- 1School of Biological Sciences, University of Utah, Salt Lake City, UT, United States
- 2California Botanic Garden, Claremont, CA, United States
- 3Department of Ecology, Evolution and Marine Biology, University of California, Santa Barbara, Santa Barbara, CA, United States
- 4Faculty of Sciences, Koç University, Istanbul, Turkey
The majority of the world’s biodiversity occurs in the tropics, but human actions in these regions have precipitated an extinction crisis due to habitat degradation, overexploitation, and climate change. Understanding which ecological, biogeographical, and life-history traits predict extinction risk is critical for conserving species. The Philippines is a hotspot of biodiversity and endemism, but it is a region that also suffers from an extremely high level of deforestation, habitat degradation, and wildlife exploitation. We investigated the biological correlates of extinction risk based on the IUCN Red List threat status among resident Philippine birds using a broad range of ecological, biogeographical, and life history traits previously identified as correlates of extinction risk in birds. We found strong support across competing models for endemism, narrower elevational ranges, high forest dependency, and larger body size as correlates significantly associated with extinction risk. Additionally, we compared observed threat status with threat status fitted by our model, finding fourteen species that are not currently recognized by the IUCN Red List as threatened that may be more threatened than currently believed and therefore warrant heightened conservation focus, and predicted threat statuses for the four Philippine Data Deficient bird species. We also assessed species described in recent taxonomic splits that are recognized by BirdLife International, finding 12 species that have a fitted threat status more severe than their IUCN-designated ones. Our findings provide a framework for avian conservation efforts to identify birds with specific biological correlates that increase a species’ vulnerability to extinction both in the Philippine Archipelago and elsewhere on other tropical islands.
Introduction
The majority of the world’s biodiversity is found in the tropics (Myers et al., 2000; Brown, 2014; Barlow et al., 2018), but human actions in these regions are expected to precipitate an extinction crisis as habitats are diminished and degraded (Pimm and Raven, 2000; Barlow et al., 2018). Extinction risk among taxa is phylogenetically non-random (e.g., Russell et al., 1998; Hughes, 1999; Von Euler, 2001; Yessoufou et al., 2012; Wang et al., 2018) and influenced by species’ biology, specifically ecological, biogeographical, and life history traits (hereafter “biological correlates”). Such ecological variables that impact extinction risk include poor dispersal ability (Weerd et al., 2003; Moore et al., 2008; Lees and Peres, 2009), ecological specialization (Norris and Harper, 2004; Şekercioğlu, 2007, 2011), and large body size (Gaston and Blackburn, 1995; Bennett and Owens, 1997; Wang et al., 2018). Biogeographical variables associated with high extinction risk include small geographical and elevational range size (Manne et al., 1999; Purvis et al., 2000; Şekercioğlu et al., 2008; White and Bennett, 2015), and endemism (Myers et al., 2000; Boyer, 2008). Life history and breeding biology variables associated with high extinction risk include nest type (Terborgh, 1974; Wilcove, 1985; Boyer, 2008) and slow life histories (Purvis et al., 2000; Webb et al., 2002; Tobias et al., 2013), including low fecundity (Bennett and Owens, 1997; Purvis et al., 2000; Kruger and Radford, 2008) which can impact a species’ vulnerability to extinction both via increased predation risk of open nests and slower population recovery from a severe reduction in numbers.
For birds, habitat specialists are most at risk from habitat loss and degradation (Sodhi et al., 2004), which reduces niche availability (Norris and Harper, 2004). Of habitat specialists, forest interior (Turner, 1996; O’Dea and Whittaker, 2007), understory, and ground-dwelling bird species are particularly sensitive to habitat changes (Lambert and Collar, 2002; Visco et al., 2015), forest fragmentation (Şekercioğlu, 2002; Şekercioğlu and Sodhi, 2007), and compression of trophic niche-widths (Edwards et al., 2013) that results from habitat degradation. Larger bird species are also at heightened risk due to having low population sizes, slower life histories, requiring larger home ranges, and tending to occupy higher trophic niches (Gaston and Blackburn, 1995). Widely distributed species, on the other hand, are usually able to exploit a wider range of habitats than those with narrow distributions and may thus be less prone to extinction (Manne et al., 1999). Forest fragmentation and deforestation can compound species’ poor dispersal abilities (Barlow et al., 2006; Şekercioğlu, 2007; Moore et al., 2008; Lees and Peres, 2009; Visco et al., 2015; Sheard et al., 2020) and push forest interior species upslope to more suitable habitat (Ocampo-Peñuela and Pimm, 2015), resulting in the extinction of small, isolated populations that are constrained by elevation (Kattan et al., 1994).
Most studies examining biological correlates of extinction risk in birds have only assessed single traits individually in models (e.g., Bennett and Owens, 1997; Norris and Harper, 2004; Şekercioğlu et al., 2004; Kruger and Radford, 2008). Yet, traits analyzed in isolation are limited in their power to predict a species’ vulnerability (Henle et al., 2004; Wang et al., 2015, 2018), making it more difficult to determine the relative importance of individual traits that may predispose species to extinction (Bennett and Owens, 1997). Furthermore, some studies have found strong synergistic interactions between natural abundance and habitat specificity, and between body size and hunting vulnerability (Wang et al., 2015, 2018), though these studies used a maximum of two traits in each model. Therefore, there is a need to analyze the combined effects of multiple traits on a species’ extinction proneness (Davies et al., 2004; Henle et al., 2004; Wang et al., 2015). Globally, the International Union for the Conservation of Nature (IUCN) Red List has become the standard for assessing extinction risk in species (Le Breton et al., 2019). IUCN Red List criteria consist of three main components: a species’ population decline rate, geographic range size, and population size (Mace et al., 2008; Le Breton et al., 2019; International Union for Conservation of Nature and Natural Resources, 2020).
Southeast Asia is predicted to lose over a third of its biodiversity over the next century due to elevated rates of deforestation (Brook et al., 2003; Sodhi et al., 2010), with the resident avifauna of the region being the most extinction-prone in the world after that of oceanic islands (Şekercioğlu et al., 2004). This is particularly true in the Philippines, where extreme habitat loss and other human actions threaten the nation’s highly endemic birdlife (Oliver and Heaney, 1996; Panopio and Pajaro, 2014). The Philippines is one of the most biodiverse countries in the world (Posa et al., 2008); a vast archipelago with diverse habitats which form a global hotspot of species diversity and endemism (Heaney, 1993; Oliver and Heaney, 1996; Stattersfield et al., 1998; Myers et al., 2000). Furthermore, the bird diversity recognized in the Philippines continues to increase with successive taxonomic revisions. The country now possesses some of the highest richness of recently split threatened species in the world (Simkins et al., 2019). Furthermore, recent genetic and phenotypic analyses have indicated that alpha taxonomy may underestimate the quantity of bird species in the Philippines by at least 50% as a result of numerous cryptic endemic species in the country (Lohman et al., 2010; Campbell et al., 2016). Bird species limits in the Philippines are complicated by the country’s large number of islands and mountains that have driven allopatric diversification (Collar, 2011; Rasmussen et al., 2012; Neate-Clegg et al., 2021).
With 594 species, the Philippines is ranked 58th in the world for bird species richness (BirdLife International, 2021). However, 93 (15.6%) Philippine bird species are threatened with extinction (BirdLife International, 2021), ranking the country 8th for globally threatened species (Vulnerable, Endangered, or Critically Endangered; Figure 1). For endemic species, the outlook is even more dire, with 75 of the 258 (29.1%) endemic species considered threatened. However, there has been minimal research assessing the extinction proneness of Philippine avifauna or even Southeast Asian avifauna as a whole (Sodhi and Brook, 2006). At the current rate of habitat destruction and biodiversity loss within the Philippines, we may lose a large amount of diversity before many cryptic species are even described (Lohman et al., 2010).
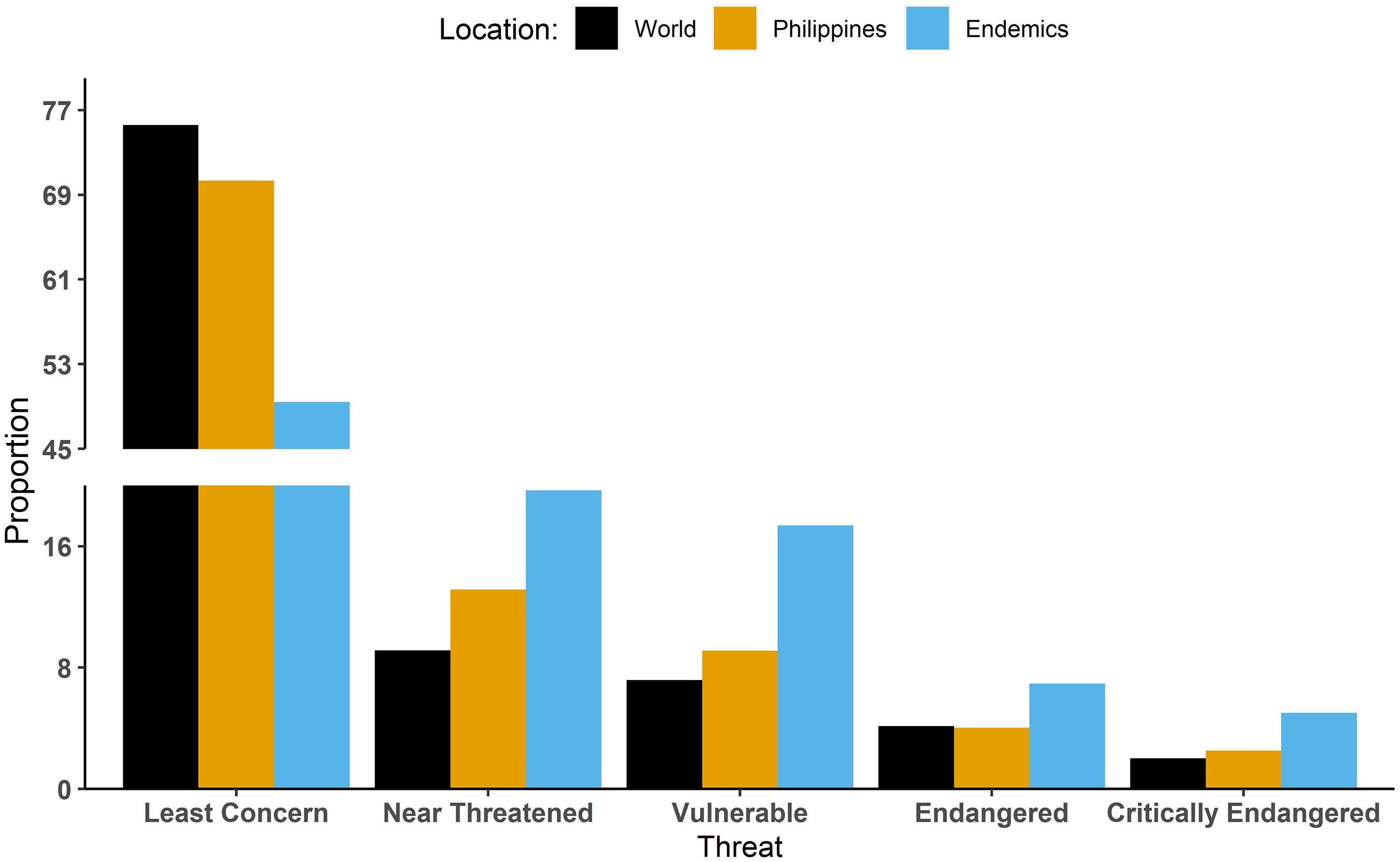
Figure 1. Percentage of bird species in the five main IUCN Red List threat categories in the world compared to all resident birds and endemic species in the Philippines. Percentages were calculated by taking the total number of bird species in each category and dividing by the total number of birds in the world, residents in the Philippines, and Philippine endemics.
