- Department of Forest and Conservation Sciences, Faculty of Forestry, University of British Columbia, Vancouver, BC, Canada
In the 2017 and 2018, 2.55 million hectares burned across British Columbia, Canada, including unanticipated large and high-severity fires in many dry forests. To transform forest and fire management to achieve resilience to future megafires requires improved understanding historical fire frequency, severity, and spatial patterns. Our dendroecological reconstructions of 35 plots in a 161-hectare study area in a dry Douglas-fir forest revealed historical fires that burned at a wide range of frequencies and severities at both the plot- and study-area scales. The 23 fires between 1619 and 1943 burned at intervals of 10–30 years, primarily at low- to moderate-severity that scarred trees but generated few cohorts. In contrast, current fire-free intervals of 70–180 years exceed historical maximum intervals. Of the six widespread fires from 1790 to 1905, the 1863 fire affected 86% of plots and was moderate in severity with patches of higher severity that generated cohorts at fine scales only. These results indicate the severity of fires varied at fine spatial scales, and offer little support for the common assertion that periodic, high-severity, stand-initiating events were a component of the mixed-severity fire regime in these forest types. Many studies consider fires in the late 1800s relatively severe because they generated new cohorts of trees, and thus, emphasize the importance of high-severity fires in a mixed-severity fire regime. In our study area, the most widespread and severe fire was not a stand-initiating fire. Rather, the post-1863 cohorts persisted due disruption of the fire regime in the twentieth century when land-use shifted from Indigenous fire stewardship and early European settler fires to fire exclusion and suppression. In absence of low- to moderate-severity fires, contemporary forests are dense with closed canopies that are vulnerable to high-severity fire. Future management should reduce forest densities and to restore stand- and landscape-level heterogeneity and increase forest resilience. The timing and size of repeat treatments such as thinning of subcanopy trees and prescribed burning, including Indigenous fire stewardship, can be guided by our refined understanding of the mixed-severity fire regime that was historically dominated by low- to moderate-severity fires in this dry forest ecosystem.
Introduction
Fire is a dominant disturbance in forests worldwide (Bowman et al., 2009) and interacts with multiple abiotic and biotic disturbances to drive forest composition, structure, and dynamics (Hessburg et al., 2019; Leclerc et al., 2021). In forests where ecosystem-based management aims to emulate the historical range and variability in disturbance (Landres et al., 1999; Swetnam et al., 1999; Keane et al., 2009), knowledge on historical fire frequency, severity and spatial patterns guides choices on stand-level even- or uneven-aged silvicultural systems and landscape-level forest age class distributions, old-growth management areas (e.g., BC Ministries of Environment and Forests, 1995), and fire suppression policies (e.g., BC Wildfire Management Branch, 2012). Historical baselines are also used to gauge departures from historical conditions and determine where ecological restoration or fire hazard mitigation are warranted (Hessburg et al., 2005, 2016; Stephens et al., 2012, 2013; Halofsky et al., 2020).
Along the steep climatic and vegetation gradients characteristic of the montane forests of western North America, mixed-severity fire regimes include fires of variable frequencies and severities forming complex spatial patterns within and among events (Perry et al., 2011; Marcoux et al., 2013; Hessburg et al., 2016, 2019). In these forests, understanding the relative importance of low- versus high-severity fire is critical for sustainable management and effective forest restoration, but remains a point of contention (Hagmann et al., 2021). Specifically, a debate has centered on whether recent high-severity fires in mixed-conifer forests are an inherent part of a mixed-severity fire regimes (Klenner et al., 2008; Williams and Baker, 2012; Odion et al., 2014; Baker, 2015, 2017) or if high-severity fires have exceeded the historical range of variability (Fulé et al., 2013; Stephens et al., 2014; Moritz et al., 2018). Different management actions are associated with these two schools of thought. If the fire regime is not altered, management actions are unnecessary or may have unintended negative consequences on forest productivity or critical habitat (Odion et al., 2014; Baker, 2015; DellaSala and Hanson, 2019). Where fire regimes have been altered, determining the causes and degree of departure is important for taking appropriate management actions (Fulé et al., 1997; Stephens et al., 2013; Hessburg et al., 2016). To avoid risk of generalizing across forest types and oversimplifying treatments, ecosystem-specific reference conditions are required to develop management and restoration treatments to enhance forest resilience to fire and climate change (Agee and Skinner, 2005; Keane et al., 2009; Schoennagel and Nelson, 2011; Hessburg et al., 2016).
Fires of a range of severities burn in the dry mixed-conifer forests of the interior of British Columbia (BC) (Marcoux et al., 2013; Hessburg et al., 2019). Historical ignitions were by lightning and Indigenous fire stewardship (Coogan et al., 2021), with low-severity surface fires burning at intervals < 50 years, with high-severity stand-initiating fires at intervals of 250 years (BC Ministries of Environment and Forests, 1995). Supporting the latter notion, modern fire records show that large, intense fires burn during extreme fire weather (Klenner et al., 2008). Across BC, an average of 1,690 fires burn 150,000 hectares annually (BC Government, 2018), although 92% of fires are successfully suppressed before exceeding 4 ha in size (BC Wildfire Management Branch, 2012). Past management focused on controlling fire under the premise that human lives, communities, critical infrastructure, and economically valuable resources in grasslands and forests required protection (BC Government, 2010). Despite highly successful fire suppression since the 1940s, 3.4 million hectares have burned in British Columbia since 2003, costing over $3 billion (CAD) in direct fire suppression and resulting in tens of thousands of evacuations (Abbott and Chapman, 2018). Combined, the 2003 and 2017 fire seasons burned almost 1.5 million ha, largely in dry mixed-conifer forests with tree mortality exceeding 80% in many areas (Abbott and Chapman, 2018). Although the 2017 fire season broke the British Columbian record for area burned in one fire season (1.2 million ha), it was surpassed in 2018 when an additional 1.35 million hectares burned (BC Government, 2018). The threat of more frequent and severe fires continues to impact the lives and property of the people living in communities surrounded by dry mixed-conifer forests (Coogan et al., 2021). There is great concern that megafires such as those of 2003, 2017, and 2018 will become more common with continued climate change (Abbott and Chapman, 2018), reinforcing recommendations for transformative changes to fire and forest management (North et al., 2015; Stephens et al., 2016, 2020; Daniels et al., 2020).
In 2017, a lightning outbreak during extreme fire weather ignited multiple high-severity fires that burned 33,181 ha of forests surrounding the city of Williams Lake in south central BC (Figure 1A). The combination of ignition locations, wind direction, and fire suppression efforts spared the forests near Knife Creek in the Alex Fraser Research Forest. Nevertheless, these fires underscored the importance of reconstructing historical fire regimes and their influences on forest dynamics. This research was conducted in the dry Douglas-fir forests of the Research Forest to address the following questions: How frequent and severe were historical fires? Did severity vary spatially within widespread fires? Has the fire regime changed during the twentieth century and what are the impacts on forest structure? Dendroecological analyses of fire scars and increment cores sampled from a dense network of 35 plots across a 161-hectare (ha) study area allowed us to reconstruct spatio-temporal variations in fire frequency, severity, and spatial patterns at a fine scale. By critically assessing alternate interpretations of contemporary forest structure in the context of historical fires, we elucidated disruptions to the historical fire regime and provide recommendations to restore forest resilience to fire.
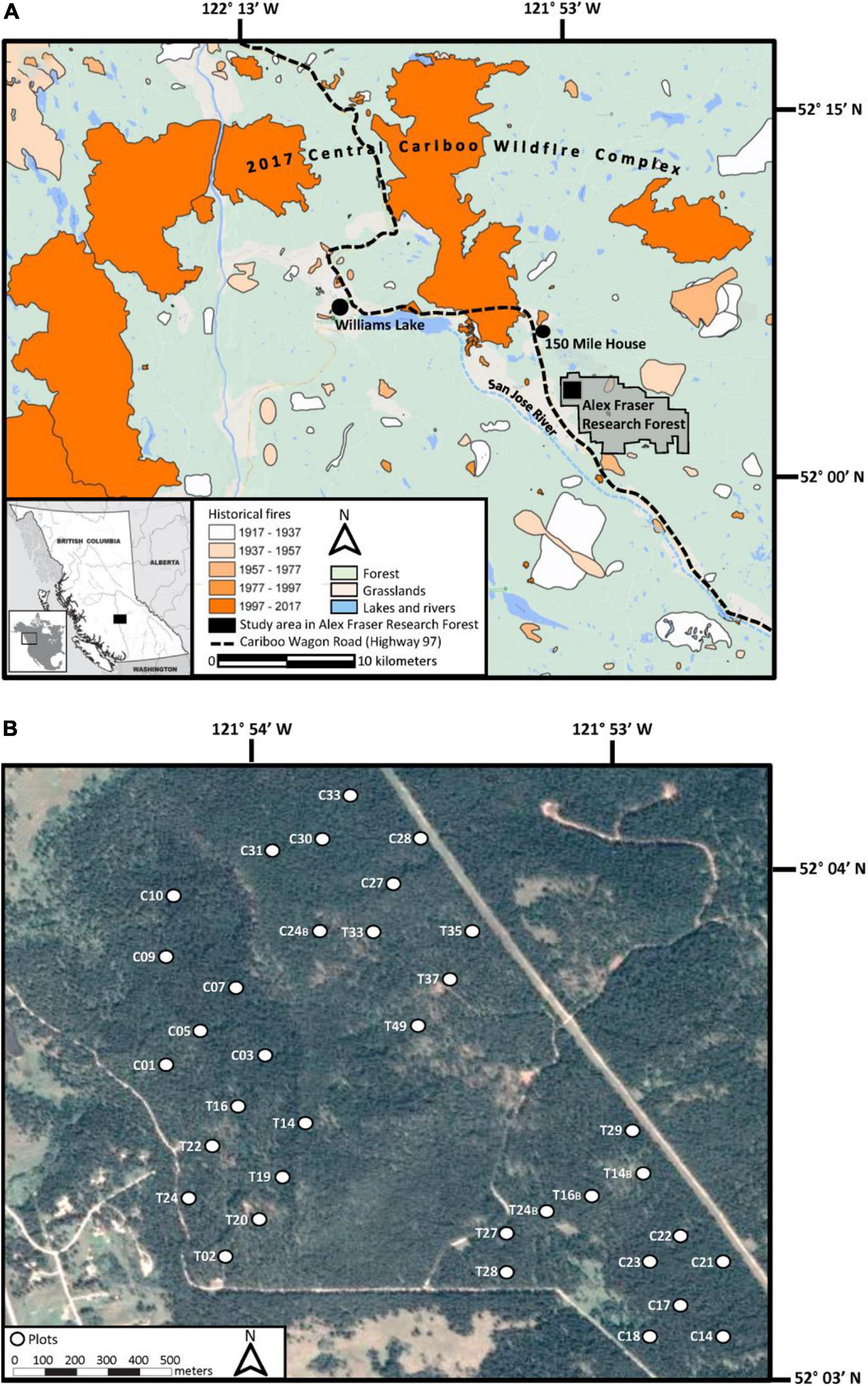
Figure 1. Location of the study area and sampled plots in the Alex Fraser Research Forest in south central British Columbia, Canada. (A) The Cariboo Wagon Road (now Highway 97) and San Jose River lie west of the study area. Polygons in white and oranges depict historical fires between 1917 and 2017. (B) Thirty-five plots were sampled in 161 ha of forest in the northeast corner of the Research Forest. T denotes plots in treated areas that were partially harvested in 1984; C denotes unharvested control plots. [Data sources (A) British Columbia Open Data Catalog and (B) Google Earth].
