- 1School of Biological, Earth and Environmental Sciences, University College Cork, Cork, Ireland
- 2Marine Institute, Newport, Co. Mayo, Ireland
- 3The Environmental Research Institute, University College Cork, Cork, Ireland
Migratory species must cope with different parasite communities in different environments, but little is known about the ecophysiological effects of parasites on migratory performance. Some species/strains of acanthocephalan parasites in the genus Pomphorhynchus use anadromous salmonids as preferred definitive hosts, perforating the intestines, destroying mucosa and inducing inflammation–all of which might affect osmoregulatory function during transition between freshwater and marine environments. We used genetic barcoding to identify acanthocephalans in the intestines of wild Irish Atlantic salmon (Salmo salar L.) smolts as being the recently taxonomically resurrected species Pomphorhynchus tereticollis. We then investigated whether natural infection intensities of this parasite were associated with reduced osmoregulatory performance, as measured by plasma chloride concentrations, or potentially elevated stress, as measured by blood glucose, of hosts in freshwater or saltwater environments (24 or 72 h in ∼26PPT salt water, reflecting salinities of coastal waters through which smolts migrate). Although infection prevalence was high amongst sampled smolts, no associations were found within or across treatment groups between parasite abundance and plasma chloride concentrations or blood glucose levels. We found no intestinal perforations that would indicate P. tereticollis had recently vacated the intestines of smolts in either of the saltwater groups. Exploratory sampling in the 2 years preceding the experiment indicated that parasite prevalence and abundance are consistently high and comparable to the experimental individuals. Collectively, these results indicate that naturally occurring abundances of P. tereticollis do not reduce osmoregulatory function or affect blood glucose content in fresh water or within 72 h of entering coastal waters, although delayed pathologies affecting marine survival may occur. Future consideration of ecophysiological interactions between anadromous fish hosts and their parasites across different osmotic environments should provide general insights into coevolution between migratory hosts and their parasites.
Introduction
Migration has evolved in a diverse array of animal taxa as a strategy for coping with, or exploiting, spatiotemporal environmental variation (Dingle, 2014). Physiological processes (e.g., metabolic and neuroendocrine pathways) drive how animals translate environmental cues into movement decisions (Goossens et al., 2020) and also mediate fitness costs of these decisions (Alerstam et al., 2003; Brownscombe et al., 2017) and consequent life-history trade-offs (Ricklefs and Wikelski, 2002). While the performance of migratory aquatic species has been clearly linked to a range of abiotic drivers such as temperature (e.g., Crossin et al., 2008; Gilbert and Tierney, 2018), oxygen limitation (e.g., Rosa and Seibel, 2010), water flow (e.g., Swanson et al., 2004), water chemistry (e.g., Borges et al., 2019), and pollution (e.g., Seewagen, 2020), the role of biotic factors in the ecophysiology of animal migration is arguably less well studied. In particular, parasites and pathogens have received relatively little attention, but are critical components of any ecosystem and are likely to exert a range of physiologically mediated influences on migration decisions and fitness outcomes (Piersma, 1997; Altizer et al., 2000; Gylfe et al., 2000; Norris and Evans, 2000; Møller and Szép, 2011).
Salmonid fishes (Family Salmonidae) are a group of broad ecological, economic, and cultural importance that exhibit a diversity of migratory strategies, ranging from residency, to potamodromy (migrations within freshwater) to anadromy (migration to sea for growth followed by return to freshwater for spawning). Their complex life cycles and amenability to experimental work and tracking studies has made salmonids the focus of much research in ecophysiology (see reviews by: McCormick et al., 1998; Poole et al., 2003; Hinch et al., 2005; Cooke et al., 2008, 2012; McCormick et al., 2009; Groot, 2010; Björnsson et al., 2011; Eliason and Farrell, 2016). The life histories of anadromous species such as Atlantic salmon (Salmo salar L.) necessitate the regulation of internal osmotic balance in hypoosmotic (freshwater) and hyperosmotic (saltwater) environments. In fresh water, osmoregulation requires excess water, which is passively absorbed through osmosis across the gills and skin, to be excreted as dilute urine (Genz et al., 2011). In contrast, osmoregulation in a marine environment requires salmon to continuously drink salt water and actively uptake H20 through the intestinal epithelium into the body, while limiting intestinal absorption of ions in order to mitigate diffusive water losses through the gills and skin (Grosell, 2007; Whittamore, 2012).
The initial period of acclimation to the marine environment necessitates significant changes to the internal physiology of salmon smolts (Stefansson et al., 2012) and provides an acute physiological challenge (Handeland et al., 2014). Plasma cortisol levels rise during the parr-smolt transformation, and this natural stress response is thought to benefit smolts by mobilizing energy reserves and increasing saltwater tolerance (Bisbal and Specker, 1991; Strand and Finstad, 2007). However, further sources of acute or chronic stress can greatly impair osmoregulatory ability in salmonid smolts (Redding and Schreck, 1983; Iversen et al., 1998) as observed with infestations of ectoparasitic sea lice (Lepeophtheirus salmonis) (Poole et al., 2000) or Gyrodactylus salaris (Bakke and Harris, 1998). Parasite-induced damage to organs involved in osmoregulation such as the skin, gills or intestines can also directly impact the ability of salmonids to maintain osmotic homeostasis in salt water, leading to disruption of physiological processes, elevated stress, and ultimately mortality (Dawson et al., 1998; Wells et al., 2006; Finstad et al., 2012; Hvas et al., 2017). Through these pathological effects, increased sea lice infestations associated with fish farming are regarded as a significant factor contributing to declines in the marine survival of many Atlantic salmon and sea trout (anadromous Salmo trutta L.) stocks in recent decades (Poole et al., 2007; Krkošek et al., 2013; Thorstad et al., 2015; Shephard and Gargan, 2017).
Pomphorhynchus tereticollis is an acanthocephalan endoparasite of various freshwater and brackish fishes with a complex (heteroxenous) life cycle that requires infection of both intermediate and definitive host species. Previously regarded as a synonym for the phenotypically similar Pomphorhynchus laevis, recent genetic characterisation has led to the resurrection of P. tereticollis as a distinct species within the (former) P. laevis complex (Špakulová et al., 2011). Based on morphological and molecular examinations of new and archived material, recent studies provide convincing evidence that P. tereticollis is the only Pomphorhynchus species present in Ireland and Britain, and that all previous literature pertaining to P. laevis in this region represents misidentifications of P. tereticollis (Perrot-Minnot et al., 2018; Andreou et al., 2020; Tierney et al., 2020). To avoid confusion, we use the term P. laevis s.l. (sensu lato) when referring to older studies in which P. tereticollis may have been misidentified as P. laevis, or to more recent studies in which the taxonomy was uncertain. Such studies have shown that P. laevis s.l. infection rates of Irish brown trout peak in spring, coinciding with the annual smolt run (Molloy et al., 1995), and that parasite abundance and prevalence are highest in smolt-aged Atlantic salmon (i.e., 2+) (Fitzgerald and Mulcahy, 1983). Pippy (1969) found that the incidence of P. laevis s.l. in Atlantic salmon smolts in Ireland was 25 times higher than in Scotland, England and Wales. Thus anadromous salmonids in Ireland have a particularly high chance of entering salt water while infected by these acanthocephalan parasites.
Pomphorhynchus tereticollis uses gammarid species as intermediate hosts and trophic transmission to a definitive host requires the consumption of an infected gammarid by a suitable fish species (Perrot-Minnot et al., 2020). Upon consumption by a salmonid, P. tereticollis use their hooked proboscis to pierce all layers of the intestinal wall and anchor themselves in place. This process creates a perforation leading from the interior to the exterior of the intestinal wall, destroying intestinal mucosa, causing a localized inflammatory response and potentially altering the physiological performance of the intestine in controlling transepithelial ion transport (Wanstall et al., 1986, 1988; Dezfuli et al., 2002b, 2008). Previous studies have concluded that, despite causing such intestinal damage, infection with these acanthocephalans does not significantly reduce growth rates in salmonids and does not directly cause mortality of the host (Hine and Kennedy, 1974; Wanstall, 1984; Wanstall et al., 1986). However, these studies have focused on the impact of acanthocephalan infection on salmonids in fresh water where osmoregulation does not require equivalent control of transepithelial ion transport or active H20 uptake through the intestinal wall, as is necessary in saltwater environments.
Exposure to sub-optimal conditions or stressors in fresh water has been shown to reduce osmoregulatory performance and increase the susceptibility of salmonids to parasitic infection and associated mortality in the marine environment (Finstad et al., 2012, 2007). In the present study, we first used genetic barcoding techniques to confirm that Atlantic salmon smolts in our Irish study system are infected by P. tereticollis. We then investigated whether natural infection intensities of P. tereticollis in wild-caught smolts affect their osmoregulatory performance in saltwater or freshwater environments, and whether such osmoregulatory impacts might also be associated with potentially increased stress. We hypothesized that perforations made by P. tereticollis in the intestinal wall would allow uncontrolled ingress of water into the peritoneum, while parasite-induced damage to mucosa, and the associated inflammatory response, would further reduce the ability of salmonids to control ion uptake or water absorption through the intestines. Through these processes, P. tereticollis infection was predicted to compromise the osmoregulatory performance of Atlantic salmon in salt water (i.e., hypo-osmoregulation), leading to elevated stress and increased ion concentrations in the blood within marine environments. While we regarded a strong reduction in hyper-osmoregulatory performance as less likely, we predicted that pathological effects of P. tereticollis infection (including reduced control of transepithelial ion transport) could also lead to elevated stress and, potentially, reduced blood ion concentrations in freshwater environments.
We characterized P. tereticollis infection prevalences and intensities in wild smolts captured from the Burrishoole catchment, Co. Mayo, over a 3-year period and investigated whether infection patterns were associated with smolt size, sex or condition. Smolts captured on the third year were held in fresh (n = 66) or salt (n = 132) water prior to sampling and blood samples were extracted shortly after euthanasia. We then tested whether higher parasite abundance (higher numbers of P. tereticollis per individual) was associated with reduced osmoregulatory performance of smolts within each osmotic environment (fresh or salt water) as indicated by plasma chloride concentrations, which provide a direct measure of internal osmotic balance (Strand and Finstad, 2007; McCormick, 2012; Archer et al., 2019). We also measured blood glucose levels as one potential indicator of physiological stress (McGeer et al., 1991) that might accompany impaired osmoregulatory performance in fresh or salt water environments.
Materials and Methods
Exploratory Sampling
Wild Atlantic salmon smolts were captured for stock assessment purposes at the tidal limit of the Burrishoole river system (NW Ireland; 53° 55′ 13 N, 9° 35′03 W) in May 2016 (n = 136) and May 2017 (n = 39). The Burrishoole system is comprised of over 45 km of small rivers and streams that link two main freshwater lakes, Bunaveela Lough (46 ha) and Lough Feeagh (410 ha), and ultimately flow into Lough Furnace (141 ha), a brackish, partially tidal lake opening into Clew Bay (Matthews et al., 1997; Whelan et al., 1998). The majority (∼89%) of salmon from the Burrishoole catchment smoltify and migrate to sea as 2 year old fish (Fealy et al., 2014; de Eyto et al., 2016). Captured smolts were dissected and their digestive tracts inspected for the presence of acanthocephalan parasites. Attached and unattached acanthocephalans in each smolt were recorded and stored in ethanol for subsequent DNA barcoding work. Smolt weight (to 0.1 g) and fork length (to 1 mm) were recorded before dissection and sex was determined by inspection of gonads during removal of the digestive tract. Chi-square and Mann-Whitney U tests were used to investigate whether there was a significant relationship between acanthocephalan prevalence or infection intensity, respectively, and sex amongst the smolts sampled in 2016, 2017 or amongst the experimental 2018 samples.
