- 1School of Biological Sciences, Royal Holloway University of London, Egham, United Kingdom
- 2Plant & Food Research Australia, Queensland University of Technology, Brisbane, QLD, Australia
- 3School of Environmental Sciences, University of Guelph, Guelph, ON, Canada
Individual animals allowed the opportunity to learn generally outperform those prevented from learning, yet, within a species the capacity for learning varies markedly. The evolutionary processes that maintain this variation in learning ability are not yet well understood. Several studies demonstrate links between fitness traits and visual learning, but the selection pressures operating on cognitive traits are likely influenced by multiple sensory modalities. In addition to vision, most animals will use a combination of hearing, olfaction (smell), gustation (taste), and touch to gain information about their environment. Some animals demonstrate individual preference for, or enhanced learning performance using certain senses in relation to particular aspects of their behaviour (e.g., foraging), whereas conspecific individuals may show different preferences. By assessing fitness traits in relation to different sensory modalities we will strengthen our understanding of factors driving observed variation in learning ability. We assessed the relationship between the olfactory learning ability of bumble bees (Bombus terrestris) and their foraging performance in their natural environment. We found that bees which failed to learn this odour-reward association had shorter foraging careers; foraging for fewer days and thus provisioning their colonies with fewer resources. This was not due to a reduced propensity to forage, but may have been due to a reduced ability to return to their colony. When comparing among only individuals that did learn, we found that the rate at which floral resources were collected was similar, regardless of how they performed in the olfactory learning task. Our results demonstrate that an ability to learn olfactory cues can have a positive impact of the foraging performance of B. terrestris in a natural environment, but echo findings of earlier studies on visual learning, which suggest that enhanced learning is not necessarily beneficial for bee foragers provisioning their colony.
Introduction
An animal’s capacity for learning can influence essentially every aspect of its behaviour, including its ability to find food, attract mates, and avoid predators (Nowicki et al., 2002; Lönnstedt et al., 2012; Sergio et al., 2014). Individuals given the opportunity to learn associations between sensory cues and risk/reward outcomes generally outperform those prevented from learning (e.g., higher mating success, growth, reproductive output; Dukas and Bernays, 2000; Dukas and Duan, 2000; Lönnstedt et al., 2012; Ward-Fear et al., 2016). Ultimately, learning enables individuals to respond to environmental change within their lifetime (Greenlees et al., 2010; Ward-Fear et al., 2016).
Despite these apparent advantages of learning, considerable variation in learning capacity can often be observed among individuals within the same species (e.g., Chittka et al., 2003; Raine et al., 2006b; van den Berg et al., 2011; White and Brown, 2014). This intraspecific variation exists because being a “good learner” does not always provide an overall fitness benefit. Cognitive function has a metabolic cost (Foley and Lee, 1991) and inherently fast-learning individuals may face trade-offs between learning and other cognitive functions (Hermer et al., 2018) or other energetically demanding processes including longevity, immune function and reproduction (Dukas, 1999; Mallon et al., 2003; Mery and Kawecki, 2003, 2004; Burger et al., 2008; Burns et al., 2011; Jaumann et al., 2013). Learning can also have an opportunity cost; the time taken for a foraging animal to learn its preferred food source and the subsequent commitment to the learned food source can mean it forgoes exploitation of other resources (Eliassen et al., 2007; Evans and Raine, 2014). While it is clear that these trade-offs can affect the learning abilities of individuals, the evolutionary processes that maintain this variation in learning ability within natural populations are not yet well understood (Raine et al., 2006a; Morand-Ferron and Quinn, 2015; Morand-Ferron, 2017; Boogert et al., 2018).
Links between learning performance and fitness traits under natural conditions have, so far, only been investigated in few species and all these studies focus on visual learning (Raine and Chittka, 2008; Evans et al., 2017; Huebner et al., 2018; Madden et al., 2018). The selection pressures operating on cognitive traits are likely influenced by multiple sensory modalities. In addition to visual cues, most animals will use a combination of sound, taste, touch, and/or smell when forming learnt associations (Dukas, 2008; De Agrò et al., 2020; Flanigan et al., 2021). The relative importance of different sensory modalities can sometimes be obvious with regards to species ecology; depending on whether the animal is active at day or night, either vision or olfaction are often a more prominent modality than the other (Balkenius et al., 2006). But the salience of different modalities can also depend on context and/or environment (Maaswinkel and Whishaw, 1999; Andersson and Dobson, 2003; Kaczorowski et al., 2012), and reliance on a particular cue can adaptively shift depending on environmental conditions (Spaethe et al., 2001; Kaczorowski et al., 2012). To add to this complexity, individual animals can favour different sensory cues than their conspecifics (Smith et al., 2004; Raine and Chittka, 2007; Sato et al., 2014), and can also exhibit better learning performance when using a particular sensory modality (Kunze and Gumbert, 2001; Smith and Raine, 2014). The relationship between learning ability and fitness may therefore differ depending on the sensory modality used to assess learning. By assessing fitness traits in relation to different sensory modalities we will strengthen our understanding of factors driving observed differences in learning ability.
Bumble bees are a useful study system for investigating fitness traits and learning though different sensory modalities, because foragers rely on multiple sensory inputs, which serve different functions. For instance, bumble bees rely on both learnt visual and olfactory cues when locating and evaluating their food sources (Chittka and Raine, 2006). Attraction to flowers at a distance is primarily due to the visual cues of the flowers (Manning, 1956; Heinrich, 1976), whereas floral scents provide a localised cue which a bee uses to discriminate between similar flowers and to reject flowers recently depleted of nectar (Manning, 1956; Wright and Schiestl, 2009). The presence of a learned floral scent determines whether a foraging bee alights and/or probes for nectar (Manning, 1956; Kunze and Gumbert, 2001). Foragers use olfactory and tactile cues to communicate with each other, both directly and indirectly (Dornhaus and Chittka, 2001; Saleh et al., 2006). For example, bees in the nest learn the floral scents carried by incoming foragers, which can influence their subsequent foraging choices (Molet et al., 2009). Foragers can also learn to use the scents produced and deposited on flowers by other bees (cuticular hydrocarbon footprints), to avoid recently visited flowers (Goulson et al., 1998; Stout and Goulson, 2001; Pearce et al., 2017).
Using proboscis extension response (PER) conditioning we assessed the olfactory learning performance of foraging naïve Bombus terrestris individuals in the lab, then monitored their subsequent foraging performance in a natural environment. In doing so, we gained insight into how odour learning affects foraging success and colony provisioning (both proxy measures of colony fitness). We discuss our results in relation to visual learning in B. terrestris, which has previously been assessed in conjunction with foraging performance (see: Raine and Chittka, 2008; Evans et al., 2017).
Materials and Methods
Experimental Setup
Five B. terrestris colonies (obtained from Biobest—Westerlo, Belgium) were each housed in split colony boxes (Figure 1A), which enabled us to assess the olfactory learning performance of foraging naïve bees in the lab and subsequently monitor the foraging performance of the same individuals in a natural environment. Each box was divided in half with mesh (mesh size: 1 × 1 mm), allowing olfactory and/or tactile connections to be maintained between bees and brood on either side of the colony box. One side of the box (internal side) was connected to an enclosed foraging arena (140 × 240 × 120 mm) containing a gravity feeder of sucrose solution (50% v/v) provided ad libitum, and 3 g per day of defrosted honey bee-collected pollen (sourced from Koppert Ltd., United Kingdom). The other (external) side was connected to the outside environment through a tube leading to an exit/entrance hole in the laboratory window (Figures 1D,E), allowing bees on this side to forage naturally (Figure 1F).
