- Institute of Evolutionary Ecology and Conservation Genomics, Ulm University, Ulm, Germany
Oligolectic bees are highly dependent on the availability of the host plants to which they are specialized. Nevertheless, females of Chelostoma rapunculi have recently been monitored occasionally to visit Malva moschata and Geranium sanguineum flowers, in addition to their well-known Campanula spp. hosts. The questions therefore arise which floral cues promote visits to non-host plants. As host-specific floral cues are key attractants for oligolectic bees, we have studied the attractiveness of olfactory and visual cues of the established host Campanula trachelium in comparison to the non-host plants G. sanguineum and M. moschata in behavioral experiments. Chemical and electrophysiological analyses of the floral scent and spectral measurements of floral colors were used to compare and contrast host and non-host plants. The behavioral experiments showed that foraging-naïve bees, in particular, were attracted by olfactory cues of the non-host plants, and that they did not favor the Campanula host scent in choice experiments. Many electrophysiologically active floral volatiles were present in common in the studied plants, although each species produced an individual scent profile. Spiroacetals, the key components that enable C. rapunculi to recognize Campanula hosts, were detected in trace amounts in Geranium but could not be proved to occur in Malva. The visual floral cues of all species were particularly attractive for foraging-experienced bees. The high attractiveness of G. sanguineum and M. moschata flowers to C. rapunculi bees and the floral traits that are similar to the Campanula host plants can be a first step to the beginning of a host expansion or change which, however, rarely occurs in oligolectic bees.
Introduction
Flowers are essential for bees, as almost all bee species gather their food exclusively from flowers. Bees are also the primary pollen vectors in most ecosystems (Michener, 2007). Nectar and pollen are the main food sources used for the bee’s own nourishment and are needed in large amounts to provision the brood (Westrich, 2018). The pollen of up to several hundred flowers is required to rear a single offspring (Müller et al., 2006). Because of the enormous amount of required pollen, flowering plants are thought to be the limiting factor for the abundance of bees in an area (Müller et al., 2006). This is especially the case for specialist (oligolectic) bees that collect pollen from only a few plants of a given genus or family (Müller and Kuhlmann, 2008) and the larvae normally fail to develop on pollen of non-host flowers (Praz et al., 2008b). The absence of specific host plants in a habitat leads to a lack of the corresponding oligolectic bee species, independently of other living conditions (Westrich, 2018). Generalist (polylectic) bees, in contrast, visit various plant taxa. They are able to switch to more abundant plant species if a preferred pollen host is not available. As floral resources are tending to decrease because of agricultural practices, bee specialists are particularly threatened at present (Müller et al., 2006).
Although strong selection is expected to act on oligolectic bees to reduce their heavy dependence upon a limited number of host plants, a broadening of their diet has occurred only occasionally in evolution (Sedivy et al., 2008). Bees seem to have evolved strong physiological adaptations to deal with only a few plant species that are similar in their pollen properties (Sedivy et al., 2008). For example, the larvae of the Campanula-specialist Chelostoma rapunculi fail to develop on the pollen of various non-host species which was also demonstrated for other oligoleges (Praz et al., 2008b). In contrast, individuals of the Asteraceae-specialist Heriades truncorum successfully developed on experimentally offered Echium or Campanula pollen (Praz et al., 2008b). Nevertheless, those individuals did not forage on the non-host plants (Praz et al., 2008a). Constraints in recognizing or handling the flowers might have prevented the bees from collecting pollen, but this suggestion awaits to be studied experimentally (Sedivy et al., 2008).
For host plant finding, oligolectic bees rely on visual and olfactory floral cues (Dötterl and Vereecken, 2010). Host-specific olfactory cues are normally used to recognize hosts and to differentiate them from non-hosts (Burger et al., 2010, 2012; Milet-Pinheiro et al., 2012). In these studies, the bees clearly preferred the scent of hosts when offered against non-host scent cues. Color cues are also involved in host finding but they are often not host-specific and do not function alone as a recognition cue (Burger et al., 2010). Foraging-naïve oligolectic C. rapunculi bees recognize their host plants, for example, by means of spiroacetals, i.e., the host-specific key components of the floral scent of different Campanula species (Milet-Pinheiro et al., 2013; Brandt et al., 2017). As soon as the newly emerged bees are foraging-experienced, they change their preference, visual cues and a bouquet of commonly occurring volatiles become reliable in the foraging behavior of experienced females (Milet-Pinheiro et al., 2012, 2013).
Chelostoma rapunculi bees are known to restrict their pollen collection to plants of the genus Campanula (Westrich, 2018). However, females have recently been monitored to occasionally visit Malva and Geranium species, among them M. moschata and G. sanguineum, in addition to their well-known Campanula hosts (observations from 1996 onward, Southern Germany; Datenbank Wildbienen-Kataster, Entomologischer Verein Stuttgart, and personal communication Hans Schwenninger). Occasional visits do not necessarily lead to a host change but could be a first step toward the incorporation of a new host. Since floral scent has been shown to play the most important role in the location of suitable flowers by C. rapunculi bees (Milet-Pinheiro et al., 2012), olfactory cues of Malva and Geranium flowers might also function as attractants to these non-hosts. Additionally, lilac and blueish colors in the range of different Campanula species are also attractants for C. rapunculi bees (Milet-Pinheiro et al., 2015).
In this study, we have investigated the attractiveness of olfactory and visual floral cues of G. sanguineum and M. moschata for foraging-naïve and experienced C. rapunculi bees and tested whether the bees show a preference when floral cues of the non-hosts are offered against those of the established host C. trachelium. Further, we have compared the floral scent bouquets between C. trachelium, and two non-hosts, M. moschata and G. sanguineum using electrophysiological and chemical analyses. As C. rapunculi bees are highly tuned to Campanula-specific volatiles (Milet-Pinheiro et al., 2013), we expected that M. moschata and G. sanguineum show similarities in their scent bouquets with C. trachelium if the olfactory cues of the non-hosts are indeed attractive to the bees. Additionally, we have modeled the floral color spectra of these plants, as visual cues are also involved in host plant finding of oligolectic bees.
Materials and Methods
Study Organisms
Chelostoma rapunculi (Lepeletier 1841) (Megachilidae) (Figure 1) is an oligolectic bee species with a distribution in many parts of Europe including Germany (Westrich, 2018). The bees nest in existing cavities, favoring deadwood and the boreholes of insects, but also accept trap nests such as the tubes of reed plants. The bees are highly specialized on Campanula spp. C. rapunculi is a univoltine bee species with its highest activity from early June until August.
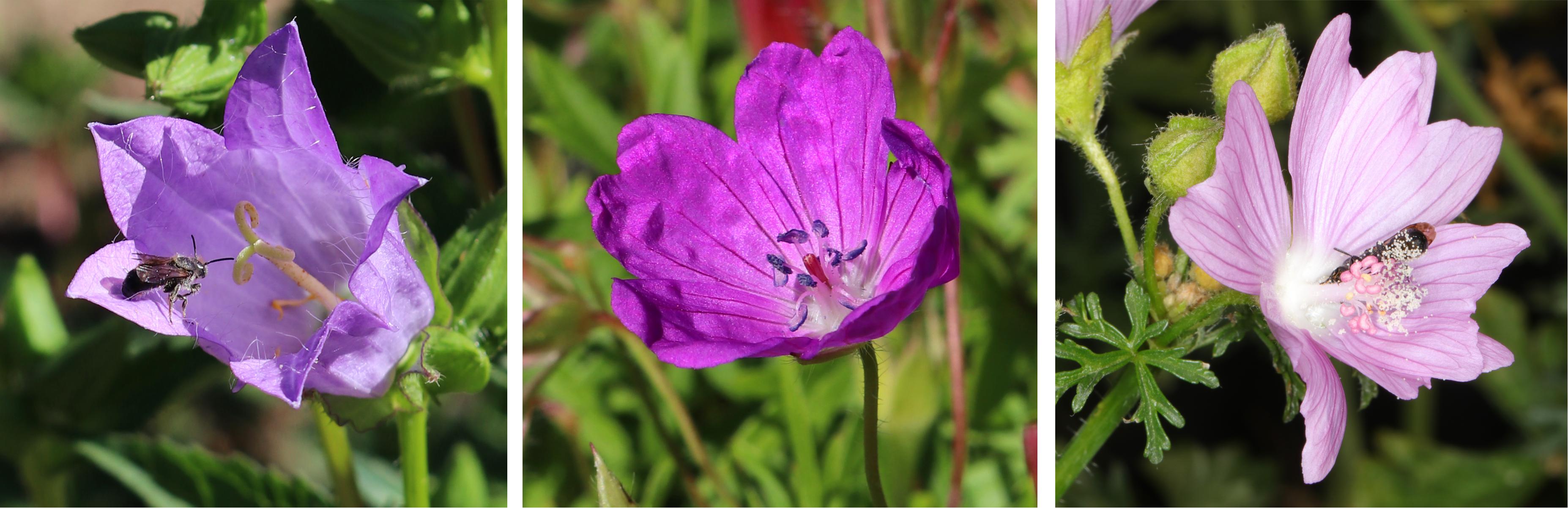
Figure 1. Flowers of the studied plant species Campanula trachelium, Geranium sanguineum, and Malva moschata and flower-visiting Chelostoma rapunculi females (left and right photograph).
