- 1Ministry of Education Key Laboratory of Ecology and Resource Use of the Mongolian Plateau and Inner Mongolia Key Laboratory of Grassland Ecology, School of Ecology and Environment, Inner Mongolia University, Hohhot, China
- 2Vegetation Ecology Group, Institute of Natural Resource Sciences, Zurich University of Applied Sciences, Wädenswil, Switzerland
- 3Ministry of Education of China and Inner Mongolia Autonomous Region, Collaborative Innovation Centre for Grassland Ecological Security, Hohhot, China
- 4College of Life Science and Technology, Inner Mongolia Normal University, Hohhot, China
Plant functional traits reflect species ecological strategies and determine how plants respond to environmental changes, however, how dominant species in the Inner Mongolia grassland adapt to different grazing systems by changing their functional traits has not been systematically investigated. In this study, we established four 7-year grazing treatments in the Inner Mongolia grassland: cattle grazing, sheep grazing, mixed sheep and cattle grazing, and no grazing. Fourteen functional traits of three dominant species (Stipa grandis, Leymus chinensis, and Cleistogenes squarrosa) were measured under the different grazing treatments. We found convergences of plant functional traits that indicate herbivory avoidance or tolerance. Plants reduced their vegetative height (VH) and stem: leaf ratio (SLR) to avoid grazing; increased their ability to acquire resources by increasing their specific leaf area (SLA), leaf nitrogen concentration (LNC), and leaf phosphorus concentration (LPC); and reduced their leaf dry matter content (LDMC) to tolerate grazing. Moreover, plants may adapt to grazing by increasing the intraspecific variability of their functional traits. Sheep-only grazing adversely affected L. chinensis growth and survival, while cattle-only grazing hindered S. grandis growth and increased the intraspecific variability of its plant functional traits. Our study emphasizes that intraspecific variability is an important indicator of the responses of plant functional traits to grazing. Since single grazing is more detrimental to the functional traits of dominant plants, we suggest that mixed cattle and sheep grazing may be a more environmentally friendly and sustainable practice for the Inner Mongolia grassland than single grazing.
Introduction
Many ecological problems can be addressed from the perspective of plant functional traits (Diaz et al., 2007b; Lavorel et al., 2011; Reichstein et al., 2014) because plants can quickly change their morphology and physiological characteristics in response to environmental changes (Wright et al., 2004). With the standardization of measurement methods and the establishment of global plant functional trait databases, plant functional traits are increasingly being applied to quantitatively analyze and simulate the effects of human activities on ecosystem structure and functions (Kattge et al., 2011; Perez-Harguindeguy et al., 2013; Diaz et al., 2016).
Managed grazing is the most widespread land use on earth and covers more than a quarter of the global land surface (Asner et al., 2004). Managed grazing is also an important driver of ecosystem succession processes and has an important “sieve effect” on the distribution and development of plant traits (von Wehrden et al., 2012). Plant traits can be used as effective indicators for studying the adaptability of species under grazing disturbances and predicting the response of communities and ecosystem functions to grazing disturbances (Diaz et al., 2007b; Zheng et al., 2010). For example, plant resistance to grazing is related to both avoidance traits (such as low plant height and leaf area) and tolerance traits [such as high specific leaf area (SLA) and leaf nitrogen concentration (LNC) (Zheng et al., 2011)]. These traits can reflect the survival strategies of plants and their ability to obtain resources and compete for nutrients under different grazing intensities (Diaz et al., 2007a). Moreover, plants with certain functional traits experience stronger herbivore selection preference, causing a considerable reduction in the populations of these plants under high-intensity grazing (Wang et al., 2011). For example, theoretical predictions and previous studies have found that the abundance of tall species tends to decrease after grazing (Westoby et al., 1999; Diaz et al., 2007a; Niu et al., 2010).
Previous studies have focused on how plants adjust their morphological structure and physiological characteristics in response to grazing disturbances (Diaz et al., 2007b; Bai et al., 2012) and avoid or tolerate livestock feeding by changing their traits (Diaz et al., 2001; Cingolani et al., 2005). Recent studies in various systems have highlighted that intraspecific trait variability (ITV) is a key factor that plays an important ecological role (Albert et al., 2011). ITV can provide meaningful information about plant functional trait responses to changes in environmental gradients (Nicotra et al., 2010; Laforest-Lapointe et al., 2014) and can increase the ability of species to establish and spread in new environments (Davidson et al., 2011). ITV also helps to explain how certain species pass through biological and abiotic filters and consequently promote species coexistence (Jung et al., 2010; de la Riva et al., 2016). Lang et al. (2020) found that the “ITV response to grazing” increases with an increase in precipitation in the Mongolian rangelands. High ITV at the population level may arise from differences in ecological factors, such as soil texture and successional stage (Albert et al., 2011; Salazar et al., 2018). Disturbance regimes (e.g., grazing) are also important to population ITV, and the greater the intensity of a disturbance is, the greater the ITV is. For example, Jung et al. (2010) found that the greater the flood pressure was, the greater the ITV was in terms of height and SLA. Disturbances can cause major shifts in understorey environmental conditions such as light availability, temperature and soil moisture status (Venier et al., 2014), which will increase the heterogeneity of the environment and increase the magnitude of ITV (Schulte and Mladenoff, 2005; Kumordzi et al., 2019).
Grasslands occupy 37% of the earth’s terrestrial area, and increasing grazing pressure has caused many of them to suffer some level of degradation (O’Mara, 2012); grassland degradation causes a series of problems, including decreased ground cover, increased populations of less-palatable species and other changes in grassland communities (Kemp et al., 2013). Plants can compensate for herbivore damage by increasing their growth rates (Knapp et al., 2012; Muthoni et al., 2014). Herbivore selectivity alters the species composition and community structure of grasslands (Bai et al., 2012; Guo et al., 2016). For example, in the Inner Mongolia grassland, one of the largest remaining grasslands on Earth (i.e., Eurasian steppe), overgrazing has degraded the Stipa grandis P. Smirn. and Leymus chinensis (Trin.) Tzvel steppe such that they have become Cleistogenes squarrosa (Trin.) Keng steppe (Tong et al., 2004). Because both herbivore selectivity and plant growth rates are related to plant functional traits (Wright et al., 2004; Cingolani et al., 2005), it is feasible to use the response of plant functional traits to grazing to reveal the mechanisms underlying community structure changes in grassland ecosystems.
Grazing in grassland ecosystems reflects the interactions of soil, livestock, and grass. Large herbivores affect the grassland ecosystem by feeding, excreting feces, and trampling (Veen et al., 2014). Moreover, different combinations of herbivores have different levels of feed intake, which leads to differences in aboveground biomass, soil water content, species composition, nitrogen availability, net ecosystem CO2 exchange and total ecosystem productivity (Bakker et al., 2004; Hodel et al., 2014; Liu et al., 2015, 2016; Risch et al., 2015). Therefore, different grazing systems affect grasslands differently. There is some evidence that mixed grazing can increase livestock production and multifunctionality in managed grasslands (Thebault and Loreau, 2003; Wang et al., 2019). In the grassland ecosystem of Inner Mongolia, researchers have paid more attention to the effects of grazing vs. non-grazing and of grazing intensity on plant functional traits (Bai et al., 2012; Zhang et al., 2018). Few studies, however, have examined the effect of different grazing systems on plant functional traits in dominant species, and few studies have focused on ITV under different grazing systems (Lang et al., 2020). Here, we characterized the response of the dominant species in the Inner Mongolia grassland to different grazing systems and asked the following questions:
(1) Which grazing systems have more adverse effects on the functional traits of dominant species in the Inner Mongolia grassland?
(2) Do plants change their intraspecific functional trait variability to resist grazing disturbance?
Materials and Methods
Study Sites
This study was conducted at the field observation station of the National Climate Observatory in Xilinhot (NCOX, 44°07′∼09′N, 116°19′∼20′E), located in Xilinhot, Inner Mongolia, China, at an altitude of 1129 m (Fan et al., 2019). This area is characterized by a typical temperate semiarid climate with wet, mild summers and dry, cold winters. Based on meteorological data provided by the National Meteorological Administration of China, the study area has a mean annual precipitation of 297 mm (1953–2013), and 60–80% of precipitation falls during the growing season (May to September). The mean annual temperature is 2.4°C, with the peak temperatures occurring during the growing season. The soil type in this area is a chestnut soil, which is similar to a calcic Chernozem according to the IUSS Working Group WRB 2006 (Pan et al., 2016). The study area consists mainly of grasslands in which the dominant species are S. grandis, L. chinensis, and C. squarrosa (Trin.) Keng.
