- Department of Biological Sciences, Texas Tech University, Lubbock, TX, United States
Optimal migration theory is a framework used to evaluate trade-offs associated with migratory strategies. Two strategies frequently considered by migration theory are time minimizing, whereby migration is completed as quickly as possible, and energy minimizing, whereby migration is completed as energetically efficiently as possible. Despite extensive literature dedicated to generating analytical predictions about these migratory strategies, identifying appropriate study systems to empirically test predictions is difficult. Theoretical predictions that compare migratory strategies are qualitative, and empirical tests require that both time-minimizers and energy-minimizers are present in the same population; spring migrating silver-haired (Lasionycteris noctivagans) and hoary bats (Lasiurus cinereus) provide such a system. As both species mate in the fall, spring-migrating males are thought to be energy-minimizers while females benefit from early arrival to summering grounds, and are thought to be time-minimizers. Thermoregulatory expression also varies between species during spring migration, as female silver-haired bats and males of both species use torpor while female hoary bats, which implant embryos earlier, are thought to avoid torpor use which would delay pregnancy. Based on optimal migration theory, we predicted that female silver-haired bats and hoary bats would have increased fuel loads relative to males and the difference between fuel loads of male and female hoary bats would be greater than the difference between male and female silver-haired bats. We also predicted that females of both species would have a greater stopover foraging proclivity and/or assimilate nutrients at a greater rate than males. We then empirically tested our predictions using quantitative magnetic resonance to measure fuel load, δ13C isotope breath signature analysis to assess foraging, and 13C–labeled glycine to provide an indicator of nutrient assimilation rate. Optimal migration theory predictions of fuel load were supported, but field observations did not support the predicted refueling mechanisms, and alternatively suggested a reliance on increased fuel loads via carry-over effects. This research is the first to validate a migration theory prediction in a system of both time and energy minimizers and uses novel methodological approaches to uncover underlying mechanisms of migratory stopover use.
Introduction
Billions of birds and bats migrate annually to take advantage of seasonally abundant resources. Many species interrupt migratory movements with periodic stopovers which are critical to the overall success of migration as they provide opportunities to rest, refuel, and seek refuge. Despite their importance, stopovers often account for the majority of time and energy costs associated with migration (Hedenström and Alerstam, 1997; Wikelski et al., 2003) and in some cases could account for greater than 90% of the total energetic costs of migration (Clerc and McGuire, 2021). Thus, to fully understand migration ecology it is necessary to consider the consequences of stopover behavior.
One approach used to generate predictions of stopover behavior is optimal migration theory, which uses an optimization modeling framework to theoretically frame the adaptive value associated with varied migratory strategies (Alerstam and Lindström, 1990). The goal of optimal migration theory is to determine behavioral expressions that maximize fitness (Alerstam and Lindström, 1990). However, because fitness is difficult to quantify during migration, alternative currencies (i.e., fitness surrogates), such as time, energy, or predation risk, are considered to generate predictions about stopover behaviors (Alerstam and Lindström, 1990; Hedenström and Alerstam, 1995, 1997; Lank et al., 2003; Schmaljohann and Dierschke, 2005; Jonker et al., 2010). For example, time may be an appropriate currency if it is adaptive for a migrant to reach their destination as quickly as possible (i.e., time minimizer) (Hedenström and Alerstam, 1997; Hedenström, 2008). Conversely, energy may be an appropriate currency if a migrant experiences selective pressure to reduce the overall energetic costs of migration (i.e., energy minimizer) (Hedenström and Alerstam, 1997). One of the foundational predictions of optimal migration theory is that time minimizers, relative to energy minimizers, will accumulate a larger fuel load at stopover in response to variable fuel deposition rates (Alerstam and Lindström, 1990; Hedenström and Alerstam, 1997).
The mechanism by which migrants manipulate fuel deposition rates and achieve different stopover departure fuel loads depends on the migration theory currency being optimized. To minimize the total time of migration, migrants are expected to increase the net energy accumulation rate at stopover (Hedenström and Alerstam, 1995; Piersma and van Gils, 2011). Conversely, to achieve the minimum total energy of migration, migrants are predicted to maximize the stopover foraging gain ratio (i.e., maximize the gross amount of energy ingested per unit of energy expended; Hedenström and Alerstam, 1995; Hedenström, 2008; Piersma and van Gils, 2011). Given differences in how individuals treat foraging opportunities, time minimizers are predicted to maximize net intake rates by having greater foraging intensity and/or greater nutrient assimilation capacity (rate at which ingested food is converted into fuel) relative to energy minimizers (Houston and McNamara, 2013).
Predictions of stopover departure fuel load, and the mechanisms by which migrants manipulate fuel load at stopover, become more complex when considering the influence of varied thermoregulatory expressions. Many migrants employ a homeothermic pattern of temperature regulation to keep body temperature relatively constant even outside the thermoneutral zone (i.e., the range of ambient temperature (Ta) at which body temperature regulation is achieved without regulatory changes in metabolic heat production; IUPS Thermal Commission, 2003). In particular, as Ta drops below the thermoneutral zone, homeotherms increase their metabolic rate to produce excess heat (e.g., Cryan and Wolf, 2003; Wikelski et al., 2003; for a full treatment of different thermoregulatory strategies in migration see Clerc and McGuire, 2021). For homeotherms, a large proportion of the energetic cost associated with stopover can be attributed to the rapid use of endogenous fuel to remain euthermic via shivering and non-shivering thermogenesis (Hedenström and Alerstam, 1997; Wikelski et al., 2003). However, many species have the capacity to use a heterothermic pattern of temperature regulation during migration and may express hypometabolic states ranging from deep torpor heterothermy (Carpenter et al., 1993; Cryan and Wolf, 2003; McGuire et al., 2014) to facultative hypothermia (or rest-time hypothermia) (McKechnie and Lovegrove, 2002; Wojciechowski and Pinshow, 2009; Carere et al., 2010). When migrants enter hypometabolic states at stopover during non-active periods, they offset anywhere from 5 to >90% of the energetic cost associated with remaining euthermic, increasing fuel deposition rates (Carpenter et al., 1993; McGuire et al., 2014; Baloun and Guglielmo, 2019) and resulting in predictions of reduced stopover departure fuel load (Clerc and McGuire, 2021). Thus differences in thermoregulatory expressions introduce previously unconsidered mechanisms by which fuel loads can be manipulated (i.e., reducing resting costs rather than increasing intake rates) and can change the magnitude of the difference between predicted differences in stopover departure fuel loads between time and energy minimizers.
Empirically comparing migration theory predictions presents a challenge because predictions are qualitative and thus need to be tested in systems where relative differences can be detected. Identifying populations of migrants with seasonally unique migratory strategies is difficult, because for many species all individuals are under similar selective pressures (e.g., arrive to summering grounds to establish territories and breed). However, due to temporal differences in reproductive investment, spring migrating bats present an opportunity to test migration theory predictions under natural conditions. Silver-haired bats (Lasionycteris noctivagans) and hoary bats (Lasiurus cinereus) are examples of migratory bat species that exhibit mismatched reproductive investment between sexes. Copulation typically occurs in autumn (Racey, 1982; Cryan et al., 2012). Females store sperm throughout the winter and begin spring migration either continuing to store sperm or in early stages of gestation. Female hoary bat reproductive phenology is approximately 1–month advanced relative to silver-haired bats, and by mid-May (spring migration) hoary bats may have well developed embryos while silver-haired bats are just implanting (Druecker, 1972). In spring, females must arrive at summering grounds such that they can identify and occupy high quality maternity roosts prior to parturition and subsequent pup-rearing, with enough time for newborn pups to develop before autumn migration. Conversely, males incur the majority of reproductive costs during autumn (Druecker, 1972), and there is unlikely strong selection to arrive to summering grounds more quickly as it would provide little fitness advantage. Thus, it should be adaptive for females to complete migration as quickly as possible (time minimizers) and males to reduce the overall energetic cost of spring migration (energy minimizers).