In this study, we examined the biological correlates of extinction risk among all resident Philippine bird species. As a natural archipelago of diverse topography and ecosystems, a global biodiversity hotspot rich in endemism, and a nation facing numerous threats that are driving rapid wildlife population declines, the Philippines is a high priority country to assess the relationships between ecological, biogeographical, and life history traits and extinction risk in birds. We analyzed a broad range of traits that have previously been linked to extinction risk, comparing the effects of multiple traits simultaneously. We predicted that species at greater risk of extinction in the Philippines would be endemic (Myers et al., 2000; Boyer, 2008), restricted to lower elevations or to limited elevational ranges (Manne et al., 1999; Şekercioğlu et al., 2008; White and Bennett, 2015), large-bodied (Bennett and Owens, 1997; Boyer, 2008; Wang et al., 2018), have limited dispersal ability (Moore et al., 2008; Lees and Peres, 2009; Sheard et al., 2020), be ecologically specialized (Weerd et al., 2003; Norris and Harper, 2004; Şekercioğlu, 2011), have exposed nests or nest on the ground (Terborgh, 1974; Wilcove, 1985; Boyer, 2008), or have low fecundity (Bennett and Owens, 1997; Kruger and Radford, 2008). While we are assessing all of the resident birdlife in the Philippines, we especially focused on species that have either been split within the last decade or have been proposed to consist of cryptic populations that may warrant species-level status (Lohman et al., 2010; Collar, 2011; Rasmussen et al., 2012; Hosner et al., 2013; Campbell et al., 2016). We also aim to identify Philippine bird species which are not currently recognized as threatened by the IUCN but may be at risk of extinction in the near future.
Materials and Methods
Dataset and Biological Correlates
We compiled a dataset of the 446 resident Philippine bird species (Supplementary Table 1) after excluding 148 species that are fully migratory and do not breed on any of the islands in the Philippines. Taxonomic classifications were based on BirdLife International (2021), which maintains its own list of the world’s bird species, reviewed and adopted by the BirdLife Taxonomic Working Group (BirdLife International, 2021) and utilized by the IUCN Red List (2020). IUCN Red List threat status for each species consists of Least Concern (LC), Near Threatened (NT), Vulnerable (VU), Endangered (EN), Critically Endangered (CR), or Data Deficient (DD). We then collated data on 11 ecological, biogeographical, and life-history traits: endemism, elevational range, average elevation, body mass, hand-wing index, habitat breadth, forest dependency, primary diet, dietary breadth, nest type, and average clutch size. While there is a strong relationship between geographic range size and extinction risk (Figure 2), we did not include geographic range size in our analyses to avoid potential circularity with a species’ IUCN threat status. Most of the data were extracted from a global dataset of avian ecological traits (Table 1; see Şekercioğlu et al., 2004, 2019 for more details of these methods and a description of this dataset) which compiled information from the literature, including ornithological books (e.g., Del Hoyo et al., 1992-2013; Kennedy et al., 2000), BirdLife International (2021), and field guides (e.g., Robson, 2000). Hand-wing index (HWI) data were taken from Sheard et al. (2020). HWI is a measure of a bird wing’s pointiness and is a proxy for dispersal ability (Sheard et al., 2020), and this dataset was also used to fill gaps in body mass. If no additional data were available, we used conspecific or congeneric values where possible to fill in gaps.
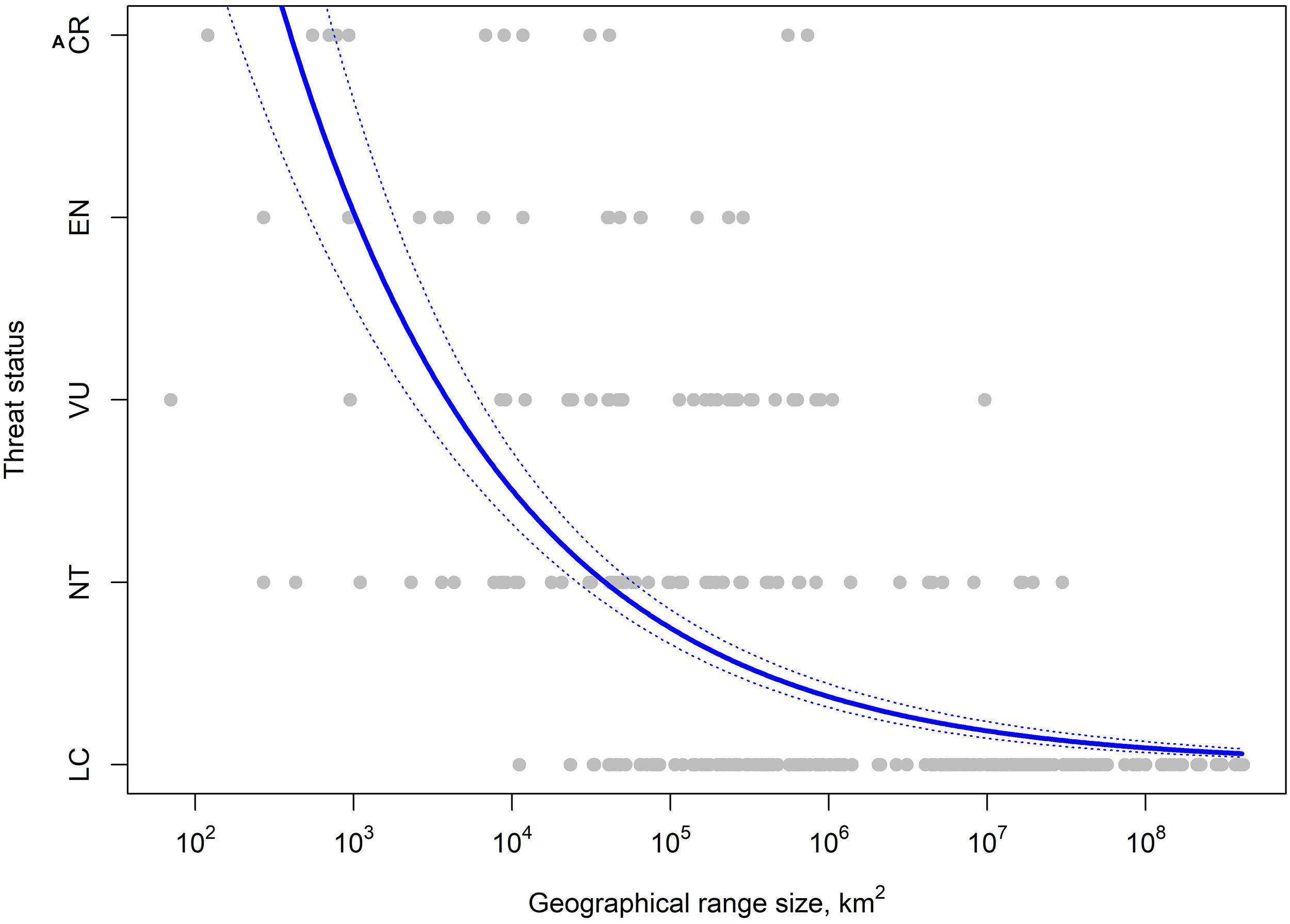
Figure 2. The correlation between threat status and geographic range size in resident Philippine birdlife.