Materials and Methods
Study Area
The study area encompasses 161 ha near Knife Creek in the Alex Fraser Research Forest, located 24 kilometers southeast of the city of Williams Lake, BC, Canada (Figure 1). It is in the traditional territory of the T’exelcemc (Williams Lake Band), members of the Northern Secwépemc te Qelmucw (Northern Shuswap Tribal Council, 2014). Elevation varies from 761 to 879 m above sea level (masl) and the terrain is flat to gently rolling. The climate is continental and strongly influenced by the rain shadow of the Coastal Mountains, yielding average annual precipitation of 450.7 mm, with approximately half falling in the growing season [1981–2000, Williams Lake Station (52°10′59″N 122°03′15″W, 940 masl), Environment Canada, 2017]. Winter months are dominated by Arctic air masses and December is the coldest month with a mean daily temperature of −7.3°C (Environment Canada, 2017). July is the warmest month with a mean daily temperature of 16.0°C and mean monthly precipitation of 52.7 mm, primarily from convective storms.
The forests near Knife Creek are dominated by interior Douglas-fir [Pseudotsuga menziesii var. glauca (Beissn.) Mayr] which forms closed-canopy stands with well-developed canopy, subcanopy, and regeneration strata. Trembling aspen [Populous tremuloides Michx.] and paper birch [Betula papyrifera Marsh.] are infrequent in the canopy. Although present in the past, living, mature lodgepole pine [Pinus contorta Dougl. var. latifolia Engelm.] are absent from the canopy due to the recent mountain pine beetle [Dendroctonus ponderosae Hopkins] epidemic. Other recent biotic disturbances include defoliation by western spruce budworm [Choristoneura occidentalis Freeman] and tree mortality due to Douglas-fir bark beetle [Dendroctonus pseudotsugae Hopkins] (Leclerc et al., 2021). Documentary fire records from 1917 to present include two fires in the Alex Fraser Research Forest, although neither burned within the boundaries of our study area (Figure 1A). A 64-ha fire was recorded in 1938 and a 6-ha fire was suppressed in September 2013 (BC Wildfire Service, 2018).
Research Design and Field Sampling
We reconstructed fire history and forest dynamics for a subset of 35 permanent sample plots near Knife Creek in the Alex Fraser Research Forest. In 1984, 80 permanent sample plots were established along a systematic, 100 × 100 m grid that covers 161 ha (Armleder and Thomson, 1984; Koot et al., 2015). Half the plots were in forests that were partially harvested to enhance mule deer winter habitat; the other half were untreated controls. We sampled 35 plots, including 17 treated and 18 control plots (Figure 1B; Brookes, 2019).
We searched a 1-ha circular plot (radius = 56.4 m) surrounding each plot center for living trees, snags, logs, or stumps with external fire scars (hereafter “fire-scarred trees”) (Brookes, 2019). To reduce impacts on living trees and to avoid potential bias due to partial harvesting in 1984, we preferentially sampled snags, logs and stumps. Up to 5 partial or full cross-sections were sampled per plot (Cochrane and Daniels, 2008) by selecting fire-scarred trees with the most and best-preserved visible scars (Swetnam and Baisan, 1996).
To quantify forest composition and structure, we sampled the species and diameter at breast-height (dbh) of the 10 live canopy (emergent, dominant, and codominant crown classes) and 10 live subcanopy (intermediate and suppressed crown classes) trees (dbh ≥ 12.5 cm) closest to each plot center (Jonsson et al., 1992). The distance from the center of the plot to the 10th tree in each canopy class formed the radius of a circular plot used for calculating tree densities (Jonsson et al., 1992). To assess tree ages and growth histories, an increment core was sampled c. 20–30 cm from the base of each tree and coring height was recorded. As needed, multiple cores were extracted to ensure each sample intersected or was close to the pith.
Reconstructing Fire History and Forest Dynamics
Following standard dendrochronological methods (Stokes and Smiley, 1996), fire-scarred sections and increment cores were air-dried, mounted on wooden supports, and sanded so that individual cells and ring boundaries were clearly visible. Using high-resolution digital images (2400–4800 dpi), the ring widths of each sample were measured using the program CooRecorder (Larsson, 2011a) and crossdated against an existing regional Douglas-fir chronology (Daniels, 2004) using the programs CDendro (Larsson, 2011b) and COFECHA (Holmes, 1986). By crossdating, accurate calendar years were assigned to the inner- and outer-rings of samples from living and dead trees, as well as the fire scars (Brookes, 2019). Fire years were determined from the position of each scar tip within annual rings of the scarred cross-sections (Brown and Swetnam, 1994). For fire scars located at the boundary between two rings, we assigned the calendar year of the completed ring, consistent with modern fires in the study area that typically burn mid- to late-summer (Heyerdahl et al., 2012; BC Wildfire Service unpublished data). In subsequent analyses, only fire years recorded by ≥ 2 trees to avoid scars possibly caused by disturbance agents other than fire (Swetnam and Baisan, 1996).
Plot-level age structures were derived using the canopy and subcanopy tree ages (Brookes, 2019). To estimate the year in which each tree established, corrections were applied to the crossdated inner-ring dates (Daniels et al., 2017). For all trees, we used an age-on-height regression developed from local Douglas-fir saplings to estimate the number of years for trees to grow to coring height (Daniels, 2004). For the subset of cores that did not intercept the pith, we accounted for missed rings based on ring geometry if the core had arced rings close to the pith (Duncan, 1989). For cores that did not include arced rings but was ≥ 90% of the geometric radius, the length of the missing radius and average ring width were used to estimate the number of missing rings (Norton et al., 1987). Eighty-eight percent of age corrections were ≤ 15 years; thus, plot-level age structures were derived using 15-year classes.
Even-aged cohorts provide evidence for moderate- to high-severity fires (Harvey et al., 2017). In our study area, Douglas-fir establishment can be prolonged because seedlings are susceptible to growing season frosts and require some protection (Klinka et al., 2000). At the plot level, an even-aged cohort was identified when ≥ 5 trees established within 15-years, preceded by ≥ 15 years with no tree establishment (after Heyerdahl et al., 2012). We tested 10- and 20-year windows but found little difference in the number of cohorts detected. To identify cohorts, we counted the number of trees that established in 15-year periods beginning with the pith date of the oldest tree in the plot and then shifted in 1-year increments until we reached the pith date of the youngest tree (Chavardès and Daniels, 2016). The year assigned to each cohort was the establishment year of the oldest tree of the cohort. Cohorts that established within 15 years of a fire year in the same plot or an adjacent plot were considered post-fire cohorts (Heyerdahl et al., 2012).
Since periods of suitable climate can facilitate tree establishment independently of disturbance, we assessed the possibility that cohorts were a consequence of favorable climate rather than fire (Heyerdahl et al., 2012). Using an independent tree-ring reconstruction of June to August precipitation in Williams Lake from 1633 to 1996 (Daniels and Watson, 2003; Daniels, 2004), we calculated 15-year moving averages reported for the first year of each 15-year window (e.g., 1901 = average values from 1901 to 1915) and compared the averaged values against the long-term mean. Positive departures indicated dry periods likely to limit establishment because a lack of summer moisture has been shown to delay seedling growth (Hermann and Lavender, 1990). Each cohort was classified as establishing during a wet or dry period. Contingency tables of the number of cohorts that established during wet versus dry periods were assessed against a random distribution using chi-squared goodness of fit (α = 0.05) (Whitlock and Schluter, 2009).
Determining Historical Fire Frequency and Severity
To quantify fire frequency, we developed plot-level fire chronologies using fire-scars and post-fire cohorts (Brookes, 2019). For each plot, the length of the fire record, number of fires, the minimum, maximum, and mean number of years between fires, and the time since last fire were calculated. The length of the fire record at each plot was determined from the age of the oldest tree. The time since last fire was the number of years between 2015 (the year of sampling) and the last fire year indicated by a scar or a post-fire cohort. The maximum fire interval from each plot was used to determine whether the current fire-free interval exceeded the historical range of variation. A visual representation of fire scar and cohort occurrence for each plot was constructed using the Fire History Analysis and Exploration System (FHAES) (Brewer et al., 2016). To discern whether reconstructed fire frequency differed between the mule deer control and treatment units at Knife Creek, we composited the plot-level fire records for the control and treatment units and used a Welch’s 2-tail t-test to compare the mean intervals between fires. They did not differ significantly (p = 0.50, α = 0.05, df = 31), therefore we composited fire records from all plots and calculated the study-area fire frequency as the mean interval between fire years. We used breakpoint analysis to test for structural changes in the cumulative number of fires from 1600 to 2015. We used ordinary least squares-based CUSUM tests as an explorative tool, Bayesian information criterion to determine the optimal number of breakpoints, and built a segmented regression model following Zeileis et al. (2003) and Zeileis (2005). Breakpoint analyses were conducted in R (version 4.0.5) statistical software (R Core Team, 2021) using the package strucchange (Zeileis et al., 2002).