The flowering times of the study plants overlap with the periods of activity of the bees. C. trachelium L. (Campanulaceae) is a European perennial herb that blooms from June to September. It is one of the main hosts of C. rapunculi and was already used as study organism in previous investigations on the flower recognition behavior of C. rapunculi bees (Milet-Pinheiro et al., 2012, 2013). G. sanguineum L. (Geraniaceae) is a persistent plant with a wide-spread distribution in Europe (Nebel et al., 1993). In Germany, the plant blooms from May to September and offer pollen and nectar (Westrich, 2018). M. moschata L. (Malvaceae) is an herbaceous perennial plant that is distributed throughout Europe (Nebel et al., 1993). The plants flowers from late June to October and provide nectar and a huge amount of pollen (Westrich, 2018). The taxa Geranium and Malva are both phylogenetically unrelated to Campanula (Asterids) but grouped together in the malvid clade of the Rosids (Angiosperm Phylogeny Website1).
Behavioral Experiments
Establishment of the Bee Population in an Experimental Flight Cage
For the behavioral studies, the bees were kept in a flight cage situated in the Botanical Garden of the University of Ulm. The flight cage consisted of a steel frame (7 m length × 3.5 m width × 2.2 m height) covered with a fine mesh (stitch density of 1 mm × 0.5 mm), the lower edges of which were buried in the soil to a depth of 0.5 m. A roof of UV permeable acrylic glass protected the cage. This setup allowed the simulation of abiotic conditions similar to those of the natural environment.
To obtain foraging-naïve bees, colonized trap nests were placed in a cage at the beginning of May, so that C. rapunculi bees emerged directly into the cage. The bees were fed with sugar water, prepared by Apiinvert (Südzucker AG, Ochsenfurt, Germany) and mixed with water to give a 40% solution. Sugar water was offered in saturated sponges placed in Petri dishes. The bees had no contact to the study plants C. trachelium, M. moschata, or G. sanguineum, which were used in the bioassays. Following the behavioral experiments with foraging-naïve bees, the same bees were allowed to forage and feed on their host plant C. trachelium to become foraging-experienced. To allow the bees to familiarize themselves with their host plant, bioassays were conducted at least 3 days after Campanula flowers were offered to the bees.
Set-Up of Behavioral Experiments
To test the attractiveness of decoupled floral cues of M. moschata, G. sanguineum, and C. trachelium for C. rapunculi bees, dual choice bioassays were conducted in the flight cage as previously described in Burger et al. (2010) and Milet-Pinheiro et al. (2012). Foraging-naïve and experienced bees were offered a choice between olfactory or visual cues of inflorescences of each species against an empty control. Furthermore, decoupled floral cues of M. moschata and G. sanguineum were tested against those of C. trachelium. The plant samples consisted of about 30 flowers each and were covered with cylinders to hide either visual or olfactory cues (Figure 2).
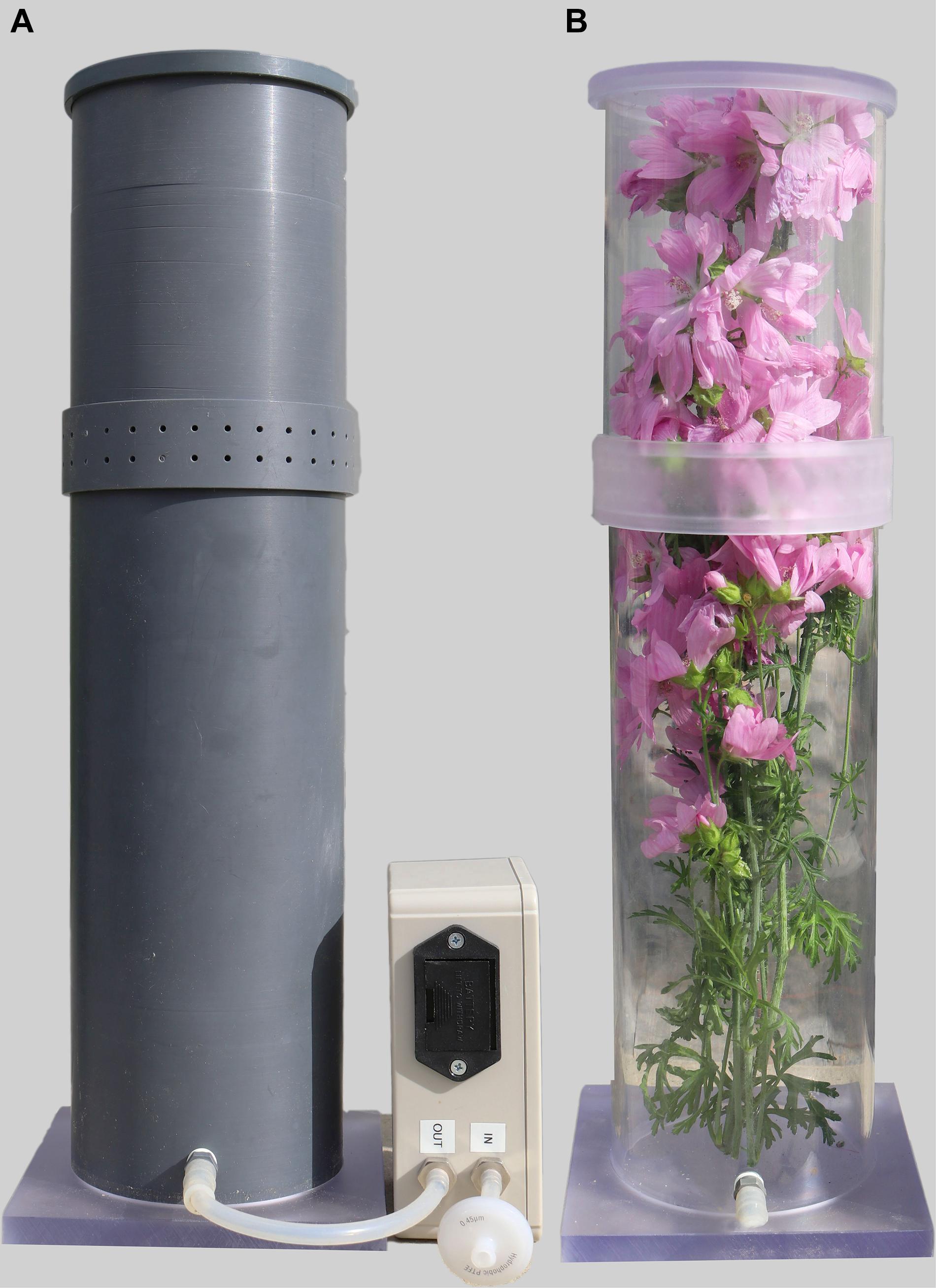
Figure 2. Cylinders to test behavioral responses to decoupled floral cues. The set-up for testing olfactory cues (A) consisted of a gray plastic cylinder that was connected to a pump and that had small holes allowing scent diffusion. For visual cues (B), transparent plexiglass cylinders were used but without holes.