Experimental Design
In 2013, a long-term grazing experiment with twelve plots was established in NCOX in a completely randomized block design. Each plot was randomly assigned one of four grazing management practices: no grazing (NG), sheep grazing (SG), cattle grazing (CG), and mixed grazing with sheep and cattle (MG), therefore, each grazing system had three repetitions (Wang et al., 2018). To control the grazing intensity, the same feed intake per unit area was utilized in all grazing treatments. According to previous studies, the feed intake of cattle in the Inner Mongolia grassland is equivalent to the feed intake of 8.5 sheep (Lin et al., 2008). Therefore, we grazed 85 adult sheep in the SG plots, 10 adult cattle in the CG plots, and 85 adult sheep and 10 adult cattle in the MG plots. The stocking rate was designed to provide a moderate grazing intensity level, and livestock were removed from the plots when approximately 50% of the aboveground plant biomass had been consumed (Wang et al., 2018). The grazing trials started at the beginning of June and ended at the end of August, with grazing intervals of 1 month on average starting at the beginning of the experiment. The experiment ran for a total of 7 years.
Species Selection
We selected three plant species S. grandis, L. chinensis, and C. squarrosa for three main reasons (Table 1). First, the total biomass of the three plants accounted for more than 60% of the total biomass at each plot, the mean proportion of aboveground biomass contributed by the three species was shown in Table 1. Second, L. chinensis and S. grandis are the most important native species in the typical grasslands of Inner Mongolia (Wang et al., 2000). Last, previous studies regarding the consequences of species turnover in changing community structures have concluded that some S. grandis and L. chinensis grasslands will degenerate into C. squarrosa grasslands after livestock overgrazing (Wang et al., 1996). Therefore, analyzing the responses of the functional traits of these species to different grazing systems should aid our understanding of the fundamental ecological mechanisms underlying grassland degradation and succession.

Table 1. Mean proportion of aboveground biomass (%) contributed by species at twelve sites on typical steppe, Inner Mongolia, with different grazing systems.
Measurement of Plant Functional Traits
Data on plant functional traits were collected in August 2019. At all grazing plots, three grazing exclosures (1.5 m × 1.5 m) were established randomly prior to livestock grazing, which started in June. Within each plot, two well-grown (non-shaded and non-damaged), mature individuals of the selected species were randomly collected from the grazing exclosures (six duplicates for each species under each grazing treatment). Five morphological traits and nine chemical traits (Table 2) of each selected species were measured at each plot, following Perez-Harguindeguy et al. (2013). The vegetative height (VH) was measured in the field, and then each selected individual was excavated with its whole root system. After cleaning the roots, each individual plant was separated into its roots, leaves, and stems; oven-dried at 65°C for 48 h; and weighed. The root/shoot ratio (RSR) and stem: leaf ratio (SLR) were then calculated. The nitrogen and phosphorus concentrations of the roots, stems and leaves (dry mass) of each species [the root nitrogen concentration (RNC), root phosphorous concentration (RPC), stem nitrogen concentration (SNC), stem phosphorous (SPC), LNC, and leaf phosphorous concentration (LPC), respectively] per plot were analyzed with an elemental analyzer (CHN-600, LECO, St. Joseph, MI, United States). The nitrogen: phosphorus ratios (NPRs) of the roots, stems and leaves (RNPR, SNPR, and LNPR, respectively) were calculated. The SLA and leaf dry matter content (LDMC) were measured using standardized protocols (Perez-Harguindeguy et al., 2013). According to leaf size, 5–20 leaves were collected as a sample to measure SLA and LDMC (Zheng et al., 2011). These two traits were also measured on six replicate samples per species per grazing system. The aboveground parts of the plant were collected, quickly placed into a box filled with water and rehydrated for at least 6 h in the dark. After wiping the water off the surface of the blades, the leaf fresh mass was measured by weighing, and the leaf area was measured with a leaf area meter (Li-3000, LI-COR, Superior St., Lincoln, NE, United States). The leaves were then oven-dried at 60°C for 48 h and weighed. The LDMC was calculated as the leaf dry mass/leaf fresh mass, and the SLA was calculated as the leaf area/leaf dry mass.
For each species and trait, we calculated the ITV as the coefficient of variation (CV), which is computed as the standard deviation of each grazing system (six replicates) divided by the mean (Lang et al., 2020).
Statistical Analysis
All statistical analyses were performed with R x 64 3.6.1 (R Development Core Team). A one-way analysis of variance (ANOVA) was performed to detect whether there were significant differences in the functional traits of the species under the different grazing treatments. Principal component analysis (PCA) was conducted on the matrix of traits × plot combinations to analyze the differences in the plant functional traits within species among plotplots and to explore the correlations among plant functional traits. Three PCA plots were drawn, one for each species. We also added confidence intervals for the functional trait composition.
Results
Morphological Traits
The grazing treatment had a significant effect on the VH of the three plants (S. grandis, F3,20 = 13.734, P < 0.001; L. chinensis, F3,20 = 15.633, P < 0.001; C. squarrosa, F3,20 = 9.534, P < 0.001, Figure 1A). The VHs of the S. grandis, L. chinensis, and C. squarrosa were highest in the NG plots, with heights of 50.67 ± 3.88 cm, 40.58 ± 3.18 cm, and 20.83 ± 4.26 cm, respectively. Except for the VH of S. grandis, which was the lowest in the MG plots, there were no significant differences in the VHs of the three species among the plots. The response of the SLR of the three species to the different grazing treatments is shown in Figure 1B. L. chinensis had the highest SLR of all species in the MG plots, while S. grandis had the highest SLR in the CG plots; both L. chinensis and S. grandis had the lowest SLRs in the NG plots. The grazing treatments had no effect on the SLR of C. squarrosa (F3,20 = 1.405, P = 0.272). The LDMCs of all three species were the highest in the NG plots (Figure 1C). The RSR of L. chinensis was highest in the SG plots and lowest in the MG plots. There was no significant difference in the RSRs of the other species among plots (S. grandis, F3,20 = 0.623, P = 0.609; C. squarrosa, F3,20 = 0.164, P = 0.920; Figure 1D). The grazing treatments had a significant effect on the SLA of the three species. L. chinensis had the highest SLA in the CG plots and the lowest SLA at the NG plots. The SLAs of S. grandis and C. squarrosa were highest in the SG plots (Figure 1E).
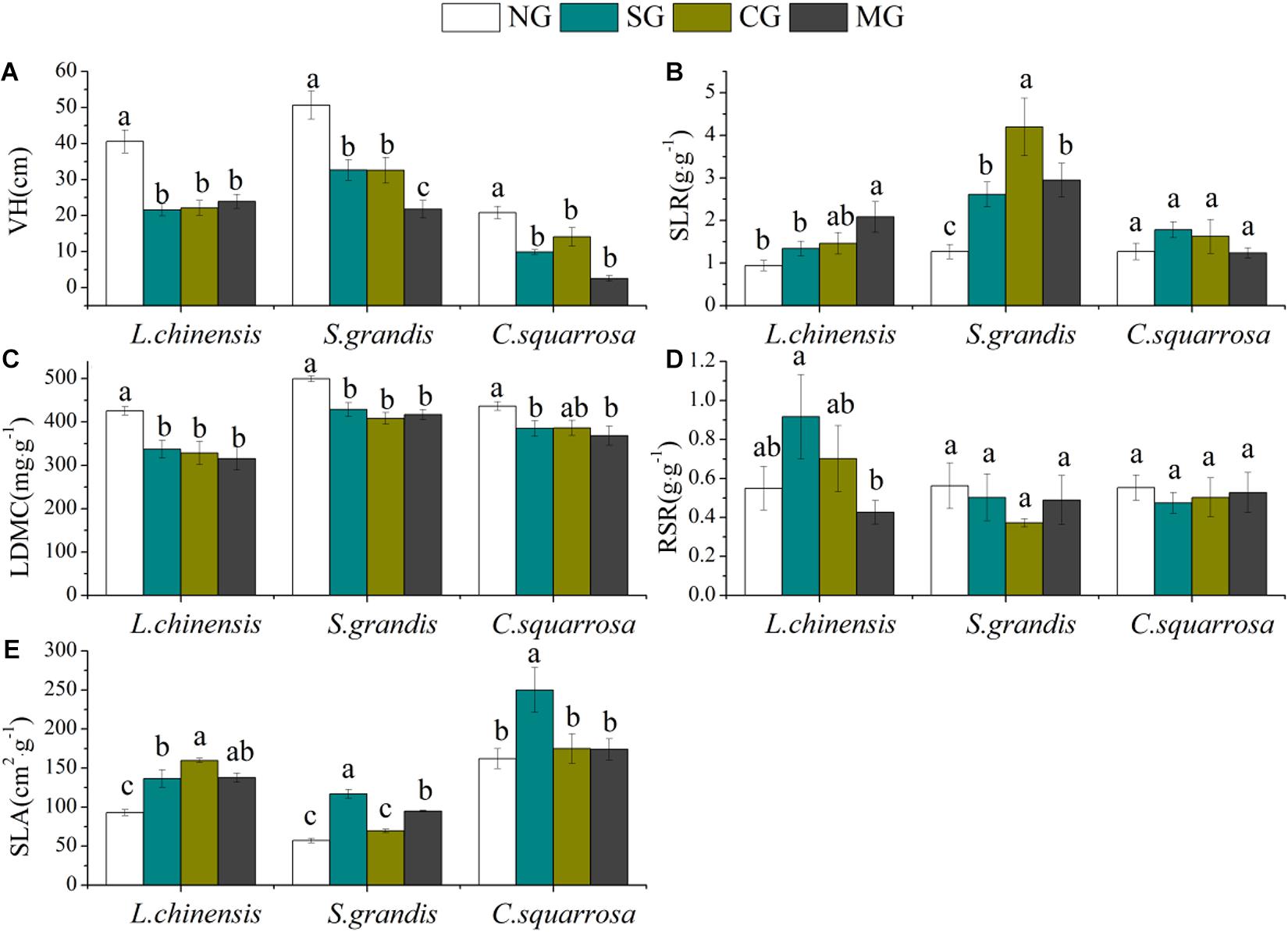
Figure 1. Morphological traits of dominant species in different plots (SG, sheep grazing; CG, cattle grazing; MG, mixed grazing with cattle and sheep; NG, no grazing) showing (A) Vegetative height, (B) Stem leaf ratio, (C) Leaf dry matter content, (D) Root shoot ratio, (E) Specific leaf area. In each graph, means with the same lowercase letters are not significantly different among plots (P > 0.05); means with different lowercase letters are significantly different among plots (P < 0.05). Capped lines represent ± standard error.
