- Department of Ecology and Evolutionary Biology, Tulane University, New Orleans, LA, United States
Epigraph: “The house is burning. We do not need a thermometer. We need a fire hose.” (P. 102, Janzen and Hallwachs, 2019). Insectivorous birds are declining widely, and for diverse reasons. Tropical insectivorous birds, more than 60% of all tropical birds, are particularly sensitive to human disturbances including habitat loss and fragmentation, intensive agriculture and pesticide use, and climate change; and the mechanisms are incompletely understood. This review addresses multiple, complementary and sometimes synergistic explanations for tropical insectivore declines, by categorizing explanations into ultimate vs. proximate, and direct versus indirect. Ultimate explanations are diverse human Anthropocene activities and the evolutionary history of these birds. This evolutionary history, synthesized by the Biotic Challenge Hypothesis (BCH), explains tropical insectivorous birds' vulnerabilities to many proximate threats as a function of both these birds' evolutionary feeding specialization and poor dispersal capacity. These traits were favored evolutionarily by both the diversity of insectivorous clades competing intensely for prey and co-evolution with arthropods over long evolutionary time periods. More proximate, ecological threats include bottom-up forces like declining insect populations, top-down forces like meso-predator increases, plus the Anthropocene activities underlying these factors, especially habitat loss and fragmentation, agricultural intensification, and climate change. All these conditions peak in the lowland, mainland Neotropics, where insectivorous bird declines have been repeatedly documented, but also occur in other tropical locales and continents. This multiplicity of interacting evolutionary and ecological factors informs conservation implications and recommendations for tropical insectivorous birds: (1) Why they are so sensitive to global change phenomena is no longer enigmatic, (2) distinguishing ultimate versus proximate stressors matters, (3) evolutionary life-histories predispose these birds to be particularly sensitive to the Anthropocene, (4) tropical regions and continents vary with respect to these birds' ecological sensitivity, (5) biodiversity concepts need stronger incorporation of species' evolutionary histories, (6) protecting these birds will require more, larger reserves for multiple reasons, and (7) these birds have greater value than generally recognized.
Introduction
Birds are one of the most thoroughly described animal taxa, and thus provide one of the best indicators of Anthropocene impacts on the environment (Robinson and Sherry, 2012), and the news is anything but good. In North America, for example, diverse types of birds are declining precipitously, including once widespread and abundant species, providing losses of ecological services even absent complete extirpation or extinction (Rosenberg et al., 2019). Many potential causes of these declines have been identified (e.g., Sodhi et al., 2007b; Rosenberg et al., 2019). The combination of these global-scale human impacts has increased the extinction rates of birds and other organisms 1,000–10,000-fold (Pimm et al., 2006; Sodhi et al., 2007b; Raven and Wagner, 2021). These environmental impacts thus constitute a mass extinction comparable to past, non-human mass extinctions that profoundly and repeatedly changed the evolutionary course of history going back hundreds of millions of years. These human impacts constitute the “sixth mass extinction” (Kolbert, 2014; Ceballos et al., 2017), a major component of the transition from the Holocene to the Anthropocene, a proposed and not yet formally recognized epoch.
Human impacts on global Biological Diversity (henceforth, “biodiversity”) are not new. Ever since humans emerged out of Africa more than 50,000 years ago, and even before, humans over-hunted megafauna, including birds, to the point of extinction on virtually every continent, and on hundreds of islands (Sodhi et al., 2007b). This resulted initially from the most primitive technologies such as stone tools. Human technologies today eclipse what was possible with stone-age technologies, and the impacts on biodiversity globally are correspondingly devastating. The very diversity of these human impacts challenges our ability to explain precisely the causality. How can one make sense of why and how humans cause environmental havoc with so many potential causes and interactions thereof? Effective conservation action requires clear diagnosis of causality, including the costs and consequences such as lost species and their ecosystem services.
Insectivorous tropical birds help dissect the present and potential future causes of species extirpations and extinctions, and the stakes of this impoverishment. These birds' extraordinary sensitivity to human impacts is particularly well-documented in the Neotropics (e.g., Robinson, 1999; Sekercioglu et al., 2002, 2004, 2019; Sodhi et al., 2004; Stratford and Robinson, 2005; Sigel et al., 2006, 2010; Stouffer et al., 2006; Kumar and O'Donnell, 2007; Laurance et al., 2011; Newbold et al., 2013; Martínez et al., 2021), which comprise the focus of this review. Nonetheless, such sensitivity likely applies to the tropics more generally (e.g., Adeney et al., 2006; Peters et al., 2008; Peters and Okalo, 2009; Bregman et al., 2014), warranting inclusion of literature from the Paleotropics. Diverse causes of tropical insectivorous birds' sensitivity to the Anthropocene have been proposed, but remain poorly understood. Degradation via fragmentation is pivotal because of poor dispersal in many tropical insectivorous birds (e.g., Sekercioglu et al., 2002; Sheard et al., 2020; Sherry et al., 2020; Stouffer, 2020), including montane species (Soh et al., 2006; Sodhi et al., 2007b), and forest edge effects on forest interior species (Pfeifer et al., 2017). However, why do these birds disperse poorly, and why is this important to their conservation?
The stakes of tropical insectivorous bird declines, extirpations, and extinctions are high. Globally, about two-thirds of all species, and 89% of all land birds, are tropical (Sodhi et al., 2007b; Sekercioglu et al., 2012). In the Neotropics, >60% of all bird species are insectivorous, comprising ~2,081 resident, endemic species, not including migrants visiting the Neotropics seasonally (Sherry et al., 2020). These insectivores have arisen multiple times evolutionarily, given the wide distribution of Neotropical insectivores among avian taxa (Supplementary Table 1). Tropical insectivores thus include thousands of species, and billions of individuals, consuming vast numbers of diverse arthropods, including herbivorous insects, year-round, intimating a potentially significant impact on vegetation.
Human population size and activities constitute ultimate mechanisms of global change impacts on all organisms, including tropical birds. However, specifically how do human activities impact tropical, especially Neotropical, insectivorous birds so profoundly? We need to better understand both the proximate and ultimate causes, as well as the direct and indirect ones. Although narrowing the focus here to just tropical, especially Neotropical insectivorous birds, risks loss of generality, it gains the potential to identify overlooked mechanisms.
This review emphasizes the importance of incorporating the tropics better into conservation diagnoses and planning, i.e., taking a more globally comprehensive approach, using insectivorous Neotropical birds as a model system to highlight these issues. The fundamental assertion is that tropical insectivorous birds face many simultaneous, interacting human threats—a perfect storm. Additionally, a particularly important and poorly recognized ultimate threat is the evolutionary history and geography of tropical, especially mainland Neotropical insectivores. This history explains many of the more immediate, proximate vulnerabilities of insectivores, including their susceptibility to top-down, bottom-up, and a host of other genetic and demographic risk factors, all as the result of feeding specializations (Sherry et al., 2020). This combination of ultimate and proximate threats illuminates consequences of these declines, and indicates a variety of conservation implications and recommendations.
Diverse tropical organisms other than insectivorous birds are evolutionarily specialized in their species interactions, which likely increases their vulnerability to many of the same threats that impact insectivorous birds. For example, tropical mutualisms involving plant pollinators and fruit dispersers can involve few interacting animal or plant species, making these species interactions highly vulnerable to forest fragmentation (e.g., Laurance, 2005; Marjakangas et al., 2019; Carreira et al., 2020). Addressing all these, and many other tropical species interactions is beyond the scope of this review, but many lessons from this review may extend beyond just insectivorous tropical birds.
Ultimate Mechanisms of Tropical Insectivorous Bird Declines
I start out distinguishing ultimate from proximate, and direct from indirect mechanisms of tropical insectivorous bird declines (Figure 1). I use “ultimate” in its general (non-biological) sense to distinguish a relatively distant from a more immediate “proximate” mechanism or trigger, although the boundary between these is arbitrary—for example, “Habitat Fragmentation Components” in the figure, especially habitat area reduction, represents an ultimate as well as a proximate factor. Ultimate mechanisms create the context and conditions for the more proximate mechanisms or triggers of population response. Two ultimate mechanisms are recognized: (1) Anthropocene environmental impacts that then trigger population declines via a variety of more proximate, demographic mechanisms of habitat loss and degradation that in turn cause declining reproductive success, survival, and/or population viability. (2) Evolved life-history traits that emphasize the legacy of tropical evolutionary history that make some populations or species particularly sensitive to the proximate mechanisms. This latter use of the term “ultimate” parallels its application to animal behavior causation, which distinguishes ultimate evolutionary adaptations from proximate endocrinological and neurobiological triggers. Ecosystem services of insectivorous birds (Section ultimate vs. proximate threats) link most directly to proximate mechanisms because of dependence on the birds' demography, although the ecosystem impacts and ecosystem services feedback into both ultimate and proximate mechanisms (Figure 2).
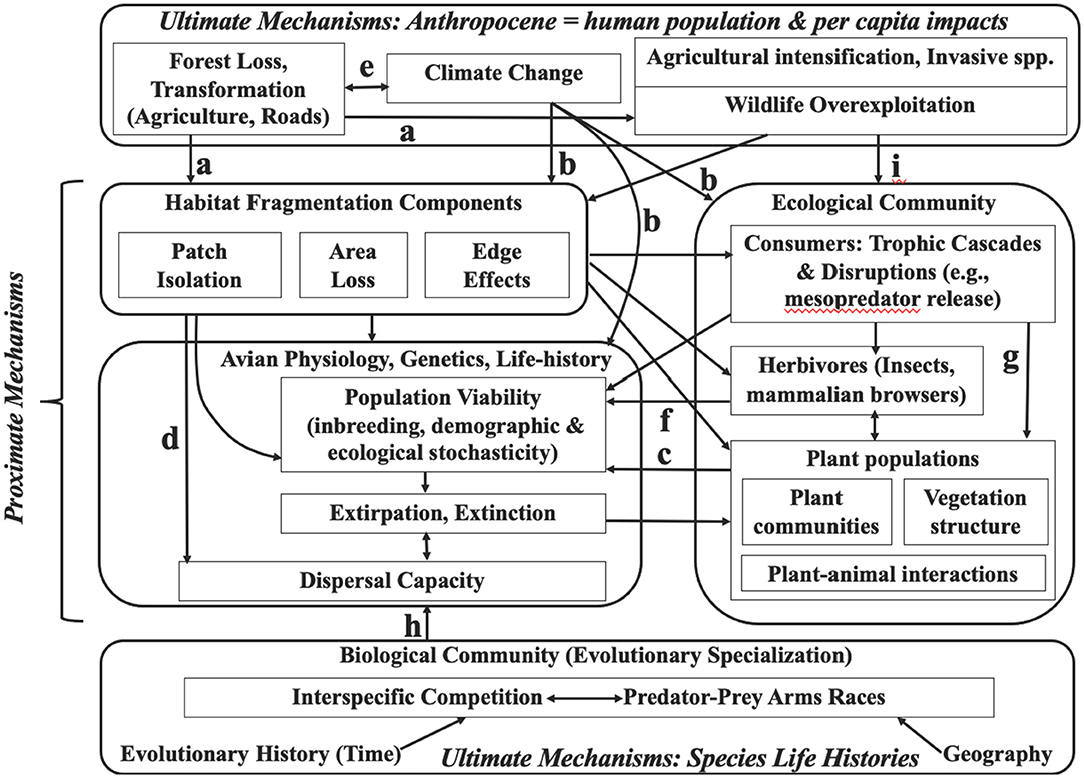
Figure 1. Diverse mechanisms (arrows) of Anthropocene global change impacts, emphasizing insectivorous birds. A variety of mechanisms (lettered) are particularly important in the tropics—see text for further explanation and references. (a) Increased forest loss and road construction dramatically increase forest accessibility to subsistence hunting and habitat degradation via a variety of mechanisms including forest fragmentation, fires, and further human exploitation of various resources. These are ultimate, mostly direct mechanisms. (b) Climate change alters avian geographic ranges, impacts that will be particularly dramatic in the tropics, given extensive elevation gradients and small geographic ranges, high species richness, and endemism (e.g., the Andes). These are ultimate, and mostly indirect impacts. (c) Indirect effects of structural and floristic vegetation change on birds due to modification of habitat and microhabitat, which in turn impacts feeding and nesting sites. Some of these are the indirect impact of trophic cascades. (d) An important ultimate mechanism highlighted in this paper involves many tropical birds poorly adapted for dispersal, making them highly vulnerable to forest patch isolation and indirectly to many demographic consequences of small population size. These are mostly indirect mechanisms. (e) Climate change interacts reciprocally with forest loss and fragmentation, which has largely ultimate, indirect impacts. (f) Bottom-up impacts of insect declines are both proximate and direct. (g) Top-down impacts of predator losses triggering trophic cascades, such as Meso-predator Release Hypothesis, indirectly reduce avian feeding and reproduction, a proximate threat. (h) The Biotic Challenge Hypothesis (BCH), explains evolutionary feeding specialization in the tropics and consequence of reduced dispersal due to tradeoff between feeding efficiency and dispersal capacity—an ultimate, indirect threat. (i) Multiple hypotheses stemming from pesticides, wildlife overexploitation, and invasive species impact insect abundances (mostly declines) and trophic chain disruptions, contributing ultimate threats that are both direct and indirect. Modified and extended from Robinson and Sherry (2012) and Sherry et al. (2020).
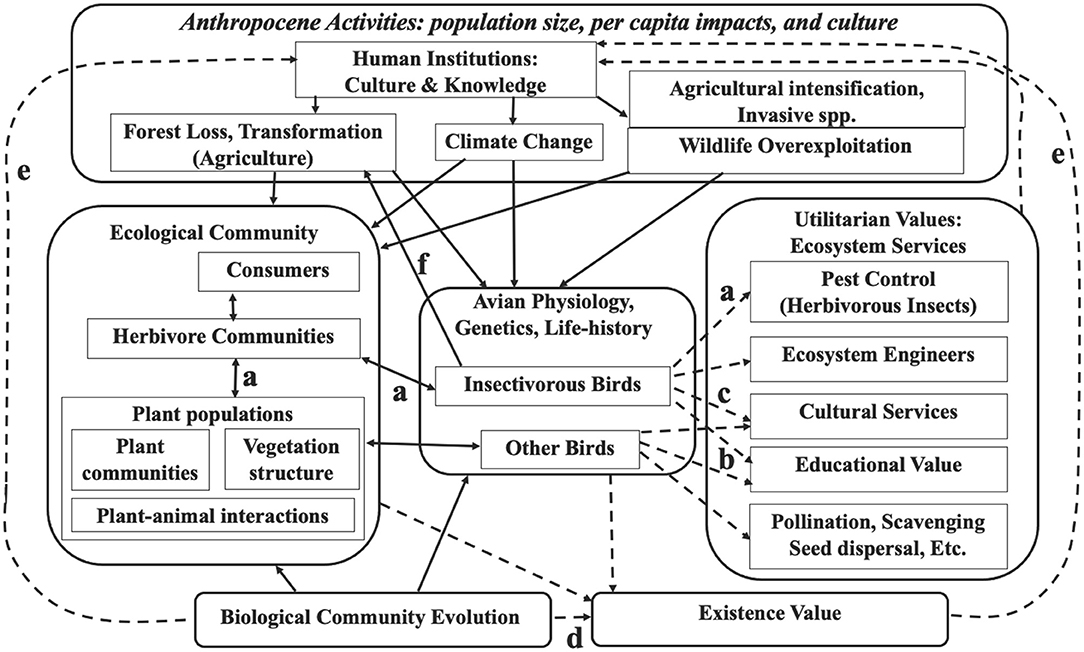
Figure 2. Simplified mechanisms of threat to tropical insectivorous birds (from Figure 1) integrated with values of these animals and their ecosystems. Biological processes shown with solid arrow-headed lines; human values and concepts shown with dashed arrow-headed lines. As in Figure 1, Anthropocene activities (upper box) all impact both species biodiversity components, the ecological community (left-middle box) and bird traits (central box). Several mechanisms or values are particularly important for tropical insectivorous birds. (a) By consuming herbivorous insects these birds potentially influence plant demography (a trophic cascade), and vice versa, hence the double-headed arrows. (b) Extraordinary tropical species richness and diverse ecological roles within communities underscore ecosystem utilitarian values of birds, including insectivorous species. (c) The diversity of tropical birds and the charismatic traits of many species, emphasize their potential cultural ecosystem value, e.g., for ecotourism and education. (d) The long evolutionary history, specializations, and life-histories of tropical birds emphasize their intrinsic value, and thus indirectly their potential to educate humans. (e) Knowledge of the evolutionary history and traits of tropical insectivorous birds can feedback into human knowledge, culture, and ultimately conservation action, and thus help humans change how we relate to nature. (f) Related to mechanism (a), insectivorous birds benefit humans directly, economically by controlling agricultural crop pests.