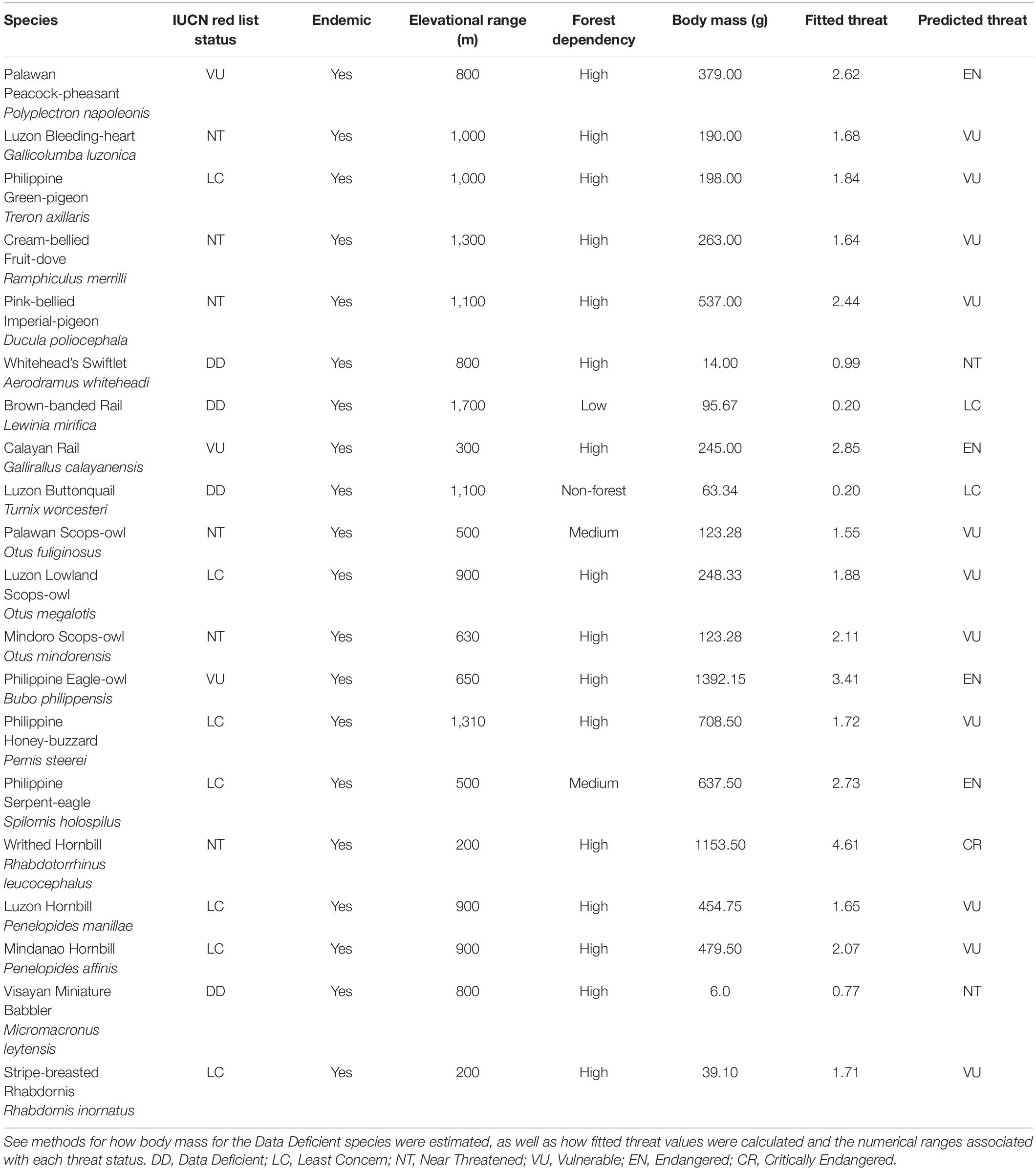
Table 1. Resident Philippine bird species not currently listed as threatened by the IUCN Red List (i.e., Least Concern or Near Threatened) which possess traits identified as correlates of extinction risk with strong support across all competing models (endemism, elevational range, forest dependency, and body mass) and have a predicted threat status that would often result in the species being classified as threatened under the IUCN Red List. Three threatened species with higher predicted threats than their IUCN designated ones, as well as the four Data Deficient species are also included in the table.
We used Kennedy et al. (2000) for each species’ elevational range in meters above sea level (hereafter “m asl”; Del Hoyo et al., 1992-2013) rather than generalizing lowland, foothill, or montane designations based on elevational ranges, as there are hundreds of islands in the Philippines and elevational ranges can vary greatly between islands and mountains (Rahbek et al., 2019). Since 71% of the species in our study are forest species, we used forest dependency (“high,” “medium,” “low,” “non-forest”; BirdLife International, 2021) as an indicator of a species’ habitat preference. We used primary diet as an indicator of a species’ trophic level (Wang et al., 2018). However, because invertivores and vertivores tend to have different sensitivity to anthropogenic change than herbivores (Şekercioğlu et al., 2002; Bregman et al., 2016; Keinath et al., 2017; Atwood et al., 2020; Sherry et al., 2020), we designated species as either “carnivores” or “herbivores.” We grouped frugivores, nectarivores, and granivores as herbivores, and grouped piscivores, invertivores, and vertivores as carnivores. For the 14 omnivorous species, we used context to specify if a species was primarily an herbivore or carnivore. We used dietary breadth and habitat breadth to calculate the ecological specialization index (Şekercioğlu, 2011). Dietary breadth is a count of the number of food types a species consumes, with categories such as invertebrates and fruit (max = 7). Habitat breadth is a count of the number of major habitats that a species can occur in, with broad categories such as forest, woodland, and grassland (max = 10). Specialization is then quantified as log10[100/(dietary breadth × habitat breadth)], with a maximum of 2 for species that eat only one type of food and live in one habitat (Şekercioğlu, 2011).
For traits related to a species’ breeding biology, we used clutch size as a proxy for fecundity (Bennett and Owens, 1997; Wang et al., 2015). We also simplified our nest type categories into nests that are either open (i.e., cup, platform, scrape, or saucer nests) or closed (i.e., burrows, cavity, dome, pendant, sphere, or mound nests). Our final set of variables included ten ecological traits: endemism (yes/no), elevational range, average elevation, body mass, HWI, forest dependency, trophic level (carnivore/herbivore), ecological specialization, nest type (open/closed), and average clutch size.
Four species were listed by International Union for Conservation of Nature and Natural Resources (2020) as DD and lacked some trait data: Whitehead’s Swiftlet (Aerodramus whiteheadi), Brown-banded Rail (Lewinia mirifica), Luzon Buttonquail (Turnix worcesteri), and Visayan Miniature Babbler (Micromacronus leytensis). Where possible, we filled in trait gaps for these four species with data from congeners. For body mass, we averaged the masses of all congeners.
To examine the threat status of recently split species, we identified the species in the literature that have been associated with taxonomic updates and proposed revisions within the last decade (Supplementary Table 2). This includes the 35 species recognized by BirdLife International (2021) that have recently been described as a result of species splits (Lohman et al., 2010; Collar, 2011; Rasmussen et al., 2012; Hosner et al., 2013; Campbell et al., 2016; Arndt et al., 2019) and, if applicable, the endemic Philippine parent species from which these birds were split (Supplementary Table 2). We also included the 19 species that consist of one or more cryptic populations that have been recommended to be split but have yet to be split and recognized by BirdLife International (2021) as separate species (Supplementary Table 2; Lohman et al., 2010; Collar, 2011; Campbell et al., 2016).
Statistical Analyses
We created a series of generalized linear mixed-effects models (GLMMs) using the “glmer” function from the R package lme4 (Bates et al., 2015) to test the relationships between IUCN threat status and our ten traits (Kruger and Radford, 2008). We excluded the four DD species, and then converted threat status into a continuous linear index from 0 to 4 (0 = LC, 1 = NT, 2 = VU, 3 = EN, 4 = CR), which served as our response variable. This numerical treatment for threat status and use of IUCN categories as surrogate measurements of extinction risk follows that of prior studies assessing predisposition to extinction in biodiversity (Purvis et al., 2000; Cardillo et al., 2004), including a recent examination of the correlates of extinction risk in birds (Wang et al., 2018). We also log-transformed body mass, since it increases geometrically, and clutch size, since this variable consists of count data; these two variables were approximately normally distributed following transformation. We then scaled all of our numerical variables to have a mean of 0 and a standard deviation of 1.
We then constructed a GLMM with a Poisson error structure. We used a Poisson GLMM because threat status was not normally distributed, consisted of integer values, and was zero-bound and zero-inflated. To control for phylogeny, we included bird genus nested within family as random intercept effects in our models. In our general model, we included all ten biological correlates, excluding any species which had gaps in data for forest dependency, nest type, or clutch size. Removing these birds with gaps, we therefore had sufficient data for 371 species to run in this model. However, due to the large number of species for which we lacked information on clutch size, we created another model for this dataset that excluded clutch size, to test the significance of this variable. We compared these two models using a likelihood ratio test and, since clutch size was not significant (χ2 = 0.482, p = 0.487), we removed it from the general model. We then created a second general GLMM with an expanded dataset that included species with no clutch size data (n = 421). We investigated variance inflation factors for the nine variables in our expanded GLMM using the function “vif” from the R package car (Fox and Weisberg, 2019), and all were below 3 (Zuur et al., 2010).
Next, we used the function “dredge” from the R package MuMIn (Bartoń, 2020) on the general model to run models for every possible subset of variables (512 models), to rank these models based on AICc, and to provide model weights for each model (Burnham and Anderson, 2002). We then subsetted these possible models to those with ΔAICc < 6 (Harrison et al., 2018). We considered the model with the lowest AICc to be the model best supported by the data. We used a likelihood ratio test to determine significance for any categorical variables with more than two categories.
We compared observed threat status with predicted (fitted) threat status from the model for two groups of species to evaluate whether some species may be more threatened than currently designated. We first assessed LC and NT species and determined whether the fitted threat status exceeded the observed threat status. For our thresholds for fitted threat status, we chose a range between 0.5 and 1.5 to indicate a species was NT, 1.5–2.5 for VU, 2.5–3.5 for EN, and 3.5 and higher for CR; a range of 0.0–0.5 indicated LC. We also used the same approach to extract and assess fitted threat values for 35 species (and their parent species, if applicable) recognized by BirdLife International (2021) that were recently split (Lohman et al., 2010; Collar, 2011; Rasmussen et al., 2012; Hosner et al., 2013; Campbell et al., 2016; Arndt et al., 2019), as well as the 19 species which consist of populations of one or more cryptic species (Lohman et al., 2010; Collar, 2011; Campbell et al., 2016) that have not yet been recognized by BirdLife International (2021). Finally, we used our model, which had excluded DD species, to predict the threat status of the four DD species.
All statistical analyses and graphing were conducted in R (version 4.0.2, 2020-06-22; R Core Team, 2020).