In addition to sex differences in optimization currencies, hoary and silver-haired bats differ in thermoregulatory expressions throughout spring migration. Males of both species use torpor at stopover (Cryan and Wolf, 2003; McGuire et al., 2014), as do female silver-haired bats (Jonasson and Guglielmo, 2019). However, female hoary bats avoid torpor use during migration (Cryan and Wolf, 2003). Although capable of using torpor while pregnant (Willis et al., 2006), female hoary bats forgo torpor use during the early stages of gestation, presumably as a strategy to ensure embryo development is not delayed (Cryan and Wolf, 2003; Willis et al., 2006). In contrast, Jonasson and Guglielmo (2019) found that silver-haired bats used torpor during spring migration. Torpor use by female silver-haired bats may be possible because of delayed implantation relative to hoary bats (Druecker, 1972), thus allowing torpor expression without trading-off embryo development.
These differences in reproductive phenology, across sex and species, lead to three migrant “types”: time-minimizing homeotherms (female hoary bats), time-minimizing heterotherms (female silver-haired bats), and energy-minimizing heterotherms (male silver-haired and hoary bats). Our objective was to use optimal migration theory to generate field predictions relating differences in fuel load and foraging tactic for the three migrant “types” and empirically field test the resulting predictions during the spring migratory period.
Materials and Methods
Study System
We captured silver-haired bats and hoary bats during the month of May from 2016 to 2018 at two sites in the Sandia-Manzano Mountains, New Mexico, United States. We captured most bats in 2016 and 2017 in David Canyon, Bernalillo County (34.99°N, 106.36°W). In 2018 we split capture time between David Canyon and San Pedro Creek (Bernalillo and Sandoval counties; 35.22°N 106.30°W) which is approximately 25 km North of David Canyon. Unfortunately, little is known about the origins and final destinations of the bats that use this stopover site. For hoary bats, historic capture rates are highest during the spring migratory period and then decrease throughout the summer (Findley and Jones, 1964; Druecker, 1972). Based on collection records, the site is likely near the beginning of the migratory journey as winter records of silver-haired bats and hoary bats exist for southern New Mexico (Cryan, 2003). Winter behavior is mostly unknown for both species, though at least some hoary bats remain semi-active throughout winter (Weller et al., 2016). Specific destinations are unknown, though hoary bats and silver-haired bats summer throughout a broad range of the continental interior (Cryan, 2003; Cryan et al., 2014; Hayes et al., 2015).
We captured bats in mist nets and placed them into cloth bags prior to processing. We recorded sex, body mass (± 0.1 g), and forearm length (± 0.1 mm) of each bat. We measured sunset Ta at our capture site for each capture occasion.
Generating Field Predictions
Based on the natural history of silver-haired and hoary bats, we hypothesized that females minimize time and males minimize the total energy cost of migration (for a full treatment of time and total energy cost of migration currencies, see Hedenström and Alerstam, 1997). We parameterized the currencies by sex and species to generate qualitative predictions of optimal fuel load (Figure 1). To determine how sensitive prediction outcomes were to changes in currency parameters we compared predictions across a range of mean ambient temperature, stopover settling costs, and daily energetic expenditures. While quantitative predictions varied, relative qualitative differences between males and females and the magnitude of the difference in fuel load between male and female hoary bats compared to the difference in fuel load between male and female silver-haired bats were robust. Qualitative predictions only changed when stopover ambient temperature, which ultimately drives differences in thermoregulatory related energy costs, approached the lower critical temperature of the thermoneutral zone (for a full treatment of the influence of thermoregulatory strategies on optimal migration theory predictions of fuel load see Clerc and McGuire, 2021). For these species the lower critical temperature is between 27 and 30°C (Cryan and Wolf, 2003; McGuire et al., 2014). We did not observe temperatures that high at our field site during this study, thus we believe our parameter values are reasonable and our qualitative predictions to be sound.
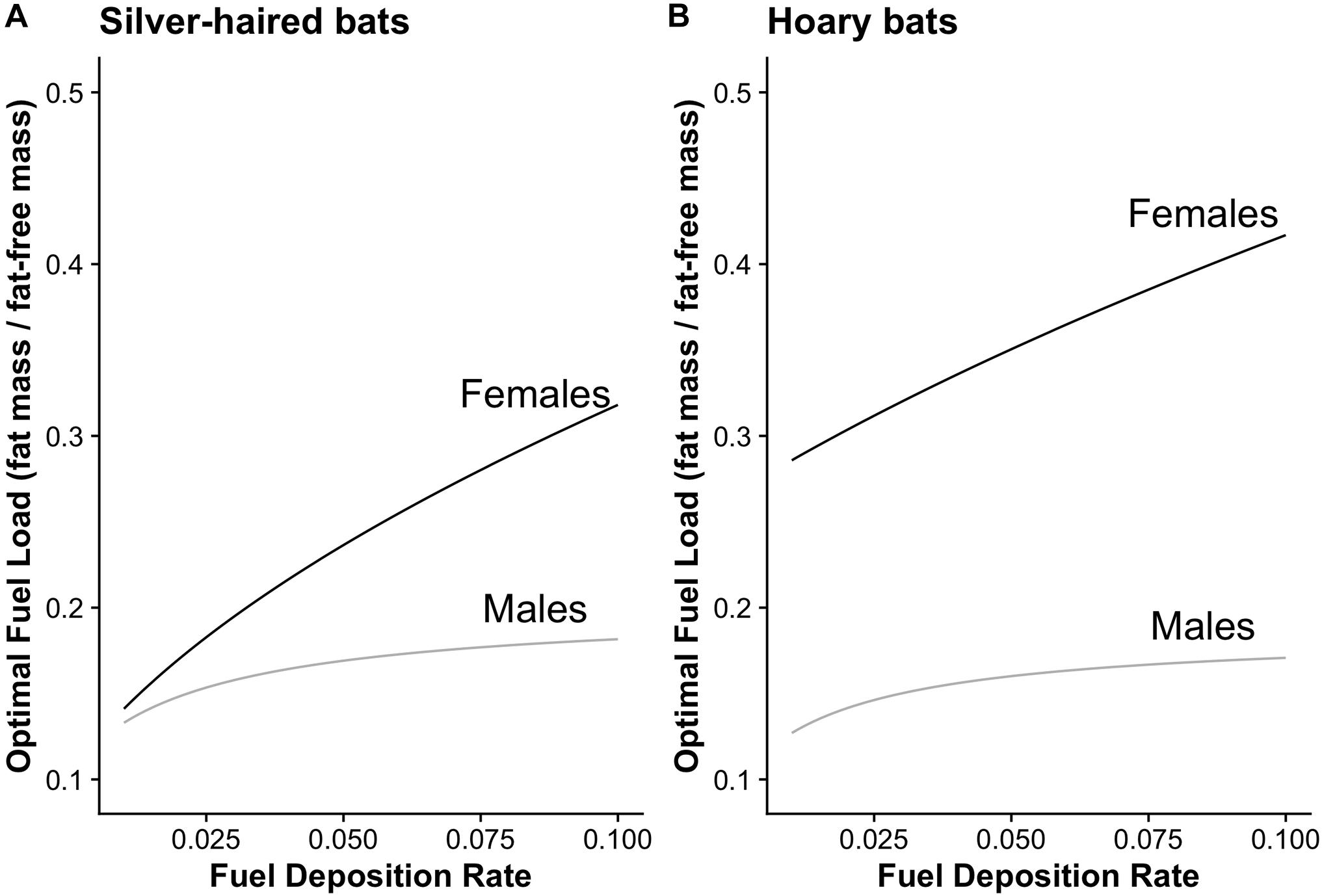
Figure 1. Qualitative predictions of optimal fuel load across a range of fuel deposition rates for silver-haired bats and hoary bats using time and energy minimizing strategies as well as heterothermic and homeothermic thermoregulatory strategies. (A) The optimal fuel load for female heterothermic time minimizing silver-haired bats (black line) is predicted to be greater than for male heterothermic energy minimizing silver-haired bats (gray line). (B) Similarly, female homeothermic time minimizing hoary bats (black line) are predicted to have greater optimal fuel load than male heterothermic energy minimizing hoary bats (gray line), with the further prediction that the observed difference would be greater than the difference for silver-haired bats.