I further recognize relatively direct versus indirect mechanisms nested within each of the ultimate and proximate mechanisms that constitute a higher level of a hierarchy of decline mechanisms. Direct mechanisms impact birds' populations without intermediary steps or organisms, illustrated by food organisms such as insects, and predators on adult birds or their nests. Indirect mechanisms operate via one or more intermediary agents, e.g., via trophic cascades and trophic downgrading of ecosystems (e.g., Estes et al., 2011; Terborgh, 2015), and can be far stronger than direct effects (Feeley and Terborgh, 2008; Sherry, 2008).
This recognition of a hierarchy of threats to insectivorous tropical birds is imperfect, but helps categorize fundamentally different causes with different challenges and solutions. For example, an important reason to highlight indirect mechanisms is that, as Estes et al. (2011) argue, ecological processes are often cryptic, e.g., unless an ecosystem is severely perturbed or the scale of a study is large enough in space or time to detect relevant interactions, making them easily overlooked. Some solutions are also relatively simple, like creating corridors for dispersal among refuges, whereas others like addressing climate change will be far more challenging given human institutions and priorities. Categorizing threats also helps identify new ones (what's missing), and will be necessary to model complex systems involving global human actions.
The Anthropocene
The first ultimate mechanism explaining tropical insectivorous bird declines is human global change phenomena, which emphasize human agency. These include the size of the human population—now more than 7.8 billion and growing—and the per capita environmental impacts (Vitousek et al., 1997; Rockström et al., 2015; Raven and Wagner, 2021), neither illustrated in Figure 1. These human phenomena then cause several subsidiary ultimate human activities, including habitat loss and conversion (to agriculture and other human-centered land-uses), climate change, pesticide use, invasive species, and wildlife overexploitation. Emphasizing these human impacts is important because of the ultimate human responsibility for the conservation of these species, and the different contributions to the problem by country and region (Davidson et al., 2012; Williston, 2019).
The term “Anthropocene” itself connotes a particular species, namely humans, as the cause of the environmental threats reviewed in this paper, which is problematic according to some social scientists, who argue instead that we must recognize that the wealthiest, and thus most politically and economically powerful, humans and nations have disproportionately caused the Anthropocene biodiversity crisis. Simultaneously, the most disenfranchised humans, such as indigenous tribes in Amazonia, are as much victims of human global change phenomena (Demos, 2017) as organisms like insectivorous tropical birds.
Species Life-Histories
The second ultimate cause of tropical insectivorous birds' extraordinary sensitivity to human disturbance is the evolutionary history responsible for species' distinctive life histories (Pavlacky et al., 2015). One of their most notable life-history traits is poor dispersal (e.g., Stratford and Robinson, 2005; Moore et al., 2008; Salisbury et al., 2012), which makes many of these species particularly vulnerable to habitat fragmentation. Sheard et al. (2020) also document a trend toward poor-dispersal wing shapes (lower Hand-Wing Index, thus less pointed wing tips) in low latitude birds globally, as well as in insect-feeders. We recently proposed an explanation for this poor dispersal, namely the Biotic Challenge Hypothesis (BCH), which derives from the ecology and evolution of insectivorous Neotropical birds (Sherry et al., 2020). According to this hypothesis, insectivorous birds' poor dispersal results from a combination of wing shapes adapted for feeding efficiency, at the expense of efficiency and endurance to travel any great distance, combined with the evolution of physiologically conservative metabolic rates in some species, which also limit endurance. The BCH thus entails a life-history tradeoff, involving dispersal ability weakened by the evolutionary necessity to compete for food with other tropical insectivores. Two relevant ecological processes intensify, particularly in the mainland equatorial lowlands, to create a challenging environment in which to forage: (1) diffuse interspecific competition with hundreds, if not thousands of other insectivores, and (2) predator-prey arms races. This competition combined with the diverse behavioral, chemical, and physical defenses by arthropods against different bird and other insectivorous species taken together challenge these predators' ability to detect, catch, handle, and digest prey (Sherry, 2016; Sherry et al., 2020). Mainland (South American and Central American) Neotropical forests comprise the most species-rich forested environments globally, for diverse kinds of organisms including insectivorous birds (at least 2,081 resident endemic insectivorous species from 14 orders and 46 families, ~60% of all Neotropical birds; Sherry et al., 2020). This combination of factors in the tropics help explain the diversity of avian feeding specializations (Supplementary Table 2) needed to survive in such environments.
Globally, avian specialization and extinction risk are linked (Sodhi et al., 2007b; Clavel et al., 2010; Sekercioglu, 2011). The BCH helps explain why Neotropical insectivorous birds' foraging specializations have made these species vulnerable to human global change phenomena, as discussed below in the context of specific proximate population decline mechanisms and dispersal limitation. A second likely cost, or tradeoff, involving feeding specializations is reduced feeding flexibility, which should exacerbate these species' vulnerability to declining arthropod abundance and the loss of feeding substrates (Ducatez et al., 2020), and to physiologically uninhabitable conditions. In becoming adapted to feed on particular substrates (Supplementary Table 2; Visco et al., 2015; Sherry et al., 2020), these birds have likely lost efficiency exploiting other prey and substrates. Betts et al. (2019) argue that tropical birds and other vertebrates are relatively sensitive to disturbances [see also Sheldon (2019)], especially those associated with fragmentation and edge effects (“extinction filters”), because of less exposure evolutionarily to disturbances compared to higher latitude species.
Multiple lines of evidence support a long evolutionary history, relatively stable (reduced-seasonality) climate, and disturbance-resistant wet forests (Cochrane and Barber, 2009) necessary to evolve the feeding specializations seen in insectivorous Neotropical birds (Sherry et al., 2020; Miller et al., 2021). These specializations, including the variety of feeding strategies plus relevant anatomical and other adaptations, have resulted from diverse adaptive radiations implicit in Supplementary Table 2. The phylogenies of these birds also indicate that these tropical insectivorous birds, and by inference their adaptations (and arthropod counter adaptations) are in some cases many tens of millions of years old, and accumulated gradually over a long time period (Salisbury et al., 2012; Harvey et al., 2020). This latter phylogeny of the suboscine passerines (non-songbird perching birds, largely endemic to the Neotropics except for the New World flycatchers–Tyrannidae—many of which migrate to the Nearctic region, and five Old World families including broadbills and pittas) puts the origin of diversification of this group at 44.5 million years ago. Even though this group is not entirely insectivorous, many of these families—including antbirds, ovenbirds and Woodcreepers, and New World flycatchers—comprise by far the greatest diversity of Neotropical insectivore species, which themselves comprise ~60% of all Neotropical birds (Sherry et al., 2020). Moreover, the suboscines in Harvey et al. (2020) are just some of the tropical insectivores (Supplementary Tables 1, 2).
Another reason to emphasize tropical insectivorous birds' life-histories is their evolutionary species interactions. Many bird-insect interactions are likely co-evolutionary, but this has yet to be as well-documented in birds as in many bat-insect interactions (e.g., Conner and Corcoran, 2012; ter Hofstede and Ratcliffe, 2016). Enhancement is another species interaction, defined as the increased accessibility of prey to one group of predators caused by altered behavior or microhabitat use caused by other predators (Charnov et al., 1976). Enhancement is pervasive in insectivorous Neotropical birds, which have evolved many specializations to exploit the anti-predator behaviors of their prey that have evolved in response to other predators (Sherry et al., 2020). Enhancement, like coevolution, likely requires long periods of time to evolve the necessary foraging traits due to their likely genetic complexity (e.g., predator and prey behavior, physiology, morphology). Some examples of enhancement are illustrative: Army ant-following birds exploit the conspicuousness of the arthropods and other animals fleeing the army ants, Epinecrophylla antwrens are specialized to feed on arthropods concealed in the suspended dead leaves that provide hiding refugia from other predators, and a variety of insectivorous birds join mixed-species flocks year-round to exploit the arthropods conspicuously fleeing other birds that act as beaters revealing prey presence. Insectivorous birds' predator-prey arms races and diffuse interspecific competition for prey, argued to have caused many tropical insectivorous birds' foraging specializations (Sherry et al., 2020), emphasize evolutionary species interactions. The evolutionary nature of all these species interactions indicates the need to expand beyond just ecological species interactions to understand the origins—and vulnerability—of tropical insectivorous birds.
Proximate Mechanisms of Tropical Avian Insectivore Declines
Proximate mechanisms of population decline are the focus of most research to date on the causes of insectivorous bird declines, in part because their proximity to the declines spatially and temporally likely makes these mechanisms easier to recognize and test. These can be direct, e.g., via altered food abundance or predation, both of which impact reproduction or survival, and thus population size; or indirect via intermediary species or circumstances. Many of these proximate causes of insectivorous tropical bird decline likely also operate synergistically, a poorly documented phenomenon. Synergies are potentially extremely important because of their multiplicative nature. Some examples are forest loss and fragmentation interacting with climate change (Laurance et al., 2014; Lawrence and Vandecar, 2015; Nobre et al., 2016; Marengo et al., 2018), and also propelling road-building and overexploitation of wildlife (e.g., Peres, 2001; Benchimol and Peres, 2014).
Insect Declines
One of the most obvious potential direct mechanism of insectivorous bird declines is the decline of their prey. Insect declines are widely documented and reviewed, probably because of their profound ecological importance (Dirzo et al., 2014; Sánchez-Bayo and Wyckhuys, 2019; Cardoso et al., 2020; Montgomery et al., 2020; Tallamy et al., 2020; van Klink et al., 2020; Wagner, 2020; Wagner et al., 2021). These references identify multiple causes of insect decline (including the ultimate cause, human impacts; Figure 1): habitat loss and fragmentation, pollutants associated with agriculture, invasive insect species (e.g., competitors), climate change, overexploitation of some insects, invasive plants that host few herbivores (Tallamy et al., 2020); and coextinction of specialized insects such as parasites on other insects, mutualists such as insect pollinators, coprophages such as dung beetles, and mycophages (fungal feeders; Cardoso et al., 2020). These multiple threats to insects amount to death by a thousand cuts (Wagner et al., 2021)—an apt phrase for insectivorous tropical bird declines as well.
What's most relevant here is whether or not tropical insects are declining, and the evidence to date is equivocal. Some studies support tropical insect declines. Janzen and Hallwachs (2019, 2021) use mostly anecdotal information, but from multiple sources, to conclude that insects are declining alarmingly from seasonal tropical forests in Costa Rica, and attribute these declines to loss of primary forests, agricultural intensification and pesticides, monocultures, and especially climate change. At La Selva Biological Station on the wetter, Caribbean side of Costa Rica, Salcido et al. (2020) document declines of moth caterpillar, and parasitoid wasp and fly species, along with tri-trophic species interactions involving these species. However, scant and locally patchy long-term insect population and diversity data preclude broad, pan-tropical generalizations (van Klink et al., 2020; Wagner, 2020). Wagner et al. (2020) emphasize that insect population dynamics vary regionally (and taxonomically), even considering just tropical microlepidoptera (moths); and note that intact Ecuadorean forests are not encountering declines. These same authors identify the steepest declines in species with food-specialist larvae, large wing-span, small geographic range, poor dispersal, and univoltism (single brood annually); and species associated with grasslands, aridlands, and nutrient-poor habitats (due to nitrogen pollution). Raven and Wagner (2021) make a compelling case for agricultural intensification coupled with climate change as the major causes of insect declines globally to date, which predicts accelerating tropical insect declines given agricultural, and human population trends.
Sekercioglu et al. (2002) failed to find evidence that insect declines caused bird declines, whereas Lister and Garcia (2018, 2019) but see Willig et al., 2019; Schowalter et al., 2021) argued for insect declines causing vertebrate predator declines in Puerto Rico. Wagner (2020) argues that scant evidence is available presently to link bird declines to insect declines. One basis for Wagner's skepticism is that neither bat nor bird declines is as great as predicted by insect declines. Similarly, Vergara et al. (2020) argue that birds and insects are impacted differently and independently by degradation of temperate, austral forests. Thus, bottom-up causes of insectivorous tropical bird declines are plausible, albeit far from certain.
Lost insect substrates and microhabitats may be as important causes of bird declines as insect declines per se (Stratford and Stouffer, 2015; Visco et al., 2015), although the two may be linked as in the case of tropical lianas (Schnitzer et al., 2020). Neotropical insectivorous birds are often substrate specialists, inferred from stereotyped feeding habits linked to morphology and behavior (Sherry, 1990, Supplementary Table 2), and the loss of which could jeopardize a variety of these birds' survival. For example, Michel et al. (2015a) argue that the most plausible explanation for the decline of insectivorous birds from some Central American forests is the local increase of collared peccaries (Pecari tajacu): This study focused on three unrelated insectivorous bird species in multiple Central American sites, and showed that (a) these species foraged preferentially in vine and liana tangles, (b) such viney substrates are more abundant where peccaries are least abundant (including experimental peccary-exclosure plots), and (c) abundant peccaries are associated with reduced abundance of these particular bird species. This liana-substrate hypothesis is reinforced by the variety of insects that frequent vines and lianas (Schnitzer et al., 2020).
Natural Enemies and Trophic Cascades
Some of the most obvious potential direct threats to insectivorous tropical birds are natural enemies like predators and diseases, some of which are addressed below in context of invasive species (section invasive species). Nest predators are important to diverse birds, including tropical birds, and have been discussed repeatedly in the context of indirect results of tropical forest fragmentation (e.g., Young et al., 2008; Spanhove et al., 2009; Newmark and Stanley, 2011; Visco and Sherry, 2015). Predation on adult birds certainly occurs, but its rarity (compared to nest depredation) and difficulty of observation makes it hard to study. Also, climate changes may alter the distributions and relative abundances of different predators, such as ectothermic predators like snakes, which are particularly important predators in Central American studies just cited. Direct human exploitation of insectivorous tropical birds for food, including bushmeat, is probably rare, at least in the Neotropics, because of their generally small size compared to hunted birds such as curassows, guans, trumpeters, parrots and macaws, toucans, and ducks (Peres and Nascimento, 2006).
Predation of a predator, i.e., multiple trophic levels, can result in turn in trophic cascades, which are defined as the indirect impacts of one consumer causing reciprocal changes of populations at multiple lower trophic levels. For example, predators like insectivorous birds benefit plants by controlling the insect herbivores of plants (e.g., Marquis and Whelan, 1994; Greenberg et al., 2000; Van Bael et al., 2003; Maas et al., 2016), i.e., “the enemy of my enemy is my friend.” Ecologists increasingly recognize the strength and pervasiveness of trophic cascades globally, including tropical ones, by documenting the often profound impacts of the loss of top predators, i.e., trophic downgrading (Terborgh et al., 2001; Estes et al., 2011; Terborgh, 2015). For example, Feeley and Terborgh (2008; see also Sherry, 2008) document how the loss of predators on small islands in Lago Guri, Venezuela, created dramatic increases in herbivores that reduced nesting sites available to birds. Another example involves peccaries in Central America limiting feeding habitat for insectivorous birds (see section insect declines). Terborgh (2015) also links these cascades to negative density-dependence, in which a variety of predators disproportionately limit populations of the most frequent prey, thereby contributing to the maintenance of species diversity. The loss or decline of some predators allows many prey populations to increase at the expense of others, and often at the expense of overall biodiversity, which often collapses. The conservation implications of maintaining these trophic cascades, and the evolved species relationships, are emphasized in sections extraordinary sensitivity of insectivorous tropical birds to Anthropocene and need for multiple large tropical reserves.