Experimental Setup
On two occasions during 2018, emigrating wild smolts (mean fork length = 138.9 mm, SD = 9.5 mm, range = 121–168 mm) were captured at the Salmon Leap fish trap located at the confluence between the freshwater component of the Burrishoole river system and the saline environment of Lough Furnace and Clew Bay (de Eyto et al., 2020). On each occasion, captured smolts were transported <100 m to an indoor Marine Institute research facility where they were transferred in an ad hoc fashion to evenly populate four 500 L aerated experimental tanks. On the first capture occasion (02 May), 66 smolts were distributed evenly amongst four tanks that had each been filled with 300 L of fresh water (i.e., 16–17 smolts per tank). After 24 h all 66 smolts were terminally sampled (see next section), at which point the experiment finished for this freshwater treatment group (24FW) and the four tanks were emptied of water.
On the second capture occasion (05 May), 132 smolts were distributed evenly amongst the same four tanks, each now pre-filled again with 50 L of fresh water (i.e., 33 smolts per tank). During the 2 h after the 132 smolts were transferred, 300 L of locally sourced sea water were gradually added to each tank, raising the salinity in each tank to 26.1–26.3 PPT at a rate that reflects the natural salinity increase experienced by wild smolts moving from the Burrishoole system to coastal waters. Twenty-four hours after the salinity had reached this peak, 66 smolts (16–17 smolts per tank) were terminally sampled and this group then comprised the 24 h in saltwater (24SW) treatment group. The remaining 66 smolts were then terminally sampled 48 h later, i.e., after a total of 72 h in saltwater (72SW). Water temperatures ranged between 8.4 and 13.9°C and dissolved oxygen was maintained at >8.5 mg/L during all phases of the experiment. The tanks were covered throughout the experiment in order to reduce exposure to potential external sources of stress.
Experimental Sampling Procedure
At each sampling time (i.e., 24FW, 24SW, and 72SW), dip nets were used to transfer 16–17 smolts from each of the four tanks into a pH buffered solution of tricaine methanesulfonate (450 mg L–1) while minimizing disturbance to the remaining smolts. Smolts were monitored until opercular movement ceased and death was confirmed by severing the spinal cord with a scalpel (completing the killing of the animal in accordance with Annex IV of EU Directive 2010/63/EU and Irish Statutory Instrument 5432 of 2012). Blood samples were extracted from the caudal vein (along midline just posterior of the anal fin) with 1 ml 21G lithium-heparinised syringes (containing ∼6 USP units of lithium-heparin and providing ∼15 USP units per ml of blood) immediately after cervical dislocation and transferred to 1 ml Eppendorf© tubes which were stored on ice. Mean duration between dip netting and blood sampling was 9 min and 24 s (SD = 261 s, max = 1110 s, min = 121 s).
Sample Processing
A commercially available meter (FreeStyle Lite: Abbott) was used to take a single measurement of the blood glucose level (mmol/L) of each smolt within 1 min of sacrifice, requiring one drop of blood from the needle of each syringe. This meter has been shown to accurately measure glucose levels in teleosts (Eames et al., 2010). Each smolt was then weighed (to 0.1 g), measured (fork length “FL” to 1 mm), and a ∼2 mm2 clip of caudal tissue was stored in ethanol for genetic sex determination. The condition factor (Fulton’s K) for each smolt was then calculated by the following formula (Ricker, 1975):
where K is condition factor, W is smolt weight (g) and FL is fork length (cm).
Carcasses were placed in individual sealable plastic bags and stored on ice until dissection. All smolts were dissected within 8 h of mortality. An incision was made along the midventral line and the alimentary tract was removed after severing its junctures with the anus and the esophagus. The phenotypic sex of each smolt was determined by visual inspection of the gonads and in any case where the designation was uncertain genetic methods were used to verify sex (as per Finlay et al. (2020)).
Once removed from the body, the alimentary tract of each smolt was temporarily filled with water and pinched at each end to create water tight seals. The oesophageal end was then compressed to pressurize the internal water and the external wall was closely inspected for “pinprick” leaks that would indicate the presence of unplugged perforations left by previously attached acanthocephalans. The alimentary tract was then opened by mesial incision with a fine-point scissors and divided into four sections; (1) stomach (esophagus to pyloric caeca), (2) anterior intestine (33% of intestinal length from post-pyloric caeca to rectum); (3) intermediate intestine (middle 33% of intestine), and (4) posterior intestine (last 33% of intestine ending at anus). Each section was examined for the presence of parasites and the number of attached and unattached acanthocephalans in each section was recorded. On each sampling date, 30 acanthocephalans were examined under a microscope within 15 min of opening the digestive tract and their status as “alive” or “dead” was determined based on the presence or absence of observable movement in response to physical stimulus. A subset of acanthocephalans (n = 264) were also weighed in groups of 2–32 individuals (each group collected from a single smolt) and mean individual parasite weight per group and in total were calculated. Parasite abundance was measured as the number of acanthocephalans in an individual host, regardless of whether the host was infected or not. We calculated the prevalence of infection as the percentage of smolts containing acanthocephalans and infection intensity as the number of acanthocephalans in infected individuals.
Plasma was separated from all blood samples within 4 h of extraction by spinning in a centrifuge (ALC PK 421) at 3000 rpm for 10 min. Where possible, 0.07 ml of plasma was extracted from each sample (12 samples provided less than 0.07 ml of plasma) with an adjustable micropipette (Nichipet Ex) and stored in a 0.5 ml tube at −20°C for chloride analysis. Plasma chloride was measured by coulometric titration using a Jenway PCLM3 chloride meter (reproducibility ±1% or ±1 mmol/l for 100 μl sample at 100 mmol/l). Where plasma quantities were sufficient (98% of samples), chloride samples were tested in duplicate, and triplicates were run for any samples showing a difference greater than three units between the first two replicates. All blood assays were conducted within 3 weeks of freezing plasma.
Molecular Species Identification
Twenty-two of the attached acanthocephalan parasites, representing two randomly selected acanthocephalans from each of eleven randomly selected experimental smolts, were selected for molecular confirmation of species identity. DNA extraction was performed on entire specimens using the DNeasy® Blood and Tissue Kit (Qiagen), following instructions provided in the kit handbook. A 558 bp region of the mitochondrial DNA COI gene was amplified using the PT/PL-COI primers described in Tierney et al. (2020). PCR was performed in a 20 μl total volume consisting of 10 μl of 2× Plain Combi PP Master Mix (Top-Bio), 1 μM each of forward and reverse primer and 10–50 ng of DNA. PCR cycling conditions were as follows; an initial denaturation step of 3 min at 95°C was followed by 35 cycles of 94°C for 30 s 51°C for 30 s and 72°C for 60 s, with final extension step of 72°C for 5 min. Electrophoresis of PCR products was performed on 1% agarose and products were excised and purified using a QIAquickTM Gel Extraction Kit (QIAGEN). Sequencing was performed from both directions using the BigDye® Terminator v3.1 Cycle Sequencing Kit (Applied Biosystems). Sequencing reactions were purified using the EDTA-ethanol precipitation method described in the sequencing kit handbook and were run on an ABI3500XL DNA analyzer.
Ethics Statement
We adhered to the ASAB/ABS Guidelines for the Use of Animals in Research throughout this project. All actions relating to the capture and sampling of smolts as well as the manipulation of environmental salinity were carried out in accordance with S.I. No. 123/2014 Animal Health and Welfare (operations and procedures) Regulations 2014 and with approval of the Marine Institute animal welfare committee (MI Establishment Authorisation No: AE19121) and the Health Professionals Regulatory Authority (HPRA Classification Request Number: 066). Procedures for euthanasia were appropriate for salmonids (Popovic et al., 2012). Sampling was carried out by personnel with appropriate training and Individual Authorisations under Scientific Animal Protection Legislation (HPRA).
Statistical Analyses
We conducted all analyses using the statistical computing software R v3.6.1 (R Core Team, 2019). We specified separate generalized least squares models (GLS) using the gls function in the nlme package (Pinheiro et al., 2019) to investigate the extent to which variation in blood parameters (blood glucose and plasma chloride) was associated with variation in two continuous (acanthocephalan count, i.e., parasite abundance, and smolt condition factor, measured as Fulton’s K) and four categorical (treatment group, sex, operator, and tank) explanatory variables. Treatment group had three levels (24FW, 24SW, and 72SW) corresponding with the three sampling dates. Operator, with two levels designating the two operators who performed the blood sampling, was included to control for potential variation in response variables resulting from differences in sampling technique among personnel. Tank had four levels reflecting the four experimental water tanks and sex had two levels, male and female. GLS models were used in order to account for differences in the variance of each of the three response variables observed amongst the three treatment groups, i.e., to control for heteroscedasticity. Initial models included an interaction between parasite abundance and treatment group in order to test whether any effect of acanthocephalan infection on chloride or blood glucose depended on treatment group (i.e., whether parasite effects on these physiological parameters varied between hyper and hypo-osmotic environments, and whether effects increased with time spent in hyper-osmotic environments). Models excluding this interaction were then run in order to test whether parasite abundance was associated with variation in these blood parameters independently of treatment group. AIC values were used to compare models including and excluding the interaction between parasite abundance and treatment group.
We used the glmmTMB function (Brooks et al., 2017) to specify generalized linear models (GLMs) to explore the degree to which the variation in infection prevalence and individual infection intensity was associated with variation in the condition factor (Fulton’s K), length, weight and sex of smolts sampled in 2016, 2017, and 2018 (n = 312, the subset for which sex and size were both recorded). The individual infection intensity model included only smolts that were infected (n = 257). We used negative binomial models with log link functions to investigate individual infection intensity, in order to account for residual overdispersion in the data, and binomial models with logit link functions to investigate infection prevalence. Due to high collinearity between fork length and weight (R2 = 0.91), two models were specified for each response variable (i.e., infection prevalence and intensity), each including either fork length or weight, and AIC values were used to compare both models. As both the infection prevalence and infection intensity models containing fork length yielded marginally lower AIC values than the models containing weight (−0.4 and −0.6, respectively), only results from the models with fork-length are presented.
Prior to model fitting, collinearity between all continuous explanatory variables in each model was explored by Pearson’s R with the cor.test function in the stats package and associations between continuous and categorical explanatory variables were examined visually. Variance inflation factors (VIFs) were calculated for all fixed effects in each GLM with the check_collinearity function in the performance package in R (Lüdecke et al., 2019). We tested for heteroscedasticity and violations of linearity amongst residuals from the GLMs by plotting fitted values against simulated (scaled) residuals with the DHARMa package (Hartig, 2019). We tested for temporal autocorrelation with the acf function in the stats package. The qqnorm and plot functions were used to investigate residual distributions from GLS models. Chi-square tests were used to investigate whether there were significant differences in acanthocephalan prevalence or abundance amongst experimental treatment groups or amongst tanks within each treatment group.
