- 1Centre for the Research and Technology of Agro-Environmental and Biological Sciences, Institute for Innovation, Capacity Building and Sustainability of Agri-Food Production, Inov4Agro, University of Trás-os-Montes e Alto Douro, Vila Real, Portugal
- 2Department of Crop Production and Engineering Projects, University of Santiago de Compostela, Lugo, Spain
- 3Laboratory of Applied Ecology, Inov4Agro, University of Trás-os-Montes and Alto Douro, Vila Real, Portugal
- 4Laboratory of Ecology and Conservation, Federal Institute of Education, Science and Technology of Maranhão, Buriticupu, Brazil
The effects of climate change are increasingly noticed worldwide, and crops are likely to be impacted in direct and indirect ways. Thus, it is urgent to adopt pliable strategies to reduce and/or mitigate possible adverse effects to meet the growing demand for sustainable and resilient food production. Monoculture cropping is globally the most common production system. However, adaptation to ongoing climate change, namely, to more extreme environmental conditions, has renewed the interest in other practices such as agroforestry, agroecology, and permaculture. This article provides an overview of ecological and physiological interactions between trees and crops in Mediterranean agroforestry systems and compares them with those from monocultures. The advantages and disadvantages of both systems are explored. The added value of modeling in understanding the complexity of interactions within agroforestry systems, supporting decision-making under current and future weather conditions, is also pinpointed. Several interactions between trees and crops might occur in agroforestry systems, leading to mutual positive and/or negative effects on growth, physiology, and yield. In this sense, selecting the most suitable combination of tree/crop species in mixtures may be best be indicated by complementary traits, which are crucial to maximizing trade-offs, improving productivity, ecosystem services, and environmental sustainability.
Introduction
Multispecies systems are an ancient cropping technique that involves the simultaneous cultivation of two or more plant species within the same field (Malézieux et al., 2009; Gaba et al., 2015; Bybee-Finley and Ryan, 2018). It was estimated that agroforestry systems cover about 1 billion hectares of land worldwide (Zomer et al., 2014, 2016): 17% of agricultural systems, if more than 30% of tree cover is considered, or 46% if more than 10% of tree cover is considered (Kumar et al., 2014). Agroforestry systems are envisaged within the European Green Deal as one of the most effective tools to mitigate and adapt to climate change (Mosquera-Losada et al., 2020), but also by their socio-ecological returns (Malézieux et al., 2009; Chapagain et al., 2020). The European Common Agrarian Policy (CAP) turnaround emerges from the evidence that previous support for the intensification of agroforestry systems (and in general to agriculture) led to many environmental problems, namely, soil and biodiversity losses as well as land degradation, and water pollution (Tsiafouli et al., 2015). In this way, mixed systems can be viewed as a nature-based solution, implying a practical application of ecological principles to agriculture, capitalizing functional biodiversity, beneficial plant interactions, and other homeostatic mechanisms (Malézieux et al., 2009). Actually, a diverse agroecosystem in genotypes, species, structures, and functions is more environmentally adaptable, resilient, and sustainable than monocrops (Supplementary Figure 1), especially when native species are involved (Manson et al., 2013).
Among the several types of multispecies systems, the combination of woody plants and herbaceous crops, often together with domesticated or semi-wild animals, in a range of temporal and spatial arrangements are defined as agroforestry systems (Mosquera-Losada et al., 2018). Agroforestry systems have a long tradition in tropical and Mediterranean regions (Jose, 2009; Mosquera-Losada et al., 2018; Rodríguez-Rigueiro et al., 2021). In the Mediterranean, the characteristic agroforestry systems include the oak agroforests (Portugal and Spain), agrosilvopasture mosaics (Italy), and the Valonian oak silvopastures (Greece) (Moreno et al., 2018). Several authors emphasized the pivotal role of agroforestry systems in biodiversity conservation (Mcneely and Schroth, 2006; Vodouhe et al., 2011; Montagnini, 2017; Mosquera-Losada et al., 2018, 2020; Udawatta et al., 2019; Dagar and Gupta, 2020; Rosati et al., 2020), carbon sequestration (Montagnini and Nair, 2004; Mosquera-Losada et al., 2015, 2017; Ferreiro-Domínguez et al., 2016; De Stefano and Jacobson, 2018; Udawatta et al., 2019; Dagar and Gupta, 2020), restoration of degraded ecosystems (Navas and Silva, 2016; Dagar and Gupta, 2020), and in the mitigation of climate change impacts (Lin, 2007; Bayala et al., 2008; Fernández-Núñez et al., 2010; Montagnini, 2017; Mosquera-Losada et al., 2017, 2018; De Stefano and Jacobson, 2018) by amending microclimates (Gomes et al., 2020). Agroforestry systems are also critical for rural development in low-income regions (Montagnini, 2017; Rodríguez-Rigueiro et al., 2021) by providing determinant ecosystem services such as soil fertility enhancement, prevention of soil erosion, water, wind, and pest regulation, and pollination (Rigueiro-Rodríguez et al., 2009; Kuyah et al., 2017). In this sense, mixing crops and tree species is an excellent way to increase crop yield and yield stability, especially within adverse climatic conditions (Sileshi et al., 2012; Nasielski et al., 2015).