The severity of fires at individual plots through time was inferred using criteria from Heyerdahl et al. (2012). We classified plots with only 1 fire year associated with a post-fire cohort and no fire scars as those recording high-severity fire through time. Mixed-severity fire histories were assigned to plots with > 1 fire year and ≥ 1 post-fire cohorts. Plots with low-severity histories were plots with > 1 fire scar years but no post-fire cohorts. For plots not meeting these criteria, severity was unclassifiable.
Trees that survived ≥ 1 fire indicated by a fire scar or post-fire cohort were classified as remnant trees (Chavardès and Daniels, 2016). The severity of the most recent fire at each plot was calculated as the percentage of remnant trees surviving that fire relative to all trees in the plot (Chavardès and Daniels, 2016). Plots with 81−100% remnant trees indicated low-severity, 20−80% indicated moderate-severity, and < 20% indicated high-severity fire (after Sherriff and Veblen, 2006).
Widespread fires were defined as those that scarred trees in ≥ 10 plots and ≥ 25% of plots with living trees present in the fire year. We used the distribution of fire scars, post-fire cohorts, and remnant trees to assess spatial variation in severity within individual widespread fires (Brookes, 2019). For each fire, the presence or absence of fire scars, post-fire cohorts, remnant trees, and potential recording trees were combined to estimate severity at the plot level. Similar to the classification of fire-severity through time, plots with fire scars but no post-fire cohort indicated local low-intensity surface fire that scarred trees but did not initiate a post-fire cohort. Plots with fire scars and a corresponding post-fire cohort indicated fire that burned at moderate-to-high intensity, sufficient to provide a suitable environment for tree establishment although some trees survived. Plots with only a post-fire cohort burned with sufficiently high intensity to initiate a new cohort, leave no local fire scars, and destroy evidence of previous fires. Lastly, plots with remnant trees that did not scar or form cohorts indicated the plot did not burn or burned with an intensity too low to scar trees. The latter evidence was strongest at plots with existing scarred trees at the time of fire, because trees that have previously scarred are more likely to scar during subsequent fires.
Results
Forest Composition and Structure
All plots comprised Douglas-fir only, except one that included trembling aspen (Table 1). Canopy-dominant trees were up to 149 cm in diameter and 457 years of age. In the control plots, densities were 182 ± 84 (mean ± standard deviation) trees ha–1 in the canopy and subcanopy densities were ≤ 581 trees ha–1, except one plot with 3,537 trees ha–1. Densities were lower in the treated area, averaging 152 ± 85 trees ha–1 in the canopy and 224 ± 299 trees ha–1 in the subcanopy due to partial harvesting in 1984 (Leclerc et al., 2021).
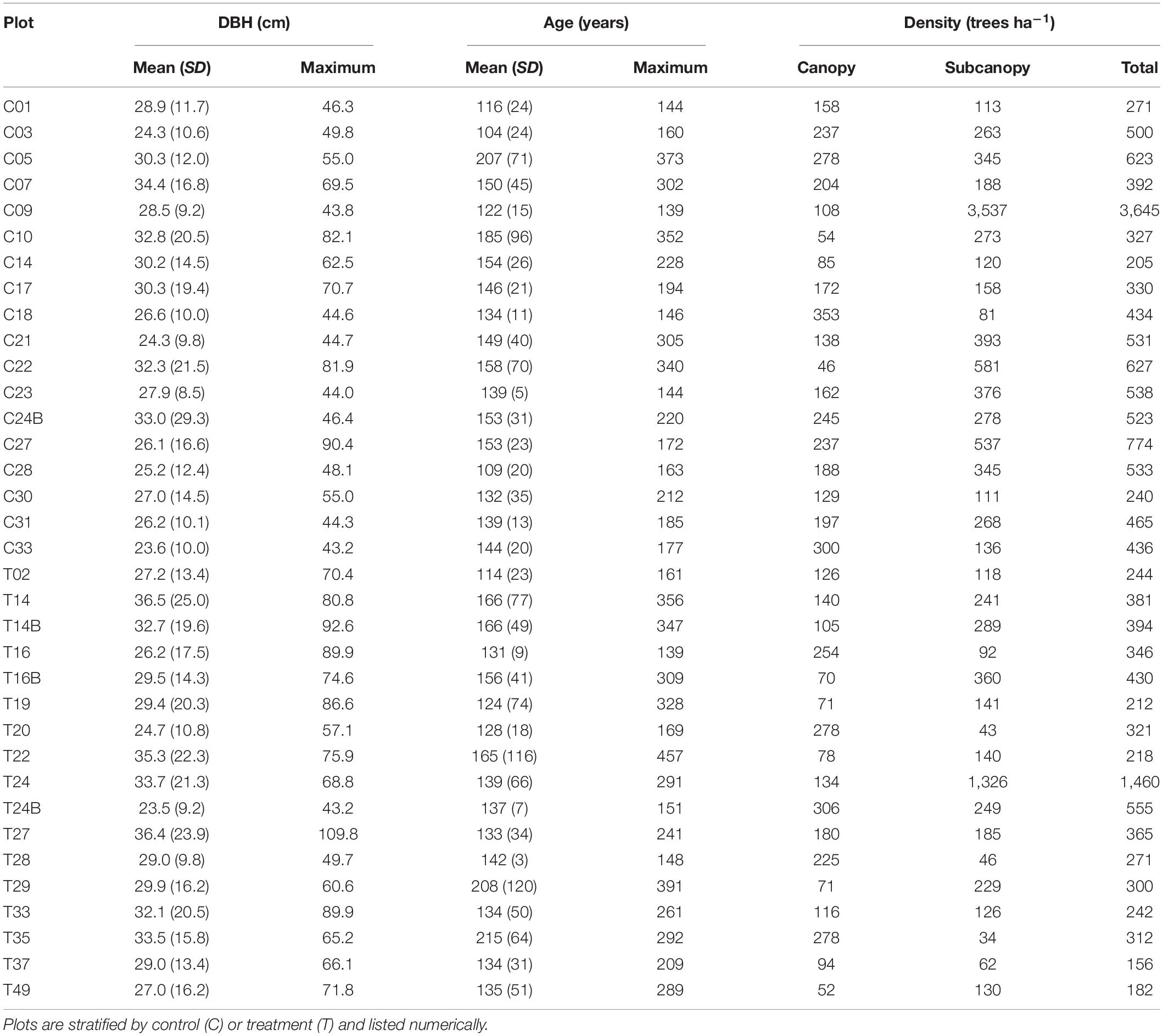
Table 1. Summary of forest attributes in 35 plots near Knife Creek in the Alex Fraser Research Forest, British Columbia.
Fire Occurrence and Frequency
Twenty-nine of 35 plots contained fire-scarred trees (Figure 2 and Table 2). Seventy-nine of 82 (96%) sections sampled from 67 fire-scarred trees were successfully crossdated. Of 305 fire scars, 282 scars formed in years when ≥ 2 trees were scarred across the study area and were included in subsequent analyses. Plot-level fire chronologies ranged from 150 to 555 years and included 1-10 fire years. At the 26 plots with ≥ 2 fires, mean fire intervals were 17-110 years and < 40 years in 73% of plots. Current fire-free intervals of 72–184 years exceeded their corresponding historical maximum fire intervals (23−110 years) at all but one plot.
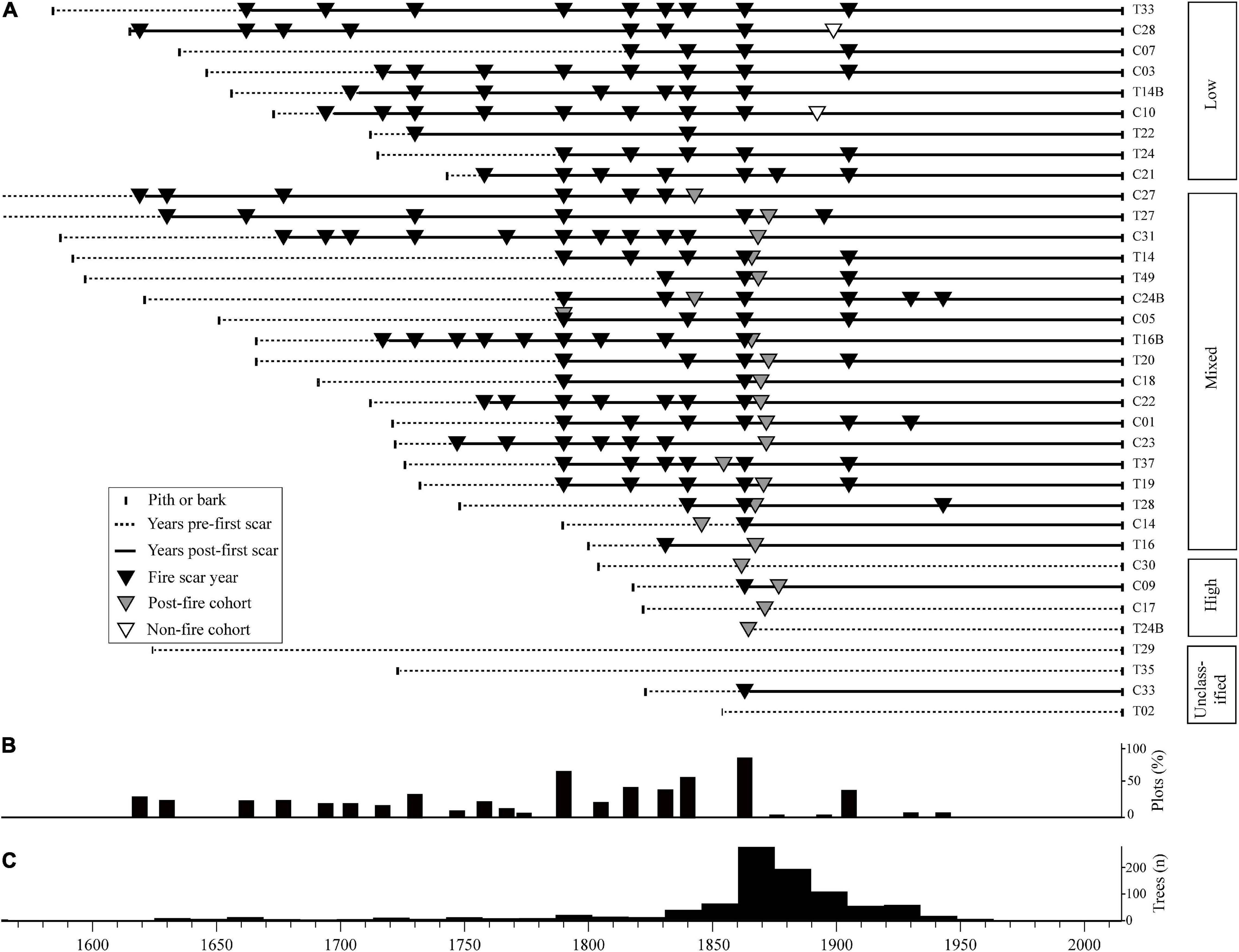
Figure 2. Fire record from 1600 to 2015 for 35 plots in the Alex Fraser Research Forest. (A) Horizontal lines represent individual plots, stratified by fire history through time and record length (top to bottom). Dashed segments of lines indicate years prior to fire-scar formation on ≥ 1 sampled trees; solid lines indicate presence of ≥1 scarred trees. Black triangles represent fire years, gray/white triangles represent the first year of each post-fire/non-fire cohort. (B) Percentage of plots recording fire scars calculated as the number of plots recording fire relative to the number of plots with trees present that could record fire. (C) Age-structure histogram (15-year bins) for all plots combined.