Chemical Traits
There was no significant difference in the RNC of S. grandis and L. chinensis among plots (S. grandis F3,20 = 0.500, P = 0.687; L. chinensis F3,20 = 0.196, P = 0.898). The RNC of C. squarrosa was lowest in the NG plots (Figure 2A). The grazing treatment had no significant effect on the RPC of L. chinensis (P > 0.05). The RPC of S. grandis was lowest in the CG plots, while the RPC of C. squarrosa was lowest in the NG plots (Figure 2B). In the CG plots, L. chinensis had the lowest RNPR, and S. grandis had the highest RNPR. The RNPR of C. squarrosa was not significantly affected by the grazing treatment (C. squarrosa F3,20 = 1.117, P = 0.368, Figure 2C).
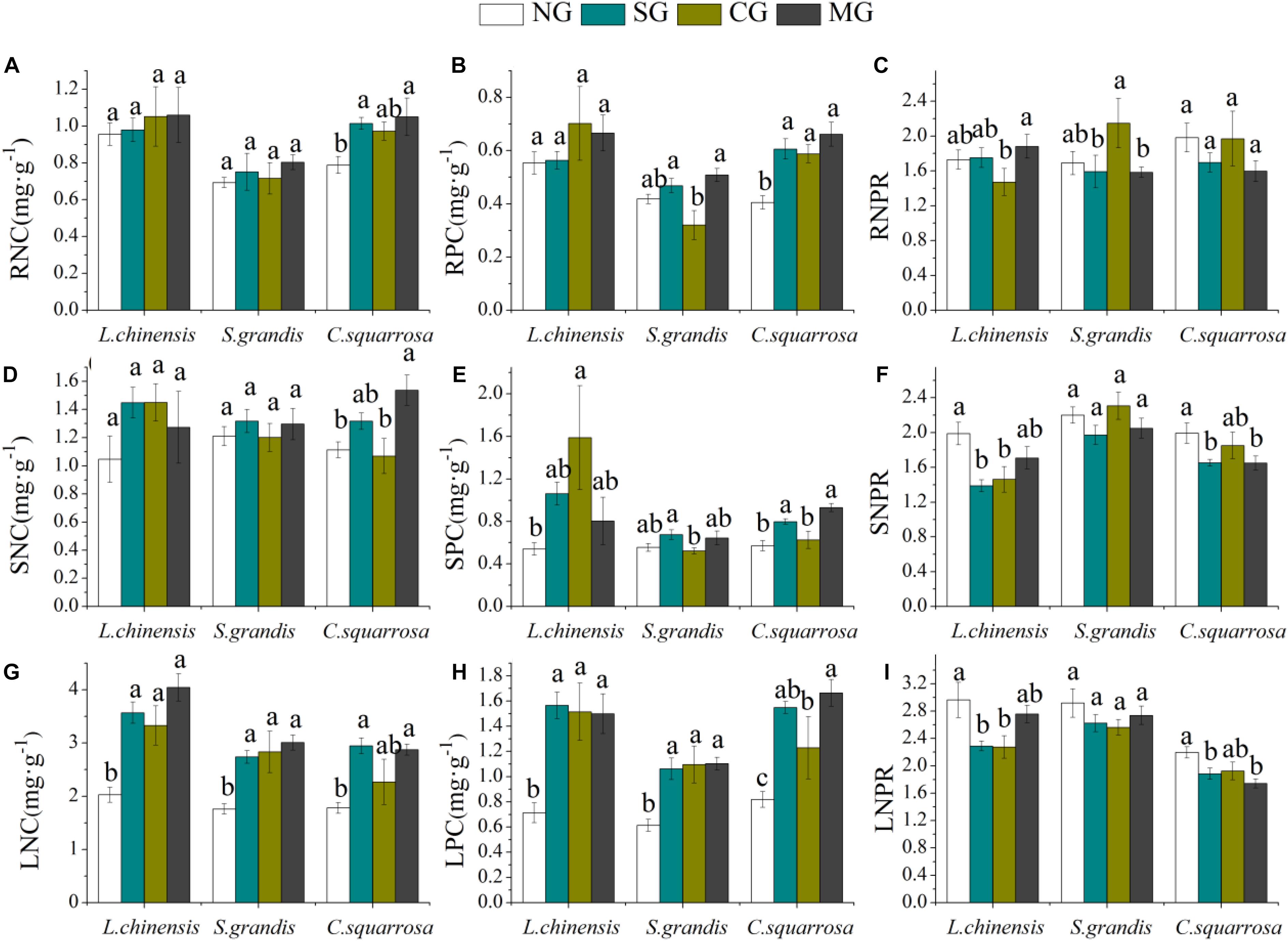
Figure 2. Chemical traits of dominant species in different plots (SG, sheep grazing; CG, cattle grazing; MG, mixed grazing with cattle and sheep; NG, no grazing) showing (A) Root nitrogen concentration, (B) Root phosphorus concentration, (C) Root nitrogen: phosphorus ratio, (D) Stem nitrogen concentration, (E) Root phosphorus concentration, (F) Stem nitrogen: phosphorus ratio, (G) Leaf nitrogen concentration, (H) Leaf phosphorus concentration, (I) Leaf nitrogen: phosphorus ratio. In each graph, means with the same lowercase letters are not significantly different among plots (P > 0.05); means with different lowercase letters are significantly different among plots (P < 0.05). Capped lines represent ± standard error.
The grazing treatment had no effect on the SNC of the other two species (S. grandis F3,20 = 0.421, P = 0.740, L. chinensis F3,20 = 1.443, P = 0.262), except that of C. squarrosa, which was highest in the MG plots (F3,20 = 5.854, P = 0.005, Figure 2D). In the CG plots, L. chinensis had the highest value of SPC, while S. grandis had the lowest value of SPC. The SPCs of C. squarrosa in the MG and SG plots were significantly higher than those in the CG and NG plots (Figure 2E). The SNPRs of L. chinensis and C. squarrosa were highest in the NG plots. The SNPR of S. grandis did not differ significantly among plots (Figure 2F).
The LNCs of the three species were the lowest in the NG plots (S. grandis, F3,20 = 6.399, P = 0.003; L. chinensis, F3,20 = 11.073, P < 0.001; C. squarrosa, F3,20 = 6.626, P = 0.003; Figure 2G), indicating that grazing can increase the LNCs of plants. Consistent with the response pattern of the LNC to the grazing treatments, the LPCs of the three species also increased significantly after grazing (S. grandis, F3,20 = 6.742, P = 0.003; L. chinensis, F3,20 = 6.556, P = 0.003; C. squarrosa, F3,20 = 9.357, P = 0.001, Figure 2H). Grazing had no significant effect on the LNPR of S. grandis but decreased the LNPR of L. chinensis and C. squarrosa (Figure 2I).
Functional Trait Composition
As shown in Figure 3A, the first two principal components of the PCA for the functional traits of S. grandis explained 53.98% of the variation under the different grazing systems. The first principal component explained 32.25% of the variation and was positively correlated with LNC, LPC, and SLA and negatively correlated with LDMC, VH, and LNPR. The second principal component explained 21.73% of the variation and was positively correlated with the nitrogen and phosphorus contents of roots and stems and negatively correlated with RNPR and SNPR. The CG plots had the lowest scores on this axis.
