Corrigendum: Epibiont assemblages on nesting hawksbill turtles show site-specificity in the Persian Gulf
- 1Department of Animal Sciences and Marine Biology, Faculty of Life Sciences and Biotechnology, Shahid Beheshti University, Tehran, Iran
- 2Qeshm Environmental Conservation Institute (QECI), Qeshm, Iran
Sea turtle epibionts can provide insights into the hosts' habitat use. However, at present, there is a lack of information on sea turtle epibiont communities in many locations worldwide. Here, we describe the epibiont communities of 46 hawksbill turtles (Eretmochelys imbricata) in the Persian Gulf. Specifically, we sampled 28 turtles from the Dayyer-Nakhiloo National Park (DNNP) in the northern Gulf and 18 turtles from Shibderaz beach in the Strait of Hormuz. A total of 54 macro, meio, and micro-epibiont taxa were identified, including 46 taxa from Shibderaz and 29 taxa from DNNP. The barnacles Chelonibia testudinaria and Platylepas hexastylos, as well as harpacticoid copepods and Rotaliid foraminifers, had the highest frequency of occurrence found on almost all turtle individuals. Harpacticoids were the most abundant epizoic taxa (19.55 ± 3.9 ind. per 9 cm2) followed by forams (Quinqueloculina spp.: 6.25 ± 1.5 ind. per 9 cm2 and Rotaliids: 6.02 ± 1.3 ind. per 9 cm2). Our results showed significant differences between the study sites in the composition of micro and macro-epibiont communities found on hawksbill turtles. We speculate that the differences in epibiont communities were largely influenced by local environmental conditions.
Introduction
Epibiosis is a symbiotic relationship where one organism (epibiont) lives on the surface of the other (basibiont) (Wahl and Mark, 1999; Harder, 2008). A wide variety of epibiont communities are found on sea turtles (Wahl, 1989; Pfaller et al., 2008b; Frick and Pfaller, 2013; Majewska et al., 2015) including macro, meio, and micro-epibionts. Macro-epibiont communities encompassing cirripeds, polychaetes, hydrozoans, bryozoans, poriferans, tunicates, periphytic algae, and some motile organisms have been widely studied on different sea turtle species (Caine, 1986; Pfaller et al., 2008b; Fuller et al., 2010; Lazo-Wasem et al., 2011; Robinson N. J. et al., 2017; Robinson et al., 2019), and meiofaunal organisms such as nematodes and copepods have recently been the focus of several studies (Aznar et al., 2010; Corrêa et al., 2013; Domènech et al., 2017; Ingels et al., 2020). Likewise, micro-epibiota on sea turtles, represented mostly by colonizing diatoms, have recently been assessed (Majewska et al., 2015, 2017; Robinson et al., 2016; van de Vijver et al., 2020). Some of these epibionts, such as the barnacle Chelonibia testudinaria, have a wide geographical distribution (Rawson et al., 2003; Lazo-Wasem et al., 2011), whereas some others, like some short-lived diatom species, may have a relatively narrow and local distribution (Abarca et al., 2014).
Barnacles are the most prominent epibionts of sea turtles (Casale et al., 2012; Frick and Pfaller, 2013). Turtle barnacles belong to the superfamily Coronuloidea and include three families: Chelonibiidae Pilsbry, 1916, Coronulidae Leach, 1817, and Platylepadidae Newman and Ross, 1976 (Hayashi, 2012, 2013). Members of Chelonibiidae are perhaps the most studied barnacle species recorded on sea turtles. Chelonibia testudinaria, the most commonly reported sea turtle barnacle, has been reported on the body surface of all extant sea turtle species (Sloan et al., 2014), sirenians (Zardus et al., 2014), and some crustaceans (Cheang et al., 2013) from distant geographical regions. It is, therefore, considered a host generalist species and should not be assumed as an obligatory turtle barnacle (Cheang et al., 2013; Zardus et al., 2014). In contrast, Chelonibia caretta, which is considered a host specialist, is reported only in association with sea turtles, especially loggerheads (Caretta caretta) (Torres-Pratts et al., 2009; Farrapeira, 2010).
Several techniques have been successfully used to study habitat use and migration patterns of sea turtles, including satellite telemetry (e.g., Rees et al., 2016; Robinson D. P. et al., 2017; Hays and Hawkes, 2018; Pilcher et al., 2020), aerial surveys (Jean et al., 2010), visual surveys via snorkeling (Roos et al., 2005), and stable isotope analysis (e.g., Nolte et al., 2020). However, most of these techniques are costly (Pfaller et al., 2014), and/or logistically difficult to implement. As an alternative, or complementary and relatively low-cost approach, epibiont assemblages living on sea turtles can roughly indicate habitat use and migratory behavior of these highly mobile marine reptiles (e.g., Pfaller et al., 2008b; Lazo-Wasem et al., 2011; Rivera et al., 2018; Robinson et al., 2019; Nolte et al., 2020; Silver-Gorges et al., 2021). For instance, some sea turtle epibionts, e.g., C. testudinaria and two lepadid barnacles Lepas hilli and Conchoderma virgatum, have been proposed to be potentially used as habitat indicators of sea turtles (Casale et al., 2012; Ten et al., 2019). According to previous studies, the barnacles L. hillii, C. virgatum (Ten et al., 2019), and Platylepas spp. (Casale et al., 2012) preferably settle on turtles inhabiting oceanic waters. In contrast, C. testudinaria, Stomatolepas elegans, and Stephanolepas muricata are mainly associated with turtles occupying neritic waters (Casale et al., 2012). Epibiotic barnacles and crabs have also been used as indicators of the distribution and movement of loggerheads (Casale et al., 2004). Thus, epibiont communities could roughly reflect the environment in which the host has recently been living (Casale et al., 2012; Frick and Pfaller, 2013; Nolte et al., 2020; Silver-Gorges et al., 2021). In addition, this method could be very useful in sea turtle conservation planning efforts, as epibionts may affect their health status. Stranded turtles were frequently utilized in studies to examine factors that affect their health and mortality (Sönmez, 2018; Cheng et al., 2019; Wang et al., 2020). Turtle epibionts may cause increased drag (Logan and Morreale, 1994; Wyneken, 1997), which could be energetically expensive for the host turtles, particularly for those undertaking long-distance migrations (Frick and Pfaller, 2013). Additionally, some turtle epibionts such as leeches and barnacles may cause infections in sea turtles (George, 1997; Greenblatt et al., 2004), or enhance their vulnerability to pathogens (George, 1997). The presence of some coronuloid barnacles on eyes and wounds, as well as their penetration into the epidermis of the host's flippers, may have a negative influence on their health (Frick et al., 2011).
The marine environment of the Persian Gulf is characterized by high and wide-ranging temperatures [sea surface temperatures (SST) from 15° to 36°C, Riegl and Purkis, 2012] and high salinities (>39 psu in most areas, Sheppard et al., 2010). This is a challenging environment for many organisms, leading to impoverished biodiversity in this semi-enclosed body of water compared to other coastal habitats of the Indian Ocean (Sheppard et al., 2010). Satellite telemetry has partially revealed habitat use and migratory behavior of the turtles in this region. Hawksbill turtles (Eretmochelys imbricata) spend most of their time feeding on foraging grounds in shallow waters near the coasts of Qatar, Saudi Arabia, and UAE while spending only a small portion of their life nesting on Iranian coasts (Pilcher et al., 2014). In summer, when the SST rises to 33°C, hawksbills leave shallow foraging grounds and move northward to deeper waters (30–50 m) of the Persian Gulf (Pilcher et al., 2014; Marshall et al., 2020).
Hawksbill turtles, along with green turtles (Chelonia mydas), are the dominant sea turtle species in the Persian Gulf. It is assumed that hawksbill turtles nesting along the Iranian shores of the Gulf may comprise one of the most important nesting populations in the Indian Ocean region (Meylan and Donnelly, 1999). Therefore, obtaining information on epibiont communities of hawksbills in the Gulf, especially those that are likely indicators of nesting ecology, can aid in their management. Additionally, epibionts could be used as bio-indicators of ecological change in the Persian Gulf. Despite this, our knowledge about epibiont communities of the Gulf's hawksbill turtles is restricted to a few studies on turtle barnacles (Loghmani-Devin and Sadeghi, 2010; Razaghian et al., 2019). In this study, we present the first comprehensive dataset on the diversity, assemblage, and abundance of macro, meio, and micro-epibionts of hawksbill turtles nesting at two distant sites along the Iranian coastline of the Persian Gulf, one at the northwest coast, and the other at the Strait of Hormuz. Due to the differences in environmental conditions of the sites, we hypothesized that epibiont assemblages of the two turtle rookeries might show site-specific differences.
Materials and Methods
Study Area
Ommolgorm (27° 50′N, 51° 33′E) and Nakhiloo (27° 51′N, 51° 26′E) islands in Dayyer-Nakhiloo National Park (DNNP) and located at the center of Iran's northwestern Persian Gulf coast, and Shibderaz (26° 41′N, 55° 55′E), a 2 km sandy beach on the south coast of Qeshm Island in the Strait of Hormuz (the entrance of the Persian Gulf; Figure 1) were used as study sites. Sea surface temperature and salinity data were obtained during 2017 and 2018 for each site (Table 1) from the Copernicus Marine Environment Monitoring Service (http://marine.copernicus.eu; product reference: CMEMS-GLO-PUM-001-024).