Stopover Settling Costs and Daily Energy Expenditure
Predictions of optimal fuel load hinge on stopover settling costs (time and energy spent finding appropriate roosting and foraging locations upon arrival at the stopover site) as well as the fuel deposition rate achieved at stopover. We generated values of settling costs and daily energy expenditure following the methods of Hedenström and Alerstam (1997) and Clerc and McGuire (2021) by combining reasonable assumptions of bat stopover behavior with allometrically scaled mass-specific estimates of metabolic costs assuming mean daytime Ta = 15°C (mean daytime Ta at our field site). We further assumed bats arrive at a stopover site near dawn and required 20 min to find a suitable day roost, upon which they rested in the roost for the daytime period (14.5 h) before emerging the following evening resulting in a settling time cost of t0 = 0.6 days for all groups. Settling fuel load costs (expressed as a ratio of fat mass and lean mass) varied among groups due to differences in mass-specific metabolic rates and thermoregulatory mode with greatly reduced settling for groups using torpor. Female and male silver-haired bats had assumed settling fuel load cost of f0 = 0.007, male hoary bats had assumed settling fuel load cost of f0 = 0.006, and female hoary bats had an assumed settling fuel load cost of f0 = 0.037. Settling costs are a combination of the time and energy spent finding appropriate roosting and foraging locations upon arrival at the stopover site (i.e., time and energy spent before the start of active refueling).
To generate predictions of daily energy expenditure (MRfield) we summed energy costs associated with 2 h of flight and 22 h resting. In reality, settling cost and daily energy expenditure will change through time and space, however, qualitative predictions of fuel load are not overly sensitive to changes in daily energy expenditure parameters (Clerc and McGuire, 2021). For example if we assume 6 h of flight and 18 h resting, quantitative values will change but qualitative differences in predicted optimal departure fuel load between time and energy minimizers will remain relatively stable (Clerc and McGuire, 2021). We calculated metabolic rates using the mean body mass values for each group following the allometric scaling equations of Speakman and Thomas (2003) and used an oxyjoule equivalent of 20.09 (J mL O2−1) assuming a mixed fuel strategy (Speakman and Thomas, 2003; Lighton, 2018).
Field Predictions
We generated predictions of optimal fuel load as a function of stopover fuel deposition rate on a scale of 0.01 – 0.1 (where 0.1 represents a 10% daily increase in fuel mass) (Figure 1). For silver-haired bats we predicted females (time-minimizing heterotherm) would have greater fuel load than males (energy-minimizing heterotherm; Figure 1A). For hoary bats we predicted females (time-minimizing homeotherm) would have a greater fuel load than males (energy-minimizing heterotherm; Figure 1B). Furthermore, we predicted that the difference in fuel load between male and female hoary bats would be greater than the difference in silver-haired bats (Figure 1).
To achieve increased fuel loads we tested migration theory predictions that female hoary bats and female silver-haired bats conform to a foraging tactic of maximum net intake and thus would have an increased foraging proclivity and/or nutrient assimilation rate relative to males of both species. We predicted males of both species to conform to a foraging tactic of maximum efficiency.
Fuel Load
We measured fat mass (2016 and 2018 only) with quantitative magnetic resonance body composition analysis following the methods of McGuire and Guglielmo (2010). We supplemented our dataset with previously published (McGuire et al., 2013) fat and body mass data on 15 female and 15 male hoary bats captured at the same site during the same month. We express fuel load as a ratio of fat mass and lean mass. We considered lean mass to be fat-free mass and thus calculated fuel load as fat mass/(body mass – fat mass).
Foraging Assay: δ13C Isotope Breath Signature
We measured the δ13C isotope signature of the exhaled breath as an indicator of recent foraging activity (Voigt and Speakman, 2007). More enriched breath isotope signatures indicate a greater reliance on recently ingested prey for current metabolic demands, while depleted signatures indicate reliance on endogenous energy stores (Voigt et al., 2012). Therefore, this method can be used to determine which groups have greater foraging proclivity. We acquired breath samples following the methods of Voigt and Speakman (2007) and Voigt et al. (2012). If target species were captured and extracted from mist nets within 3 min (to avoid potential capture stress effects) we placed bats into a 500 ml glass container with a gas entry port connected to a tank of CO2-free air, an exit valve, and a breath sample port. With the bat in the container, we pumped CO2-free air through the container for 60 s to remove ambient CO2 (a flow time empirically determined to evacuate containers of detectable CO2 in the lab prior to field data collection). We then allowed exhaled breath to accumulate in the container for 240 s such that the CO2 concentration reached 4,000 – 6,000 ppm, and collected the breath sample by penetrating the septum of two 13 mL exetainers (Labco Ltd, United Kingdom). Samples were stored at room temperature for up to 3 months prior to analysis on a CO2 isotope analyzer run in batch mode (model CCIA-38-EP; LGR, Los Gatos, United States).
Nutrient Assimilation Rate Assay
To determine how rapidly bats oxidized recently ingested nutrients we fed fasted bats 13C-labeled glycine (Glycine-1-13 C 99%, Sigma-Aldrich, United States) and measured the change in δ13C of exhaled breath over a period of at least 45 min post-ingestion. We recorded the enrichment of exhaled breath at 10 min post-ingestion (arbitrarily selected common time point at which enrichment was rapidly increasing for all individuals) as an index of nutrient assimilation rate. Glycine is a non-essential (dispensable) amino acid that is preferentially metabolized by mammals, compared with essential (indispensable) amino acids, carbohydrates that can be stored as glycogen, or fat that can be stored in adipocytes (Welch et al., 2015). The rate at which a fasted bat assimilates ingested glycine can thus act as a proxy for the functional capacity of the gut under the assumption that there could be differences in the size of the gut (McGuire et al., 2013) or the density of transporters. Either increased gut surface area or greater density of transporters could lead to increased rate of appearance of oxidized glycine, allowing the rate of appearance to act as an index of gut functional capacity. Among Vespertilionid bats, sexual size dimorphism in body size (female > male) is common (Williams and Findley, 1979), and must be accounted for in statistical analyses (see below). Size dimorphism is especially pronounced for hoary bat females that may weigh ∼30 – 50% more than males. Larger females presumably have larger digestive tracts, and therefore, all else being equal, should have increased rate of 13C-labeled glycine appearance in the breath.