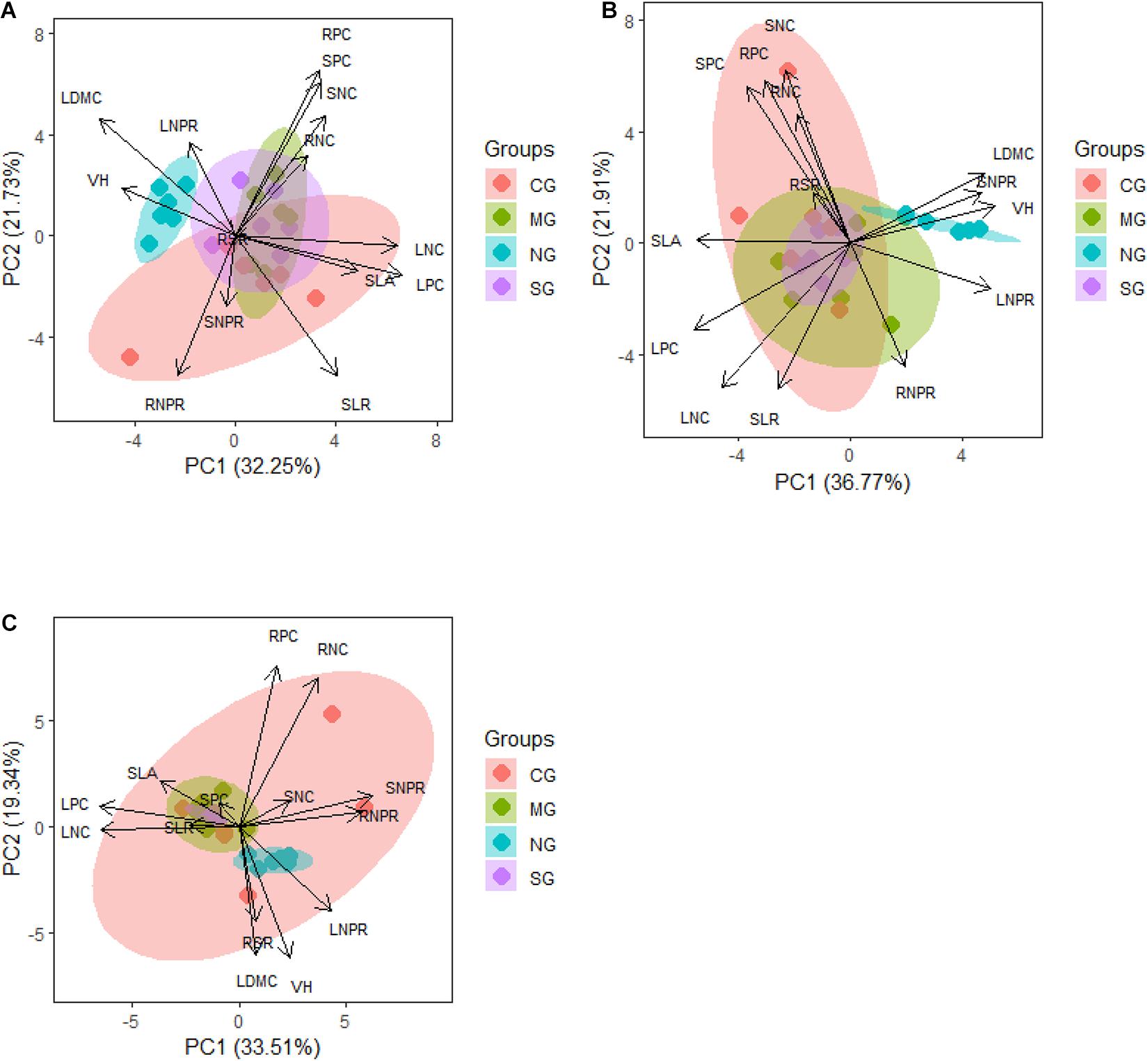
Figure 3. PCA of plant functional traits within Stipa grandis (A), L. chinensis (B), and Cleistogenes squarrosa (C). Plot CG means cattle grazing; plot SG means sheep grazing; plot MG means mixed grazing of cattle and sheep; and NG means no grazing. VH denotes vegetative height; SLR indicates stem-to-leaf ratio; RSR represents root biomass over aboveground biomass; LDMC denotes leaf dry matter content; SLA indicates specific leaf area; RNC represents root nitrogen content; RPC denotes root phosphorus content; RNPR indicates root nitrogen to phosphorus ratio; SNC represents stem nitrogen content; SPC denotes stem nitrogen content; SNPR indicates stem nitrogen content; LNC represents leaf nitrogen content; LPC denotes leaf phosphorus content; and LNPR indicates leaf nitrogen phosphorus ratio.
Figure 3B showed the PCA of the functional traits of L. chinensis under the different grazing systems. The first two principal components explained 58.68% of the variation. The first principal component explained 36.77% of the variation and was positively correlated with VH, LDMC, and SNPR and negatively correlated with SLA. The second principal component explained 21.91% of the variation and was negatively correlated with RNPR and SLR. Therefore, the RNPR and SLR for the MG plots below the horizontal axis were higher than those for the CG plots.
The PCA of the functional traits of C. squarrosa under the different grazing treatments was shown in Figure 3C. The first two principal components explained 52.85% of the variation. The first principal component accounted for 33.51% of the variation and was positively correlated with SNPR and RNPR and negatively correlated with LNC, LPC, and SLR. The second principal component explained 19.34% of the variation and was negatively correlated with LDMC and RSR. Therefore, the LDMC and RSR for the NG plots below the horizontal axis were higher than those for the other plots.
Intraspecific Trait Variability (ITV)
The ITV results calculated for each plant were displayed on a polar map. As shown in Figure 4, for each functional trait of L. chinensis, the ITV was generally high in the CG plots and low in the NG plots. Specifically, the maximum intraspecific variation was found for the SPC (51%) in the SG plots. A minimum of 3% was obtained for the SLA in the NG plots. For all functional traits of S. grandis, the ITV was generally high in the CG plots and low in the NG plots. Specifically, a maximum intraspecific variation of 50% was found for the SLA in the CG plots. A minimum of 2% was obtained for the SLA in the NG plots. For each functional trait of C. squarrosa, the ITV was generally high in the CG plots and low at the NG plots. Specifically, a maximum intraspecific variation of 54% was found for the RSR in the CG plots. A minimum of 2% was obtained for the LDMC in the SG plots.
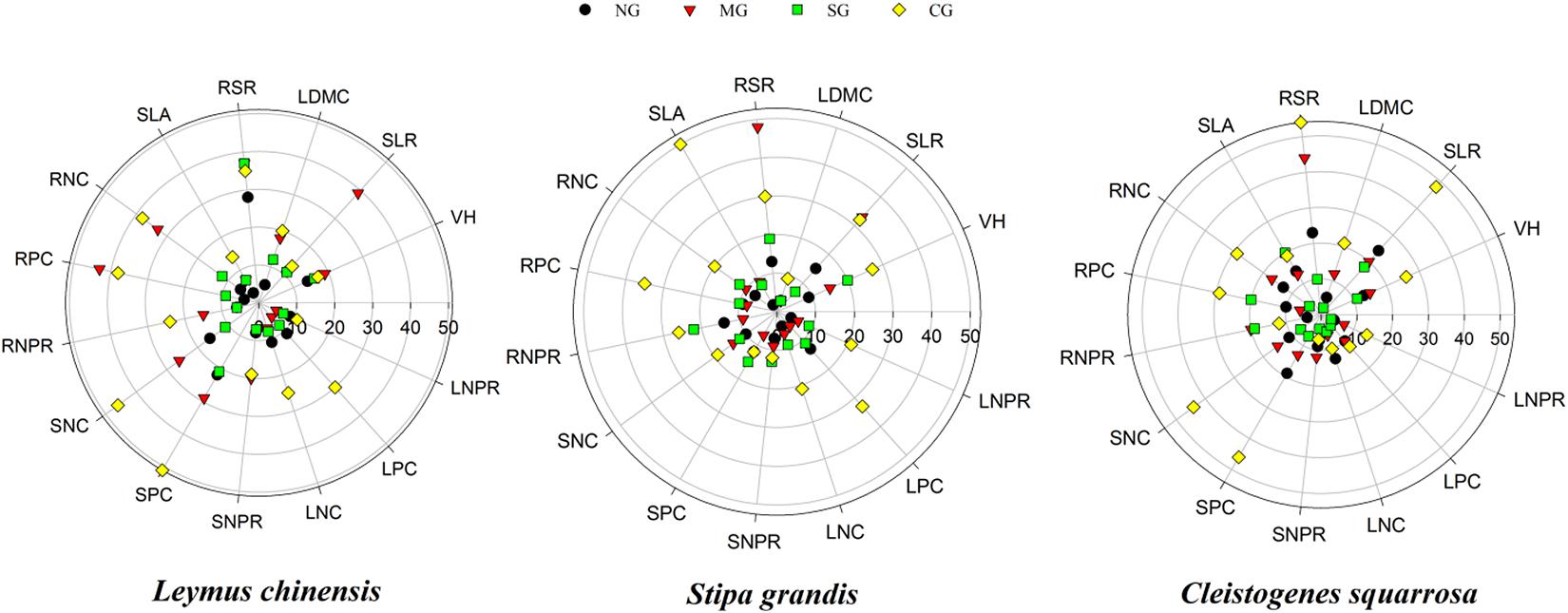
Figure 4. Polar figure of ITV in dominant species at each plot. Plot NG means no grazing; plot MG means mixed grazing of cattle and sheep; plot SG means grazing sheep alone and CG means grazing cattle alone. VH indicates vegetative height, SLR indicater stem-to-leaf ratio, LDMC indicate leaf dry matter content, RSR indicate root biomass over above-ground biomass, SLA indicate specific leaf area, RNC indicate root nitrogen content, RPC indicate root phosphorus content, RNPR indicate root nitrogen to phosphorus ratio, SNC indicate stem nitrogen content, SPC indicate stem nitrogen content, SNPR indicate stem nitrogen content, LNC indicate leaf nitrogen content, LPC indicater leaf phosphorus content, LNPR indicate leaf nitrogen phosphorus ratio.