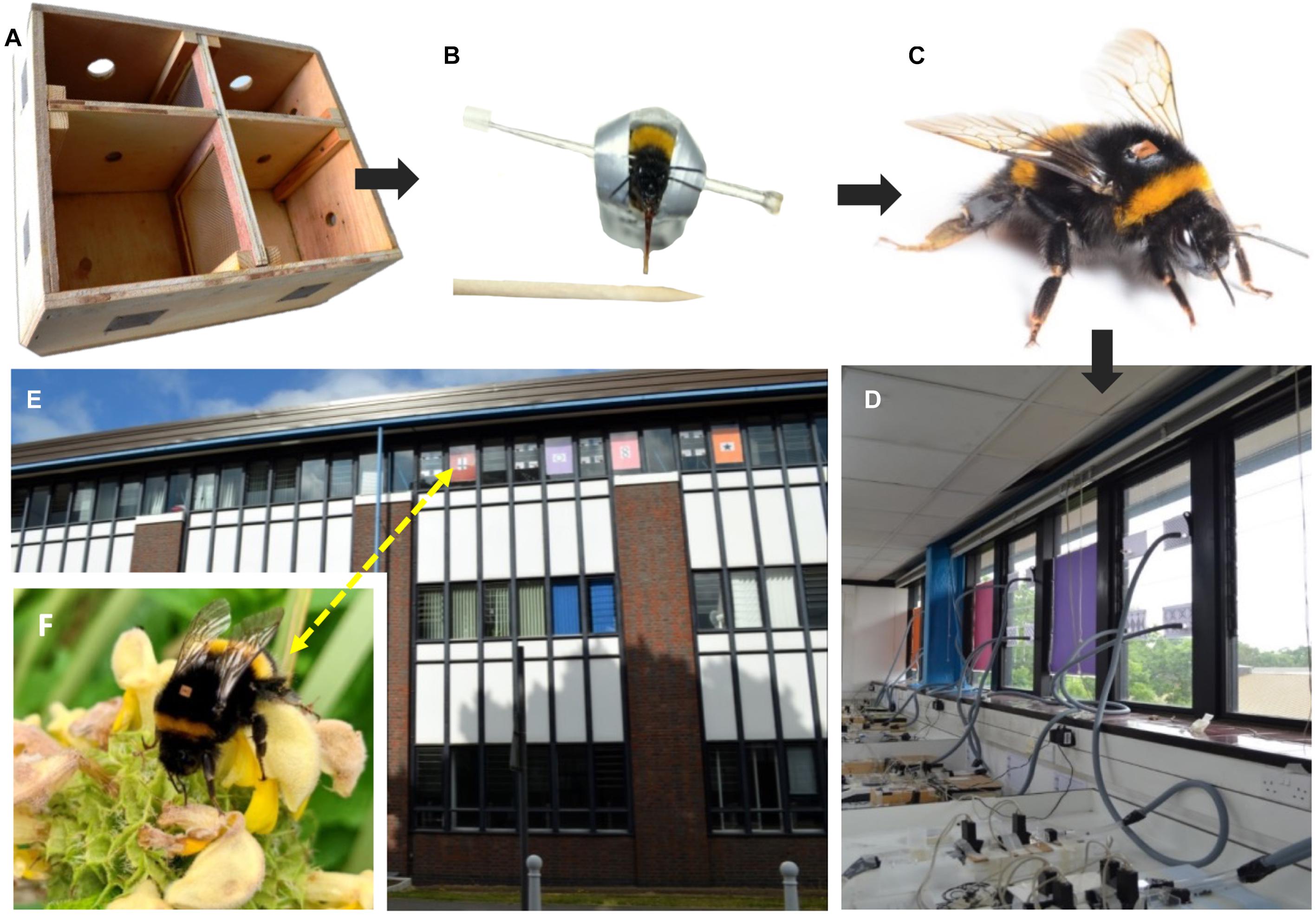
Figure 1. | Diagram showing experimental setup. (A) Divided colony box with a mesh partition, which contained the split colonies (colourless, transparent lid removed for clarity). (B) Bees identified as foragers, based on frequency of foraging in an indoor foraging arena, were harnessed and tested on their olfactory learning ability using proboscis extension response (PER) conditioning paradigm. (C) Assessed individuals were tagged with an RFID tag to enable us to track their subsequent foraging performance. (D) After being assessed the bees were transferred to the (external) side of their divided colony box that was connected to the laboratory window. (E) Each window exit/entrance was marked with a unique pattern to assist retuning foragers in finding their colony. (F) The RFID reader system allowed us to record the foraging behaviour of the bees in the rural/urban landscape surrounding Royal Holloway University of London (Egham Hill, Egham TW20 0EX, United Kingdom). The RFID tag on the bee in image (F) has been added digitally for illustrative purposes. Photos (B,C) provided by Dylan Smith and Brian Cutting, respectively.
At the beginning of the trial, each colony had a queen, brood and an average of 30 workers (range = 23–37), which were divided evenly between the two sides of the colony box. Each queen was moved between sides of the colony box every 24 h to encourage normal queen-right colony behaviour and reduce aggression when tested worker bees were moved between sides (Schmid-Hempel and Schmid-Hempel, 1998; Evans et al., 2017). For the purpose of identification, newly emerged workers on the internal side were marked daily with uniquely identifiable numbered tags (Opalith tags; Christian Graze KG, Germany). Foraging individuals were identified in the foraging arena, assessed in an olfactory learning paradigm (described below), then re-tagged with an Radio Frequency Identification (RFID) tag (details below; Figure 1C) and transferred to the external side of the colony box. Workers emerging in the external side had one of their wings clipped to prevent them from flying and foraging, thus ensuring that only our tested bees (from the internal side) could forage outside. To encourage foraging in our initially small colonies, the external side was provided with no pollen, and only enough sucrose solution to fill three nectar pots—pipetted directly into nectar pots (for a more detailed description of the setup see: Evans et al., 2017).
Assessing Olfactory Learning Performance of Foragers
The five foraging arenas (each connected to the internal side of a different split colony box) were visually checked throughout the day and the identities of any bees on the sucrose or pollen feeders were recorded. Bees were defined as foragers if they had been observed on a feeder on at least three separate occasions, across multiple days. Bees that met these criteria (n = 93 across the five colonies) were assessed on their olfactory learning performance.
Olfactory learning performance was assessed using a PER absolute conditioning paradigm (Riveros and Gronenberg, 2009; Evans et al., 2016). Identified foragers were caught and chilled on ice until they became quiescent and then harnessed within plastic syringe tubes (Figure 1B). The bees were fed with sucrose solution (50% v/v) 3 h after being harnessed. When feeding, their antennae were touched with a pipette containing sucrose. If the bee responded by extending its proboscis it was presented with sucrose solution for 2 s. Each bee was given four opportunities to feed. The harnessed bees were left overnight in a ventilated container. The following morning (ca. 18 h after harnessing) we again checked their responsiveness to sucrose. If the bee responded it was given a small droplet of sucrose and progressed to the training phase. If a bee failed to respond after four attempts it was removed from the trial (n = 7/93; 7.5%).
A bee was assessed on its ability to learn to associate a fruit odour—lemon (essential oil, Calmer solutions), with a sucrose reward. Lemon was chosen as our conditioning odour as it is unlikely that these bees would encounter this odour while foraging outside after their learning assessment (as this could potentially influence their foraging decisions). Prior to every training event each harnessed bee was placed individually in an odour extraction hood. The odour stimulus was released from an odour tube, containing 1 μl of the essential oil on filter paper. The volume of air, flow rate, and duration of the odour presentation was controlled by a Programmable Logic Controller computer. During each trial a bee was exposed to 5 s of unscented air then 10 s of odour-containing air. The bee was presented with 0.8 μl of sucrose solution (using a Gilmont syringe) after approximately 6 s of exposure to the odour-containing air (Evans et al., 2016).
Each bee was subjected to 15 trials in which it was exposed to the odour, with a 12 min interval between trials. After each trial we recorded the bees’ response; whether it extended its proboscis before being presented with reward (conditioned response or learning event), after it was presented with reward (unconditioned response or non-learning event), or not at all (unresponsive). Bees that did not respond for three consecutive trials were removed from the experiment as they were assumed to be senescing or otherwise no longer responding to foraging cues (n = 6/86; 7%). On completion of the 15 trials all the bees were removed from their harnesses and tagged with an RFID tag (Microsensys GmbH: mic3-Tag; Figure 1C), on the back of their thorax over the top of their Opalith tag. Each bee was then placed within the external side of its corresponding colony so that their foraging could be monitored outside the laboratory in a natural environment.