We randomly selected up to 6 individuals each night (prioritizing females due to male bias at our capture site) for the glycine assay [n = 64 silver-haired (2017 only) and n = 35 hoary bats (2017–2018)]. Bats were first fasted for at least 90 min to ensure that individuals voided recently ingested prey that may have interfered with their ability to digest and assimilate glycine (Buchler, 1975). We fed each individual 5 mg of 13C-labeled glycine (Sigma Aldrich, United States) suspended in 200 μl of water. We placed up to 2 individuals in separate respirometry chambers and ran 0.60 L min–1 of CO2-free air through the chambers and into the isotope analyzer. Flow-through mode acquired a semi-continuous reading of the δ13C, using a flow multiplexer (Sable Systems, United States) to switch between each chamber every 30 s, such that we were able to document the rate of appearance of 13C enriched breath. We provided mealworms to bats following all measurements and released all bats at the capture site.
Statistical Analysis
All analyses were completed with the program R (v 3.5.1; R Core Team, 2018). To test predictions of sex-based differences in fuel load, and that the difference would be greater in hoary bats than silver-haired bats, we used a linear model including sex, species, and the interaction between the two. We compared δ13C isotope signature of exhaled breath with linear models including body mass, Ta (measured at sunset), and capture year as covariates. Because there is little overlap in body mass between male and female hoary bats, sex and body mass are confounded so we did not include mass in our initial model and then separately tested the effect of mass on 13C isotope enrichment within each sex. We used a linear model to test for the effects of sex on assimilation rate, controlling for mass, capture date, and year. As above, we tested for the effect of mass within sexes. We used a backward stepwise model selection procedure for all linear models, sequentially removing non-significant terms until only significant terms remained. Because there is very little overlap in the body mass between sexes of hoary bats, sex and body mass are confounded so for both the digestive assay and glycine assay hoary bat analyses we first omitted mass in our models and then separated the sexes and tested mass effects on δ13C isotope signature within males and females. We report Cohen’s effect size values for all significant sex comparisons. All values are reported as mean ± s.e.m.
Results
Fuel Load
Female silver-haired bats (n = 85) had a fuel load (0.11 ± 0.004) that was 34% greater (F1,283 = 27.17, p < 0.001, Cohen’s effect size d = 0.67) than male (n = 200) fuel load (0.082 ± 0.002) (Figure 2A). Similarly, female hoary bats (n = 45) had a fuel load (0.17 ± 0.008) that was 42% greater (F1,72 = 21.97, p < 0.001, Cohen’s effect size d = 1.12) than male (n = 29) fuel load (0.12 ± 0.007) (Figure 2B). The difference between female and male hoary bat fuel load was 79% greater than the difference between female and male silver-haired bats (F1,355 = 7.96, p = 0.005).
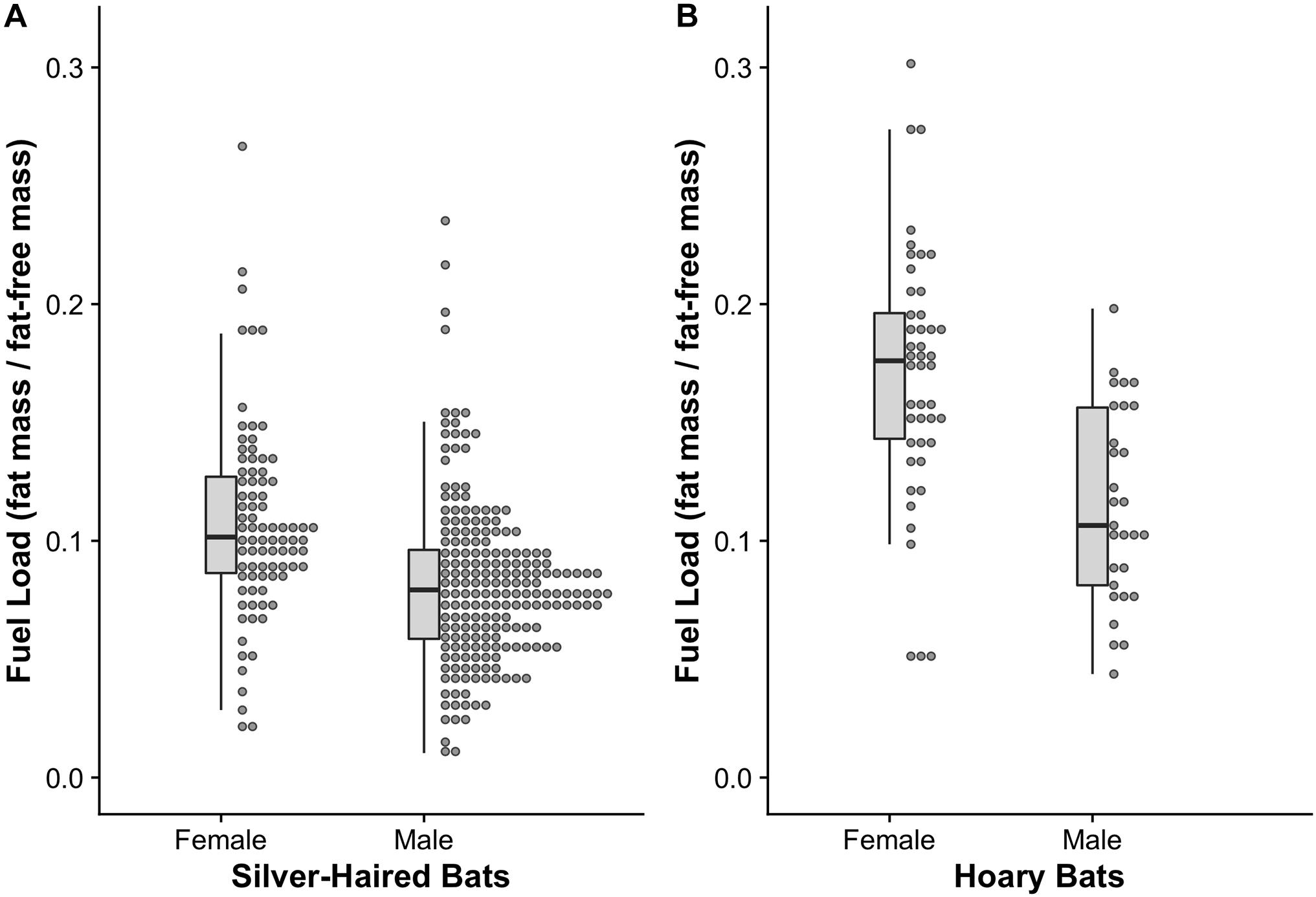
Figure 2. Fuel loads of (A) spring migrating silver-haired bats (Lasionycteris noctivagans) and (B) hoary bats (Lasiurus cinereus). In both species, female fuel loads were greater than male fuel loads, consistent with optimal migration theory predictions based on females adopting a time-minimizing strategy and males adopting an energy minimizing strategy. Furthermore, the difference in fuel load is greater in hoary bats than silver-haired bats, consistent with predictions based on homeothermic female hoary bats and heterothermy among all others.
Foraging Assay: δ13C Isotope Breath Signature
Our results suggest that females and males of both study species forage during stopover (Figure 3). Female silver-haired bats (n = 132) had a mean δ13C isotope signature of -27.09 ± 0.18 ‰ and male silver-haired bats (n = 67) had a mean isotope signature of −26.79 ± 0.13 ‰ (Figure 3A). Male and female silver-haired bats did not differ in mean breath sample δ13C enrichment (F1,181 = 0.29, p = 0.588), nor did sample 13C enrichment vary across years (F1,182 = 2.16, p = 0.143). We detected a negative association of foraging with body mass (F1,183 = 6.15, p = 0.014), suggesting that individuals with lower mass were more likely to forage. We also detected a positive association of foraging with Ta (F1,183 = 14.12, p < 0.001), which aligns with prey availability increases as a function of Ta (Anthony and Kunz, 1977).