Agroforestry systems can also reduce evaporation (by temperature and wind reduction) (Lin, 2010), improve water and nutrient cycling and radiation protection, and increase soil organic carbon and the activity of beneficial soil organisms, leading to nutrient supply by nitrogen fixation and enhanced decomposition (Barrios et al., 2012). Additionally, plants with deep roots can lift or redistribute water to the upper layers through a process known as hydraulic lift, potentially acting as “bioirrigators” to adjacent plants (Bayala and Prieto, 2020). Biodiverse ecosystems are generally more efficient in terms of resource use (water, nutrients) and more resilient to diverse environmental stresses (e.g., weather extremes, pests, or diseases) than monocrops (Gaba et al., 2015), likely due to different traits associated with complementary functions (Lohbeck et al., 2016). Despite all the positive aspects of agroforestry systems, several ecological interactions between trees and crops (Supplementary Figure 2; Jose et al., 2004) can also lead to adverse effects on the physiology of both cultures. According to van Noordwijk et al. (2015), the water/nutrient use efficiency does not differ significantly between trees and C3 crops in most agroforestry systems. Being a perennial crop, trees have more developed root systems, which can explore larger soil volumes, water, and/or nutrients, thus allowing their full growth. On the other hand, tree roots can assist the weathering of saprolite or bed-rock layers inaccessible to crops and intercept water and nutrients leaching down the soil profile below the crop rooting zone. Therefore, both spatial and temporal complementarity and competition can occur (Ong et al., 2014). According to van Noordwijk et al. (1996), belowground competition occurs especially when two or more species have developed a specialized root system that directs them to explore the same rhizosphere for resources. If unsuitable combinations of species were chosen, it can result in the poor growth of both cultures (FAO and IAEA, 2008). In fact, resource competition is the most important factor for plant community diversity and dynamics as it usually reduces the marketable productivity of the system (Tilman, 1982; Schluter, 2000; Supplementary Figure 2). Indeed, competition or competitive behaviors can affect the plant at several levels, leading to morphological responses (plant growth), biochemical responses (plant defense), and resource allocation (Novoplansky, 2009; Yamawo, 2015). According to Jose et al. (2004), competition for nutrients is minimal in systems managed with high input of inorganic or organic nutrient supplements. Thus, competitive interactions involving water seem to be the most influential driving force of productivity in alley-cropping and silvopastoral systems. Therefore, agroforestry’s effects on crop yields depend on complex interactions between trees, crops, soil, climate, and management (Bayala et al., 2012) and the balances between positive and negative interactions determine the overall sustainability and yield of the agroforests. Rosenstock et al. (2014) pointed out that the integration of N2-fixing trees in agroforestry systems promotes excessive production of gaseous N but can improve crop yields and reduce dependence on mineral fertilization. The objectives of this manuscript were (i) to summarize the ecological and physiological processes in tree–crop interactions with respect to climate change, and (ii) to give a brief overview of process-based models for agroforestry systems.
Ecological and Physiological Processes of Tree–Crop Combinations in Agroforestry Systems
Agricultural intensification can be technically defined as an increase in agricultural production per unit of inputs (FAO, 2004). In several regions of the world, particularly in the Portuguese region of Alentejo, holm-oak and cork-oak agroforests (Montados) have been replaced by olive groves. However, the association between trees, crops, or pastures has multiple economic, environmental, social, and cultural benefits (Jose, 2009; Pasalodos-Tato et al., 2009; Santiago-Freijanes et al., 2018), namely by diversifying productions, income, and services, yet remaining a low input system (Lehmann et al., 2020). Diversifying the production can reduce the risks of yield losses (and yield cycles) imposed by pests, diseases, and climate change (Lin, 2011; Santiago-Freijanes et al., 2018). Agroforestry practices such as silvopasture or silvoarable with fast- and slow-growing species might help farmers to reap multiple benefits. The diversification of species can support non-productive phases of crops and exploit timber and more valuable understorey products while reducing soil erosion risks and providing shelter and protection against frost or pests (Montagnini et al., 2004; Petit and Montagnini, 2006). Providing shade can also buffer climatic extremes (van Oijen et al., 2010) by reducing the energy expended in thermoregulation, leading to higher biomass conversion and weight gain (Jose et al., 2004). Plants can perceive their surrounding environment by using the information on the distribution of essential resources (light, nutrients, and water), chemical cues (volatile compounds, root exudates, and leachates) (Novoplansky, 2009; Weston and Mathesius, 2013), or even hormones, such as abscisic acid or ethylene (Wahid et al., 2007). According to this signaling, plants display several responses to optimize their performances upon exposure to biotic stress (Pierik et al., 2013). So, woody perennials in agroforestry systems modify microclimate conditions, which, in turn, benefit livestock and wildlife (Moreno et al., 2018) and affect crops’ physiology (Righi et al., 2007). Furthermore, volatile cues in a plant’s environment can lead to physiological changes that alter their volatile profile, influencing the neighboring plants to respond differently, adapting to neighbors’ presence (Pierik et al., 2014; Ninkovic et al., 2019).
In this context, the reduction of solar radiation, extreme temperatures, wind speed, and soil evaporation in the understory can improve water status, gas exchange, and water use efficiency by the system (Lasco et al., 2014), depending on the tree (Muthuri et al., 2009) and crop species (Arenas-Corraliza et al., 2018). Campi et al. (2009) studied the effect of tree canopy provided by Cupressus arizonica on durum wheat in a Mediterranean environment. They concluded that the woody tree’s windbreak barrier significantly affected the water use efficiency and yield. Other work performed by Mahieu et al. (2016) in a Mediterranean agroforestry system indicated that the use of walnut trees was beneficial for chickpea growth, seed biomass, seed quality, and production due to higher mineral N availability proportioned by the trees. Recent studies in Galicia (NW Spain) have shown that the combination of broadleaf trees (Juglans regia L.) with wheat (Triticum aestivum) increased the production of this cereal compared with the tree-less areas. This might be linked to a reduction in understory light availability, which decreased the weed establishment in the understory, and the competition for light, nutrients, and water between the cereals and the weeds (Ferreiro-Domínguez et al., 2021). However, Kaushal and Verma (2003), in a study of above and belowground interactions of Grewia optiva and wheat, found that growth and yield of wheat were negatively affected below the tree crown while increasing with distance from the tree trunk. Therefore, tree species and crops’ choice is important and should be one of the first decisions. Selection of the “right species for the right place” depends on the goals, site conditions, and species traits and should be focused on complementary traits that maximize positive and minimize negative interactions between system components (Liu et al., 2018). It is expected that different aspects of climate change (e.g., higher temperatures and atmospheric CO2 concentration, and reduced rainfall), acting as a disturbance factor, may affect all system components and interactions between them (Luedeling et al., 2014), further complicating its management. In this sense, the selection of trees and crops better adapted to future environmental and climatic conditions (e.g., especially resistant to water, heat, and light stresses) is also extremely important in areas with drought periods, such as the Mediterranean, as was highlighted in the AFINET project by stakeholders. This selection should be based on identifying and quantifying functional traits associated with drought resistance (e.g., rooting volume, leaf arrangement, and leaf water potential).