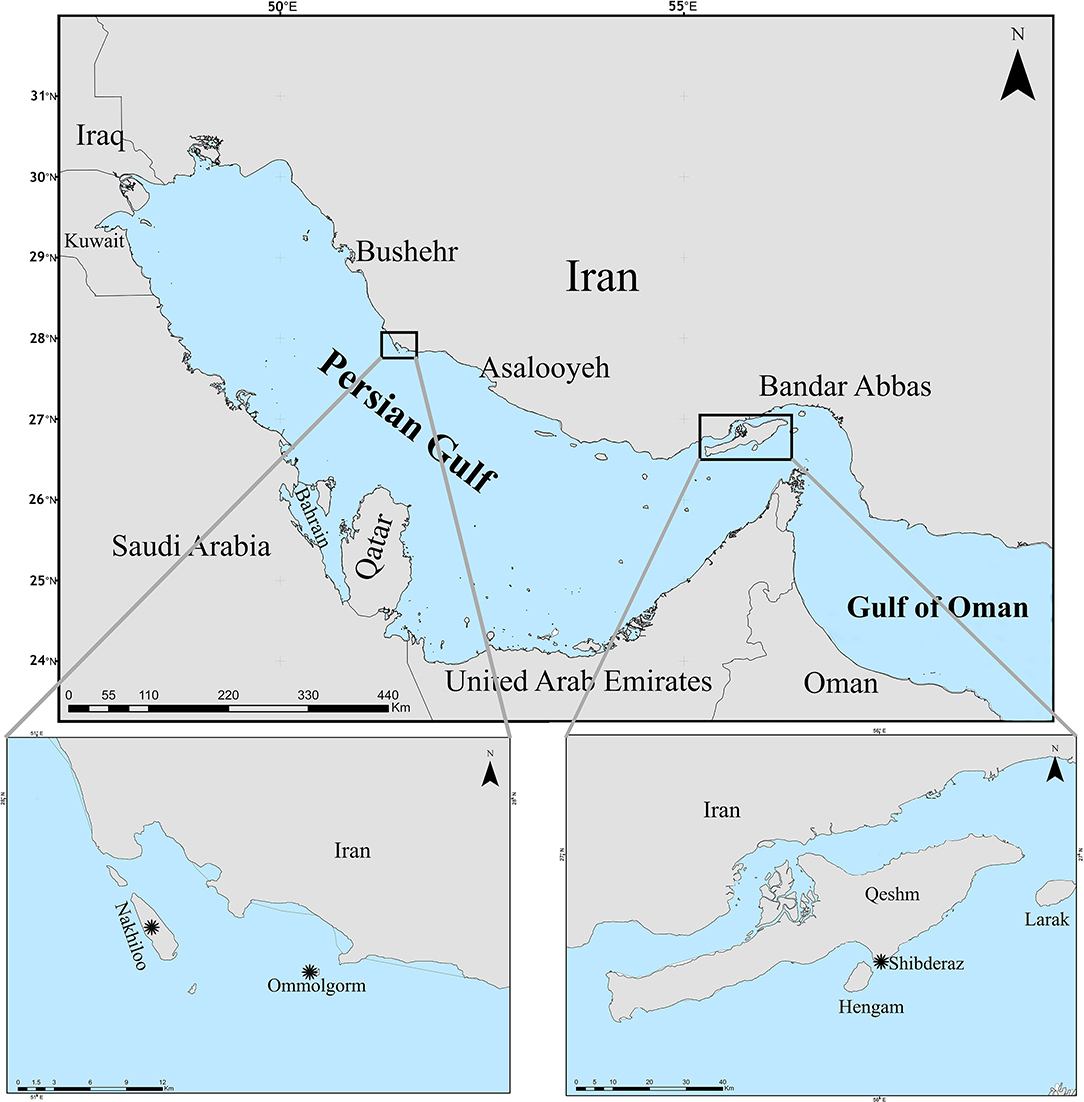
Figure 1. Sampling sites (marked by asterisks) of hawksbill turtle epibionts, Ommolgorm, and Nakhiloo islands in Dayyer-Nakhiloo National Park (DNNP) and Shibderaz on Qeshm Island in the Iranian coasts of the Persian Gulf.
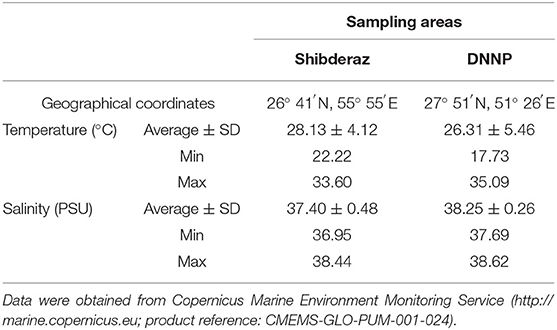
Table 1. Sea surface temperature and salinity values at Shibderaz and Dayyer-Nakhiloo National Park (DNNP) during 2017 and 2018.
Field Surveys and Sample Collection
The beach areas of both sites were patrolled between March and June in 2017 and 2018. All encountered nesting turtles were examined after the completion of oviposition to avoid interrupting the nesting process. Each turtle was first measured for curved carapace length (CCL) to the nearest 1 mm, and its body was gently washed with clean seawater to remove sand and particles. Following that, a digital camera (Sony DSC-HX9V) was used to photograph the carapace, plastron, head, and soft parts to measure barnacle abundance on each body part using a non-invasive approach. Further, three randomly chosen portions of the carapace surface (9 cm2) were gently shaved and the keratin materials collected were preserved in 4% formaldehyde solution diluted with filtered seawater. To study diatoms, ~4 cm2 of the outer-most layer of the three different scutes were taken and immediately fixed in vials containing 4% formaldehyde solution diluted by filtered seawater (Majewska et al., 2015). For precise identification of the barnacles, in addition to using photographs, a few barnacle individuals from visually distinct species were physically removed with a safe plastic knife and preserved in vials containing 96% ethanol for laboratory examinations. In total, epibiont samples were collected from 46 nesting hawksbill sea turtles (28 turtles from DNNP and 18 turtles from Shibderaz).
Species Identification and Quantification
Zooepibionts of each collected sample were isolated from algal mats under a stereomicroscope with a magnification of 80x. Specimens were then identified to the lowest possible taxonomic level and their abundance was determined. Scute samples for diatoms identification were subsampled to ca. 1 cm2, dehydrated through 25, 50, 60, 70, 80, 90, and 100% ethanol series. The samples were then air-dried in a desiccator containing silica gel, placed on microscope slides, sputter-coated with gold, and identified using images taken with a Hitachi SU3500 (Hitachi High-Technologies, Tokyo, Japan) scanning electron microscope (SEM), operating at 15 kV.
We used standard morphological keys following Chan et al. (2009) and Shahdadi et al. (2014) to identify the barnacle species. Sea turtle foraminifera epibionts were identified using the Atlas of Benthic Foraminifera (Holbourn et al., 2013). To identify macroalgae epibionts on hawksbill turtles we utilized the Atlas of the sea algae of the Persian Gulf and Oman Sea coasts (Gharanjik and Rohani Ghadikolaei, 2009) and the Field Guide of Marine Macroalgae of Kuwait (Al-Yamani et al., 2014). Other epibiont taxa were identified using the relevant literature (e.g., Taylor et al., 2007; Guerra-García et al., 2010; Martin et al., 2014).
As the most prominent and visible epibiont taxa, barnacles were analyzed in greater detail. Total and mean barnacle abundance were recorded on each body part (head, carapace, plastron, supra-caudals, and soft parts) using photographs (see above). Image J software (version 1.43 u) was used to measure the basal diameter (Nasrolahi et al., 2013) of each individual barnacles found on turtles.
Statistical Analysis
A Kolmogorov-Smirnov test was used to check for normality, and revealed that the data did not exhibit a normal distribution even after being transformed. The Kruskal-Wallis non-parametric test was performed to compare barnacle abundances, and a Mann-Whitney U-test was used to evaluate differences in C. testudinaria rostro-carinal diameter (RCD) among different body parts (head, carapace, plastron, supra-caudals, and soft parts). A Mann-Whitney U-test was also used to compare P. hexastylos RCD between plastron and soft parts of hawksbill turtles encountered in Shibderaz and DNNP.
A PERMANOVA statistical test was used to compare assemblage structure and species composition of sea turtle epibionts between the two study sites. Except for diatoms and other algal taxa, for which only presence-absence data were recorded, the analysis of epibiont structure was based on absolute abundance data. Species composition of the entire epibiont community (including micro, meio, and macro-epibionts) was evaluated based on presence-absence data. A SIMPER (similarity percentage) test was performed to identify the relative contribution of each epibiont taxon to any dissimilarity values between the epibiont assemblages of hawksbill turtles nesting on the two sites. Graphical representation of the similarity was carried out using non-metric multidimensional scaling (nMDS) based on the square-root-transformed abundance data and the Bray–Curtis similarity measure of all identified epibiont taxa for each turtle. Furthermore, a PERMANOVA was used to compare species composition of the macro, meio, and micro-epibionts between the two study sites. Following this, a SIMPER analysis was used to reveal the dissimilarity of epibiont groups between the two sites as well as the contribution of each taxon to the dissimilarity. All the analyses were performed and graphs generated using the statistical software SPSS 26 (George and Mallery, 2019) and Primer 6.0+PERMANOVA (Clarke and Gorley, 2006; Anderson et al., 2008). A significance level of <0.05 was used to reject null hypotheses for all tests.
Results
Examined Turtles
A total of 46 hawksbill turtles were examined from both nesting sites. At Shibderaz, the mean CCL (±SE) was 73.6 ± 0.6 cm (range 69.5–78.0 cm). At DNNP, the mean CCL ± SE was 71.9 ± 0.5 cm (range 67.5–77.0 cm). The overall mean CCL (±SE) for both sites was 72.6 ± 0.4 cm, ranging from 67.5 to 78.0 cm.
Composition and Structure of Epibiont Communities
In total, 54 macro-, meio-, and micro-epibiont taxa including 28 diatoms, five filamentous algae, four barnacles, three foraminifers, and two amphipod species. In addition, single-taxon representatives of bivalves, copepods, cumaceans, gastropods, haptophytes, leeches, hydrozoans, nematodes, ostracods, polychaetes, sponges, and tanaids were identified on hawksbill sea turtles at both nesting sites (Table 2). From these, 46 taxa were found on turtles from Shibderaz, whereas only 29 taxa were identified on turtles from DNNP. The difference was largely driven by diatoms. Of the 28 total diatom taxa belonging to 17 genera, 25 taxa were identified in samples collected from Shibderaz whereas only five taxa were observed from DNNP (Table 2). Chaetomorpha sp. and Ozobranchus sp. were recorded only from Shibderaz and Polysiphonia sp., Caprella sp., a bivalve, and a polychaete were only identified in DNNP. Examples of different epibiont taxa are shown in Figure 2.