Results
Our general model contained 421 species. Following multi-model comparison, we found 29 competing models within ΔAICc <6. The top-ranked model contained four covariates that had a significant effect on threat status: endemism, elevational range, forest dependency, and body mass. These covariates were present in all 29 competing models. Specialization (0.173 ± 0.097, z = 1.783, p = 0.075) was also included in our top-ranked model, but it was non-significant.
Endemism was significantly associated with threat status in our top-ranked model (Figure 3B; 1.560 ± 0.276 se, z = 5.656, p < 0.001), with endemic species being more threatened with extinction than non-endemic residents (average threat: endemic = 1.00, non-endemic = 0.11). Elevational range was significantly associated with threat status in our top-ranked model (Figure 3A; −0.541 ± 0.097 se, z = −5.603, p < 0.001), with species that have narrower elevational ranges being more threatened than those that occur at broader elevational ranges. Forest dependency was significantly associated with threat status in our top-ranked model (Figure 3A; χ2 = 16.361, p = 0.001), such that species that have high forest dependency are more threatened than species with lower forest dependency (average threat: high = 1.20, medium = 0.50, low = 0.10, non-forest = 0.06). Finally, body mass was significantly associated with threat status in our top-ranked model (Figure 3B; 0.451 ± 0.069, z = 6.550, p < 0.001), with larger-bodied species more threatened than smaller-bodied birds.
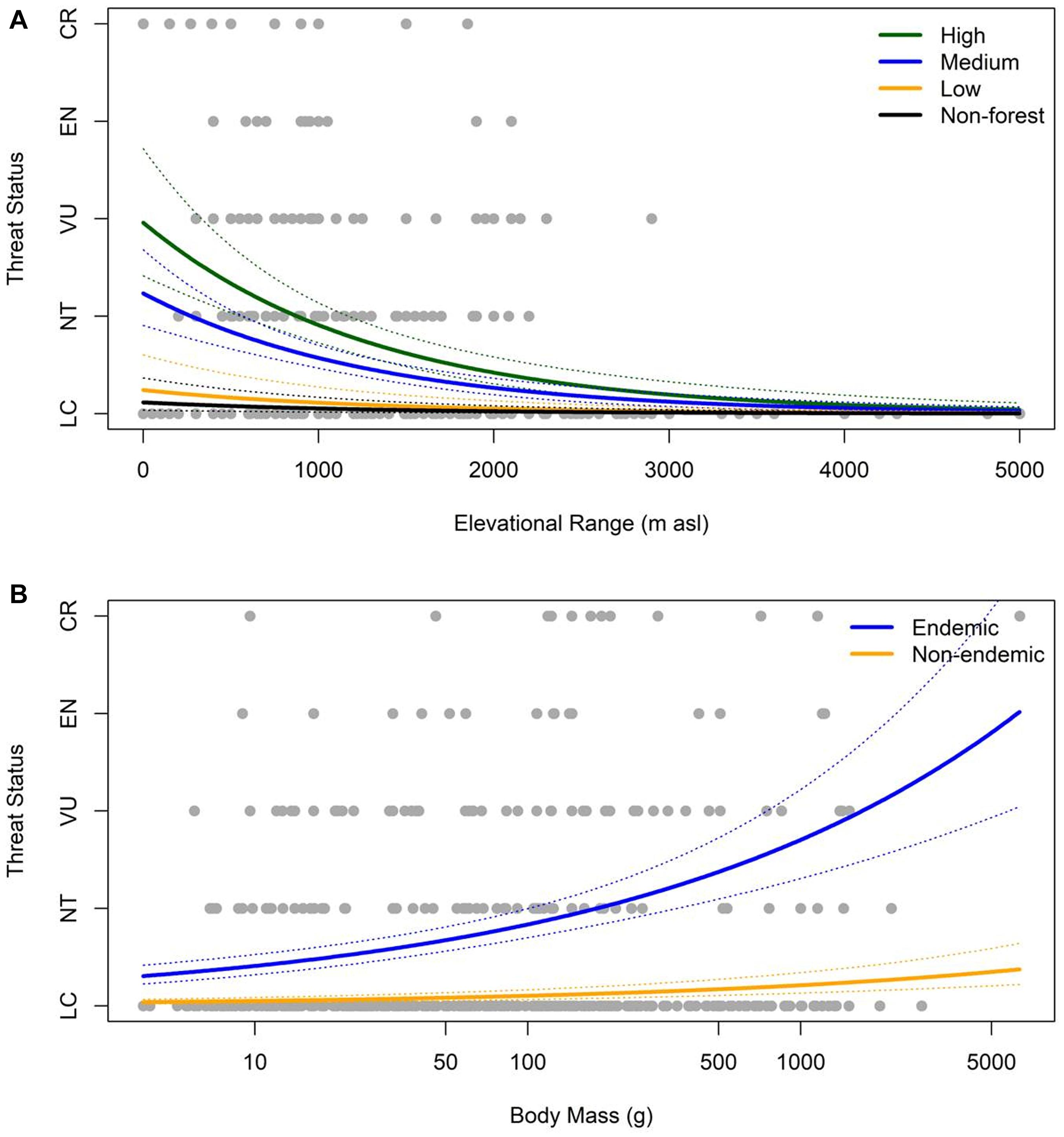
Figure 3. The correlation between threat status and (A) a species’ elevational range size and forest dependency, and (B) a species’ endemicity and body mass in resident Philippine birdlife. We hold the other variables from our top model to be equal to their mean values (0). All four variables were significantly correlated with threat status: endemism (1.560 ± 0.276, z = 5.656, p < 0.001), elevational range (–0.541 ± 0.097, z = –5.603, p < 0.001), forest dependency (χ2 = 16.361, p = 0.001), and body mass (0.451 ± 0.069, z = 6.550, p < 0.001).
We found 84 species of resident Philippine birds not listed as threatened by the International Union for Conservation of Nature and Natural Resources (2020) that had fitted numerical threats more severe than their currently designated ones, including 71 LC species that would classify as NT. Of these 84 species, fourteen had a predicted threat status that would result in the species being classified as threatened under the IUCN Red List (Table 1). In particular, Philippine Serpent-eagle (Spilornis holospilus) would classify as EN, while Writhed Hornbill (Rhabdotorrhinus leucocephalus) would classify as CR (Table 1). There were also three threatened species, all currently designated as VU, which would classify as EN (Table 1): Palawan Peacock-pheasant (Polyplectron napoleonis), Calayan Rail (Gallirallus calayanensis), and Philippine Eagle-owl (Bubo philippensis). Finally, for the four DD species, we predicted a fitted threat of LC for Brown-banded Rail and Luzon Buttonquail, and NT for Whitehead’s Swiftlet and Visayan Miniature Babbler (Table 1).
Additionally, we found 12 species, which are all products of recent splits (Collar, 2011; Rasmussen et al., 2012; Hosner et al., 2013; Campbell et al., 2016) and are recognized by BirdLife International (2021) that have predicted threat statuses more severe than their current IUCN-designated statuses (Table 2). Similarly, we found two species, Red-crested Malkoha (Dasylophus superciliosus) and Buff-spotted Flameback (Chrysocolaptes lucidus), each consisting of one or more cryptic populations with high phenotypic divergence (Campbell et al., 2016), that had a higher predicted threat status than their IUCN-designated one (Table 2). Eleven parent species from which one or more species were recently split also had a more severe predicted threat status than their IUCN ones (Supplementary Table 2).
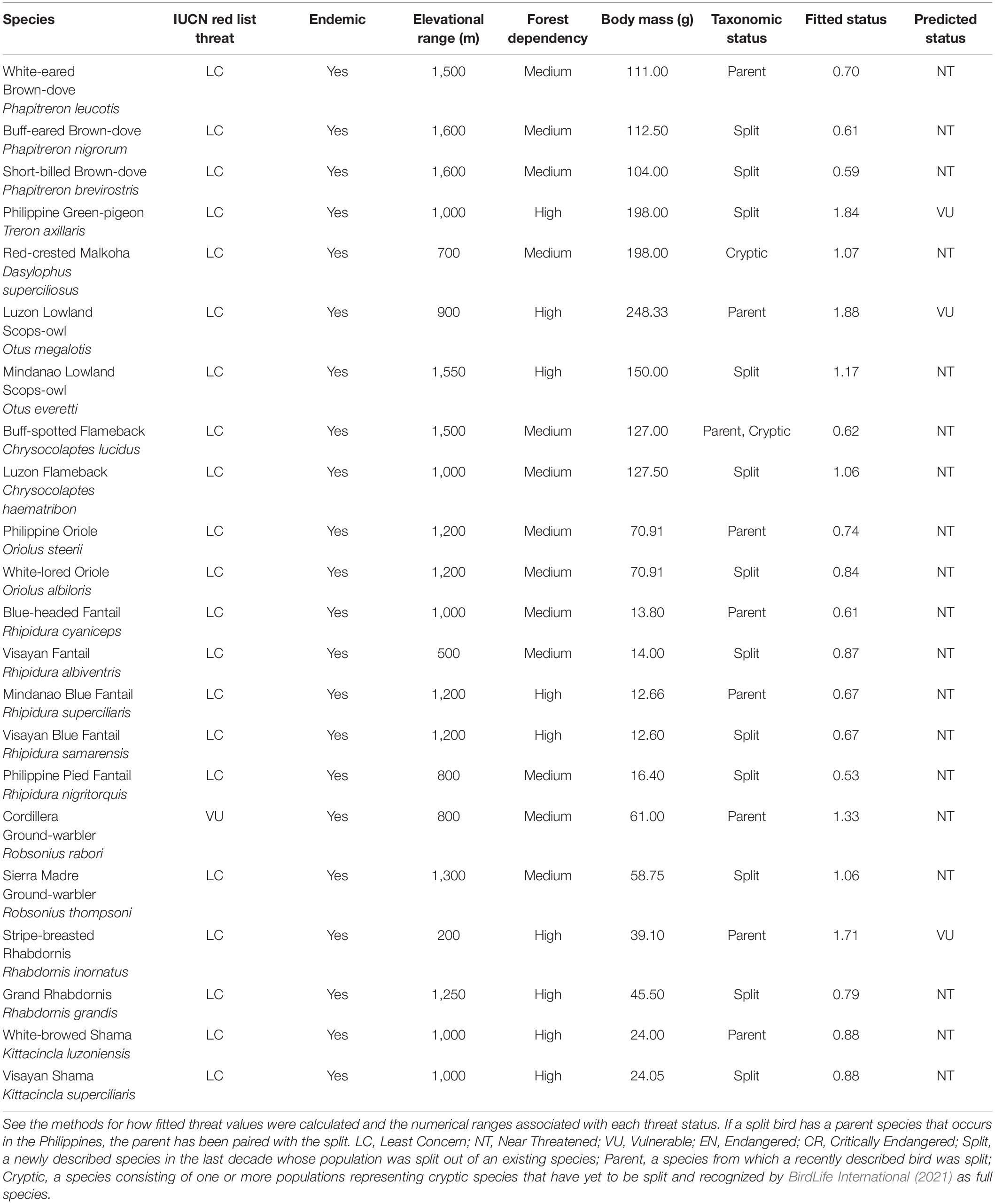
Table 2. Endemic Philippine bird species, described within the last decade as a result of a taxonomic split and recognized by BirdLife International (2021) or species that consist of one or more cryptic populations, which possess traits identified as correlates of extinction risk with strong support across all competing models (endemism, elevational range, forest dependency, and body mass) and have a predicted threat status that is numerically higher than their current IUCN designated one.