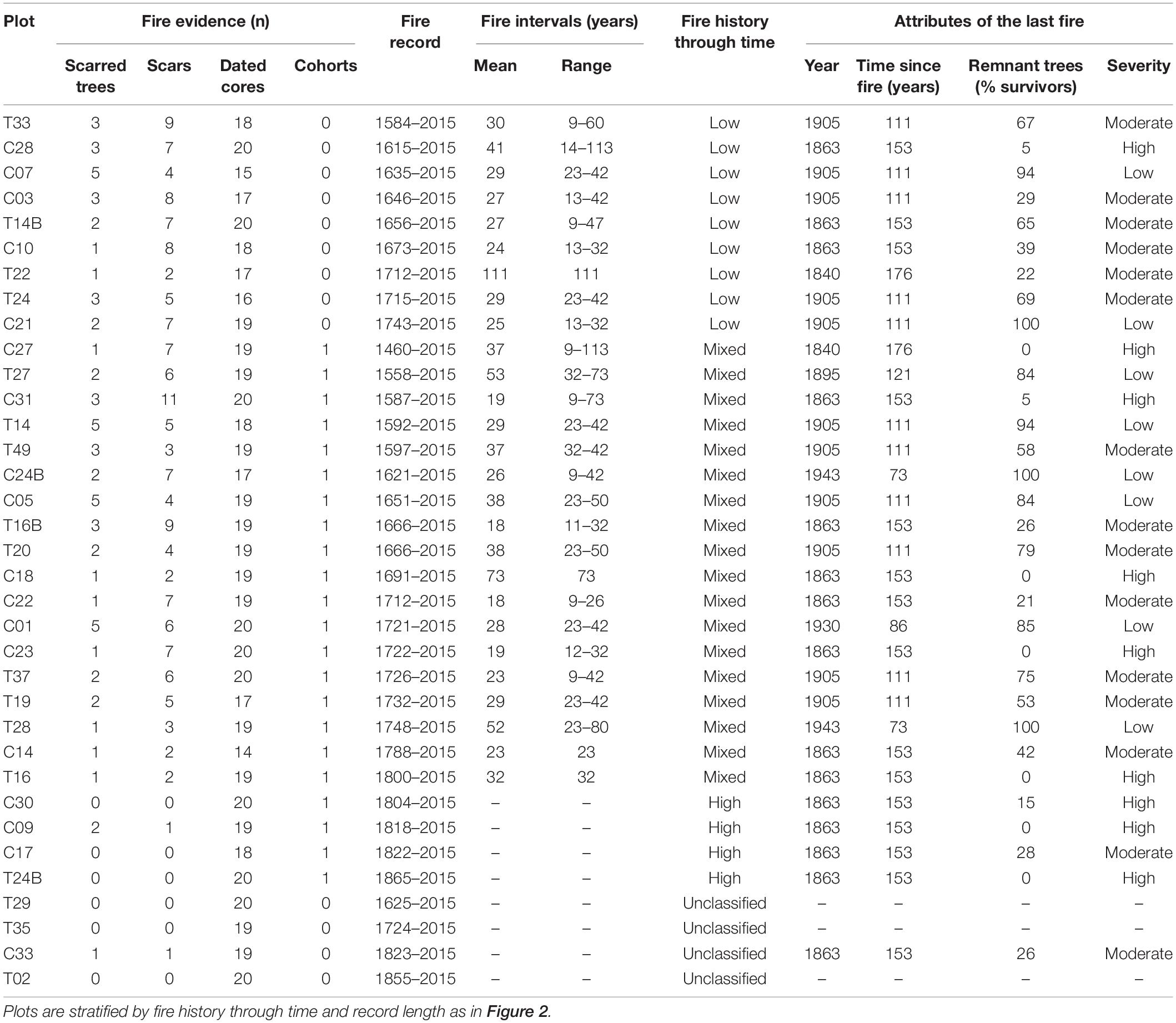
Table 2. Fire records for 35 plots near Knife Creek in the Alex Fraser Research Forest, British Columbia.
The composite fire record for the study area indicated 23 years from 1619 to 1943 when ≥ 2 trees were scarred, with intervals of 7−32 years (Figure 2). We detected two significant structural changes in the cumulative number of fires over time, with breakpoints in 1757 (95% CI: 1756, 1760) and 1942 (95% CI could not be computed) (Table 3). The cumulative number of fires increased from 1600 to 1757 at a rate of 0.05 fires per year. The rate of fire accumulation increased significantly to 0.07 fires per year from 1758 to 1942, but stagnated after 1943 when no fires burned. Six widespread fires burned in 1790, 1817, 1831, 1840, 1863, and 1905 (Table 4), during the period of rapid fire accumulation. Evidence of the 1863 fire was most widespread and persistent, including scars on 41% of sampled trees in 86% of plots.
Tree Establishment and Cohorts
We successfully estimated the ages of 656 of 700 (94%) trees that we cored; 44 trees missing inner rings due to substantive heartwood decay or with correlation coefficients < 0.25 relative to the regional chronology were omitted from subsequent analyses (Table 2). Trees established from 1559 to 1962, with 76% establishing after the widespread 1863 fire (Figure 3 and Table 1). Only 2% of trees recruited into the canopy since the last fire in 1943. Among the 35 plots, 24 cohorts established from 1844 to 1899, 22 of which were classified as post-fire cohorts and 9 included the oldest trees in their plots. Cohort establishment was significantly associated with dry, rather than wet, periods (p = 0.07, α = 0.05, df = 1), corroborating the inference that most cohorts established following fires.
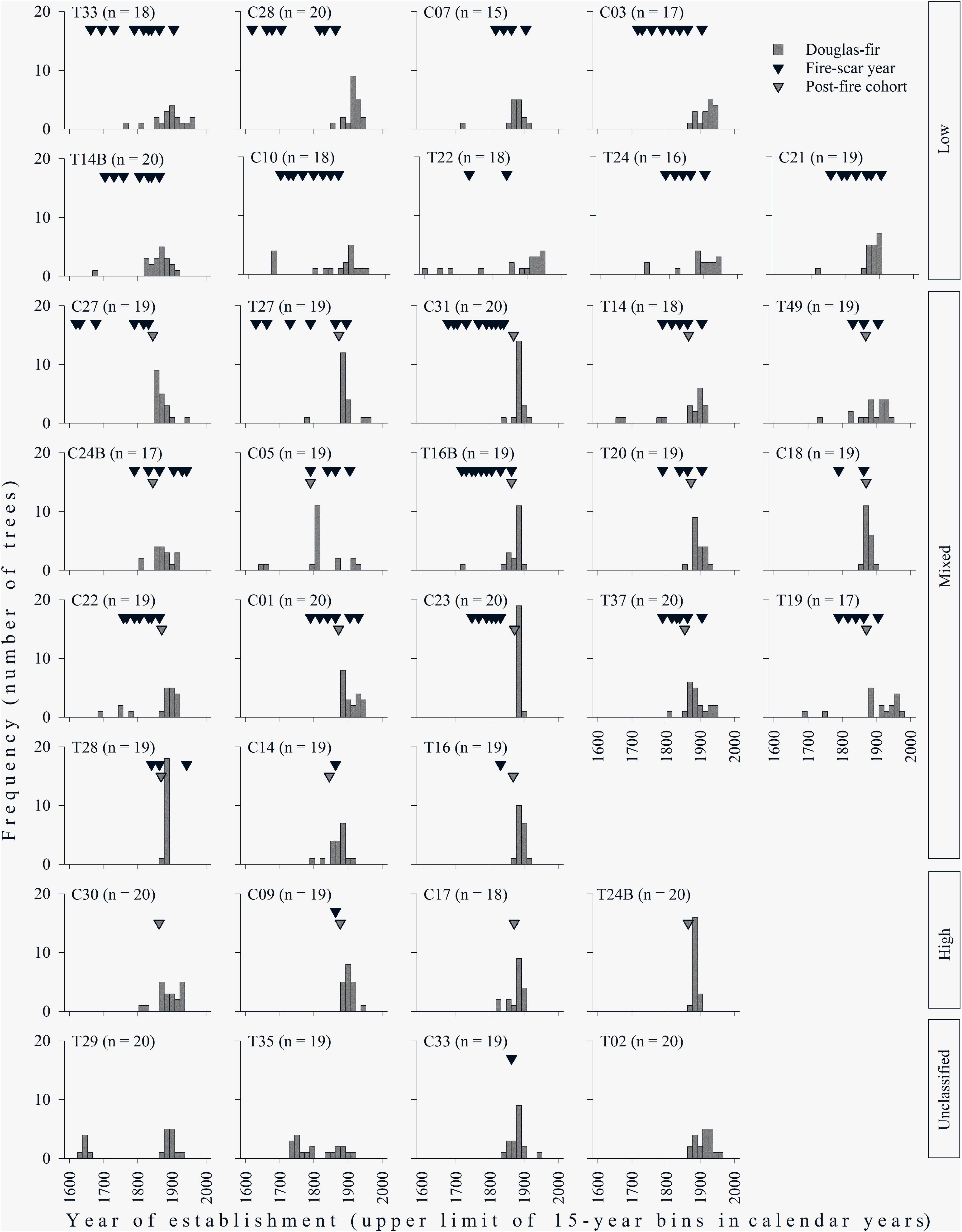
Figure 3. Plot-level fire history reconstructed from fire scars and post-fire cohorts. Plot numbers are followed by the number of trees in the age histogram in parentheses. T denotes plots in treated areas that were partially harvested in 1984; C denotes unharvested control plots. Plots are stratified by fire history through time (low, mixed, high and unclassified, from top to bottom).