Modeling Tree–Crop Interactions in Agroforestry Systems
Agroforests are complex systems whose management depends on their components and the interactions between them (Lehmann et al., 2020). Climate change will alter the interactions among components (Luedeling et al., 2014), which will inevitably affect the sustainability (Mbow et al., 2014) and productivity of the agroforestry systems (Jose et al., 2004) while contributing to climate change mitigation (Mbow et al., 2014). The growing need to consider the inherent complexity of agroforestry systems has fostered an increased interest in modeling approaches. The development of modeling tools that can incorporate multiple objectives, alternatives, and interests might be of great help for farmers, landowners, researchers, and policy-makers. These tools can integrate very diverse and complex interactions, typical of agroforestry systems, at a relatively low cost, effort, and time, for assisting in management decisions (Ellis et al., 2004). However, this is a very challenging task. When trees and crops are grown together, several changes occur over time and space, mainly in response to biophysical interactions, environmental conditions, and management options (e.g., the exact arrangement and placement of trees and crops). Integration of all these issues in modeling approaches is also challenging since models should represent processes accurately, and at the same time, be easy to use.
Several models have been developed for a wide range of environmental conditions, agroforestry practices, and purposes in recent years. Such models can be organized into six categories according to their goals (Burgess et al., 2019): (i) allometric models – models for estimating aboveground biomass and volume; (ii) models for describing the environmental impact of agroforestry practices on soil carbon, soil nutrients, and water flow (e.g., CO2FIX and SCUAF models); (iii) models for exploring tree and crop growth (e.g., ALWAYS, HyPAR, WaNuLCAS, Yield-SAFE, and Hi-sAFE); (iv) models for describing aboveground canopy architecture (e.g., AMAP model), belowground architecture (e.g., FracRoot), or both (e.g., NOTG model described by Simioni et al., 2016), i.e., architectural models; (v) models for assisting whole-farm decision-making (e.g., ARBUSTRA, Farm-SAFE, and Forage-SAFE), and finally, (vi) landscape models – models for determining the effect of agroforestry systems at the landscape scale. Most existing models present inadequate flexibility and require numerous parameters, many of which are hard to obtain, making model calibration and validation difficult (Luedeling et al., 2016). Under such circumstances, the risk of making maladaptive decisions is much higher, limiting model adoption, and application. Furthermore, most of the models mentioned above ignore the interactions within agroforestry systems, indicating that they are unlikely to capture their complexity adequately. In this sense, the model’s accuracy will inevitably be affected (Mishra et al., 2021). The need to correctly address the complex dynamics of tree–crop interactions and predict responses beyond known conditions has fostered process-based models instead of empirical ones (Oreske, 2003). Such models can simulate physiological processes involved in growth in response to abiotic factors such as soil, climate, or management (Luedeling et al., 2014). Among the many models that consider the interactions between trees and crops and include physiological oriented high-resolution approaches, the most relevant are WaNuLCAS – a model for water, nutrient, and light capture in agroforestry systems (van Noordwijk and Lusiana, 1998), Hi-sAFE, developed in the EU SAFE project (Silvoarable Agroforestry for Europe) that explores the competition between trees and crops for light, water, and nitrogen (Dupraz et al., 2019), Yield-SAFE, also created under the SAFE project, is designed to describe tree and crop yields (van der Werf et al., 2007), and HyPAR, which combines the continuous-canopy forest (Hybrid) and crop (PARCH) models, taking into account the competition for light and water (Mobbs et al., 1998). The overall characteristics of these models are presented in Figure 1.
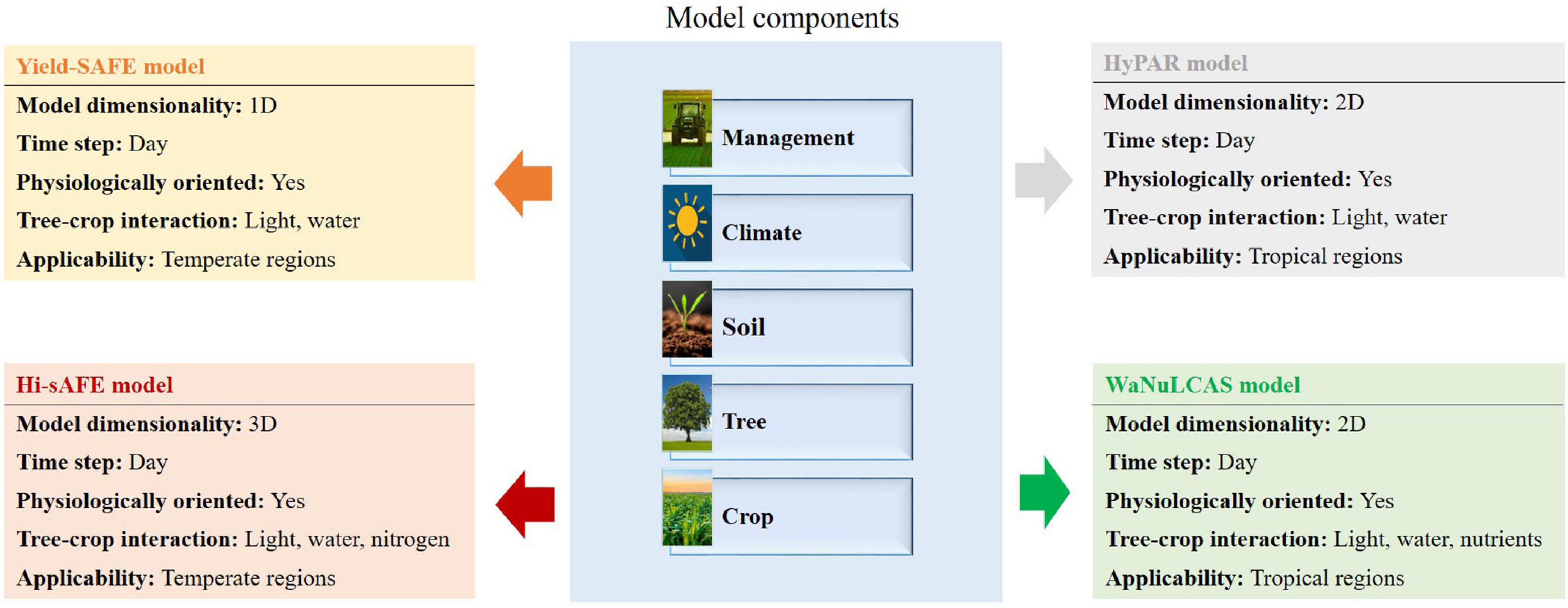
Figure 1. Overview of the most used agroforestry models, in relation to model components, model dimensionality, time steps, integration of physiological process, tree–crop interactions, and applicability.