A special case of such trophic cascades is the Meso-predator Release Hypothesis, in which top predators lost due to overexploitation (over-hunting) or fragmentation of habitats that leave insufficiently large habitat area to maintain the top predators, release their prey populations to increase (Prugh et al., 2009). In the Neotropics, these top predators consist of cats like jaguars (Panthera onca), ocelots (Leopardus pardalis), and margays (Leopardus wiedii); harpy eagles (Harpia harpyja), and large snakes, among others. Declines of top predators from diverse ecosystems for various reasons (e.g., Estes et al., 2011; Dirzo et al., 2014) are expected to contribute to the increased abundance of mesopredators [the prey of the top predators, including a variety of snakes, opossum species, and coatimundi (Nasua narica)], which in turn depredate birds' nests, and occasional adult birds. Robinson and Sherry (2012) found this hypothesis to be plausible, albeit with little empirical support to date in most tropical forests given the large sample sizes required to show a significant effect. Another example involves the bird-eating snake (Pseustes poecilinotus), whose impacts on nesting success of tropical insectivorous understory birds is revealed by fragmentation impacts in Central America (Visco and Sherry, 2015). Terborgh (2015) argues that the top-down impacts of predators can release herbivore populations to become so abundant as to alter vegetation in ways that make it more difficult for birds to forage and conceal nests. Similarly, the decline in apex predators was hypothesized to contribute to the increase in collared peccary populations—mentioned above—that in turn caused the decline of vine tangles necessary for foraging and nesting by some insectivorous tropical birds (Michel et al., 2015a).
Habitat Loss and Fragmentation
The literature on tropical forest habitat loss and fragmentation is daunting, probably in part because this is a major cause of tropical species extirpations and extinctions, particularly for understory, forest interior bird species (Tobias et al., 2013; Powell et al., 2015; Visco et al., 2015). This research includes diverse landscapes. For example, the “arc of deforestation” in southern Amazonia is experiencing expanding agriculture and consequent loss and fragmentation of native forest habitat with important negative impacts on diverse birds (Lees and Peres, 2006, 2008, 2009, 2010; Davidson et al., 2012; Neate-Clegg and Sekercioglu, 2020) and mammals (Palmeirim et al., 2020). Moreover, tropical habitat fragmentation is predicted to accelerate in the next 50 years (Taubert et al., 2018). This Amazonian landscape illustrates how humans are ultimately responsible for habitat loss and resulting fragmentation of native habitat, such as tropical rainforest, primarily via conversion to agriculture.
Habitat loss is widely regarded as the preeminent cause of animal species population declines globally (Betts et al., 2019). Habitat loss almost invariably causes habitat fragmentation, and both engender habitat deterioration, linking all three phenomena. Habitat fragmentation can be further subdivided into reduced habitat patch area, patch isolation, diverse edge effects that themselves interact with the surrounding matrix such as agricultural habitats or secondary growth woodlands, and altered trophic structure. These combined, interrelated, and often synergistic mechanisms comprise the major class of indirect proximate causes of insectivorous tropical bird declines (Gibson et al., 2011). This is a class of mechanisms largely independent of the direct causes of threats like overexploitation of wildlife (Dirzo and Raven, 2003).
Early approaches to habitat loss began with the species-area relationship, a mathematical power function that describes species richness as a function of real island areas as well as isolated or nested subsets of habitat patches (“habitat islands”) (e.g., Desouza et al., 2001). Some tropical forest “islands” fit this species-area relationship for bird species richness (e.g., Visco et al., 2015), but often more is involved than just area effects, such as edge and matrix effects (between forest islands; e.g., Stouffer, 2020). Consideration of habitat loss, and thus area effects, should also recognize the heterogeneity of the tropics, a reality captured to some extent by conservation “hotspots” (e.g., Myers et al., 2000), defined as regions of relatively high species richness, many endemic species, and high human impact. Half these original hotspots are tropical (Laurance et al., 2014), and human pressures on these areas are intense and growing due to disproportionate human population expansion in the tropics. The stakes of habitat loss and deterioration are greatest in these hotspots.
Tropical mountains contribute importantly to environmental heterogeneity and to our understanding of species richness and endemism, and tropical montane birds often exhibit high species turnover with elevation (Jankowski et al., 2010). Tropical mountains thus constitute an additional category of conservation hotspot, namely by concentrating threatened small-range species, including Andean vertebrates (amphibians, birds, and mammals; Jenkins et al., 2013). Although much research on tropical habitat fragmentation focuses on low elevations, habitat loss, deterioration, and fragmentation are also important at higher elevations, and often impact insectivorous birds disproportionately (Restrepo and Gómez, 1998; Renjifo, 1999, 2001; Pattanavibool and Dearden, 2002; Soh et al., 2006; Kumar and O'Donnell, 2007; Colorado and Rodewald, 2015). A related conservation concern is the loss of habitat area at higher elevations associated with climate change and upwardly shifted geographic ranges (see section climate change). Fragmentation at particular elevations can also threaten the elevational movements (e.g., Forero-Medina et al., 2011) and migrations of tropical birds, but insectivorous species generally decrease in relative abundance with elevation (e.g., Blake and Loiselle, 2000). Also, many tropical elevational migrants are frugivorous as well as physiologically sensitive to changing food and weather conditions at higher elevations (Boyle et al., 2010), much as insectivores are sensitive generally.
The sensitivity of tropical insectivorous birds to forest fragmentation is particularly well-documented in the vicinity of Manaus, Brazil, where Tom Lovejoy initiated the Biological Dynamics of Forest Fragments Project (BDFFP). The importance of this project for purposes of the present study is its longevity, experimental nature, and integration of birds—and especially insectivorous birds—with plants and a variety of other components of the biotic and abiotic environment. This study took advantage of researcher-informed logging clear-cuts to produce replicate squares of lowland rainforest of 1, 10, and 100 hectares; and compared them with nearby contiguous (unfragmented) rainforest habitat as control treatment [see Bierregaard et al. (1992), Bierregaard et al. (2001), Stouffer (2020) and Stouffer et al. (2020) for recent reviews of findings most relevant to birds].
The following brief review of the avian BDFFP results emphasizes insectivorous birds. Bird crowding increased initially, particularly in the smallest forest fragments, due to an influx of individuals displaced in the process of logging nearby areas (Stouffer, 2020). Subsequently, bird communities were highly dynamic in all fragments, but particularly in the smallest ones, due largely to non-random extirpations and many recolonizations (Laurance et al., 2011, 2017; Stouffer, 2020; Stouffer et al., 2020), for various reasons including fluctuating climates over the years of the studies. Some of the bird species most susceptible to extirpation were matrix-intolerant, widely ranging species (Laurance et al., 2017); and a variety of insectivores including army ant followers, mixed species flocking species, and solitary and terrestrial species [Laurance et al., 2017; but see Stouffer et al. (2020)]. Stouffer et al. (2020) found terrestrial insectivores to be the least likely to recolonize patches, even after regrowth of the matrix. A variety of forest interior insectivores were rare in remnant forest patches, except occasionally as young birds, indicating the general decline in habitat quality within patches and the area-sensitivity of these birds. With time, regrowth of the matrix between old growth forest patches, especially Cecropia trees, facilitated recolonizations, except by non-forest bird species, and contributed to the community dynamics within patches (Stouffer, 2020). Forest patch edge effects that reduced bird abundance ameliorated over time, likely because of increased secondary growth between patches; and these edge effects were often subtle, probably involving reduced quality foraging opportunities (Stouffer, 2020). There were also particularly strong reductions in bird populations resulting from 50 to 100 m wide strips of cut vegetation, implicating isolation effects on forest interior bird populations. Stouffer et al. (2020) also found that communities within unfragmented forest continued to deteriorate, suggesting directional climate change impacts. Taken together, these studies indicate negative impacts on forest interior birds in particular, resulting from area reductions, isolation of patches, edge effects, and possibly climate change. The BDFFP thus yields many insights, especially about patterns and less about mechanisms, but the impacts of fragmentation may be relatively mild in this study system compared to other areas of Amazonia for a variety of reasons: These include fewer high-disturbance impacts in the surrounding matrix such as fire and road-building, proximity to large forested source areas for recolonizations, absence of cattle pastures in the deforested areas, and rapid revegetation of cut areas reducing the duration of experimental treatments (Barlow et al., 2006).
Sensitivity of tropical insectivorous birds to forest loss and fragmentation is widespread in the Neotropics (Maldonado-Coelho and Marini, 2004; Barlow et al., 2006; Robinson and Sherry, 2012; Tobias et al., 2013; Visco et al., 2015; Sekercioglu et al., 2019) and Paleotropics (Lens et al., 2002; Bregman et al., 2014; Powell et al., 2015; Martínez et al., 2021). This literature repeatedly emphasizes the relatively great vulnerability of tropical insectivorous birds, and adds perspective to the BDFFP results. For example, ant-following birds are generally relatively sensitive to habitat fragmentation, indicated by the loss of some or most of these ant-following birds, often in concert with declines or changes in behavior of the army ants [or driver ants in Africa; Stouffer and Bierregaard, 1995; Laurance et al., 2002; Maldonado-Coelho and Marini, 2004; Stouffer et al., 2006; Kumar and O'Donnell, 2007; Peters et al., 2008; Peters and Okalo, 2009; Bregman et al., 2014; reviewed by Martínez et al. (2021)]. Ant-following bird declines imply food declines as pivotal, because the ants make prey conspicuously available to the birds, but other factors include poor dispersal by these birds (patch isolation), large home-ranges, and edge effects on habitat quality (Visco et al., 2015). Additionally, both the army ants and ant-following birds are sensitive to changing rainfall and temperatures, making these birds vulnerable to climate change threats (Martínez et al., 2021).
Poor dispersal, and consequent isolation of tropical insectivorous bird populations within remnant forest fragments, often accompanied by declines, are a common theme (e.g., Sekercioglu et al., 2002; Stratford and Robinson, 2005; Moore et al., 2008; Woltmann et al., 2012a,b; Pavlacky et al., 2015; Visco et al., 2015; Sherry et al., 2020; Stouffer, 2020), emphasizing the importance of the BCH. Isolation of these birds within forest fragments should subject them to the well-documented small population threats, namely loss of genetic variability from genetic drift and inbreeding, and demographic stochasticity (Figure 1), although relatively few studies have documented this [but see Brown et al. (2004) and Schlaepfer et al. (2018)]. Woltmann et al. (2012a) showed significant, relatively strong genetic differentiation of an understory Neotropical insectivore population within the most isolated patch in the study, a small (80 ha) patch isolated by banana plantations for only about 60 years. A number of other studies have shown genetic differentiation of populations separated by large Neotropical rivers, if not always in the river headwaters (e.g., Weir et al., 2015). Experimental studies support the inability or reluctance of many tropical forest interior birds to cross even small breaks in forest (Robinson and Sherry, 2012). A revealing exception that helps prove the rule of poor dispersal in these birds comes from species endemic to white sands soils, which constitute natural “islands” of often low nutrient content and stunted vegetation surrounded by more lush rainforest (Capurucho et al., 2020): Birds inhabiting these long-isolated habitat patches tend to be endemic to it, and to disperse well, a trait necessitated by the long-term, natural isolation of these habitat patches (see section natural enemies and trophic cascades below).
A last potential proximal impact of tropical forest fragmentation is physiological sensitivity to changing microclimatic conditions within fragments. This should be particularly important for resident, tropical forest interior species (Stratford and Robinson, 2005; Robinson and Sherry, 2012; Betts et al., 2019) and understory species (Powell et al., 2015; Stratford and Stouffer, 2015). These microhabitats are considered relatively buffered year-round by the forest canopy, and are minimally seasonal, in lowland, low latitude forests (Kricher, 2011; Sheldon, 2019). Any such buffering of the abiotic (temperatures, humidity, rainfall, and windiness) and biotic (habitat, prey, natural enemies) environment should facilitate the persistence of specialized physiologies directly, and ecologies indirectly, both over animals' lifetimes and evolutionarily (Betts et al., 2019). As Janzen (1967) first argued in a genetic context, lower latitude animals should be poorly adapted to disperse, e.g., through a mountain pass with cool temperatures, because of weak adaptation to such variable conditions [reviewed by Sheldon (2019)]. Accordingly, tropical species should experience greater genetic differentiation across mountain ranges.
Applying this argument to fragmented landscapes, species lacking physiological flexibility could suffer demographically when conditions fluctuate in a fragment, particularly when dispersal is precluded (e.g., Karr and Freemark, 1983). Fragments could exacerbate this effect due to edge effects, which can extend up to 400 m inside a habitat patch from the edge (Laurance et al., 2002; Betts et al., 2019). Edge effects typically warm and desiccate forest habitat, and decrease the forest flammability threshold (Barlow et al., 2006), potentially enough to stress birds [Visco et al., 2015; but see Pollock et al. (2015)]. Despite these predictions about potential physiological sensitivity of tropical birds, recent empirical studies of tropical birds fail to support this physiological sensitivity (e.g., Khaliq et al., 2014; Freeman, 2016; Londoño et al., 2017; Pollock et al., 2020), making it more likely that ecological changes, such as food and predators, associated with forest fragmentation will impact birds sooner than physiological.
Agriculture
Conversion of tropical rainforest to agriculture including pastures, and thus increasing forest fragmentation, constitutes one of the most important threats to tropical insectivorous birds, as discussed above (Figure 1). Some agricultural land uses such as selectively logged forests and some agro-forestry and mixed cropping protect some biodiversity, and mitigate against climate change (e.g., Porro et al., 2012; Buechley et al., 2015; see section climate change), but most agriculture has strongly negative impacts that are increasing, particularly where the human population is growing most in sub-Saharan Africa and Latin America (Sekercioglu, 2012; Laurance et al., 2014; Raven and Wagner, 2021). Agriculture is also particularly important regionally in the southeastern portion of Amazonia (Davidson et al., 2012). Agriculture threatens not just tropical forests, but also wetlands, savannas, and seasonal woodlands like Brazil's Pantanal and Cerrado regions. All forms of agriculture degrade biodiversity, particularly specialized forest interior birds including insectivores (Laurance et al., 2014; Neate-Clegg and Sekercioglu, 2020). The worst agricultural land uses from this perspective, are monocultures, including plantations of Eucalyptus (Barros et al., 2019; Neate-Clegg and Sekercioglu, 2020), rubber, oil palm (Lees et al., 2015; Neate-Clegg and Sekercioglu, 2020), sugar cane, and soybeans. Agricultural expansion is exacerbated by new technological advances, such as tropically adapted soybeans that are in especially high demand by humans (Laurance et al., 2014). Pesticide-dependent crops like pineapples and bananas are insidious to biodiversity due to pesticide drift that can kill non-target arthropods. Many agricultural threats to biodiversity interact with other threats. High demand for inexpensive beef, particularly for fast food restaurants in developed countries, fueled both high rates of deforestation contributing to both biological deserts (Neate-Clegg and Sekercioglu, 2020) and emissions of methane, a far more potent greenhouse gas than CO2. Agricultural expansion and roadbuilding typically advance in concert, and both in turn foster direct exploitation of wildlife, e.g., bushmeat, as humans expand into formerly forested areas (Laurance et al., 2014).
Moreover, intensification of crop production that could minimize the need for new agricultural lands depends on inexpensive energy, which will need to become less carbon-dependent in order to reduce greenhouse gas emissions, and thus not exacerbate climate change impacts (Raven and Wagner, 2021). Climate change in turn threatens to reduce crop production locally (Laurance et al., 2014), creating demand for more deforestation, contributing to a positive feedback loop for habitat loss.
Fire
Fire contributes significantly to habitat loss and modification impacting Neotropical birds (Barlow et al., 2006), and understory insectivores in Sumatra, which are replaced by open field species (Adeney et al., 2006). Fire also impacts tropical rainforest birds indirectly by influencing the habitat matrix between fragments: Cecropia secondary growth, which rapidly formed woodlands with continuous canopy, facilitated bird movement between forest patches, whereas Vismia secondary growth, which dominated previously burned pasture areas did not facilitate such movement as much (Stouffer, 2020). Neotropical fires have diverse causes and consequences (Barlow et al., 2020), but most are anthropogenic (Cochrane and Barber, 2009). Fires are exacerbated by multiple factors, including road-building in both unprotected and protected areas (particularly in close proximity to roads; Adeney et al., 2009; Silvestrini et al., 2011; Laurance et al., 2014), increased forest fragmentation often associated with increased roads and pastures, and the warming and drying that result locally from climate change (Davidson et al., 2012; Laurance et al., 2014; Barlow et al., 2020) and El Niño-Southern Oscillation (ENSO) and other droughts that are potentially increasing in intensity with climate change (Laurance and Williamson, 2001; Cochrane and Barber, 2009; Davidson et al., 2012). Amazonian fires are particularly forest habitat-destructive because of positive feedbacks in the susceptibility to fire and fire intensity with recurrence; and fires' importance is often underestimated because of misclassification of satellite imagery as deforested land that was actually burned (Cochrane et al., 1999). Besides short-term habitat destruction involving many positive feedbacks of all the foregoing factors (Laurance and Williamson, 2001; Davidson et al., 2012), fires convert biomass carbon to CO2, accelerating the atmospheric accumulation of greenhouse gases (Ometto et al., 2011), which thereby contributes to another positive climate change feedback loop.