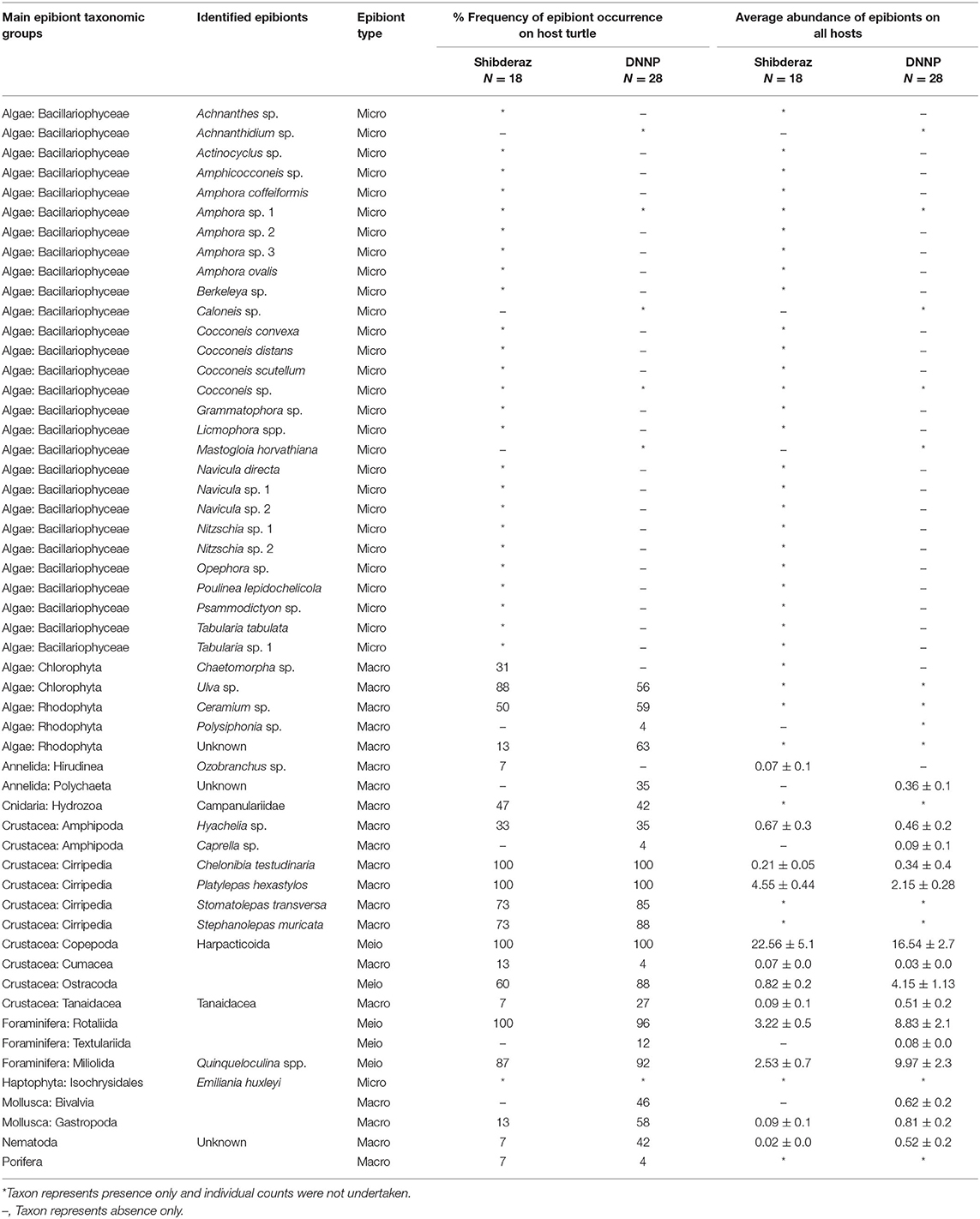
Table 2. Epibiont species list, abundance (ind. per 9 cm2), and frequency of occurrence on hawksbill (Eretmochelys imbricata) turtles (N = 46) nesting on Shibderaz (Qeshm Island) and Dayyer-Nakhiloo National Park (DNNP; Bushehr) beaches, Iran.
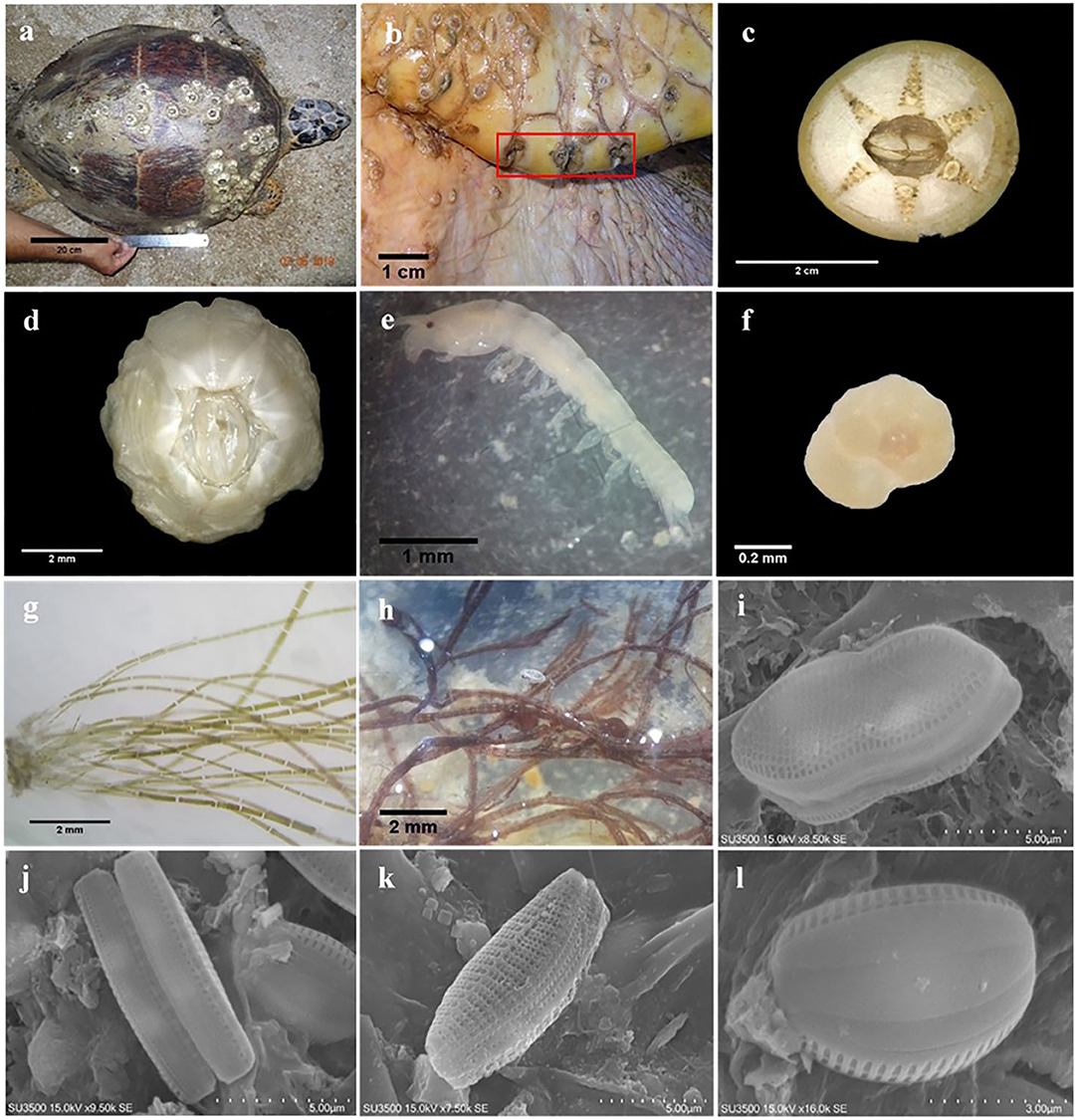
Figure 2. Examples of epibiont taxa recorded on the body surface of hawksbill sea turtles in the Iranian coasts of the Persian Gulf: (a) C. testudinaria on the carapace of hawksbill sea turtle; (b) specimens of Stephanolepas muricata; (c) Chelonibia testudinaria; (d) Platylepas hexastylos; (e) Tanaid; (f) Rotaliid foraminifer; (g) Chaetomorpha sp.; (h) Polysiphonia sp.; (i) Psammodictyon sp.; (j) Nitzschia sp.; (k) Tabularia sp.1; (l) Amphora sp.1.
Among macrofauna, C. testudinaria and P. hexastylos were present on all examined turtles. Among the meiofauna, harpacticoid copepods, and Rotaliid foraminifers were also observed on almost all sea turtle individuals. Likewise, the filamentous alga Ulva sp. showed a high frequency of occurrence on turtles (88 and 56% at Shibderaz and DNNP, respectively, Table 2). Harpacticoids (64.5%) followed by P. hexastylos (13%) and Rotaliids (9.3%) were the most abundant epizoic taxa on turtles from Shibderaz, whereas harpacticoids (36.4%), Quinqueloculina spp. (22%) and Rotaliids (19.5%) were the most dominant taxa on turtles at DNNP (Figure 3).
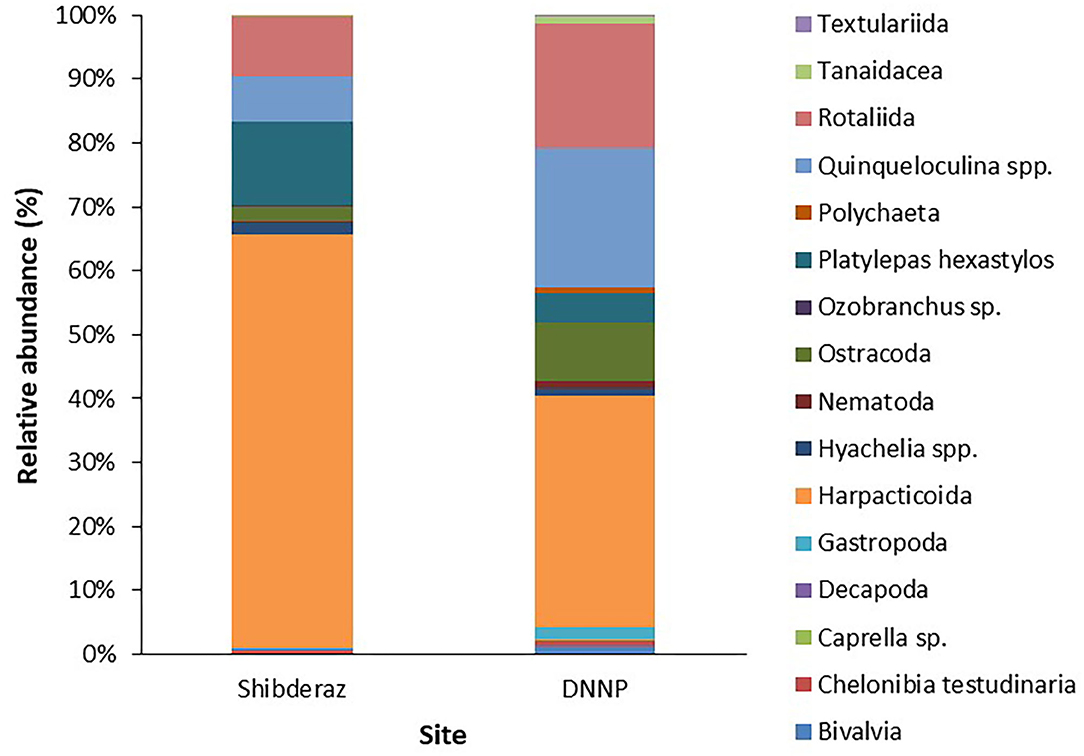
Figure 3. Relative abundance (%) of epibiont taxa on the body surface of the nesting hawksbill turtles in Shibderaz and Dayyer-Nakhiloo National Park (DNNP).