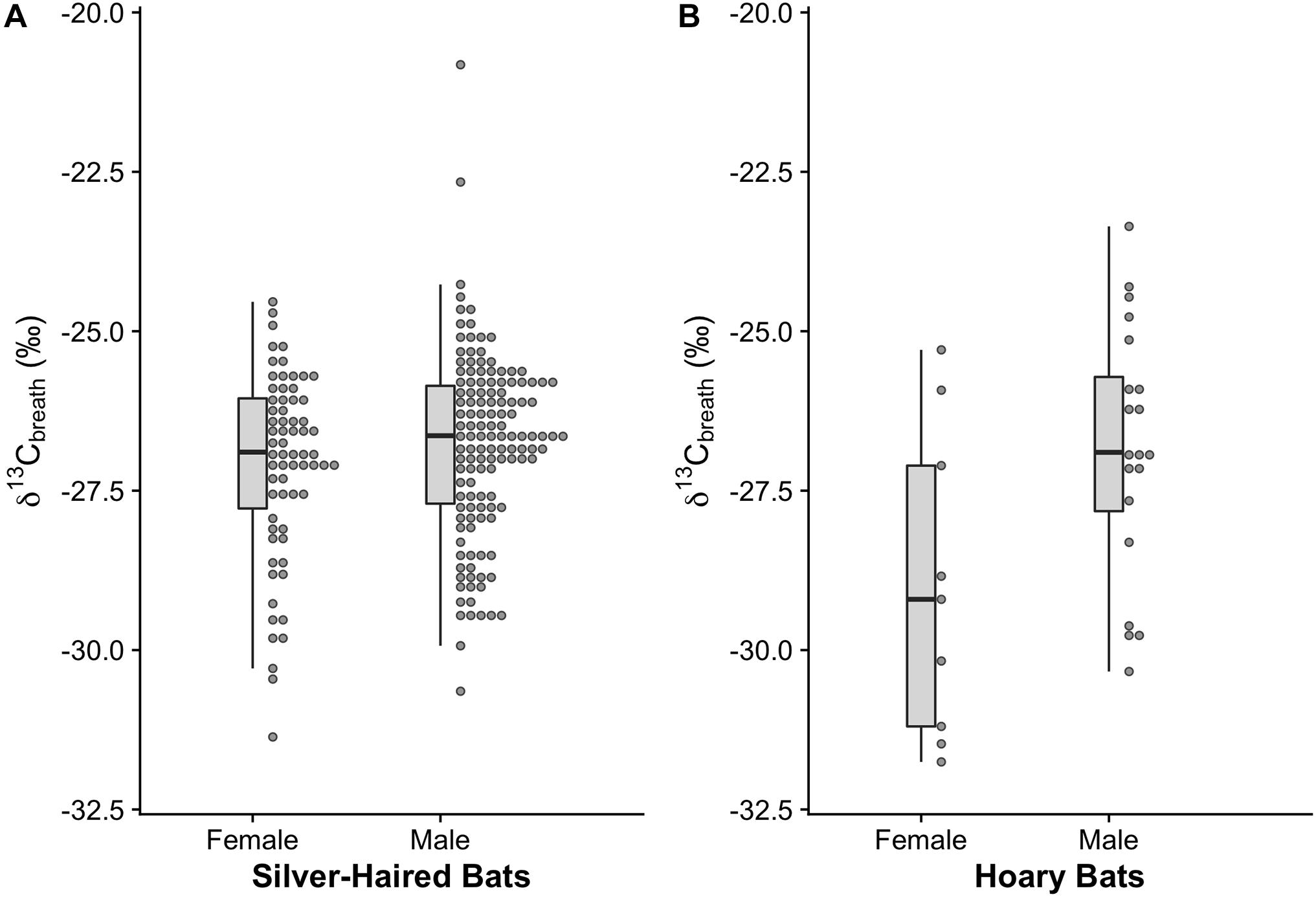
Figure 3. 13C enrichment in exhaled breath of spring migrating silver-haired and hoary bats. Less negative δ13C values are associated with metabolism of exogenous nutrients (foraging) and more negative δ13C values are associated with metabolism of endogenous fuel sources (relying on stored fat). Using relative 13C enrichment as a proxy for foraging proclivity we find that (A) female and male silver-haired bats do not show differences in their proclivity to forage at stopover but that (B) female hoary bats are less likely to forage relative to male hoary bats.
Female hoary bats (n = 9) had a more depleted δ13C breath signature (−28.99 ± 0.81 ‰) than male hoary bats (n = 20; −26.84 ± 0.44 ‰; F1,27 = 6.43, p = 0.017; Cohen’s effect size, d = −1.02) (Figure 3B) signifying a greater reliance on endogenous fuel stores rather than recently ingested prey (Voigt et al., 2012; Baloun et al., 2020). Further, we did not find any effect of body mass on foraging proclivity for males (F1,27 = 0.03, p = 0.865) or females (F1,27 = 0.82, p = 0.396) suggesting a real effect of sex on foraging proclivity and that females are less reliant on recently ingested exogenous nutrients at our capture site than males. We did not detect any effects of year (F1,25 = 0.23, p = 0.632), or Ta (F1,25 = 0.99, p = 0.329) on hoary bat foraging proclivity.
Nutrient Assimilation Rate Assay
Glycine assimilation varied widely between the sexes of both species (Figure 4). There was no difference in mean glycine assimilation rate between male (72.68 ± 3.46 ‰/min; n = 45) and female (63.06 ± 6.29 ‰/min; n = 19) silver-haired bats (F1,61 = 0.85, p = 0.360) (Figure 4A). Further, there was no effect of mass on glycine assimilation rate (F1,60 = 0.01, p = 0.919). Glycine assimilation rate was positively related to capture date (F1,62 = 18.42, p < 0.001), suggesting that either late migrants have greater gut functional capacity or that functional capacity increases over the course of migration.