Fire is also important to the evolution and ecology of diverse tropical ecosystems other than rainforests. Specifically, fire is critical to understand the plant forms and distinctive species composition of a variety of lowland environments globally that are more seasonal than equatorial rainforests. In the Neotropics these range from grasslands to savannas, seasonally dry and scrub woodlands, and even deserts; and include pine savannas (in Central America and the Caribbean), and the Llanos, Pantanal, Campos Cerrados, and Caatinga. These diverse ecosystems are shaped by complex interactions of quantity and seasonality of rainfall, fire, soil properties, herbivory, and humans both prehistorically and presently [reviewed by Kricher (2011)]. The avifaunas of tropical fire-maintained ecosystems are as distinctive as their plants (e.g., Prado and Gibbs, 1993; da Silva and Bates, 2002; Marini et al., 2009; Franchin et al., 2017; Norambuena and Van Els, 2020). Threats to avifaunas in these areas, especially Cerrado ecosystems—comprising a conservation savanna hotspot (Franchin et al., 2017)—include conversion to agriculture, especially soybeans (Kricher, 2011), and Climate change (Marini et al., 2009).
Climate Change
Climate change is likely the single most potent threat to the future of tropical insectivorous birds, as indicated by four different arrows connecting it to all the other categories of environmental degradation (Figure 1). Climate change is part of the ultimate, Anthropocene threats to overall biodiversity, as well as causing a variety of proximate changes in temperature and rainfall that exacerbate threats to biodiversity from agriculture, reductions in arthropod abundance, and habitat loss and deterioration from multiple mechanisms including fire. Climate change will interact with habitat fragmentation differently in different landscapes, leading to variable and difficult-to-predict community trajectories (Davidson et al., 2012), and likely leading to many more extinctions than attributable to either factor alone (Forero-Medina et al., 2011; Laurance et al., 2017). Interactions of local (fragment size, shape, edge, and matrix) and larger scale phenomena (directional climate change, rare meteorological events like droughts, intense rain, and windstorms) are all a part of climate change impacts. Recent long-term studies have detected directional changes to bird communities even in non-fragmented “control” areas in Brazil (Stouffer et al., 2020) and Ecuador (Blake and Loiselle, 2015). Stouffer et al. provide evidence for declines in ground-level leaftossers (genus Sclerurus) and upward-strikers feeding on leaf undersides, implicating changing substrate availability in tropical insectivores. Brawn et al. (2017) point to increasing dry season length in Panama as an important cause of demographic problems in rainforest birds, also independent of habitat loss. Tropical species are also particularly vulnerable to climate change due to their restricted geographic options, for example in the Andes Mountains (Colwell et al., 2008; Sekercioglu et al., 2012; Jenkins et al., 2013; Freeman et al., 2018), but the foregoing examples illustrate that far more is afoot than simply altered climate envelopes shrinking—e.g., lowland biotic attrition (Colwell et al., 2008)—or shifting off of mountain tops. Sekercioglu et al. (2012) projected that as many as 100–500 bird species may go extinct for every 1°C increase in temperature, and most of these will be tropical birds. Most of the few studies addressing rates of range shifts in tropical mountains find that species range shifts are unlikely to keep up with climate shifts (Sheldon, 2019). We have much to learn about the mechanisms of directional climate threats, necessitating coordinated, long-term studies of climate change impacts on tropical montane forest birds (Neate-Clegg et al., 2018, 2021).
These just mentioned studies of otherwise undisturbed tropical rainforest are the climate-change canary in the coal mine. Climate change threatens to overwhelm all the other mechanisms of decline in these birds. Increased temperatures associated with climate change may be greatest at high latitudes, but the diverse manifestations of climate change profoundly threaten tropical ecosystems where so many species are vulnerable, and in some cases highly sensitive. These threats include increasingly frequent and extended (“100-year”) droughts, altered rainfall including heavy downpours in unexpected areas, and even seemingly miniscule temperature increases that can reduce tree growth (Clark et al., 2003, 2010; Davidson et al., 2012; Wagner et al., 2014).
Invasive Species
Invasive species are another factor causing insectivorous tropical bird declines (Sodhi et al., 2004; Dayer et al., 2020). Invasive bird species can threaten other birds, and create a variety of indirect effects (Sodhi et al., 2004, 2007a). Invasive mammals such as rats and cats are especially destructive, particularly on islands (Harper and Bunbury, 2015; Dayer et al., 2020). Three species of invasive rats, and particularly black rats (Rattus rattus), have caused documented declines and/or extinctions, including a number of insectivorous bird species, on a variety of tropical islands globally; and have likely caused undetected avian extinctions due to how much earlier these rats reached tropical islands than humans could document bird species there (Harper and Bunbury, 2015).
Invasive grass species that accumulate biomass contribute to the intensity and frequency of habitat-destructive fires (Sodhi et al., 2007a, 2011), which are likely contributing to Neotropical forest loss (see Section fire). For example, invasive Melinis minutiflora grass is associated with greater fire intensity and gallery forest loss in Brazilian Cerrado (Hoffmann et al., 2004), reducing habitat for insect herbivores and birds. A variety of invasive pathogens threaten birds, as illustrated by West Nile Virus (e.g., Pinto et al., 2008; George et al., 2015), and avian malaria that has devastated Hawaiian endemic birds (e.g., Samuel et al., 2015). Neotropical birds also suffer from various diseases (Pinto et al., 2008; Sehgal, 2010; Blake and Loiselle, 2015).
Conservation Implications and Recommendations
The fundamental argument of this review is that the better we understand the diverse threats to biodiversity, focusing here on tropical insectivorous birds, the better we can protect it. Tropical insectivorous birds are highly sensitive to human activities, i.e., to the Anthropocene, as emphasized by the BCH, but understanding the causes, and their relative importance better is challenging considering their variety and many indirect pathways (Figure 1). The variety of mechanisms and their interactions (Figure 1) suggests seven conservation implications.
Extraordinary Sensitivity of Insectivorous Tropical Birds to Anthropocene
First, multiple causes of this sensitivity are important, as summarized in Figure 1, that are complimentary, potentially synergistic, and in many cases interrelated—a perfect storm of threats. Examples of complimentary threats include forest habitat loss plus fragmentation, intensification of agriculture including the use of pesticides, potential declines in insect prey at least locally as documented in Costa Rica, loss of foraging substrates such as lianas and habitat patch edges, meso-predator release, and climate change. Every one of these proximate mechanisms is likely important in particular circumstances, and these proximate mechanisms generally apply to bats, which, like insectivorous birds, peak in species richness in the tropics (Frick et al., 2019). Added to these proximate threats are the extraordinarily poor dispersal ability of many tropical forest interior insectivorous birds and their specialized, stereotyped foraging behaviors and physiological sensitivity to altered conditions, explained by the BCH (Sherry et al., 2020). A potential example of synergistic threats, best understood in this evolutionary context, is the isolation of suitable forest interior habitat in fragmented landscapes—essentially habitat jails to species incapable of dispersing—coupled with pesticide drift from the growing intensive agricultural activities in the habitat matrix, including crops such as bananas, pineapples, and oil palms. That said, we are far from understanding which tropical insectivorous birds are declining, where, and why. For example, far less is known about montane and seasonal tropical forests than the wet tropics. We also need to better understand the natural history of tropical communities, including the arthropods, the birds that feed on them (and their diets). Monitoring these birds and insects, and linking real ecological trends with potential direct and indirect causes will be critical to prescribe specific remedies. Wagner et al. (2021) emphasize the need for standardized long-term monitoring of insects, particularly in the tropics, which is challenging due to funding shortages, plus hyperdiversity of tropical insects coupled with a large proportion of undescribed species—probably at least 85% of all tropical insects (Raven and Wagner, 2021). A critical next step in tropical insectivorous bird conservation is prioritization of the threats, which will be facilitated by the relatively low proportion (<4%) of “data deficient” bird species globally according to International Union for Conservation of Nature (IUCN) data—see Frick et al. (2019) for an example with bats.
Ultimate vs. Proximate Threats
Second, the distinction of ultimate and proximate threats merits increased attention. The proximate direct threats are relatively straightforward to detect, and in some cases to address. For example, trophic downgrading of tropical forests due to loss of top predators like jaguars and harpy eagles can be addressed in theory with large reserves (see section need for multiple large tropical reserves). Poor dispersal by many tropical forest interior insectivorous birds is amenable to mitigation via corridors between viable habitat patches (Lees and Peres, 2008, 2009), minimizing edge effects like fire and threats within the surrounding matrix, establishing larger reserves without dispersal barriers, restoring native rainforest habitat, and switching to organic agriculture. These are all possible, if economically and politically challenging. The direct threat of declining tropical insects will be more challenging to address given the growing impacts of accelerating agricultural intensification plus climate change (Raven and Wagner, 2021).
The first of the two ultimate threats, the totality of Anthropocene human global change impacts, particularly climate change and loss of biodiversity, is extremely challenging by implicating the need to change entrenched political and economic activities. Changing these will be strongly resisted, necessitating, for example, reducing the human population and our global carbon footprint. This is a “wicked problem” (O'Brien, 2017) involving diverse, entrenched human institutions.
Indirect threats like trophic cascades, and synergistic threats will be particularly difficult to address because these are arguably challenging to document. These mechanisms warrant particular research focus, such as investigating predators and nest predators of birds, the mesopredator-release hypothesis, and the role of predators generally maintaining diversity in tropical terrestrial ecosystems (Robinson and Sherry, 2012; Terborgh, 2015).
Threats Arising From Life-Histories and Rarity
Third, tropical insectivorous birds' life-histories (Stratford and Robinson, 2005; Betts et al., 2019; Sherry et al., 2020) provide important risk factors that amplify vulnerability to the Anthropocene. These life-history traits also unify a variety of what have often been treated as independent threats: poor dispersal ability, stereotyped foraging (Sherry, 1990), and relative rarity (Figure 1). Consequences of poor dispersal and stereotyped foraging specialization are emphasized above by the BCH (section species life-histories). An important future need is to identify more precisely which species disperse poorly, and why, including detailed physiological constraints to flight so as to help establish mechanisms (Robinson et al., 2021).
Rarity of many insectivorous tropical birds emphasizes this third implication for conservation, and itself has multiple causes. Low population densities of Neotropical, forest-dependent birds are often associated with relatively large home ranges (Terborgh et al., 1990; Stratford and Robinson, 2005) probably in part due to sparse effective food availability, and to the higher species richness in Amazonian sites—closer to the Equator—compared to Panamanian (Robinson et al., 2000). Tropical latitudes may appear lush with nectar, fruit, seeds, and diverse kinds of animal protein—especially of insects—due to reduced temperature seasonality, and even reduced rainfall seasonality close to the equator, and thus year-round availability of these resources in the least seasonal locations. However, birds share these resources, and thus compete diffusely with diverse other birds, and other organisms. Insectivorous birds compete with hundreds of other insectivorous birds for insects, as well as with diverse tropical bats, rodents, small primates, amphibians, snakes, other insects, and even plants (Sherry et al., 2020). Moreover, tropical insectivorous birds comprise a relatively reduced biomass because the largest-bodied species tend to be granivores and frugivores, emphasizing the challenge of resource scarcity for insectivorous bird species (W.D. Robinson, pers. comm.).
Insectivorous tropical bird rarity in South America is exacerbated by low soil fertility in high-precipitation, ancient, and thus highly weathered soils, such as found in the Guiana Shield and parts of Central and Eastern Amazonia. Such soils yield relatively low primary productivity, which limits the biomass available to higher trophic levels (Laurance et al., 1999, 2017) and limits plant productivity overall (Huston, 2012). Patchy edaphic conditions also contribute to low-density populations of tropical species (Laurance et al., 2011, 2017). White sand soils are relatively extreme in terms of low soil fertility and low bird diversity.
Tropical insectivorous birds' life-histories also help explain the rarity that makes these birds vulnerable to the Anthropocene (Pavlacky et al., 2015). A variety of these birds are adapted to a restricted set of arthropod taxa and/or prey substrates (Supplementary Table 2). Many arthropod prey are well-defended against visually hunting insectivores, such as most birds, making these prey effectively unavailable to most birds (Sherry et al., 2020). Many of these substrates are patchily available. For example, Checker-throated Antwrens (Epinecrophylla fulviventris), Dot-winged Antwrens (Microrhopias quixensis), and Ruddy-tailed Flycatchers (Terenotriccus erythrurus) depend on lianas and vines to concentrate the arthropod foods on which these birds feed (Michel et al., 2015a; Schnitzer et al., 2020), and potentially for safe nesting sites from snakes and other nest predators. In old-growth tropical forests lianas and vines are limited to disturbances like old treefall gaps, making this microhabitat temporally and spatially patchy. Another example: Some Army-ant-following birds, which compete intensely for food at the foraging front of the ants, have large, albeit overlapping home ranges, linked to the challenges of finding enough food at enough ant swarms (e.g., Sherry, 2016). Rufous-tailed Jacamars (Galbula fulvicauda), which feed on many other insects than butterflies, but are particularly reliant on them as relatively large prey, feed on just the palatable butterfly species, a fraction of the total (Pinheiro and Campos, 2019). The rarity of these, and many other groups of insectivorous birds exacerbates the impacts of population isolation resulting from habitat fragmentation, and that contribute to demographic and genetic bottlenecks well-known to threaten small, isolated populations (e.g., Westemeier et al., 1998; Woltmann et al., 2012a,b).
Tropical Heterogeneity
Fourth, tropical communities appear to differ considerably both within and among continents. South America probably accumulated greater species richness than Paleotropical and Australian tropical regions because of its relatively long history as a tropical landmass (Betts et al., 2019), its large area, and its many mountains—especially the Andes Mountains—and rivers, including tributaries to the Amazon, all of which likely contributed to speciation rates exceeding extinction rates long enough to allow the net accumulation of insectivorous bird clades (Sherry et al., 2020). Tropical regions on other continents have fewer species per unit area, and as a result may have fewer specialists with the life history traits that make Neotropical insectivorous birds so sensitive to human impacts (Figure 1). Thus, history and geography matter. In the extreme, small and isolated oceanic islands at tropical latitudes have very few species, that are extraordinary generalists, as illustrated by the Cocos Finch of Costa Rica (Werner and Sherry, 1987; Sherry, 2016). Species in different regions should be differentially sensitive to many of the threats to biodiversity depicted in Figure 1. Dispersal abilities, degree of evolutionary specialization, physiological traits, extent of migration vs. residency, and other traits differ considerably within and among continents, and should caution us against simplistic comparisons involving species richness. Much of the research cited in the present review comes from the lowland wet tropics, implicating far more research needed in seasonal, drier, and higher elevation tropical regions (Sheldon, 2019).
Expanding Understanding of Biodiversity
Fifth, an implication of including the ultimate evolutionary threats to species, as illustrated by insectivorous tropical birds, is the need to broaden the definition of biodiversity, a pivotal concept in conservation biology. A typical definition of biodiversity is “the complete range of species, biological communities, and their ecosystem interaction and genetic variation within species” (Sher and Primack, 2019). The inclusion of biological communities in this definition, and implicit ecological species interactions, emphasize ecological, and too infrequently evolutionary species interactions [but see Jarzyna and Jetz (2016) and Jarzyna and Jetz (2017)]. The present review reinforces the need to better incorporate evolutionary time, evolutionary species interactions like enhancement, genetic distinctiveness, and related species traits that impact vulnerability to Anthropocene threats.