The PERMANOVA analysis identified statistically significant site-based differences in the epibiont species composition and community structure on studied turtles [Pseudo-F = 5.89, P (perm) < 0.001; Pseudo-F = 17.51, P (perm) < 0.001, respectively, Supplementary Table S1]. Similarly, the nMDS plot shows that species composition and community structure were noticeably different between the two sites (Figure 4). The SIMPER analysis showed 35.71% dissimilarity between the two sites. Rhodophyta (7.1%), Gastropoda (6.32%), Bivalvia (5.64%), Campanulariidae (5.55%), Ceramium sp. (5.54%), Ulva sp. (5.11%), and Hyachelia sp. (4.93%) contributed to more than 40% of the difference (Table 3). When separating the epibionts into macro, meio, and micro-epibiont groups, a significant difference between the two sites in species composition of the micro and macro-epibionts was detected [Pseudo-F = 15.32, P (perm) < 0.001, Pseudo-F = 9.02, P (perm) = 0.001, respectively]. The SIMPER analysis revealed 97.68 and 39.37% dissimilarity between the two sites, respectively. Diatom species—including Cocconeis spp. (23.83%), Caloneis sp. (9.43%), Amphora sp. 1 (7.14%), and Amphora ovalis (6.80%)—contributed around 47% to the differences of the micro-epibionts (Table 3). Rhodophyta (10.45%), Gastropoda (9.31%), Ceramium sp. (8.28%), Campanulariidae (8.23%), Bivalvia (8.19%), and Ulva sp. (7.66%) explained 52% of the macro-epibiont variances (Table 3).
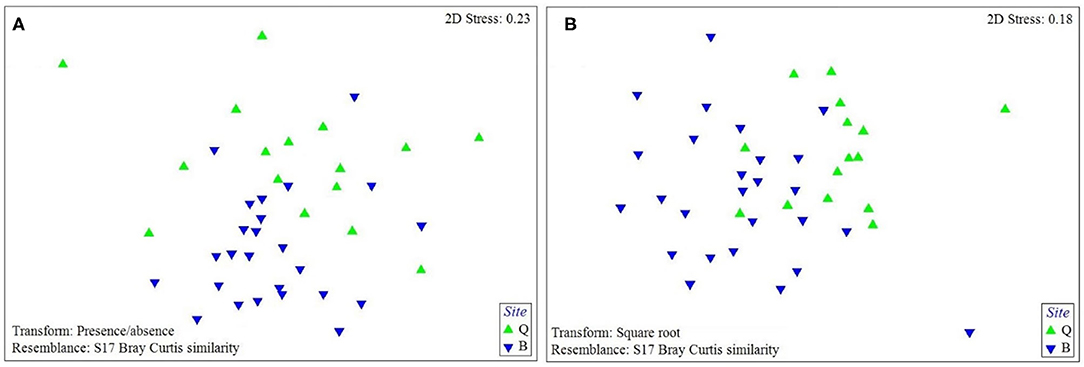
Figure 4. The nMDS plot of (A) the species composition and (B) assemblage structure of sea turtle epibionts at each study site based on Bray-Curtis similarity matrix performed on presence-absence data for species composition and square-root transformed data for assemblage structure (Q: Shibderaz, Qeshm Island; and B: DNNP, Bushehr province).
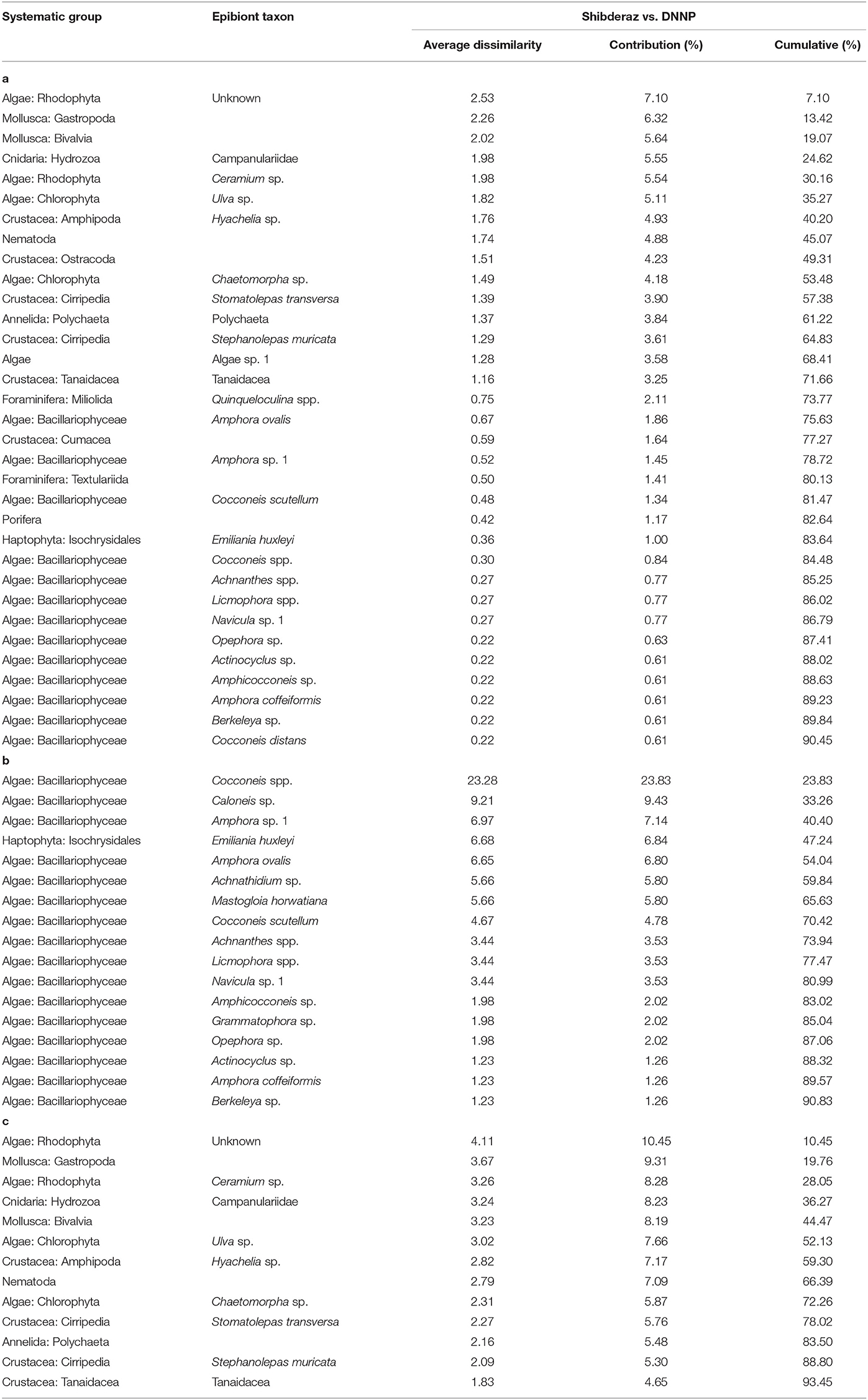
Table 3. Results of the SIMPER procedure to identify the relative contribution of each epibiont taxa to the dissimilarity between the epibiont assemblages of hawksbills (Eretmochelys imbricata) nesting on Shibderaz (Qeshm Island) and Dayyer-Nakhiloo National Park (DNNP; Bushehr) beaches, Iran: (a) all epibionts, (b) micro-epibionts and (c) macro-epibionts.
Barnacle Composition and Distribution
Four barnacle species, including P. hexastylos, C. testudinaria, Stomatolepas transversa, and Stephanolepas muricata were identified on the body surface of examined turtles. About 95% of P. hexastylos individuals were found on the flippers and soft parts, while only 5% were recorded on the plastron scutes; no individuals were observed on the carapace. C. testudinaria individuals were distributed more broadly, with 51% distributed on the plastron, 37% on the carapace, 10% under the supracaudals, and 2% on the head. Individuals of S. transversa were only observed along the plastral sutures and S. muricata was only found attached to the leading edges of the front flippers. In general, 85.3% of all barnacles were attached on flippers and soft parts, 9.7% on the plastron, 3.8% on the carapace, 1% under supracaudals, and 0.2% on the head (Table 4).
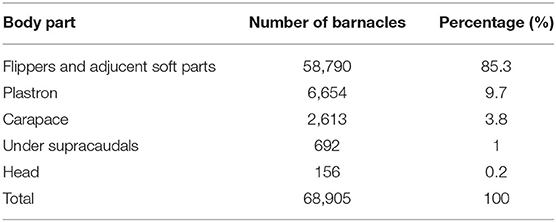
Table 4. Occurrence of barnacles on different body parts of the hawksbill sea turtles nesting on Shibderaz (Qeshm Island) and Dayyer-Nakhiloo National Park (DNNP) in the Persian Gulf.
Barnacle Abundance
As S. transversa and S. muricata were small and difficult to distinguish from each other, which made it challenging to precisely count them using the images. We visually estimated their total abundance to be <2%. Thus, we only counted P. hexastylos and C. testudinaria. A total of 68,905 individual barnacles were counted on body parts (including carapace, plastron, head, neck, flippers, and soft parts) of turtles. Of these, there were 61,837 (90%) and 7,068 (10%) individuals of P. hexastylos and C. testudinaria, respectively. The greatest barnacle load was found on a 72 cm (CCL) turtle that had 3,774 barnacles (3,659 P. hexastylos and 115 C. testudinaria) and the lowest measured barnacle load was from a 70.5 cm (CCL) turtle that was carrying 212 barnacles (146 P. hexastylos and 66 C. testudinaria).
