Hrbek, T., Caura, S., De Greef, J., and Roff, D. (2007). Life history of Xenodexia ctenolepis: implications for life history evolution in the family Poeciliidae. Biol. J. Linn. Soc. 92, 77–85. doi: 10.1111/j.1095-8312.2007.00869.x
Reznick, D. N., Mateos, M., and Springer, M. S. (2002). Independent origins and rapid evolution of the placenta in the fish genus Poeciliopsis. Science 298, 1018–1020. doi: 10.1126/science.1076018
Riesch, R., Martin, R. A., and Langerhans, R. B. (2013). Predation’s role in life-history evolution of a livebearing fish and a test of the Trexler-DeAngelis model of maternal provisioning. Am. Nat. 181, 78–93. doi: 10.1086/668597
Saleh-Subaie, N., and Zúñiga-Vega, J. J. (2019). Are superfetation and matrotrophy more prominent in small species? Evol. Ecol. Res. 20, 403–425.
Schrader, M., and Travis, J. (2008). Testing the viviparity-driven-conflict hypothesis: parent-offspring conflict and the evolution of reproductive isolation in a poeciliid fish. Am. Nat. 172, 806–817. doi: 10.1086/592999
Schrader, M., and Travis, J. (2012). Assessing the roles of population density and predation risk in the evolution of offspring size in populations of a placental fish. Ecol. Evol. 2, 1480–1490. doi: 10.1002/ece3.255
Shine, R., and Bull, J. J. (1979). The evolution of live-bearing in lizards and snakes. Am. Nat. 113, 905–923. doi: 10.1086/283444
Simpkins, D. G., and Hubert, W. A. (2000). Drifting invertebrates, stomach contents, and body conditions of juvenile rainbow trout from fall through winter in a Wyoming tailwater. Trans. Am. Fish. Soc. 129, 1187–1195. doi: 10.1577/1548-86592000129<1187:DISCAB<2.0.CO;2
Torres-Mejia, R. M. (2011). Ecomorphology of Body Shape and Life History in Females of the Genus Gambusia (Poeciliidae) and in Guppies (Poecilia reticulata, Poeciliidae). Doctoral dissertation. Riverside, CA: University of California.
Trexler, J. C., and DeAngelis, D. L. (2003). Resource allocation in offspring provisioning: an evaluation of the conditions favoring the evolution of matrotrophy. Am. Nat. 162, 574–585. doi: 10.1086/378822
Trexler, J. C., and DeAngelis, D. L. (2010). “Modeling the evolution of complex reproductive adaptations in Poeciliid fishes: matrotrophy and superfetation,” in Viviparous Fishes II, eds M. C. Uribe and H. J. Grier (Florida: New Life Publications), 231–240.
Turcotte, M. M., Pires, M. N., Vrijenhoek, R. C., and Reznick, D. N. (2008). Pre-and post- fertilization maternal provisioning in livebearing fish species and their hybrids (Poeciliidae: Poeciliopsis). Funct. Ecol. 22, 1118–1124. doi: 10.1111/j.1365-2435.2008.01461.x
Van Dyke, J. U., Griffith, O. W., and Thompson, M. B. (2014). High food abundance permits the evolution of placentotrophy: evidence from a placental lizard, Pseudemoia entrecasteauxii. Am. Nat. 184, 198–210. doi: 10.1086/677138
Velando, A., and Alonso-Alvarez, C. (2003). Differential body condition regulation by males and females in response to experimental manipulations of brood size and parental effort in the blue-footed booby. J. Anim. Ecol. 72, 846–856. doi: 10.1046/j.1365-2656.2003.00756.x
Veggetti, A., Mascarello, F., Scapolo, P. A., Rowlerson, A., and Carnevali, M. C. (1993). Muscle growth and myosin isoform transitions during development of a small teleost fish, Poecilia reticulata (Peters) (Atheriniformes, Poeciliidae): a histochemical, immunohistochemical, ultrastructural and morphometric study. Anat. Embryol. 187, 353–361. doi: 10.1007/BF00185893
Wake, M. H. (2015). Fetal adaptations for viviparity in amphibians. J. Morphol. 276, 941–960. doi: 10.1002/jmor.20271
Young, B. J., López, G. C., Cristos, D. S., Crespo, D. C., Somoza, G. M., and Carriquiriborde, P. (2017). Intersex and liver alterations induced by long-term sublethal exposure to 17 α-ethinylestradiol in adult male Cnesterodon decemmaculatus (Pisces: Poeciliidae). Environ. Toxicol. Chem. 36, 1738–1745. doi: 10.1002/etc.3547
Zúñiga-Vega, J. J., Olivera-Tlahuel, C., and Molina-Moctezuma, A. (2017). Superfetation increases total fecundity in a viviparous fish regardless of the ecological context. Acta Oecol. 84, 48–56. doi: 10.1016/j.actao.2017.08.002
Keywords: lecithotrophy, matrotrophy, maternal provisioning, Poeciliidae, Poeciliopsis, reproductive modes, viviparous fishes
Citation: Saleh-Subaie N, Ramírez-Cruz GA and Zúñiga-Vega JJ (2021) Examination of the Trexler-DeAngelis Model of Maternal Provisioning Reveals That Matrotrophy Is Costly. Front. Ecol. Evol. 9:690430. doi: 10.3389/fevo.2021.690430
Received: 02 April 2021; Accepted: 01 September 2021;
Published: 23 September 2021.
Edited by:
Michael Tobler, Kansas State University, United StatesReviewed by:
Zachary Culumber, University of Alabama in Huntsville, United StatesRudiger Riesch, University of London, United Kingdom
Daniel Blackburn, Trinity College, United States
Copyright © 2021 Saleh-Subaie, Ramírez-Cruz and Zúñiga-Vega. This is an open-access article distributed under the terms of the Creative Commons Attribution License (CC BY). The use, distribution or reproduction in other forums is permitted, provided the original author(s) and the copyright owner(s) are credited and that the original publication in this journal is cited, in accordance with accepted academic practice. No use, distribution or reproduction is permitted which does not comply with these terms.
*Correspondence: J. Jaime Zúñiga-Vega, jzuniga@ciencias.unam.mx