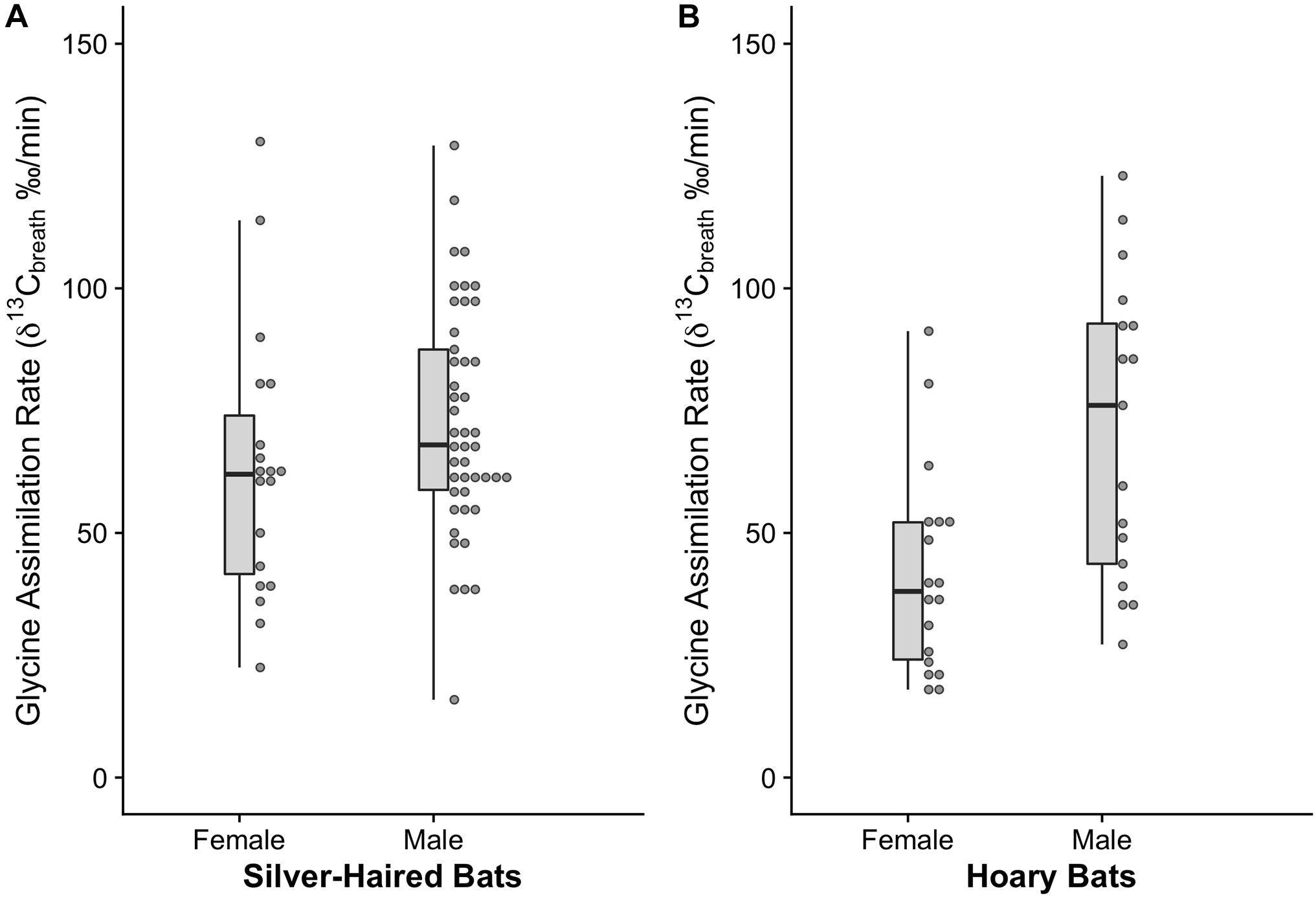
Figure 4. 13C enrichment 10 min post-ingestion of 13C-labeled glycine (indicator of assimilation rate) in spring migrating silver-haired (Lasionycteris noctivagans) and hoary bats (Lasiurus cinereus). (A) Enrichment did not differ between female and male silver-haired bats, but (B) female hoary bats had depleted 13C relative to males, suggesting reduced digestive capacity.
Female hoary bats had a lower rate of 13C-labeled glycine assimilation (41.79 ± 4.97 ‰/min, n = 18) relative to males (71.436 ± 7.45 ‰/min; n = 17; F1,33 = 11.20, p = 0.002) and the difference was associated with a large effect size (Cohen’s effect size, d = −1.13) (Figure 4B). There was no effect of body mass on the rate of apparent oxidation of 13C-labeled glycine for males (F1,15 = 0.001, p = 0.970) or females (F1,16 = 0.07, p = 0.792). The rate of apparent oxidation of 13C-labeled glycine was not affected by capture date (F1,31 = 0.16, p = 0.691) or year (F1,32 = 2.06, p = 0.161).
Discussion
Our results are consistent with the migration theory predictions that females would have increased fuel loads relative to males and the difference between fuel loads between male and female hoary bats would be greater than the difference between male and female silver-haired bats. Fuel loads in both species qualitatively conformed with a time minimizing hypothesis for females and an energy minimizing hypothesis for males. Furthermore, relative differences in fuel load conform to the predicted effects of thermoregulatory expressions.
The prediction that female time minimizers maximize net energy intake and male energy minimizers maximize the foraging gain ratio at stopover was not supported. Despite increased fuel loads in females of both species, we found no evidence that females deposited more fuel than males at our capture site, either through increased foraging or increased digestive efficiency. We found no difference in foraging proclivity or nutrient assimilation in male and female silver-haired bats, and counter to our predictions found lower foraging intensity and nutrient assimilation in female hoary bats relative to males. The observed patterns of foraging and nutrient assimilation at our capture site are potentially explained by an inability to achieve positive energy balance (i.e., our study site may have been a low-quality stopover site), or that both sexes use the same foraging tactic (maximize net energy intake or maximize the foraging gain ratio). Explanations of the observed differential fuel loads then may include greater reliance on ephemeral blooms of high prey density, and/or reliance on carryover fuel from the pre-migratory period (see below).
Migration theory assumes that stopovers are used for refueling only when individuals can achieve positive energy balance. Unlike nocturnally migrating birds that can forage during the day and migrate at night, bats must manage both refueling and migratory flights during nocturnal periods, undoubtedly leading to foraging period time constraints. The challenges of gaining positive energy balance in a constrained time period are likely exacerbated when foraging quality is low. Torpor use may allow some migrants to overcome these constraints and build fuel at stopover, however, maintaining euthermia [i.e., as observed in pregnant hoary bats, Cryan and Wolf (2003)] may tip stopover energetics into negative balance across a large proportion of stopovers during migration. Increased stopover costs coupled with reduced foraging proclivity and a decrease in gut functional capacity suggest female hoary bats may not achieve positive energy balance on most occasions at our field site and may forgo foraging in favor of rapid departure. That females are also pregnant may further inhibit individual ability to achieve positive energy balance as increases in mass may make foraging flight energetically prohibitive unless prey availability and quality is high. Foraging proclivity, as indicated by 13C enrichment in the breath, was low but variable in female hoary bats, suggesting opportunistic foraging may be a tactic used to mitigate rapid loss of endogenous fuel stores.
In our study, groups that presumably use torpor during migration exhibited a greater foraging proclivity than those that forgo torpor use. However, in the case of silver-haired bats there was no evidence that males and females used different foraging tactics. In conditions of low foraging quality and foraging time constraints, it is possible that maximizing net intake is the only foraging tactic that allows migrants to achieve positive energy balance. If this is the case, we might expect that rather than exhibiting a fixed foraging tactic throughout migration, individuals will adjust their foraging habits based on prey availability and time constraints. In conditions of high prey availability, males may switch tactic to maximize stopover foraging ratio, however, it is unclear if females would do the same or continue to maximize the net intake rate, as time-minimizers are predicted to. A logical extension of this research will be to sample across a wider range of habitat foraging qualities, to determine if males switch tactics to maximize efficiency when foraging quality is high, and if females maintain a net intake foraging tactic.
Females had greater fuel loads than males, but foraged less and did not have greater digestive efficiency. This begs the question of how females deposit or maintain greater fuel loads. One explanation is that females rely on ephemeral pulses of extreme prey abundance (Valdez and Cryan, 2009). Valdez and Cryan (2009) found that Geometrid and Noctuid moths make up the majority of hoary bat diets during spring migration at our study site. Females may only reach positive energy balance under foraging conditions where they encounter either emerging diapausal moths or migratory moths as they too move northward along the east slope of the Rocky Mountains (Valdez and Cryan, 2009; Krauel et al., 2015, 2017). When encountered, abundant moths would offer a more ephemeral, but substantial, foraging opportunity than might be encountered on average across the landscape. At our capture site we observed one such diapause emergence, where the ground surrounding the capture site was blanketed in presumably newly emerged Geometrid moths. In nearly 80 capture nights we have only encountered one such emergence, reinforcing the fact that bat prey is spatiotemporally ephemeral during spring migration.