The overall mean (±SE) barnacle abundance (1497.9 ± 133.7) was significantly different on various body parts of turtles (p < 0.05, Supplementary Table S2). Mean barnacle abundance was 1278.0 ± 123.4 on the flippers and adjacent soft parts, 144.7 ± 16.4 on the plastron, 56.8 ± 8.2 on the carapace, 15.0 ± 2.5 under the supracaudal scutes, and 3.4 ± 1.1 on the head. The mean abundance for C. testudinaria and P. hexastylos was 153.7 ± 15.7 and 1344.3 ± 130.1, respectively.
Barnacle Rostro-Carinal Diameter
The results of the Mann-Whitney U-test showed that there was no significant difference in the mean RCD of C. testudinaria on different body parts of turtles nesting at Shibderaz vs. DNNP (p > 0.05). Therefore, the data for both sites were pooled. The RCD of C. testudinaria was significantly different among different body parts (i.e., head, carapace, plastron, and supracaudal) (Figure 5A, p < 0.05, Supplementary Table S2). The highest mean barnacle RCD (22.57 ± 9.47) was observed on the head and the lowest (12.27 ± 5.24) on the supracaudal scutes (Figure 5A). The mean RCD of P. hexastylos was significantly higher in Shibderaz compared to DNNP (Figure 5B, p < 0.05, Supplementary Table S2). The size-frequency distribution of C. testudinaria showed a skewness by some large barnacle individuals (Figure 6). It showed a peak at 5.01–10 mm followed by two smaller peaks at 10.01–15 and 15.01–20 mm. There were few large barnacles with a size range of 55.01–60 mm (Figure 6).
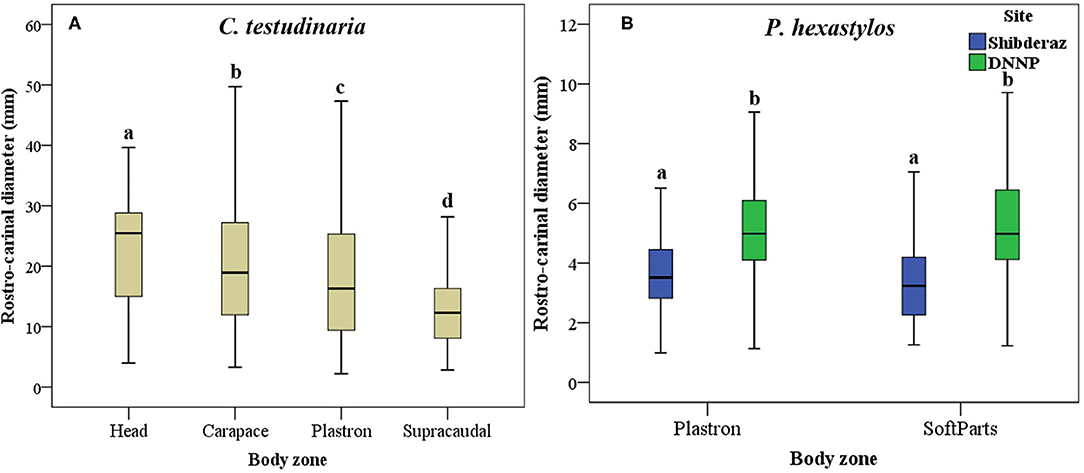
Figure 5. Box plot showing Rostro-Carinal Diameter (RCD) of the turtle barnacles on different body parts of hawksbill turtles (E. imbricata) in Shibderaz and Dayyer-Nakhiloo National Park (DNNP): (A) Chelonibia testudinaria on head, carapace, plastron, and supracaudal and, (B) Platylepas hexastylos on plastron and soft parts.
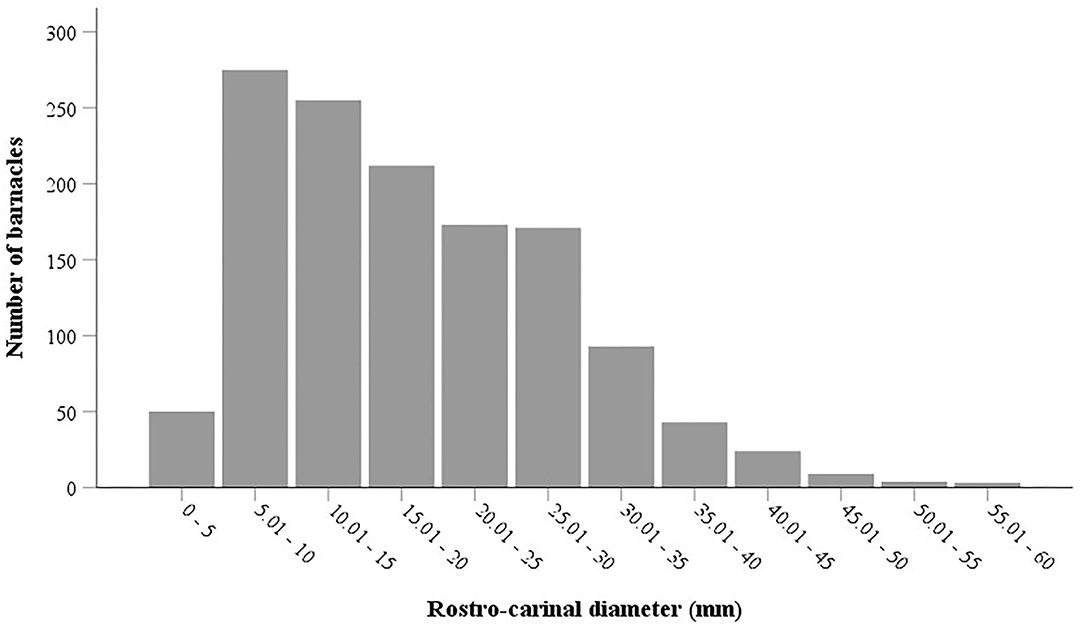
Figure 6. Size frequency distribution of the barnacle Chelonibia testudinaria on different body surfaces of the hawksbill sea turtles nesting on Shibderaz and Dayyer-Nakhiloo National Park (DNNP).
Discussion
The majority of research on turtle epibionts has focused on the epibiont loads on the carapace (see Caine, 1986; Pfaller et al., 2008a,b; Fuller et al., 2010), presuming that the abundance of epibionts is highest on this body part. However, this notion has been contradicted by more recent studies (e.g., Robinson et al., 2019) that have found epibiont abundance on soft skin to be higher than on the carapace and plastron. To provide a holistic qualitative or quantitative data set of the epibiont communities of sea turtles, it is therefore essential to conduct a full-body examination (Robinson et al., 2019). Although most prior research concentrated on macro-epibiota (e.g., Frazier et al., 1985; Fuller et al., 2010; Lazo-Wasem et al., 2011; Casale et al., 2012), meio and micro-epibiota have recently received increasing attention due to their high diversity and abundance in sea turtles, as well as advancements in microscopic techniques that have facilitated greater study of these smaller organisms (e.g., Corrêa et al., 2013; Majewska et al., 2015, 2017; Robinson et al., 2016; Azari et al., 2020; Ingels et al., 2020; Silver-Gorges et al., 2021). Some of these taxa, such as diatoms, are found on all sea turtle species (Majewska et al., 2015; Robinson et al., 2016) and are considered ecological indicators (El-Semary, 2016; Majewska et al., 2017). A comprehensive baseline study on the epibionts of sea turtles should, therefore, encompass both macroscopic and microscopic epibiota to depict a better picture of the turtle epibiont assemblages (Majewska et al., 2015). To the best of our knowledge, our study is the first study that has simultaneously assessed macro-, meio-, and micro-epibionts on sea turtles. Some of these epibionts may distinguish groups of sea turtles (see Ingels et al., 2020) and reveal their movement pathways. Sea turtle conservation and management might benefit from research into the identification and origin of epibiont species or communities that are likely to be indicators of feeding or nesting sites.
Our results showed a statistically significant difference in the structure and species composition of epibiont assemblages in the two study sites (Supplementary Table S1), with higher species diversity in the Shibderaz at the entrance of the Persian Gulf compared to that of DNNP at the mid part of the sea. We suggest that these differences in turtle epibiont assemblages among different habitats in the Persian Gulf might result from differences in environmental conditions at each study site. Extreme and wide-ranging temperature fluctuations and high salinity in the Persian Gulf have led to the selection of tolerant taxa, which may result in impoverished biodiversity in the region (Sheppard et al., 2010). However, the environmental extremes are not similar in all marine habitats of the sea. The Gulf receives incoming currents from the Gulf of Oman via the Strait of Hormuz, which flow counterclockwise through the Gulf and exit via the bottom of the Strait (Sheppard et al., 2010). Along the Iranian coastline of the Gulf, temperature and salinity increase with incrementing distance from the Strait (Reynolds, 1993). This is also evident from the temperature and salinity data presented in this study for the sites investigated (Table 1). Further, as a result of shape, bathymetry, and wind regime, waters close to the Strait of Hormuz are nutrient-rich (German and Elderfield, 1990; Longhurst et al., 1995).
Azari et al. (2020) studied diatoms on foraging green turtles in the Persian Gulf and found that diatom abundance on turtles collected from the Strait of Hormuz was higher than that of on turtles collected from the Gulf habitats found farther from the Strait. However, their findings were based on green turtles that dwell in foraging habitats, while our study examined hawksbill turtles in their nesting habitats, where they reside temporarily. The results of a previous post-nesting satellite tracking study showed that most of the Gulf hawksbills nesting along the Iranian coastline migrate to foraging grounds in the southeastern Persian Gulf and establish home ranges of 40 to 60 km2 (Pilcher et al., 2014). The same study revealed that the Gulf hawksbill turtles spend only 6% of their time at the nesting grounds, whereas they spend about 68% in foraging grounds, about 20% conducting summer seasonal movements, and 5% migrating between foraging and nesting areas (Pilcher et al., 2014). Therefore, variable epibiont taxonomic composition at each nesting site is thought to be the outcome of various environmental conditions at the nesting grounds during a short period of time (i.e., about 6% of their time as reported by Pilcher et al., 2014). This mostly includes the short-living taxa such as diatoms.
In this research, we found more diverse diatoms on hawksbills and recorded only 11 taxa that were also found by Azari et al. (2020). Although these studies were carried out almost in the same area, the host species was different; hawksbills were studied here whereas green turtles were the focus of Azari et al. (2020). We, therefore, speculate that the difference in diatom species composition between our study and that of Azari et al. (2020) is partially due to differences in the behavior and local habitat use between these two Gulf turtle species. However, we acknowledge that it could also be a result of annual variations in the abundance and composition of diatom communities.