The WaNuLCAS and the Hi-sAFE models are the most commonly used in tropical and European regions, respectively (Dupraz et al., 2019). WaNuLCAS is a dynamic model that has been used in a wide range of agroforestry systems ranging from hedgerow intercropping [e.g., maize (Hussain et al., 2016) and sugarcane (Pinto et al., 2005)] to fallow-crop mosaics or even isolated trees in parklands (van Noordwijk and Lusiana, 1998). Apart from successfully simulating soil erosion and runoff, nutrient dynamics, and carbon sequestration (Pansak et al., 2010), the WaNuLCAS model may also be used to answer questions related to vulnerability and adaptation associated with climate change mitigation (Luedeling et al., 2014). In turn, the Hi-sAFE model is a mechanistic 3D model that explores the interactions between the above and belowground components that govern the dynamics of light, water, and nitrogen (Dupraz et al., 2019). Simulations using the Hi-sAFE model proved to be a powerful tool for examining different agroforestry designs, management strategies, and environmental variation, including climate change (Dupraz et al., 2019). Compared to the WaNuLCAS model, the Hi-sAFE is easier to use, but it cannot predict long-term dynamics (Malézieux et al., 2009). For more details in both agroforestry models, please see van Noordwijk and Lusiana (1998); Malézieux et al. (2009), and Dupraz et al. (2019).
Final Considerations
Agroforestry systems have high landscape and ecological values and are strategic for biodiversity conservation and the livelihood of farmers, especially in tropical and Mediterranean regions. However, there is an urgent need to enhance their productivity and resource-use efficiency in the context of increasing populations and limited land. The selection of tree/crop combination is crucial for the success of agroforestry systems. Many researchers explored the potential mutual benefits to gain a better understanding and enable sustainable use of various species in agroforestry. However, one of the major obstacles to the widespread use of agroforestry systems is insufficient knowledge about the interactions between trees and crops, ranging from competition to facilitation. Therefore, more effort should be put into the characterization of species involved, for example, concerning their tolerance to shade, drought, heat, pests, diseases, and compatibility with other species, to reverse the negative impacts and maximize the positive influences of trees on crops. Since this requires dealing with trade-offs that depend on the environment, considering envisaged environmental changes in the near future is of particular importance. A change in aboveground resource availability necessarily changes the struggle for belowground resources and thus the relation between trees and crops. However, such effects are not well documented. Therefore, it will be of paramount importance to increase our knowledge of key processes that determine belowground interactions, such as root expansion and nutrient dynamics, and environmental dependencies.
To prepare agroforestry systems for more extreme conditions related to climate change, it might also be necessary to: (a) test new species combinations or management options; (b) to study improved crop technology that minimizes competition and maximizes facilitation; and (c) to breed and genetically modify plants to increase productivity and nutritional quality. Moreover, the development of agroforestry models capable of simulating the complex interactions between trees and crops at aboveground and belowground levels under future climate conditions, although difficult to achieve, should be a research priority. Similarly, the integration of physiological parameters, such as photosynthesis or transpiration, is a significant challenge but should be considered in modeling. With climate change, shifts in distribution and impacts of biotic constraints (pests, diseases, and invasive species) are an increasing concern, so these aspects should also be accounted for when modeling. A key future challenge of modeling agroforestry systems will be to balance the trade-offs between model complexity and the variety of interactions that are possible to simulate.
Author Contributions
BG: conceptualization and writing – original draft and review and editing. MM and SP: writing – review and editing. MM-L and MS: conceptualization and writing – review and editing. All authors contributed to the article and approved the submitted version.
Funding
This work was supported by the National Funds by FCT – Portuguese Foundation for Science and Technology, under the project UIDB/04033/2020. The authors also acknowledge the support of Ministerio de Economía y Competitividad (CTM2016-80176-C2-1-R) “Sistemas Agroforestales para la Producción de Cereal como Estrategia de Adaptación y Mitigación al Cambio Climático en el Ámbito de la Península Ibérica” (AFCLIMA).
Conflict of Interest
The authors declare that the research was conducted in the absence of any commercial or financial relationships that could be construed as a potential conflict of interest.
Publisher’s Note
All claims expressed in this article are solely those of the authors and do not necessarily represent those of their affiliated organizations, or those of the publisher, the editors and the reviewers. Any product that may be evaluated in this article, or claim that may be made by its manufacturer, is not guaranteed or endorsed by the publisher.
Acknowledgments
María Rosa Mosquera-Losada was supported by the Spanish Ministry of Economy and Competitivity through “Sistemas agroforestales para la producción de cereal como estrategia de adaptación y mitigación al cambio climático en el ámbito de la península ibérica” (AFCLIMA) (CTM2016-80176-C2-1-R) from the Retos Investigación Programme.
Supplementary Material
The Supplementary Material for this article can be found online at: https://www.frontiersin.org/articles/10.3389/fevo.2021.661978/full#supplementary-material
Supplementary Figure 1 | Advantages and constraints associated with agroforestry systems in comparison to monocultures.
Supplementary Figure 2 | Ecological interactions and potential effects on species. + and − denote positive and negative effects, respectively. 0 denotes no effect (adapted from Jose et al., 2004).