Discussion
In biodiverse regions threatened with anthropogenic change, it is critical to assess the predictors of extinction risk. In this study, we conducted the first comprehensive investigation into the biological correlates of extinction risk in the Philippine avifauna. For resident Philippine birds, we found strong support across competing models for endemic, elevationally-restricted, highly forest dependent, and larger-bodied species being predisposed to extinction (Figure 3).
Endemic species tend to be well-adapted to their local environments, but become rare when rapid changes create new habitats (Jones et al., 2001) or forest fragments (Weerd et al., 2003) to which they often cannot adapt. Endemic species also tend to have smaller geographic ranges than co-occurring resident, non-endemic species. While a number of Philippine endemics have large ranges across the archipelago and are not listed as globally threatened, geographic range size is one of the main criteria used by the IUCN to assess a species’ extinction risk (Mace et al., 2008; Le Breton et al., 2019; International Union for Conservation of Nature and Natural Resources, 2020). Consequently, many Philippine endemic birds are restricted to a single island such as Palawan or Luzon. We also found that birds which occur at narrower elevational ranges are at a heightened risk of extinction, consistent with prior studies (Şekercioğlu et al., 2008; White and Bennett, 2015). This may be amplified by the fact that eighty-seven percent of Philippine bird species occur in lowland areas below 500 m asl (Supplementary Table 1), with many species with narrow elevational ranges occurring partially or entirely across lower elevations. Deforestation for timber and crop cultivation (Kummer, 1992; Weerd et al., 2003; Panopio and Pajaro, 2014) has reduced the extent of lowland forest in the Philippines by over 90% (Ong et al., 2002; Tanalgo et al., 2015). Between 2002 and 2020, the Philippines lost 151kha of humid primary forest (Turubanova et al., 2018). This level of habitat destruction may especially impact species that have smaller elevational ranges and are thus constrained by elevation (Kattan et al., 1994). Extensive habitat destruction also greatly reduces niche availability, and species with narrow ecological niches are less adaptable to the changes brought about by habitat loss and degradation (McKinney, 1997; Kruger and Radford, 2008; Edwards et al., 2013). We likewise found that high forest dependency was a correlate of extinction risk in Philippine birds.
We found that larger-bodied birds are at increased risk of extinction, consistent with previous studies (e.g., Gaston and Blackburn,1995; Bennett and Owens, 1997; Wang et al., 2018). Larger species may recover more slowly from reductions in population size as a result of slower life histories (Gaston and Blackburn, 1995; Bennett and Owens, 1997), particularly in insular species (Boyer, 2008). Likewise, larger body size may limit population density and therefore a species’ total population size on islands (Boyer, 2008). Since population size is one of the main criteria used by the IUCN to assess a species’ extinction risk (Mace et al., 2008; Le Breton et al., 2019; International Union for Conservation of Nature and Natural Resources, 2020), both of these effects of larger body size would be important for predisposition to extinction. Additionally, larger species are often targeted for hunting and the pet trade, such as Visayan Hornbill (Penelopides panini), Philippine Cockatoo (Cacatua haematuropygia), Blue-naped Parrot (Tanygnathus lucionensis), and Philippine Serpent-eagle (Asian Development Bank, 2018) are thus more susceptible to overexploitation than are smaller species (Beissinger, 2000; Peres and Palacios, 2007; Wang et al., 2018). For example, three large pigeon species disappeared in the early twentieth century from Sibuyan Island in the Philippines, species that were likely locally extirpated despite their broad elevational ranges (Goodman et al., 1995). Intense hunting pressure coupled with the destruction of lowland habitat could have played a large part in the disappearances of these three pigeons (Goodman et al., 1995).
When examining predicted threat status, we found fourteen Philippine endemics not currently listed as threatened by the IUCN Red List (2020) that have predicted threat statuses that would qualify these birds as globally threatened according to our model (Table 1). We also found three threatened species that had higher predicted threats than their current designated ones. These fifteen species may be more threatened with extinction than currently believed based on their ecological correlates of extinction risk, and so should receive heightened conservation attention. Of particular note, we predicted that the LC Philippine Serpent-eagle and NT Writhed Hornbill are, respectively, EN and CR. Both birds are recognized as undergoing population declines, though Philippine Serpent-eagle is not classified as threatened since it occurs over a large range whereas it is noted that Writhed Hornbill is a poorly known species that should be carefully monitored (BirdLife International, 2021). These two species should therefore become bird conservation priorities in the Philippines.
Additionally, there are currently four DD birds in the Philippines, all of which are poorly known, with only a handful of records each within the last couple decades. Using our fitted model, we predicted that two of these birds would have a status of LC and the other two would have a status of NT (Table 1). However, there are some caveats with these findings. While we used congeners of these four species to help fill in information gaps for certain key traits, having more accurate trait information will allow for better predictions of threat status. We also know that IUCN threat status takes into account geographic range size and population size (Mace et al., 2008; Le Breton et al., 2019; International Union for Conservation of Nature and Natural Resources, 2020), which are both likely to be very small for these species. Likewise, elevational ranges used for these species may not be truly reflective for these birds with little-known distributions. Thus, these species are likely to be more threatened than estimated by our models. Future surveys should aim for a better understanding of the distributional limits and biological traits of these DD species in order to better predict and assess their threat status.
The Philippine avifauna has also undergone much taxonomic revision in recent years. In 2000, the number of endemic birds in the Philippines was 172 species (Kennedy et al., 2000), whereas today there are 258 endemic species (BirdLife International, 2021). Much of this change has occurred within the past decade, as birds have been split from extant recognized species (Lohman et al., 2010; Collar, 2011; Rasmussen et al., 2012; Hosner et al., 2013; Campbell et al., 2016; Arndt et al., 2019). We found 12 recently described splits (Table 2) and 11 parent species (Supplementary Table 2) which are likely to have worse threat statuses than their IUCN-designated ones. It is also predicted that the Philippines has a high number of cryptic species that have yet to be formally recognized (Lohman et al., 2010; Campbell et al., 2016), with recent proposals for some cryptic populations being elevated to species (Supplementary Table 2; Lohman et al., 2010; Collar, 2011; Campbell et al., 2016). We found two species with proposed cryptic splits that had higher predicted threat statuses than their IUCN-designated ones (Table 2). As bird species are split, newly split populations will have both a smaller number of individuals and more restricted geographic distributions (Robuchon et al., 2019) than the original parent species. For example, the Philippine clade of the LC parrot species Tanygnathus sumatranus was recently split (Arndt et al., 2019), resulting in the now endemic Blue-backed Parrot (Tanygnathus everetti) being designated as EN by the IUCN Red List (2020). We can therefore expect the number of threatened birds in the Philippines to increase in the future with new taxonomic arrangements.
Between 1988 and 2012, the number of threatened endemic birds in the Philippines increased from 34 (Collar and Andrew, 1988) to 84 species (Panopio and Pajaro, 2014). Today, 93 (36%) endemic species are threatened with extinction in the Philippine Archipelago (BirdLife International, 2021), a 10% increase in the number of endemic avifauna facing extinction in these islands in just under a decade. The families with the highest number of threatened birds in the Philippines are Columbidae, Muscicapidae, Strigidae, and Bucerotidae, with the latter having the highest proportion of threatened species (Figure 4). Approximately 71% of Philippine birds in our study are forest species, so the numbers of threatened birds can be expected to increase if deforestation and degradation of habitats continue at a rate similar to or greater than the recent trends of destruction.