The behavioral experiments were conducted on sunny days between 9:30 and 15:00 h, when the bees were most active. Two cylinders were offered at a distance of 1 m from each other in each choice experiment. Each test was conducted for a total of 30 min, during which the position of the two cylinders was exchanged after 15 min. As only limited numbers of bees were available for the experiments each season (approx. 50 or 20 individuals in the first or later seasons, respectively, at the beginning of the experimental series), all tests were repeated either 1 or 2 years later, and the responses were summed up within each experiment. Responses were pooled, as individuals of both years responded equally in all bioassays (Fisher’s Exact tests2: 0.16 < P < 1.00). The number of bees approaching the set-up at a maximum distance of 10 cm and landing on the cylinder was recorded as the behavioral response. To ensure that an individual bee was counted only once in a specific two-choice test, the approaching or landing bees were caught. An exact binomial test (see text footnote 2) was used to test for difference in total bee response in all dual-choice experiments.
Scent Analysis
Collection of Scent Samples
The volatiles of M. moschata, G. sanguineum, and C. trachelium inflorescences were collected using dynamic headspace methods (Dötterl and Jürgens, 2005). The samples were obtained to compare scent bouquets between species and to identify the components (samples for thermal desorption), as well as for electrophysiological investigations (solvent samples). Floral scents were collected in situ from potted plants or from cut inflorescences that were placed in water. Approximately 20 different plant individuals of each species were available for scent collection. For each sample, six inflorescences were enclosed in a polyester oven bag (20 × 30 cm; Toppits®).
To obtain samples for thermal desorption, the scent of enclosed flowers was enriched for half an hour. Volatiles were then trapped for 1 h in an adsorbent tube through which air was drawn at a rate of 150 ml/min by using a membrane pump (G12/01 EB, Rietschle Thomas, Puchheim, Germany). The adsorbent tube consisted of glass tubes (length 2 cm, inner diameter 2 mm) that were filled with a mixture of 1.5 mg Tenax-TA (mesh 60–80; Supelco, Bellefonte, PA, United States) and 1.5 mg Carbotrap B (mesh 20–40, Supelco, Bellefonte, PA, United States), which were fixed with glass wool (Dötterl and Jürgens, 2005). In total, 10 samples of each plant species were collected. As the analyses revealed that the scent concentration was relatively low in the samples, we collected three more samples of M. moschata and G. sanguineum, each sample containing 30 inflorescences. Additionally, scent from non-flowering plant parts (N = 3) and blank controls (N = 3) without plant material were collected in order to identify flower-specific compounds.
Additionally, solvent headspace samples for the electrophysiological analyses were collected for 6 h in a larger adsorbent tube (length 9 cm, inner diameter 2 mm) at a flow rate of 100 ml/min–1. The adsorbent tubes were filled with 10 mg of the absorbent Super Q (mesh 80/100, Alltech Associates Inc., United States). Volatiles were eluted with 100 μl dichloromethane (99.9%, Merck KGaA, Darmstadt, Germany). Three adsorbent tubes were pooled to obtain one sample, which was concentrated under a gentle stream of nitrogen and stored at −20°C. In total, six samples of each plant species were collected.
Electrophysiological Analysis
To determine the floral compounds that trigger antennal responses in C. rapunculi bees, gas chromatography coupled to electroantennographic detection (GC-EAD) experiments were performed for C. trachelium (N = 3), G. sanguineum (N = 10), and M. moschata (N = 7). The GC-EAD system consisted of an HP 6890 Hewlett-Packard gas chromatograph (Agilent Technologies, United States) equipped with a flame-ionization detector (FID) and an EAD set-up (Syntech, Hilversum, Netherlands). The separation of compounds was performed on a DB-5 column (30 cm long; 0.25 mm i.d.; 0.25 μm film thickness; J&W, United States). Hydrogen was used as the carrier gas at a constant flow of 2 ml/min. Two microliters of the solvent headspace samples were injected in splitless mode into the GC injector at an initial temperature of 40°C. After 1 min, the splitter was opened, and the oven temperature was increased at a rate of 10°C/min to a final temperature of 250°C, which was held for 3 min. In order to record the FID and EAD responses simultaneously, the GC effluent was split (split ratio FID:EAD = 1:1) under a make-up gas supply (nitrogen, 25 ml/min). The effluent was humidified with a filtered airflow of 100 ml/min and directed to the antennal preparation set-up via a glass tube of 95 mm length. Antennae of foraging-naïve C. rapunculi females were cut at the base and the tip and mounted between two glass capillaries that were connected to gold electrodes closing an electric circuit. The capillaries were filled with insect Ringer’s solution (5 g NaCl; 0.42 g KCl; 0.19 g CaCl2, in 1,000 ml demineralized water). New antennae were prepared for each run. A compound was considered to be EAD-active if a response was detected in at least three replicates. The EAD-active compounds were identified using gas chromatography coupled to mass spectrometry (GC-MS).
Chemical Analysis
To identify and compare the volatiles in the floral scent bouquet of the plants, the headspace samples were analyzed using gas chromatography (7890B GC system, Agilent Technologies, United States) coupled to mass spectrometry (Agilent 5977A mass selective detector). The GC was equipped with a thermal desorption unit (TDU, Gerstel, Mühlheim a. d. Ruhr, Germany) and a cold injection system (CIS 4C, Gerstel). A quartz micro-vial (length: 15 mm; inner diameter: 2 mm; Varian) containing 1 μl of a solvent sample or a thermal desorption sample was inserted into the injection unit by using an autosampler (Gerstel MAS Modular Analytical Systems Controller C506). The analytes were injected in splitless mode onto a non-polar column (DB-5ms, 30 m length, 250 μm inner diameter, 0.25 μm film thickness, J&W, United States). Analytes were thermally desorbed at 300°C for 8 min and refocused with liquid nitrogen. Helium was used as carrier gas at a constant flow of 1.5 ml per min. The oven program started at 40°C (held for 2 min) and was increased at a rate of 6°C per min to 200°C (held for 25 min; total run time 50 min). The MS interface and the ion source had temperatures of 250 and 230°C, respectively. Mass spectra were taken at 70 eV (in EI mode) from m/z 30 to 350.
Active compounds were assigned to GC-MS runs of the solvent scent samples that were used for the GC-EAD analyses by comparing the elution sequence and Kovats retention indices. An alkane series was run on all used systems. Compounds were identified based on their mass spectra by using multiple references from the NIST11 library and on published Kovats retention indices. Spiroacetals were identified based on the mass spectra and retention index described in Milet-Pinheiro et al. (2013). Additionally, the identification of individual components was confirmed by comparison of both mass spectrum and GC retention data on all used systems with those of authentic standards if available. The GC-MS runs were analyzed using Amdis 2.71 (Automated Mass Spectral Deconvolution and Identification System). Absolute amounts were calculated based on an alkane standard (dodecane, 0.1 μg) that was added to a clean thermal desorption tube (N = 6).
Comparison of Scent Samples
EAD-active floral scent compounds in the solvent headspace samples were considered for the analysis of the same compounds in thermal desorption samples. Volatiles were categorized as floral compounds when they were only found in inflorescence samples or when they occurred in smaller amounts in leaf samples. EAD-active compounds found in blank controls were excluded from further analyses. Inflorescence scent bouquets of the investigated species were compared using a semi-quantitative approach based on the Bray-Curtis similarity index. The relative ratios of the compounds were transformed to their square root. An analysis of similarity (ANOSIM, 9999 permutations) was performed using species as fixed factors. Non-metric multidimensional scaling, based on the similarity matrices generated, were used to display graphically the differences in scent-profiles among species. SIMPER analysis (similarities percentages routine) was used to reveal those components of the scent bouquet that contributed to the differences between or similarities within (with species as nested factor) the species. The software PRIMER 6.1.6 was used for the analyses (Clarke and Gorley, 2006).