Foraging Performance in a Natural Environment
A pair of RFID readers (Microsensys GmbH: Maja IV reader modules with optimized antenna for mic3-Tag transponders) were attached to the entrance of each colony which recorded when our previously tested and RFID-tagged foragers left and re-entered their nest. This experimental set up yielded data on individual foraging trip frequency and duration. Each colony was also observed for 3 h a day between: 09:00 and 12:00, 12:00 and 15:00 or 15:00 and 18:00, for the duration of the trial (5 days a week for 4 weeks), to estimate the nectar and pollen loads collected by our tagged bees. To control for differences in forager activity levels over the course of a day, the observation period was randomised across colonies. We recorded the mass of any RFID-tagged bee leaving or entering their colony, as they walked over a balance pan (Ohaus AdventurerTM Pro, Ohaus NavigatorTM, and Sartorius Practum 213-IS x 2 all accurate to the nearest 0.001 g). Using the balance’s dynamic weighing function (designed for weighing moving animals), three mass recordings were taken for each bee and the average of these values used in the analysis. A stopwatch synchronized with the time on the RFID readers was used to record the time of each bee observation, enabling us to identify individuals. The size of pollen loads brought back to the colony were non-invasively estimated, so as not to disrupt normal foraging activity. Each pollen load was classified as being either small, medium, large, or very large, relative to the size of the bee (Gill et al., 2012; Gill and Raine, 2014; Evans et al., 2017).
Analysis
Learning Performance
Learning performance scores were generated for bees tested with the PER paradigm by summing each individual’s responses across their 15 conditioning trials. Each correct response (i.e., when a bee extended their proboscis in response to the odour prior to being offered sucrose solution) was given a score of 1, so a learning score of 14 is the maximum a bee could obtain given that no learnt association could have been formed before the first trial. The learning scores were split into four categories: A = 0 (non-learners), B = 1–5, C = 6–10, and D = 11–14 (fastest learners). Learning performance scores were not normally distributed and non-parametric tests were used to assess differences within and among colonies.
Individual Foraging Performance in a Natural Environment
Foraging performance was quantified using the RFID data log of when each tagged bee left and re-entered a colony. These data were manually sorted to determine the number, duration, and timing of the foraging trips made by each bee. As we only tagged foragers, we assumed that all trips away from the nest were foraging bouts (trips), provided that the bee was gone for ≥ 8 min and, once they returned, they stayed in the nest (to off-load pollen and/or nectar) for ≥ 1 min. These thresholds were based on the duration and sequence of activity of visually confirmed foragers during the observation periods (Evans et al., 2017). Bees were only included in our analyses if they completed at least five foraging bouts.
For each forager we determined the colony that it foraged for the most frequently—their “majority colony,” because all foragers visited multiple colonies (mean ± SE = 4.09 ± 0.17 colonies). This drifting is typical for closely situated bumble bee colonies (Zanette et al., 2014), and is comparable to the extent of drifting observed by others using a similar setup to assess foraging activity (Gill et al., 2012; Gill and Raine, 2014; Stanley et al., 2016). For 50% of foragers their majority colony was also their natal colony. On average, foragers performed 57.73 ± 3.92% of their foraging trips for their majority colony, compared to 39.90 ± 5.39% for their natal colony. For this reason “majority colony” was used in all subsequent analysis.
Foraging Efficiency
All formal analyses were conducted in R v 3.0.2 (R Core Development Team., 2014). Using a series of general linear mixed models (GLMM’s, using the lme function in the package nlme: Pinheiro et al., 2014), we determined whether learning ability predicts nectar collection efficiency and/or pollen collection efficiency. Our basic model contained just majority colony as a random effect. This was compared with four different candidate models that contained the basic model and one of the following possible covariates as a fixed effect: worker size, worker age, foraging experience, and the age of colony when the forager was introduced (see Table 1 for variable descriptions). We calculated AICc values (Akaike Information Criterion—corrected for small sample size) for each model (selMod function from the pgirmess package: Giraudoux, 2014) and selected the model with the lowest AICc value. Olfactory learning performance was added (as a factor) to the best model to determine whether it significantly lowered (i.e., ΔAICc > 2 units: Burnham et al., 2011) the model’s AICc. If it did, we concluded that learning performance was predicting the response variable. This bottom-up model building approach is more conservative than a stepwise deletion, but given our limited sample size it is more appropriate as it avoids over-parameterization inherent in small data sets (Raihani and Bshary, 2012). The fit of the best model was checked by plotting the fitted values against the residual values of the model.
Foraging Activity
Mean daily number of bouts and mean bout duration were log10 transformed (to normalise residuals) and analysed with a general mixed model as described above. A generalised linear mixed model (using the glmer function in package lme4: Bates et al., 2014) was used to analyse count data (assumed to have a Poisson error distribution) for number of days spent foraging. A basic model was generated and then compared with three additional models that contained either: worker size, worker age, and majority colony age, in addition to the basic model. Learning performance was added to the model with the lowest AICc. The fit of the best model was checked by plotting the fitted values against the residual values of the model.
Results
Learning Performance
We assessed the olfactory learning performance of 80 foragers (mean = 16, range = 13–19 foragers per natal colony) across five colonies. Seventy-five percent of the bees (n = 60) exhibited at least one learnt response. The proportion of correct choices increased with trial number across all five colonies; rising from 0 to 8% in trial 2 to 6–46% in trial 7, and finally 50–77% in trial 15 (Figure 2A). Whilst learning performance varied within colonies, there was no significant variation in learning performance among colonies (Kruskal-Wallis, H4 = 6.064, p = 0.19; Figure 2B). Forager learning performance was not predictably affected by factors such as worker age (Spearman’s ρ = −0.021, p = 0.92; Supplementary Figure 1A), worker body mass (Spearman’s ρ = −0.145, p = 0.46; Supplementary Figure 1B) or colony age (Spearman’s ρ = 0.037, p = 0.53; Supplementary Figure 1C).
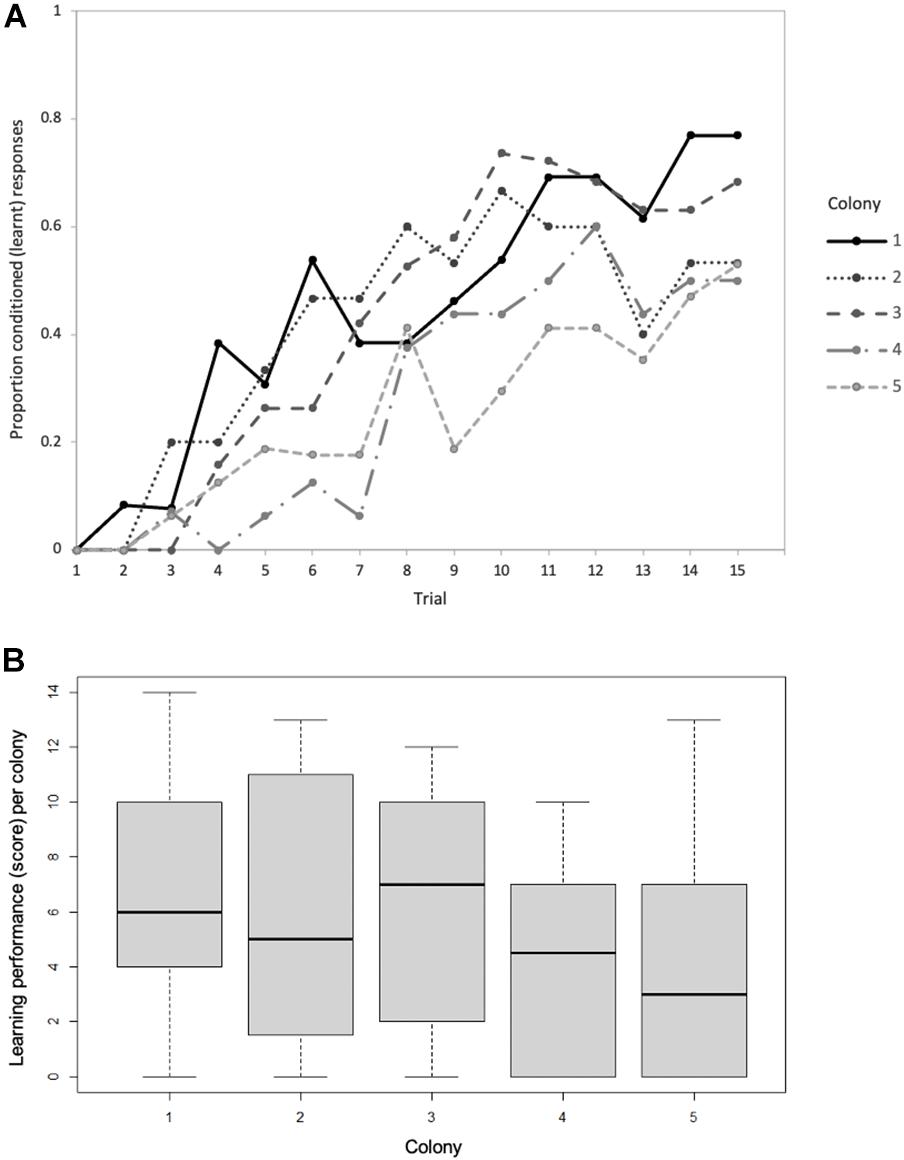
Figure 2. (A) Proportion of conditioned (learnt) responses of Bombus terrestris individuals from five (natal) colonies during 15 sequential proboscis extension response (PER) trials. (B) Variation in learning performance (learning score) of the five natal colonies based on the number of learnt responses per bee. In each box the thick horizontal bar is the colony median, whilst the lower and upper edges represent the 25 and 75% quartiles, respectively. Whiskers indicate the maximum and minimum values that are not outliers. The numbers of bees tested per colony = 13, 15, 19, 16, and 17, respectively.