Fire Severity Through Time
Fire scars and post-fire cohorts varied among plots and indicated fires burned at a range of severities through time. Eighteen plots (54%) were classified as having mixed-severity fires through time, 4 (11%) as high severity, 9 (26%) as low severity, and 4 (11%) were unclassifiable (Table 2). Eleven mixed-severity plots recorded ≥ 5 fire-scar years and a single post-fire cohort that established after the 1840 (n = 3) and 1863 (n = 8) fires (Table 2). The plots with low-severity fires through time recorded 2−9 fires. The most recent fire at 8 plots was classified as low-severity, 15 were moderate- and 6 were high-severity, based on the percentage of remnant trees. Four plots with neither a fire-scar or post-fire cohort could not be classified. At the study area scale, both plot-level fire histories through time and severity of the most recent fire indicate a mixed-severity fire regime.
Variability in Historical Widespread Fires
The spatial distribution of fire scars, combined with post-fire cohorts, revealed variation in fire severity within and among the 6 widespread fires (Figure 4 and Table 4). As well, plots lacking fire evidence were adjacent to and between plots with scars or cohorts, providing indirect evidence of spatial variation in severity. The 1790 fire scarred trees in 19 plots distributed across the study area and a post-fire cohort established in one plot. The 1817 fire scarred trees in 13 plots, 12 of which were in the north and west of the study area. The 1840 fire scarred trees in 16 plots, one of which included a post-fire cohort. Cohorts established in 3 other plots. Plots with cohorts were in the north and east of the study area. This fire was the last recorded in 2 plots; severity was classified as moderate in 1 plot and high in the other. Scars and cohorts in 30 plots throughout the study area provide evidence that the 1863 fire was relatively severe and widespread. Trees were scarred in 24 plots, 11 of which included post-fire cohorts. Cohorts established in another 6 plots. This fire was the last recorded in 15 plots; severity was classified as moderate in 7 plots and high in 8 plots. In contrast with 1863, the 1905 fire was relatively low in severity. Trees were scarred in 13 plots, but no post-fire cohorts established. All but one plot with scars were in the north and west of the study area, where young trees had established after the 1840 and 1863 fires. The 1905 fire was the last recorded in 11 plots; severity was classified as low in 4 plots and moderate in 7 plots.
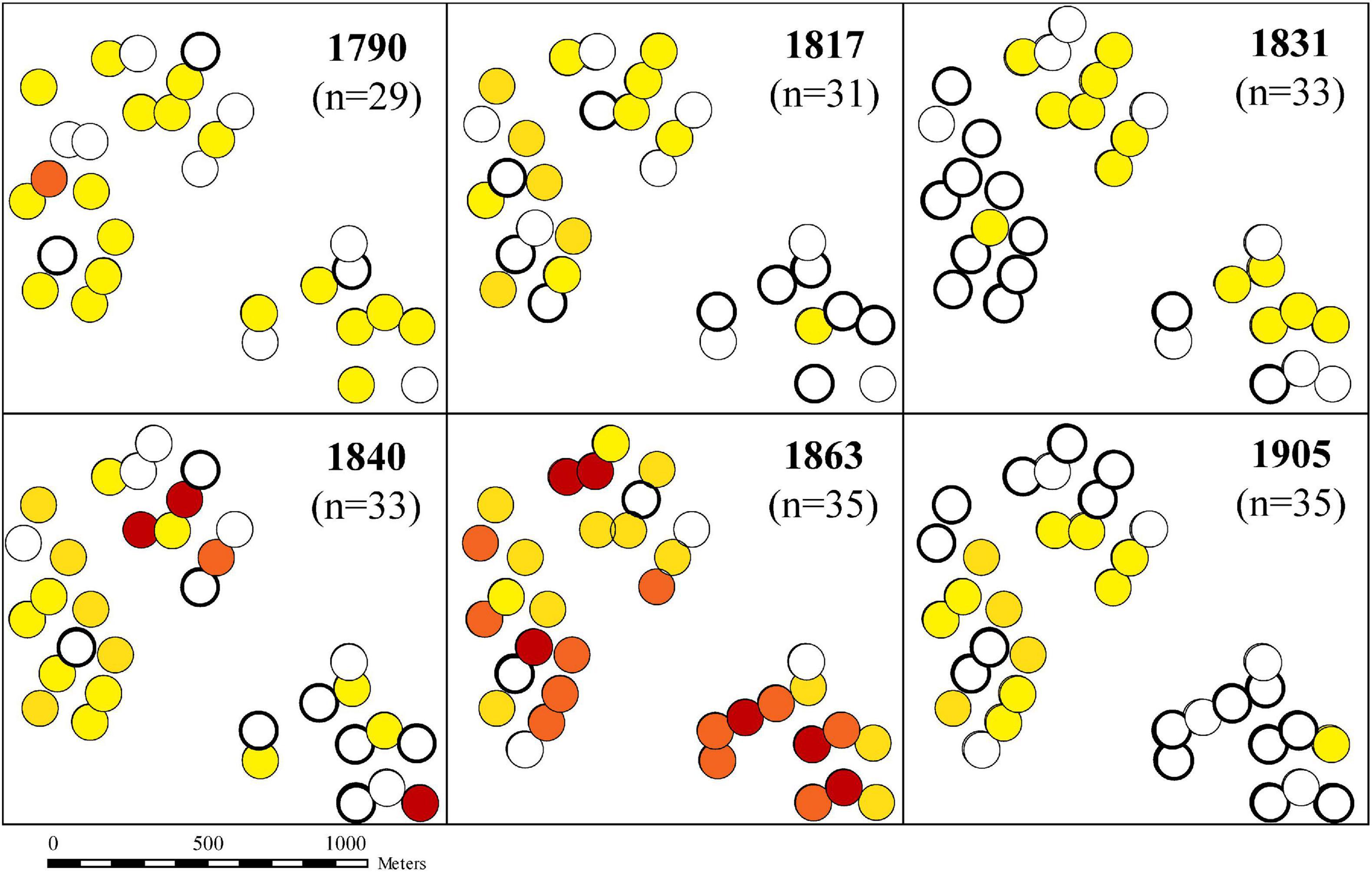
Figure 4. Spatial pattern of fire severity for six widespread fires. Only plots containing trees old enough to record each fire are shown (n). Colors of one-hectare circular plots indicate severity: yellow plots include fire scars only indicating low severity; orange plots include fire scars and post-fire cohorts indicating moderate severity; and, red plots include post-fire cohorts only indicating high severity. White indicate plots with no evidence of fire; bold boundaries indicate plots with trees that had previously been scarred and were susceptible to fire damage.
Discussion
Historical Mixed-Severity Fire Regime
In stark contrast with the predominantly severe, stand-initiating megafires of 2017 that burned 33,181 ha of Douglas-fir forests surrounding our study area (Figure 1A; Abbott and Chapman, 2018; BC Wildfire Service, 2018), low- and moderate-severity fires frequently burned, dominating the historical mixed-severity fire regime and driving forest dynamics in the Douglas-fir forests near Knife Creek (Table 2). The composite fire-scar record indicated surface fires burned every 10–30 years prior to the 1940s, comparable to other Douglas-fir-dominated forests in warm and dry climates of interior British Columbia (Figure 2; Daniels, 2004; Heyerdahl et al., 2007, 2012; Marcoux et al., 2013; Greene and Daniels, 2017; Harvey et al., 2017) and, more broadly, across the western United States (Heyerdahl et al., 2008; Perry et al., 2011; Hessburg et al., 2019).
Our fine-scale spatio-temporal reconstruction of historical fires detected no evidence of a stand-initiating fire across the 161-ha study area over the 396-year reconstruction (Figures 2, 3). Fire scars, cohorts, and remnant trees indicated the six widespread fires from 1790 to 1905 included substantive internal heterogeneity at scales of 10–100s of meters (Figure 4). Individuals and patches of trees survived even the two most widespread fires in 1840 and 1863, while cohorts initiated following fires in 1790, 1840, and 1863 (Table 4). Cohort establishment suggests that some patches of high-intensity fire were sufficient to cause overstory tree mortality and improve levels of light and nutrients available to survivors and seedlings (Harvey et al., 2017). Nevertheless, these fine-scale patches were embedded in an uneven-aged forest matrix maintained by frequent low- or moderate-severity fires, a characteristic noted in other dry forests in south central BC (Daniels, 2004; Heyerdahl et al., 2007; Harvey et al., 2017). Given the small size of our study area, replicating our research across a network of sites at landscape-to-regional scales will improve understanding of the frequency and relative importance of fires of a range of severities.
Inferring Fire Severity From Scars and Cohorts
The presence and location of fire-scarred trees and post-fire cohorts are used to infer the timing, spatial patterns, and severity of past fires (Swetnam et al., 1999; Daniels et al., 2017). The absence of scars and cohorts can be equally informative, but yield multiple possible interpretations: (i) fire did not burn, (ii) fire burned at low intensity leaving no scars or cohorts, or (iii) fire burned at sufficient intensity to leave scars or cohorts but the evidence no longer persists (Falk et al., 2007; Yocom Kent, 2014). The latter interpretations are confounded by diminishing fire evidence due to subsequent disturbances, tree mortality, and wood decay through time, a widely recognized limitation of dendroecological reconstructions (Swetnam et al., 1999; Falk et al., 2011; Daniels et al., 2017). Below, we discuss how the presence, absence, and assumptions underlying fire evidence yield alternate interpretations of severity of the two widespread fires in 1790 and 1863.
The 1790 fire illustrates the challenges and limitations of inferring the severity of fires in the distant past (Figure 4), although it burned during the period of rapid fire accumulation, when ≥ 77% of plots included recorder trees (Table 3). Scars in 19 plots but a post-fire cohort in only one plot suggest a low-severity fire (e.g., interpretations i and ii, above). Alternately, had the 1790 fire burned at higher intensity and generated post-fire cohorts in multiple plots, subsequent fires eliminated that evidence from contemporary age structures (e.g., interpretation iii, above). Over the 50 years from 1790 to 1840, five fires burned at short intervals of 9-15 years and 66% of plots that burned in 1790 re-burned up to four times. Trees that established after 1790 would have been young, small, and lacking thick bark to resist subsequent fires. In fact, the single persistent post-1790 cohort was in the only plot that remained fire-free until 1840. Thus, it is plausible that other post-1790 cohorts established but were consumed by subsequent fires. Conversely, such cohorts may have persisted without repeat fires from 1790 to 1840.