Need for Multiple Large Tropical Reserves
Sixth, it is imperative to establish multiple, large reserves in the tropics, not a novel recommendation. This follows from the species-area relationship, in which larger areas (whether parts of reserves or of unfragmented landscapes) tend to contain more species (e.g., Brook et al., 2003). The multiple, interconnected proximate and ultimate threats to insectivorous tropical birds (Figure 1) recognized in the present study also emphasize the need for large reserves: The threats emphasized here include large home ranges and rarity of many species, patchy distributions, low primary productivity (and resources) in areas with infertile soils (and high elevations), specialization on rare arthropods or feeding substrates, weak bird dispersal, and small geographic ranges particularly in Neotropical mountains. Large reserves also minimize edge effects that threaten tropical species in particular (Betts et al., 2019), and protect against forest-destructive fires (Cochrane and Barber, 2009). Reserves must also be comprised of intact ecosystems like primary forest (e.g., Renjifo, 1999; Gibson et al., 2011; Watson et al., 2018), which provide the ecological conditions where organisms evolved, and where both top-down (trophic cascades) and bottom-up (prey base) ecological relationships are maintained intact.
Large reserves may not by themselves protect species, given projected climate changes that will necessitate topographic complexity that encompasses climate refugia (Blake and Loiselle, 2015; Brawn et al., 2017; Raven and Wagner, 2021). Large reserves, particularly indigenous and limited-human-impact ones, also minimize tropical moist forest-destructive fires that are associated with roads, fragmentation, agriculture, and other human impacts (Adeney et al., 2009; Cochrane and Barber, 2009). Directional changes in species abundances and species richness, even in relatively non-fragmented landscapes, indicate the critical need for systematic monitoring, especially in large tropical study areas (Robinson and Curtis, 2020). Large reserves protect the most biologically diverse terrestrial environments on the planet, diverse human cultures, and potential carbon sinks to buy humans time to address climate change (Ometto et al., 2011).
Importance of Species Values
A seventh and final conservation implication of the diverse proximate and ultimate threats to insectivorous tropical birds derives from their values. Values are necessary for conservation action by prioritizing and protecting biodiversity. The kinds and strengths of values humans associate with biodiversity cut both ways: They allow the degradation of nature, and all the threats to insectivorous tropical species reviewed in this paper, which is why the Anthropocene impacts are stressed here as one ultimate cause of the threats. Values also link to human culture (Figure 2), and thus provide the information that can inform societies to transform themselves enough to protect biodiversity (Johnson and Hackett, 2016). Better understanding of these values can fuel hope, which motivates increased conservation action (O'Brien, 2010).
The most straightforward values to understand, and probably best studied, are utilitarian or instrumental, i.e., those important to humans (Figure 2). Of these, ecosystem services are relatively well-studied, including those provided by tropical birds (Michel et al., 2020). Although much remains to be learned about insectivorous birds' ecosystem services, a conspicuous one is regulatory, via pest control. Insectivorous birds annually consume an estimated 400–500 million metric tons of prey globally, 75% of which involves forest-based birds, especially during the nesting season when demand for protein food to feed nestlings is greatest (Nyffeler et al., 2018). Moreover, tropical forests account for almost half (48.7%) of this consumption, due to the relatively high diversity of birds and large area of tropical forests globally, and a substantial proportion of this consumption targets herbivorous insects. By such consumption tropical birds benefit plants via trophic cascades (section species life-histories). For example, tropical vertebrates reduced abundance of predatory and herbivorous vertebrates by 38 and 39%, respectively, reducing the damage to plants by 40% [Mooney et al., 2010; cited by Powell et al. (2015)]. Insectivorous tropical birds consume enough herbivorous arthropods to favor particular plants via trophic cascades (Michel et al., 2014, 2015b). Investment by tropical plants in defenses against herbivores are substantial, reinforcing humans' appreciation for herbivores' role evolutionarily shaping plant speciation and coexistence patterns, and likely tropical plant communities, exemplified by Becerra (2007) for Bursera (Burseraceae); Kursar et al. (2009), Endara et al. (2017), and Coley and Kursar (2014) for Inga (Fabaceae); Fine et al. (2013) for Protium (Proteaceae); and Sedio et al. (2018) for Psychotria (Rubiaceae). Tropical insectivorous birds benefit humans directly as consumers of agricultural pests, exemplified by coffee (Johnson et al., 2009; Karp et al., 2013; Sherry et al., 2016).
Tropical birds also provide cultural services, in support of education and ecotourism, that provide substantial economic value for many tropical countries. A variety of insectivorous tropical birds are both charismatic (e.g., jacamars, some tanagers) and rare, both of which attract eco-tourists and their economic assets. The impending extirpation and extinction of tropical insectivorous bird species will deprive humanity of all their values, not to mention the possibility to understand how the most species-diverse tropical communities on the planet arose, emphasizing these birds' educational value: “The simplification of habitats by humans will be more devastating in the tropics than in the temperate zone because biotic interactions have shaped a behavioral and morphological diversity in tropical birds that is far richer than that found in temperate zone birds. Biotic interactions produce complex evolutionary results of the sort most interesting to behavioral ecologists” (Stutchbury and Morton, 2001, p. 130). Janzen and Hallwachs (2021) describe BioAlfa, a Cost Rican bioliteracy program, in the context of insects, but the same applies to birds: This exemplifies the kind of social paradigm shift necessary to protect biodiversity.
A fundamental tenet of Conservation Biology is species' existence value (Johnson and Hackett, 2016; Picolo, 2017; Prendergast, 2020). This value derives from evolutionary history, an emphasis in the present review. Better understanding of the long evolutionary history of many tropical birds, and thus their enhanced intrinsic value, will inform us of fundamental questions about the origins of biodiversity, and how profoundly human global change threatens it (Figure 2). To the extent that Neotropical insectivorous birds are highly specialized evolutionarily (Sherry et al., 2020), they are irreplaceable, each species adapted to its particular environment, a survivor in some cases of tens of millions of years of history and intense tropical species interactions. Awareness of the antiquity and unique evolutionary specializations of these birds will hopefully enhance their value in the same way that human cultural artifacts often acquire value with age and uniqueness.
Moreover, the very evolutionary specializations of many tropical insectivorous bird species inform us extraordinarily about the functions of their adaptations: Foraging specialization clarifies the nature of form-function relationships, e.g., to a particular prey type or substrate, which is less obvious in generalist species. Evolutionary antiquities, including relict species and “living fossils” likely also contain distinctive genomes, informative and potentially useful in themselves. We cannot easily “re-evolve” extinct species by way of restoring habitats, because the “habitat” of these species for tens of millions of years is often comprised of all the other species with which these insectivorous birds co-evolved, including their competitors, prey, and diverse feeding substrates (Sherry et al., 2020).
The combination of the vulnerability of tropical insectivorous birds to multiple Anthropocene threats coupled with their arguably incalculable—and at present inestimable—value emphasize the urgency of effective conservation action. Tropical deforestation rates are presently increasing, particularly in Brazil (https://blog.globalforestwatch.org/data-and-research/global-tree-cover-loss-data-2020/), and many threats discussed in this paper are also increasing, or are likely to increase in the future, given increased tropical forest loss, human agriculture necessitated by the growing human population, and myriad climate-related threats. Keeping Earth's average temperature below 1.5°C, so as to avoid massively increased climate and related ecosystem disruptions, will require nearly halving humans' carbon emissions from 2010 levels by the year 2030, less than a decade hence (IPCC, 2018).
Summary and Conclusions
Tropical insectivorous birds, particularly those adapted to forest interior environments, are widely recognized as disproportionately sensitive to diverse human Anthropocene activities. I have argued that these declines should no longer surprise us, considering the number of both ultimate and proximate threats, both direct and indirect, and the growing intensity of many of these. Ultimate mechanisms include both the human dimension—that has thrust us into the Anthropocene—and the more subtle evolutionary history that has predisposed these tropical insectivorous birds to be extraordinarily vulnerable to the Anthropocene threats. Proximate mechanisms include declining insect populations, altered trophic structure, loss and fragmentation of tropical forest habitats, and climate change. We know enough about tropical insectivorous birds, and the stakes of protecting them, to warrant immediate strong conservation actions. Protecting these birds is important because of their intrinsic value as well as their strong impacts on plants via herbivorous insects, including economically important plants.
The threats to tropical insectivorous birds are daunting, and require fundamental changes in how humans understand and interact with the biosphere (Figure 2). Understanding the multiple, interacting mechanisms of decline in these animals, both the ultimate and proximate evolutionary mechanisms, is an important advance. A thorough, prioritized set of recommendations is beyond the scope of this paper, but the diversity and interconnectedness of threats identify seven conservation lessons and recommendations.
Understanding the mechanisms of insectivorous tropical birds' declines is necessary, but not sufficient to protect their extraordinary biodiversity and species interactions. The necessary conservation actions will require intense effort and strong collaboration with social scientists and the instigators of cultural change. Communicating the uniqueness and values of insectivorous birds and their ecosystems is needed at every scale, from local communities and schools to national and international decision makers. We need more and better storytellers, to help the scientific community communicate the stakes of protecting these organisms, along with their extraordinary history and resulting sensitivity to diverse human impacts.
Data Availability Statement
The original contributions presented in the study are included in the article/Supplementary Material, further inquiries can be directed to the corresponding author.
Author Contributions
The author confirms being the sole contributor of this work and has approved it for publication.
Funding
NSF LTREB grants 1242584, 1242588, and 8008614 Tulane University, Department of Ecology and Evolutionary Biology Charles Bullard Fellowship, Harvard University.
Conflict of Interest
The author declares that the research was conducted in the absence of any commercial or financial relationships that could be construed as a potential conflict of interest.
Acknowledgments
I thank the editors of this special feature for the invitation to participate. Writing of this manuscript was supported by the Department of Ecology and Evolutionary Biology, Tulane University. The research supporting this effort spans decades, and includes many colleagues, mostly recently C. M. Kent, R. T. Holmes, and Ç. Sekercioglu. I am also grateful for other organizations that helped fund my research on tropical birds, especially the University of California at Los Angeles (and my dissertation supervisor, H. A. Hespenheide) and the Organization for Tropical Studies, the U.S. National Science Foundation, and a Charles Bullard Fellowship from Harvard University. I thank all three reviewers for numerous constructive criticisms and suggestions that improved the manuscript substantially.
Supplementary Material
The Supplementary Material for this article can be found online at: https://www.frontiersin.org/articles/10.3389/fevo.2021.662873/full#supplementary-material
References
Adeney, J. M., Christensen, N. L. Jr., and Pimm, S. L. (2009). Reserves protect against deforestation fires in the Amazon. PLoS ONE 4:e5014. doi: 10.1371/journal.pone.0005014
Adeney, J. M., Ginsberg, J. R., Russell, G. J., and Kinnaird, M. F. (2006). Effects of an ENSO-related fire on birds of a lowland tropical forest in Sumatra. Anim. Conserv. 9, 292–301. doi: 10.1111/j.1469-1795.2006.00035.x
Barlow, J., Berenguer, E., Carmenta, R., and França, F. (2020). Clarifying Amazonia's burning crisis. Global Change Biol. 26, 319–321. doi: 10.1111/gcb.14872
Barlow, J., Peres, C. A., Henriques, L. M. P., Stouffer, P. C., and Wunderle, J. M. (2006). The responses of understorey birds to forest fragmentation, logging and wildfires: an Amazonian synthesis. Biol. Conserv. 128, 182–192. doi: 10.1016/j.biocon.2005.09.028
Barros, F. M., Peres, C. A., Pizo, M. A., and Ribeiro, M. C. (2019). Divergent flows of avian-mediated ecosystem services across forest-matrix interfaces in human-modified landscapes. Landscape Ecol. 34, 879–894. doi: 10.1007/s10980-019-00812-z
Becerra, J. X. (2007). The impact of herbivore-plant coevolution on plant community structure. Proc. Natl. Acad. Sci. U. S. A. 104, 7483–7488. doi: 10.1073/pnas.0608253104
Benchimol, M., and Peres, C. A. (2014). Predicting primate local extinctions within “realworld” forest fragments: a pan-neotropical analysis. Am. J. Primatol. 76, 289–302. doi: 10.1002/ajp.22233
Betts, M. G., Wolf, C., Pfeifer, M., Banks-Leite, C., Arroyo-Rodríguez, V., Bandini Ribeiro, D., et al. (2019). Extinction filters mediate the global effects of habitat fragmentation on animals. Science 366, 1236–1239. doi: 10.1126/science.aax9387
Bierregaard, R.O. Jr., Gascon, C., Lovejoy, T.W., and Mesquita, R.C.G. (2001). Lessons From Amazonia: The Ecology and Conservation of a Fragmented Forest. New Haven, CT: Yale University Press.
Bierregaard, R. O. Jr., Lovejoy, T. E., Kapos, V., Dossantos, A. A., and Hutchings, R. W. (1992). The biological dynamics of tropical rainforest fragments. BioScience 42, 859–866. doi: 10.2307/1312085
Blake, J. G., and Loiselle, B. A. (2000). Diversity of birds along an elevational gradient in the Cordillera Central, Costa Rica. Auk 117, 663–686. doi: 10.1093/auk/117.3.663
Blake, J. G., and Loiselle, B. A. (2015). Enigmatic declines in bird numbers in lowland forest of eastern Ecuador may be a consequence of climate change. PeerJ 3:e1177. doi: 10.7717/peerj.1177
Boyle, W. A., Norris, D. R., and Guglielmo, C. G. (2010). Storms drive altitudinal migration in a tropical bird. Proc. Roy. Soc. B-Biol. Sci. 277, 2511–2519. doi: 10.1098/rspb.2010.0344
Brawn, J. D., Benson, T. J., Stager, M., Sly, N. D., and Tarwater, C. E. (2017). Impacts of changing rainfall regime on demography of tropical birds. Nat. Clim. Change 7, 133–136. doi: 10.1038/nclimate3183
Bregman, T. P., Sekercioglu, C. H., and Tobias, J.A. (2014). Global patterns and predictors of bird species responses to forest fragmentation: implications for ecosystem function and conservation. Biol. Conserv. 169, 372–383. doi: 10.1016/j.biocon.2013.11.024
Brook, B. W., Sodhi, N. S., and Ng, P. K. L. (2003). Catastrophic extinctions follow deforestation in Singapore. Nature 424, 420–423. doi: 10.1038/nature01795
Brown, L. M., Ramey, R. R., Tamburini, B., and Gavin, T. A. (2004). Population structure and mitochondrial DNA variation in sedentary Neotropical birds isolated by forest fragmentation. Cons. Gen. 5, 743–757. doi: 10.1007/s10592-004-1865-x
Buechley, E. R., Sekercioglu, Ç. H., Atickem, A., Gebremichael, G., Ndungu, J. K., Mahamued, B.A., et al. (2015). Importance of Ethiopian shade coffee farms for forest bird conservation. Biol. Conserv. 188, 50–60. doi: 10.1016/j.biocon.2015.01.011
Capurucho, J. M. G., Ashley, M. V., Tsuru, B. R., Cooper, J. C., and Bates, J. M. (2020). Dispersal ability correlates with range size in Amazonian habitat-restricted birds. Proc. Roy. Soc. B-Biol. Sci. 287:1450. doi: 10.1098/rspb.2020.1450
Cardoso, P., Barton, P. S., Birkhofer, K., Chichorro, F., Deacon, C., Fartmann, T., et al. (2020). Scientists' warning to humanity on insect extinctions. Biol. Conserv. 242, doi: 10.1016/j.biocon.2020.108426
Carreira, D. C., Dáttilo, W., Bruno, D. L., Percequillo, A. R., Ferraz, K. M. P. M.B., and Galetti, M. (2020). Small vertebrates are key elements in the frugivory networks of a hyperdiverse tropical forest. Sci. Rep. 10:10594. doi: 10.1038/s41598-020-67326-6
Ceballos, G., Ehrlich, P. R., and Dirzo, R. (2017). Biological annihilation via the ongoing sixth mass extinction signaled by vertebrate population losses and declines. Proc. Natl. Acad. Sci. U. S. A. 114, E6089–E6096. doi: 10.1073/pnas.1704949114
Charnov, E., Orians, G. H., and Hyatt, K. (1976). Ecological implications of resource depression. Am. Nat. 110, 247–259. doi: 10.1086/283062
Clark, D. A., Piper, S. C., Keeling, C. D., and Clark, D. B. (2003). Tropical rain forest tree growth and atmospheric carbon dynamics linked to interannual temperature variation during 1984–2000. Proc. Natl. Acad. Sci. U. S. A. 100, 5852–5857. doi: 10.1073/pnas.0935903100
Clark, D. B., Clark, D. A., and Oberbauer, S. F. (2010). Annual wood production in a tropical rain forest in NE Costa Rica linked to climatic variation but not to increasing CO2. Glob. Change Biol. 16, 747–759. doi: 10.1111/j.1365-2486.2009.02004.x
Clavel, J., Julliard, R., and Devictor, V. (2010). Worldwide decline of specialist species: toward a global functional homogenization? Front. Ecol. Environ. 9, 222–228. doi: 10.1890/080216
Cochrane, M. A., Alencar, A., Schulze, M. D., Souze, C. M. Jr., Nepstad, D. C., Lefebvre, P., et al. (1999). Positive feedbacks in the fire dynamic of closed canopy tropical forests. Science 284, 1832–1835. doi: 10.1126/science.284.5421.1832
Cochrane, M. A., and Barber, C. P. (2009). Climate change, human land use and future fires in the Amazon. Glob. Change Biol. 15, 601–612. doi: 10.1111/j.1365-2486.2008.01786.x
Coley, P. D., and Kursar, T. A. (2014). Ecology: On tropical forests and their pests: interactions with pest species may help to explain the high plant species diversity in tropical forests. Science 343, 35–36. doi: 10.1126/science.1248110
Colorado, G. J., and Rodewald, A. D. (2015). Response of mixed-species flocks to habitat alteration and deforestation in the Andes. Biol. Cons. 188, 72–81. doi: 10.1016/j.biocon.2015.02.008
Colwell, R. K., Brehm, G., Cardelus, C. L., Gilman, A. C., and Longino, J. T. (2008). Global warming, elevational range shifts, and lowland biotic attrition in the wet tropics. Science 322, 258–261. doi: 10.1126/science.1162547
Conner, W. E., and Corcoran, A. J. (2012). Sound strategies: the 65-million-year old battle between bats and insects. Annu. Rev. Entomol. 57, 21–39. doi: 10.1146/annurev-ento-121510-133537
da Silva, J. M. C., and Bates, J. M. (2002). Biogeographic patterns and conservation in the South American Cerrado: a tropical savanna hotspot. Bioscience 52, 225–234. doi: 10.1641/0006-3568(2002)052[0225:BPACIT]2.0.CO;2
Davidson, E. A., de Araújo, A. C., Artaxo, P., Balch, J. K., Brown, I. F., Bustamante, M. M. C., et al. (2012). The Amazon Basin in transition. Nature 481, 321–328. doi: 10.1038/nature10717
Dayer, A. A., Silva-Rodríguez, E. A., Albert, S., Chapman, M., Zukowski, B., Ibarra, J. T., et al. Applying conservation social science to study the human dimensions of Neotropical bird conservation. Condor Ornithol. Appl. (2020) 122, 1–15. doi: 10.1093/condor/duaa021
Demos, T. J. (2017). Against the Anthropocene: Visual Culture and Environment Today. Cambridge: MIT Press.