Individual Foraging Efficiency in a Natural Environment
Individual patterns of foraging activity were recorded when each RFID-tagged bee left and returned to their nest. We found that 48.8% (n = 39/80) of our tagged bees completed at least five foraging bouts (flights outside the colony lasting at least 8 min). During daily observations, we recorded the efficiency of pollen/nectar collection in 10.27% of the foraging bouts undertaken by 84.6% (n = 33/39) of the tagged foragers. The number of foraging bouts for which pollen/nectar collection was observed per bee ranged between 2 and 22, and was directly proportional to the total number of foraging bouts undertaken by each bee (Spearman’s ρ = 0.73, n = 33, p < 0.001).
Twenty two tagged bees were further classified as nectar (n = 15) and/or pollen (n = 14) foragers, based on having recorded at least two nectar or pollen-collecting bouts (range = 2–17) during our observations. Thirty two percent (n = 7/22) of these bees foraged for both nectar and pollen, in separate trips. The nectar and pollen collection rates of these bees were positively correlated (Pearson’s Correlation, r = 0.81, n = 7, p = 0.03), but this relationship was driven by a single bee that collected both floral resources at a high rate (Supplementary Figure 2).
Our best model provides no strong evidence that olfactory learning performance predicts nectar collection efficiency or pollen collection efficiency (Table 2). For nectar collection, the best model was the basic model (which represents the null hypothesis). For pollen collection, although the best model did include learning score, the AICc score of this model was not significantly (>2 AIC units) lower than that of the basic model (ΔAICc 0.57; Table 2; raw data presented in Supplementary Figure 3 for nectar and pollen collection).
Individual Foraging Activity in a Natural Environment
When comparing all bees, a binomial test indicates that there was no difference in the proportion of non-learners (0.50) and learners (0.55) to forage (p = 0.90, 95% CI [−0.34, 0.24]). Assessed bees foraged for between 1 and 15 days (mean ± [SE] = 5.64 ± 0.58), completing between 1 and 26 foraging bouts per day (mean ± [SE] = 10.37 ± 0.98), with each bout lasting between 28.67 and 184 min (mean ± [SE] = 67.46 ± 5.57). Once foraging outside of the laboratory, 97% of the bees continued foraging for consecutive days, with the exception of one bee that had two, 1-day breaks during its 15 days of foraging.
Comparing candidate models, learning performance was also not a good predictor of mean number of bouts per day, mean bout duration, or number of foraging days (Table 3; raw data presented in Supplementary Figure 4). However, visual inspection of the raw data suggested that learning per se did appear to affect Number of foraging days as bees that showed some learning (learning scores 1–5: mean days foraging = 7.08 ± 0.98 [SE]; learning scores 6–10: mean days foraging = 6.22 ± 0.83 [SE]) foraged for more days than non-learning individuals (mean days foraging = 3.91 ± 1.19 [SE]) Figure 3A). Accordingly, when bees were included in the models as non-learners or learners (i.e., a binomial category), learning ability was a good predictor of days foraged. The best model, which contained majority colony, age and learning (model estimate for non-learners: 8.86 ± 4.71[SE] and for learners: 14.66 ± 3.72[SE]) was a significant improvement on the basic model and all tested alternative models (ΔAICc 5.95; Table 4; raw data are summarised in Figure 3B and presented in full in Supplementary Figure 4). Learning was also added as a binomial category in models for mean number of bouts per day and mean bout duration, however, this did not alter the model’s predictions (Supplementary Table 1).
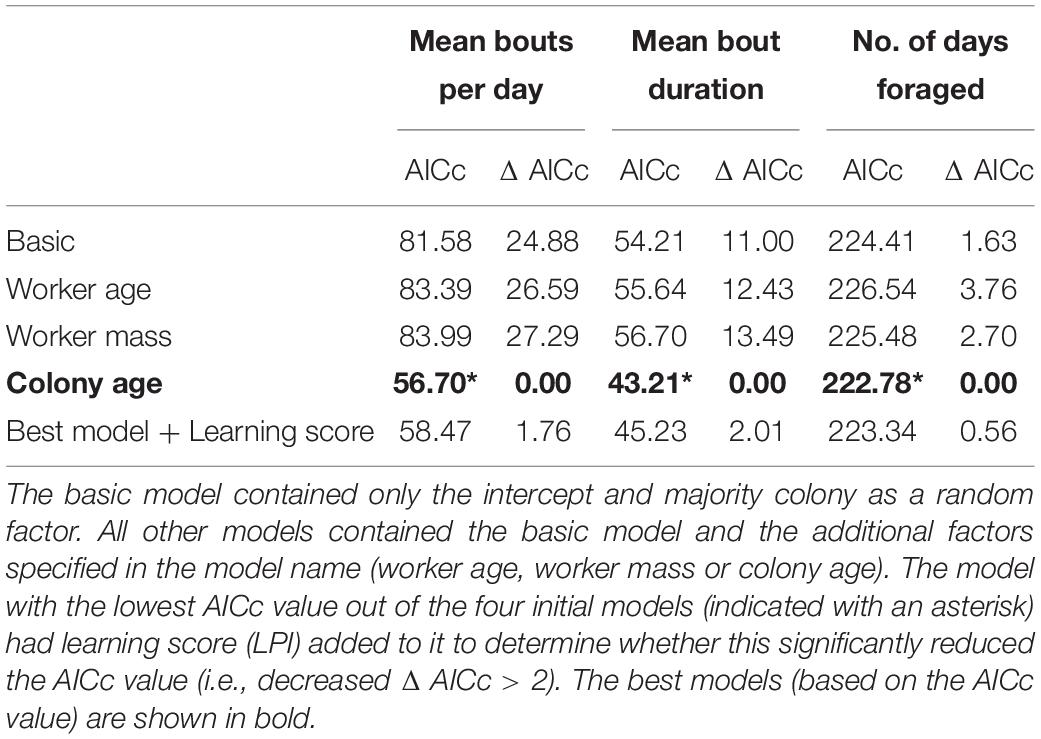
Table 3. Candidate models to predict the mean number of foraging bouts conducted per day, mean foraging bout duration, and number of days spent foraging by tested foragers.