Discussion
How Do Plants Adjust Their Functional Traits to Adapt to Grazing Disturbance?
Diaz et al. (2001) proposed that some easy-to-measure plant traits can be applied to predict plant responses to grazing and that the responses of some of these traits to grazing are consistent at the global level (Diaz et al., 2007b). Here, we found that compared with NG, regardless of the grazing treatment, the VH, LDMC, SLA, SLR, LNC, and LPC of the three plant species changed significantly. The change trends of the traits were consistent among species after grazing, i.e., a decline in VH and LDMC and an increase in SLA, SLR, LNC, and LPC. Therefore, our study supports the inference that the response of plant functional traits to grazing is consistent from the perspective of the dominant species in the Inner Mongolia grassland (Diaz et al., 2007b).
Changes in plant functional traits reflect both herbivore avoidance and tolerance strategies in plants (Diaz et al., 2001; Cingolani et al., 2005). The plant height at grazed plots was significantly lower than that in the control plot after grazing, and the SLR values increased (Figure 1). This result is consistent with the results of other studies performed in the Inner Mongolia grassland (Zhang et al., 2018). Changes in these two plant functional traits indicate that plants avoid being consumed by livestock by reducing their height and the distribution ratio of their aboveground components (Cingolani et al., 2005; Zheng et al., 2010). For livestock, shorter plants require more bites to consume, which increases the difficulty of their consumption; moreover, the transfer of more aboveground biomass to the stem also reduces the palatability of the plant (Cingolani et al., 2005). Here, we found that the avoidance strategies of the studied species rely heavily on changes in biomass allocation; therefore, more insight into this strategy will need to be gained by evaluating plant traits, including the above- and belowground fractions (de la Riva et al., 2016). On the other hand, we found that after grazing, LDMC decreased, while SLA and LNC increased. The combined changes in these traits indicate that the photosynthetic rate of the plants increased and that their growth rate accelerated (Reich et al., 1997; Quetier et al., 2007). These responses increase the ability of each plant to obtain resources to restore its lost tissues and ensure its growth (Garnier et al., 2004) and thus reflect the ability of plants to adapt to the environment. In addition, high LNC and low LDMC also indicate increased palatability in plant leaves (Perez-Harguindeguy et al., 2003), which increases herbivore selectivity toward these plants; these responses indicate that a species is tolerant to grazing (Diaz et al., 2007b). Changes in plant traits with which plants avoid grazing decrease the aboveground biomass of grassland ecosystems, but the traits related to grazing tolerance generate compensatory growth. These opposing processes keep the biomass and ecosystem functions of grassland ecosystems relatively stable under appropriate grazing pressure. Our results indicated a tradeoff between plant functional traits and ecosystem functions that helps to maintain the stability and sustainability of the ecosystem.
Studying the changes in plant nutrient concentrations and nutrient allocation is helpful in explaining the impact of grazing on grassland ecosystems (Zechmeister-Boltenstern et al., 2015). Here, we found that sheep grazing, cattle grazing, and mixed grazing with sheep and cattle increased the LNC and LPC of the three plants compared to the no grazing treatment (Figure 2). The return of nutrient-rich feces and urine of livestock can directly provide rapid nutrient replenishment, thereby significantly improving nutrient use efficiency and possibly stimulating plant production and soil microbial activities (Frost and Hunter, 2004; Van der Waal et al., 2011). On the other hand, herbivores have an indirect effect on nutrient dynamics by affecting the rate of litter decomposition. Previous studies have found that grazing can affect the quality and quantity of litter (e.g., the quality of litter is higher in the presence of grazing); in addition, the feces and urine of herbivores can accelerate the decomposition rate of litter by returning highly decomposable resources (Bakker et al., 2004). Herbivores thus have an important role in regulating nutrient cycling in grassland ecosystems, which cause a significant increase in soil mineral nitrogen after grazing (Wang et al., 2018). Therefore, the increase in soil mineral nitrogen may be the main reason for the increase in leaf nitrogen caused by grazing. Leaf phosphorous and nitrogen generally increase consistently with an increase in soil fertility (Ordonez et al., 2009), which causes leaf phosphorous to respond to grazing in the same manner as leaf nitrogen. Grazing also has an effect on root and stem nutrient traits, however, nutrient traits of roots and stems were not as obvious as those of leaf traits in response to grazing (Figure 2). In this study, we also found that the leaf nitrogen and phosphorus concentration of the three plants has a weak correlations with the root and stem nitrogen and phosphorus concentrations (Figure 3). These results seem to be inconsistent with the result of Kramer-Walter et al. (2016), who found that root, stem and leaf tissue traits are functionally coordinated to maximize the efficiency of acquiring resources. This coordination may be attributed to the notion that the responses of plant roots and stems to grazing disturbances exhibits a certain lag effect, rendering the traits of roots and stems more stable than those of leaves (Milchunas and Vandever, 2013).
Our results indicate that the intraspecific variability of plant functional traits increased after grazing. The intraspecific variation in plant functional traits was the lowest under non-grazing conditions Figure 4). Plants adapt to biological and abiotic gradients primarily through ITV (Ackerly and Cornwell, 2007; Martinez-Vilalta et al., 2009). ITVs affect their ability to respond to environmental changes as well as their evolutionary development and ultimately determine the breadth of their ecological niche (Nicotra et al., 2010; Laforest-Lapointe et al., 2014). Albert et al. (2010) found a high ITV under certain environmental conditions; here, we emphasize the importance of disturbance factors such as grazing. Plants make their functional traits more “adaptive” to grazing disturbance by increasing their intraspecific variability, thereby expanding their niche to obtain more resources and pass through environmental filters (Jung et al., 2010; de la Riva et al., 2016). Therefore, in addition to adjusting their functional traits (values) to adapt to environmental pressures, plants may also survive adverse environmental conditions by increasing their intraspecific variability (Messier et al., 2010).
Overall, the investigated plant species exhibited higher SLA, LNC, and LPC and lower LDMC after grazing. These responses show that the plants adapted to the environment by changing their growth strategies and providing compensatory growth (Reich et al., 1997; Garnier et al., 2004; Wright et al., 2004). These changes promote the accumulation of ecosystem biomass and accelerate the ecosystem cycling rate (Zheng et al., 2010; Bai et al., 2012), specifically by improving biodiversity, primary productivity and water use efficiency. Thus, we recommend adopting moderate-intensity grazing for grassland management in Inner Mongolia.
Does Grazing With Only One Livestock Type Have a Stronger Adverse Effect on Plant Functional Traits Than Mixed Grazing?
Compared with its SLA under the other two grazing treatments, L. chinensis had its lowest SLA under the SG treatment, and more of its biomass was distributed to the roots (Figure 1). Moreover, the LDMC of L. chinensis under SG was higher than that under the other two grazing treatments, while its average VH was the lowest (although the difference was not significant, Figure 1). The combination of these trait changes leads to the following conclusion: L. chinensis is more inclined to avoid sheep grazing than to tolerate it. Zhao et al. (2009) found that long-term grazing altered both the morphological and functional traits of L. chinensis; here, our research further shows that grazing only sheep will strengthen these effects. Pan et al. (2017) found that sheep greatly damaged the growth points of L. chinensis, leaving residual stems that were unable to continue growing, and that sheep grazing inhibited L. chinensis growth more than it inhibited the growth of other species. L. chinensis, which is highly palatable for livestock animals and has considerable economic value, is one of the most important native perennial forage species of the Inner Mongolia grassland (Wang et al., 2004; Zheng et al., 2011). Therefore, to reduce the further consumption and destruction of L. chinensis populations, it is recommended to limit the practice of grazing only sheep.