Color Analyses
To measure and compare the spectral reflection of the petals of C. trachelium, G. sanguineum, and M. moschata, we used an Ocean Optics JAZ spectrometer (Ocean Optics Inc., Dunedin, FL, United States) equipped with a pulsed xenon light source (JAZ-PX) and attached to a fiber optic cable (UV/VIS 400 μm; World Precision Instruments Inc., Saracota, FL, United States). The optical fiber was fixed onto an attachment, so that the light touched the investigated object at an angle of 45°. A plate with barium sulfate (Merck KGaA, Darmstadt, Germany) and an open black film canister were used as a white and black standard, respectively. The spectral reflection was recorded from 300 to 700 nm, which corresponds to the color spectrum perceived by bees (Peitsch et al., 1992). For each species, three measurements were taken from petals from three freshly collected plant individuals. The R package pavo (Rx64 Version 3.3.1) was used to process the obtained raw data (Maia et al., 2013).
The mean reflections of the petals, based on the three measurements, were used to determine the loci of the measured colors within the hexagon color space according to Chittka (1992). Bee colors were modeled using the spectral sensitivity of the honeybee because bees have in general similar vision (Peitsch et al., 1992). Hexagon distances were calculated as Euclidean distances between the loci of the color stimuli and between the non-colored point, which was the background stimulus with constant reflection (Chittka, 1992). Bumblebees can effectively discriminate colors with distances of at least 0.1 hexagon units (Dyer and Chittka, 2004). The reflectance function of a typical green leaf was used as a background color (Chittka et al., 1994).
Results
Attractiveness of Floral Cues
In the behavioral experiments, the olfactory cues of all plant species were significantly more attractive than an empty control for naïve and experienced bees (for number of responding bees, see Figure 3A). Visual cues tested against a control were significantly more attractive for all plants in experienced bees, but for naïve ones, only for C. trachelium.
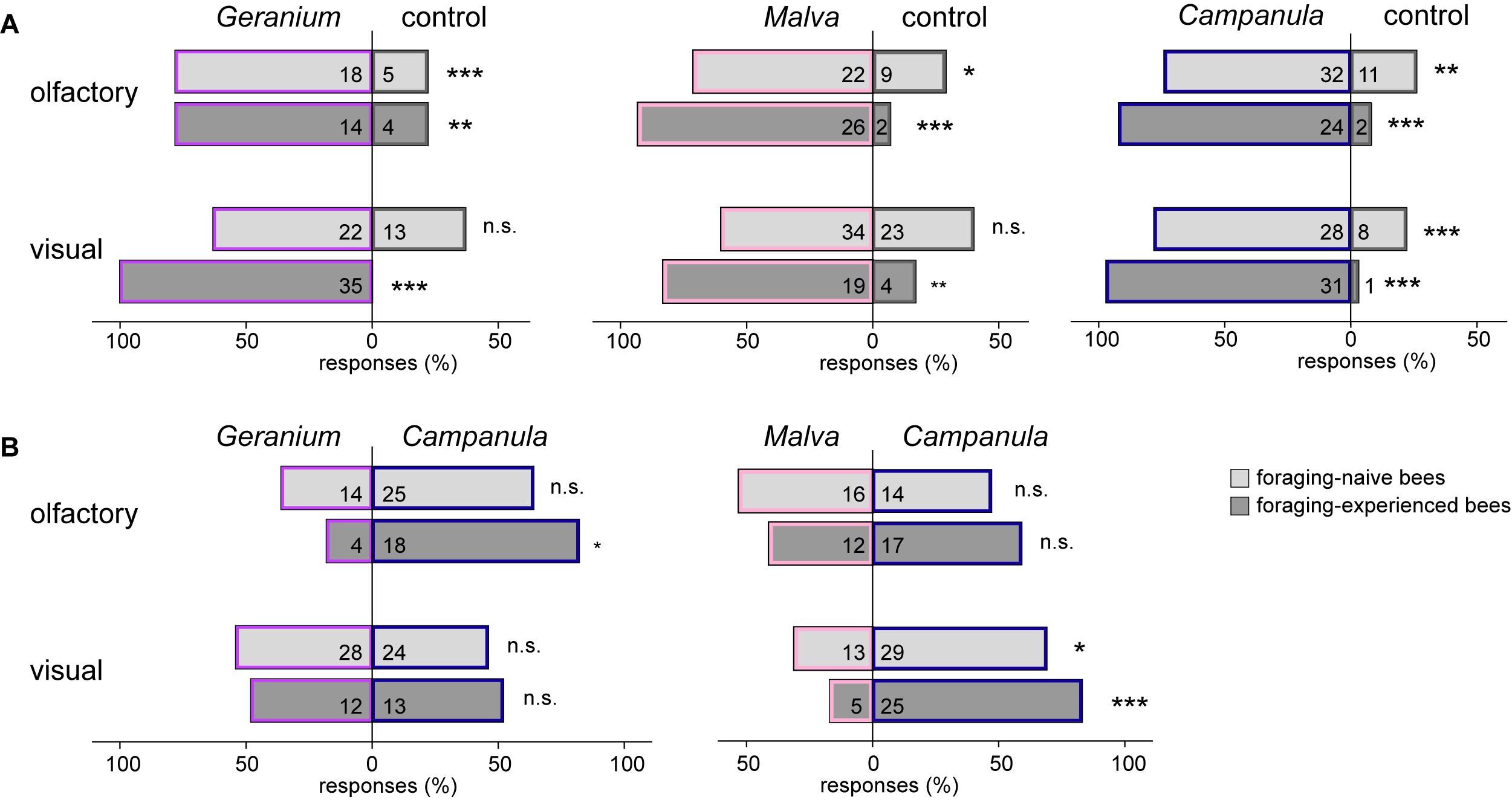
Figure 3. Behavioral responses (in percent) of foraging-naïve (light gray) and foraging-experienced (dark gray) males and females of C. rapunculi to olfactory and visual cues of (A) G. sanguineum, M. moschata, and C. trachelium inflorescences tested against an empty control and (B) G. sanguineum and M. moschata against C. trachelium (exact binomial test: n.s.: P > 0.05, *P < 0.05, **P < 0.01, ***P < 0.001).
Naïve bees showed no preference for one plant species when olfactory cues of either G. sanguineum or M. moschata were tested against those of C. trachelium (for number of responding bees, see Figure 3B). Experienced bees also showed no preference when M. moschata was tested against C. trachelium, but significantly more experienced bees preferred the olfactory cues of C. trachelium when tested against G. sanguineum. Regarding visual cues, both naïve and experienced bees did not prefer G. sanguineum over C. trachelium but significantly preferred C. trachelium over M. moschata.
Electrophysiologically Active Compounds and Comparison of Floral Scent Bouquets
In the GC-EAD analysis, 21 different antennal responses of C. rapunculi were registered in total (Figure 4). The responses were assigned to 32 compounds belonging to the following chemical classes: 2 aliphatic compounds, 18 terpenes, 2 spiroacetals, 3 benzenoids and phenylpropanoids, 1 nitrogen-containing compound, and 6 unknowns (Table 1). The two spiroacetals 1,6-dioxaspiro[4.5]decane and (Z)-7-methyl-1,6-dioxaspiro[4.5]decane (Z-conophthorin) were detected in quantifiable amounts in C. trachelium and G. sanguineum (Table 1). Overall, the scent bouquets significantly differed between the study species in the semi-quantitative comparison (ANOSIM R = 0.65, p < 0.001; Figure 5). (E)-β-ocimene was the main compound in all three plant species and contributed most to the similarity of samples within each plant species. This compound, followed by (Z)-β-ocimene and (E)-4,8-dimethyl-1,3,7-nonatriene, also contributed most to the similarity between the species. Dissimilarities between G. sanguineum and C. trachelium were mostly attributable to (E)-4,8-dimethyl-1,3,7-nonatriene and one unknown compound (m/z 81, 67, 55, RI 1023) between M. moschata and C. trachelium, followed by α-copaene and limonene in both groups.