Deciphering the relative severity of the widespread 1863 fire was key for understanding the contemporary forest age structure and concluding the historical fire regime has been disrupted. Many studies consider fires in the late 1800s relatively severe because they generated new cohorts of trees, and thus, emphasize the importance of high-severity fires in a mixed-severity fire regime (Daniels et al., 2017). We classified the 1863 as moderate-to-high severity since the resulting post-fire cohorts account for >70% of contemporary canopy and subcanopy trees (Sherriff and Veblen, 2006; Chavardès and Daniels, 2016). However, emphasizing the origin versus persistence of those cohorts yields contrasting interpretations of the contemporary fire regime. A focus on cohort origin supports an interpretation that the 1863 fire was a periodic high-severity event in a mixed-severity regime that has not been disrupted (Odion et al., 2014). This interpretation inherently assumes a high rate of fire-caused tree mortality predicated abundant post-fire tree establishment, justifying the classification of high severity. Yet, the survival of remnant trees in 29 of 35 plots is inconsistent with this assumption. Alternately, a focus on cohort persistence supports an interpretation that tree survival rates have been exceptionally high due to subsequent reductions in fire occurrence, size, and severity (Guiterman et al., 2018). In the 70 years preceding 1863, five widespread fires reburned plots multiple times (Figures 2, 4 and Table 4). In contrast, in the 150 years since 1863, small fires in 1876, 1895, 1930, and 1943 scarred trees in only one or two plots and 15 plots with post-1863 cohorts have not reburned. The last widespread fire in 1905 was low in severity, only scarring trees without initiating new cohorts. Excessive fire-free intervals of 72–184 years indicate individual plots have missed up to six fires relative to historical frequencies (Tables 2, 3). Absent surface fires to drive tree mortality and maintain low forest densities, we conclude the persistence of the post-1863 cohorts both result from and strongly indicate disruption of the historical fire regime during the twentieth century.
Human Disruption of the Fire Regime
The absence of fire scars since 1943 or establishment of new post-fire cohorts in the twentieth century provide strong evidence of a disrupted fire regime at Knife Creek (Figure 2). All plots included young, small subcanopy trees and 19 plots included live, fire-scarred trees that would have been highly susceptible to scarring had fires burned (Figure 3). The lack of fire is consistent with other dendroecological reconstructions, a period of cooler and wetter regional climate from 1946 to 1976 (Daniels, 2004; Heyerdahl et al., 2012; Harvey et al., 2017), and historical land use in the region (Parminter, 1991; Day, 1998). Specifically, the arrival of Europeans to this area following the Gold Rush in the 1860s severely restricted Indigenous fire stewardship (Parminter, 1991; Day, 1998). This part of the T’exelcmec traditional territory includes sacred ceremonial grounds and a well-established travel route between seasonal camps along the San Jose River (oral history shared with and reported by Day, 2007), which lies immediately west of our study area (Figure 1A). Travel corridors are generally known to have a high prevalence of Indigenous fire (Lake and Christianson, 2019). Therefore, the historical fire record reconstructed in this study likely reflects Indigenous fire stewardship, including the practice of intentionally setting fires to modify fire regimes and increase resource availability (Turner, 1999; Turner et al., 2000; Lake and Christianson, 2019). Indigenous fire stewardship in dry forest ecosystems is well documented in oral histories in BC (Simmons, 2012; Lewis et al., 2018; Xwisten Nation et al., 2018), and fire was likely used to manage forests for food and medicinal plants and for hunting animals at or near Knife Creek.
During the Gold Rush and continuing through to the 1940s, the region surrounding our study area was rapidly colonized by Europeans as ranches and road houses were established along the Cariboo Wagon Road (currently Highway 97, the main north-south highway in BC), which is adjacent to the Knife Creek study area (Figure 1A; Day, 1998). Controlled burning continued to be used by ranchers to promote the expansion of meadows and grasslands to provide feed for livestock (Parminter, 1991; Day, 1998). In 1874, the Bush Fire Act introduced fines or imprisonment if a purposely set fire resulted in damage to private or crown land and the Forest Act of 1912 provided financial support to equip and maintain a fire prevention force (Parminter, 1991). Since the 1940s, firefighting technology improved substantially, and both fire-scar and documentary records indicate the majority of fires have been successfully suppressed (Daniels, 2004; BC Wildfire Service unpublished data).
Tree-ring evidence, consistent with oral histories and documentary records, indicates changes in human land-use and fire suppression have disrupted the fire regime at Knife Creek relative to the historical range of variation. As observed in other dry mixed-conifer forests, contemporary fire regimes have led to increased tree densities, especially in subcanopy strata, that have made dry forests less resilient to fire (Hessburg et al., 2019). There is compelling evidence that in the absence of frequent fires, a substantial degree of forest structural diversity in Knife Creek has been lost (Hessburg et al., 2005, 2016; Spies et al., 2006; Harvey et al., 2017). Stands near Knife Creek that have not been harvested have closed canopies and forest densities generally range from 200 to 775 trees ha–1, with the densest stands exceeding 3400 trees ha–1 (Table 1). Such dense canopies leave Knife Creek at increased risk of a high-severity stand-initiating fire (Leclerc et al., 2021) and justify treatments to mitigate hazardous fuels and restore ecosystem structure and function (Hessburg et al., 2005, 2016; Stephens et al., 2012; Halofsky et al., 2020).
Implications for Forest Restoration and Management
Our reconstruction of the historical fire regime provides a framework for future management and restoration of the Knife Creek forest and other Douglas-fir forests in the surrounding landscape. Since surface fires have been essentially eliminated from the contemporary fire regime, priority actions should aim to reduce stand densities, increase stand-level heterogeneity, and diversify forest structures across the landscape (Halofsky et al., 2011; Stephens et al., 2012, 2020; Churchill et al., 2013; Hessburg et al., 2016, 2019). Uneven-aged silviculture, thinning, and prescribed burning (Stephens et al., 2012), including the re-introduction of Indigenous fire stewardship (Lake and Christianson, 2019; Long et al., 2020), can be used in combination to achieve these goals. Specifically, thinning the subcanopy emulates the common, widespread effects of low-severity fires and selected removal of canopy trees emulates the patchy effects of moderate-to-high-severity that we reconstructed. Removing up to 76% of the relative density of the unharvested forests of Knife Creek would yield tree densities of 50–190 trees ha–1, congruent with historical densities (Hessburg et al., 2005; Leclerc et al., 2021). Recurring maintenance treatments at intervals of 10-30 years that vary in size and within-treatment severity at scales of 1-100 ha will maintain forest structures consistent with the historical mixed-severity fire regime. Long-term management that emulates or includes frequent surface fires will maintain low levels of flammable fuels and an open canopy structure, reducing crown fire potential and increasing forest resilience against future megafires.
Data Availability Statement
The raw data supporting the conclusions of this article will be made available by the authors, without undue reservation.
Author Contributions
WB organized the data collection and analyzed the data with guidance from LDD and ALC. WB and LDD led the writing of the manuscript. All authors contributed to discussing and revising the manuscript.
Funding
This research was funded by Natural Sciences and Engineering Research Council of Canada (NSERC) Discovery Grants to LDD (RGPIN-2020-06310) and ALC (RGPIN-2015-04376), and a grant from an anonymous foundation.
Conflict of Interest
The authors declare that the research was conducted in the absence of any commercial or financial relationships that could be construed as a potential conflict of interest.
Acknowledgments
This manuscript includes excerpts from the Master of Science thesis by WB, completed at the University of British Columbia, Vancouver, BC, Canada. We respectfully acknowledge that the land on which we conducted our research is the unceded ancestral territory of the T’exelcemc (Williams Lake Band) of the Secwepemc (Shuswap) Nation. We thank the staff at the Alex Fraser Research Forest, K. Day, C. Koot, and S. Ewan, for facilitating this research; T. Dergousoff, A. Kaufman, M-A. Leclerc, M-E. Leclerc, A. Weixelman, E. Xu, and R. Chavardès for assistance in the field and lab; G. Preston for assistance with the maps; and B. Eskelson, J. Rhemtulla, and two reviewers for helpful comments that improved the manuscript.
References
Abbott, G., and Chapman, M. (2018). Addressing the New Normal: 21st Century Disaster Management in British Columbia. Available online at: https://www2.gov.bc.ca/assets/gov/public-safety-and-emergency-services/emergency-preparedness-response-recovery/embc/bc-flood-and-wildfire-review-addressing-the-new-normal-21st-century-disaster-management-in-bc-web.pdf (accessed May 1, 2018).
Agee, J. K., and Skinner, C. N. (2005). Basic principles of forest fuel reduction treatments. Forest. Ecol. Manag. 211, 83–96. doi: 10.1016/j.foreco.2005.01.034
Armleder, H. M., and Thomson, R. (1984). Experiment Harvesting of Dry-belt Douglas-fir on a Mule Deer Winter Range at Knife Creek: Working Plan and Progress Report. Victoria, B.C: British Columbia Ministry of Forests Research Branch Report WHR-17.
Baker, W. L. (2015). Are high-severity fires burning at much higher rates recently than historically in dry-forest landscapes of the western USA? PLoS One 10:e0136147. doi: 10.1371/journal.pone.0136147
Baker, W. L. (2017). Restoring and managing low-severity fire in dry-forest landscapes of the western USA. PLoS One 12:e0172288. doi: 10.1371/journal.pone.0172288
BC Government (2010). Wildland Fire Management Strategy. Available online at: http://www2.gov.bc.ca/assets/gov/farming-natural-resources-and-industry/forestry/wildfiremanagement/governance/bcws_wildland_fire_mngmt_strategy.pdf [accessed August 28, 2017]
BC Government (2018). Wildfire averages. Available online at: https://www2.gov.bc.ca/gov/content/safety/wildfire-status/about-bcws/wildfire-statistics/wildfire-averages (accessed November 22, 2018).