References
Arenas-Corraliza, M. G., López-Díaz, M. L., and Moreno, G. (2018). Winter cereal production in a Mediterranean silvoarable walnut system in the face of climate change. Agric. Ecosyst. Environ. 264, 111–118. doi: 10.1016/j.agee.2018.05.024
Barrios, E., Sileshi, G. W., Shepherd, K., and Sinclair, F. (2012). “Agroforestry and soil health: linking trees, soil biota and ecosystem services,” in Soil Ecology and Ecosystem Services, ed. D. H. Wall (Oxford: Oxford University Press), 315–330.
Bayala, J., and Prieto, I. (2020). Water acquisition, sharing and redistribution by roots: applications to agroforestry systems. Plant Soil 453, 17–28. doi: 10.1007/s11104-019-04173-z
Bayala, J., Heng, L. K., Noordwijk, M. V., and Ouedraogo, S. J. (2008). Hydraulic redistribution study in two native tree species of agroforestry parklands of West African dry savanna. Acta Oecol. 34, 370–378. doi: 10.1016/j.actao.06.2008010
Bayala, J., Sileshi, G., Coe, R., Kalinganire, A., Tchoundjeu, Z., Sinclair, F., et al. (2012). Cereal yield response to conservation agriculture practices in drylands of West Africa: a quantitative synthesis. J. Arid Environ. 78, 13–25. doi: 10.1016/j.jaridenv.2011.10.011
Burgess, P. J., Graves, A., García de Jalón, S., Palma, J. H. N., Dupraz, C., and van Noordwijk, M. (2019). “Modeling agroforestry systems,” in Agroforestry for Sustainable Agriculture. Burleigh Dodds Series in Agricultural Science 55, eds M. R. Mosquera-Losada and R. Prabhu (Cambridge: Burleigh Dodds Science Publishing), 209–238.
Bybee-Finley, K. A., and Ryan, M. R. (2018). Advancing intercropping research and practices in industrialized agricultural landscapes. Agriculture 8:80. doi: 10.3390/agriculture8060080
Campi, P., Palumbo, A. D., and Mastrorilli, M. (2009). Effects of tree windbreak on microclimate and wheat productivity in a Mediterranean environment. Eur. J. Agron. 30, 220–227. doi: 10.1016/j.eja.2008.10.004
Chapagain, T., Lee, E. A., and Raizada, M. N. (2020). The potential of multi-species mixtures to diversify cover crop benefits. Sustainability 12:2058. doi: 10.3390/su12052058
Dagar, J. C., and Gupta, S. R. (2020). “Agroforestry developments for degraded landscapes: a synthesis,” in Agroforestry for Degraded Landscapes, eds J. C. Dagar, S. R. Gupta, and D. Teketay (Singapore: Springer), 447–458.
De Stefano, A., and Jacobson, M. G. (2018). Soil carbon sequestration in agroforestry systems: a meta-analysis. Agrofor. Syst. 2, 285–299. doi: 10.1007/s10457-017-0147-9
Dupraz, C., Wolz, K. J., Lecomte, I., Talbot, G., Vincent, G., Mulia, R., et al. (2019). Hi-sAFe: a 3D agroforestry model for integrating dynamic tree–crop interactions. Sustainability 11:2293. doi: 10.3390/su11082293
Ellis, E. A., Bentrup, G., and Schoeneberger, M. M. (2004). Computer-based tools for decision support in agroforestry: current state and future needs. Agrofor. Syst. 61, 401–421.
FAO and IAEA (2008). Management of Agroforestry Systems for Enhancing Resource use Efficiency and Crop Productivity. Joint FAO/IAEA Division of Nuclear Techniques in Food and Agriculture, Vienna. Available online at: https://www-pub.iaea.org/MTCD/Publications/PDF/te_1606_web.pdf (accessed April 15, 2021).
FAO. (2004). The Ethics of Sustainable Agricultural Intensification. FAO Ethics Series 3.Rome: Food and Agriculture Organization of the United Nations, 3rd Edn. doi: ISBN 92-5-105067-8
Fernández-Núñez, E., Rigueiro-Rodríguez, A., and Mosquera-Losada, M. R. (2010). Carbon allocation dynamics one decade after afforestation with Pinus radiata D. Don and Betula alba L. under two stand densities in NW Spain. Ecol. Eng. 36, 876–890. doi: 10.1016/j.ecoleng.2010.03.007
Ferreiro-Domínguez, N., Papadopoulus, P., Rigueiro-Rodíguez, A., and Mosquera-Losada, M. R. (2021). “Wheat varieties established under walnut of different ages in Galicia (NW Spain),” in Proceedings of the 5th EURAF conference. Nuoro.
Ferreiro-Domínguez, N., Rigueiro-Rodríguez, A., Rial-Lovera, K. E., Romero-Franco, R., and Mosquera-Losada, M. R. (2016). Effect of grazing on carbon sequestration and tree growth that is developed in a silvopastoral system under wild cherry (Prunus avium L.). Catena 142, 11–20. doi: 10.1016/j.catena.2016.02.002
Gaba, S., Lescourret, F., Boudsocq, S., Enjalbert, J., Hinsinger, P., Journet, E. P., et al. (2015). Multispecies cropping systems as drivers for providing multiple ecosystem services: from concepts to design. Agron. Sustain. Dev. 35, 607–623. doi: 10.1007/s13593-014-0272-z
Gomes, L. C., Bianchi, F. J. J. A., Cardoso, I. M., Fernandes, R. B. A., Fernandes, E. I., and Schulte, R. P. O. (2020). Agroforestry systems can mitigate the impacts of climate change on coffee production: a spatially explicit assessment in Brazil. Agric. Ecosyst. Environ. 294:106858. doi: 10.1016/j.agee.2020.106858
Hussain, K., Wongleecharoen, C., Hilger, T., Ahmad, A., Kongkaew, T., and Cadisch, G. (2016). Modelling resource competition and its mitigation at the crop-soil-hedge interface using WaNuLCAS. Agrofor. Syst. 90, 1–20. doi: 10.1007/s10457-015-9881-z
Jose, S. (2009). Agroforestry for ecosystem services and environmental benefits: an overview. Agrofor. Syst. 76, 1–10. doi: 10.1007/s10457-009-9229-7
Jose, S., Gillespie, A. R., and Pallardy, S. G. (2004). Interspecific interactions in temperate agroforestry. Agrofor. Syst. 61, 237–255. doi: 10.1023/B:AGFO.0000029002.85273.9b
Kaushal, R., and Verma, K. S. (2003). Tree-crop interaction studies in natural agroforestry system: a case study from western Himalayas in India. Paper presented at the XII World Forestry Congress, Quebec, 0234–B1.