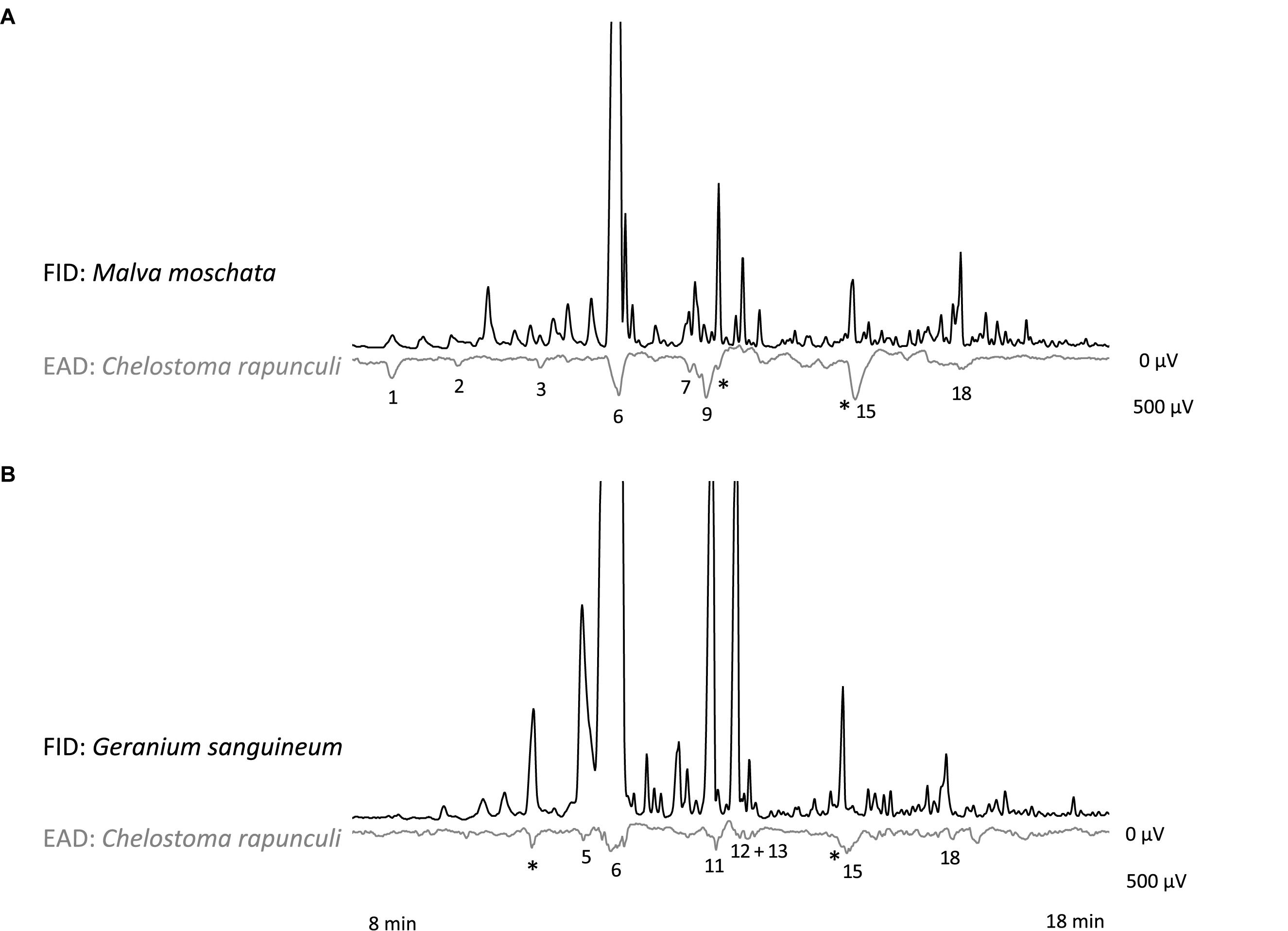
Figure 4. Simultaneous recordings of gas chromatographic (FID) and electroantennographic (EAD) signals for antennae of C. rapunculi females and a headspace sample of (A) M. moschata and (B) G. sanguineum inflorescences. Responses of the shown individuals are labeled with numbers that correspond to numbers given in Table 1. Responses to components of control samples are indicated by an asterisk.
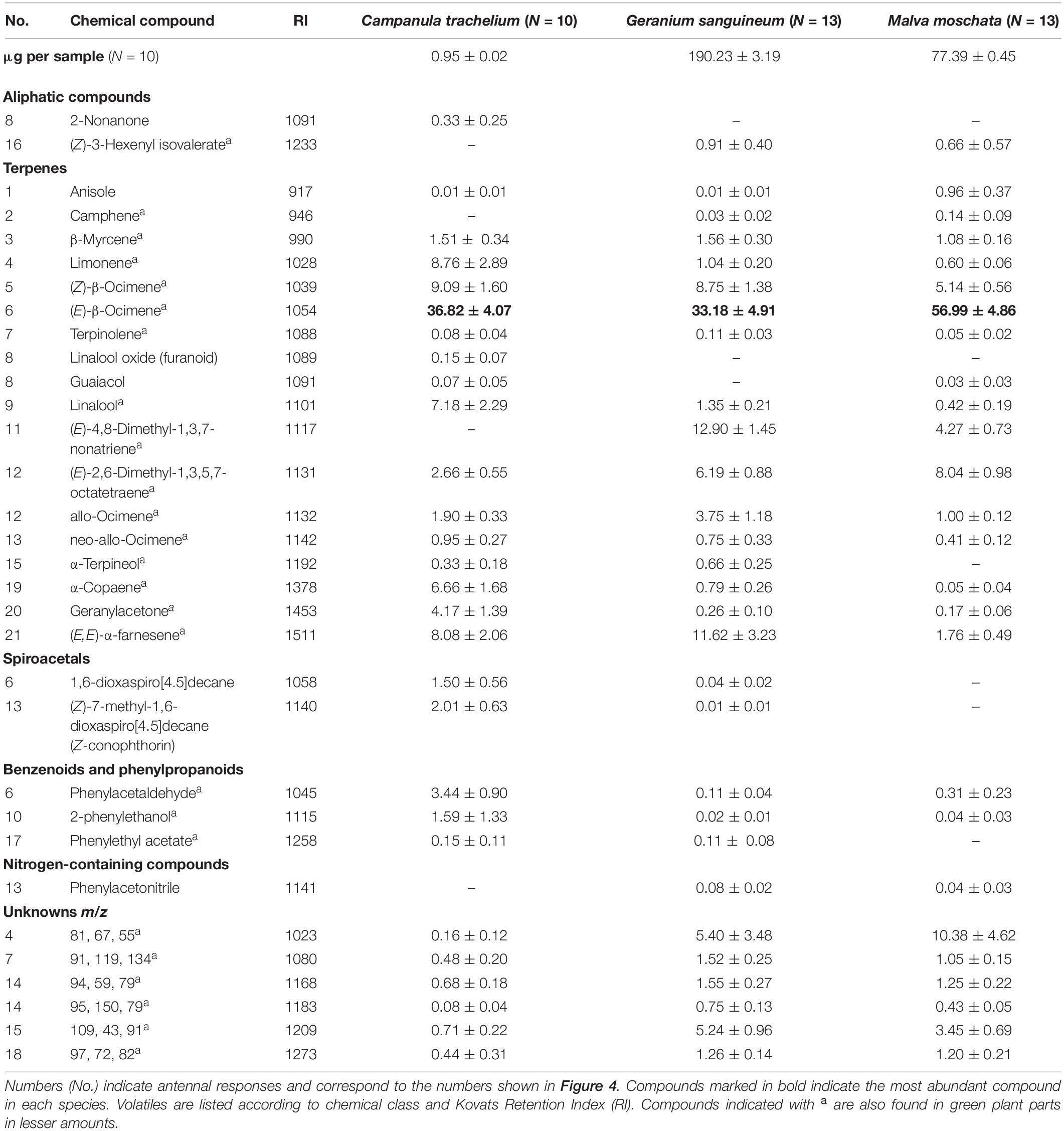
Table 1. Absolute (first line) and relative amounts (mean ± standard error) of GC-EAD active compounds in the headspace samples of inflorescences from C. trachelium, G. sanguineum, and M. moschata.
