- 1Bargerveen Foundation, Nijmegen, Netherlands
- 2Department of Animal Ecology and Physiology, Radboud University Nijmegen, Nijmegen, Netherlands
- 3B-WARE Research Centre, Radboud University Nijmegen, Nijmegen, Netherlands
Background: Nitrogen (NOx, NHy) and acidifying (NOx, NHy, SOx) deposition has reduced the biodiversity of European dry heathlands. Restoration efforts such as sod-cutting (removal of vegetation, litter and humus layer) often shifted these systems from N to P limitation and have had limited success in restoring the invertebrate community. Possible reasons for this include the unresolved acidification and a change in food plant stoichiometry. Here, we investigate how liming and P addition change food nutritional quality and their consequences for invertebrate performance.
Methods: We performed feeding experiments with field crickets (Gryllus campestris), using plant material collected from a full factorial field experiment with liming and P addition. We related female reproduction as measure of individual fitness to elemental ratios of plants fed to the crickets.
Results: P addition stimulated cricket daily reproduction and shortened their reproductive period, resulting in no difference in total reproduction. Liming greatly reduced both daily and total reproduction and resulted in more females cannibalizing on their male mates. Females that did so could partly offset the liming induced reduction in reproduction, suggesting dietary deficiency. P-addition improved food quality (lower N:P ratios) while liming led to skewed Mn:Mg and Fe:Mg ratios that compare unfavorably to ratios found in terrestrial invertebrates.
Conclusion: Increased plant N:P ratio following sod-cutting constrains the reproductive potential in Gryllus campestris in a non-linear way. Liming reduced nutritional quality, likely by inducing deficiencies in Fe or Mn.
Management Implications: High-impact restoration management practices such as sod cutting and liming cause new problems for invertebrates rooted in ecological stoichiometry. Since P-addition only partially offsets these negative effects, we instead advocate the use of less intensive N removal management and weaker buffering agents to reduce soil acidification. Furthermore, a reduction in N emission is paramount as it will remove the need for disruptive interventions.
Introduction
Increased deposition of nitrogen (NOx, NHy) alter soil biochemical cycles (Penuelas et al., 2020), result in increased acidification rates (Stevens et al., 2009; Tian and Niu, 2015) and impact plant and invertebrate diversity (Haddad et al., 2000; Bobbink et al., 2010; Stevens et al., 2010). Western European dry heathlands are naturally oligotrophic and poorly buffered systems and therefore highly vulnerable to increased N (NOx, NHy) and acidifying (NOx, NHy, SOx) deposition (Bobbink and Heil, 1993). In North Western Europe, current annual N deposition rates often exceed critical loads, and intensified management is needed to prevent N accumulation (Jones et al., 2017). In dry heathland areas subjected to high N deposition, restoration management focuses on maximizing removal of biomass and consequently N, using high-impact measures such as sod cutting (removal of vegetation, litter and humus layer) across large scales (Diemont, 1996; Webb, 1998; Härdtle et al., 2006). Such high-impact, large scaled management practices have been criticized however, as they can harm invertebrate populations due to disturbance or habitat destruction and homogenization (Maes et al., 2017). Also, current management aimed at maximizing N removal often indiscriminately removes other nutrients as well, including P and base cations. This has led to undesirable shifts in soil nutrient stoichiometry, shifting these systems from N to P limitation (De Graaf et al., 1998; Härdtle et al., 2009; Vogels et al., 2020). Increased availability of inorganic N greatly increases aboveground plant tissue N:P ratios (Penuelas et al., 2020) and decreased plant available P has been found to further increase plant tissue N:P ratios in dry heathlands (Vogels et al., 2017). Such shifts in plant tissue N:P ratio has been implicated in the decline of dry heathland invertebrate diversity (Vogels et al., 2017). Compared to plants, invertebrates have in general a lower tissue N:P ratio and also a lower plasticity in this and other macronutrient ratios (Elser et al., 2000a). Consequently, shifts in plant tissue N:P ratio can greatly impact invertebrate population composition and dynamics (Sterner and Elser, 2002). In European dry heathlands that underwent sod-cutting management, there is a need to address how stoichiometric mismatches created by sod-cutting can be mitigated, and to test whether such mitigation is effective in restoring invertebrate diversity.