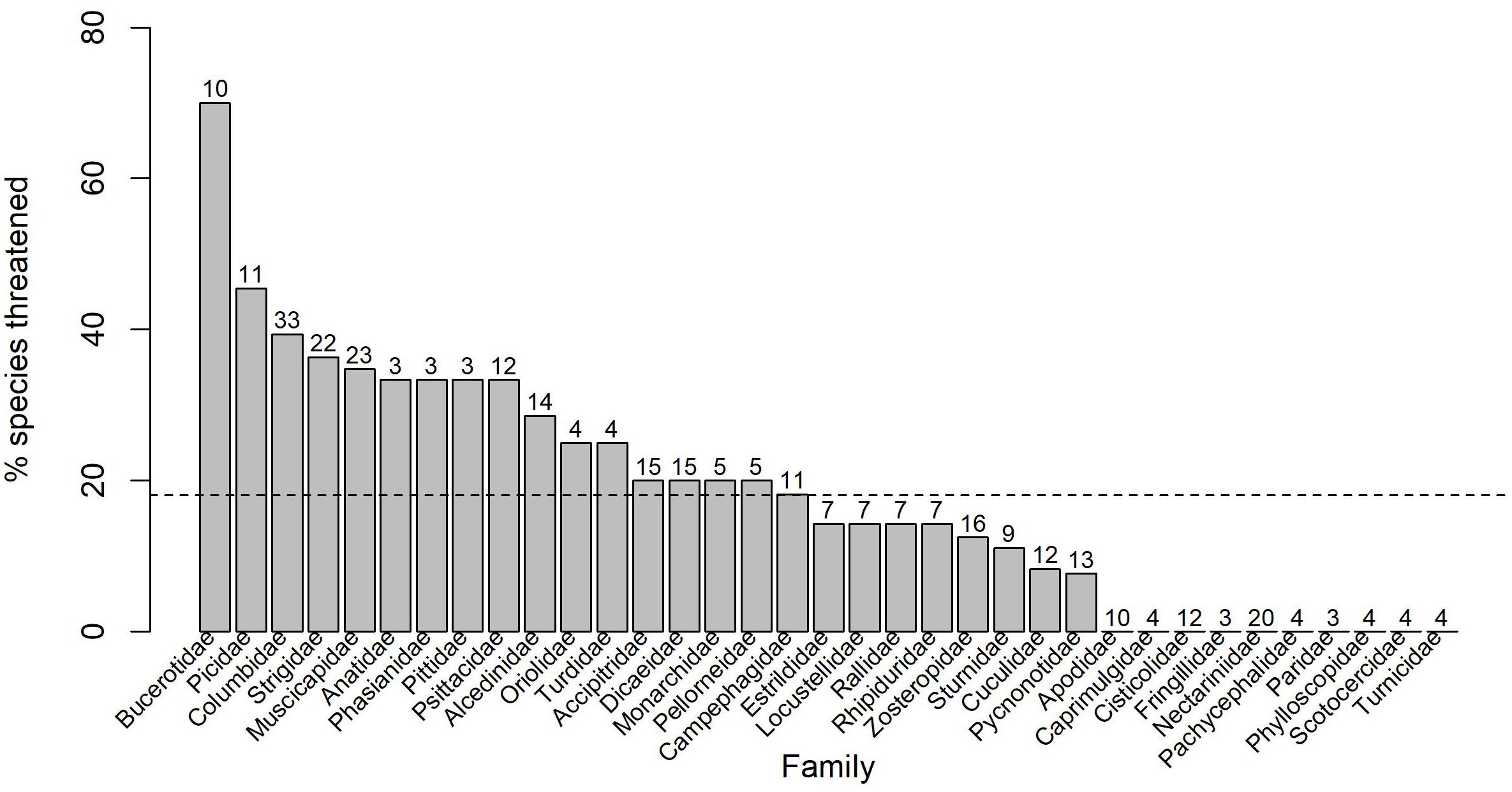
Figure 4. The percentage of species threatened with extinction (IUCN Red List statuses of Vulnerable, Endangered, or Critically Endangered) in each family of Philippine birds. Only families with at least three resident Philippine species are shown. The number of species in each family is shown above each bar. The dashed line represents the mean proportion of threatened birds across these families.
Beyond the Philippines, Southeast Asia contains thousands of islands rich in diversity and endemism, and anthropogenic threats such as habitat degradation and exploitation are severe. In the Philippines, there are 258 endemic species (43% of resident avifauna) and 141 species (24%) with high forest dependence. Across Southeast Asia and Papua New Guinea, this number is 880 (34% of resident avifauna) and 852 species (33%) with high forest dependence. The median elevational range among Philippine avifauna is 1,100 m, and there are 222 species (37%) with a range of 1,100 or less meters. Likewise, there are 503 species (19%) across the broader region with a range of 1,100 or narrower. The median body mass of Philippine birds is also 53 g, and there are 223 species (38%) in the archipelago that weigh this much or more. Likewise, of the birds across the broader region for which there is available information on weight (65% of species), there are441 species (26%) that have a large body size. Our findings thus have important conservation implications for birdlife on islands throughout Southeast Asia, Oceania, and the rest of the tropics. As human activities continue to cause global declines and losses in avian biodiversity, assessing and predicting extinction risk will be even more important as a pre-emptive conservation strategy.
Conclusion
In this study, we identify from a broad range of ecological, biogeographical, and life history traits the most important biological correlates of extinction risk in resident Philippine birdlife. Many species are typically affected by more than one type of threat (Davies et al., 2004; Henle et al., 2004; Wang et al., 2015, 2018), and our study is one of the few that collectively analyzes the effects of multiple traits in birds as potential correlates of extinction risk. Endemics, species with narrower elevational ranges, highly forest dependent species, and larger birds are most prone to population declines, and we can use our analyses to predict which species may be at more risk of extinction now and in the near future (Tables 1, 2) to help bring attention to these species and better inform conservation strategies for Philippine birds. With many recently described bird species in the Philippines in the last decade and an increased understanding of the number of cryptic species in the archipelago that have yet to be elevated to species-level (Lohman et al., 2010; Collar, 2011; Campbell et al., 2016), identifying avian ecological traits of extinction risk can be crucial for the conservation of newly described species in the future. If deforestation continues and habitats are further diminished and degraded, particularly in lowland regions, species currently at risk in the Philippines may become extinct and the threat of extinction will expand to other species, including those that have yet to be described. Furthermore, we have a limited understanding of the effects of climate change on tropical birds and the future impacts of climate change on tropical montane forest endemics are likely to be underestimated (Harris et al., 2011; Wormworth and Şekercioğlu, 2011). Urgent action to curtail human impact on biodiversity, especially deforestation, degradation, exploitation and climate change, is needed if the Philippine Archipelago is to retain its unique and diverse avian biodiversity.
Data Availability Statement
The original dataset analyzed for this study can be found in Supplementary Table 1 included in the article/Supplementary Material, further inquiries can be directed to the corresponding author/s.
Ethics Statement
Ethical review and approval was not required for the animal study because our study relied on a dataset of trait-based information for bird species and did not involve handling of or experiments on animals.
Author Contributions
KK wrote the manuscript with assistance from MN-C and ÇŞ. ÇŞ and MP conceived the original idea for the project, with later support from KK and MN-C. ÇŞ acquired funding and resources. JM, JB, KK, and MN-C compiled the dataset for this manuscript. KK and MN-C statistically analyzed the data and created the figures and tables. All co-authors contributed to and gave final approval of the manuscript.
Funding
We thank the Christensen Fund for their support in the early stages of this project. We are grateful for the generous support of Hamit Batubay Özkan Conservation Ecology Graduate Fellowship, Barbara J. Watkins Environmental Studies Graduate Fellowship and the University of Utah Global Change and Sustainability Center.
Conflict of Interest
The authors declare that the research was conducted in the absence of any commercial or financial relationships that could be construed as a potential conflict of interest.
Acknowledgments
We thank our good friend and colleague, the late Navjot Sodhi for his involvement in an earlier version of this manuscript. We also thank Michael T. Mathieson for his feedback and assistance with obtaining information for the four Data Deficient species.
Supplementary Material
The Supplementary Material for this article can be found online at: https://www.frontiersin.org/articles/10.3389/fevo.2021.664764/full#supplementary-material
References
Arndt, T., Collar, N. J., and Wink, M. (2019). The taxonomy of Tanygnathus sumatranus. Bull. Br. Ornithol. Club 139:a8. doi: 10.25226/bboc.v139i4.2019.a8
Asian Development Bank (2018). Addressing the Illegal Wildlife Trade in the Philippines. Global Wildlife Program. Available online at: http://pubdocs.worldbank.org/en/997621542735912298/-Illegal-Wildlife-trade-brochure-ADBDENR18NovforWEB.pdf. (accessed November 25, 2020)
Atwood, T. B., Valentine, S. A., Hammill, E., McCauley, D. J., Madin, E. M. P., Beard, K. H., et al. (2020). Herbivores at the highest risk of extinction among mammals, birds, and reptiles. Sci. Adv. 6:8458. doi: 10.1126/sciadv.abb8458
Barlow, J., Peres, C. A., Henriques, L. M. P., Stouffer, P. C., and Wunderle, J. M. (2006). The responses of understorey birds to forest fragmentation, logging and wildfires: an Amazonian synthesis. Biol Conserv. 128, 182–192. doi: 10.1016/j.biocon.2005.09.028
Barlow, J., França, F., Gardner, T. A., Hicks, C. C., Lennox, G. D., Berenguer, E., et al. (2018). The future of hyperdiverse tropical ecosystems. Nature 559, 517–526. doi: 10.1038/s41586-018-0301-1
Bates, D., Mächler, M., Bolker, B., and Walker, S. (2015). Fitting linear mixed-effects models using lme4. J. Statist. Soft. 67, 1–48. doi: 10.18637/jss.v067.i01
Beissinger, S. R. (2000). Ecological mechanisms of extinction. Proc. Natl. Acad. Sci. U S A 97, 11688–11689. doi: 10.1073/pnas.97.22.11688
Bennett, P. M., and Owens, I. P. F. (1997). Variation in extinction risk among birds: chance or evolutionary disposition? Proc. R Soc. B: Biol. Sci. 264, 401–408. doi: 10.1098/rspb.1997.0057
BirdLife International (2021). BirdLife Data Zone. Available online at: http://www.birdlife.org/datazone [accessed January 24, 2021]
Boyer, A. G. (2008). Extinction patterns in the avifauna of the Hawaiian islands. Div. Distribut. 14, 509–517. doi: 10.1111/j.1472-4642.2007.00459.x
Bregman, T. P., Lees, A. C., MacGregor, H. E. A., Darski, B., de Moura, N. G., Aleixo, A., et al. (2016). Using avian functional traits to assess the impact of land-cover change on ecosystem processes linked to resilience in tropical forests. Proc. R Soc B: Biol. Sci. 2016:1289. doi: 10.1098/rspb.2016.1289
Brook, B. W., Sodhi, N. S., and Ng, P. K. L. (2003). Catastrophic extinctions follow deforestation in Singapore. Nature 424, 420–423. doi: 10.1038/nature01795
Brown, J. H. (2014). Why are there so many species in the tropics? J. Biogeogr. 2014:12228. doi: 10.1111/jbi.12228
Burnham, K. P., and Anderson, D. R. (2002). Model Selection and Multimodel Inference: A Practical Information-Theoretic Approach, 2nd Edn. New York, NY: Springer.