Some turtle epizoic taxa have a wide geographic distribution. Barnacle species including C. testudinaria, P. hexastylos, Stomatolepas sp., and S. muricata, for example, have been found on sea turtles from various locations (see Hayashi and Tsuji, 2008; Fuller et al., 2010; Lazo-Wasem et al., 2011; Casale et al., 2012; Domènech et al., 2015; Robinson et al., 2016, 2019). In addition to barnacles, harpacticoids (especially, Balaenophilus manatorum) and the amphipod, Caprella sp. have also shown a wide range of distribution on sea turtles (Caine, 1986; Pfaller et al., 2008b; Sezgin et al., 2009; Aznar et al., 2010; Casale et al., 2012; Domènech et al., 2015). The presence of these epibionts is seemingly not affected strongly by local environmental conditions. This wide distribution has also been locally observed in our study shown by the frequency of occurrence of some macro- and meio-epizoic taxa including barnacles (C. testudinaria and P. hexastylos), harpacticoid copepods, and rotaliid foraminifers. Our results revealed that while macro- and meio-epibiont taxa assemblages are relatively similar at both sites (16 macro- and 4 meio-epibiont taxa at Shibderaz; 18 macro- and 4 meio-epibiont taxa at DNNP, Table 1), micro-epibionts (26 taxa at Shibderaz and 6 taxa at DNNP, Table 1), represented mostly by diatoms, differ significantly. This was also evident by the high dissimilarity in species composition of micro-epibionts between the two sites (>97%, Table 3). We suggest that micro-epibionts may be considered as more sensitive bioindicators.
The most prominent turtle epibionts, barnacles, have shown contrasting spatial patterns on different body parts of studied turtles (Hayashi and Tsuji, 2008; Pfaller et al., 2008b; Fuller et al., 2010; Nájera-Hillman et al., 2012; Razaghian et al., 2019; Robinson et al., 2019). We also found a relative niche partitioning among different barnacle species. P. hexastylos individuals were observed mostly on the flippers and soft parts, while S. transversa was seen along the plastral sutures and S. muricata was mostly embedded in the gaps between scales in the leading edges of the front flippers. C. testudinaria showed a wider distribution attaching to both plastron and carapace. These distribution patterns are mainly driven by factors associated with feeding and attachment, including water flow (Pfaller et al., 2008a) and substratum characteristics (Fuller et al., 2010). These factors may also influence the barnacle size as was reflected by the RCD of our measured barnacles. Our results show that the most frequent RCD size range of C. testudinaria was 5–10 mm with a unimodal size-frequency distribution probably indicating only a single-age class of barnacles. These results are in line with those of Lim et al. (2020) on the size-frequency distribution of C. testudinaria on sea turtles, but are contradictory to Ewers-Saucedo et al. (2015) and Ten et al. (2019) who detected a bimodal size-frequency distribution of C. testudinaria in their studies. We speculate that the year-round reproduction of C. testudinaria in the Persian Gulf as a warm subtropical sea is the reason for the lack of age classes compared to those from more seasonally affected areas.
In this research, the most abundant barnacle species on sea turtle bodies was P. hexastylos. A similar result was also found by Habibi Motlagh et al. (2020) who studied foraging green turtles in the Gulf. Similar to Robinson et al. (2019), we found that barnacle abundance on soft parts, including flippers, neck, and tail was considerably higher than on the carapace and plastron (Table 4). In contrast, Razaghian et al. (2019) studied the distribution pattern of epibiont barnacles on nesting hawksbills in DNNP and found that barnacle abundance was much higher on the plastron and carapace than on soft parts. The latter authors did not report P. hexastylos in their research but rather introduced only C. testudinaria as the epibiont barnacle of the examined turtles. We believe that this might be due to the lack of accurate identification of barnacle species which resulted in the taxonomic assignment of all individuals to C. testudinaria. We suggest that, in addition to the hard parts (carapace and plastron), soft parts of sea turtles should also be considered when assessing distribution and abundance of epibionts. Recently, Lim et al. (2020) examined different body parts of hawksbill turtles in Mabul Island (southeastern Sabah, Malaysia). They only examined barnacles larger than 5 mm on the carapace, plastron, and head of the turtles and concluded that C. testudinaria mainly settled on the plastron (94.6%) and just a few individuals tended to dwell on the carapace (1.4%) and head (4%). We also found a relatively similar pattern (but with different data values) in the settlement of C. testudinaria, with more individuals on the plastron (51%) compared to carapace and head (37 and 2%, respectively). The difference in data values may be a result of differences in the local barnacle larval supply, migratory behavior of turtles, and possibly barnacle removal by local people in some areas.
As a complementary study, these baseline data on turtle epibionts might be highly beneficial for future directions in adopting proper management strategies and making effective conservation decisions for these threatened species. In the face of climate change, the data are highly relevant considering the naturally harsh environment of the Persian Gulf. Furthermore, conducting such qualitative and quantitative assessments as regular monitoring studies can be used to track potential ecological changes in the Gulf. The epibiont assemblages of the two examined nesting turtle rookeries were significantly different, as revealed in this study, and may necessitate separate conservation approaches for the two populations. We encourage assessing epibionts of the other common turtle species in the region, the green turtle, to provide a clearer picture of sea turtle epibionts in the Persian Gulf and to better understand sea turtle habitat use and behavior in the region.
Data Availability Statement
The original contributions presented in the study are included in the article/Supplementary Materials, further inquiries can be directed to the corresponding authors.
Ethics Statement
Ethical review and approval was not required for the animal study because we did the sampling under the Environmental Protection Organization role and received help from their local expert while sampling. As we have done this kind of sampling several times (and have published them), we used totally non-invasive protocols.
Author Contributions
JL: conceptualization, methodology, sampling, analyses, and writing the first draft. AN: conceptualization, methodology, analyses, supervision, writing, review, and editing. BK: conceptualization, methodology, and supervision. MR-A: conceptualization, methodology, sampling, supervision, writing, review, and editing. All authors contributed to the article and approved the submitted version.
Funding
This study was financially supported by Iran National Science Foundation (INSF) (Grant Number: 97014616).
Conflict of Interest
The authors declare that the research was conducted in the absence of any commercial or financial relationships that could be construed as a potential conflict of interest.
The handling editor SD and author AN declare a shared professional partnership at the time of review. This collaboration was ongoing during the review process.
Publisher's Note
All claims expressed in this article are solely those of the authors and do not necessarily represent those of their affiliated organizations, or those of the publisher, the editors and the reviewers. Any product that may be evaluated in this article, or claim that may be made by its manufacturer, is not guaranteed or endorsed by the publisher.
Acknowledgments
We would like to thank the Department of Environment of Qeshm Free Area Organization (QDOE of QFAO) and Iran's Department of Environment (DOE) – Bushehr Office, for providing logistical help. We greatly appreciate Dr. Mohammad Javidkar, Dr. Koen Van Waerebeek and Dr. Jeffrey A. Seminoff for editing the English text. We also thank all members of Naturan Khamis NGO located at the Shibderaz study site, Qeshm Island, Iran, for their collaboration. Finally, we appreciate two reviewers for their constructive comments.
Supplementary Material
The Supplementary Material for this article can be found online at: https://www.frontiersin.org/articles/10.3389/fevo.2021.690022/full#supplementary-material
References
Abarca, N., Jahn, R., Zimmermann, J., and Enke, N. (2014). Does the cosmopolitan diatom Gomphonema parvulum (Kützing) Kützing have a biogeography? PLoS ONE 9:e86885. doi: 10.1371/journal.pone.0086885
Al-Yamani, F. Y., Polikarpov, I., Al-Ghunaim, A., and Mikhaylova, T. (2014). Field Guide of Marine Macroalgae (Chlorophyta, Rhodophyta, Phaeophyceae) of Kuwait. Kuwait: Kuwait Instiute for Scientific Research.
Anderson, M., Gorley, R. N., and Clarke, K. R. (2008). PERMANOVA + for PRIMER: Guide to Software and Statistical Methods, Vol. 1. Plymouth, 218.
Azari, M., Farjad, Y., Nasrolahi, A., De Stefano, M., Ehsanpour, M., Dobrestov, S., et al. (2020). Diatoms on sea turtles and floating debris in the Persian Gulf (Western Asia). Phycologia 59, 292–304. doi: 10.1080/00318884.2020.1752533
Aznar, F. J., Badillo, F. J., Mateu, P., and Raga, J. A. (2010). Balaenophilus manatorum (Ortíz, Lalana and Torres, 1992) (Copepoda: Harpacticoida) from loggerhead sea turtles, Caretta caretta, from Japan and the Western Mediterranean: amended description and geographical comparison. J. Parasitol. 96, 299–307. doi: 10.1645/GE-2246.1
Caine, E. A. (1986). Carapace epibionts of nesting loggerhead sea turtles: atlantic coast of U.S.A. J. Exp. Mar. Biol. Ecol. 95, 15–26. doi: 10.1016/0022-0981(86)90084-5
Casale, P., D'Addario, M., Freggi, D., and Argano, R. (2012). Barnacles (Cirripedia, Thoracica) and associated epibionts from sea turtles in the central Mediterranean. Crustaceana 85, 533–549. doi: 10.1163/156854012X634393
Casale, P., Freggi, D., Basso, R., and Argano, R. (2004). Epibiotic barnacles and crabs as indicators of Caretta caretta distribution and movements in the Mediterranean Sea. J. Mar. Biol. Assoc. United Kingdom 84, 1005–1006. doi: 10.1017/S0025315404010318h
Chan, B. K. K., Prabowo, R. E., and Lee, K. S. (2009). Crustacean Fauna of Taiwan: Barnacles, Volume I - Cirripedia: Thoracica Excluding the Pyrgomatidae and Acastinae. Keelung: National Taiwan Ocean University.