Reliance on ephemeral prey pulses as the primary means of building large fuel loads is an inherently risky tactic. To consistently achieve increased fuel loads in this manner we would predict that gut functional capacity would be preemptively maintained at a high-performance level such that when pulses of high-density prey are encountered, individuals can take full advantage of their energic benefits. However, our nutrient assimilation assay suggested that females did not physiologically anticipate encounters with high density foraging opportunities. Females likely seek out high density pulses of prey, but there may be a trade-off in maintaining excess functional capacity in the gut (and the flight cost of carrying that extra load) that precludes maintaining high digestive efficiency throughout migration (Piersma and Gill, 1998; McGuire et al., 2013).
Another mechanism that would account for differential fuel loads is that females carry-over fuel from the overwintering period into spring migration. Unfortunately, little is known about the overwinter ecology of these species (Weller et al., 2016), so the feasibility of either increasing winter energy intake/reducing energy output relative to males or exhibiting pre-migratory hyperphagia is unknown. Despite gaps in the natural history, it is likely that overwintering behavior plays an important role in spring migration.
Theoretical models suggest silver-haired bats could achieve travel distances of >1500 km on fuel loads similar to those observed in the present study (McGuire et al., 2012). Thus, with maximum net migration distances of 1000 – 2000 km (Cryan, 2003; Cryan et al., 2014), hoary bat females may be able to arrive at summer grounds with minimal stopover refueling. However, if fuel stores become depleted, due to tortuous routing, or extended periods of inclement weather, and individuals can’t locate high-density foraging opportunities, female hoary bats still have the ability to use torpor to save energy in emergency situations (Willis et al., 2006).
Our study highlights the strengths and limitations in generating predictions of stopover behavior under an optimal migration theory framework. Our target species appear to conform to the migration theory predictions of differential fuel load, however, we are unable to conclusively identify the mechanism by which differential fuel loads are achieved. In the case of migratory bat systems, we may need greater flexibility in the framework to incorporate previously unaccounted for mechanisms of fuel accumulation. Migration theory has been effectively used to generate predictions of stopover physiology and behavior, and with the extension of migration theory to account for heterothermic thermoregulatory strategies the framework can be used for an ever more diverse range of migrants. Empirical tests are critical to evaluate model predictions and determine how well the models fit observed patterns of migration. Through an iterative process of modeling and empirical testing we can refine migration theory to develop a comprehensive understanding of the ecological patterns of migration.
Data Availability Statement
The raw data supporting the conclusions of this article will be made available by the authors, without undue reservation.
Ethics Statement
All procedures were approved by the Texas Tech University Institutional Animal Care and Use Committee (protocols 16013-04 and 19043-05) and permits from the New Mexico Department of Game and Fish (permit 3424).
Author Contributions
JC and LM contributed to conception and design of the study and performed the statistical analysis. JC, ER, and LM contributed to data collection. JC wrote the first draft of the manuscript. All authors contributed to manuscript revision, read, and approved the submitted version.
Funding
This research was supported by funds from the Texas Tech University and University of Waterloo to LM and student research grants to JM from the Animal Behavior Society and American Society of Mammalogists.
Conflict of Interest
JC is currently employed by the company Normandeau Associates Inc.
The remaining authors declare that the research was conducted in the absence of any commercial or financial relationships that could be construed as a potential conflict of interest.
Publisher’s Note
All claims expressed in this article are solely those of the authors and do not necessarily represent those of their affiliated organizations, or those of the publisher, the editors and the reviewers. Any product that may be evaluated in this article, or claim that may be made by its manufacturer, is not guaranteed or endorsed by the publisher.
Acknowledgments
We would like to thank Nate Fuller, Amie Sommers, and Emma Kunkel for their help in the field. A previous version of this manuscript appeared in the dissertation of JC (Clerc, 2020).
References
Alerstam, T., and Lindström, Å (1990). “Optimal bird migration: the relative importance of time, energy, and safety,” in Bird Migration, ed. E. Gwinner (Heidelberg: Springer), 331–351. doi: 10.1007/978-3-642-74542-3_22
Anthony, E. L., and Kunz, T. H. (1977). Feeding strategies of the little brown bat, Myotis lucifugus, in southern New Hampshire. Ecology 58, 775–786. doi: 10.2307/1936213
Baloun, D. E., and Guglielmo, C. G. (2019). Energetics of migratory bats during stopover: a test of the torpor-assisted migration hypothesis. J. Exp. Biol. 222:jeb196691.
Baloun, D. E., Hobson, K. A., and Guglielmo, C. G. (2020). Temporal patterns of foraging by silver-haired bats during migratory stopover revealed by isotopic analyses (δ 13 C) of breath CO 2. Oecologia 193, 67–75. doi: 10.1007/s00442-020-04650-8
Buchler, E. R. (1975). Food transit time in Myotis lucifugus chiroptera: vespertilionidae. J. Mammal. 56, 252–255. doi: 10.2307/1379630
Carere, C., Costantini, D., Fusani, L., Alleva, E., and Cardinale, M. (2010). Hypothermic abilities of migratory songbirds at a stopover site. Rend. Lincei 21, 323–334. doi: 10.1007/s12210-010-0094-0
Carpenter, F. L., Hixon, M. A., Beuchat, C. A., Russell, R. W., and Paton, D. C. (1993). Biphasic mass gain in migrant hummingbirds: body composition changes, torpor, and ecological significance. Ecology 74, 1173–1182. doi: 10.2307/1940487
Clerc, J. (2020). Theoretical and Empirical Investigation of the Influence of Heterothermy on Bat Migration Ecology. Doctoral dissertation. Lubbock, TX: Texas Tech University.
Clerc, J., and McGuire, L. P. (2021). Considerations of varied thermoregulatory expressions in migration theory. Oikos (in press).
Cryan, P. M. (2003). Seasonal distribution of migratory tree bats (Lasiurus and Lasionycteris) in North America. J. Mammal., 84, 579–593. doi: 10.1644/1545-1542(2003)084<0579:SDOMTB>2.0.CO;2
Cryan, P. M., Jameson, J. W., Baerwald, E. F., Willis, C. K. R., Barclay, R. M. R., Snider, E. A., et al. (2012). Evidence of late-summer mating readiness and early sexual maturation in migratory tree-roosting bats found dead at wind turbines. PLoS One 7:e47586. doi: 10.1371/journal.pone.0047586
Cryan, P. M., Stricker, C. A., and Wunder, M. B. (2014). Continental-scale, seasonal movements of a heterothermic migratory tree bat. Ecol. Appl. 24, 602–616. doi: 10.1890/13-0752.1
Cryan, P. M., and Wolf, B. O. (2003). Sex differences in the thermoregulation and evaporative water loss of a heterothermic bat, Lasiurus cinereus, during its spring migration. J. Exp. Biol. 206, 3381–3390. doi: 10.1242/jeb.00574
Druecker, J. D. (1972). Aspects of Reproduction in Myotis Volans, Lasionycteris Noctivagans, and Lasiurus Cinereus. Doctoral dissertation. Albuquerque, NM: University of New Mexico.