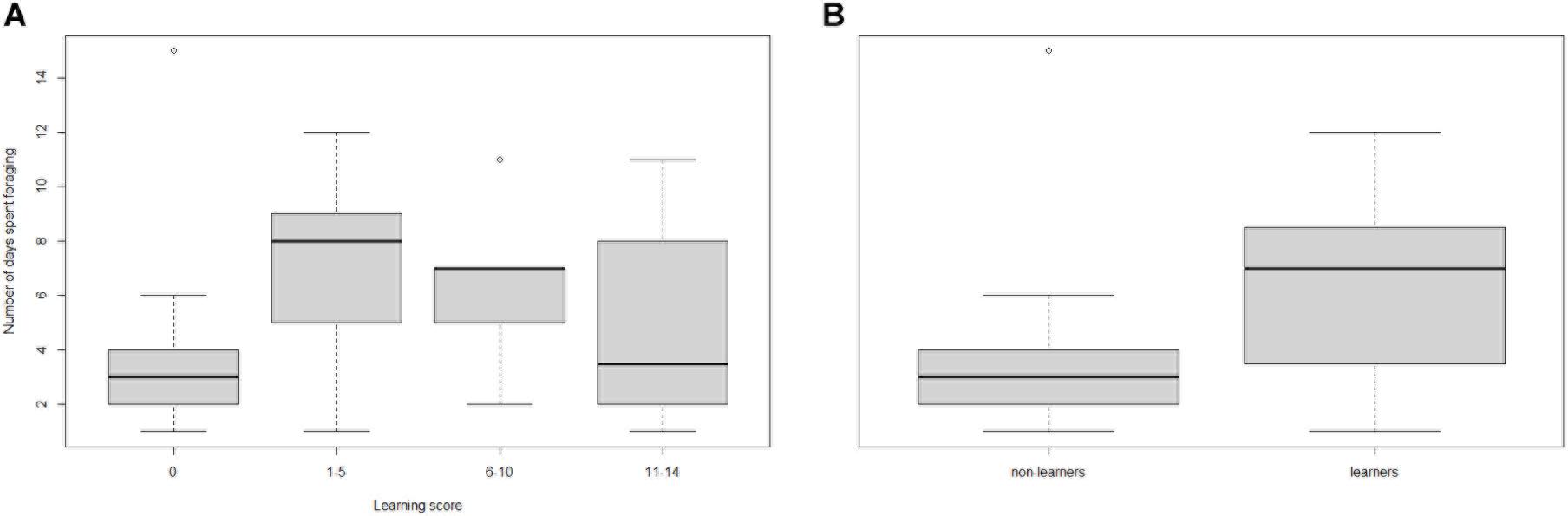
Figure 3. Box and whisker plot showing (A) the number of days spent foraging against olfactory learning performance index score, and (B) the number of days spent foraging by non-learners vs. learners (raw data). High learning scores are indicative of fast learning individuals, whilst a score of zero means they showed no sign of learning. The number of bees in each learning category: 0 = 11, 1–5 = 13, 6–10 = 9, and 11–14 = 6. In each box the thick horizontal line indicates the median, whilst the lower and upper edges represent the 25 and 75% quartiles, respectively. Whiskers indicate minimum and maximum values that are not outliers. Outliers (± 1.5*IQR) are represented by open circles. (A) learning score 0: mean days foraging 3.91 ± 1.19 [SE]; learning score 1–5: 7.08 ± 0.98 [SE]; learning score 6–10: 6.22 ± 0.83 [SE]; learning score 11–15: 4.83 ± 1.62 [SE], (B) Non-learners mean days foraging: 3.91 ± 1.19 [SE], learners: 6.32 ± 0.63 [SE].
Discussion
To determine whether the olfactory learning abilities of B. terrestris individuals predict their foraging performance in a natural environment, PER conditioning was used to assess olfactory learning in the laboratory before the foraging performance of the same individuals was monitored in the field. When comparing all individuals that demonstrated odour learning, we found that their learning performance did not predict their foraging efficiency (i.e., nectar or pollen collection rates), daily foraging activity (numbers of bouts completed), or forage-bout duration. The daily rate at which foragers collected floral resources (nectar or pollen) was similar, regardless of how they performed in the olfactory learning task. However, olfactory learning per se predicted the duration of their foraging career. Bees that demonstrated some ability to use odour cues as a predictor of sucrose solution reward (learning scores of 1–14) foraged for more days than non-learning individuals (learning score = 0). Consequently, odour learning individuals provided food resources for their colony over a longer period of time.
It is not clear why the non-learning bees foraged for fewer days compared to bees that exhibited some olfactory learning. These bees did not have a lower propensity to forage in their natural environment; they were just as likely to forage as bees demonstrating learning. It is possible that these non-learning individuals were in poor condition and therefore not motivated to learn and more likely to not forage for long or to die early. However, whilst these bees did not learn, they were still responsive/motivated by sucrose throughout the laboratory PER conditioning assessment. It is reasonable to expect that if the bees had been in poor condition they would have been generally less responsive. The non-learning bees were also a similar size and age to their nest mates, meaning it is unlikely that they had a reduced ability to detect the olfactory cues in the learning assessment because they had lower olfactory sensitivity (Spaethe et al., 2007), or because their olfactory systems were less developed (Ray and Ferneyhough, 1997; Laloi et al., 2001). It is possible that the bees demonstrating no olfactory learning were ill equipped for foraging in their natural foraging environment. As well as being important for flower selection, olfactory learning is likely to be important for predator avoidance; enabling bees to detect and avoid potentially lethal encounters with predatory insects (Reader et al., 2006; Bray and Nieh, 2014; Li et al., 2014). Olfactory learning is also necessary for homing/navigation; olfactory cues near the nest are learned as guides for returning foragers (Foster and Gamboa, 1989; Saleh et al., 2007). Consequently, these bees might have been more likely to succumb to predation or become lost whilst foraging.
Despite the olfactory and visual sensory systems in bees serving some distinct functions (Wright and Schiestl, 2009), and there being differences in the way these cues are learned and retained (Menzel and Greggers, 1985; Kunze and Gumbert, 2001), the relationship between learning ability and foraging performance among B. terrestris individuals was similar, regardless of whether learning was assessed using an olfactory (this study) or a visual (colour learning) task (the latter results presented in Evans et al., 2017). Like olfactory learning performance, visual learning performance did not predict floral resource collection rates, daily foraging activity levels, or foraging bout duration (Evans et al., 2017). However, we did find differences in the relationship between olfactory/visual learning performance and the amount of foraging undertaken overall. When comparing only among individuals that learnt the olfactory cues (i.e., non-learners were excluded), we found that the duration of their foraging careers was not predicted by how they performed in the olfactory learning task. In contrast, visual (colour) learning performance did predict foraging career duration, with the fastest visual learners foraging for fewer days overall (Evans et al., 2017).
The shorter foraging careers of faster visual learners (Evans et al., 2017) was thought to have resulted from the energetic cost associated with enhanced cognitive performance, which can negatively impact other energetically demanding processes (Mery and Kawecki, 2003; Mery and Kawecki, 2004; Snell-Rood et al., 2011; Jaumann et al., 2013). Another study provides evidence of a “trade-off” in the opposite direction—increased foraging time lowered olfactory learning performance (reversal learning) among honey bees (Cabirol et al., 2018), further support for an inverse relationship between learning and foraging duration. In the current study, the fastest olfactory learners also had a tendency to forage for fewer days than “average” learners, although this trend was not statistically significant. It is possible that this relationship was less pronounced in the current study because of the smaller number of foragers monitored (compared to Evans et al., 2017). It should be noted that whilst PER is a well-established method of assessing classical conditioning for honey bees and bumble bees (e.g., Takeda, 1961; Riveros and Gronenberg, 2009; Giurfa and Sandoz, 2012), this is the first time the foraging performance of bees has been assessed after completing PER. Our data suggest that the PER assay could have affected the performance of foragers. In comparison with the bees for which colour learning was assessed (by Evans et al., 2017), 10% fewer individuals in the olfactory PER assay foraged, and those that did forage completed 25 percent fewer foraging bouts and foraged for two thirds as long. Reducing the time the bees spend in a harness for PER conditioning may improve results obtained in future studies.
While we have shown that learning is associated with the foraging career duration of B. terrestris workers, we have not demonstrated a relationship between olfactory learning performance and rate of resource collection by individual bees. Such a relationship between these variables might be expected because the ability to rapidly learn salient floral cues is thought to enable foragers to better track changes in floral resources that vary across time and space and among plant species (Laverty, 1980; Menzel, 1993; Chittka, 1998). Even a slight decrease in the time spent locating or handling each flower may be an advantage because in a single day individuals will visit thousands of flowers to support themselves and their colony (Raine et al., 2006b). However, it is possible that any benefits in flower-handling efficiency are negligible compared to other time-intensive elements of foraging, including travel between the colony and multiple resource patches (Lihoreau et al., 2010, 2012). It is also possible that we have not used the best measure for assessing foraging efficiency. In addition to needing a sufficient quantity of food, bees require diverse and high quality protein and micronutrients for maintaining healthy workers and to rear their brood (Alaux et al., 2010; Di Pasquale et al., 2013; Vaudo et al., 2016). In future, it may be more useful to consider foraging efficiency in terms of the quality of the floral resource (e.g., the sucrose content of nectar and protein content of pollen), and/or the diversity of pollen sources collected as well as the amount of pollen and nectar collected.
Another possible explanation for our results is that the ability to learn odours more quickly in the rural/residential landscape surrounding the test site (Royal Holloway; Egham TW20 0EX, United Kingdom) simply may not have conferred an advantage in terms of foraging efficiency. Because of the costs associated with learning, its adaptation is expected to be fined-tuned to prevailing ecological (and social) conditions; leading it to be more important in some environments than others (Stephens, 1991; Dunlap and Stephens, 2016; Morand-Ferron et al., 2019). This remains a possible explanation for the apparent lack of relationship between individual learning (either olfactory or visual) and foraging (olfactory assessed in this study and visual in Evans et al., 2017), while (visual) learning and nectar foraging performance of 12 B. terrestris colonies were strongly correlated in an urban habitat in central London (Raine and Chittka, 2008). In this urban experiment, bumble bee colonies containing the fastest colour learning individuals also brought in nectar at significantly higher rates in those environmental conditions.