In addition to creating stoichiometric imbalances, management aimed at N removal does not restore soil buffer capacity. Increased soil acidity is known to hamper the restoration of characteristic heathland flora and fauna as well (Bobbink et al., 1998; Roem and Berendse, 2000; Roem et al., 2002; De Graaf et al., 2009; Nijssen et al., 2017), and additional measures to increase soil buffer capacity are often necessary in order to achieve restoration goals (Dorland et al., 2003, 2004; Vogels et al., 2020). Increased acidification results in changed elemental content of plant tissue, reducing cations (Ca, Mg, Na, and K), and increasing Al and heavy metal (Cd and Pb) content of plants (Gjengedal et al., 2015). Depending on soil type and species, concentrations and ratios of trace elementals (Mn, Cu, and Zn) can also shift considerably. Such shifts in elemental content or ratios can ultimately result in a significant reduction in uptake of essential trace elements by invertebrate consumers. For example, reduced Ca intake resulting from soil acidification has been linked to reduced hatchling success in insectivorous birds (Graveland et al., 1994; Graveland and vanderWal, 1996; Graveland, 1998). Whether such changes also negatively impact fitness in invertebrates is however largely unexplored (Nijssen et al., 2017). Variation in plant (trace) elemental content has however been found to influence invertebrate herbivore density and their species composition (Joern et al., 2011), the degree of herbivory (Borer et al., 2019) and the fitness of honeybees (Filipiak et al., 2017), suggesting that the availability of these elements play a role in the ecological functioning of invertebrate communities. Deficiencies that arise may however likely relate to trace elements other than Ca, as demands are much lower in invertebrates compared to birds and other vertebrates (Sterner and Elser, 2002).
Here we test the effectiveness of additional management following sod-cutting from an invertebrate’s perspective. Specifically we examine the effects of liming (to ameliorate acidification), P addition (to resolve P limitation created by sod-cutting) and their interaction, using the field cricket (Gryllus campestris) as model species. This is a large insect and mostly confined to xero-thermophilic habitats in its northern distribution range. Dry heathland is the typical habitat of this species in the area of study (Kleukers et al., 1997). It overwinters as half grown nymph, adults emerge in early to mid-spring. Female crickets are able to produce a large amount of eggs, which develop without diapause and hatch approximately 2 weeks after oviposition (Ingrisch and Köhler, 1998). Earlier feeding experiments with plant tissue differing in N:P ratio indicated that this species can exhibit P limited growth (Vogels et al., 2013). We expected that P replenishment as well as liming would improve the nutritional quality of plant tissue quality for female Gryllus campestris, by reducing plant N:P ratio to more optimal conditions (P-replenishment), and by increasing Ca, Mg and trace elemental concentration in food plants via reduced root Al stress, increasing root elemental uptake efficiency. We tested this by performing a climate controlled feeding experiment using field collected plant material from a full factorial management experiment in recently sod cut dry heathland vegetation (see Siepel et al., 2018; Vogels et al., 2020 for soil mesofauna and vegetation effects) as food source and used female reproductive success as measure of fitness response.
Materials and Methods
Field Experiment
Experimental plots were established in Hoge Veluwe National Park, in the center of the Netherlands (52°.2’0.30”N; 5°.49’0.50”) at a site that underwent sod cutting management in the first quarter of 2012. Before sod cutting the vegetation was highly dominated by Molinia caerulea. At the start of the experiment in autumn 2012, the soil was still bare, no vegetation had yet established. For more detailed information on the experimental site and set-up see Siepel et al. (2018) and Vogels et al. (2020).
Experimental plots measured 15 m × 15 m in size and were placed in a randomized block design each consisting of four plots. In total five blocks were installed (n = 5). On the plots, the reduction of soil acidity treatment (further referred as Ca+, but note that Mg was also added in this treatment) was achieved by addition of dolomite (200 g.m–2 granular Dolokal; 80% CaCO3 and 20% MgCO3). P-replenishment treatment (further referred as P+) was achieved by adding 5 g P⋅m–2 by spraying an aqueous solution of NaH2PO4⋅2H2O, adding in total 5.67 kg NaH2PO4⋅2H2O per plot. P+ treatment (early April 2013) was carried out substantially later than Ca+ treatment (December 2012), in order to avoid high PO43– sorption resulting from liming acid soils, as reported by Haynes (1982). Each plot received either P+ or no addition (P–), Ca+ or no addition (Ca–), resulting in four treatment combinations P+Ca+, P+ Ca–, P–Ca+ and P–Ca–. Vegetation was then allowed to develop naturally for two growing seasons in order to provide sufficient amount of food for the feeding experiment.