In this study, we found that the CG treatment had a greater impact on the functional traits of S. grandis and a smaller effect on the functional traits of C. squarrosa. S. grandis showed a higher SLR and lower SLA, SPC, and RPC under CG than under the other treatments. The increase in the SLR indicates that the proportion of S. grandis leaves decreased. Because leaves are the photosynthetic organs of a plant, this change in biomass allocation inevitably cause a decrease in the photosynthetic rate of S. grandis and a slowed growth rate (Garnier et al., 2004). Similarly, SLA, SPC, and RPC are positively correlated with the photosynthetic efficiencies and growth rates of plants (Reich et al., 1997; Quetier et al., 2007). Therefore, compared with the other two grazing treatments, CG significantly reduced the growth efficiency of S. grandis, causing very adverse effects on S. grandis. In contrast, the morphological and chemical trait values of C. squarrosa in the CG plots were most similar to those in the control plots (Figures 1, 2), indicating that cattle had a minimal effect on the functional traits of C. squarrosa. Because cattle graze plants with their tongues, they tend to eat plants that are taller and have more biomass (Schwartz and Ellis, 1981). Due to its low VH, C. squarrosa successfully avoided being consumed by cattle. The Inner Mongolia grassland is an important part of the Eurasian temperate grassland area, which is the largest terrestrial biome in the world, and S. grandis is one of the most important species in this area (Bai et al., 2004). Some S. grandis communities in this area have been degraded by overgrazing cattle or sheep, and some of them are likely to become C. squarrosa communities (Pan et al., 2016). The results of this study explain the internal mechanism of this change and emphasize that cattle grazing may be an important reason for this kind of community transformation.
Our results indicate that the intraspecific variability of plant functional traits increases more obviously under cattle-only grazing than under the other grazing systems tested (Figure 4). Plants will exhibit greater intraspecies trait variability when they are subject to increased abiotic or biological constraints (Violle et al., 2007). Jung et al. (2010) found that the intraspecific variability in SLA and height increased with an increase in flooding stress. Our results are similar to their results: the plants in the control plot were the least disturbed; their species traits were the most clustered together in the PCA; and the value of intraspecific variability of plant functional traits was low (Figures 3, 4). Therefore, to a certain extent, the intraspecies variability of functional traits can indicate the magnitude of environmental pressure on the species in a habitat, and increased intraspecies variability indicates greater environmental pressure. In this study, the plant species traits were the most dispersed under the CG treatment (Figure 3B), indicating that the plants under this treatment were under the most grazing pressure. Albert et al. (2010) believe that intraspecific effects are more important along short environmental gradients that include minimal changes in species composition than under other conditions. Therefore, for regional grassland management, it is recommended that cattle-only grazing be reduced to alleviate the pressure on grasslands.
Large-herbivore grazing is a compound stressor that affects grassland ecosystems mainly through feeding, feces and trampling (Veen et al., 2014). Different combinations of herbivores exhibit differences in feed intake, which yields differences in the aboveground biomass, soil water content, species composition, and nitrogen availability; these differences further affect the net ecosystem CO2 exchange and the total ecosystem productivity (Bakker et al., 2004; Hodel et al., 2014; Liu et al., 2015, 2016; Risch et al., 2015). Mixed grazing by a combination of different livestock species affects grassland ecosystems in diverse ways rather than having only a single effect, which is helpful in reducing the negative impact of grazing activities on grassland ecosystems. In the context of achieving appropriate grazing pressure, mixed grazing can not only maintain the plant community structure but also promote the compensatory growth of plants and accelerate material circulation and energy flow. Therefore, we recommend that mixed cattle and sheep grazing be implemented in the management of the Inner Mongolia grassland. Our study provides evidence from the perspective of plant functional trait responses to different grazing systems in the Inner Mongolia grassland. The evidence suggests that compared with single grazing, mixed cattle and sheep grazing should be the preferred grazing system for the Inner Mongolia grassland.
Conclusion
Our research shows that VH, LDMC, SLA, plant biomass allocation fractions, and plant nutrients can be used to predict plant responses to grazing and that these traits exhibit consistent responses to grazing in the dominant plant species on the Inner Mongolia grassland. Plants avoid grazing by becoming smaller and by reducing their leaf allocation ratio. They tolerate grazing by increasing their SLA and nitrogen and phosphorous contents and by reducing the LDMC. In addition, plants may also adapt to unfavorable living conditions by increasing the intraspecific variability of their functional traits. In addition, our research found that compared with other grazing methods, grazing only sheep had a greater impact on the functional traits of the L. chinensis population than on those of the other species and adversely affected its survival and growth. Grazing only cattle had a greater impact on the functional traits of S. grandis than on those of the other species. The functional traits of C. squarrosa plants were less affected by grazing than those of the other species, which may be an important mechanism by which S. grandis grasslands degenerate into C. squarrosa grasslands. Because grazing can promote plant growth, mixed grazing had milder effects than single grazing on the functional traits of dominant plant species in the Inner Mongolia grassland. Therefore, we conclude that mixed cattle and sheep grazing should be adopted for the management of the Inner Mongolia grassland.
Data Availability Statement
The raw data supporting the conclusions of this article will be made available by the authors, without undue reservation.
Author Contributions
JZ conceived the idea of the study. ZW and JZ conducted the statistical analyses and led the writing with major inputs from ZL, HL, LW, and CL. All authors revised, read, and agreed to the published version of the manuscript.
Funding
This research was funded by the National Natural Science Foundation of China (grant number 31960243), the Natural Science Foundation of Inner Mongolia (grant number 2018BS03006), the Science and Technology Major Project of Inner Mongolia grant number ZDZX2018054, and Science Technology Project of Inner Mongolia (grant number 201802100).
Conflict of Interest
The authors declare that the research was conducted in the absence of any commercial or financial relationships that could be construed as a potential conflict of interest.
The reviewer WM declared a shared affiliation, though no other collaboration, with one of the authors with the authors to the handling editor.
Acknowledgments
The authors thank Yantao Wu, Hao Li, Xing Li, and Zeyu Yang for their contributions to the data collection. We appreciate the helpful comments of Jürgen Dengler and Idoia Biurrun, which improved the quality of the manuscript.
References
Ackerly, D. D., and Cornwell, W. K. (2007). A trait-based approach to community assembly: partitioning of species trait values into within- and among-community components. Ecol. Lett. 10, 135–145. doi: 10.1111/j.1461-0248.2006.01006.x
Albert, C. H., Grassein, F., Schurr, F. M., Vieilledent, G., and Violle, C. (2011). When and how should intraspecific variability be considered in trait-based plant ecology? Perspect. Plant Ecol. 13, 217–225. doi: 10.1016/j.ppees.2011.04.003
Albert, C. H., Thuiller, W., Yoccoz, N. G., Soudant, A., Boucher, F., Saccone, P., et al. (2010). Intraspecific functional variability: extent, structure and sources of variation. J. Ecol. 98, 604–613. doi: 10.1111/j.1365-2745.2010.01651.x
Asner, G. P., Elmore, A. J., Olander, L. P., Martin, R. E., and Harris, A. T. (2004). Grazing systems, ecosystem responses, and global change. Annu. Rev. Env. Resour. 29, 261–299. doi: 10.1146/annurev.energy.29.062403.102142