BC Ministries of Environment and Forests (1995). Biodiversity Guidebook – Forest Practices Code of British Columbia. Available online at: https://www.for.gov.bc.ca/hfd/library/documents/bib19715.pdf (accessed May 20, 2021)
BC Wildfire Management Branch. (2012). Wildfire Management Branch Strategic Plan 2012-2017. Available online at: https://www2.gov.bc.ca/assets/gov/public-safety-and-emergency-services/wildfire-status/governance/bcws_strategic_plan_2012_17.pdf (accessed November 10, 2016).
BC Wildfire Service (2018). Historical fire perimeters (1917 to 2017). British Columbia Open Data Catalogue (updated April 2018). Available online at: https://catalogue.data.gov.bc.ca/dataset/bc-wildfire-fire-centres (Accessed May 15, 2021).
Bowman, D. M. J. S., Balch, J. K., Artaxo, P., Bond, W. J., Carlson, J. M., Cochrane, M. A., et al. (2009). Fire in the earth system. Science 324, 481–484. doi: 10.1126/science.1163886
Brewer, P. W., Velasquez, M. E., Sutherland, E. K., and Falk, D. A. (2016). Fire History Analysis and Exploration System (FHAES) version 2.0.2. Available online at: http://www.fhaes.org (accessed June 1, 2017)
Brookes, W. (2019). Historical and Contemporary Disturbance Regimes in Central Interior Dry Forests of British Columbia. Master’s thesis. Vancouver, BC: University of British Columbia.
Brown, P. M., and Swetnam, T. W. (1994). A cross-dated fire history from coast redwood near Redwood National Park, California. Can. J. Forest. Res. 24, 21-31. doi: 10.1139/x94-004
Chavardès, R., and Daniels, L. D. (2016). Altered mixed-severity fire regime has homogenized montane forests of Jasper National Park. Int. J. Wildland Fire 25, 433-444. doi: 10.1071/WF15048
Churchill, D. J., Larson, A. J., Dahlgreen, M. C., Franklin, J. F., Hessburg, P. F., and Lutz, J. A. (2013). Restoring forest resilience: from reference spatial patterns to silvicultural prescriptions and monitoring. Forest. Ecol. Manag. 291, 442-457. doi: 10.1016/j.foreco.2012.11.007
Cochrane, J., and Daniels, L. D. (2008). Striking a balance: safe sampling of partial stem cross-sections in British Columbia. BC JEM 9, 38–46.
Coogan, S. C. P., Daniels, L. D., Boychuk, D., Burton, P. J., Flannigan, M. D., Gaulthier, S., et al. (2021). Fifty years of wildland fire science in Canada. Can. J. Forest. Res. 51, 283–302. doi: 10.1139/cjfr-2020-0314
Daniels, L. D. (2004). “Climate and fire: a case study of the Cariboo forest, British Columbia,” in Proceedings of the Mixed Severity Fire Regimes: Ecology and Management Conference, eds L. Taylor, J. Zelnik, S. Cadwallader, and B. Hughes (Pullman, WA: Washington State University), 235–246.
Daniels, L. D., Gray, R. W., and Burton, P. J. (2020). “2017 Megafires in British Columbia - Urgent need to adapt and improve resilience to wildfire,” in Proceedings of the Fire Continuum – Preparing for the Future of Wildland Fire, eds S. M. Hood, S. Drury, T. Steelman, and R. Steffens (Fort Collins, CO: Rocky Mountain Research Station).
Daniels, L. D., Sherriff, R. L., Yocom-Kent, L., and Heyerdahl, E. H. (2017). “Deciphering the complexity of historical fire regimes: Diversity among forests of western North America,” in Dendroecology: Tree-ring Analyses Applied to Ecological Studies, eds M. M. Amoroso, L. D. Daniels, P. J. Baker, and J. J. Camerero (Switzerland: Springer Nature), 185–210. doi: 10.1007/978-3-319-61669-8_8
Daniels, L. D., and Watson, E. (2003). Climate-Fire-Vegetation Interactions in the Cariboo Forests: A Dendrochronological Analysis. Vancouver, BC: Forest Innovation and Investment, Forest Research Program.
Day, J. K. (1998). Selection Management of Interior Douglas-fir for Mule Deer Winter Range. Master’s Thesis. Vancouver, BC: University of British Columbia.
Day, K. (2007). Management and Working Plan #3 for UBC Alex Fraser Research Forest. Vancouver, BC: UBC Faculty of Forestry.
DellaSala, D. A., and Hanson, C. T. (2019). Are wildland fires increasing large patches of complex early seral forest habitat?”. Diversity 11:157. doi: 10.3390/d11090157
Duncan, R. P. (1989). An evaluation of errors in tree age estimates based on increment cores in kahikatea (Dacrycarpus dacrydioides). New Zeal. Nat. Sci. 16, 31–37.
Environment Canada (2017). Canadian climate normals 1981 – 2010. Available online at: http://climate.weather.gc.ca/climate_normals/results_1981_2010_e.html [Accessed May 24, 2017]
Falk, D. A., Heyerdahl, E. K., Brown, P. M., Farris, C., Fulé, P. Z., McKenzie, D., et al. (2011). Multi-scale controls of historical forest-fire regimes: new insights from fire scar networks. Front. Ecol. Environ. 9:446–454. doi: 10.2307/23034492
Falk, D. A., Miller, C., McKenzie, D., and Black, A. E. (2007). Cross-scale analysis of fire regimes. Ecosystems 10, 809–823. doi: 10.1007/s10021-007-9070-7
Fulé, P. Z., Covington, W. W., and Moor, M. M. (1997). Determining reference conditions for ecosystem management of southwestern ponderosa pine forests. Ecol. Appl. 7, 895-908.
Fulé, P. Z., Swetnam, T. W., Brown, P. M., Falk, D. A., Peterson, D. L., Allen, C. D., et al. (2013). Unsupported inferences of high-severity fire in historical dry forests of the western United States: response to Williams and Baker. Glob. Ecol. Biogeog. 23, 825–830. doi: 10.1111/geb.12136
Greene, G. A., and Daniels, L. D. (2017). Spatial interpolation and mean fire interval analyses quantify historical mixed-severity fire regimes. Int. J. Wildland Fire 26, 136–147. doi: 10.1071/WF16084
Guiterman, C. H., Margolis, E. Q., Allen, C. D., Falk, D. A., and Swetnam, T. W. (2018). Long-term persistence and fire resilience of oak shrubfields in dry conifer forests of northern New Mexico. Ecosystems 21, 943–959. doi: 10.1007/s10021-017-0192-2
Hagmann, R. K., Hessburg, P. F., Prichard, S., Povak, N. A., Brown, P. B., Fulé, P. Z., et al. (2021). Evidence for widespread changes in the structure, composition, and fire regimes of western North American forests. Ecol. Appl.
Halofsky, J. E., Donato, D. C., Hibbs, D. E., Campbell, J. L., Cannon, M. D., Fontaine, J. B., et al. (2011). Mixed-severity fire regimes: lessons and hypotheses from the Klamath-Siskiyou Ecoregion. Ecosphere 2, 1-19. doi: 10.1890/ES10-00184.1
Halofsky, J. E., Peterson, D. L., and Harvey, B. J. (2020). Changing wildfire, changing forests: the effects of climate change on fire regimes and vegetation in the Pacific Northwest, USA. Fire Ecol. 16:4. doi: 10.1186/s42408-019-0062-8
Harvey, J. E., Smith, D. J., and Veblen, T. T. (2017). Mixed-severity fire history at a forest-grassland ecotone in west central British Columbia, Canada. Ecol. Appl. 27:6. doi: 10.1002/eap.1563
Hermann, R. M., and Lavender, D. P. (1990). “Douglas-fir,” in Silvics of North America: 1. Conifers, eds R. M. Burns and B. H. Honkala (Washington, DC: USDA Forest Service).
Hessburg, P. F., Agee, J. K., and Franklin, J. F. (2005). Dry forests and wildland fires of the inland Northwest USA: contrasting the landscape ecology of the pre-settlement and modern eras. Forest Ecol. Manag. 211, 117-139. doi: 10.1016/j.foreco.2005.02.016
Hessburg, P. F., Miller, C. L., Parks, S. A., Povak, N. A., Taylor, A. H., Higuera, P. E., et al. (2019). Climate, environment, and disturbance history govern resilience of western North American Forests. Front. Ecol. Evol. 7:239. doi: 10.3389/fevo.2019.00239
Hessburg, P. F., Spies, T. A., Perry, D. A., Skinner, C. N., Taylor, A. H., Brown, P. M., et al. (2016). Tamm review: Management of mixed-severity fire regime forests in oregon, Washington, and Northern California. Forest Ecol. Manag. 366, 221-250. doi: 10.1016/j.foreco.2016.01.034
Heyerdahl, E. K., Lertzman, K. P., and Karpuk, S. (2007). Local-scale controls of a low-severity fire regime (1750-1950), southern British Columbia, Canada. Ecoscience 14, 40-47.
Heyerdahl, E. K., Lertzman, K. P., and Wong, C. M. (2012). Mixed-severity fire regimes in dry forests of southern interior British Columbia, Canada. Can. J. For. Res. 42, 88–98. doi: 10.1139/x11-160
Heyerdahl, E. K., McKenzie, D., Daniels, L. D., Hessl, A. E., Littell, J. S., and Mantua, N. J. (2008). Climate drivers of regionally synchronous fires in the inland Northwest (1651-1900). Int. J. Wildland Fire 17, 40–49. doi: 10.1071/WF07024
Holmes, R. L. (1986). “Quality control of crossdating and measuring,” in User’s Manual for Computer Program COFECHA - Laboratory of Tree-Ring Research, eds R. L. Holmes, R. K. Adams, and H. C. Fritts (Tucson, AZ: University of Arizona), 41–49.
Jonsson, B., Holm, S., and Kallur, H. (1992). A forest inventory method based on density-adapted circular plot size. Scand. J. For. Res. 7, 405-421. doi: 10.1080/02827589209382733
Keane, R. E., Hessburg, P. F., Landres, P. B., and Swanson, F. J. (2009). The use of historical range and variability (HRV) in landscape management. Forest Ecol. Manag. 258, 1025-1037. doi: 10.1016/j.foreco.2009.05.035
Klenner, W., Walton, R., Arsenault, A., and Kremsater, L. (2008). Dry forests in the Southern Interior of British Columbia: Historic disturbances and implications for restoration and management. Forest Ecol. Manag. 256, 1711–1722. doi: 10.1016/j.foreco.2008.02.047
Klinka, K., Worral, J., Skoda, L., and Varga, P. (2000). The Distribution and Synopsis of Ecological and Silvical Characteristics of Tree Species of British Columbia’s Forests. Available online at: https://www2.gov.bc.ca/gov/content/industry/forestry/managing-our-forest-resources/silviculture/tree-species-selection/tree-species-compendium-index (accessed July 15, 2017).