Results
Molecular Identification of Acanthocephalan Parasite Species
All 22 acanthocephalan specimens examined were identified unambiguously as P. tereticollis, with 97–100% sequence match to voucher specimens. Sequence match with P. laevis was less than 90% in all cases.
Parasite Prevalence, Infection Intensity and Locations Within the Alimentary Tract
Acanthocephalan infection prevalences amongst smolts sampled in 2016, 2017, and 2018 were 74.2, 64.1, and 66.2%, respectively. Mean infection intensities amongst the 2016, 2017, and 2018 samples, respectively were 9.23, 7.28, and 6.9 acanthocephalans per infected smolt. Infection prevalence amongst male and female smolts, respectively was 76.2 and 75% in 2016 (χ2 = 0.77, p = 0.68), 93.8 and 40.9% in 2017 (χ2 = 11.82, p = 0.003), and 71.1 and 63.6% in 2018 (χ2 = 0.84, p = 0.358). Mean infection intensity per infected smolt amongst males and females, respectively was 12.8 and 4.9 in 2016 (Mann-Whitney U test: W = 1171, p = 0.044), 7.9 and 7.0 in 2017 (W = 53.5, p = 0.416) and 6.0 and 7.6 in 2018 (W = 2063, p = 0.942). Ten of the 198 smolts sampled in 2018 contained unattached acanthocephalans with nine of these smolts also containing attached acanthocephalans. A total of 899 attached and 15 unattached acanthocephalans were recorded amongst the 2018 experimental samples and the number of attached worms per smolt ranged from 1 to 42 (Figure 1).
Almost all (93.64%) attached acanthocephalans were in the intermediate (central 33%) section of the intestine while 3.11 and 3.25% were located in the anterior and posterior sections, respectively. No attached or unattached acanthocephalans were found in the esophagus, stomach or pyloric caeca. A few (n = 7) acanthocephalans were attached to the muscle along the inside wall of the peritoneal cavity, having presumably passed completely through the wall of the digestive tract (Figure 2A). The mean weight of individual attached worms was 3.1 mg (SD of mean individual weight per smolt = 1.5 mg, max = 7 mg, min = 1.5 mg). All worms from all treatment groups that were observed under a microscope directly after removal from the intestines were found to be alive. Infection prevalence in the 24FW, 24SW, and 72SW treatment groups were 63.1, 56.9, and 77.6%, respectively (χ2 = 6.7, p = 0.036). Mean acanthocephalan counts per smolt in the 24FW, 24SW, and 72SW treatment groups were 4.29, 3.48, and 5.88, respectively (χ2 = 40.5, p = 0.769). Acanthocephalan prevalence did not differ significantly amongst the four tanks in the 24FW (χ2 = 4.9, p = 0.183), 24SW (χ2 = 6.7, p = 0.084), or 72SW (χ2 = 0.8, p = 0.841) treatment groups. Acanthocephalan count did not differ significantly amongst the four tanks in the 24FW (χ2 = 53.2, p = 0.507), 24SW (χ2 = 43.9, p = 0.390), or 72SW (χ2 = 49.3, p = 0.657) treatment groups.
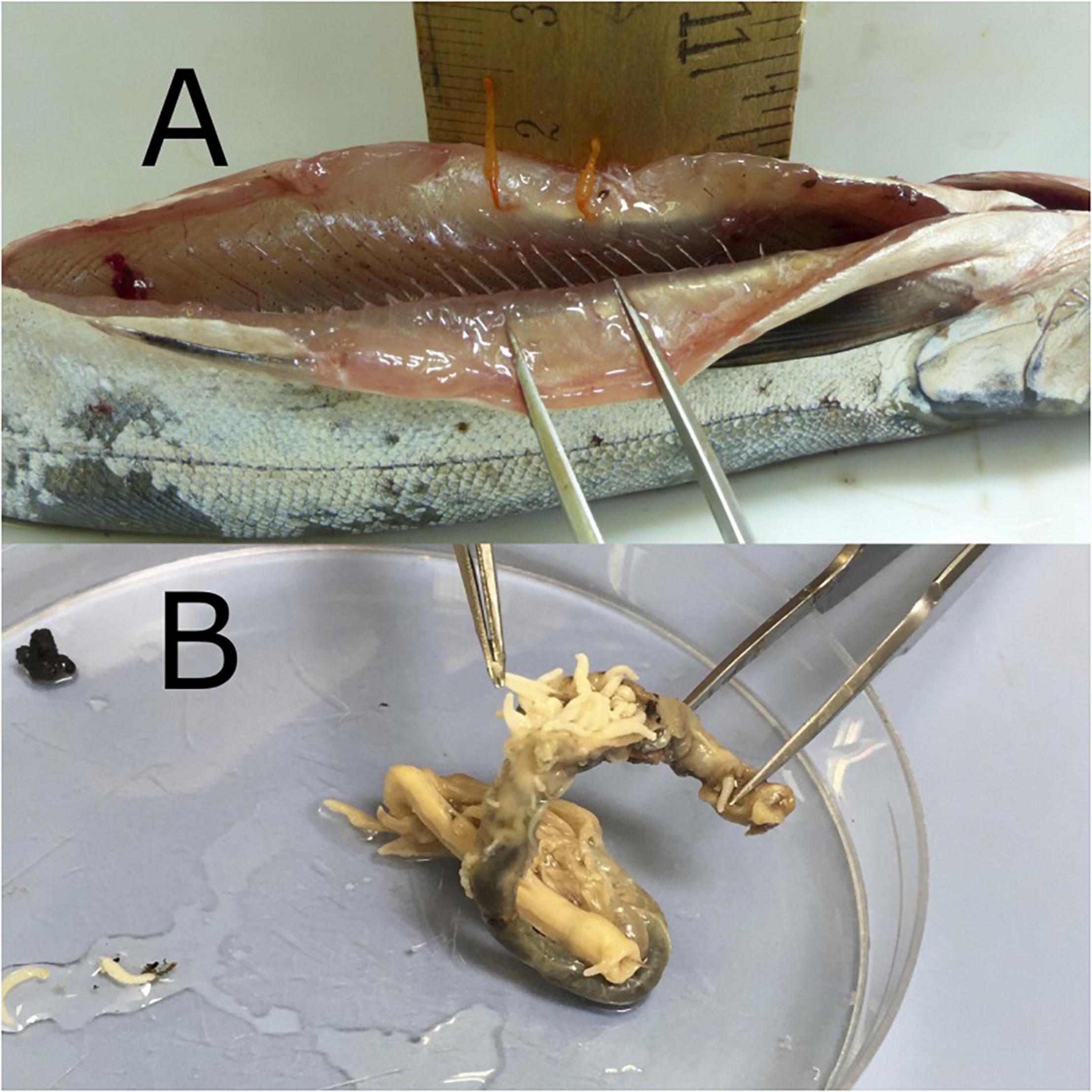
Figure 2. (A) Photograph of P. tereticollis outside the alimentary tract of salmon smolt and attached to the peritoneum and muscle wall. (B) Salmon smolt intestine, heavily infected by P. tereticollis.
No evidence of damage to intestine walls (i.e., pinprick leaks or visible perforations) from recently expelled acanthocephalans was observed in sampled smolts. Additionally, no leakage was observed through intestinal perforations that were plugged by the probosces of acanthocephalans. No mortality of smolts occurred in any treatment group prior to sampling. The binomial model revealed a significant positive association between infection prevalence and Fulton’s K (p = 0.012) (Figure 3A) but no evidence of significant associations with sex or fork length (Table 1). The negative binomial model (Table 2) revealed a significant association between individual infection intensity and sex, with infected males having higher infection intensities than infected females (p = 0.043). This model also revealed a non-significant positive association between Fulton’s K and infection intensity (p = 0.091) (Figure 3B).
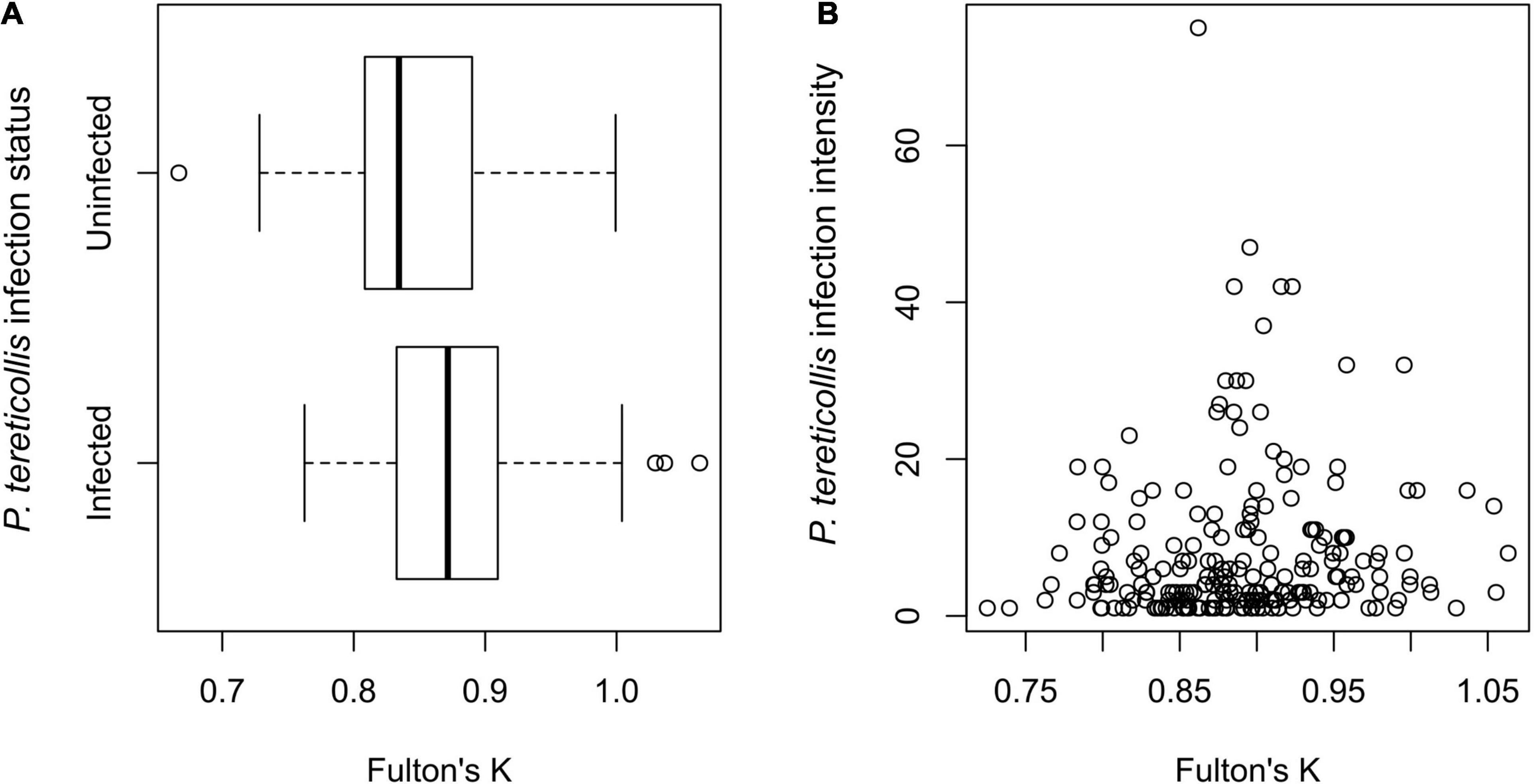
Figure 3. (A) Boxplot of infection status (i.e., whether individual smolts contain P. tereticollis or not) vs. Fulton’s K (condition factor) (n = 312) and (B) scatterplot of individual infection intensity (i.e., the number of P. tereticollis per infected smolt) vs. Fulton’s K (n = 218). Data include smolts sampled in 2016, 2017, and 2018.