Cheang, C. C., Tsang, L. M., Chu, K. H., Cheng, I. J., and Chan, B. K. K. (2013). Host-specific phenotypic plasticity of the turtle barnacle Chelonibia testudinaria: a widespread generalist rather than a specialist. PLoS ONE 8:e57592. doi: 10.1371/journal.pone.0057592
Cheng, I. J., Wang, H. Y., Hsieh, W. Y., and Chan, Y. T. (2019). Twenty-three years of sea turtle stranding/bycatch research in Taiwan. Zool. Stud. 58, 1–14. doi: 10.6620/ZS.2019.58-44
Corrêa, G. V. V., Ingels, J., Valdes, Y. V., Fonsêca-Genevois, V. G., Farrapeira, C. M. R., and Santos, G. A. P. (2013). Diversity and composition of macro- and meiofaunal carapace epibionts of the hawksbill sea turtle (Eretmochelys imbricata Linnaeus, 1822) in Atlantic waters. Mar. Biodivers. 44, 391–401. doi: 10.1007/s12526-013-0189-9
Domènech, F., Badillo, F. J., Tomás, J., Raga, J. A., and Aznar, F. J. (2015). Epibiont communities of loggerhead marine turtles (Caretta caretta) in the western Mediterranean: influence of geographic and ecological factors. J. Mar. Biol. Assoc. United Kingdom 95, 851–861. doi: 10.1017/S0025315414001520
Domènech, F., Tomas, J., Crespo-picazo, L., Garcia-Parraga, D., Raga, J. A., and Aznar, F. J. (2017). To swim or not to swim: potential transmission of Balaenophilus manatorum (Copepoda: Harpacticoida) in marine turtles. PLoS ONE 12:e170789. doi: 10.1371/journal.pone.0170789
El-Semary, N. A. (2016). Diatoms as bioindicators of littoral zone: A case study. Bangladesh J. Bot. 45, 1113–1121.
Ewers-Saucedo, C., Arendt, M. D., Wares, J. P., and Rittschof, D. (2015). Growth, mortality, and mating group size of an androdioecious barnacle: implications for the evolution of dwarf males. J. Crust. Biol. 35, 166–176. doi: 10.1163/1937240X-00002318
Farrapeira, C. M. R. (2010). Shallow water Cirripedia of the northeastern coast of Brazil: the impact of life history and invasion on biogeography. J. Exp. Mar. Bio. Ecol. 392, 210–219. doi: 10.1016/j.jembe.2010.04.021
Frazier, J., Margarttoulis, D., Muldoon, K., Potter, C. W., Rosewater, J., Ruckdeschel, C., et al. (1985). Epizoan communities on marine turtles: I. Bivalve and Gastropod mollusks. Mar. Ecol. 6, 127–140. doi: 10.1111/j.1439-0485.1985.tb00134.x
Frick, M. G., and Pfaller, J. B. (2013). “15 sea turtle epibiosis,” in The Biology of Sea Turtles, eds J. Wyneken, K. J. Lohmann, and J. A. Musick (Boca Raton, FL: CRC Press), 399–426.
Frick, M. G., Zardus, J. D., Ross, A., Senko, J., Montano-Valdez, D., Bucio-Pacheco, M., et al. (2011). Novel records and observations of the barnacle Stephanolepas muricata (Cirripedia: Balanomorpha: Coronuloidea); including a case for chemical mediation in turtle and whale barnacles. J. Nat. Hist. 45, 629–640. doi: 10.1080/00222933.2010.534563
Fuller, W. J., Broderick, A. C., Enever, R., Thorne, P., and Godley, B. J. (2010). Motile homes: a comparison of the spatial distribution of epibiont communities on Mediterranean sea turtles. J. Nat. Hist. 44, 1743–1753. doi: 10.1080/00222931003624820
George, D., and Mallery, P. (2019). IBM SPSS Statistics 26 Step by Step: A Simple Guide and Reference. New York, NY: Routledge.
George, R. H. (1997). “Health problems and disease of sea turtles,” in The Biology of the Sea Turtles, eds P. L. Lutz and J. A. Musick (Boca Raton, FL: CRC Press), 363–385.
German, C. R., and Elderfield, H. (1990). Rare earth elements in the NW Indian Ocean. Geochim. Cosmochim. Acta 54, 1929–1940. doi: 10.1016/0016-7037(90)90262-J
Gharanjik, B. M., and Rohani Ghadikolaei, K. (2009). Atlas of the sea algae of the Persian Gulf and Oman sea coasts. Iran. Fish. Res. Organ. 47–77.
Greenblatt, R. J., Work, T. M., Balazs, G. H., Sutton, C. A., Casey, R. N., and Casey, J. W. (2004). The Ozobranchus leech is a candidate mechanical vector for the fibropapilloma-associated turtle herpesvirus found latently infecting skin tumors on Hawaiian green turtles (Chelonia mydas). Virology 321, 101–110. doi: 10.1016/j.virol.2003.12.026
Guerra-García, J. M., Ganesh, T., Jaikumar, M., and Raman, A. V. (2010). Caprellids (Crustacea: Amphipoda) from India. Helgol. Mar. Res. 64, 297–310. doi: 10.1007/s10152-009-0183-6
Habibi Motlagh, S., Nasrolahi, A., and Rezaie-Atagholipour, M. (2020). Species assemblage and distribution of turtle barnacles (Cirripedia: Coronuloidea) on foraging green sea turtles (Chelonia mydas) in the Persian Gulf. J. Nat. Hist. 54, 2113–2123. doi: 10.1080/00222933.2020.1837276
Harder, T. (2008). “Marine epibiosis: concepts, ecological consequences and host defence,” in Marine and Industrial Biofouling, eds H.-C. Flemming, P. S. Murthy, R. Venkatesan, and K. Cooksey (Berlin; Heidelberg: Springer), 219–231.
Hayashi, R. (2012). Atlas of the barnacles on marine vertebrates in Japanese waters including taxonomic review of superfamily Coronuloidea (Cirripedia: Thoracica). J. Mar. Biol. Assoc. United Kingdom 92, 107–127. doi: 10.1017/S0025315411000737
Hayashi, R. (2013). A checklist of turtle and whale barnacles (Cirripedia: Thoracica: Coronuloidea). J. Mar. Biol. Assoc. United Kingdom 93, 143–182. doi: 10.1017/S0025315412000847
Hayashi, R., and Tsuji, K. (2008). Spatial distribution of turtle barnacles on the green sea turtle, Chelonia mydas. Ecol. Res. 23, 121–125. doi: 10.1007/s11284-007-0349-0
Hays, G. C., and Hawkes, L. A. (2018). Satellite tracking sea turtles: opportunities and challenges to address key questions. Front. Mar. Sci. 5:432. doi: 10.3389/fmars.2018.00432
Holbourn, A., Henderson, A. S., and MacLeod, N. (2013). Atlas of Benthic Foraminifera. London: John Wiley & Sons.
Ingels, J., Valdes, Y., Pontes, L. P., Silva, A. C., Neres, P. F., Corrêa, G. V. V., et al. (2020). meiofauna life on loggerhead sea turtles-diversely structured abundance and biodiversity hotspots that challenge the meiofauna paradox. Diversity 12, 203–223. doi: 10.3390/d12050203
Jean, C., Ciccione, S., Ballorain, K., Georges, J. Y., and Bourjea, J. (2010). Ultralight aircraft surveys reveal marine turtle population increases along the west coast of Reunion Island. Oryx 44, 223–229. doi: 10.1017/S003060530999072X
Lazo-Wasem, E. A., Pinou, T., de Niz, A. P., and Feuerstein, A. (2011). Epibionts associated with the nesting marine turtles Lepidochelys olivacea and Chelonia mydas in Jalisco, Mexico: a review and field guide. Bull. Peabody Museum Nat. Hist. 52, 221–240. doi: 10.3374/014.052.0203
Lim, K. K., Syed Hussein, M. A., and Palaniappan, P. (2020). Abundance, placement and sexual identity of the epizoic barnacle Chelonibia testudinaria relative to the size and species of host turtles in Mabul Island, Malaysia. J. Mar. Biol. Assoc. United Kingdom 100, 1299–1309. doi: 10.1017/S0025315420001198
Logan, P., and Morreale, S. J. (1994). Hydrodynamic Drag Characteristics of Juvenile. L. kempii, C. mydas (Jekyll Island), 205–208.
Loghmani-Devin, M., and Sadeghi, P. (2010). Barnacles on hawksbill sea turtles, Eretmochelys imbricata, in Hormoz Island, Iran. Zool. Middle East 45–48. doi: 10.1080/09397140.2010.10638388
Longhurst, A., Sathyendranath, S., Platt, T., and Caverhill, C. (1995). An estimate of global primary production in the ocean from satellite radiometer data. J. Plankton Res. 17, 1245–1271. doi: 10.1093/plankt/17.6.1245
Majewska, R., de Vijver, B., Van Nasrolahi, A., Ehsanpour, M., Afkhami, M., Bolaños, F., et al. (2017). Shared epizoic taxa and differences in diatom community structure between green turtles (Chelonia mydas) from distant habitats. Microb. Ecol. 74, 969–978. doi: 10.1007/s00248-017-0987-x
Majewska, R., Santoro, M., Bolaños, F., Chaves, G., and De Stefano, M. (2015). Diatoms and other epibionts associated with Olive Ridley (Lepidochelys olivacea) sea turtles from the Pacific Coast of Costa Rica. PLoS ONE 10:e130351. doi: 10.1371/journal.pone.0130351
Marshall, C. D., Cullen, J. A., Al-Ansi, M., Hamza, S., and Abdel-Moati, M. A. R. (2020). Environmental drivers of habitat use by hawksbill turtles (Eretmochelys imbricata) in the Arabian Gulf (Qatar). Front. Mar. Sci. 7:549575. doi: 10.3389/fmars.2020.549575
Martin, J. W., Olesen, J., Høeg, J. T., and Høeg, J. (eds). (2014). Atlas of Crustacean Larvae. Baltimore, MD: Johns Hopkins University Press.
Meylan, A. B., and Donnelly, M. (1999). Status justification for listing the hawksbill turtle (Eretmochelys imbricata) as critically endangered on the 1996 IUCN Red List of Threatened Animals. Chelonian Conserv. Biol. 3, 200–224.