Campbell, K., Braile, T., and Winker, K. (2016). Integration of genetic and phenotypic data in 48 lineages of philippine birds shows heterogenous divergence processes and numerous cryptic species. PLoS One 11:e0159325. doi: 10.1371/journal.pone.0159325
Cardillo, M., Purvis, A., Sechrest, W., Gittleman, J. L., Bielby, J., and Mace, G. M. (2004). Human Population Density and Extinction Risk in the World’s Carnivores. PLoS Biol. 2:909–914. doi: 10.1371/journal.pbio.0020197
Collar, N. J., and Andrew, P. (1988). Birds to watch: the ICBP world checklist of threatened birds. ICBP technical publication No. 8. Cambridge, MA: International Council for Bird Preservation.
Collar, N. J. (2011). Species limits in some Philippine birds including the Greater Flameback Chrysocolaptes lucidus. Forktail 27, 29–38.
Davies, K. F., Margules, C. R., and Lawrence, J. F. (2004). A synergistic effect puts rare, specialized species at greater risk of extinction. Ecology 85, 265–271. doi: 10.1890/03-0110
Del Hoyo, J., Elliot, A., and Sargatal, J. (1992-2013). Handbook of Birds of the World. Barcelona: Lynx Edicions, 1–13.
Edwards, D. P., Woodcock, P., Newton, R. J., Edwards, F. A., Andrews, D. J. R., Docherty, T. D. S., et al. (2013). Trophic flexibility and the persistence of understory birds in intensively logged rainforest. Conserv. Biol. 27, 1079–1086. doi: 10.1111/cobi.12059
Fox, J., and Weisberg, S. (2019). An {R} Companion to Applied Regression, Third Edition. Thousand Oaks, CA: Sage.
Gaston, K. J., and Blackburn, T. M. (1995). Birds, body size and the threat of extinction. Philosop. Trans. R. Soc. B: Biol. Sci. 347, 205–212. doi: 10.1098/rstb.1995.0022
Goodman, S. M., Willard, D. E., and Gonzales, P. C. (1995). The birds of Sibuyan Island, Romblon province, Philippines, with particular reference to elevational distribution and biogeographic affinities. Fieldiana Zool. 82, 1–57.
Harris, J. B. C., Şekercioğlu, ÇH., Sodhi, N. S., Fordham, D. A., Paton, D. C., and Brook, B. W. (2011). The tropical frontier in avian climate impact research. Ibis 153, 877–882. doi: 10.1111/j.1474-919X.2011.01166.x
Harrison, X. A., Donaldson, L., Correa-Cano, M. E., Evans, J., Fisher, D. N., Goodwin, C. E. D., et al. (2018). A brief introduction to mixed effects modelling and multi-model inference in ecology. PeerJ. 2018:4794. doi: 10.7717/peerj.4794
Heaney, L. R. (1993). Biodiversity patterns and the conservation of mammals in the Philippines. Asia Life Sci. 2, 261–274.
Henle, K., Davies, K. F., Kleyer, M., Margules, C., and Settele, J. (2004). Predictors of species sensitivity to fragmentation. Biodiv. Conserv. 13, 207–251. doi: 10.1023/B:BIOC.0000004319.91643.9e
Hosner, P. A., Boggess, N. C., Alviola, P., Sánchez-González, L. A., Oliveros, C. H., Urriza, R., et al. (2013). Phylogeography of the robsonius ground-warblers (passeriformes: Locustellidae) reveals an undescribed species from Northeastern Luzon, Philippines. Condor 155, 630–639. doi: 10.1525/cond.2013.120124
Hughes, A. L. (1999). Differential human impact on the survival of genetically distinct avian lineages. Bird Conserv. Intern. 9, 147–154. doi: 10.1017/S0959270900002264
International Union for Conservation of Nature and Natural Resources (2020). The IUCN Red List of Threatened Species. Available online at: http://www.redlist.org [accessed January 24, 2021]
Jones, M. J., Sullivan, M. S., Marsden, S. J., and Linsley, M. D. (2001). Correlates of extinction risk of birds from two Indonesian islands. Biolog. J. Linnean Soc. 73, 65–79. doi: 10.1006/bijl.2001.0525
Kattan, G. H., Alvarez-Lopez, H., and Giraldo, M. (1994). Forest Fragmentation and Bird Extinctions: San Antonio Eighty Years Later. Conserv. Biol. 1994:08010138. doi: 10.1046/j.1523-1739.1994.08010138.x
Keinath, D. A., Doak, D. F., Hodges, K. E., Prugh, L. R., Fagan, W., Şekercioğlu, ÇH., et al. (2017). A global analysis of traits predicting species sensitivity to habitat fragmentation. Glob. Ecol. Biogeogr. 2017, 115–127. doi: 10.1111/geb.12509
Kennedy, R. S., Gonzales, P. C., Dickinson, E. C., Miranda, H. C. Jr., and Fisher, T. H. (2000). A Guide to the Birds of the Philippines. New York, NY: Oxford University Press.
Kruger, O., and Radford, A. N. (2008). Doomed to die? Predicting extinction risk in the true hawks Accipitridae. Anim. Conserv. 11, 83–91. doi: 10.1111/j.1469-1795.2007.00155.x
Kummer, D. (1992). Deforestation in the postwar Philippines. Chicago: University of Chicago Press, doi: 10.2307/2759399
Lambert, F. R., and Collar, N. J. (2002). The future for Sundaic lowland forest birds: long-term effects of commercial logging and fragmentation. Forktail 18, 127–146.
Le Breton, T. D., Zimmer, H. C., Gallagherm, R. V., Cox, M., Allen, S., and Auld, T. D. (2019). Using IUCN criteria to perform rapid assessments of at-risk taxa. Biodiv. Conserv. 28, 863–883.
Lees, A. C., and Peres, C. A. (2009). Gap-crossing movements predict species occupancy in Amazonian forest fragments. Oikos 118, 280–290. doi: 10.1111/j.1600-0706.2008.16842.x
Lohman, D. J., Ingram, K. K., Prawiradilaga, D. M., Winker, K., Sheldon, F. H., Moyle, R. G., et al. (2010). Cryptic genetic diversity in ‘widespread’ Southeast Asian birds suggests that Philippine avian endemism is gravely underestimated. Biol. Conserv. 143, 1885–1890. doi: 10.1016/j.biocon.2010.04.042
Mace, G. M., Collar, N. J., Gaston, K. J., Hilton-Taylor, C., Akçakaya, H. R., Leader-Williams, N., et al. (2008). Quantification of Extinction Risk: IUCN’s System for Classifying Threatened Species. Conserv. Biol. 22, 1424–1442. doi: 10.1111/j.1523-1739.2008.01044.x
Manne, L. L., Brooks, T. M., and Pimm, S. L. (1999). Relative risk of extinction of passerine birds on continents and islands. Nature 399, 258–261. doi: 10.1038/20436
McKinney, M. L. (1997). Extinction vulnerability and selectivity: combining ecological and paleontological views. Annu. Rev. Ecol. Syst. 28, 495–516. doi: 10.1146/annurev.ecolsys.28.1.495
Moore, R. P., Robinson, W. D., Lovette, I. J., and Robinson, T. R. (2008). Experimental evidence for extreme dispersal limitation in tropical forest birds. Ecol. Lett. 11:01196. doi: 10.1111/j.1461-0248.2008.01196.x
Myers, N., Mittermeier, R. A., Mittermeier, C. G., da Fonseca, G. A. B., and Kent, J. (2000). Biodiversity hotspots for conservation priorities. Nature 403, 853–858. doi: 10.1038/35002501
Neate-Clegg, M. H. C., Blount, J. D., and Şekercioğlu, ÇH. (2021). Ecological and biogeographical predictors of taxonomic discord across the world’s birds. Glob. Ecol. Biogeogr. 4:13300. doi: 10.1111/geb.13300
Norris, K., and Harper, N. (2004). Extinction processes in hot spots of avian biodiversity and the targeting of pre-emptive conservation action. Proc. R. Soc. B: Biol. Sci. 271, 123–130. doi: 10.1098/rspb.2003.2576
Ocampo-Peñuela, N., and Pimm, S. L. (2015). Elevational ranges of montane birds and deforestation in the Western Andes of Colombia. PLoS One 2015:0143311. doi: 10.1371/journal.pone.0143311
O’Dea, N., and Whittaker, R. J. (2007). How resilient are Andean montane forest bird communities to habitat degradation? Biodiv. Conserv. 2007:9095. doi: 10.1007/s10531-006-9095-9
Oliver, W. L. R., and Heaney, L. R. (1996). Biodiversity and conservation in the Philippines. International. Zoo News 43, 329–337.
Ong, P. S., Afuan, L. E., and Rosell-Ambal, R. G. (2002). Philippine Biodiversity Conservation Priorities: A second iteration of the national biodiversity strategy and national plan. Department of Environment and Natural Resources – Protected Areas and Wildlife Bureau; Conservation International; Biodiversity Conservation Program – University of the Philippines Center for Integrative and Development Studies; & Foundation for the Philippine Environment.. Quezon City.
Panopio, J. K. B., and Pajaro, M. (2014). The State of Philippine Birds. Haribon Foundation, & BirdLife International. It was a joint publication.. Quezon City.
Peres, C. A., and Palacios, E. (2007). Basin-wide effects of game harvest on vertebrate population densities in Amazonian forests: implications for animal-mediated seed dispersal. Biotropica 39, 304–315. doi: 10.1111/j.1744-7429.2007.00272.x
Pimm, S. L., and Raven, P. (2000). Extinction by numbers. Nature 403, 848–845. doi: 10.1038/35002708
Posa, M. R. C., Diesmos, A. C., Sodhi, N. S., and Brooks, T. M. (2008). Hope for threatened tropical biodiversity: lessons from the Philippines. BioScience 58, 231–240. doi: 10.1641/B580309
Purvis, A., Gittleman, J. L., Cowlishaw, G., and Mace, G. M. (2000). Predicting extinction risk in declining species. Proc. R. Soc. B: Biol. Sci. 267, 1947–1952. doi: 10.1098/rspb.2000.1234
R Core Team (2020). R: A language and environment for statistical computing. R Foundation for Statistical Computing. Vienna: R Core Team.