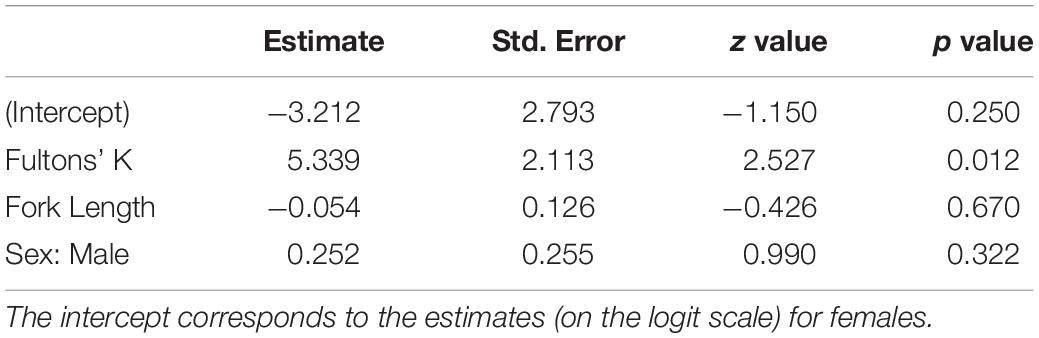
Table 1. Parameter estimates for the binomial prevalence GLM where individual infection status (i.e., infected or uninfected) is the binary response variable.
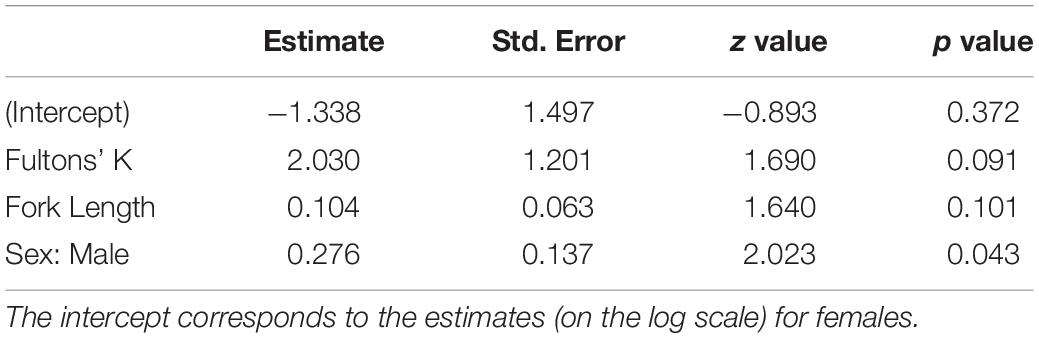
Table 2. Parameter estimates for the negative binomial GLM where individual infection intensity (i.e., the number of P. tereticollis per infected smolt) is the response variable.
Blood Parameters
The mean blood parameters in each treatment group fell within reported ranges for Atlantic salmon (Table 3; Bowers et al., 2000; Finstad et al., 2012; Kolarevic et al., 2014). Our initial chloride model revealed no significant interaction between acanthocephalan count and treatment group (p = 0.447). When this interaction was excluded, the model AIC value decreased by ∼7 and the main effect of acanthocephalan count was non-significant (p = 0.26) (Figure 4). The model without an interaction revealed significantly higher plasma chloride concentrations in the 24SW and 72SW treatment groups relative to the 24FW group (Tables 3, 4). This model also revealed a significant negative relationship between Fulton’s K and plasma chloride (Table 4). However, acanthocephalan count was not associated with variation in plasma chloride.
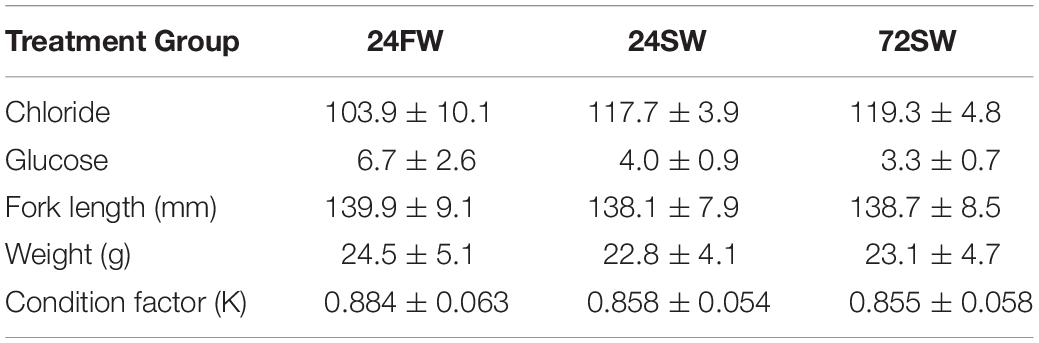
Table 3. Mean and standard deviations for each blood parameter and physical measurements for each treatment group.
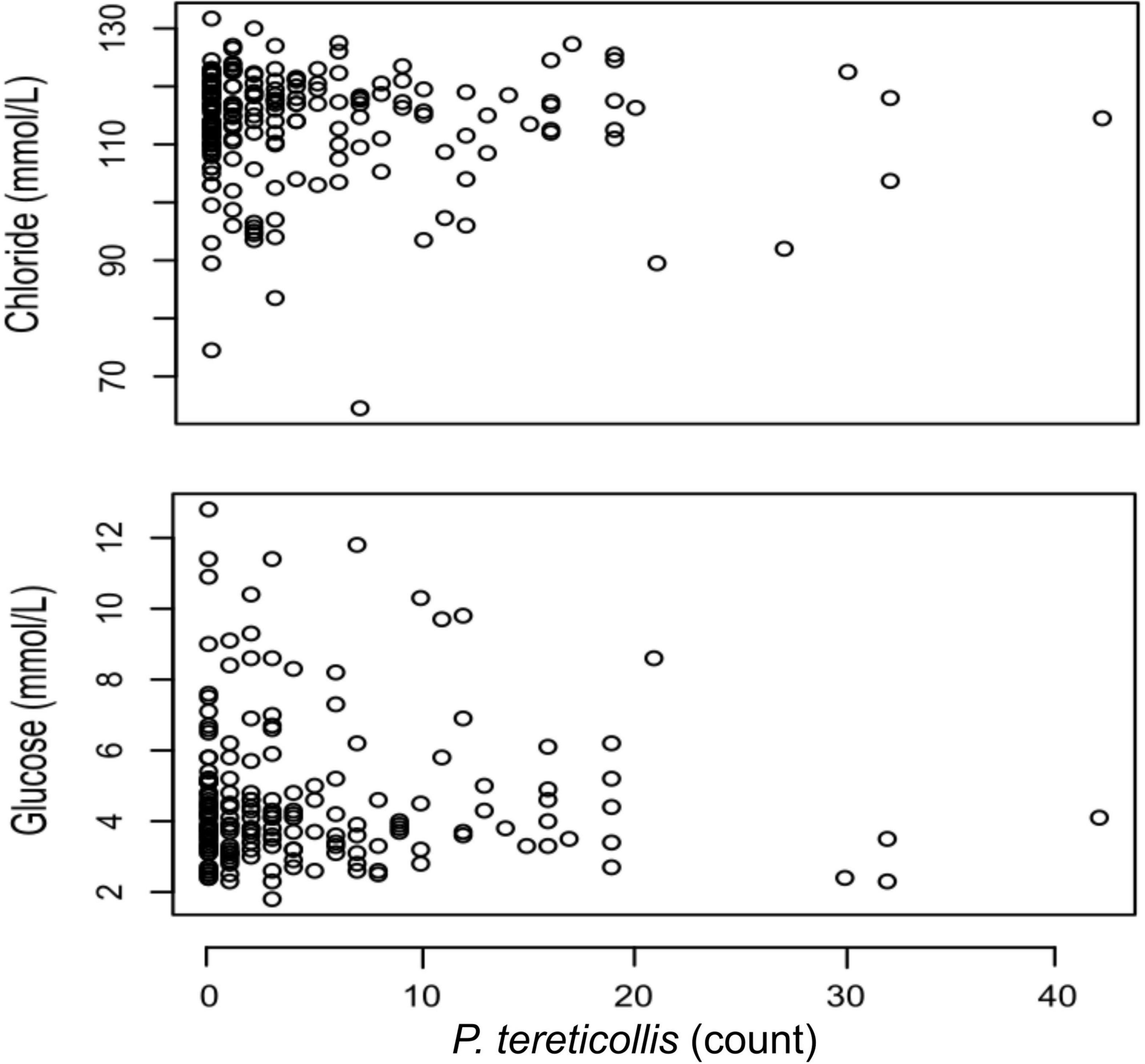
Figure 4. Plasma chloride concentration (mmo/l) and blood glucose concentration (mmol/l) vs. P. tereticollis count for all smolts used in the experiment (n = 198).
Our initial glucose model revealed no significant interaction between acanthocephalan count and treatment group (p = 0.391). Removal of the acanthocephalan count by treatment group interaction term lowered the model AIC value by ∼12.1. No significant association was found between blood glucose and acanthocephalan count in this model (Table 5 and Figure 4). Glucose levels in the 24SW and 72SW groups were significantly lower than in the 24FW group (Tables 3, 5). This model also revealed that blood glucose was significantly negatively related to Fulton’s K (Table 5).
Discussion
We found little evidence that natural infection by acanthocephalan parasites affected the osmoregulatory performance of Atlantic salmon smolts immediately prior to, or within the first 72 h of, entry into salt water. Moreover, there was no evidence of physiological stress associated with acanthocephalan infection–at least as captured by blood glucose levels, which is one of several possible secondary physiological responses linked to general stress in fishes (Barton, 2002). Infection prevalence amongst experimental smolts was 66.2% and parasite abundance exhibited greater-than-Poisson variance (raw variance 10.3 times greater than raw mean), with few smolts containing many parasites (Figure 2B) and many individuals containing zero parasites: a common finding in parasitology in general (Poulin, 2007). The acanthocephalan parasites, which our DNA barcoding indicated to be P. tereticollis, consistently survived the first 72 h that smolts spent in salt water. However, although salinity in the saltwater tanks (∼26 PPT) was representative of local coastal waters, it was lower than in the open ocean habitat of the North Atlantic (∼35 PPT). This lower salinity may have made the environment in the saltwater tanks more tolerable for these acanthocephalan parasites than open ocean environments but was representative of local conditions experienced by these smolts in the wild in their first few days in coastal waters. However, given that the PTL1 strain of P. tereticollis (the only strain recorded in Ireland thus far) is believed to have co-evolved with salinity tolerant hosts (O’Mahony et al., 2004; Perrot-Minnot et al., 2018; Andreou et al., 2020), it is also possible that these acanthocephalans can survive for prolonged periods while their hosts are in open ocean environments.