Overall our results suggest that olfactory learning plays a role in foraging success for B. terrestris. Individuals that were able to learn the scent-reward association had a longer foraging career and as a consequence collected more floral resources for their colony overall. The reason that non-learners foraged for fewer days remains unclear, it was not due to a reduced propensity to forage, and further work would be needed to determine if it could have been due to a reduced ability of foragers to return to their colony. We did not find statistical support for faster olfactory learners being more efficient or active foragers. Instead our results echo the findings of studies using visual learning (Evans et al., 2017); that suggest a balance exists between the benefits and costs associated with learning.
Data Availability Statement
The original contributions presented in the study are included in the article/Supplementary Material, further inquiries can be directed to the corresponding author/s.
Author Contributions
LE and KS conceived the project, carried-out the experiment and statistical analyses. LE, KS, and NR designed the research. LE and NR wrote the manuscript. All authors contributed to the article and approved the submitted version.
Funding
The authors gratefully acknowledge the support of the Natural Sciences and Engineering Research Council of Canada in the form of a Discovery Grant (RGPIN-2015-06783) and the Food from Thought: Agricultural Systems for a Healthy Planet Initiative, by the Canada First Research Excellence Fund (grant 000054). LE was supported by The New Zealand Institute for Plant and Food Research and the following scholarships: C. Alma Baker, A.G.K. Overseas Research, and a Queen Elizabeth’s Technicians Award. KS was supported by a Crosslands Research Scholarship and the Atkins bequest from Royal Holloway University of London. NR was supported as the Rebanks Family Chair in Pollinator Conservation by the Weston Family Foundation.
Conflict of Interest
The authors declare that the research was conducted in the absence of any commercial or financial relationships that could be construed as a potential conflict of interest.
Publisher’s Note
All claims expressed in this article are solely those of the authors and do not necessarily represent those of their affiliated organizations, or those of the publisher, the editors and the reviewers. Any product that may be evaluated in this article, or claim that may be made by its manufacturer, is not guaranteed or endorsed by the publisher.
Acknowledgments
We would like to thank Emily Parsons for her help monitoring field foraging bees, Oscar Ramos-Rodriguez for technical assistance, and also Brian Cutting and Jenny Jandt for commenting on the manuscript.
Supplementary Material
The Supplementary Material for this article can be found online at: https://www.frontiersin.org/articles/10.3389/fevo.2021.676289/full#supplementary-material
References
Alaux, C., Ducloz, F., Crauser, D., and Le Conte, Y. (2010). Diet effects on honeybee immunocompetence. Biol. Lett. 6, 562–565. doi: 10.1098/rsbl.2009.0986
Andersson, S., and Dobson, H. E. M. (2003). Behavioral foraging responses by the butterfly Heliconius melpomene to Lantana camara floral scent. J. Chem. Ecol. 29, 2303–2318. doi: 10.1023/A:1026226514968
Balkenius, A., Rosén, W., and Kelber, A. (2006). The relative importance of olfaction and vision in a diurnal and a nocturnal hawkmoth. J. Comp. Physiol. A Neuroethol. Sens. Neural. Behav. Physiol. 192, 431–437. doi: 10.1007/s00359-005-0081-6
Bates, D., Maechler, M., Bolker, B., and Walker, S. (2014). lme4: Linear Mixed - Effects Models Using Eigen and s4. R package Version 1.1-6. Available online at: http://CRAN.R-project.org/package=lme4 (accessed June 22, 2021)
Boogert, N. J., Madden, J. R., Morand-Ferron, J., and Thornton, A. (2018). Measuring and understanding individual differences in cognition. Philos. Trans. R. Soc. B Biol. Sci. 373:20170280. doi: 10.1098/rstb.2017.0280
Bray, A., and Nieh, J. (2014). Non-consumptive predator effects shape honey bee foraging and recruitment dancing. PLoS One 9:e87459. doi: 10.1371/journal.pone.0087459
Burger, J. M. S., Kolss, M., Pont, J., and Kawecki, T. J. (2008). Learning ability and longevity: a symmetrical evolutionary trade-off in Drosophila. Evolution 62, 1294–1304. doi: 10.1111/j.1558-5646.2008.00376.x
Burnham, K. P., Anderson, D. R., and Huyvaert, K. P. (2011). AIC model selection and multimodel inference in behavioral ecology: some background, observations, and comparisons. Behav. Ecol. Sociobiol. 65, 23–35. doi: 10.1007/s00265-010-1029-6
Burns, J. G., Foucaud, J., and Mery, F. (2011). Costs of memory: lessons from ‘mini’ brains. Proc. Biol. Sci. 278, 923–929. doi: 10.1098/rspb.2010.2488
Cabirol, A., Cope, A. J., Barron, A. B., and Devaud, J. M. (2018). Relationship between brain plasticity, learning and foraging performance in honey bees. PLoS One 13:e0196749. doi: 10.1371/journal.pone.0196749
Chittka, L. (1998). Sensorimotor learning in bumblebees: long-term retention and reversal training. J. Exp. Biol. 201, 515–524. doi: 10.1242/jeb.201.4.515
Chittka, L., Dyer, A. G., Bock, F., and Dornhaus, A. (2003). Psychophysics – bees trade off foraging speed for accuracy. Nature 424, 388–388. doi: 10.1038/424388a
Chittka, L., and Raine, N. E. (2006). Recognition of flowers by pollinators. Curr. Opin. Plant Biol. 9, 428–435. doi: 10.1016/j.pbi.2006.05.002
De Agrò, M., Oberhauser, F. B., Loconsole, M., Galli, G., Dal Cin, F., Moretto, E., et al. (2020). Multi-modal cue integration in the black garden ant. Anim. Cogn. 23, 1119–1127. doi: 10.1007/s10071-020-01360-9
Di Pasquale, G., Salignon, M., Le Conte, Y., Belzunces, L. P., Decourtye, A., Kretzschmar, A., et al. (2013). Influence of pollen nutrition on honey bee health: do pollen quality and diversity matter? PLoS One 8:e72016. doi: 10.1371/journal.pone.0072016
Dornhaus, A., and Chittka, L. (2001). Food alert in bumblebees (Bombus terrestris): possible mechanisms and evolutionary implications. Behav. Ecol. Sociobiol. 50, 570–576. doi: 10.1007/s002650100395
Dukas, R. (1999). Costs of memory: ideas and predictions. J. Theor. Biol. 197, 41–50. doi: 10.1006/jtbi.1998.0856
Dukas, R. (2008). Evolutionary biology of insect learning. Annu. Rev. Entomol. 53, 145–160. doi: 10.1146/annurev.ento.53.103106.093343
Dukas, R., and Bernays, E. A. (2000). Learning improves growth rate in grasshoppers. Proc. Natl. Acad. Sci. U. S. A. 97, 2637–2640. doi: 10.1073/pnas.050461497
Dukas, R., and Duan, J. J. (2000). Potential fitness consequences of associative learning in a parasitoid wasp. Behav. Ecol. 11, 536–543. doi: 10.1093/beheco/11.5.536
Dunlap, A. S., and Stephens, D. W. (2016). Reliability, uncertainty, and costs in the evolution of animal learning. Curr. Opin. Behav. Sci. 12, 73–79. doi: 10.1016/j.cobeha.2016.09.010
Eliassen, S., Jorgensen, C., Mangel, M., and Giske, J. (2007). Exploration or exploitation: life expectancy changes the value of learning in foraging strategies. Oikos 116, 513–523. doi: 10.1111/j.2006.0030-1299.15462.x
Evans, L. J., and Raine, N. E. (2014). Foraging errors play a role in resource exploration by bumble bees (Bombus terrrestris). J. Comp. Physiol. A 200, 475–484. doi: 10.1007/s00359-014-0905-3
Evans, L. J., Raine, N. E., and Leadbeater, E. (2016). Reproductive environment affects learning performance in bumble bees. Behav. Ecol. Sociobiol. 70, 2053–2060. doi: 10.1007/s00265-016-2209-9