Feeding Experiment
Food Acquirement and Preparation
Vegetation for food preparation was collected at the experimental sites in April and May 2014. In all plots, the dominant graminoid (Molinia caerulea) and ericoid (Calluna vulgaris) was collected separately by clipping aboveground living biomass. The obtained plant tissue was pooled by treatment combination, using an equal volume of plant material per individual plot. In addition to bulk collected aboveground biomass for food preparation, smaller samples of Calluna and Molinia shoots were collected as well and stored for all individual plots and for both species separately for later analysis of elemental content. At the same day of collection, all obtained bulk vegetation samples were dried for 48 h at 60°C. Dried samples were then finely ground and homogenized at equal amounts based on dry mass. In order to produce food pellets that were easier to handle, the following procedure was done for each experimentally manipulated food. A 5 g l–1 agar solution was prepared in distilled water at 100°C. Each food stock was suspended in the agar solution after cooling of the solution to 60°C in batches of 15 g of stock in 45 ml solution. Each batch was transferred on a 10 × 10 cm patch of filter paper, spread out to a maximum thickness of 0.5 cm, cut in small squares of approximately 1 cm2 and subsequently dried in an oven for 48 h at 60°C. After drying, each food batch was separated from the filter paper and broken into pellets using the cut lines. Pellets from each treatment combination were stored at 5°C in closed jars.
Breeding Experiment
A climate controlled breeding chamber was used in which temperature was kept constant at 20°C and relative humidity at 55% (±5%). In order to reflect the light/dark regime in the field during the peak reproductive period of field crickets, artificial light regime was held constant at 16/8 h L/D. Field crickets were obtained in April 2014 by collecting penultimate and ultimate nymphal stages in the field in a nearby site within the National Park The Hoge Veluwe. Crickets were then transported to a climate controlled breeding chamber and transferred to separate plastic containers, which were randomly assigned to receive one of the four foods obtained from the field experiment. Containers (Figure 1) measured 17 × 10 × 7 cm (L × W × H). In each container, ca. 0.9 g of food pellets were offered on a 3 cm diameter petri dish. To allow for drinking, a water filled petri dish covered with gauze in which a piece of filter paper was stuck into the water was placed in each container. To allow for hiding, a tube of 4.7 cm length and 1.4 cm diameter covered in black plastic tape was placed in the container. To allow females to deposit eggs, each container was filled with 3 cm of sand which was kept moist by regular spraying with tap water. At intervals of (2)–3–4 days, water was refreshed and food pellets were replaced in each container. The remaining food pellets were often too fragmented to allow for weighing of residual food biomass. In general, food pellets were eaten to a high degree (90% or more of food consumed), unless pellets showed clear signs of fungal decay. Occurrence of fungal decay did not differ between treatments (32.6; 34.4; 31.0; and 35.5% for P+Ca+; P+Ca–; P–Ca+ and P– Ca–, respectively), and was often limited to one of the several food pellets offered. At inset of the experiment, and at each refreshment period, nymphs and adults were weighed on a microbalance. At the start of the experiment, 14 female nymphs were assigned to each food type, resulting in a total of 56 individuals. Following molt into the adult stage, females were kept from mating with males in a 2-week abstinence period in order to minimize prior field effects on nutrient and energy reserves and to allow for eggs to fully develop. After this period, males were placed with females in the same container in order to allow for mating to take place. Initially, males were kept with females constantly. However, in the first week of the experiment, a high degree of cannibalism by the females became apparent. Subsequently, males were kept with females for 1 day each week, which proved effective in eliminating the occurrence of cannibalism, while still allowing females to successfully mate (confirmed by the presence of spermatophores). During the reproductive period, containers were replaced at each of the 3–4 days refreshment intervals by new containers with a layer of fresh sand. Each rearing container was labeled by time period of female present, female identity and food treatment. Containers were subsequently incubated under the same climate regime and regularly sprayed with tap water to prevent dehydration of eggs. Containers were subsequently checked for hatched first instar nymphs at least once every 2 days. First hatchlings typically emerged two and a half weeks after replacement. Hatched nymphs were removed from each container using an insect exhauster and counted regularly until no nymphs were found for 17 consecutive days in the respective container. For each individual, date of mortality was recorded in order to obtain the length of the reproductive period for each individual. The feeding experiment ended when the last female died (88 days after adult eclosion), but counting of hatched nymphs continued for a longer period, by following the aforementioned procedure.