The apparently widespread distribution of P. tereticollis (formerly called P. laevis) in Ireland (Hine and Kennedy, 1974; Munro et al., 1990; Molloy et al., 1993; Byrne et al., 2003), combined with the preferential use of salmonid definitive hosts by the PTL1 strain in Ireland (Pippy, 1969; Perrot-Minnot et al., 2018; Andreou et al., 2020; Tierney et al., 2020), implies that anadromous Irish salmonids often enter the marine environment while infected with these acanthocephalan parasites. Indeed, we found that infection prevalence amongst salmon smolts that were captured at the tidal limit of the Burrishoole catchment during the 2016, 2017, and 2018 smolt runs exceeded 65% in all years, with mean infection intensities of 9.2, 7.3, and 6.9, respectively. These infection rates are in line with those reported from other Irish catchments where S. salar and P. laevis s.l. coexist (Pippy, 1969), and previous studies have also found strong overdispersion in P. laevis s.l. abundance amongst host fish (Kennedy, 1974, 1996; Brown, 1989). Some authors have suggested that post-cyclic transmission (i.e., transmission occurring when a definitive host eats another definitive host) causes such acanthocephalan overdispersion patterns in definitive host species (Lassiere and Crompton, 1988; Valtonen and Crompton, 1990; Kennedy, 1999). However, the small body size and therefore presumably pre-piscivorous diet of the sampled smolts (no fish parts were observed in the stomach contents of the 175 smolts dissected at time of capture in 2016 and 2017) makes post-cyclic transmission from other host fish unlikely in this case. Given the extensive habitat heterogeneity within the Burrishoole catchment (ranging from fast-flowing streams to deep lakes) (Whelan et al., 1998), it is perhaps more likely that differing feeding behavior in areas with differing densities of the intermediate host Gammarus duebeni resulted in contrasting infection opportunities amongst these smolts.
Infection intensity amongst infected smolts was not associated with significant variation in any measure of smolt size (fork length, weight or Fulton’s K). Infected males sampled in 2016 contained significantly more parasites per individual than infected females from the same year (12.8 vs. 4.9) although no sex bias in infection intensity was evident in 2017 or 2018. While no significant associations were found between infection prevalence and absolute measures of smolt size (i.e., fork length and weight), infected smolts were actually in better condition (at least as expressed by Fulton’s K) than uninfected smolts. At first glance this is surprising, given that smolt condition might be expected to be negatively impacted by parasitic infection. However, salmon are known to undergo a rapid increase in length during smoltification that is not matched by an equivalent increase in weight, leading to a reduction in condition factor (Wedemeyer et al., 1980). Thus, if infection by P. tereticollis caused a reduction in growth (i.e., length gain) during the parr-smolt transformation period, it could account for the comparatively high condition factor observed amongst infected individuals relative to uninfected individuals. Alternatively, though, this finding could simply reflect the fact that pre-smolts that feed more actively in the weeks or months preceding their marine migration may attain increased condition relative to less active feeders but also have higher chances of consuming intermediate hosts (i.e., G. duebeni) infected with P. tereticollis, given that new infections of salmonids in Irish waters tend to peak in spring (Fitzgerald and Mulcahy, 1983; Molloy et al., 1995). Such seasonal patterns of parasite infections in salmonids are often associated with temporal changes in diet (Prati and Henriksen, 2020). Previous research has shown that many species of acanthocephalan parasites cause substantial damage to their hosts’ intestines (Kim et al., 2011), reducing growth rates when infection intensities are high and leading to mortality in extreme cases (Latham and Poulin, 2002; Mayer et al., 2003). However, if many of the P. tereticollis found in the sampled smolts were relatively recent infections, there may have been insufficient time for their presence to cause a discernible effect on growth. As marine survival can be strongly associated with smolt size (Jonsson et al., 2017; Gregory et al., 2018), any parasite-induced impact on growth or condition factor is likely to have fitness consequences.
We found that P. tereticollis deeply penetrated all layers of their host’s intestinal wall with their praesoma (hooked proboscis), and in some cases even penetrated the peritoneum and adjacent muscle wall, passing completely out of the intestines in the process (Figure 2A), similar to previous reports (Dezfuli et al., 2002a). The anchoring method used by P. laevis s.l. has been shown to destroy intestinal mucosa (Wanstall et al., 1988), eliciting a localized inflammatory response (Wanstall et al., 1986; Dezfuli et al., 2008, 2011) and copious mucus secretion (Harris, 1972; Dezfuli et al., 2016). Although there is only limited evidence indicating that salmonids infected with P. laevis s.l. suffer reduced growth (Wanstall, 1984), it appears that these infections may cause modifications to the physiological functioning of their host’s alimentary tract, potentially reducing control of transepithelial ion transport (Dezfuli et al., 2002a). Thus, we expected that negative impacts from P. tereticollis infection might only manifest when the host entered salt water, where effective osmoregulation requires efficient control of transepithelial ion transport by the intestine (Whittamore, 2012).
As anticipated (i.e., Bowers et al., 2000; Urke et al., 2014; Stewart et al., 2016), plasma chloride concentrations were significantly higher in smolts sampled after 24 and 72 h in salt water than in smolts sampled after 24 h in fresh water. However, P. tereticollis abundance was not associated with variation in chloride levels in any treatment group, indicating that any intestinal damage caused by P. tereticollis was insufficient to cause hyper or hypo-osmoregulatory failure. The range of plasma chloride concentrations in the freshwater and saltwater treatment groups were similar to those reported from other studies of Atlantic salmon in freshwater and saltwater environments (Oppedal et al., 1999; Wells et al., 2006; Kolarevic et al., 2014). However, no sampled smolts displayed highly elevated chloride levels in line with levels that have been recorded in salmon smolts infected with high numbers of salmon lice (L. salmonis), which would indicate compromised hypo-osmoregulatory function (Grimnes and Jakobsen, 1996; Wagner et al., 2003).
Hyperglycemia (elevated blood glucose) is a secondary stress response in fish that has been widely used as an indicator of parasite-induced stress in smolts (Wagner et al., 2003; Finstad et al., 2007; Long et al., 2019). No association between glucose and P. tereticollis count was detected in any of the treatment groups, which would suggest that P. tereticollis infection intensities observed in this study were insufficient to cause a discernible stress response in smolts in hypo-osmotic environments or within 24- to 72-h of entry into hyper-osmotic environments. However, blood glucose is just one of many possible indicators of a complex, multi-dimensional stress response and thus it remains possible that other measures such as plasma cortisol could reveal parasite-induced effects on host stress levels that we were unable to detect.
Glucose levels were lowest in the group sampled after 72-h in salt water, which might relate to the longer period of fasting that this group experienced prior to sampling (72 h vs. 24 h in the other treatment groups). Plasma glucose is known to be affected by the feeding history and metabolic status of fish (Wells et al., 2006) and so it is possible that the comparatively low glucose levels found in the 72SW treatment group resulted from increased caloric deficit.
The results of this study are based on the use of four replicate tanks for each treatment group. However, despite having 66 smolts in each treatment group (i.e., moderate to large sample sizes), only a small number of smolts had high infection intensities (Figure 1), and this may have reduced our ability to detect subtle parasite-induced changes in the blood parameters that we investigated. Also, all P. tereticollis found in each treatment group were alive and the vast majority were securely anchored to the intestinal wall by their praesomae, with no sign that others had recently detached (i.e., no vacant perforations in the intestinal wall). When P. tereticollis are anchored to the intestinal wall, their praesoma and inflated proboscis bulb appear to form an effective plug, preventing movement of liquid through the surrounding intestinal perforation. The P. tereticollis that parasitize juvenile salmon in the Burrishoole catchment are generally absent from adult salmon when they return to the river system for spawning (Deirdre Cotter, pers. obs.). Similarly, Pippy (1980) found no P. laevis s.l. in adult salmon caught off Greenland, concluding that the parasites could not endure the prolonged migration period of their host. Furthermore, Molloy et al. (1993) found that sea trout returning to the Burrishoole catchment had lower infection prevalence and intensity than were found in emigrating trout smolts, indicating that P. tereticollis are lost during the marine migration. Given that the lifespan of P. laevis s.l. in freshwater barbel (Barbus barbus) hosts has been estimated at between 6 and 8 months (Nachev and Sures, 2016), it is likely that P. tereticollis generally die before migrating salmon hosts return to fresh water. Presumably, after some period in the marine environment these acanthocephalans detach from their hosts’ intestines, leaving intestinal perforations unplugged and thereby potentially facilitating ingress of salt water into the peritoneal cavity. However, as P. tereticollis in the sampled smolts remained alive and attached after 72 h in salt water we were unable to investigate this possible delayed pathology. As suggested by Pippy (1969), it would be useful for future studies to accurately establish the lifespan of P. laevis s.l. in Atlantic salmon at sea.
The shifts between freshwater and marine environments that define diadromous fishes are inherently stressful, demanding complex physiological responses from migrants that are simultaneously exposed to unfamiliar predators, parasites and pathogens. Mortality rates during this transitional period can be particularly high, and any pre-existing factor that increases stress or interferes with physiological processes such as osmoregulation may compromise long-term survival at sea (Finstad et al., 2012, 2007; Hostetter et al., 2012, 2011). While the contribution of non-lethal stressors to delayed marine mortality is often difficult to detect, particularly in cases where multiple stressors have a cumulative effect, their impacts are likely to play an important role in determining the performance of anadromous populations. We have shown that a high proportion of wild Atlantic salmon smolts entering the marine environment from the Burrishoole catchment in recent years are infected with P. tereticollis and, based on the literature, it appears likely that similar infection rates of smolts are common in Ireland but not elsewhere. However, we found no evidence to indicate that the infection intensities observed amongst the sampled smolts were associated with altered osmoregulatory performance or blood glucose levels in freshwater or saltwater environments. Despite this, it is possible that infection by P. tereticollis causes pathologies that we did not test for or that occur later in the marine environment as salinity increases or as the parasites detach from the intestine. Given the high prevalence of P. tereticollis infection amongst anadromous salmonid populations in Ireland, it would be valuable to investigate whether such delayed parasite-induced pathologies occur at sea. Such an investigation could be undertaken in a similar manner to the present study, but conducted over a longer time period at a higher salinity, potentially providing results with applied relevance in the context of fishery management and aquaculture (e.g., marine rearing of farmed Atlantic salmon).
In conclusion, our study adds to a growing body of work examining ecophysiological processes underpinning the performance of migratory fishes (McCormick et al., 1998, 2009; Hinch et al., 2005; Cooke et al., 2008, 2012; Groot, 2010; Björnsson et al., 2011; Eliason and Farrell, 2016) and draws particular attention to the potential role of parasites in determining osmoregulatory abilities. The physiological impacts of parasitism often depend on the extent and patterns of historical coevolution between hosts and their parasites (Prenter et al., 2004; Britton et al., 2011; but see Lymbery et al., 2014). Migratory species are particularly interesting in this context as they are exposed to native and non-native parasites in multiple geographic locations and habitat types. Consequently, infection or infestation may have delayed fitness consequences that occur in a different habitat to where the parasites are initially encountered. We therefore encourage further ecophysiological work on the impacts of parasites on the performance of migratory animals across variable and rapidly changing environments.