Evans, L. J., Smith, K. E., and Raine, N. E. (2017). Fast learning in free-foraging bumble bees is negatively correlated with lifetime resource collection. Sci. Rep. 7:496. doi: 10.1038/s41598-017-00389-0
Flanigan, K. A. S., Wiegmann, D. D., Hebets, E. A., and Bingman, V. P. (2021). Multisensory integration supports configural learning of a home refuge in the whip spider Phrynus marginemaculatus. J. Exp. Biol. 224:jeb238444. doi: 10.1242/jeb.238444
Foley, R. A., and Lee, P. C. (1991). Ecology and energetics of encephalization in hominid evolution. Philos. Trans. R. Soc. Lond. B Biol. Sci. 334, 223–232. doi: 10.1098/rstb.1991.0111
Foster, R. L., and Gamboa, G. J. (1989). Nest entrance marking with colony specific odors by the bumble bee Bombus occidentalis (Hymenoptera: Apidae). Ethology 81, 273–278. doi: 10.1111/j.1439-0310.1989.tb00773.x
Gill, R. J., and Raine, N. E. (2014). Chronic impairment of bumblebee natural foraging behaviour induced by sublethal pesticide exposure. Funct. Ecol. 28, 1459–1471. doi: 10.1111/1365-2435.12292
Gill, R. J., Ramos-Rodriguez, O., and Raine, N. E. (2012). Combined pesticide exposure severely affects individual- and colony-level traits in bees. Nature 491, 105–108. doi: 10.1038/nature11585
Giraudoux, P. (2014). pgirmess: Data Analysis in Ecology. R Package Version 1.5.9. Available online at: http://CRAN.R-project.org/package=pgirmess (accessed April 5,2021)
Giurfa, M., and Sandoz, J.-C. (2012). Invertebrate learning and memory: fifty years of olfactory conditioning of the proboscis extension response in honeybees. Learn. Mem. 19, 54–66. doi: 10.1101/lm.024711.111
Goulson, D., Hawson, S. A., and Stout, J. C. (1998). Foraging bumblebees avoid flowers already visited by conspecifics or by other bumblebee species. Anim. Behav. 55, 199–206. doi: 10.1006/anbe.1997.0570
Greenlees, M. J., Phillips, B. L., and Shine, R. (2010). Adjusting to a toxic invader: native Australian frogs learn not to prey on cane toads. Behav. Ecol. 21, 966–971. doi: 10.1093/beheco/arq095
Heinrich, B. (1976). The foraging specializations of individual bumblebees. Ecol. Monogr. 46, 105–128. doi: 10.2307/1942246
Hermer, E., Cauchoix, M., Chaine, A. S., and Morand-Ferron, J. (2018). Elevation-related differences in serial reversal learning ability in a nonscatter hoarding passerine. Behav. Ecol. 29, 840–847. doi: 10.1093/beheco/ary067
Huebner, F., Fichtel, C., and Kappeler, P. M. (2018). Linking cognition with fitness in a wild primate: fitness correlates of problem-solving performance and spatial learning ability. Philos. Trans. R. Soc. B 373:20170295. doi: 10.1098/rstb.2017.0295
Jaumann, S., Scudelari, R., and Naug, D. (2013). Energetic cost of learning and memory can cause cognitive impairment in honeybees. Biol. Lett. 9:20130149. doi: 10.1098/rsbl.2013.0149
Kaczorowski, R. L., Leonard, A. S., Dornhaus, A., and Papaj, D. R. (2012). Floral signal complexity as a possible adaptation to environmental variability: a test using nectar-foraging bumblebees. Bombus impatiens. Anim. Behav. 83, 905–913. doi: 10.1016/j.anbehav.2012.01.007
Kunze, J., and Gumbert, A. (2001). The combined effect of color and odor on flower choice behavior of bumble bees in flower mimicry systems. Behav. Ecol. 12, 447–456. doi: 10.1093/beheco/12.4.447
Laloi, D., Gallois, M., Roger, B., and Pham-Delègue, M.-H. (2001). Changes with age in olfactory conditioning performance of worker honey bees (Apis mellifera). Apidologie 32, 231–242. doi: 10.1051/apido:2001125
Laverty, T. M. (1980). The flower-visiting behaviour of bumble bees: floral complexity and learning. Can. J. Zool. 58, 1324–1335. doi: 10.1139/z80-184
Li, J., Wang, Z., Tan, K., Qu, Y., and Nieh, J. C. (2014). Giant Asian honeybees use olfactory eavesdropping to detect and avoid ant predators. Anim. Behav. 97, 69–76. doi: 10.1016/j.anbehav.2014.08.015
Lihoreau, M., Chittka, L., and Raine, N. E. (2010). Travel optimization by foraging bumblebees through readjustments of traplines after discovery of new feeding locations. Am. Nat. 176, 744–757. doi: 10.1086/657042
Lihoreau, M., Raine, N. E., Reynolds, A. M., Stelzer, R. J., Lim, K. S., Smith, A. D., et al. (2012). Radar tracking and motion-sensitive cameras on flowers reveal the development of pollinator multi-destination routes over large spatial scales. PLoS Biol. 10:e1001392. doi: 10.1371/journal.pbio.1001392
Lönnstedt, O. M., McCormick, M. I., Meekan, M. G., Ferrari, M. C. O., and Chivers, D. P. (2012). Learn and live: predator experience and feeding history determines prey behaviour and survival. Proc. Biol. Sci. 279, 2091–2098. doi: 10.1098/rspb.2011.2516
Maaswinkel, H., and Whishaw, I. Q. (1999). Homing with locale, taxon, and dead reckoning strategies by foraging rats: sensory hierarchy in spatial navigation. Behav. Brain Res. 99, 143–152. doi: 10.1016/s0166-4328(98)00100-4
Madden, J. R., Langley, E. J. G., Whiteside, M. A., Beardsworth, C. E., and van Horik, J. O. (2018). The quick are the dead: pheasants that are slow to reverse a learned association survive for longer in the wild. Philos. Trans. R. Soc. B 373:20170297. doi: 10.1098/rstb.2017.0297
Mallon, E. B., Brockmann, A., and Schmid-Hempel, P. (2003). Immune response inhibits associative learning in insects. Proc. Biol. Sci. 270, 2471–2473. doi: 10.1098/rspb.2003.2456
Manning, A. (1956). Some aspects of the foraging behaviour of bumble bees. Behaviour 9, 164–201. doi: 10.1163/156853956x00291
Menzel, R. (1993). Associative learning in honey bees. Apidologie 24, 157–168. doi: 10.1051/apido:19930301
Menzel, R., and Greggers, U. (1985). Natural phototaxis and its relationship to color-vision in honeybees. J. Comp. Physiol. A Sens. Neural. Behav. Physiol. 157, 311–321. doi: 10.1007/bf00618121
Mery, F., and Kawecki, T. J. (2003). A fitness cost of learning ability in Drosophila melanogaster. Proc. Biol. Sci. 270, 2465–2469. doi: 10.1098/rspb.2003.2548
Mery, F., and Kawecki, T. J. (2004). An operating cost of learning in Drosophila melanogaster. Anim. Behav. 68, 589–598. doi: 10.1016/j.anbehav.2003.12.005
Molet, M., Chittka, L., and Raine, N. E. (2009). How floral odours are learned inside the bumblebee (Bombus terrestris) nest. Naturwissenschaften 96, 213–219. doi: 10.1007/s00114-008-0465-x
Morand-Ferron, J. (2017). Why learn? The adaptive value of associative learning in wild populations. Curr. Opin. Behav. Sci. 16, 73–79. doi: 10.1016/j.cobeha.2017.03.008
Morand-Ferron, J., Hermer, E., Jones, T. B., and Thompson, M. J. (2019). Environmental variability, the value of information, and learning in winter residents. Anim. Behav. 147, 137–145. doi: 10.1016/j.anbehav.2018.09.008
Morand-Ferron, J., and Quinn, J. L. (2015). The evolution of cognition in natural populations. Trends Cogn. Sci. 19, 235–237. doi: 10.1016/j.tics.2015.03.005
Nowicki, S., Searcy, W. A., and Peters, S. (2002). Quality of song learning affects female response to male bird song. Proc. Biol. Sci. 269, 1949–1954. doi: 10.1098/rspb.2002.2124
Pearce, R. F., Giuggioli, L., and Rands, S. A. (2017). Bumblebees can discriminate between scent-marks deposited by conspecifics. Sci. Rep. 7:43872. doi: 10.1038/srep43872
Pinheiro, J., Bates, D., DebRoy, S., and Sarkar, D. R Core Team (2014). nlme: Linear and Nonlinear Mixed Effects Models_. R Package Version 3.1-117. Available online at: http://CRAN.R-project.org/package=nlme (accessed February4, 2021)
R Core Development Team. (2014). R: A Language and Environment for Statistical Computing. Vienna: R Foundation for Statistical Computing.