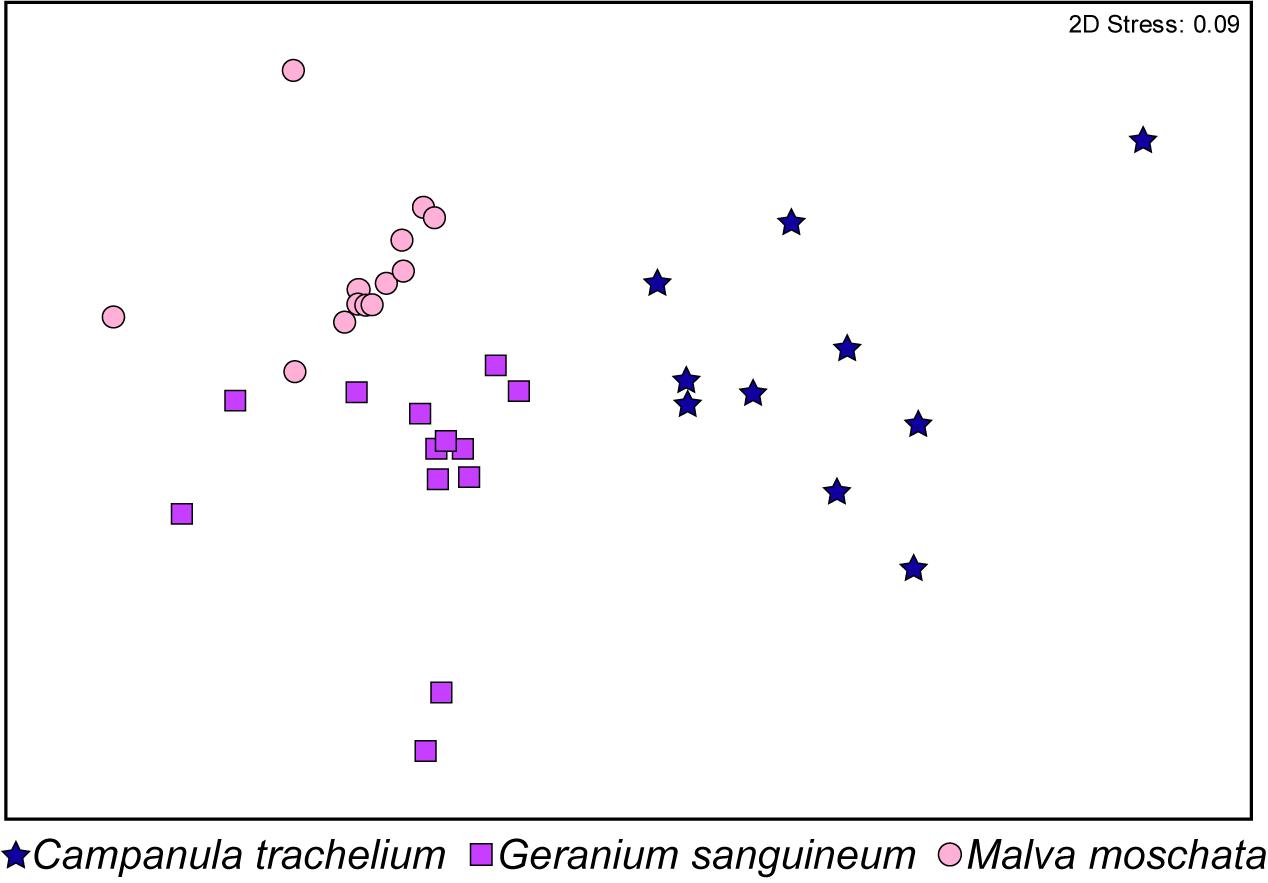
Figure 5. Non-metric multidimensional scaling based on the Bray-Curtis similarities of the semi-quantitative amounts of EAD-active compounds identified in headspace samples from C. trachelium (N = 10), G. sanguineum (N = 13), and M. moschata (N = 13) inflorescences (ANOSIM R = 0.65, P < 0.001).
Comparison of Floral Colors
The corollas of the investigated plant species were colored UV-blue or blue based on the categories of the bee color hexagon (Figure 6). The loci of C. trachelium was located at the intersection of both categories, whereas those of G. sanguineum and M. moschata lay in the UV-blue section and in the blue section, respectively. The smallest distance between species was between C. trachelium and M. moschata (0.08 units) and largest between G. sanguineum and M. moschata (0.32 units). The distance to the center of the hexagon (background colors) was smallest for M. moschata (0.16 units), intermediate for C. trachelium (0.21 units), and largest for G. sanguineum (0.38 units).
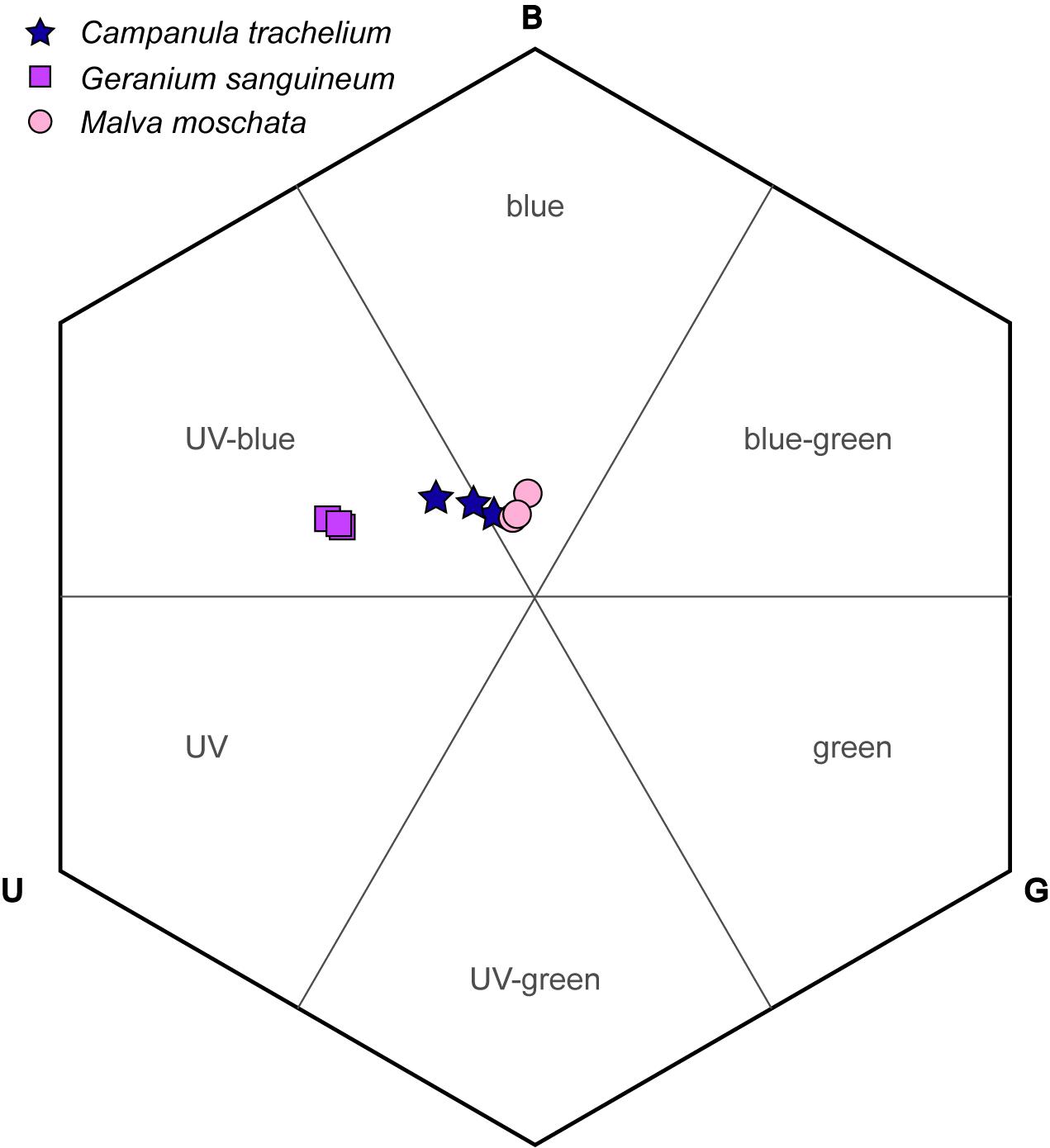
Figure 6. Corolla color loci of C. trachelium, G. sanguineum, and M. moschata in the bee color hexagon based on the excitations of the UV, blue, and green receptors (U, B, G).