Rahbek, C., Borregaard, M. K., Colwell, R. K., Dalsgaard, B., Holt, B. G., Morueta-Holme, N., et al. (2019). Humboldt’s enigma: What causes global patterns of mountain biodiversity? Science 365, 1108–1113. doi: 10.1126/science.aax0149
Rasmussen, P. C., Allen, D. N. S., Collar, N. J., Demeulemeester, B., Hutchinson, R. O., Jakosalem, P. G. C., et al. (2012). Vocal divergence and new species in the Philippine Hawk Owl Ninox philippensis complex. Forktail 28, 1–20.
Robson, C. (2000). A Field Guide to the Birds of South-east Asia. United Kingdom: New Holland Publishers.
Robuchon, M., Faith, D. P., Julliard, R., Leroy, B., Pellens, R., Robert, A., et al. (2019). Species splitting increases estimates of evolutionary history at risk. Biol. Conserv. 235, 27–35. doi: 10.1016/j.biocon.2019.03.041
Russell, G. J., Brooks, T. M., McKinney, M. M., and Anderson, C. G. (1998). Present and future taxonomic selectivity in bird and mammal extinctions. Conserv. Biol. 12, 1365–1376. doi: 10.1111/j.1523-1739.1998.96332.x
Şekercioğlu, ÇH. (2002). Forest fragmentation hits insectivorous birds hard. Direct. Sci. 1, 62–64. doi: 10.1100/tsw.2002.190
Şekercioğlu, ÇH. (2007). Conservation ecology: Area trumps mobility in fragment bird extinctions. Curr. Biol. 17, R283–R286. doi: 10.1016/j.cub.2007.02.019
Şekercioğlu, ÇH. (2011). Functional extinctions of bird pollinators cause plant declines. Science 6020, 1019–1020. doi: 10.1126/science.1202389
Şekercioğlu, ÇH., and Sodhi, N. S. (2007). Conservation biology: Predicting birds’ responses to forest fragmentation. Curr. Biol. 17, R838–R840. doi: 10.1016/j.cub.2007.07.037
Şekercioğlu, ÇH., Ehrlich, P. R., Daily, G. C., Aygen, D., Goehring, D., and Sandí, R. F. (2002). Disappearance of insectivorous birds from tropical forest fragments. PNAS 99, 263–267.
Şekercioğlu, ÇH., Daily, G. C., and Ehrlich, P. R. (2004). Ecosystem consequences of bird declines. Proc. Natl. Acad. Sci. U S A 101, 18042–18047. doi: 10.1073/pnas.0408049101
Şekercioğlu, ÇH., Schneider, S. H., Fay, J. P., and Loarie, S. R. (2008). Climate change, elevational range shifts and bird extinctions. Conserv. Biol. 22, 140–150. doi: 10.1111/j.1523-1739.2007.00852.x
Şekercioğlu, ÇH., Mendenhall, C. D., Brenes, F. O., Horns, J. J., Ehrlich, P. R., and Daily, G. C. (2019). Long-term declines in bird populations in tropical agricultural countryside. PNAS 116, 9903–9912. doi: 10.1073/pnas.1802732116
Sheard, C., Neate-Clegg, M. H. C., Alioravainen, N., Jones, S. E. I., Vincent, C., MacGregor, H. E. A., et al. (2020). Ecological drivers of global gradients in avian dispersal inferred from wing morphology. Nature Communications 2020, 16313. doi: 10.1038/s41467-020-16313-6
Sherry, T. W., Kent, C. M., Sánchez, N. V., and Şekercioğlu, ÇH. (2020). Insectivorous birds in the Neotropics: Ecological radiations, specialization, and coexistence in species-rich communities. Auk 137:ukaa049. doi: 10.1093/auk/ukaa049
Simkins, A. T., Buchanan, G. M., Davies, R. G., and Donald, P. F. (2019). The implications for conservation of a major taxonomic revision of the world’s birds. Anim. Conserv. 23, 345–352. doi: 10.1111/acv.12545
Sodhi, N. S., and Brook, B. W. (2006). Southeast Asian Biodiversity in Crisis. Cambridge, MA: Cambridge University Press.
Sodhi, N. S., Liow, L. H., and Bazzaz, F. A. (2004). Avian extinctions from tropical and subtropical forests. Ann. Rev.Ecol. Evol. Syst. 35, 323–345. doi: 10.1146/annurev.ecolsys.35.112202.130209
Sodhi, N. S., Posa, M. R. C., Lee, T. M., Bickford, D., Koh, L. P., and Brook, B. W. (2010). The state and conservation of Southeast Asian biodiversity. Biodiv. Conserv. 19, 317–328. doi: 10.1007/978-94-007-0168-7_2
Stattersfield, A. J., Crosby, M. J., Long, M. J., and Wege, D. C. (1998). Endemic Bird Areas of the world: priorities for biodiversity conservation. Cambridge, UK: BirdLife International.
Tanalgo, K., Pineda, J., Agravante, M., and Amerol, Z. M. (2015). Bird Diversity and Structure in Different Land-use Types in Lowland South-Central Mindanao, Philippines. Trop. Life Sci. Res. 26, 85–103.
Terborgh, J. (1974). Preservation of Natural Diversity: The Problem of Extinction Prone Species. BioScience 24, 715–722. doi: 10.1023/B
Tobias, J., Şekercioğlu, ÇH., and Vargas, F. H. (2013). Bird conservation in tropical ecosystems: challenges and opportunites. Key Topics Conserv. Biol. 2, 258–276.
Turner, I. M. (1996). Species Loss in Fragments of Tropical Rain Forest: A Review of the Evidence. J. Appl. Ecol. 1996:2404743. doi: 10.2307/2404743
Turubanova, S., Potapov, P. V., Tyukavina, A., and Hansen, M. C. (2018). Ongoing primary forest loss in Brazil, Democractic Republic of the Congo, and Indonesia. Environ. Res. Lett. 13:074028. doi: 10.1088/1748-9326/aacd1c
Visco, D. M., Michel, N. L., Boyle, W. A., Sigel, B. J., Woltmann, S., and Sherry, T. W. (2015). Patterns and causes of understory bird declines in human-disturbed tropical forest landscapes: A case study from Central America. Biol. Conserv. 191, 117–129. doi: 10.1016/j.biocon.2015.05.018
Von Euler, F. (2001). Selective extinction and rapid loss of evolutionary history in the bird fauna. Proc. R. Soc. B: Biol. Sci. 268, 127–130. doi: 10.1098/rspb.2000.1340
Wang, Y., Thornton, D. H., Ge, D., Wang, S., and Ding, P. (2015). Ecological correlates of vulnerability to fragmentation in forest birds on inundated subtropical land-bridge islands. Biol. Conserv. 191, 251–257. doi: 10.1016/j.biocon.2015.06.041
Wang, Y., Si, X., Bennett, P. M., Chen, C., Zeng, D., Zhao, Y., et al. (2018). Ecological correlates of extinction risk in Chinese birds. Ecography 41, 782–794. doi: 10.1111/ecog.03158
Webb, J. K., Brook, B. W., and Shine, R. (2002). What makes a species vulnerable to extinction? Comparative life-history traits of two sympatric snakes. Ecol. Res. 17, 59–67. doi: 10.1046/j.1440-1703.2002.00463.x
Weerd, M. V., Strijk, J., and Snelder, D. (2003). The importance of forest fragments for birds and local communities in Northeast Luzon, Philippines. Sylvatrop Tech. J. Philippine Ecosyst. Nat. Resour. 13, 1–30.
White, R. L., and Bennett, P. M. (2015). Elevational distribution and extinction risk in birds. PLoS One 10, 1–17. doi: 10.1371/journal.pone.0121849
Wilcove, D. S. (1985). Nest Predation in Forest Tracts and the Decline of Migratory Songbirds. Ecology 66, 1211–1214. doi: 10.2307/1939174
Wormworth, J., and Şekercioğlu, ÇH. (2011). Winged Sentinels: Birds and Climate Change. Cambridge, MA: University Press, doi: 10.1017/CBO9781139150026
Yessoufou, K., Daru, B. H., and Davies, J. T. (2012). Phylogenetic Patterns of Extinction Risk in the Eastern Arc Ecosystems, an African Biodiversity Hotspot. PLoS One 2012:0047082. doi: 10.1371/journal.pone.0047082
Keywords: cryptic species, deforestation, island biogeography, bird conservation, endemism, elevational range, forest dependency, body size
Citation: Kittelberger KD, Neate-Clegg MHC, Blount JD, Posa MRC, McLaughlin J and Şekercioğlu ÇH (2021) Biological Correlates of Extinction Risk in Resident Philippine Avifauna. Front. Ecol. Evol. 9:664764. doi: 10.3389/fevo.2021.664764
Received: 06 February 2021; Accepted: 11 May 2021;
Published: 14 June 2021.
Edited by:
David Jack Coates, Department of Biodiversity, Conservation, and Attractions (DBCA), AustraliaReviewed by:
Andres Garcia, National Autonomous University of Mexico, MexicoZuzana Burivalova, Princeton University, United States
Copyright © 2021 Kittelberger, Neate-Clegg, Blount, Posa, McLaughlin and Şekercioğlu. This is an open-access article distributed under the terms of the Creative Commons Attribution License (CC BY). The use, distribution or reproduction in other forums is permitted, provided the original author(s) and the copyright owner(s) are credited and that the original publication in this journal is cited, in accordance with accepted academic practice. No use, distribution or reproduction is permitted which does not comply with these terms.
*Correspondence: Kyle D. Kittelberger, kyle.kittelberger@utah.edu