Desouza, O. G., Schoereder, J. H., Brown, V., and Bierregaard, R. O. Jr. (2001). “A theoretical overview of the processes determining species richness in forest fragments. Ch. 2,” in Lessons From Amazonia: The Ecology and Conservation of a Fragmented Forest, eds R. O. Jr. Bierregaard, C. Gascon, T. W. Lovejoy, and R. C. G. Mesquita (New Haven, CT: Yale University Press), 13–21.
Dirzo, R., and Raven, P. H. (2003). Global state of biodiversity and loss. Annu. Rev. Env. Resour. 28, 137–167. doi: 10.1146/annurev.energy.28.050302.105532
Dirzo, R., Young, H. S., Galetti, M., Ceballos, G., Isaac, N. J. B., and Cohen, B. (2014). Defaunation in the Anthropocene. Science 345, 401–406. doi: 10.1126/science.1251817
Ducatez, S., Sol, D., Sayol, F., and Lefebvre, L. (2020). Behavioral plasticity is associated with reduced extinction risk in birds. Nat. Ecol. Evol. 4, 788–793. doi: 10.1038/s41559-020-1168-8
Endara, M.-J., Coley, P. D., Ghabash, G., Nicholls, J. A., Dexter, K. G., Donoso, D. A., et al. (2017). Coevolutionary arms race versus host defense chase in a tropical herbivore-plant system. Proc. Natl. Acad. Sci. U. S. A. 114, E7499–E7505. doi: 10.1073/pnas.1707727114
Estes, J. A., Terborgh, J., Brashares, J. S., Power, M. E., Berger, J., Bond, W. J., et al. (2011). Downgrading of planet Earth. Science 333, 301–306. doi: 10.1126/science.1205106
Feeley, K. J., and Terborgh, J. W. (2008). Direct vs. indirect effects of habitat reduction on the loss of avian species from tropical forest fragments. Anim. Conserv. 11, 353–360. doi: 10.1111/j.1469-1795.2008.00182.x
Fine, P. V. A., Metz, M. R., Lokvam, J., Mesones, I., Zuñiga, M. A., Lamarre, G. P. A., et al. (2013). Insect herbivores, chemical innovation, and the evolution of habitat specialization in Amazonian trees. Ecology 94, 1764–1775. doi: 10.1890/12-1920.1
Forero-Medina, G., Joppa, L., and Pimm, S. L. (2011). Constraints to species' elevational range shifts as climate changes. Cons. Biol. 25, 163–171. doi: 10.1111/j.1523-1739.2010.01572.x
Franchin, A. G., Juliano, R. de. F., Kanegae, M. F., and Marçal, O. Jr. (2017). “Birds in the tropical savannas. Vol. X,” in International Commission on Tropical Biology and Natural Resources in Encyclopedia of Life Support Systems (EOLSS), eds K. Del Claro, P. S. Oliveira, V. Rico-Gray, A. A. A. Barbosa, A. Bonet, F. R. Scarano, F. J. M. Garzon, G. C. Villarnovo, L. Coelho, M. V. Sampaio, M. Quesada, M. R. Morris, O. Ramirez, R. H. F. Macedo, R. J. Marquis, R. P. Martins, S. C. Rodrigues, Luttge, and O. Jr Marçcal (UNESCO EOLSS).
Freeman, B. G. (2016). Thermal tolerances to cold do not predict upper elevational limits in New Guinean montane birds. Diversity Distrib. 22, 309–317. doi: 10.1111/ddi.12409
Freeman, B. G., Scholer, M. N., Ruiz-Gutierrez, V., and Fitzpatrick, J. W. (2018). Climate change causes upslope shifts and mountaintop extirpations in a tropical bird community. Proc. Natl. Acad. Sci. U. S. A 115, 11982–11987. doi: 10.1073/pnas.1804224115
Frick, W. F., Kingston, T., and Flanders, J. (2019). A review of the major threats and challenges to global bat conservation. Ann. N. Y. Acad. Sci. 1469, 5–25. doi: 10.1111/nyas.14045
George, T. L., Harrigan, R. J., LaManna, J. A., DeSante, D. F., Sarraco, J. F., and Smith, T. B. (2015). Persistent impacts of West Nile Virus on North American bird populations. Proc. Natl. Acad. Sci. U. S. A. 112, 14290–14294. doi: 10.1073/pnas.1507747112
Gibson, L., Lee, T. M., Koh, L. P., Brook, B. W., Gardner, T. A., Barlow, J., et al. (2011). Primary forests are irreplaceable for protecting tropical biodiversity. Nature 478, 378–381. doi: 10.1038/nature10425
Greenberg, R., Bichier, P., Angon, A. C., MacVean, C., Perez, R., and Cano, E. (2000). The impact of avian insectivory on arthropods and leaf damage in some Guatemalan coffee plantations. Ecology 81, 1750–1755. doi: 10.1890/0012-9658(2000)081[1750:TIOAIO]2.0.CO;2
Harper, G. A., and Bunbury, N. (2015). Invasive rats on tropical islands: their population biology and impacts on native species. Glob. Ecol. Cons. 3, 607–627. doi: 10.1016/j.gecco.2015.02.010
Harvey, M. G., Bravo, G. A., Claramunt, S., Cuervo, A. M., Derryberry, G. E., Battilana, J., et al. (2020). The evolution of a tropical biodiversity hotspot. Science 370, 1343–1348. doi: 10.1126/science.aaz6970
Hoffmann, W. A., Lucatelli, V. M. P. C., Silva, F. J., Azeuedo, I. N. C., Marinho, da S. M., Albuquerque, A. M. S., et al. (2004). Impact of the invasive alien grass Melinis minutiflora at the savanna-forest ecotone in the Brazilian Cerrado. Divers. Distrib. 10, 91–103. doi: 10.1111/j.1366-9516.2004.00063.x
Huston, M. (2012). Precipitation, soils, NPP, and biodiversity: resurrection of Albrecht's curve. Ecol. Monogr. 82, 277–296. doi: 10.1890/11-1927.1
IPCC (2018). IPCC Special Report on Global Warming of 1.5°C. Available online at: https://unfccc.int/topics/science/workstreams/cooperation-with-the-ipcc/ipcc-special-report-on-global-warming-of-15-degc (accessed April 1, 2021).
Jankowski, J. E., Robinson, S. K., and Levey, D. J. (2010). Squeezed at the top: interspecific aggression may constrain elevational ranges in tropical birds. Ecology 91, 1877–1884. doi: 10.1890/09-2063.1
Janzen, D. H. (1967). Why mountain passes are higher in the tropics. Am. Nat. 101, 233–249. doi: 10.1086/282487
Janzen, D. H., and Hallwachs, W. (2019). Perspective: where might be many tropical insects? Biol. Conserv. 233, 102–108. doi: 10.1016/j.biocon.2019.02.030
Janzen, D. H., and Hallwachs, W. (2021). To us insectometers, it is clear that insect decline in our Costa Rican tropics is real, so let's be kind to the survivors. Proc. Natl. Acad. Sci. U. S. A. 118:2e2002546117. doi: 10.1073/pnas.2002546117
Jarzyna, M. A., and Jetz, W. (2016). Detecting the multiple facets of biodiversity. Trends Ecol. Evol. 31, 527–538. doi: 10.1016/j.tree.2016.04.002
Jarzyna, M. A., and Jetz, W. (2017). A near half-century of temporal change in different facets of avian diversity. Glob. Change Biol. 23, 2999–3011. doi: 10.1111/gcb.13571
Jenkins, C. N., Pimm, S. L., and Joppa, L. N. (2013). Global patterns of terrestrial vertebrate diversity and conservation. Proc. Natl. Acad. Sci. U. S. A. 110, E2608–E2610. doi: 10.1073/pnas.1302251110
Johnson, M. D., and Hackett, S. C. (2016). “Why birds matter economically,” in Why Birds Matter: Avian Ecological Function and Ecosystem Services, eds Ç. H. Sekercioglu, D. G. Wenny, and C. J. Whelan (Chicago: University of Chicago Press), 27–48.
Johnson, M. D., Levy, N. J., Kellermann, J. L., and Robinson, D. E. (2009). Effects of shade and bird exclusion on arthropods and leaf damage on coffee farms in Jamaica's Blue Mountains. Agroforest. Syst. 76, 139–148. doi: 10.1007/s10457-008-9198-2
Karp, D. S., Mendenhall, C. D., Sandí, R. F., Chaumont, N., Ehrlich, P. R., Hadly, E. A., et al. (2013). Forest bolsters bird abundance, pest control and coffee yield. Ecol. Lett. 16, 1339–1347. doi: 10.1111/ele.12173
Karr, J. R., and Freemark, K. E. (1983). Habitat selection and environmental gradients: dynamics in the stable tropics. Ecology 64, 1481–1494. doi: 10.2307/1937503
Khaliq, I., Hof, C., Prinzinger, R., Böhning-Gaese, K., and Pfenninger, M. (2014). Global variation in thermal tolerances and vulnerability of endotherms to climate change. Proc. Royal Soc. B 281:1097. doi: 10.1098/rspb.2014.1097
Kumar, A., and O'Donnell, S. (2007). Fragmentation and elevation effects on bird–army-ant interactions in Neotropical montane forest of Costa Rica. J. Trop. Ecol. 23, 581–590. doi: 10.1017/S0266467407004270
Kursar, T. A., Dexter, K. G., Lokvam, J., Pennington, R. T., Richardson, J. E., Weber, M. G., et al. (2009). The evolution of antiherbivore defenses and their contribution to species coexistence in the tropical tree genus Inga. Proc. Natl. Acad. Sci. USA 106, 18073–18078. doi: 10.1073/pnas.0904786106
Laurance, W. F. (2005). “The alteration of biotic interactions in fragmented tropical forests,” in Biotic Interactions in the Tropics: Their Role in the Maintenance of Species Diversity, eds D. Burslem, M. Pinard, and S. Hartley (Cambridge: Cambridge University Press), 441–458. doi: 10.1017/CBO9780511541971.019
Laurance, W. F., Camargo, J. L. C., Fearnside, P. M., Lovejoy, T. E., Williamson, G. B., Mesquita, R. C. G., et al. (2017). An Amazonian rainforest and its fragments as a laboratory of global change. Biol. Rev. 93, 223–247. doi: 10.1111/brv.12343
Laurance, W. F., Camargo, J. L. C., Luizão, R. C. C., Laurance, S. G., Pimm, S. L., Bruna, E. M., et al. (2011). The fate of Amazonian forest fragments: a 32-year investigation. Biol. Conserv. 144, 56–67. doi: 10.1016/j.biocon.2010.09.021
Laurance, W. F., Fearnside, P., Laurance, S. G., Delamonica, P., Lovejoy, T. E., Rankin-de Merona, J. M., et al. (1999). Relationships between soils and Amazon forest biomass: a landscape-scale study. Forest Ecol. Manag. 119, 127–138. doi: 10.1016/S0378-1127(98)00494-0
Laurance, W. F., Lovejoy, T. E., Vasconcelos, H. L., Bruna, E. M., Didham, R. K., Stouffer, P. C., et al. (2002). Ecosystem decay of Amazonian forest fragments: a 22-year investigation. Conserv. Biol. 16, 605–618. doi: 10.1046/j.1523-1739.2002.01025.x
Laurance, W. F., Sayer, J., and Cassman, K. G. (2014). Agricultural expansion and its impacts on tropical nature. Trends Ecol. Evol. 29, 107–116. doi: 10.1016/j.tree.2013.12.001
Laurance, W. F., and Williamson, B. (2001). Positive feedbacks among forest fragmentation, drought, and climate change in the Amazon. Cons. Biol. 15, 1529–1535. doi: 10.1046/j.1523-1739.2001.01093.x
Lawrence, D., and Vandecar, K. (2015). Effects of tropical deforestation on climate and agriculture. Nat. Clim. Change 5, 27–36. doi: 10.1038/nclimate2430
Lees, A. C., Moura, N. G., de Almeida, A. S., and Vieira, I. C. G. (2015). Poor prospects for avian biodiversity in Amazonian oil palm. PLoS ONE. 10:e0122432. doi: 10.1371/journal.pone.0122432
Lees, A. C., and Peres, C. A. (2006). Avifaunal collapse along the Amazonian deforestation frontier. Biol. Conserv. 133, 198–2011. doi: 10.1016/j.biocon.2006.06.005
Lees, A. C., and Peres, C. A. (2008). Conservation value of remnant riparian forest corridors of varying quality for Amazonian birds and mammals. Conserv. Biol. 22, 439–449. doi: 10.1111/j.1523-1739.2007.00870.x
Lees, A. C., and Peres, C. A. (2009). Gap-crossing movements predict species occupancy in Amazonian forest fragments. Oikos 118, 280–290. doi: 10.1111/j.1600-0706.2008.16842.x
Lees, A. C., and Peres, C. A. (2010). Habitat and life history determinants of antbird occurrence in variable-sized amazonian forest fragments. Biotropica 42, 614–621. doi: 10.1111/j.1744-7429.2010.00625.x
Lens, L., Van Dongen, S., Norris, K., Githiru, M., and Matthysen, E. (2002). Avian persistence in fragmented rainforest. Science 298, 1236–1238. doi: 10.1126/science.1075664
Lister, B. C., and Garcia, A. (2018). Climate-driven declines in arthropod abundance restructure a rainforest food web. Proc. Natl. Acad. Sci. U. S. A. 115, E10397–E10406. doi: 10.1073/pnas.1722477115
Lister, B. C., and Garcia, A. (2019). Reply to Willig et al.: long-term population trends in the Luquillo rainforest. Proc. Natl. Acad. Sci. U. S. A. 116, 12145–12146. doi: 10.1073/pnas.1904582116
Londoño, G. A., Chappell, M. A., Jankowski, J. E., and Robinson, S. K. (2017). Do thermoregulatory costs limit altitude distributions of Andean forest birds? Func. Ecol. 31, 204–215. doi: 10.1111/1365-2435.12697
Maas, B., Karp, D. S., Bumrungsri, S., Darras, K., Gonthier, D., Huang, J. C.-C., et al. (2016). Bird and bat predation services in tropical forests and agroforestry landscapes. Biol. Rev. Camb. Philos. Soc. 91, 1081–1101. doi: 10.1111/brv.12211