Data Availability Statement
The raw data supporting the conclusions of this article will be made available by the authors, without undue reservation.
Ethics Statement
The animal study was reviewed and approved by Marine Institute Animal Welfare Committee (MI Establishment Authorisation No: AE19121) and the Health Professionals Regulatory Authority (HPRA Classification Request Number: 066).
Author Contributions
RF, TR, and RP conceived and designed the experiment. RF, RP, and GR collected the data. ED conducted the genetic barcoding. RF and TR analyzed the data. RF wrote the first draft of the manuscript. All authors contributed to drafts of the manuscript and gave final approval for publication.
Funding
This work was funded by the Marine Institute Cullen Fellowship award CF/15/06. TR was funded by an ERC Starting Grant (ERC-2014-StG-639192-ALH) and an SFI ERC Support Award.
Conflict of Interest
The authors declare that the research was conducted in the absence of any commercial or financial relationships that could be construed as a potential conflict of interest.
Publisher’s Note
All claims expressed in this article are solely those of the authors and do not necessarily represent those of their affiliated organizations, or those of the publisher, the editors and the reviewers. Any product that may be evaluated in this article, or claim that may be made by its manufacturer, is not guaranteed or endorsed by the publisher.
Acknowledgments
We thank Phil McGinnity, Elvira de Eyto, the staff of the Marine Institute Burrishoole research facility, Newport, and the field staff of the FishEye Research Group. This manuscript benefitted from thoughtful advice and comments provided by Celia Holland, Bengt Finstad, and Joshka Kaufmann.
References
Alerstam, T., Hedenström, A., and Åkesson, S. (2003). Long-distance migration: evolution and determinants. Oikos 103, 247–260. doi: 10.1034/j.1600-0706.2003.12559.x
Altizer, S. M., Oberhauser, K. S., and Brower, L. P. (2000). Associations between host migration and the prevalence of a protozoan parasite in natural populations of adult monarch butterflies. Ecol. Entomol. 25, 125–139. doi: 10.1046/j.1365-2311.2000.00246.x
Andreou, D., Antognazza, C. M., Williams, C. F., Bradley, H., Reading, A. J., Hardouin, E. A., et al. (2020). Vicariance in a generalist fish parasite driven by climate and salinity tolerance of hosts. Parasitology 147, 1658–1664. doi: 10.1017/s0031182020001663
Archer, L. C., Hutton, S. A., Harman, L., O’Grady, M. N., Kerry, J. P., Poole, W. R., et al. (2019). The interplay between extrinsic and intrinsic factors in determining migration decisions in brown trout (Salmo trutta): an experimental study. Front. Ecol. Evol. 7:222. doi: 10.3389/fevo.2019.00222
Bakke, T. A., and Harris, P. D. (1998). Diseases and parasites in wild Atlantic salmon (Salmo salar) populations. Can. J. Fish. Aquat. Sci. 55, 247–266. doi: 10.1139/d98-021
Barton, B. A. (2002). Stress in fishes: a diversity of responses with particular reference to changes in circulating corticosteroids. Integr. Comp. Biol. 42, 517–525. doi: 10.1093/icb/42.3.517
Bisbal, G. A., and Specker, J. L. (1991). Cortisol stimulates hypo−osmoregulatory ability in Atlantic salmon, Salmo salar L. J. Fish Biol. 39, 421–432. doi: 10.1111/j.1095-8649.1991.tb04373.x
Björnsson, B. T., Stefansson, S. O., and McCormick, S. D. (2011). Environmental endocrinology of salmon smoltification. Gen. Comp. Endocrinol. 170, 290–298. doi: 10.1016/j.ygcen.2010.07.003
Borges, F. O., Santos, C. P., Sampaio, E., Figueiredo, C., Paula, J. R., Antunes, C., et al. (2019). Ocean warming and acidification may challenge the riverward migration of glass eels. Biol. Lett. 15:20180627. doi: 10.1098/rsbl.2018.0627
Bowers, J. M., Mustafa, A., Speare, D. J., Conboy, G. A., Brimacombe, M., Sims, D. E., et al. (2000). The physiological response of Atlantic salmon, Salmo salar L., to a single experimental challenge with sea lice, Lepeophtheirus salmonis. J. Fish Dis. 23, 165–172. doi: 10.1046/j.1365-2761.2000.00225.x
Britton, J. R., Pegg, J., and Williams, C. F. (2011). Pathological and ecological host consequences of infection by an introduced fish parasite. PLoS One 6:e26365. doi: 10.1371/journal.pone.0026365
Brooks, M. E., Kristensen, K., van Benthem, K. J., Magnusson, A., Berg, C. W., Nielsen, A., et al. (2017). {glmmTMB} Balances speed and flexibility among packages for zero-inflated generalized linear mixed modeling. R J. 9, 378–400. doi: 10.32614/rj-2017-066
Brown, A. F. (1989). Seasonal dynamics of the acanthocephalan Pomphorhynchus laevis (Muller, 1776) in its intermediate and preferred definitive hosts. J. Fish Biol. 34, 183–194. doi: 10.1111/j.1095-8649.1989.tb03301.x
Brownscombe, J. W., Cooke, S. J., Algera, D. A., Hanson, K. C., Eliason, E. J., Burnett, N. J., et al. (2017). Ecology of exercise in wild fish: integrating concepts of individual physiological capacity, behavior, and fitness through diverse case studies. Integr. Comp. Biol. 57, 281–292. doi: 10.1093/icb/icx012
Byrne, C. J., Holland, C. V., Kennedy, C. R., and Poole, W. R. (2003). Interspecific interactions between Acanthocephala in the intestine of brown trout: are they more frequent in Ireland? Parasitology 127, 399–409. doi: 10.1017/s0031182003003846
Cooke, S. J., Hinch, S. G., Donaldson, M. R., Clark, T. D., Eliason, E. J., Crossin, G. T., et al. (2012). Conservation physiology in practice: how physiological knowledge has improved our ability to sustainably manage Pacific salmon during up-river migration. Philos. Trans. R. Soc. B Biol. Sci. 367, 1757–1769. doi: 10.1098/rstb.2012.0022
Cooke, S. J., Hinch, S. G., Farrell, A. P., Patterson, D. A., Miller-Saunders, K., Welch, D. W., et al. (2008). Developing a mechanistic understanding of fish migrations by linking telemetry with physiology, behavior, genomics and experimental biology: an interdisciplinary case study on adult fraser river sockeye salmon. Fisheries 33, 321–339. doi: 10.1577/1548-8446-33.7.321
Crossin, G. T., Hinch, S. G., Cooke, S. J., Welch, D. W., Patterson, D. A., Jones, S. R. M., et al. (2008). Exposure to high temperature influences the behaviour, physiology, and survival of sockeye salmon during spawning migration. Can. J. Zool. 86, 127–140. doi: 10.1139/z07-122
Dawson, L. H. J., Pike, A. W., Houlihan, D. F., and McVicar, A. H. (1998). Effects of salmon lice Lepeophtheirus salmonis on sea trout Salmo trutta at different times after seawater transfer. Dis. Aquat. Organ. 33, 179–186. doi: 10.3354/dao033179
de Eyto, E., Connolly, P., Cotter, D., Dalton, C., Jennings, E., McGinnity, P., et al. (2020). “The Burrishoole Catchment,” in Ireland’s Rivers, eds M. Kelly-Quinn and J. Reynolds (Dublin: University College Dublin press), 357–382.
de Eyto, E., Dalton, C., Dillane, M., Jennings, E., McGinnity, P., O’Dwyer, B., et al. (2016). The response of North Atlantic diadromous fish to multiple stressors, including land use change: a multidecadal study. Can. J. Fish. Aquat. Sci. 73, 1759–1769. doi: 10.1139/cjfas-2015-0450
Dezfuli, B. S., Bosi, G., DePasquale, J. A., Manera, M., and Giari, L. (2016). Fish innate immunity against intestinal helminths. Fish Shellfish Immunol. 50, 274–287. doi: 10.1016/j.fsi.2016.02.002
Dezfuli, B. S., Castaldelli, G., Bo, T., Lorenzoni, M., and Giari, L. (2011). Intestinal immune response of Silurus glanis and Barbus barbus naturally infected with Pomphorhynchus laevis (Acanthocephala). Parasite Immunol. 33, 116–123. doi: 10.1111/j.1365-3024.2010.01266.x
Dezfuli, B. S., Giari, L., Simoni, E., Bosi, G., and Manera, M. (2002a). Histopathology, immunohistochemistry and ultrastructure of the intestine of Leuciscus cephalus (L.) naturally infected with Pomphorhynchus laevis (Acanthocephala). J. Fish Dis. 25, 7–14. doi: 10.1046/j.1365-2761.2002.00332.x
Dezfuli, B. S., Lui, A., Boldrini, P., Pironi, F., and Giari, L. (2008). The inflammatory response of fish to helminth parasites. Parasite 15, 426–433. doi: 10.1051/parasite/2008153426
Dezfuli, B. S., Pironi, F., Giari, L., Domeneghini, C., and Bosi, G. (2002b). Effect of Pomphorhynchus laevis (Acanthocephala) on putative neuromodulators in the intestine of naturally infected Salmo trutta. Dis. Aquat. Organ. 51, 27–35. doi: 10.3354/dao051027
Dingle, H. (2014). Migration: The Biology of Life on the Move. New York, NY: Oxford University Press.
Eames, S. C., Philipson, L. H., Prince, V. E., and Kinkel, M. D. (2010). Blood sugar measurement in zebrafish reveals dynamics of glucose homeostasis. Zebrafish 7, 205–213. doi: 10.1089/zeb.2009.0640
Eliason, E. J., and Farrell, A. P. (2016). Oxygen uptake in Pacific salmon Oncorhynchus s: when ecology and physiology meet. J. Fish Biol. 88, 359–388. doi: 10.1111/jfb.12790
Fealy, R., Allott, N., Broderick, C., de Eyto, E., Dillane, M., Erdil, R. M., et al. (2014). RESCALE: Review and Simulate Climate and Catchment Responses at Burrishoole. Galway: Marine Institute.
Finlay, R., Poole, R., Coughlan, J., Phillips, K. P., Prodöhl, P., Cotter, D., et al. (2020). Telemetry and genetics reveal asymmetric dispersal of a lake-feeding salmonid between inflow and outflow spawning streams at a microgeographic scale. Ecol. Evol. 10, 1762–1783. doi: 10.1002/ece3.5937
Finstad, B., Kroglund, F., Bjørn, P. A., Nilsen, R., Pettersen, K., Rosseland, B. O., et al. (2012). Salmon lice-induced mortality of Atlantic salmon postsmolts experiencing episodic acidification and recovery in freshwater. Aquaculture 362–363, 193–199. doi: 10.1016/j.aquaculture.2010.10.037
Finstad, B., Kroglund, F., Strand, R., Stefansson, S. O., Bjørn, P. A., Rosseland, B. O., et al. (2007). Salmon lice or suboptimal water quality–reasons for reduced postsmolt survival? Aquaculture 273, 374–383. doi: 10.1016/j.aquaculture.2007.10.019
Fitzgerald, R. D., and Mulcahy, M. F. (1983). “Parasites of salmon Salmo salar L. and trout Salmo trutta L. in the River Shournagh,” in Irish Fisheries Investigations: Advances in Fish Biology in Ireland, ed. C. Moriarty (Dublin: The National Committee for Biology of the Royal Irish Academy), 24–31.