Nájera-Hillman, E., Bass, J. B., and Buckham, S. (2012). Distribution patterns of the barnacle, Chelonibia testudinaria, on juvenile green turtles (Chelonia mydas) in Bahia Magdalena, Mexico. Rev. Mex. Biodivers. 83, 1171–1179. doi: 10.22201/ib.20078706e.2012.4.1272
Nasrolahi, A., Pansch, C., Lenz, M., and Wahl, M. (2013). Temperature and salinity interactively impact early juvenile development: a bottleneck in barnacle ontogeny. Mar. Biol. 160, 1109–1117. doi: 10.1007/s00227-012-2162-8
Nolte, C. R., Pfaff, M. C., de Lecea, A. M., le Gouvello, D., and Nel, R. (2020). Stable isotopes and epibiont communities reveal foraging habitats of nesting loggerhead turtles in the South West Indian Ocean. Mar. Biol. 167, 1–12. doi: 10.1007/s00227-020-03767-x
Pfaller, J. B., Alfaro-Shigueto, J., Balazs, G. H., Ishihara, T., Kopitsky, K., Mangel, J. C., et al. (2014). Hitchhikers reveal cryptic host behavior: new insights from the association between Planes major and sea turtles in the Pacific Ocean. Mar. Biol. 161, 2167–2178. doi: 10.1007/s00227-014-2498-3
Pfaller, J. B., Bjorndal, K. A., Reich, K. J., Williams, K. L., and Frick, M. G. (2008a). Distribution patterns of epibionts on the carapace of loggerhead turtles, Caretta caretta. Mar. Biodivers. Rec. 1, 1–4. doi: 10.1017/S1755267206003812
Pfaller, J. B., Frick, M. G., Reich, K. J., Williams, K. L., and Bjorndal, K. A. (2008b). Carapace epibionts of loggerhead turtles (Caretta caretta) nesting at Canaveral National Seashore, Florida. J. Nat. Hist. 42, 1095–1102. doi: 10.1080/00222930701877565
Pilcher, N. J., Antonopoulou, M., Perry, L., Abdel-Moati, M. A., Al Abdessalaam, T. Z., Albeldawi, M., et al. (2014). Identification of important sea turtle areas (ITAs) for hawksbill turtles in the Arabian region. J. Exp. Mar. Bio. Ecol. 460, 89–99. doi: 10.1016/j.jembe.2014.06.009
Pilcher, N. J., Rodriguez-Zarate, C. J., Antonopoulou, M. A., Mateos-Molina, D., Das, H. S., and Bugla, I. A. (2020). Combining laparoscopy and satellite tracking: successful round-trip tracking of female green turtles from feeding areas to nesting grounds and back. Glob. Ecol. Conserv. 23:e01169. doi: 10.1016/j.gecco.2020.e01169
Rawson, P. D., Macnamee, R., Frick, M. G., and Williams, K. L. (2003). Phylogeography of the coronulid barnacle, Chelonibia testudinaria, from loggerhead sea turtles, Caretta caretta. Mol. Ecol. 12, 2697–2706. doi: 10.1046/j.1365-294X.2003.01940.x
Razaghian, H., Shams, B., and Askari, M. (2019). Distribution patterns of epibiotic barnacles on the hawksbill turtle, Eretmochelys imbricata, nesting in Iran. Reg. Stud. Mar. Sci. 27, 100527. doi: 10.1016/j.rsma.2019.100527
Rees, A. F., Alfaro-Shigueto, J., Barata, P. C. R., Bjorndal, K. A., Bolten, A. B., Bourjea, J., et al. (2016). Are we working towards global research priorities for management and conservation of sea turtles? Endanger. Species Res. 31, 337–382. doi: 10.3354/esr00801
Reynolds, R. M. (1993). Physical oceanography of the Gulf, Strait of Hormuz, and the Gulf of Oman-results from the Mt Mitchell expedition. Mar. Pollut. Bull. 27, 35–59. doi: 10.1016/0025-326X(93)90007-7
Riegl, B. M., and Purkis, S. J. (2012). “Coral reefs of the Gulf: adaptation to climatic extremes in the world's hottest sea,” in Coral Reefs of the Gulf, eds B. Riegl and S. J. Purkis (Dordrecht: Springer Netherlands), 1–4. doi: 10.1007/978-94-007-3008-3
Rivera, S. F., Vasselon, V., Ballorain, K., Carpentier, A., Wetzel, C. E., Ector, L., et al. (2018). DNA metabarcoding and microscopic analyses of sea turtles biofilms: complementary to understand turtle behavior. PLoS ONE 13:e195770. doi: 10.1371/journal.pone.0195770
Robinson, D. P., Jabado, R. W., Rohner, C. A., Pierce, S. J., Hyland, P., and Baverstock, W. R. (2017). Satellite tagging of rehabilitated green sea turtles Chelonia mydas from the United Arab Emirates, including the longest tracked journey for the species. PLoS ONE 12:e184286. doi: 10.1371/journal.pone.0184286
Robinson, N. J., Lazo-Wasem, E. A., Paladino, F. V., Zardus, J. D., and Pinou, T. (2017). Assortative epibiosis of leatherback, olive ridley and green sea turtles in the eastern tropical Pacific. J. Mar. Biol. Assoc. United Kingdom 97, 1–8. doi: 10.1017/S0025315416000734
Robinson, N. J., Lazo-Wasem, E. M., Butler, B. O., Lazo-wasem, E. A., Zardus, J. D., and Pinou, T. (2019). Spatial distribution of epibionts on olive ridley sea turtles at Playa Ostional, Costa Rica. PLoS ONE 14:e218838. doi: 10.1371/journal.pone.0218838
Robinson, N. J., Majewska, R., Lazo-Wasem, E. A., Nel, R., Paladino, F. V., Rojas, L., et al. (2016). Epibiotic diatoms are universally present on all sea turtle species. PLoS ONE 11:e157011. doi: 10.1371/journal.pone.0157011
Roos, D., Pelletier, D., Ciccione, S., Taquet, M., and Hughes, G. (2005). Aerial and snorkelling census techniques for estimating green turtle abundance on foraging areas: a pilot study in Mayotte Island (Indian Ocean). Aquat. Living Resour. 18, 193–198. doi: 10.1051/alr:2005021
Sezgin, M., Ateş, A. S., Katagan, T., Bakir, K., and Özdilek, S. Y. (2009). Notes on amphipods Caprella andreae Mayer, 1890 and Podocerus chelonophilus (Chevreux & Guerne, 1888) collected from the loggerhead sea turtle, Caretta caretta, off the Mediterranean and the Aegean coasts of Turkey. Turkish J. Zool. 33, 433–437. doi: 10.3906/zoo-0807-3
Shahdadi, A., Sari, A., and Naderloo, R. (2014). A checklist of the barnacles (Crustacea: Cirripedia: Thoracica) of the Persian Gulf and Gulf of Oman with nine new records. Zootaxa 3784, 201–223. doi: 10.11646/zootaxa.3784.3.1
Sheppard, C., Al-Husiani, M., Al-Jamali, F., Al-Yamani, F., Baldwin, R., Bishop, J., et al. (2010). The Gulf: a young sea in decline. Mar. Pollut. Bull. 60, 13–38. doi: 10.1016/j.marpolbul.2009.10.017
Silver-Gorges, I., Ingels, J., dos Santos, G. A. P., Valdes, Y., Pontes, L. P., Silva, A. C., et al. (2021). Epibionts reflect spatial and foraging ecology of gulf of mexico loggerhead turtles (Caretta caretta). Front. Ecol. Evol. 9:696412. doi: 10.3389/fevo.2021.696412
Sloan, K., Zardus, J. D., and Jones, M. L. (2014). Substratum fidelity and early growth in Chelonibia testudinaria, a turtle barnacle especially common on debilitated loggerhead (Caretta caretta) sea turtles. Bull. Mar. Sci. 90, 581–597. doi: 10.5343/bms.2013.1033
Sönmez, B. (2018). Sixteen year (2002-2017) record of sea turtle strandings on Samandag Beach, the eastern Mediterranean coast of Turkey. Zool. Stud. 57, 1–15. doi: 10.6620/ZS.2018.57-53
Taylor, J. C., Harding, W. R., and Archibald, C. G. M. (2007). An Illustrated Guide to Some Common Diatom Species From South Africa. Pretoria: Water Research Commission.
Ten, S., Pascual, L., Pérez-Gabaldón, M. I., Tomás, J., Domènech, F., and Aznar, F. J. (2019). Epibiotic barnacles of sea turtles as indicators of habitat use and fishery interactions: an analysis of juvenile loggerhead sea turtles, Caretta caretta, in the western Mediterranean. Ecol. Indic. 107:105672. doi: 10.1016/j.ecolind.2019.105672
Torres-Pratts, H., Schärer, M. T., and Schizas, N. V. (2009). Genetic diversity of Chelonibia caretta, commensal barnacles of the endangered hawksbill sea turtle Eretmochelys imbricata from the Caribbean (Puerto Rico). J. Mar. Biol. Assoc. United Kingdom 89, 719–725. doi: 10.1017/S0025315409000046
van de Vijver, B., Robert, K., Majewska, R., Frankovich, T. A., Panagopoulou, A., and Bosak, S. (2020). Geographical variation in the diatom communities associated with loggerhead sea turtles (Caretta caretta). PLoS ONE 15:e236513. doi: 10.1371/journal.pone.0236513
Wahl, M. (1989). Marine epibiosis. I. Fouling and antifouling: Some basic aspects. Mar. Ecol. Prog. Ser. 58, 175–189. doi: 10.3354/meps058175
Wahl, M., and Mark, O. (1999). The predominantly facultative nature of epibiosis: experimental and observational evidence. Mar. Ecol. Prog. Ser. 187, 59–66. doi: 10.3354/meps187059
Wang, Y. F., Li, T. H., Jiang, Y. F., Chi, C. H., Cheng, I. J., Cheng, C. H., et al. (2020). Light microscopic and ultrastructural characteristics of heterophil toxicity and leftshifting in green sea turtles (Chelonia mydas) from Taiwan. Zool. Stud. 59, 1–12. doi: 10.6620/ZS.2020.59-52
Wyneken, J. (1997). Sea turtle locomotion: Mechanisms, behavior, and energetics. Biol. Sea Turtles 1, 165–198.
Keywords: barnacles, epibionts, environmental extremes, Strait of Hormuz, sea turtles
Citation: Loghmannia J, Nasrolahi A, Rezaie-Atagholipour M and Kiabi BH (2021) Epibiont Assemblages on Nesting Hawksbill Turtles Show Site-Specificity in the Persian Gulf. Front. Ecol. Evol. 9:690022. doi: 10.3389/fevo.2021.690022
Received: 01 April 2021; Accepted: 31 August 2021;
Published: 08 October 2021.
Edited by:
Sergey Dobretsov, Sultan Qaboos University, OmanReviewed by:
Benny K. K. Chan, Academia Sinica, TaiwanAdnan Shahdadi, University of Hormozgan, Iran
Daniel Rittschof, Nicholas School of the Environment, United States
Copyright © 2021 Loghmannia, Nasrolahi, Rezaie-Atagholipour and Kiabi. This is an open-access article distributed under the terms of the Creative Commons Attribution License (CC BY). The use, distribution or reproduction in other forums is permitted, provided the original author(s) and the copyright owner(s) are credited and that the original publication in this journal is cited, in accordance with accepted academic practice. No use, distribution or reproduction is permitted which does not comply with these terms.
*Correspondence: Ali Nasrolahi, YV9uYXNyb2xhaGlAc2J1LmFjLmly