Bai, Y. F., Han, X. G., Wu, J. G., Chen, Z. Z., and Li, L. H. (2004). Ecosystem stability and compensatory effects in the inner mongolia grassland. Nature 431, 181–184. doi: 10.1038/nature02850
Bai, Y. F., Wu, J. G., Clark, C. M., Pan, Q. M., Zhang, L. X., Chen, S. P., et al. (2012). Grazing alters ecosystem functioning and C:N:P stoichiometry of grasslands along a regional precipitation gradient. J. Appl. Ecol. 49, 1204–1215. doi: 10.1111/j.1365-2664.2012.02205.x
Bakker, E. S., Olff, H., Boekhoff, M., Gleichman, J. M., and Berendse, F. (2004). Impact of herbivores on nitrogen cycling: contrasting effects of small and large species. Oecologia 138, 91–101. doi: 10.1007/s00442-003-1402-5
Cingolani, A. M., Posse, G., and Collantes, M. B. (2005). Plant functional traits, herbivore selectivity and response to sheep grazing in Patagonian steppe grasslands. J. Appl. Ecol. 42, 50–59. doi: 10.1111/j.1365-2664.2004.00978.x
Cornelissen, J. H. C., Lavorel, S., Garnier, E., Diaz, S., Buchmann, N., Gurvich, D. E., et al. (2003). A handbook of protocols for standardised and easy measurement of plant functional traits worldwide. Aust. J. Bot. 51, 335–380. doi: 10.1071/bt02124
Davidson, A. M., Jennions, M., and Nicotra, A. B. (2011). Do invasive species show higher phenotypic plasticity than native species and, if so, is it adaptive? A meta-analysis. Ecol. Lett. 14, 419–431. doi: 10.1111/j.1461-0248.2011.01596.x
de la Riva, E. G., Perez-Ramos, I. M., Tosto, A., Navarro-Fernandez, C. M., Olmo, M., Maranon, T., et al. (2016). Disentangling the relative importance of species occurrence, abundance and intraspecific variability in community assembly: a trait-based approach at the whole-plant level in Mediterranean forests. Oikos 125, 354–363. doi: 10.1111/oik.01875
Diaz, S., Kattge, J., Cornelissen, J. H. C., Wright, I. J., Lavorel, S., Dray, S., et al. (2016). The global spectrum of plant form and function. Nature 529, 167–173. doi: 10.1038/nature16489
Diaz, S., Lavorel, S., de Bello, F., Quetier, F., Grigulis, K., and Robson, M. (2007a). Incorporating plant functional diversity effects in ecosystem service assessments. Proc. Natl. Acad. Sci. U.S.A. 104, 20684–20689. doi: 10.1073/pnas.0704716104
Diaz, S., Lavorel, S., McIntyre, S., Falczuk, V., Casanoves, F., Milchunas, D. G., et al. (2007b). Plant trait responses to grazing - a global synthesis. Glob. Change Biol. 13, 313–341. doi: 10.1111/j.1365-2486.2006.01288.x
Diaz, S., Noy-Meir, I., and Cabido, M. (2001). Can grazing response of herbaceous plants be predicted from simple vegetative traits? J. Appl. Ecol. 38, 497–508. doi: 10.1046/j.1365-2664.2001.00635.x
Fan, F., Liang, C. Z., Tang, Y. K., Harker-Schuch, I., and Porter, J. R. (2019). Effects and relationships of grazing intensity on multiple ecosystem services in the Inner Mongolian steppe. Sci. Total Environ. 675, 642–650. doi: 10.1016/j.scitotenv.2019.04.279
Frost, C. J., and Hunter, M. D. (2004). Insect canopy herbivory and frass deposition affect soil nutrient dynamics and export in oak mesocosms. Ecology 85, 3335–3347. doi: 10.1890/04-0003
Garnier, E., Cortez, J., Billes, G., Navas, M. L., Roumet, C., Debussche, M., et al. (2004). Plant functional markers capture ecosystem properties during secondary succession. Ecology 85, 2630–2637. doi: 10.1890/03-0799
Guo, Y. J., Du, Q. F., Li, G. D., Ni, Y., Zhang, Z., Ren, W. B., et al. (2016). Soil phosphorus fractions and arbuscular mycorrhizal fungi diversity following long-term grazing exclusion on semi-arid steppes in inner mongolia. Geoderma 269, 79–90. doi: 10.1016/j.geoderma.2016.01.039
Hodel, M., Schuetz, M., Vandegehuchte, M. L., Frey, B., Albrecht, M., Busse, M. D., et al. (2014). Does the aboveground herbivore assemblage influence soil bacterial community composition and richness in subalpine grasslands? Microb. Ecol. 68, 584–595. doi: 10.1007/s00248-014-0435-0
Jung, V., Violle, C., Mondy, C., Hoffmann, L., and Muller, S. (2010). Intraspecific variability and trait-based community assembly. J. Ecol. 98, 1134–1140. doi: 10.1111/j.1365-2745.2010.01687.x
Kattge, J., Diaz, S., Lavorel, S., Prentice, C., Leadley, P., Boenisch, G., et al. (2011). TRY - a global database of plant traits. Glob. Change Biol. 17, 2905–2935. doi: 10.1111/j.1365-2486.2011.02451.x
Kemp, D. R., Han, G., Hou, X., Michalk, D. L., Hou, F., Wu, J., et al. (2013). Innovative grassland management systems for environmental and livelihood benefits. Proc. Natl. Acad. Sci. U.S.A. 110, 8369–8374. doi: 10.1073/pnas.1208063110
Knapp, A. K., Hoover, D. L., Blair, J. M., Buis, G., Burkepile, D. E., Chamberlain, A., et al. (2012). A test of two mechanisms proposed to optimize grassland aboveground primary productivity in response to grazing. J. Plant Ecol. 5, 357–365. doi: 10.1093/jpe/rts020
Kramer-Walter, K. R., Bellingham, P. J., Millar, T. R., Smissen, R. D., Richardson, S. J., and Laughlin, D. C. (2016). Root traits are multidimensional: specific root length is independent from root tissue density and the plant economic spectrum. J. Ecol. 104, 1299–1310. doi: 10.1111/1365-2745.12562
Kumordzi, B. B., Aubin, I., Cardou, F., Shipley, B., Violle, C., Johnstone, J., et al. (2019). Geographic scale and disturbance influence intraspecific trait variability in leaves and roots of North American understorey plants. Funct. Ecol. 33, 1771–1784. doi: 10.1111/1365-2435.13402
Laforest-Lapointe, I., Martinez-Vilalta, J., and Retana, J. (2014). Intraspecific variability in functional traits matters: case study of Scots pine. Oecologia 175, 1337–1348. doi: 10.1007/s00442-014-2967-x
Lang, B., Ahlborn, J., Oyunbileg, M., Geiger, A., von Wehrden, H., Wesche, K., et al. (2020). Grazing effects on intraspecific trait variability vary with changing precipitation patterns in Mongolian rangelands. Ecol. Evol. 10, 678–691. doi: 10.1002/ece3.5895
Lavorel, S., Grigulis, K., Lamarque, P., Colace, M.-P., Garden, D., Girel, J., et al. (2011). Using plant functional traits to understand the landscape distribution of multiple ecosystem services. J. Ecol. 99, 135–147. doi: 10.1111/j.1365-2745.2010.01753.x
Leoni, E., Altesor, A., and Paruelo, J. M. (2009). Explaining patterns of primary production from individual level traits. J. Veg. Sci. 20, 612–619. doi: 10.1111/j.1654-1103.2009.01080.x
Lin, B., Tan, Z. L., Tang, S. X., Sun, Z. H., and Wang, M. (2008). Research progress in methodologies for carrying capacity and proper stocking rate in grassland ecological system. Pratacult. Sci. 8, 91–99. doi: 10.1007/s10499-007-9164-4
Liu, C., Song, X. X., Wang, L., Wang, D. L., Zhou, X. M., Liu, J., et al. (2016). Effects of grazing on soil nitrogen spatial heterogeneity depend on herbivore assemblage and pre-grazing plant diversity. J. Appl. Ecol. 53, 242–250. doi: 10.1111/1365-2664.12537
Liu, J., Feng, C., Wang, D. L., Wang, L., Wilsey, B. J., and Zhong, Z. W. (2015). Impacts of grazing by different large herbivores in grassland depend on plant species diversity. J. Appl. Ecol. 52, 1053–1062. doi: 10.1111/1365-2664.12456
Martinez-Vilalta, J., Cochard, H., Mencuccini, M., Sterck, F., Herrero, A., Korhonen, J. F. J., et al. (2009). Hydraulic adjustment of Scots pine across Europe. New Phytol. 184, 353–364. doi: 10.1111/j.1469-8137.2009.02954.x
Messier, J., McGill, B. J., and Lechowicz, M. J. (2010). How do traits vary across ecological scales? A case for trait-based ecology. Ecol. Lett. 13, 838–848. doi: 10.1111/j.1461-0248.2010.01476.x
Milchunas, D. G., and Vandever, M. W. (2013). Grazing effects on aboveground primary production and root biomass of early-seral, mid-seral, and undisturbed semiarid grassland. J. Arid Environ. 92, 81–88. doi: 10.1016/j.jaridenv.2013.01.012
Muthoni, F. K., Groen, T. A., Skidmore, A. K., and van Oel, P. (2014). Ungulate herbivory overrides rainfall impacts on herbaceous regrowth and residual biomass in a key resource area. J. Arid. Environ. 100, 9–17. doi: 10.1016/j.jaridenv.2013.09.007
Nicotra, A. B., Atkin, O. K., Bonser, S. P., Davidson, A. M., Finnegan, E. J., Mathesius, U., et al. (2010). Plant phenotypic plasticity in a changing climate. Trends Plant Sci. 15, 684–692. doi: 10.1016/j.tplants.2010.09.008
Niu, K. C., Zhang, S. T., Zhao, B. B., and Du, G. Z. (2010). Linking grazing response of species abundance to functional traits in the Tibetan alpine meadow. Plant Soil 330, 215–223. doi: 10.1007/s11104-009-0194-8
O’Mara, F. P. (2012). The role of grasslands in food security and climate change. Ann. Bot. London 110, 1263–1270. doi: 10.1093/aob/mcs209