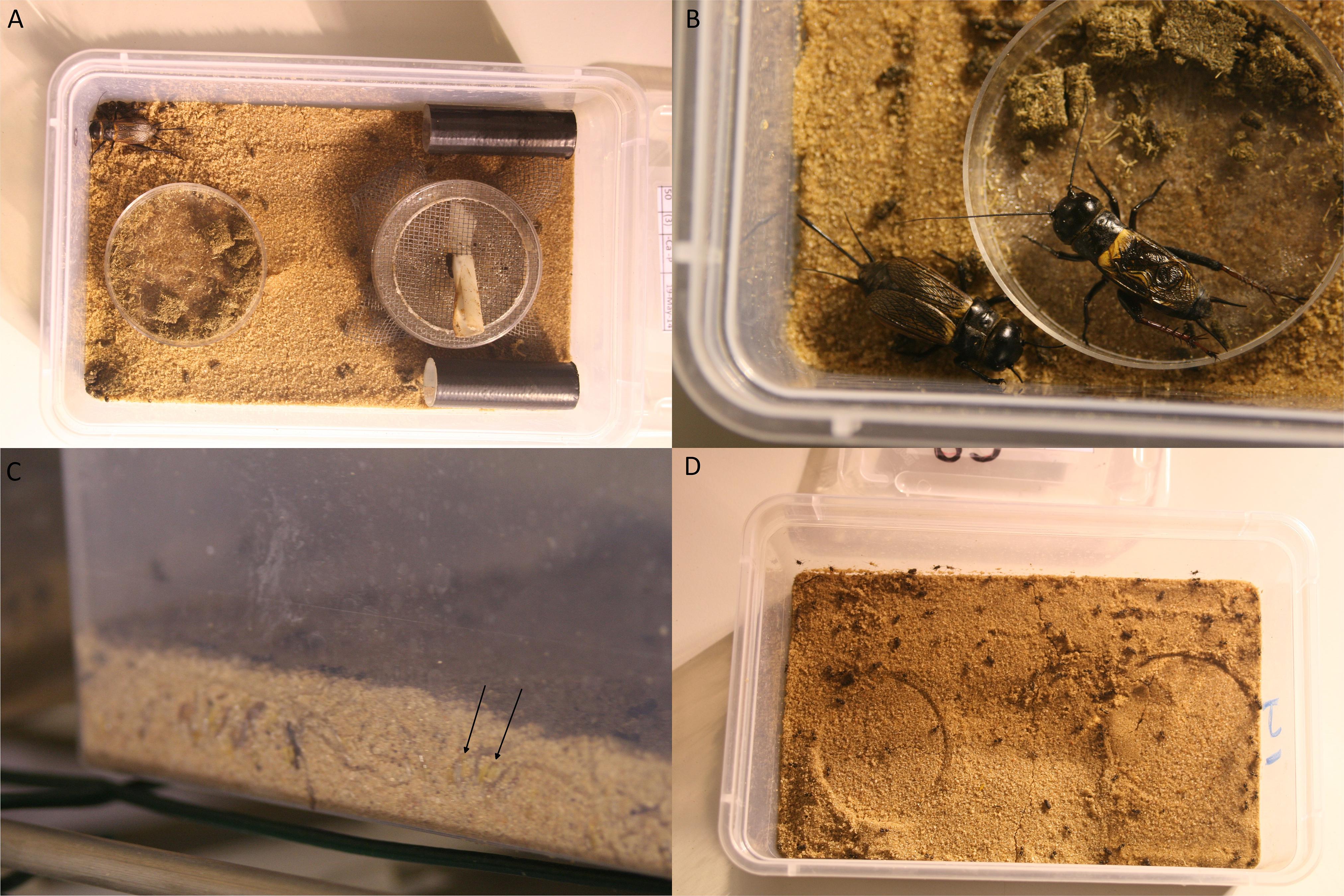
Figure 1. Overview of the experimental setup of the feeding experiment. (A) Overview of experimental container (lid removed). (B) Adult male and female field cricket in feeding container. (C) Deposited eggs visible at the sand/container interface (2 of them indicated with arrows). (D) Hatched first instar nymphs in container.