Findley, J. S., and Jones, C. (1964). Seasonal distribution of the hoary bat. J. Mammal. 45, 461–470. doi: 10.2307/1377421
Hayes, M. A., Cryan, P. M., and Wunder, M. B. (2015). Seasonally-dynamic presence-only species distribution models for a cryptic migratory bat impacted by wind energy development. PLoS One 10:e0132599. doi: 10.1371/journal.pone.0132599
Hedenström, A. (2008). Adaptations to migration in birds: behavioural strategies, morphology and scaling effects. Philos. Trans. R. Soc. B Biol. Sci. 363, 287–299. doi: 10.1098/rstb.2007.2140
Hedenström, A., and Alerstam, T. (1995). Optimal flight speed of birds. Philos. Trans. R. Soc. B Biol. Sci. 348, 471–487. doi: 10.1098/rstb.1995.0082
Hedenström, A., and Alerstam, T. (1997). Optimum fuel loads in migratory birds: distinguishing between time and energy minimization. J. Theor. Biol. 189, 227–234. doi: 10.1006/jtbi.1997.0505
Houston, A. I., and McNamara, J. M. (2013). Foraging currencies, metabolism and behavioural routines. J. Anim. Ecol. 83, 30–40. doi: 10.1111/1365-2656.12096
IUPS Thermal Commission (2003). IUPS thermal commission glossary of terms for thermal physiology. J. Therm. Biol. 28, 75–106. doi: 10.1016/s0306-4565(02)00055-4
Jonasson, K. A., and Guglielmo, C. G. (2019). Evidence for spring stopover refuelling in migrating silver-haired bats (Lasionycteris noctivagans). Can. J. Zool. 97, 961–970. doi: 10.1139/cjz-2019-0036
Jonker, R. M., Eichhorn, G., vanLangevelde, F., and Bauer, S. (2010). Predation danger can explain changes in timing of migration: the case of the barnacle goose. PLoS One 5:e11369. doi: 10.1371/journal.pone.0011369
Krauel, J. J., Brown, V. A., Westbrook, J. K., and McCracken, G. F. (2017). Predator–prey interaction reveals local effects of high-altitude insect migration. Oecologia 186, 49–58. doi: 10.1007/s00442-017-3995-0
Krauel, J. J., Westbrook, J. K., and McCracken, G. F. (2015). Weather–driven dynamics in a dual–migrant system: moths and bats. J. Anim. Ecol. 84, 604–614. doi: 10.1111/1365-2656.12327
Lank, D. B., Butler, R. W., Ireland, J., and Ydenberg, R. C. (2003). Effects of predation danger on migration strategies of sandpipers. Oikos 103, 303–319. doi: 10.1034/j.1600-0706.2003.12314
Lighton, J. R. (2018). Measuring Metabolic Rates: A Manual for Scientists. Oxford: Oxford University Press.
McGuire, L. P., and Guglielmo, C. G. (2010). Quantitative magnetic resonance: a rapid, noninvasive body composition analysis technique for live and salvaged bats. J. Mammal. 91, 1375–1380. doi: 10.1644/10-MAMM-A-051.1
McGuire, L. P., Fenton, M. B., and Guglielmo, C. G. (2013). Phenotypic flexibility in migrating bats: seasonal variation in body composition, organ sizes and fatty acid profiles. J. Exp. Biol. 216, 800–808. doi: 10.1242/jeb.072868
McGuire, L. P., Jonasson, K. A., and Guglielmo, C. G. (2014). Bats on a budget: torpor-assisted migration saves time and energy. PLoS One 9:e115724. doi: 10.1371/journal.pone.0115724
McKechnie, A. E., and Lovegrove, B. G. (2002). Avian facultative hypothermic responses: a review. Condor 104, 705–724. doi: 10.1093/condor/104.4.705
Piersma, T., and Gill, R. E. Jr. (1998). Guts don’t fly: small digestive organs in obese bar-tailed godwits. Auk 115, 196–203. doi: 10.2307/4089124
Piersma, T., and van Gils, J. A. (2011). The Flexible Phenotype: A Body-Centered Integration of Ecology, Physiology, and Behaviour. Oxford: Oxford University Press.
R Core Team (2018). R: A Language and Environment for Statistical Computing. R Foundation for Statistical Computing. Vienna: R Core Team.
Racey, P. A. (1982). “Ecology of bat reproduction,” in Ecology of Bats, ed. T. H. Kunz (Boston, MA: Springer), 57–104. doi: 10.1007/978-1-4613-3421-7_2
Schmaljohann, H., and Dierschke, V. (2005). Optimal bird migration and predation risk: a field experiment with northern wheatearsOenanthe oenanthe. J. Anim. Ecol. 74, 131–138. doi: 10.1111/j.1365-2656.2004.00905
Speakman, J. R., and Thomas, D. W. (2003). “Physiological ecology and energetics of bats,” in Ecology of Bat, eds T. H. Kunz and M. B. Fenton (Chicago: University of Chicago Press), 430–490.
Valdez, E. W., and Cryan, P. M. (2009). Food habits of the hoary bat (Lasiurus cinereus) during spring migration through New Mexico. Southwest. Nat. 54, 195–200. doi: 10.1894/ps-45.1
Voigt, C. C., Sörgel, K., Šuba, J., Keišs, O., and Pçtersons, G. (2012). The insectivorous bat Pipistrellus nathusii uses a mixed-fuel strategy to power autumn migration. Proc. R. Soc. B Biol. Sci. 279, 3772–3778. doi: 10.1098/rspb.2012.0902
Voigt, C. C., and Speakman, J. R. (2007). Nectar-feeding bats fuel their high metabolism directly with exogenous carbohydrates. Funct. Ecol. 21, 913–921. doi: 10.1111/j.1365-2435.2007.01321.x
Welch, K. C. Jr., Péronnet, F., Hatch, K. A., Voigt, C. C., and McCue, M. D. (2015). Carbon stable-isotope tracking in breath for comparative studies of fuel use. Ann. N. Y. Acad. Sci. 1365, 15–32. doi: 10.1111/nyas.12737
Weller, T. J., Castle, K. T., Liechti, F., Hein, C. D., Schirmacher, M. R., and Cryan, P. M. (2016). First direct evidence of long-distance seasonal movements and hibernation in a migratory bat. Sci. Rep. 6, 1–7. doi: 10.1038/srep34585
Wikelski, M., Tarlow, E. M., Raim, A., Diehl, R. H., Larkin, R. P., and Visser, G. H. (2003). Avian metabolism: costs of migration in free-flying songbirds. Nature 423, 704–705. doi: 10.1038/423704a
Williams, D. F., and Findley, J. S. (1979). Sexual size dimorphism in vespertilionid bats. Am. Midl. Nat. 102, 113–126. doi: 10.2307/2425072
Willis, C. K., Brigham, R. M., and Geiser, F. (2006). Deep, prolonged torpor by pregnant, free-ranging bats. Naturwissenschaften 93, 80–83. doi: 10.1007/s00114-005-0063-0
Keywords: optimal migration theory, thermoregulation, stopoverecology, physiological ecology, bats
Citation: Clerc J, Rogers EJ and McGuire LP (2021) Testing Predictions of Optimal Migration Theory in Migratory Bats. Front. Ecol. Evol. 9:686379. doi: 10.3389/fevo.2021.686379
Received: 26 March 2021; Accepted: 13 July 2021;
Published: 09 August 2021.
Edited by:
Arne Hegemann, Lund University, SwedenReviewed by:
Jeremy Ryan Shipley, Max Planck Institute of Ornithology, GermanyLara Keicher, Max Planck Institute of Animal Behaviour, Germany
Julia Nowack, Liverpool John Moores University, United Kingdom
Copyright © 2021 Clerc, Rogers and McGuire. This is an open-access article distributed under the terms of the Creative Commons Attribution License (CC BY). The use, distribution or reproduction in other forums is permitted, provided the original author(s) and the copyright owner(s) are credited and that the original publication in this journal is cited, in accordance with accepted academic practice. No use, distribution or reproduction is permitted which does not comply with these terms.
*Correspondence: Jeff Clerc, amVmZi5vbS5jbGVyY0BnbWFpbC5jb20=
†Present address: Jeff Clerc, Normandeau Associates Inc., Gainesville, FL, United States Elizabeth J. Rogers, Organismic and Evolutionary Biology, University of Massachusetts Amherst, Amherst, MA, United States Liam P. McGuire, Department of Biology, University of Waterloo, Waterloo, ON, Canada