Ordonez, J. C., Van Bodegom, P. M., Witte, J. P. M., Wright, I. J., Reich, P. B., and Aerts, R. (2009). A global study of relationships between leaf traits, climate and soil measures of nutrient fertility. Glob. Ecol. Biogeogr. 18, 137–149. doi: 10.1111/j.1466-8238.2008.00441.x
Pan, Q. M., Tian, D. S., Naeem, S., Auerswald, K., Elser, J. J., Bai, Y. F., et al. (2016). Effects of functional diversity loss on ecosystem functions are influenced by compensation. Ecology 97, 2293–2302. doi: 10.1002/ecy.1460
Pan, Y., Gong, J. R., Baoyin, T. G. T., Luo, Q. P., Zhai, Z. W., Xu, S., et al. (2017). Effect of seasonal grazing on trade-off among plant functional traits in root, stem and leaf of leymus chinensis in the temperate grassland of inner mongolia, China. Chin. Bull. Bot. 52, 307–321. doi: 10.11983/CBB16109
Perez-Harguindeguy, N., Diaz, S., Garnier, E., Lavorel, S., Poorter, H., Jaureguiberry, P., et al. (2013). New handbook for standardised measurement of plant functional traits worldwide. J. Bot. 64, 715–716. doi: 10.1071/bt12225_co
Perez-Harguindeguy, N., Diaz, S., Vendramini, F., Cornelissen, J. H. C., Gurvich, D. E., and Cabido, M. (2003). Leaf traits and herbivore selection in the field and in cafeteria experiments. Austral. Ecol. 28, 642–650. doi: 10.1046/j.1442-9993.2003.01321.x
Quetier, F., Lavorel, S., Thuiller, W., and Davies, I. (2007). Plant-trait-based modeling assessment of ecosystem-service sensitivity to land-use change. Ecol. Appl. 17, 2377–2386. doi: 10.1890/06-0750.1
Reich, P. B., Walters, M. B., and Ellsworth, D. S. (1997). From tropics to tundra: global convergence in plant functioning. Proc. Natl. Acad. Sci. U.S.A. 94, 13730–13734. doi: 10.1073/pnas.94.25.13730
Reichstein, M., Bahn, M., Mahecha, M. D., Kattge, J., and Baldocchi, D. D. (2014). Linking plant and ecosystem functional biogeography. Proc. Natl. Acad. Sci. U.S.A. 111, 13697–13702. doi: 10.1073/pnas.1216065111
Risch, A. C., Schuetz, M., Vandegehuchte, M. L., van der Putten, W. H., Duyts, H., Raschein, U., et al. (2015). Aboveground vertebrate and invertebrate herbivore impact on net N mineralization in subalpine grasslands. Ecology 96, 3312–3322. doi: 10.1890/15-0300.1
Salazar, P. C., Navarro-Cerrillo, R. M., Cruz, G., and Villar, R. (2018). Intraspecific leaf functional trait variability of eight Prosopis pallida tree populations along a climatic gradient of the dry forests of northern Peru. J. Arid Environ. 152, 12–20. doi: 10.1016/j.jaridenv.2018.01.010
Schulte, L. A., and Mladenoff, D. J. (2005). Severe wind and fire regimes in northern forests: historical variability at the regional scale. Ecology 86, 431–445. doi: 10.1890/03-4065
Schwartz, C. C., and Ellis, J. E. (1981). Feeding ecology and niche separation in some native and domestic ungulates on the short grass prairie. J. Appl. Ecol. 18, 343–354. doi: 10.2307/2402399
Thebault, E., and Loreau, M. (2003). Food-web constraints on biodiversity-ecosystem functioning relationships. Proc. Natl. Acad. Sci. U.S.A. 100, 14949–14954. doi: 10.1073/pnas.2434847100
Tong, C., Wu, J., Yong, S., Yang, J., and Yong, W. (2004). A landscape-scale assessment of steppe degradation in the Xilin River Basin, Inner Mongolia, China. J. Arid Environ. 59, 133–149. doi: 10.1016/j.jaridenv.2004.01.004
Van der Waal, C., Kool, A., Meijer, S. S., Kohi, E., Heitkonig, I. M. A., de Boer, W. F., et al. (2011). Large herbivores may alter vegetation structure of semi-arid savannas through soil nutrient mediation. Oecologia 165, 1095–1107. doi: 10.1007/s00442-010-1899-3
Veen, G. F., de Vries, S., Bakker, E. S., van der Putten, W. H., and Olff, H. (2014). Grazing-induced changes in plant-soil feedback alter plant biomass allocation. Oikos 123, 800–806. doi: 10.1111/j.1600-0706.2013.01077.x
Venier, L. A., Thompson, I. D., Fleming, R., Malcolm, J., Aubin, I., Trofymow, J. A., et al. (2014). Effects of natural resource development on the terrestrial biodiversity of Canadian boreal forests. Environ. Rev. 22, 457–490. doi: 10.1139/er-2013-0075
Violle, C., Navas, M.-L., Vile, D., Kazakou, E., Fortunel, C., Hummel, I., et al. (2007). Let the concept of trait be functional! Oikos 116, 882–892. doi: 10.1111/j.2007.0030-1299.15559.x
von Wehrden, H., Hanspach, J., Kaczensky, P., Fischer, J., and Wesche, K. (2012). Global assessment of the non-equilibrium concept in rangelands. Ecol. Appl. 22, 393–399. doi: 10.1890/11-0802.1
Wang, J. Z., Wang, D. L., Li, C. Q., Seastedt, T. R., Liang, C. Z., Wang, L., et al. (2018). Feces nitrogen release induced by different large herbivores in a dry grassland. Ecol. Appl. 28, 201–211. doi: 10.1002/eap.1640
Wang, L., Delgado-Baquerizo, M., Wang, D. L., Isbell, F., Liu, J., Feng, C., et al. (2019). Diversifying livestock promotes multidiversity and multifunctionality in managed grasslands. Proc. Natl. Acad. Sci. U.S.A. 116, 6187–6192. doi: 10.1073/pnas.1807354116
Wang, L., Wang, D. L., Liu, J. S., Huang, Y., and Hodgkinson, K. C. (2011). Diet selection variation of a large herbivore in a feeding experiment with increasing species numbers and different plant functional group combinations. Acta Oecol. 37, 263–268. doi: 10.1016/j.actao.2011.02.010
Wang, W., Liang, C. Z., Liu, Z. L., and Hao, D. Y. (2000). Mechanism of degradation succession in Leymus chinensis + Stipa grandis steppe community. Acta Phytoecol. Sin. 24, 468–472.
Wang, W., Liu, Z. L., Hao, D. Y., and Liang, C. Z. (1996). Research on the restoring succession of the degenerated grassland in inner mongolia—i. basic characteristics and driving force for restoration of the degenerated grassland. Acta Phytoecol. Sin. 5, 449–459.
Wang, Z. W., Li, L. H., Han, X. G., and Ming, D. (2004). Do rhizome severing and shoot defoliation affect clonal growth of Leymus chinensis at ramet population level? Acta Oecol. 26, 255–260. doi: 10.1016/j.actao.2004.08.007
Westoby, M., Eldridge, D., and Freudenberger, D. (1999). The LHS strategy scheme in relation to grazing and fire. Proc. Int. Rangeland Cong. 2, 893–896.
Wright, I. J., Reich, P. B., Westoby, M., Ackerly, D. D., Baruch, Z., Bongers, F., et al. (2004). The worldwide leaf economics spectrum. Nature 428, 821–827. doi: 10.1038/nature02403
Zechmeister-Boltenstern, S., Keiblinger, K. M., Mooshammer, M., Penuelas, J., Richter, A., Sardans, J., et al. (2015). The application of ecological stoichiometry to plant-microbial-soil organic matter transformations. Ecol. Monogr. 85, 133–155. doi: 10.1890/14-0777.1
Zhang, J. H., Huang, Y. M., Chen, H. Y., Gong, J. R., Qi, Y., Li, E. G., et al. (2018). Response of plant functional traits at species and community levels to grazing exclusion on Inner Mongolian steppe, China. Rangeland J. 40, 179–189. doi: 10.1071/rj16086
Zhao, W., Chen, S. P., Han, X. G., and Lin, G. H. (2009). Effects of long-term grazing on the morphological and functional traits of Leymus chinensis in the semiarid grassland of Inner Mongolia, China. Ecol. Res. 24, 99–108. doi: 10.1007/s11284-008-0486-0
Zheng, S. X., Lan, Z. C., Li, W. H., Shao, R. X., Shan, Y. M., Wan, H. W., et al. (2011). Differential responses of plant functional trait to grazing between two contrasting dominant C3 and C4 species in a typical steppe of Inner Mongolia, China. Plant Soil. 340, 141–155. doi: 10.1007/s11104-010-0369-3
Keywords: functional traits, grazing systems, intraspecific variability, adaption strategies, Inner Mongolia grassland
Citation: Wang Z, Zhang J, Li Z, Liu H, Wang L, Wang W, Wang Y and Liang C (2021) Single Grazing Is More Detrimental to Grasslands Than Mixed Grazing: Evidence From the Response of Functional Traits of Dominant Plants to Grazing Systems. Front. Ecol. Evol. 9:682289. doi: 10.3389/fevo.2021.682289
Received: 18 March 2021; Accepted: 31 May 2021;
Published: 01 July 2021.
Edited by:
Yun Jäschke, Senckenberg Museum of Natural History Görlitz, GermanyReviewed by:
Wenhong Ma, Inner Mongolia University, ChinaJirui Gong, Beijing Normal University, China
Yongmei Huang, Beijing Normal University, China
Copyright © 2021 Wang, Zhang, Li, Liu, Wang, Wang, Wang and Liang. This is an open-access article distributed under the terms of the Creative Commons Attribution License (CC BY). The use, distribution or reproduction in other forums is permitted, provided the original author(s) and the copyright owner(s) are credited and that the original publication in this journal is cited, in accordance with accepted academic practice. No use, distribution or reproduction is permitted which does not comply with these terms.
*Correspondence: Jinghui Zhang, amh6aGFuZzEwMDFAMTI2LmNvbQ==