Genz, J., Esbaugh, A. J., and Grosell, M. (2011). Intestinal transport following transfer to increased salinity in an anadromous fish (Oncorhynchus mykiss). Comp. Biochem. Physiol. 159, 150–158. doi: 10.1016/j.cbpa.2011.02.011
Gilbert, M. J. H., and Tierney, K. B. (2018). Warm northern river temperatures increase post-exercise fatigue in an Arctic migratory salmonid but not in a temperate relative. Anim. Physiol. Ecol. 32, 687–700. doi: 10.1111/1365-2435.13006
Goossens, S., Wybouw, N., Van Leeuwen, T., and Bonte, D. (2020). The physiology of movement. Mov. Ecol. 8, 1–14. doi: 10.5005/jp/books/10538_1
Gregory, S. D., Armstrong, J. D., and Britton, J. R. (2018). Is bigger really better? Towards improved models for testing how Atlantic salmon Salmo salar smolt size affects marine survival. J. Fish Biol. 92, 579–592. doi: 10.1111/jfb.13550
Grimnes, A., and Jakobsen, P. J. (1996). The physiological effects of salmon lice infection on post-smolt of Atlantic salmon. J. Fish Biol. 49, 1179–1194. doi: 10.1111/j.1095-8649.1996.tb01813.x
Grosell, M. (2007). “Intestinal transport processes in marine fish osmoregulation,” in Fish Osmoregulation, 1st Edn, ed. B. Baldisserotto (Boca Raton, FL: CRC Press), 333–357. doi: 10.1201/9780429063909-12
Gylfe, Å, Bergström, S., Lundström, J., and Olsen, B. (2000). Reactivation of Borrelia infection in birds. Nature 403, 724–725. doi: 10.1038/35001663
Handeland, S. O., Imsland, A. K., Nilsen, T. O., Ebbesson, L. O. E., Hosfeld, C. D., Pedrosa, C., et al. (2014). Osmoregulation in Atlantic salmon Salmo salar smolts transferred to seawater at different temperatures. J. Fish Biol. 85, 1163–1176. doi: 10.1111/jfb.12481
Harris, J. E. (1972). The immune response of a cyprinid fish to the acanthocephalan Pomphorhynchys Laevis. Int. J. Parasitol. 2, 459–469. doi: 10.1016/0020-7519(72)90091-4
Hartig, F. (2019). DHARMa: Residual Diagnostics for Hierarchical (Multi-Level / Mixed). Available online at: https://cran.r-project.org/package=DHARMa (accessed December 19, 2019).
Hinch, S. G., Cooke, S. J., Healey, M. C., and Farrell, A. P. (2005). Behavioural physiology of fish migrations: salmon as a model approach. Fish Physiol. 24, 239–295. doi: 10.1016/s1546-5098(05)24007-4
Hine, P. M., and Kennedy, C. R. (1974). Observations on the distribution, specificity and pathogenicity of the acanthocephalan Pomphorhynchus laevis (Muller). J. Fish Biol. 6, 521–535. doi: 10.1111/j.1095-8649.1974.tb04569.x
Hostetter, N. J., Evans, A. F., Roby, D. D., and Collis, K. (2012). Susceptibility of juvenile steelhead to avian predation: the influence of individual fish characteristics and river conditions. Trans. Am. Fish. Soc. 141, 1586–1599. doi: 10.1080/00028487.2012.716011
Hostetter, N. J., Evans, A. F., Roby, D. D., Collis, K., Hawbecker, M., Sandford, B. P., et al. (2011). Relationship of external fish condition to pathogen prevalence and out-migration survival in juvenile steelhead. Trans. Am. Fish. Soc. 140, 1158–1171. doi: 10.1080/00028487.2011.613303
Hvas, M., Karlsbakk, E., Mæhle, S., Wright, D. W., and Oppedal, F. (2017). The gill parasite Paramoeba perurans compromises aerobic scope, swimming capacity and ion balance in Atlantic salmon. Conserv. Physiol. 5, 1–12. doi: 10.1016/j.aquaculture.2016.01.019
Iversen, M., Finstad, B., and Nilssen, K. J. (1998). Recovery from loading and transport stress in Atlantic salmon (Salmo salar L.) smolts. Aquaculture 168, 387–394. doi: 10.1016/s0044-8486(98)00364-0
Jonsson, B., Jonsson, M., and Jonsson, N. (2017). Influences of migration phenology on survival are size dependent in juvenile Atlantic salmon. Can. J. Zool. 95, 581–587. doi: 10.1139/cjz-2016-0136
Kennedy, C. R. (1974). The importance of parasite mortality in regulating the population size of the Acanthocephalan Pomphorhynchus laevis in goldfish. Parasitology 68, 93–101. doi: 10.1017/s0031182000045406
Kennedy, C. R. (1996). Colonization and establishment of Pomphorhynchus laevis (Acanthocephala) in an isolated English river. J. Helminthol. 70, 27–31. doi: 10.1017/s0022149x00015091
Kennedy, C. R. (1999). Post-cyclic transmission in Pomphorhynchus laevis (Acanthocephala). Folia Parasitol. 46, 111–116.
Kim, S. R., Lee, J. S., Kim, J. H., Oh, M. J., Kim, C. S., Park, M. A., et al. (2011). Fine structure of Longicollum pagrosomi (Acanthocephala: Pomphorhynchidae) and intestinal histopathology of the red sea bream, Pagrus major, infected with acanthocephalans. Parasitol. Res. 109, 175–184. doi: 10.1007/s00436-010-2241-z
Kolarevic, J., Baeverfjord, G., Takle, H., Ytteborg, E., Reiten, B. K. M., Nergård, S., et al. (2014). Performance and welfare of Atlantic salmon smolt reared in recirculating or flow through aquaculture systems. Aquaculture 432, 15–25. doi: 10.1016/j.aquaculture.2014.03.033
Krkošek, M., Revie, C. W., Gargan, P. G., Skilbrei, O. T., Finstad, B., and Todd, C. D. (2013). Impact of parasites on salmon recruitment in the Northeast Atlantic Ocean. Proc. R. Soc. B Biol. Sci. 280, 1–8.
Lassiere, O. L., and Crompton, D. W. T. (1988). Evidence for post-cyclic transmission in the life-history of Neoechinorhynchus rutili (Acanthocephala). Parasitology 97, 339–343.
Latham, A. D. M., and Poulin, R. (2002). Field evidence of the impact of two acanthocephalan parasites on the mortality of three species of New Zealand shore crabs (Brachyura). Mar. Biol. 141, 1131–1139. doi: 10.1007/s00227-002-0913-7
Long, A., Garver, K. A., and Jones, S. R. M. (2019). Differential effects of adult salmon lice Lepeophtheirus salmonis on physiological responses of sockeye salmon and Atlantic Salmon. J. Aquat. Anim. Health 31, 75–87. doi: 10.1002/aah.10053
Lüdecke, D., Makowski, D., and Waggoner, P. (2019). Performance: Assessment of Regression Models Performance. Available online at: https://cran.r-project.org/package=performance (accessed December 19, 2019).
Lymbery, A. J., Morine, M., Kanani, H. G., Beatty, S. J., and Morgan, D. L. (2014). Co-invaders: the effects of alien parasites on native hosts. Int. J. Parasitol. Parasites Wildl. 3, 171–177. doi: 10.1016/j.ijppaw.2014.04.002
Matthews, M. A., Poole, W. R., Dillane, M. G., and Whelan, K. F. (1997). Juvenile recruitment and smolt output of brown trout (Salmo trutta L.) and Atlantic salmon (Salmo salar L.) from a lacustrine system in western Ireland. Fish. Res. 31, 19–37. doi: 10.1016/s0165-7836(97)00013-1
Mayer, K. A., Dailey, M. D., and Miller, M. A. (2003). Helminth parasites of the southern sea otter Enhydra lutris nereis in central California: abundance, distribution and pathology. Dis. Aquat. Organ. 53, 77–88. doi: 10.3354/dao053077
McCormick, S. D. (2012). Smolt physiology and endocrinology. Euryhaline Fish. Fish Physiol. 32, 199–251. doi: 10.1016/b978-0-12-396951-4.00005-0
McCormick, S. D., Hansen, L. P., Quinn, T. P., and Saunders, R. L. (1998). Movement, migration, and smolting of Atlantic salmon (Salmo salar). Can. J. Fish. Aquat. Sci. 55, 77–92. doi: 10.1139/d98-011
McCormick, S. D., Lerner, D. T., Monette, M. Y., Nieves-Puigdoller, K., Kelly, J. T., and Björnsson, B. T. (2009). Taking it with you when you go: how perturbations to the freshwater environment, including temperature, dams, and contaminants, affect marine survival of salmon. Am. Fish. Soc. Symp. 69, 195–214.
McGeer, J. C., Baranyi, L., and Iwama, G. K. (1991). Physiological responses to challenge tests in six stocks of coho salmon (Oncorhynchus kisutch). Can. J. Fish. Aquat. Sci. 48, 1761–1771. doi: 10.1139/f91-208
Møller, A. P., and Szép, T. (2011). The role of parasites in ecology and evolution of migration and migratory connectivity. J. Ornithol. 152, 141–150. doi: 10.1007/s10336-010-0621-x
Molloy, S., Holland, C., and O’Regan, M. (1995). Population biology of Pomphorhynchus laevis in brown trout from two lakes in the west of Ireland. J. Helminthol. 69, 229–235. doi: 10.1017/s0022149x00014188
Molloy, S., Holland, C., and Poole, R. (1993). Helminth parasites of brown and sea trout Salmo trutta L. from the west coast of Ireland. Proc. R. Irish Acad. B Biol. Environ. 93B, 137–142.
Munro, M. A., Reid, A., and Whitfield, P. J. (1990). Genomic divergence in the ecologically differentiated English freshwater and marine strains of Pomphorhynchus laevis (Acanthocephala: Palaeacanthocephala): a preliminary investigation. Parasitology 101, 451–454. doi: 10.1017/s0031182000060650
Nachev, M., and Sures, B. (2016). Seasonal profile of metal accumulation in the acanthocephalan Pomphorhynchus laevis: a valuable tool to study infection dynamics and implications for metal monitoring. Parasit. Vectors 9:300.
Norris, K., and Evans, M. R. (2000). Ecological immunology: life history trade-offs and immune defense in birds. Behav. Ecol. 11, 19–26. doi: 10.1093/beheco/11.1.19
O’Mahony, E. M., Bradley, D. G., Kennedy, C. R., and Holland, C. V. (2004). Evidence for the hypothesis of strain formation in Pomphorhynchus laevis (Acanthocephala): an investigation using mitochondrial DNA sequences. Parasitology 129, 341–347. doi: 10.1017/s0031182004005748
Oppedal, F., Taranger, G. L., Juell, J.-E., and Hansen, T. (1999). Growth, osmoregulation and sexual maturation of underyearling Atlantic salmon smolt Salmo salar L. exposed to different intensities of continuous light in sea cages. Aquac. Res. 30, 491–499. doi: 10.1046/j.1365-2109.1999.00362.x
Perrot-Minnot, M. J., Bollache, L., and Lagrue, C. (2020). Distribution of Pomphorhynchus laevis s.l. (Acanthocephala) among fish species at a local scale: importance of fish biomass density. J. Helminthol. 94, 1–10.