Kumar, P., Singh, R. P., Singh, A. K., and Kumar, V. (2014). Quantification and distribution of agroforestry systems and practices at global level. HortFlora Res. Spectr. 3, 1–6.
Kuyah, S., Oborn, I., and Jonsson, M. (2017). “Regulating ecosystem services delivered in agroforestry systems,” in Agroforestry, eds J. C. Dagar and V. P. Tewari (Singapore: Springer Nature), 797–815.
Lasco, R. D., Delfino, R. J. P., and Espaldon, M. L. O. (2014). Agroforestry systems: helping smallholders adapt to climate risks while mitigating climate change. Wires Clim. Change 5, 825–833. doi: 10.1002/wcc.301
Lehmann, L. M., Smith, J., Westaway, S., Pisanelli, A., Russo, G., Borek, R., et al. (2020). Productivity and economic evaluation of agroforestry systems for sustainable production of food and non-food products. Sustainability 12:5429.
Lin, B. (2011). Resilience in agriculture through crop diversification: adaptive management for environmental change. BioScience 61, 183–193. doi: 10.1525/bio.2011.61.3.4
Lin, B. B. (2007). Agroforestry management as an adaptive strategy against potential microclimate extremes in coffee agriculture. Agric. For. Meteorol. 144, 85–94. doi: 10.1016/j.agrformet.2006.12.009
Lin, B. B. (2010). The role of agroforestry in reducing water loss through soil evaporation and crop transpiration in coffee agroecosystems. Agric. For. Meteorol. 150, 510–518. doi: 10.1016/j.agrformet.2009.11.010
Liu, C. L. C., Kucma, O., and Krutovsky, K. V. (2018). Mixed-species versus monocultures in plantation forestry: development, benefits, ecosystem services and perspectives for the future. Glob. Ecol. Conserv. 15:e00419. doi: 10.1016/j.gecco.2018.e00419
Lohbeck, M., Bongers, F., Martinez-Ramos, M., and Poorter, L. (2016). The importance of biodiversity and dominance for multiple ecosystem functions in a human-modified tropical landscape. Ecology 97, 2772–2779. doi: 10.1002/ecy.1499
Luedeling, E., Kindt, R., Huth, N. I., and Koenig, K. (2014). Agroforestry systems in a changing climate challenges in projecting future performance. Curr. Opin. Environ. Sustain. 6, 1–7. doi: 10.1016/j.cosust.2013.07.013
Luedeling, E., Philip, J. S., Frédéric, B., Jules, B., Neil, I. H., Meine, V. N., et al. (2016). Field-scale modeling of tree-crop interactions: challenges and development needs. Agric. Syst. 142, 51–69. doi: 10.1016/j.agsy.2015.11.005
Mahieu, S., Metay, A., Brunel, B., and Dufour, L. (2016). Nitrogen fluxes in chickpea grown in Mediterranean agroforestry systems. Agrofor. Syst. 90, 313–324. doi: 10.1007/s10457-015-9856-0
Malézieux, E., Crozat, Y., Dupraz, C., Laurans, M., Makowski, D., Ozier-Lafontaine, H., et al. (2009). Mixing plant species in cropping systems: concepts, tools and models. A review. Agron. Sustain. Dev. 29, 43–62. doi: 10.1051/agro:2007057
Manson, D. G., Schmidt, S., Bristow, M., Erskine, P. D., and Vanclay, J. K. (2013). Species-site matching in mixed species plantations of native trees in tropical Australia. Agrofor. Syst. 87, 233–250. doi: 10.1007/s10457-012-9538-0
Mbow, C., Smith, P., Skole, D., Duguma, L., and Bustamante, M. (2014). Achieving mitigation and adaptation to climate change through sustainable agroforestry practices in Africa. Curr. Opin. Environ. Sustain. 6, 8–14. doi: 10.1016/j.cosust.2013.09.002
Mcneely, J., and Schroth, G. (2006). Agroforestry and biodiversity conservation traditional practices, present dynamics, and lessons for the future. Biodivers. Conserv. 15, 549–554. doi: 10.1007/s10531-005-2087-3
Mishra, A. K., Sinha, B., Kumar, R., Barth, M., Hakkim, H., Kumar, V., et al. (2021). Cropland trees need to be included for accurate model simulations of land-atmosphere heat fluxes, temperature, boundary layer height, and ozone. Sci. Total Environ. 751:141728. doi: 10.1016/j.scitotenv.2020.141728
Mobbs, D. C., Cannell, M. G. R., Crout, N. M. J., Lawson, G. J., Friend, A. D., and Arah, J. (1998). Complementarity of light and water use in tropical agroforests I. Theoretical model outline, performance and sensitivity. For. Ecol. Manag. 102, 259–274.
Montagnini, F. (2017). “Introduction: challenges for agroforestry in the new millennium,” in Integrating Landscapes: Agroforestry for Biodiversity, ed. F. Montagnini (New York, NY: Springer International Publishing), 3–10.
Montagnini, F., and Nair, P. K. R. (2004). Carbon sequestration: an underexploited environmental benefit of agroforestry systems. Agrofor. Syst. 61, 281–295. doi: 10.1023/B:AGFO.0000029005.92691.79
Montagnini, F., Cusack, D., Petit, B., and Kanninen, M. (2004). Environmental services of native tree plantations and agroforestry systems in Central America. J. Sustain. For. 21, 51–67. doi: 10.1300/J091v21n01_03
Moreno, G., Aviron, S., Berg, S., Crous-Duran, J., Franca, A., García de Jalón, S., et al. (2018). Agroforestry systems of high nature and cultural value in Europe: provision of commercial goods and other ecosystem services. Agrofor. Syst. 92, 877–891. doi: 10.1007/s10457-017-0126-1
Mosquera-Losada, M. R., Borek, R., Balaguer, F., Mezzrila, G., and Ramos-Font, E. (2017). Agroforestry as a Mitigation and Adaptation Tool. EIP-AGRI Focus Group Agroforestry. Available online at: https://ec.europa.eu/eip/agriculture/sites/default/files/fg22 (accessed April 15, 2021).