Koot, C., Day, K., Ewen, S., and Skea, D. (2015). Harvesting on Mule Deer Winter Range Under General Wildlife Measures for Shallow and Moderate Snowpack Zones: Approach and Lessons Learned Following a Second Harvest Entry After 30 Years. Williams Lake, BC: Report to Ministry of Forests, Lands and Natural Resource Operations, Cariboo Region, 38.
Lake, F., and Christianson, A. C. (2019). “Indigenous fire stewardship,” in Encyclopedia of Wildfires and Wildland-Urban Interface (WUI) Fires, ed. S. L. Manzello (Switzerland: Springer).
Landres, P. B., Morgan, P., and Swanson, F. J. (1999). Overview of the use of natural variability concepts in managing ecological systems. Ecol. Apps. 9, 1179-1188.
Larsson, L. (2011a). CooRecorder Program of the CDendro Package Version 7.8. Cybis Elektronik & Data AB. Sweden: Saltsjöbaden.
Larsson, L. (2011b). CDendro Program of the CDendro Package Version 7.8. Cybis Elektronik & Data AB. Sweden: Saltsjöbaden.
Leclerc, M.-A. F., Daniels, L. D., and Carroll, A. L. (2021). Managing wildlife habitat: complex interactions with biotic and abiotic disturbances. Front. Ecol. Evol. 9:613371. doi: 10.3389/fevo.2021.613371
Lewis, M., Christianson, A., and Spinks, M. (2018). Return to flame: reasons for burning in lytton first nation, British Columbia. J. Forest. 116, 143-150. doi: 10.1093/jofore/fvx007
Long, J. W., Lake, F. K., Goode, R. W., and Burnette, B. M. (2020). How traditional tribal perspectives influence ecosystem restoration. Ecopsychology 12, 71–82. doi: 10.1089/eco.2019.0055
Marcoux, H. M., Gergel, S. E., and Daniels, L. D. (2013). Mixed-severity fire regimes: how well are they represented by existing fire-regime classification systems? Can. J. For. Res. 43, 658-668. doi: 10.1139/cjfr-2012-0449
Moritz, M. A., Topik, C., Allen, C. D., Hessburg, P. F., Morgan, P., Odion, D. C., et al. (2018). A Statement of Common Ground Regarding the Role of Wildfire in Forested Landscapes of the Western United States. Fire Research Consensus Working Group Final Report. Available online http://www.nwfirescience.org/biblio/statement-common-ground-regarding-role-wildfire-forested-landscapes-western-united-states. (accessed February 25, 2021)
North, M. P., Stephens, S. L., Collins, B. M., Agee, J. K., Aplet, G., Franklin, J. F., et al. (2015). Reform forest fire management. Science 349, 1280–1281. doi: 10.1126/science.aab2356
Northern Shuswap Tribal Council (2014). History and Lands. Available online at: https://nstq.ca/history/ (accessed June 3, 2020).
Norton, D. A., Palmer, J. G., and Ogden, J. (1987). Dendroecological studies in New Zealand 1. An evaluation of tree age estimates based on increment cores. New Zeal. J. Bot. 25, 373–383. doi: 10.1080/0028825X.1987.10413355
Odion, D. C., Hanson, C. T., Arsenault, A., Baker, W. L., DellaSala, D. A., Hutto, R. L., et al. (2014). Examining historical and current mixed-severity fire regimes in ponderosa pine and mixed-conifer forests of western North America. PLoS One 9:e87852. doi: 10.1371/journal.pone.0087852
Parminter, J. (1991). Burning Alternatives Panel: A Review of Fire Ecology, Fire History and Prescribed Burning in Southern British Columbia. Available online at: https://www.for.gov.bc.ca/hre/pubs/docs/sifmc.pdf (accessed November 7, 2016)
Perry, D. A., Hessburg, P. F., Skinner, C. N., Spies, T. A., Stephens, S. L., Tayler, A. H., et al. (2011). The ecology of mixed severity fire regimes in Washington, Oregon, and Northern California. Forest Ecol. Manag. 262, 703-717. doi: 10.1016/j.foreco.2011.05.004
R Core Team (2021). R: A Language and Environment for Statistical Computing (4.0.5) [Computer software]. Available online at: http://www.R-project.org
Schoennagel, T., and Nelson, C. R. (2011). Restoration relevance of recent national fire plan treatments in forests of the western United States. Front. Ecol. Environ. 9:271-277. doi: 10.1890/090199
Sherriff, R. L., and Veblen, T. T. (2006). Ecological effects of changes in fire regimes in Pinus ponderosa ecosystems in the Colorado Front Range. J. Veg. Sci. 17, 705-718. doi: 10.1111/j.1654-1103.2006.tb02494.x
Spies, T. A., Hemstrom, M. A., Youngblood, A., and Hummel, S. (2006). Conserving old-growth forest diversity in disturbance-prone landscapes. Conserv. Biol. 20, 351-362. doi: 10.1111/j.1523-1739.2006.00389.x
Stephens, S. L., Agee, J. K., Fulé, P. Z., North, M. P., Romme, W. H., Swetnam, T. W., et al. (2013). Managing forests and fire in changing climates. Science 342, 41–42. doi: 10.1126/science.1240294
Stephens, S. L., Burrows, H., Buyantuyev, A., Gray, R. W., Keane, R. E., Kubian, R., et al. (2014). Temperate and boreal forest mega-fires: characteristics and challenges. Front. Ecol. Environ. 12, 115–122. doi: 10.1890/120332
Stephens, S. L., Collins, B. M., Biber, E., and Fulé, P. Z. (2016). U.S. federal fire and forest policy: emphasizing resilience in dry forests. Ecosphere 7:e01584.
Stephens, S. L., McIver, J. D., Boerner, R. E. J., Fettig, C. J., Fontaine, J. B., Hartsough, B. R., et al. (2012). Effects of forest fuel reduction treatments in the United States. BioScience 62, 549-560. doi: 10.1525/bio.2012.62.6.6
Stephens, S. L., Westerling, A. L., Hurteau, M. D., Peery, Z. M., Schultz, C. A., and Thompson, S. (2020). Fire and climate change: conserving seasonally dry forests is still possible. Front. Ecol. Environ. 18, 354–360. doi: 10.1002/fee.2218
Stokes, M. A., and Smiley, T. L. (1996). An Introduction to Tree-Ring Dating. Tucson, AZ: University of Arizona Press.
Swetnam, T. W., Allen, C. D., and Betancourt, J. L. (1999). Applied historical ecology: using the past to manage for the future. Ecol. Apps. 9, 1189- 1206.
Swetnam, T. W., and Baisan, C. (1996). “Historical fire regime patterns in the southwestern United States since AD 1700,” in Proceedings of the Fire Effects in Southwestern Forests: Fire Symposium, ed. C. D. Allen (Fort Collins, CO: Rocky Mountain Research Station), 11–32.
Turner, N. J. (1999). “Time to burn: Traditional Use of Fire to Enhance Resource Production by Aboriginal Peoples in British Columbia,” in Indians, Fire, and the Land in the Pacific Northwest, ed. R. Boyd (Corvallis, OR: Oregon State University Press), 185–219.
Turner, N. J., Ignace, M. B., and Ignace, R. (2000). Traditional ecological knowledge and wisdom of aboriginal peoples in British Columbia. Ecol. Appl. 10, 1275-1287.
Whitlock, M. C., and Schluter, D. (2009). The Analysis of Biological Data. Greenwood Village, CO: Roberts and Company Publishers.
Williams, M. A., and Baker, W. L. (2012). Spatially extensive reconstructions show variable-severity fire and heterogeneous structure in historical western United States dry forests. Glob. Ecol. Biogeogr. 21, 1042-1052. doi: 10.1111/j.1466-8238.2011.00750.x
Xwisten Nation, Christianson, A. C., Andrew, D., Caverley, N., and Eustache, J. (2018). Burn Plan Framework Development: Re-Establishing Indigenous Cultural Burning Practices to Mitigate Risk From Wildfire and Drought. Canadian Institute of Forestry e-lecture. Available online at http://cif-ifc.adobeconnect.com/pcrqtu6d3fgk/ (Accessed June 3, 2020)
Yocom Kent, L. L. (2014). An Evaluation of Fire Regime Reconstruction Methods. ERI Working Paper No. 32. Ecological Restoration Institute and Southwest Fire Science Consortium. Flagstaff, AZ: Northern Arizona University, 15.
Zeileis, A. (2005). A unified approach to structural change tests base on ML Scores, F Statistics, and OLS residuals. Econom. Rev. 24, 445–466. doi: 10.1080/07474930500406053
Zeileis, A., Kleiber, C., Krämer, W., and Hornik, K. (2003). Testing and dating of structural changes in practice. Comput. Sta.t Data An. 44, 109–123. doi: 10.1016/S0167-9473(03)00030-6
Keywords: dendroecological reconstruction, Douglas-fir, fire scars, post-fire cohort, frequency, severity, human impacts, mixed-severity fire regime
Citation: Brookes W, Daniels LD, Copes-Gerbitz K, Baron JN and Carroll AL (2021) A Disrupted Historical Fire Regime in Central British Columbia. Front. Ecol. Evol. 9:676961. doi: 10.3389/fevo.2021.676961
Received: 06 March 2021; Accepted: 04 June 2021;
Published: 28 June 2021.
Edited by:
Miguel Montoro Girona, Université du Québec en Abitibi-Témiscamingue, CanadaReviewed by:
Alexandra Rouillard, Arctic University of Norway, NorwayAndy Hennebelle, Université du Québec à Rimouski, Canada
Copyright © 2021 Brookes, Daniels, Copes-Gerbitz, Baron and Carroll. This is an open-access article distributed under the terms of the Creative Commons Attribution License (CC BY). The use, distribution or reproduction in other forums is permitted, provided the original author(s) and the copyright owner(s) are credited and that the original publication in this journal is cited, in accordance with accepted academic practice. No use, distribution or reproduction is permitted which does not comply with these terms.
*Correspondence: Lori D. Daniels, bG9yaS5kYW5pZWxzQHViYy5jYQ==