Discussion
Our experiments show that oligolectic C. rapunculi bees are not only attracted by floral cues of the established host plant C. trachelium, but also by those of G. sanguineum and M. moschata. The floral scent and color of the plant species were species-specific but had some of the traits in common.
Pollen specialist bees visit sometimes or even regularly further plant species, besides their pollen hosts to collect nectar, which can explain the attractiveness of non-host floral cues in the absence of host plants. When offered, however, against an attractive host, oligolectic bees clearly prefer the olfactory cues of their host as shown in previous studies (Burger et al., 2010; Milet-Pinheiro et al., 2012). Accordingly, the olfactory cues of C. trachelium were more attractive for C. rapunculi bees than the ones of Echium vulgare and Potentilla recta non-hosts (Milet-Pinheiro et al., 2012). The specialized bees seem to be highly tuned in their search image to detect host plants on which they depend for a successful reproduction. Interestingly, in our experiments naïve C. rapunculi bees showed no preference for scent cues of its established host C. trachelium when it was offered together with either G. sanguineum or M. moschata.
We hypothesized that the attractiveness to non-host floral cues is explained by similarities in floral traits with Campanula hosts. The scent profiles of C. trachelium, G. sanguineum, and M. moschata were species-specific but also showed similarities. Many of the electrophysiologically active compounds, for example (E)-β-ocimene, linalool, or 2-phenylethanol, were shared by the analyzed host and non-hosts. However, these compounds are widely distributed floral scent compounds (Knudsen et al., 2006) and probably do not explain why naïve C. rapunculi bees were highly attracted by olfactory cues of G. sanguineum and M. moschata but not by synthetic mixtures or floral scent of Echium sp., that contained or emitted these compounds (Milet-Pinheiro et al., 2012, 2013). Instead, naïve bees responded only to samples that contained spiroacetals, which are characteristic scent compounds for Campanula (Milet-Pinheiro et al., 2013). Spiroacetals are rarely produced by flowers of other plant species than Campanula (Knudsen et al., 2006) and are the key components enabling C. rapunculi to find and recognize their hosts (Milet-Pinheiro et al., 2013). In addition, the antennae of C. rapunculi are highly sensitive to these compounds, and the bees are capable of detecting tiny amounts (Brandt et al., 2017). Interestingly, we also found small amounts of spiroacetals in the headspace samples of G. sanguineum, which might explain the attractiveness of this non-host species to C. rapunculi females. In floral scent samples of M. moschata, we detected only parts of the characteristic masses for spiroacetals in the chemical analyses, in amounts that were not quantifiable. Therefore, uncertainty remains as to whether this species emits spiroacetals, too. As we did not identify floral scent attractants that are shared by the studied species, behavioral experiments with synthetic compounds are needed to identify the behaviorally attractive compounds in M. moschata and to test whether spiroacetals are indeed involved in the attractiveness of G. sanguineum scent cues.
After the C. rapunculi bees gained experience in foraging on Campanula in the flight cage, they were still attracted by the olfactory cues of G. sanguineum and M. moschata when presented against an empty control although G. sanguineum wasn’t that attractive any more than their Campanula host. Once the bees have found a reliable pollen source, they are probably not motivated to seek further plant species and prefer the olfactory cues of hosts over non-hosts as observed for G. sanguineum when presented against C. trachelium. In contrast, the scent of M. moschata still had the same attractiveness as that of C. trachelium. We cannot explain this behavior based on our results but the bees might have been seeking nectar because we had removed all food sources during the performance of the bioassays.
Behavioral changes with foraging experience were also observed for visual floral traits. As long as the bees were foraging-naïve, visual cues of Malva and Geranium were not attractive for C. rapunculi, but they were later on for foraging-experienced bees. Oligolectic bees are attracted by a range of different wavelengths within a color category, for example blue and UV-blue stimuli attract C. rapunculi bees (Milet-Pinheiro et al., 2015), but these colors are not host-specific and are therefore not used to discriminate hosts and non-hosts (Burger et al., 2010). This might explain why the bees showed varying attractiveness toward the tested visual cues depending on the foraging state (naïve vs. experienced) and tested plant species, although all measured color loci were in the range of different Campanula species (Milet-Pinheiro et al., 2015). G. sanguineum colors could be clearly discriminated from C. trachelium by the bees based on the color modeling, but had the same attractiveness as C. trachelium when the visual cues were tested against each other. M. moschata flowers were similarly colored but the visual cues of M. moschata inflorescences were less attractive than C. trachelium. It seems that not only the specific color (dominant wavelength) of the petals explains the attractiveness of visual cues but further visual traits such as shape and size. As the strength of the contrast that a floral color makes to its background is correlated with attractiveness in other bees (Lunau et al., 1996), this visual trait might also influence the choice behavior of C. rapunculi. The color modeling showed that the spectrum of M. moschata is less detectable against background colors compared with that of the other studied plant species, and, accordingly, in the bioassays, M. moschata were less attractive when offered in a choice with C. trachelium.
Our own observations in the flight cage showed that C. rapunculi bees visited M. moschata flowers frequently in the absence of Campanula although the bees removed the pollen carefully before they returned to their nest. Interestingly, we also observed increasing numbers of individuals continuously visiting M. moschata flowers in the years after our experiments have been performed when we established a bee population outside the flight cage, and M. moschata plants were still present at a high density in the close surroundings. The bees had pollen attached to their scopa, but if the bees indeed actively collected pollen or were only contaminated while consuming nectar was not examined. Malvaceae pollen is mechanically protected by spines against collection by at least corbiculate bees (Lunau et al., 2015), but some Malvaceae oligoleges are able to transport their pollen (Schlindwein et al., 2009; Gaglianone, 2000). The observed visits do not mean that the bees are incorporating Malva and Geranium spp. as new hosts, but it can be a first step. As the host choice behavior in Chelostoma bees is thought to be restricted by physiological limits to digest different pollen diets (Praz et al., 2008b; Sedivy et al., 2008), an important experiment would be to study the development of C. rapunculi larvae that are fed with pure or different ratios of Malva and Geranium pollen in future studies. Beside pollen properties, other floral traits were also hypothesized to influence the floral preferences in this bee clade (Sedivy et al., 2008). When Chelostoma bees incorporated a new host that is phylogenetically unrelated to previous hosts, the new host had a striking high floral similarity to the previous one (Sedivy et al., 2008). Our study also revealed similarities in floral traits between host and each of the non-hosts: The floral colors of G. sanguineum and M. moschata were within the range of Campanula colors and we found an overlap of floral scent components of that, particularly, spiroacetals in G. sanguineum might be an important floral signal for C. rapunculi. Other floral cues were in contrast taxon-specific. So, it remains unknown whether the identified floral cues explain the attractiveness of the non-host plants. Follow-up studies and monitoring events are needed to fully understand the interaction between C. rapunculi bees and Malva and Geranium flowers. As more than 70% of plant species, including several of C. rapunculi’s host species, have declined in Germany during the last decades (Eichenberg et al., 2020), a broadening of the host range might reduce the high dependence of C. rapunculi on Campanula plants.
Data Availability Statement
The raw data supporting the conclusions of this article will be made available by the authors, without undue reservation.
Author Contributions
MA and HB designed the study. NJ and HB performed the experiments and analyzed the data. HB wrote the manuscript with contributions from NJ and MA. All authors contributed to the article and approved the submitted version.
Conflict of Interest
The authors declare that the research was conducted in the absence of any commercial or financial relationships that could be construed as a potential conflict of interest.
Publisher’s Note
All claims expressed in this article are solely those of the authors and do not necessarily represent those of their affiliated organizations, or those of the publisher, the editors and the reviewers. Any product that may be evaluated in this article, or claim that may be made by its manufacturer, is not guaranteed or endorsed by the publisher.