Maldonado-Coelho, M., and Marini, M. A. (2004). Mixed-species bird flocks from Brazilian Atlantic forest: the effects of forest fragmentation and seasonality on their size, richness and stability. Biol. Conserv. 116, 19–26. doi: 10.1016/S0006-3207(03)00169-1
Marengo, J. A., Souza, C. M. Jr., Thonicke, K., Burton, C., Halladay, K., Betts, R. A., et al. (2018). Changes in climate and land use over the Amazon region: current and future variability and trends. Front. Earth Sci. 2018:228. doi: 10.3389/feart.2018.00228
Marini, M. A., Barbet-Massin, M., Lopes, L., and Jiguet, F. (2009). Predicted climate-driven bird distribution changes and forecasted conservation conflicts in a Neotropical savanna. Cons. Biol. 23, 1558–1567. doi: 10.1111/j.1523-1739.2009.01258.x
Marjakangas, E.-L., Abrego, N., Grøtan, V., de Lima, R. A. F., Bello, C., Bovendorp, R. S., et al. (2019). Fragmented tropical forests lose mutualistic plant–animal interactions. Diversity Distribut. 26, 154–168. doi: 10.1111/ddi.13010
Marquis, R. J., and Whelan, C. J. (1994). Insectivorous birds increase growth of white oak through consumption of leaf-chewing insects. Ecology 75, 2007–2014. doi: 10.2307/1941605
Martínez, A. E., Pollock, H. S., Rodrigues, P., and Touchton, J. M. (2021). Ant-following in Neotropical birds: a review and prospectus. Ornithology 138:ukaa078. doi: 10.1093/ornithology/ukaa078
Michel, N. L., Carson, W. P., and Sherry, T. W. (2015a). Do collared peccaries reduce understory insectivorous rainforest bird abundance indirectly via lianas and vines? Biotropica 47, 745–757. doi: 10.1111/btp.12261
Michel, N. L., Robinson, W. D., and Sherry, T. W. (2015b). “Liana-bird relationships: a review,” in Ecology of Lianas, eds S. A. Schnitzer, F. Bongers, R. J. Burnham, and F. E. Putz (Oxford: Wiley-Blackwell), 362–397. doi: 10.1002/9781118392409.ch25
Michel, N. L., Sherry, T. W., and Carson, W. P. (2014). The omnivorous collared peccary negates an insectivore-generated trophic cascade in Costa Rican wet tropical forest understory. J. Trop. Ecol. 30, 1–11. doi: 10.1017/S0266467413000709
Michel, N. L., Whelan, C. J., and Verutes, G. M. (2020). Ecosystem services provided by Neotropical birds. Condor Ornithol. Appl. 122:duaa022. doi: 10.1093/condor/duaa022
Miller, M. J., Bermingham, E., Turner, B. L., Touchon, J. C., Johnson, A. B., and Winker, K. (2021). Demographic consequences of foraging ecology explain genetic diversification in Neotropical bird species. Ecol. Lett. 24, 563–571. doi: 10.1111/ele.13674
Montgomery, G. A., Dunn, R. R., Fox, R., Jongejans, E., Leather, S. R., Saunders, M. E., et al. (2020). Is the insect apocalypse upon us? How to find out. Biol. Conserv. 241:108327. doi: 10.1016/j.biocon.2019.108327
Mooney, K. A., Gruner, D. S., Barber, N. A., Van Bael, S. A., Philpott, S. M., and Greenberg, R. (2010). Interactions among predators and the cascading effects of vertebrate insectivores on arthropod communities and plants. Proc. Natl. Acad. Sci. U. S. A 107, 7335–7340. doi: 10.1073/pnas.1001934107
Moore, R. P., Robinson, W. D., Lovette, I. J., and Robinson, T. R. (2008). Experimental evidence for extreme dispersal limitation in tropical forest birds. Ecol. Lett. 11, 960–968. doi: 10.1111/j.1461-0248.2008.01196.x
Myers, N., Mittermeier, R. A., Mittermeier, C. G., da Fonseca, G. A. B., and Kent, J. (2000). Biodiversity hotspots for conservation. Nature 403, 853–858. doi: 10.1038/35002501
Neate-Clegg, M., Jones, S. E. I., Tobias, J. A., Newmark, W. D., and Sekercioglu, Ç. H. (2021). Ecological drivers of elevational shifts in tropical birds. Front. Ecol. Evol. 9:215. doi: 10.3389/fevo.2021.621749
Neate-Clegg, M. H. C., Jones, S. E. I., Burdekin, O., Jocque, M., and Sekercioglu, Ç. H. (2018). Elevational changes in the avian community of a Mesoamerican cloud forest park. Biotropica 50, 805–815. doi: 10.1111/btp.12596
Neate-Clegg, M. H. C., and Sekercioglu, Ç. H. (2020). Agricultural land in the Amazon basin supports low bird diversity and is a poor replacement for primary forest. Condor Ornithol. Appl. 122, 1–11. doi: 10.1093/condor/duaa020
Newbold, T., Scharlemann, J. P. W., Butchart, S. H. M., Sekercioglu, Ç. H., Alkemade, R., Booth, H., et al. (2013). Ecological traits affect the response of tropical forest bird species to land-use intensity. P. Roy. Soc. B-Biol. Sci. 280:2131. doi: 10.1098/rspb.2012.2131
Newmark, W. D., and Stanley, T. R. (2011). Habitat fragmentation reduces nest survival in an Afrotropical bird community in a biodiversity hotspot. Proc. Natl. Acad. Sci. U. S. A 108, 11488–11493. doi: 10.1073/pnas.1104955108
Nobre, C. A., Sampaio, G., Borma, L. S., Castilla-Rubio, J. C., Silva, J. S., and Cardoso, M. (2016). Land-use and climate change risks in the Amazon and the need of a novel sustainable development paradigm. Proc. Natl. Acad. Sci. U. S. A. 113, 10759–10768. doi: 10.1073/pnas.1605516113
Norambuena, H. V., and Van Els, P. (2020). A general scenario to evaluate evolution of grassland birds in the Neotropics. Ibis 163, 722–727. doi: 10.1111/ibi.12905
Nyffeler, M., Sekercioglu, Ç. H., and Whelan, C. J. (2018). Insectivorous birds consume an estimated 400–500 million tons of prey annually. Sci. Nat.-Heidelberg 105:47. doi: 10.1007/s00114-018-1571-z
O'Brien, K. J. (2010). An Ethics of Biodiversity: Christianity, Ecology, and the Diversity of Life. Washington, DC: Georgetown University Press.
O'Brien, K. J. (2017). The Violence of Climate Change: Lessons of Resistance From Nonviolent Activists. Washington, DC: Georgetown University Press.
Ometto, J. P., Aguiar, A. D. P., and Martinelli, L. A. (2011). Amazon deforestation in Brazil: effects, drivers and challenges. Carbon Manag. 2, 575–585. doi: 10.4155/cmt.11.48
Palmeirim, A. F., Santos-Filho, M., and Peres, C. A. (2020). Marked decline in forest-dependent small mammals following habitat loss and fragmentation in an Amazonian deforestation frontier. PLoS ONE 15:e0230209. doi: 10.1371/journal.pone.0230209
Pattanavibool, A., and Dearden, P. (2002). Fragmentation and wildlife in montane evergreen forests, northern Thailand. Biol. Cons. 107, 155–164. doi: 10.1016/S0006-3207(02)00056-3
Pavlacky, D. C. Jr., Possingham, H. P., and Goldizen, A. W. (2015). Integrating life history traits and forest structure to evaluate the vulnerability of rainforest birds along gradients of deforestation and fragmentation in eastern Australia. Biol. Conserv. 188, 89–99. doi: 10.1016/j.biocon.2014.10.020
Peres, C. A. (2001). Synergistic effects of subsistence hunting and habitat fragmentation on Amazonian forest vertebrates. Cons. Biol. 15, 1490–1505. doi: 10.1046/j.1523-1739.2001.01089.x
Peres, C. A., and Nascimento, H. S. (2006). Impact of game hunting by the Kayapó of south-eastern Amazonia: implications for wildlife conservation in tropical forest indigenous reserves. Biol. Cons. 15, 2627–2653. doi: 10.1007/s10531-005-5406-9
Peters, M. K., Likare, S., and Kraemer, M. (2008). Effects of habitat fragmentation and degradation on flocks of African ant-following birds. Ecol. Appl. 18, 847–858. doi: 10.1890/07-1295.1
Peters, M. K., and Okalo, B. (2009). Severe declines of ant-following birds in African rainforest fragments are facilitated by a subtle change in army-ant communities. Biol. Conserv. 142, 2050–2058. doi: 10.1016/j.biocon.2009.03.035
Pfeifer, M., Lefebvre, V., Peres, C. A., Banks-Leite, C., Wearn, O. R., Marsh, C. J., et al. (2017). Creation of forest edges has a global impact on forest vertebrates. Nature 551, 187–191. doi: 10.1038/nature24457
Picolo, J. J. (2017). Intrinsic values in nature: objective good or simply half of an unhelpful dichotomy? J. Nat. Conserv. 37, 8–11. doi: 10.1016/j.jnc.2017.02.007
Pimm, S., Raven, P., Peterson, A., Sekercioglu, Ç. H., and Ehrlich, P.R. (2006). Human impacts on the rates of recent, present, and future bird extinctions. Proc. Natl. Acad. Sci. U. S. A. 103, 10941–10946. doi: 10.1073/pnas.0604181103
Pinheiro, C. E. G., and Campos, V. C. (2019). The responses of wild jacamars (Galbula ruficauda, Galbulidae) to aposematic, aposematic and cryptic, and cryptic butterflies in central Brazil. Ecol. Entomol. 44, 441–450. doi: 10.1111/een.12723
Pinto, J., Bonacic, C., Hamilton-West, C., Romero, J., and Lubroth, J. (2008). Climate change and animal diseases in South America. Rev. Sci. Tech. Off. Int. Epiz. 27, 599–613. doi: 10.20506/rst.27.2.1813
Pollock, H. S., Brawn, J. D., and Cheviron, Z. A. (2020). Heat tolerances of temperate and tropical birds and their implications for susceptibility to climate warming. Funct. Ecol. 35, 93–104. doi: 10.1111/1365-2435.13693
Pollock, H. S., Cheviron, Z. A., Agin, T. J., and Brawn, J. D. (2015). Absence of microclimate selectivity in insectivorous birds of the Neotropical forest understory. Biol. Conserv. 188, 116–125. doi: 10.1016/j.biocon.2014.11.013
Porro, R., Miller, R. P., Tito, M. R., Donovan, J. A., Vivan, J. L., Trancoso, R., et al. (2012). “Agroforestry in the Amazon region: a pathway for balancing conservation and development,” in Agroforestry - The Future of Global Land Use, Advances in Agroforestry 9, eds P. K. R. Nair, and D. Garrity (Dordrecht: Springer). doi: 10.1007/978-94-007-4676-3_20
Powell, L. L., Cordeiro, J. J., and Stratford, J. A. (2015). Ecology and conservation of avian insectivores of the rainforest understory: a pantropical perspective. Biol. Conserv. 188, 1–10. doi: 10.1016/j.biocon.2015.03.025
Prado, D. E., and Gibbs, P. E. (1993). Patterns of species distributions in the dry seasonal forests of South America. Ann. Missouri Bot. 80, 902–927. doi: 10.2307/2399937
Prendergast, K. S. (2020). Beyond ecosystem services as justification for biodiversity conservation. Austral Ecol. 45, 141–143. doi: 10.1111/aec.12882
Prugh, L. R., Stoner, C. J., Epps, C. W., Bean, W. T., Ripple, W. J., Laliberte, A. S., et al. (2009). The rise of the mesopredator. BioScience 59, 779–791. doi: 10.1525/bio.2009.59.9.9
Raven, P. H., and Wagner, D. L. (2021). Agricultural intensification and climate change are rapidly decreasing insect biodiversity. Proc. Natl. Acad. Sci. U. S. A. 118:2e2002548117. doi: 10.1073/pnas.2002548117
Renjifo, L. M. (1999). Composition changes in a subandean avifauna after long-term forest fragmentation. Conserv. Biol. 13, 1124–1139. doi: 10.1046/j.1523-1739.1999.98311.x
Renjifo, L. M. (2001). Effect of natural and anthropogenic landscape matrices on the abundance of subandean bird species. Ecol. Appl. 11, 14–31. doi: 10.1890/1051-0761(2001)011[0014:EONAAL]2.0.CO;2
Restrepo, C., and Gómez, N. (1998). Responses of understory birds to anthropogenic edges in a Neotropical montane forest. Ecol. Appl. 8, 170–183. doi: 10.1890/1051-0761(1998)008[0170:ROUBTA]2.0.CO;2
Robinson, W. D. (1999). Long-term changes in the avifauna of Barro Colorado Island, Panama, a tropical isolate. Cons. Biol. 13, 85–97 doi: 10.1046/j.1523-1739.1999.97492.x
Robinson, W. D., Brawn, J. D., and Robinson, S. K. (2000). Forest bird community structure in central Panama: influence of spatial scale and biogeography. Ecol. Monogr. 70, 209–235. doi: 10.1890/0012-9615(2000)070[0209:FBCSIC]2.0.CO;2
Robinson, W. D., and Curtis, J. R. (2020). Creating benchmark measurements of tropical forest bird communities in large plots. Condor Ornithol. Appl. 122:duaa015. doi: 10.1093/condor/duaa015
Robinson, W. D., Rourke, B., and Stratford, J. A. (2021). Put some muscle behind it: understanding movement capacity of tropical birds. Ornithology 138, 1–11. doi: 10.1093/ornithology/ukaa068
Robinson, W. D., and Sherry, T. W. (2012). Mechanisms of avian population decline and species loss in tropical forest fragments. J. Ornithol. 153, S141–S152. doi: 10.1007/s10336-011-0806-y
Rockström, J., Klum, M., and Miller, P. (2015). Big World Small Planet: Abundance Within Planetary Boundaries. New Haven. CT: Yale University Press.