Raihani, N. J., and Bshary, R. (2012). A positive effect of flowers rather than eye images in a large-scale, cross-cultural dictator game. Proc. Biol. Sci. 279, 3556–3564. doi: 10.1098/rspb.2012.0758
Raine, N. E., and Chittka, L. (2007). The adaptive significance of sensory bias in a foraging context: floral colour preferences in the bumblebee Bombus terrestris. PLoS One 2:e556. doi: 10.1371/journal.pone.0000556
Raine, N. E., and Chittka, L. (2008). The correlation of learning speed and natural foraging success in bumble-bees. Proc. Biol. Sci. 275, 803–808. doi: 10.1098/rspb.2007.1652
Raine, N. E., Ings, T. C., Dornhaus, A., Saleh, N., and Chittka, L. (2006a). Adaptation, genetic drift, pleiotropy, and history in the evolution of bee foraging behavior. Adv. Study Behav. 36, 305–354. doi: 10.1016/s0065-3454(06)36007-x
Raine, N. E., Ings, T. C., Ramos-Rodriguez, O., and Chittka, L. (2006b). Intercolony variation in learning performance of a wild British bumblebee population (Hymenoptera: Apidae: Bombus terrestris audax). Entomol. Gen. 28, 241–256. doi: 10.1127/entom.gen/28/2006/241
Ray, S., and Ferneyhough, B. (1997). The effects of age on olfactory learning and memory in the honey bee Apis mellifera. Neuroreport 8, 789–793. doi: 10.1097/00001756-199702100-00042
Reader, T., Higginson, A. D., Barnard, C. J., and Gilbert, F. S. (2006). The effects of predation risk from crab spiders on bee foraging behavior. Behav. Ecol. 17, 933–939. doi: 10.1093/beheco/arl027
Riveros, A. J., and Gronenberg, W. (2009). Olfactory learning and memory in the bumblebee Bombus occidentalis. Naturwissenschaften 96, 851–856. doi: 10.1007/s00114-009-0532-y
Saleh, N., Ohashi, K., Thomson, J. D., and Chittka, L. (2006). Facultative use of the repellent scent mark in foraging bumblebees: complex versus simple flowers. Anim. Behav. 71, 847–854. doi: 10.1016/j.anbehav.2005.06.014
Saleh, N., Scott, A. G., Bryning, G. P., and Chittka, L. (2007). Distinguishing signals and cues: bumblebees use general footprints to generate adaptive behaviour at flowers and nest. Arthropod Plant Interact. 1, 119–127. doi: 10.1007/s11829-007-9011-6
Sato, A., Ozawa, N., and Karino, K. (2014). Variation in female guppy preference for male olfactory and visual traits. J. Ethol. 32, 137–143. doi: 10.1007/s10164-014-0402-8
Schmid-Hempel, R., and Schmid-Hempel, P. (1998). Colony performance and immunocompetence of a social insect, Bombus terrestris, in poor and variable environments. Funct. Ecol. 12, 22–30. doi: 10.1046/j.1365-2435.1998.00153.x
Sergio, F., Tanferna, A., De Stephanis, R., Jimenez, L. L., Blas, J., Tavecchia, G., et al. (2014). Individual improvements and selective mortality shape lifelong migratory performance. Nature 515, 410–413. doi: 10.1038/nature13696
Smith, C., Barber, I., Wootton, R. J., and Chittka, L. (2004). A receiver bias in the origin of three–spined stickleback mate choice. Proc. Biol. Sci. 271, 949–955. doi: 10.1098/rspb.2004.2690
Smith, K. E., and Raine, N. E. (2014). A comparison of visual and olfactory learning performance in the bumblebee Bombus terrestris. Behav. Ecol. Sociobiol. 68, 1549–1559. doi: 10.1007/s00265-014-1765-0
Snell-Rood, E. C., Davidowitz, G., and Papaj, D. R. (2011). Reproductive tradeoffs of learning in a butterfly. Behav. Ecol. 22, 291–302. doi: 10.1093/beheco/arq169
Spaethe, J., Brockmann, A., Halbig, C., and Tautz, J. (2007). Size determines antennal sensitivity and behavioral threshold to odors in bumblebee workers. Naturwissenschaften 94, 733–739. doi: 10.1007/s00114-007-0251-1
Spaethe, J., Tautz, J., and Chittka, L. (2001). Visual constraints in foraging bumblebees: flower size and color affect search time and flight behavior. Proc. Natl. Acad. Sci. U. S. A. 98, 3898–3903. doi: 10.1073/pnas.071053098
Stanley, D. A., Russell, A. L., Morrison, S. J., Rogers, C., and Raine, N. E. (2016). Investigating the impacts of field−realistic exposure to a neonicotinoid pesticide on bumblebee foraging, homing ability and colony growth. J. Appl. Ecol. 53, 1440–1449. doi: 10.1111/1365-2664.12689
Stephens, D. W. (1991). Change, regularity, and value in the evolution of animal learning. Behav. Ecol. 2, 77–89. doi: 10.1093/beheco/2.1.77
Stout, J. C., and Goulson, D. (2001). The use of conspecific and interspecific scent marks by foraging bumblebees and honeybees. Anim. Behav. 62, 183–189. doi: 10.1006/anbe.2001.1729
Takeda, K. (1961). Classical conditioned response in the honey bee. J. Insect Physiol. 6, 168–179. doi: 10.1016/0022-1910(61)90060-9
van den Berg, M., Duivenvoorde, L., Wang, G. H., Tribuhl, S., Bukovinszky, T., Vet, L. E. M., et al. (2011). Natural variation in learning and memory dynamics studied by artificial selection on learning rate in parasitic wasps. Anim. Behav. 81, 325–333. doi: 10.1016/j.anbehav.2010.11.002
Vaudo, A. D., Patch, H. M., Mortensen, D. A., Tooker, J. F., and Grozinger, C. M. (2016). Macronutrient ratios in pollen shape bumble bee (Bombus impatiens) foraging strategies and floral preferences. Proc. Natl. Acad. Sci. U. S. A. 113, E4035–E4042. doi: 10.1073/pnas.1606101113
Ward-Fear, G., Pearson, D. J., Brown, G. P., Rangers, B., and Shine, R. (2016). Ecological immunization: in situ training of free-ranging predatory lizards reduces their vulnerability to invasive toxic prey. Biol. Lett. 12:20150863. doi: 10.1098/rsbl.2015.0863
White, G. E., and Brown, C. (2014). A comparison of spatial learning and memory capabilities in intertidal gobies. Behav. Ecol. Sociobiol. 68, 1393–1401. doi: 10.1007/s00265-014-1747-2
Wright, G. A., and Schiestl, F. P. (2009). The evolution of floral scent: the influence of olfactory learning by insect pollinators on the honest signalling of floral rewards. Funct. Ecol. 23, 841–851. doi: 10.1111/j.1365-2435.2009.01627.x
Keywords: bumblebee behavior, cognitive ecology, olfaction, pollinator behaviour, resource collection, social insects
Citation: Evans LJ, Smith KE and Raine NE (2021) Odour Learning Bees Have Longer Foraging Careers Than Non-learners in a Natural Environment. Front. Ecol. Evol. 9:676289. doi: 10.3389/fevo.2021.676289
Received: 05 March 2021; Accepted: 25 June 2021;
Published: 27 July 2021.
Edited by:
Johannes Spaethe, Julius Maximilian University of Würzburg, GermanyReviewed by:
Fabio Santos Nascimento, University of São Paulo Ribeirão Preto, BrazilAmelie Cabirol, University of Trento, Italy
Copyright © 2021 Evans, Smith and Raine. This is an open-access article distributed under the terms of the Creative Commons Attribution License (CC BY). The use, distribution or reproduction in other forums is permitted, provided the original author(s) and the copyright owner(s) are credited and that the original publication in this journal is cited, in accordance with accepted academic practice. No use, distribution or reproduction is permitted which does not comply with these terms.
*Correspondence: Lisa J. Evans, bGlzYS5ldmFuc0BwbGFudGFuZGZvb2QuY28ubno=
†ORCID: Lisa J. Evans, orcid.org/0000-0001-7353-9750; Nigel E. Raine, orcid.org/0000-0001-6343-2829