Acknowledgments
We gratefully acknowledge K. Brandt, K. C. Heuel, and K. Babucke for their help to perform behavioral experiments, P. Milet-Pinheiro and S. Dötterl for advice in chemical analyses, and the people of the Botanical Garden of Ulm to grow plants for the experiments. We also thank Theresa Jones for linguistic advice and K. Brandt and two reviewers for valuable comments. All experiments comply with the current laws of the country in which they were performed: in this case, Germany.
Footnotes
References
Brandt, K., Dötterl, S., Francke, W., Ayasse, M., and Milet-Pinheiro, P. (2017). Flower visitors of campanula: are oligoleges more sensitive to host-specific floral scents than polyleges? J. Chem. Ecol. 43, 4–12. doi: 10.1007/s10886-016-0802-z
Burger, H., Dötterl, S., and Ayasse, M. (2010). Host-plant finding and recognition by visual and olfactory floral cues in an oligolectic bee. Funct. Ecol. 24, 1234–1240. doi: 10.1111/j.1365-2435.2010.01744.x
Burger, H., Dötterl, S., Häberlein, C. M., Schulz, S., and Ayasse, M. (2012). An arthropod deterrent attracts specialised bees to their host plants. Oecologia 168, 727–736. doi: 10.1007/s00442-011-2136-4
Chittka, L. (1992). The colour hexagon: a chromaticity diagram based on photoreceptor excitations as a generalized representation of colour opponency. J. Compar. Physiol. A Sens. Neural Behav. Physiol. 170, 533–543.
Chittka, L., Shmida, A., Troje, N., and Menzel, R. (1994). Ultraviolet as a component of flower reflections, and the colour perception of Hymenoptera. Vis. Res. 34, 1489–1508. doi: 10.1016/0042-6989(94)90151-1
Dötterl, S., and Jürgens, A. (2005). Spatial fragrance patterns in flowers of Silene latifolia: lilac compounds as olfactory nectar guides? Plant Syst. Evol. 255, 99–109. doi: 10.1007/s00606-005-0344-2
Dötterl, S., and Vereecken, N. J. (2010). The chemical ecology and evolution of bee-flower interactions: a review and perspectives. Can. J. Zool. 88, 668–697. doi: 10.1139/Z10-031
Dyer, A. G., and Chittka, L. (2004). Biological significance of distinguishing between similar colours in spectrally variable illumination: bumblebees (Bombus terrestris) as a case study. J. Compar. Physiol. A Neuroethol. Sens. Neural Behav. Physiol. 190, 105–114. doi: 10.1007/s00359-003-0475-2
Eichenberg, D., Bowler, D. E., Bonn, A., Bruelheide, H., Grescho, V., Harter, D., et al. (2020). Widespread decline in Central European plant diversity across six decades. Glob. Chang. Biol. 27, 1097–1110. doi: 10.1111/gcb.15447
Gaglianone, M. C. (2000). Behavior on flowers, structures associated to pollen transport and nesting biology of Perditomorpha brunerii and Cephalurgus anomalus (Hymenoptera: Colletidae, Andrenidae). Rev. Biol.Trop. 48, 89–99.
Knudsen, J. T., Eriksson, R., Gershenzon, J., and Stahl, B. (2006). Diversity and distribution of floral scent. Bot. Rev. 72:1. doi: 10.1663/0006-8101(2006)72[1:dadofs]2.0.co;2
Lunau, K., Wacht, S., and Chittka, L. (1996). Colour choices of naive bumble bees and their implications for colour perception. J. Compar. Physiol. A Sens. Neural Behav. Physiol. 178, 477–489.
Lunau, K., Piorek, V., Krohn, O., and Pacini, E. (2015). Just spines—mechanical defense of malvaceous pollen against collection by corbiculate bees. Apidologie 46, 144–149.
Maia, R., Eliason, C. M., Bitton, P. P., Doucet, S. M., and Shawkey, M. D. (2013). pavo: an R package for the analysis, visualization and organization of spectral data. Methods Ecol. Evol. 4, 906–913.
Milet-Pinheiro, P., Ayasse, M., Dobson, H., Schlindwein, C., Francke, W., and Dötterl, S. (2013). The chemical basis of host-plant recognition in a specialized bee pollinator. J. Chem. Ecol. 39, 1347–1360. doi: 10.1007/s10886-013-0363-3
Milet-Pinheiro, P., Ayasse, M., Schlindwein, C., Dobson, H., and Dötterl, S. (2012). Host location by visual and olfactory floral cues in an oligolectic bee: innate and learned behavior. Behav. Ecol. 23, 531–538. doi: 10.1093/beheco/arr219
Milet-Pinheiro, P., Ayasse, M., and Dötterl, S. (2015). Visual and olfactory floral cues of Campanula (Campanulaceae) and their significance for host recognition by an oligolectic bee pollinator. PLoS One 10:e0128577. doi: 10.1371/journal.pone.0128577
Müller, A., Diener, S., Schnyder, S., Stutz, K., Sedivy, C., and Dorn, S. (2006). Quantitative pollen requirements of solitary bees: implications for bee conservation and the evolution of bee-flower relationships. Biol. Conserv. 130, 604–615. doi: 10.1016/j.biocon.2006.01.023
Müller, A., and Kuhlmann, M. (2008). Pollen hosts of western palaearctic bees of the genus Colletes (Hymenoptera: Colletidae): the Asteraceae paradox. Biol. J. Linnean Soc. 95, 719–733. doi: 10.1111/j.1095-8312.2008.01113.x
Nebel, M., Philippi, G., Quinger, B., Rösch, M., Schiefer, J., Sebald, O., et al. (1993). Die Farn-und Blütenpflanzen Baden-Württembergs. Stuttgart: E. Ulmer.
Peitsch, D., Fietz, A., Hertel, H., de Souza, J., Ventura, D. F., and Menzel, R. (1992). The spectral input systems of Hymenopteran insects and their receptor-based colour vision. J. Compar. Physiol. A Sens. Neural Behav. Physiol. 170, 23–40. doi: 10.1007/BF00190398
Praz, C. J., Müller, A., and Dorn, S. (2008a). Host recognition in a pollen-specialist bee: evidence for a genetic basis. Apidologie 39, 547–557. doi: 10.1051/apido:2008034
Praz, C. J., Müller, A., and Dorn, S. (2008b). Specialized bees fail to develop on non-host pollen: do plants chemically protect their pollen? Ecology 89, 795–804. doi: 10.1890/07-0751.1
Sedivy, C., Praz, C. J., Müller, A., Widmer, A., and Dorn, S. (2008). Patterns of host-plant choice in bees of the genus Chelostoma: the constraint hypothesis of host-range evolution in bees. Evolution 62, 2487–2507. doi: 10.1111/j.1558-5646.2008.00465.x
Schlindwein, C., Pick, R. A., and Martins, C. F. (2009). Evaluation of oligolecty in the Brazilian bee Ptilothrix plumata (Hymenoptera, Apidae, Emphorini). Apidologie 40, 106–116.
Keywords: floral scent, color, spiroacetals, Campanula, pollen-specialist bee, Malva, Geranium
Citation: Burger H, Joos N and Ayasse M (2021) Floral Cues of Non-host Plants Attract Oligolectic Chelostoma rapunculi Bees. Front. Ecol. Evol. 9:682960. doi: 10.3389/fevo.2021.682960
Received: 19 March 2021; Accepted: 06 July 2021;
Published: 30 July 2021.
Edited by:
Sara Diana Leonhardt, Technical University of Munich, GermanyReviewed by:
Tamar Keasar, University of Haifa - Oranim, IsraelKelsey Jaye Ruth Paularena Byers, John Innes Centre, United Kingdom
Copyright © 2021 Burger, Joos and Ayasse. This is an open-access article distributed under the terms of the Creative Commons Attribution License (CC BY). The use, distribution or reproduction in other forums is permitted, provided the original author(s) and the copyright owner(s) are credited and that the original publication in this journal is cited, in accordance with accepted academic practice. No use, distribution or reproduction is permitted which does not comply with these terms.
*Correspondence: Hannah Burger, info@hannah-burger.de