Rosenberg, K. V., Dokter, A. M., Blancher, P. J., Sauer, J. R., Smith, A. C., Smith, P. A., et al. (2019). Decline of North American avifauna. Science 366, 120–124. doi: 10.1126/science.aaw1313
Salcido, D. M., Forister, M. L., Lopez, H. G., and Dyer, L. A. (2020). Loss of dominant caterpillar genera in a protected tropical forest. Sci. Rep. 10:422. doi: 10.1038/s41598-019-57226-9
Salisbury, C. L., Seddon, N., Cooney, C. R., and Tobias, J. A. (2012). The latitudinal gradient in dispersal constraints: ecological specialisation drives diversification in tropical birds. Ecolo. Lett. 15, 847–855. doi: 10.1111/j.1461-0248.2012.01806.x
Samuel, M. D., Woodworth, B. L., Atkinson, C. T., Hart, P., and LaPointe, D. A. (2015). Avian malaria in Hawaiian forest birds: Infection and population impacts across species and elevations. Ecosphere 6:aart104. doi: 10.1890/ES14-00393.1
Sánchez-Bayo, F., and Wyckhuys, K. A. G. (2019). Worldwide decline of the entomofauna: a review of its drivers. Biol. Conserv. 232, 8–27. doi: 10.1016/j.biocon.2019.01.020
Schlaepfer, D. R., Braschler, B., Rusterholz, H.-P., and Baru, B. (2018). Genetic effects of anthropogenic habitat fragmentation on remnant animal and plant populations: a meta-analysis. Ecosphere 9:2488. doi: 10.1002/ecs2.2488
Schnitzer, S. A., Michel, N. L., Powers, J. S., and Robinson, W. D. (2020). Lianas maintain insectivorous bird abundance and diversity in a Neotropical forest. Ecology 101:e01376. doi: 10.1002/ecy.3176
Schowalter, T. D., Pandey, M., Presley, S. J., Willig, M. R., and Zimmerman, J. K. (2021). Arthropods are not declining but are responsive to disturbance in the Luquillo Experimental Forest, Puerto Rico. Proc. Natl. Acad. Sci. U. S. A. 118:2e2002556117. doi: 10.1073/pnas.2002556117
Sedio, B. E., Parker, J. D., McMahon, S. M., and Wright, S. J. (2018). Comparative metabolomics of a tropical and a temperate forest community. Ecology 99:2647–2653. doi: 10.1002/ecy.2533
Sehgal, R. N. M. (2010). Deforestation and avian infectious diseases. J. Exper. Biol. 213, 955–960. doi: 10.1242/jeb.037663
Sekercioglu, Ç. H. (2011). Functional extinctions of bird pollinators cause plant declines. Science 331, 1019–1020. doi: 10.1126/science.1202389
Sekercioglu, Ç. H. (2012). Bird functional diversity and ecosystem services in tropical forests, agroforests and agricultural areas. J. Ornithol. 153, 153–161. doi: 10.1007/s10336-012-0869-4
Sekercioglu, Ç. H., Daily, G. C., and Ehrlich, P.R. (2004). Ecosystem consequences of bird declines. Proc. Natl. Acad. Sci. U. S. A. 101, 18042–18047. doi: 10.1073/pnas.0408049101
Sekercioglu, Ç. H., Ehrlich, P. R., Daily, G. C., Aygen, D., Goehring, D., and Sandi, R.F. (2002). Disappearance of insectivorous birds from tropical forest fragments. Proc. Natl. Acad. Sci. U. S. A. 99, 263–267. doi: 10.1073/pnas.012616199
Sekercioglu, Ç. H., Mendenhall, C. D., Brenes, F. O., Horns, J. J., Ehrlich, P. R., and Daily, G. C. (2019). Long-term declines in bird populations in tropical agricultural countryside. Proc. Natl. Acad. Sci. U. S. A. 116, 9903–9912. doi: 10.1073/pnas.1802732116
Sekercioglu, Ç. H., Primack, R. B., and Wormworth, J. (2012). The effects of climate change on tropical birds. Biol. Conserv. 148, 1–18. doi: 10.1016/j.biocon.2011.10.019
Sheard, C., Neate-Clegg, M. H. C., Alioravainen, N., Jones, S. E. I., Vincent, C., MacGregor, H. E. A., et al. (2020). Ecological drivers of global gradients in avian dispersal inferred from wing morphology. Nat. Commun. 11:2463, doi: 10.1038/s41467-020-16313-6
Sheldon, K. S. (2019). Climate change in the tropics: ecological and evolutionary responses at low latitudes. Ann. Rev. Ecol. Evol. Syst. 50, 303–333. doi: 10.1146/annurev-ecolsys-110218-025005
Sher, A. A., and Primack, R. B. (2019). An Introduction to Conservation Biology, 2nd Edn. New York, NY: Sinauer Associates and Oxford University Press.
Sherry, T. W. (1990). When are birds dietarily specialized? Distinguishing ecological from evolutionary perspectives. Stud. Avian Biol. 13, 337–352.
Sherry, T. W. (2008). Community approach to tropical forest fragmentation gets us closer to understanding mechanisms by revealing strong indirect effects. Anim. Conserv. 11, 361–363. doi: 10.1111/j.1469-1795.2008.00205.x
Sherry, T. W. (2016). “Chapter 8. avian food and foraging,” in The Cornell Lab of Ornithology Handbook of Bird Biology, 3rd Edn, eds I. J. Lovette, and J. W. Fitzpatrick (West Sussex: John Wiley & Sons), 264–310.
Sherry, T. W., Johnson, M. D., Williams, K., Kaban, J., McAvoy, C., Medori, A., et al. (2016). Dietary opportunism, resource partitioning, and consumption of coffee-berry borers by five migratory wood warblers (Parulidae) wintering in Jamaican shade coffee plantations. J. Field Ornithol. 87, 273–292. doi: 10.1111/jofo.12160
Sherry, T. W., Kent, C. M., Sánchez, N. V., and Sekercioglu, Ç. H. (2020). Insectivorous birds in the Neotropics: ecological radiations, specialization, and coexistence in species-rich communities. Auk Ornithol. Adv. 137:ukaa049. doi: 10.1093/auk/ukaa049
Sigel, B. J., Robinson, W. D., and Sherry, T. W. (2010). Comparing bird community responses to forest fragmentation in two lowland Central American reserves. Biol. Conserv. 143, 340–350. doi: 10.1016/j.biocon.2009.10.020
Sigel, B. J., Sherry, T. W., and Young, B. E. (2006). Avian community response to lowland tropical rainforest isolation: 40 years of change at La Selva Biological Station, Costa Rica. Cons. Biol. 20, 111–121. doi: 10.1111/j.1523-1739.2005.00293.x
Silvestrini, R. A., Soares-Filho, B. S., Nepstad, D., Coe, M., Rodrigues, H., and Assunçao. (2011). Simulating fire regimes in the Amazon in response to climate change and deforestation. Ecol. Appl. 21, 1573–1590. doi: 10.1890/10-0827.1
Sodhi, N. S., Brook, B. W., and Bradshaw, C. J. A. (2007a). “Chapter 5, Alien invaders,” in Tropical Conservation Biology (Oxford: Blackwell Publishing), 89–110.
Sodhi, N. S., Brook, B. W., and Bradshaw, C. J. A. (2007b). “Chapter 9, lost without a trace: the tropical extinction crisis,” in Tropical Conservation Biology (Oxford: Blackwell Publishing), 208–238.
Sodhi, N. S., Liow, L. H., and Bazzaz, F. A. (2004). Avian extinctions from tropical and subtropical forests. Annu. Rev. Ecol. Syst. 35, 323–345. doi: 10.1146/annurev.ecolsys.35.112202.130209
Sodhi, N. S., Sekercioglu, Ç. H., Barlow, J., and Robinson, S. K. (2011). Conservation of Tropical Birds. Oxford: Wiley-Blackwell). doi: 10.1002/9781444342611
Soh, M. C. K., Sodhi, N. S., and Lim, S. L. H. (2006). High sensitivity of montane bird communities to habitat disturbance in Peninsular Malaysia. Biol. Conserv. 129, 149–166. doi: 10.1016/j.biocon.2005.10.030
Spanhove, T., Lehouck, V., and Lens, L. (2009). Inverse edge effect on nest predation in a Kenyan forest fragment: an experimental case study. Bird Cons. Intl. 19, 367–378. doi: 10.1017/S0959270909008752
Stouffer, P. C. (2020). Birds in fragmented Amazonian rainforest: lessons from 40 years at the Biological Dynamics of Forest Fragments Project. Condor Ornithol. Appl. 122, 1–15. doi: 10.1093/condor/duaa005
Stouffer, P. C., and Bierregaard, R. O. Jr. (1995). Use of Amazonian forest fragments by understory insectivorous birds. Ecology 76, 2429–2445. doi: 10.2307/2265818
Stouffer, P. C., Bierregaard, R. O. Jr., Strong, C., and Lovejoy, T. E. (2006). Long-term landscape change and bird abundance in Amazonian rainforest fragments. Conserv. Biol. 20, 1212–1223. doi: 10.1111/j.1523-1739.2006.00427.x
Stouffer, P. C., Jirinec, V., Rutt, C. L., Bierregaard, R. O. Jr., Hernández-Palma, A., Johnson, E. I., et al. (2020). Long-term change in the avifauna of undisturbed Amazonian rainforest: ground-foraging birds disappear and the baseline shifts. Ecol. Lett. 24, 186–195. doi: 10.1111/ele.13628
Stratford, J. A., and Robinson, W. D. (2005). Gulliver travels to the fragmented tropics: geographic variation in mechanisms of avian extinction. Frontiers Ecol. Envir. 3, 91–98. doi: 10.2307/3868515
Stratford, J. A., and Stouffer, P. C. (2015). Forest fragmentation alters microhabitats availability for Neotropical terrestrial insectivorous birds. Biol. Conserv. 188, 109–115. doi: 10.1016/j.biocon.2015.01.017
Stutchbury, B. J. M., and Morton, E. S. (2001). Behavioral Ecology of Tropical Birds. San Diego, CA: Academic Press.
Tallamy, D. W., Narango, D. L., and Mitchell, A. B. (2020). Do non-native plants contribute to insect declines? Ecol. Entomol. 2020, 1–14. doi: 10.1111/een.12973
Taubert, F., Fischer, R., Groeneveld, J., Lehmann, S., Müller, M. S., Rödig, E., et al. (2018). Global patterns of tropical forest fragmentation. Nature 554, 519–522. doi: 10.1038/nature25508
ter Hofstede, H. M., and Ratcliffe, J. M. (2016). Evolutionary escalation: the bat-moth arms race. J. Exper. Biol. 219, 1589–1602. doi: 10.1242/jeb.086686
Terborgh, J., Robinson, S. K., Parker, T. A. I. I. I., Munn, C. A., and Pierpont, N. (1990). Structure and organization of an Amazonian forest bird community. Ecol. Monogr. 60, 213–238. doi: 10.2307/1943045
Terborgh, J. W. (2015). Toward a trophic theory of species diversity. Proc. Natl. Acad. Sci. U. S. A. 112, 10415–10422. doi: 10.1073/pnas.1501070112
Terborgh, J. W., Lopez, L., Nuñez, P., Rao, M., Shahabuddin, G., Orihuela, G., et al. (2001). Ecological meltdown in predator-free forest fragments. Science 294, 1923–1926. doi: 10.1126/science.1064397
Tobias, J. A., Sekercioglu, Ç. H., and Vargas, F.H. (2013). “Bird conservation in tropical ecosystems: challenges and opportunities,” in Key Topics in Conservation Biology, vol. 2, ed D. MacDonald (Oxford: Wiley-Blackwell), 258–276. doi: 10.1002/9781118520178.ch15
Van Bael, S. A., Brawn, J. D., and Robinson, S. K. (2003). Birds defend trees from herbivores in a Neotropical forest canopy. Proc. Royal Soc. B 100, 8304–8307. doi: 10.1073/pnas.1431621100
van Klink, R., Bowler, D. E., Gongalsky, K. B., Swenger, A. B., Gentile, A., and Chase, J. M. (2020). Meta-analysis reveals declines in terrestrial but increases in freshwater insect abundances. Science 368, 417–420. doi: 10.1126/science.aax9931
Vergara, P. M., Fierro, A., Alaniz, A. J., Carvajal, M. A., Lizama, M., and Llanos, L. (2020). Landscape-scale effects of forest degradation on insectivorous birds and invertebrates in austral temperate forests. Landscape Ecol. 36, 191–208. doi: 10.1007/s10980-020-01133-2
Visco, D. M., Michel, N. L., Boyle, A. W., Sigel, B. J., Woltmann, S., and Sherry, T. W. (2015). Patterns and causes of understory bird declines in human-disturbed tropical forest landscapes: a case study from Central America. Biol. Conserv. 191, 117–129. doi: 10.1016/j.biocon.2015.05.018
Visco, D. M., and Sherry, T. W. (2015). Increased abundance, but reduced nest predation in the chestnut-backed antbird in Costa Rican rainforest fragments: surprising impacts of a pervasive snake species. Biol. Conserv. 188, 22–31. doi: 10.1016/j.biocon.2015.01.015
Vitousek, P. M., Mooney, H. A., Lubchenko, J., and Melillo, J. M. (1997). Human domination of Earth's ecosystems. Science 277, 494–499. doi: 10.1126/science.277.5325.494
Wagner, D. L. (2020). Insect declines in the Anthropocene. Annu. Rev. Entomol. 65, 457–480. doi: 10.1146/annurev-ento-011019-025151
Wagner, D. L., Fox, R., Salcedo, D. M., and Dyer, L. A. (2020). A window to the world of global insect declines: moth biodiversity trends are complex and heterogeneous. Proc. Natl. Acad. Sci. U. S. A. 118:e2002549117. doi: 10.1073/pnas.2002549117
Wagner, D. L., Grames, E. M., Forister, M. L., Berenbaum, M. R., and Stopak, D. (2021). Insect decline in the Anthropocene: death by a thousand cuts. Proc. Natl. Acad. Sci. U. S. A. 118:e2023989118. doi: 10.1073/pnas.2023989118
Wagner, F., Rossi, V., Aubry-Kientz, M., Bonal, D., Dalitz, H., Gliniars, R., et al. (2014). Pan-tropical analysis of climate effects on seasonal tree growth. PLoS ONE Biol. 9:e92337. doi: 10.1371/journal.pone.0092337
Watson, J. E. M., Evans, T., Venter, O., Williams, B., Tulloch, A., Stewart, C., et al. (2018). The exceptional value of intact forest ecosystems. Nat. Ecol. Evol. 2, 599–610. doi: 10.1038/s41559-018-0490-x
Weir, J. T., Faccio, M. S., Pulido-Santacruz, P., Barrera-Guzmán, A., and Aleixo, A. (2015). Hybridization in headwater regions, and the role of rivers as drivers of speciation in Amazonian birds. Evolution 69, 1823–1834. doi: 10.1111/evo.12696
Werner, T. K., and Sherry, T. W. (1987). Behavioral feeding specialization in Pinaroloxias inornata, the “Darwin's Finch” of Cocos Island, Costa Rica. Proc. Nat. Acad. Sci. U. S. A. 84, 5506–5510. doi: 10.1073/pnas.84.15.5506
Westemeier, R. L., Brawn, S. A., Simpson, J. D., Esker, T. L., Jansen, R. W., Walk, J. W., et al. (1998). Tracking the long-term decline and recovery of an isolated population. Science 282, 1695–1698. doi: 10.1126/science.282.5394.1695
Willig, M. R., Woolbright, L., Pesley, S. J., Schowalter, T. D., Waide, R. B., Heartsill Scalley, T., et al. (2019). Populations are not declining and food webs are not collapsing at the Luquillo Experimental Forest. Proc. Nat. Acad. Sci. U. S. A. 116, 12143–12144. doi: 10.1073/pnas.1820456116
Williston, B. (2019). The Ethics of Climate Change: An Introduction. New York, NY: Routledge, Taylor & Francis Group.
Woltmann, S., Kreiser, B. R., and Sherry, T. W. (2012a). Fine-scale genetic population structure of an understory rainforest bird in Costa Rica. Cons. Gen. 13, 925–935. doi: 10.1007/s10592-012-0341-2
Woltmann, S., Sherry, T. W., and Kreiser, B. R. (2012b). A genetic approach to estimating natal dispersal distances and self-recruitment in resident rainforest birds. J. Avian Biol. 43, 33–42. doi: 10.1111/j.1600-048X.2011.05572.x
Keywords: biological diversity, biotic challenge hypothesis, conservation, ecosystem services, insectivorous bird declines, intrinsic value, neotropics, tropics
Citation: Sherry TW (2021) Sensitivity of Tropical Insectivorous Birds to the Anthropocene: A Review of Multiple Mechanisms and Conservation Implications. Front. Ecol. Evol. 9:662873. doi: 10.3389/fevo.2021.662873
Received: 01 February 2021; Accepted: 16 April 2021;
Published: 17 May 2021.
Edited by:
Çagan H. Sekercioglu, The University of Utah, United StatesReviewed by:
W. Douglas Robinson, Oregon State University, United StatesMonte H. C. Neate-Clegg, The University of Utah, United States
Jeffrey D. Brawn, University of Illinois at Urbana-Champaign, United States
Copyright © 2021 Sherry. This is an open-access article distributed under the terms of the Creative Commons Attribution License (CC BY). The use, distribution or reproduction in other forums is permitted, provided the original author(s) and the copyright owner(s) are credited and that the original publication in this journal is cited, in accordance with accepted academic practice. No use, distribution or reproduction is permitted which does not comply with these terms.
*Correspondence: Thomas W. Sherry, tsherry@tulane.edu