Mosquera-Losada, M. R., Rigueiro-Rodríguez, A., and Ferreiro-Domínguez, N. (2015). Effect of liming and organic and inorganic fertilization on soil carbon sequestered in macro-and microaggregates in a 17-year old Pinus radiata silvopastoral system. J. Environ. Manage. 150, 28–38. doi: 10.1016/j.jenvman.2014.10.015
Mosquera-Losada, M. R., Santiago-Freijanes, F. J., Rigueiro-Rodríguez, A., Rodríguez-Rigueiro, F. J., Arias-Martínez, D., Pantera, A., et al. (2020). “The importance of agroforestry systems in supporting biodiversity conservation and agricultural production: a European perspective,” in Reconciling Agricultural Production with Biodiversity Conservation, eds P. Barberi and A. C. Moonen (Cambridge: Burleigh Dodds Series in Agricultural Science).
Mosquera-Losada, M. R., Santiago-Freijanes, J. J., Rois-Díaz, M., Moreno, G., Herder, M. D., Aldrey-Vázquez, J. A., et al. (2018). Agroforestry in Europe: a land management policy tool to combat climate change. Land Use Policy 78, 603–613. doi: 10.1016/j.landusepol.2018.06.052
Muthuri, C., Ong, C., Craigon, J., Mati, B., Ngumi, V. W., and Black, C. R. (2009). Gas exchange and water use efficiency of trees and maize in agroforestry systems in semi-arid Kenya. Agric. Ecosyst. Environ. 129, 497–507. doi: 10.1016/j.agee.2008.11.001
Nasielski, J., Furze, J. R., Tan, J., Bargaz, A., Thevathasan, N. V., and Isaac, M. E. (2015). Agroforestry promotes soybean yield stability and N2-fixation under water stress. Agron. Sustain. Dev. 35, 1541–1549. doi: 10.3390/agronomy10010052
Navas, R., and Silva, R. J. (2016). Ecological restoration indicators in agroforestry systems in the Atlantic forest. Ciência e Natura, Santa Maria 38, 656–664. doi: 10.5902/2179-460X19666
Ninkovic, V., Rensing, M., Dahlin, I., and Markovic, D. (2019). Who is my neighbor? Volatile cues in plant interactions. Plant Signal. Behav. 14:1634993. doi: 10.1080/15592324.2019.1634993
Novoplansky, A. (2009). Picking battles wisely: plant behaviour under competition. Plant Cell Environ. 32, 726–741. doi: 10.1111/j.1365-3040.2009.01979.x
Ong, C., Black, C. R., Wilson, J., Muthuri, C., Bayala, J., and Jackson, N. A. (2014). “Agroforestry: hydrological impacts,” in Encyclopedia of Agriculture and Food Systems, ed. N. van Alfen (San Diego, CA: Elsevier), 244–252.
Oreske, N. (2003). “The role of quantitative models in science,” in Models in Ecosystem Science, eds C. D. Canham, J. Cole, and W. Lauenroth (Princeton, NJ: Princeton University Press), 13–31.
Pansak, W., Hilger, T., Lusiana, B., Kongkaew, T., Marohn, C., and Cadisch, G. (2010). Assessing soil conservation strategies for upland cropping in Northeast Thailand with the WaNuLCAS model. Agrofor. Syst. 79, 123–144. doi: 10.1007/s10457-010-9290-2
Pasalodos-Tato, M., Pukkala, T., Rigueiro-Rodríguez, A., Fernández-Núñez, E., and Mosquera-Losada, M. R. (2009). Optimal management of Pinus radiata silvopastoral systems established on abandoned agricultural land in Galicia (North-Western Spain). Silva Fennicia. 43, 831–845.
Petit, B., and Montagnini, F. (2006). Growth in pure and mixed plantations of tree species used in reforesting rural areas of the humid region of Costa Rica, Central America. For. Ecol. Manage. 233, 338–343. doi: 10.1016/j.foreco.2006.05.030
Pierik, R., Ballare, C. L., and Dicke, M. (2014). Ecology of plant volatiles: taking a plant community perspective. Plant Cell Environ. 37, 1845–1853. doi: 10.1111/pce.12330
Pierik, R., Mommer, L., and Voesenek, L. A. (2013). Molecular mechanisms of plant competition: neighbour detection and response strategies. Funct. Ecol. 27, 841–853. doi: 10.1111/1365-2435.12010
Pinto, L. F., Bernardes, M., van Noordwijk, M., Pereira, A., Lusiana, B., and Mulia, R. (2005). Simulation of agroforestry systems with sugarcane in Piracicaba. Brazil. Agric. Syst. 86, 275–292.
Righi, C. A., Bernardes, M. S., Lunz, A. M. P., Pereira, C. R., Dourado Neto, D., and Favarin, J. L. (2007). Measurement and simulation of solar radiation availability in relation to the growth of coffee plants in an agroforestry system with rubber trees. Revista Árvore 31, 195–207. doi: 10.1590/S0100-67622007000200002
Rigueiro-Rodríguez, A., Fernández-Núñez, E., González-Hernández, P., McAdam, J. H., and Mosquera-Losada, M. R. (2009). “Agroforestry systems in Europe: productive, ecological and social perspectives,” in Agroforestry in Europe. Current Status and Futures Prospects, eds A. Rigueiro-Rodróguez, J. McAdam, and M. R. Mosquera-Losada (Dordrecht: Springer), 43–66.