Perrot-Minnot, M. J., Špakulová, M., Wattier, R., Kotlík, P., Düşen, S., Aydoğdu, A., et al. (2018). Contrasting phylogeography of two Western Palaearctic fish parasites despite similar life cycles. J. Biogeogr. 45, 101–115. doi: 10.1111/jbi.13118
Piersma, T. (1997). Do global patterns of habitat use and migration strategies co-evolve with relative investments in immunocompetence due to spatial variation in parasite pressure? Oikos 80:623. doi: 10.2307/3546640
Pinheiro, J., Bates, D., DebRoy, S., Sarkar, D., Heisterkamp, S., and Van Willigen, B. (2019). nlme: Linear and Nonlinear Mixed Effects Models. Available online at: https://cran.r-project.org/package=nlme (accessed December 19, 2019).
Pippy, J. (1969). Pomphorhynchus laevis (Zoega) Müller, 1776 (Acanthocephala) in Atlantic Salmon (Salmo salar) and its use as a biological tag. J. Fish. Res. Board Can. 26, 909–919. doi: 10.1139/f69-088
Pippy, J. H. C. (1980). The value of parasites as biological tags in Atlantic salmon at West Greenland. Ra P.-v. Réun. Cons. Int. Explor. Mer 176, 76–81.
Poole, W. R., Dillane, M., Deeyto, E., Rogan, G., Mcginnity, P., and Whelan, K. (2007). “Characteristics of the Burrishoole Sea trout population: census, marine survival, enhancement and stock-recruitment relationship, 1971-2003,” in Sea Trout: Biology, Conservation and Management, eds G. Harris and N. Milner (Oxford: Wiley-Blackwell), 279–306. doi: 10.1002/9780470996027.ch19
Poole, W. R., Nolan, D., and Tully, O. (2000). Modelling the effects of capture and sea lice [Lepeophtheirus salmonis (Kroyer)] infestation on the cortisol stress response in trout. Aquac. Res. 31, 835–841. doi: 10.1046/j.1365-2109.2000.00516.x
Poole, W. R., Nolan, D. T., Wevers, T., Dillane, M., Cotter, D., and Tully, O. (2003). An ecophysiological comparison of wild and hatchery-raised Atlantic salmon (Salmo salar L.) smolts from the Burrishoole system, western Ireland. Aquaculture 222, 301–314. doi: 10.1016/s0044-8486(03)00129-7
Popovic, N. T., Strunjak-Perovic, I., Coz-Rakovac, R., Barisic, J., Jadan, M., Berakovic, A. P., et al. (2012). Tricaine methane-sulfonate (MS-222) application in fish anaesthesia. J. Appl. Ichthyol. 28, 553–564. doi: 10.1111/j.1439-0426.2012.01950.x
Poulin, R. (2007). Are there general laws in parasite ecology? Parasitology 134, 763–776. doi: 10.1017/s0031182006002150
Prati, S., and Henriksen, E. H. (2020). Seasonal dietary shifts enhance parasite transmission to lake salmonids during ice cover. Ecol. Evol. 10, 4031–4043. doi: 10.1002/ece3.6173
Prenter, J., MacNeil, C., Dick, J. T. A., and Dunn, A. M. (2004). Roles of parasites in animal invasions. Trends Ecol. Evol. 19, 385–390. doi: 10.1016/j.tree.2004.05.002
R Core Team (2019). R: A Language and Environment for Statistical Computing. Vienna: R Foundation for Statistical Computing.
Redding, J. M., and Schreck, C. B. (1983). Influence of ambient salinity on osmoregulation and cortisol concentration in yearling coho salmon during stress. Trans. Am. Fish. Soc. 112, 800–807. doi: 10.1577/1548-8659(1983)112<800:ioasoo>2.0.co;2
Ricker, W. E. (1975). Computation and Interpretation of Biological Statistics of Fish Populations. Bulletin of the Fisheries Research Board of Canada. Ottawa, ON: Fisheries and Marine Service.
Ricklefs, R. E., and Wikelski, M. (2002). The physiology/life-history nexus. Trends Ecol. Evol. 17, 462–468. doi: 10.1016/s0169-5347(02)02578-8
Rosa, R., and Seibel, B. A. (2010). Metabolic physiology of the Humboldt squid, Dosidicus gigas: implications for vertical migration in a pronounced oxygen minimum zone. Prog. Oceanogr. 86, 72–80. doi: 10.1016/j.pocean.2010.04.004
Seewagen, C. L. (2020). The threat of global mercury pollution to bird migration: potential mechanisms and current evidence. Ecotoxicology 29, 1254–1267. doi: 10.1007/s10646-018-1971-z
Shephard, S., and Gargan, P. (2017). Quantifying the contribution of sea lice from aquaculture to declining annual returns in a wild Atlantic salmon population. Aquac. Environ. Interact. 9, 181–192. doi: 10.3354/aei00223
Špakulová, M., Perrot-Minnot, M. J., and Neuhaus, B. (2011). Resurrection of Pomphorhynchus tereticollis (Rudolphi, 1809) (Acanthocephala: Pomphorhynchidae) based on new morphological and molecular data. Helminthologia 48, 268–277. doi: 10.2478/s11687-011-0038-y
Stefansson, S. O., Haugland, M., Björnsson, B. T., McCormick, S. D., Holm, M., Ebbesson, L. O. E., et al. (2012). Growth, osmoregulation and endocrine changes in wild Atlantic salmon smolts and post-smolts during marine migration. Aquaculture 362–363, 127–136. doi: 10.1016/j.aquaculture.2011.07.002
Stewart, H. A., Noakes, D. L. G., Cogliati, K. M., Peterson, J. T., Iversen, M. H., and Schreck, C. B. (2016). Salinity effects on plasma ion levels, cortisol, and osmolality in Chinook salmon following lethal sampling. Comp. Biochem. Physiol. A 192, 38–43. doi: 10.1016/j.cbpa.2015.11.011
Strand, R., and Finstad, B. (2007). Migratory behaviour in relation to smolt development and releasing strategies in Atlantic salmon (Salmo salar L.) smolts. Aquaculture 273, 277–283. doi: 10.1016/j.aquaculture.2007.10.010
Swanson, C., Young, P. S., and Cech, J. J. (2004). Swimming in two-vector flows: performance and behavior of juvenile chinook salmon near a simulated screened water diversion. Trans. Am. Fish. Soc. 133, 265–278. doi: 10.1577/03-068
Thorstad, E. B., Todd, C. D., Uglem, I., Bjørn, P. A., Gargan, P. G., Vollset, K. W., et al. (2015). Effects of salmon lice Lepeophtheirus salmonis on wild sea trout salmo trutta–a literature review. Aquac. Environ. Interact. 7, 91–113. doi: 10.3354/aei00142
Tierney, P. A., Caffrey, J. M., Vogel, S., Matthews, S. M., Costantini, E., and Holland, C. V. (2020). Invasive freshwater fish (Leuciscus leuciscus) acts as a sink for a parasite of native brown trout Salmo trutta. Biol. Invasions 22, 2235–2250. doi: 10.1007/s10530-020-02253-1
Urke, H. A., Arnekleiv, J. V., Nilsen, T. O., and Nilssen, K. J. (2014). Development of seawater tolerance and subsequent downstream migration in wild and stocked young-of-the-year derived Atlantic salmon Salmo salar smolts. J. Fish Biol. 84, 178–192. doi: 10.1111/jfb.12276
Valtonen, E. T., and Crompton, D. W. T. (1990). Acanthocephala in fish from the Bothnian Bay, Finland. J. Zool. 220, 619–639. doi: 10.1111/j.1469-7998.1990.tb04739.x
Wagner, G. N., McKinley, R. S., Bjørn, P. A., and Finstad, B. (2003). Physiological impact of sea lice on swimming performance of Atlantic salmon. J. Fish Biol. 62, 1000–1009. doi: 10.1046/j.1095-8649.2003.00091.x
Wanstall, S. T. (1984). A Study of the Pathobiology of Pomphorhynchus laevis Muller in Freshwater Fish. Ph. D. thesis. Derby: Derbyshire College of Further Education.
Wanstall, S. T., Robotham, P. W. J., and Thomas, J. S. (1986). Pathological changes induced by Pomphorbynchus laevis Muller (Acanthocephala) in the gut of rainbow trout, Salmo gairdneri Richardson. Parasitenkunde 72, 105–114. doi: 10.1007/bf00927741
Wanstall, S. T., Thomas, J. S., and Robotham, P. W. J. (1988). The pathology caused by Pomphorhynchus laevis Muller in the alimentary tract of the stone loach, Noemacheilus harbatulus (L.). J. Fish Dis. 11, 511–523. doi: 10.1111/j.1365-2761.1988.tb00750.x
Wedemeyer, G. A., Saunders, R. L., and Clarke, W. C. (1980). Environmental factors affecting smoltification and early marine survival of anadromous salmonids. Mar. Fish. Rev. 4, 1–14.
Wells, A., Grierson, C. E., MacKenzie, M., Russon, I. J., Reinardy, H., Middlemiss, C., et al. (2006). Physiological effects of simultaneous, abrupt seawater entry and sea lice (Lepeophtheirus salmonis) infestation of wild, sea-run brown trout (Salmo trutta) smolts. Can. J. Fish. Aquat. Sci. 63, 2809–2821. doi: 10.1139/f06-160
Whelan, K. F., Poole, W. R., McGinnity, P., Rogan, G., and Cotter, D. (1998). “The Burrishoole System,” in Studies of Irish Rivers and Lakes, Essays on the occasion of the XXVII Congress of Societas Internationalis Limnologiae (SIL), (Dublin: Congress of Societas Internationalis Limnologiae), 191–212.
Keywords: parasite, stress, osmoregulation, anadromy, salmonid, Pomphorhynchus tereticollis
Citation: Finlay RW, Poole R, Rogan G, Dillane E, Cotter D and Reed TE (2021) Hyper- and Hypo-Osmoregulatory Performance of Atlantic Salmon (Salmo salar) Smolts Infected With Pomphorhynchus tereticollis (Acanthocephala). Front. Ecol. Evol. 9:689233. doi: 10.3389/fevo.2021.689233
Received: 31 March 2021; Accepted: 20 July 2021;
Published: 16 August 2021.
Edited by:
Arne Hegemann, Lund University, SwedenReviewed by:
Henning Urke, INAQ AS, Trondheim, NorwayMarie-jeanne Perrot-minnot, Université de Bourgogne, France
Copyright © 2021 Finlay, Poole, Rogan, Dillane, Cotter and Reed. This is an open-access article distributed under the terms of the Creative Commons Attribution License (CC BY). The use, distribution or reproduction in other forums is permitted, provided the original author(s) and the copyright owner(s) are credited and that the original publication in this journal is cited, in accordance with accepted academic practice. No use, distribution or reproduction is permitted which does not comply with these terms.
*Correspondence: Ross W. Finlay, cm9maW5sYXlAdGNkLmll