Rodríguez-Rigueiro, F. J., Santiago-Freijanes, J. J., Mosquera-Losada, M. R., Castro, M., Silva-Losada, P., Pisanelli, A., et al. (2021). Silvopasture policy promotion in European Mediterranean Areas. PLoS One 16:e0245846. doi: 10.1371/journal.pone.0245846
Rosati, A., Borek, R., and Canali, S. (2020). Agroforestry and organic agriculture. Agrofor. Syst. 95, 805–821. doi: 10.1007/s10457-020-00559-6
Rosenstock, T. S., Tully, K. L., Arias-Navarro, C., Neufeldt, H., Butterbach-Bahl, K., and Verchot, L. V. (2014). Agroforestry with N2-fixing trees: sustainable development’s friend or foe? Curr. Opin. Environ. Sustain. 6, 15–21. doi: 10.1016/j.cosust.2013.09.001
Santiago-Freijanes, J. J., Mosquera-Losada, M. R., Rois-Díaz, M., Ferreiro-Domínguez, N., Pantera, A., Aldrey, J. A., et al. (2018). Global and European policies to foster agricultural sustainability: agroforestry. Agrofor. Syst. 95, 775–790. doi: 10.1007/s10457-018-0215-9
Schluter, D. (2000). Ecological character displacement in adaptive radiation. Am. Nat. 156, 4–16. doi: 10.1016/0169-5347(93)90098-A
Sileshi, G. W., Debusho, L. K., and Akinnifesi, F. K. (2012). Can integration of legume trees increase yield stability in rainfed maize cropping systems in Southern Africa? Agron. J. 104, 1392–1398. doi: 10.2134/agronj2012.0063
Simioni, G., Marie, G., and Huc, R. (2016). Influence of vegetation spatial structure on growth and water fluxes of a mixed forest: results from the NOTG 3D model. Ecol. Model. 328, 119–135. doi: 10.1016/j.ecolmodel.2016.02.004
Tilman, D. (1982). Resource Competition and Community Structure. Princeton, NJ: Princeton University Press.
Tsiafouli, M. A., Thébault, E., Sgardelis, S. P., de Ruiter, P. C., van der Putten, W. H., Birkhofer, K., et al. (2015). Intensive agriculture reduces soil biodiversity across Europe. Glob. Change Biol. 21, 973–985. doi: 10.1111/gcb.12752
Udawatta, R. P., Rankoth, L., and Jose, S. (2019). Agroforestry and biodiversity. Sustainability 11:2879. doi: 10.3390/su11102879
van der Werf, W., Keesman, K., Burgess, P., Graves, A., Pilbeam, D., Incoll, L. D., et al. (2007). Yield-SAFE: a parameter-sparse, process-based dynamic model for predicting resource capture, growth, and production in agroforestry systems. Ecol. Eng. 29, 419–433. doi: 10.1016/j.ecoleng.2006.09.017
van Noordwijk, M., and Lusiana, B. (1998). WaNuLCAS, a model of water, nutrient and light capture in agroforestry systems. Agric. Syst. 43, 217–242. doi: 10.1023/A:1026417120254
van Noordwijk, M., Lawson, G., Hairiah, K., and Wilson, J. (2015). “Root distribution of trees and crops: competition and/or complementarity,” in Tree-Crop Interactions: Agroforestry in a Changing Climate, eds C. K. Ong, C. R. Black, and J. Wilson (Wallingford: CAB International), 221–257.
van Noordwijk, M., Lawson, G., Soumaré, A., Groot, J. J. R., and Hairiah, K. (1996). “Root distribution of trees and crops: competition and/or complementarity,” in Tree–Crop Interactions: A Physiological Approach, eds C. K. Ong and P. Huxley (Wallingford: CAB International), 319–364.
van Oijen, M., Dauzat, J., Harmand, J. M., Lawson, G., and Vaast, P. (2010). Coffee agroforestry systems in Central America: i. A review of quantitative information on physiological and ecological processes. Agrofor. Syst. 80, 341–359. doi: 10.1007/s10457-010-9294-y
Vodouhe, F., Ousmane, C., Biaou, G., and Sinsin, B. (2011). Traditional agroforestry systems and biodiversity conservation in Benin (West Africa). Agrofor. Syst. 82, 1–13. doi: 10.1007/s10457-011-9377-4
Wahid, A., Gelani, S., Ashraf, M., and Foolad, M. R. (2007). Heat tolerance in plants: an overview. Environ. Exp. Bot. 61, 199–223. doi: 10.1016/j.envexpbot.2007.05.011
Weston, L. A., and Mathesius, U. (2013). Flavonoids: their structure, biosynthesis and role in the rhizosphere, including allelopathy. J. Chem. Ecol. 39, 283–297. doi: 10.1007/s10886-013-0248-5
Yamawo, A. (2015). Relatedness of neighboring plants alters the expression of indirect defense traits in an extrafloral nectary-bearing plant. Evol. Biol. 42, 12–19. doi: 10.1007/s11692-014-9295-2
Zomer, R. J., Neufeldt, H., Xu, J., Ahrends, A., Bossio, D., Trabucco, A., et al. (2016). Global tree cover and biomass carbon on agricultural land: the contribution of agroforestry to global and national carbon budgets. Sci. Rep. 6, 1–12. doi: 10.1038/srep29987
Keywords: modeling, ecosystem service, plant physiology, global warming, agroforestry systems, monocultures
Citation: Gonçalves B, Morais MC, Pereira S, Mosquera-Losada MR and Santos M (2021) Tree–Crop Ecological and Physiological Interactions Within Climate Change Contexts: A Mini-Review. Front. Ecol. Evol. 9:661978. doi: 10.3389/fevo.2021.661978
Received: 31 January 2021; Accepted: 22 September 2021;
Published: 08 October 2021.
Edited by:
Ruscena P. Wiederholt, Everglades Foundation, United StatesReviewed by:
Rüdiger Grote, Karlsruhe Institute of Technology (KIT), GermanyBradley S. Case, Auckland University of Technology, New Zealand
Copyright © 2021 Gonçalves, Morais, Pereira, Mosquera-Losada and Santos. This is an open-access article distributed under the terms of the Creative Commons Attribution License (CC BY). The use, distribution or reproduction in other forums is permitted, provided the original author(s) and the copyright owner(s) are credited and that the original publication in this journal is cited, in accordance with accepted academic practice. No use, distribution or reproduction is permitted which does not comply with these terms.
*Correspondence: Berta Gonçalves, bertag@utad.pt