Food Quality
Collected Molinia and Calluna shoots were analyzed separately for elemental content. 200 mg of dried plant material was digested using 4 ml HNO3 (65%) and 1 ml H2O2 (35%) in teflon vessels using an Ethos D microwave (1200 MLS, Milestone, Sorisole, Italy), after which digests were analyzed for phosphorus (P), calcium (Ca), aluminum (Al), iron (Fe), potassium (K), magnesium, (Mg), manganese (Mn), sulfur (S), silicon (Si) and zinc (Zn) using an inductively coupled plasma emission spectrophotometer (ICP-OES; model IRIS Intrepid II XDL, Thermo Fisher Scientific, Franklin, United States). C and N contents of 3 mg homogenized dried plant material were determined by an elemental CNS analyzer (model NA 1500, Carlo Erba; Thermo Fisher Scientific, Franklin, United States). Food elemental content was calculated using the mean of Calluna + Molinia nutrient content, thus reflecting the food quality of the food mixture offered in the experiment.
Data Analysis
Breeding Experiment
Prior to analyzing the effect of treatments, we assessed whether other life-history parameters could have influenced reproduction, either dependent or independent of treatment. We analyzed whether initial weight, weight at start of mating, weight change and female reproductive lifespan (time between start of mating and death of the female in days) differed between food treatments, by fitting P×Ca, P+Ca, P, Ca and intercept only predictor models and compared these using the model AIC scores and AIC-derived model fit parameters (Burnham and Anderson, 2002). We subsequently analyzed the model output of the minimum adequate model or second ranked model, in case the intercept only model was the minimum adequate model. Only for female reproductive lifespan, a significant negative treatment effect of P addition was apparent (Supplementary Figure 1 and Supplementary Tables 1, 2). Apart from life history parameters, we analyzed whether the observed incidences of cannibalism were influenced by the experimental food treatments. Based on the preliminary analyses, we used total number of hatched nymphs as well as daily reproduction, calculated as total number of hatched nymphs/female reproductive lifespan as measure of reproductive success. Since occurrence of cannibalism was significantly influenced by Ca+ treatment (see Results section “Occurrence of cannibalism”), we also included the occurrence of cannibalism as a covariate in the models, and present the results separately for all females and all non- cannibalistic females. Responses in reproductive parameters from cannibalistic females only are provided in Supplementary Figure 3. In assessing the effects of treatments on reproduction, we used an information theoretic approach by fitting generalized linear models varying between maximum (P × Ca × cannibalism) and minimum (intercept only) number of parameters and comparing the degree of fit of these models using the model AIC scores and AIC-derived model fit parameters (Burnham and Anderson, 2002). In order to compare the effect of additional parameters on model outcome with the minimum adequate model, second ranking model outcomes are reported as well. A total overview of model ranking results is provided in Supplementary Table 3. In order to further assess the influence of cannibalism on model outcome, the same model procedures, excluding cannibalism as covariate, was performed on reproduction data from non-cannibalistic females. The results of this secondary analysis procedure are summarized in Supplementary Tables 4, 5. Total hatched eggs models were fitted using a negative binomial GLM with log link, daily reproduction models were fitted using a Gamma GLM with identity link.
Food Quality
Treatment effects on food elemental content and elemental ratios were analyzed using linear regression using an initial full factorial (P × Ca) model formulation. When no significant effect of the interaction term was apparent from this model, this parameter was dropped from the final model. An exception was made for plant tissue P, where a near significant effect for the P × Ca interaction was kept in the model in order to better compare model results with tissue N:P ratio (containing a significant P × Ca interaction term). In order to meet normality assumptions, elemental ratios were log-transformed prior to the analysis.
Comparisons of Food and Invertebrate Elemental Stoichiometry
We explored possible causal mechanisms underlying the observed responses in the feeding experiment by comparing data of invertebrate macro and micro-elemental content from literature (Elser et al., 2000a), a previously obtained dataset of elemental content of invertebrate taxa (Supplementary Table 6), and by comparing results with a heathland vegetation feeding experiment with Gryllus campestris performed earlier, which differed markedly in plant tissue N:P stoichiometry (for results and methods, see Vogels et al., 2013).
Results
Breeding Experiment
Occurrence of Cannibalism
The frequency of occurrence of cannibalism in female crickets during the first week of allowed male mating was significantly higher in the lime addition (Ca+ treatment) groups compared to the Ca– groups (Figure 2, Ca+: 46.4%, Ca–: 14.3%, χ2 = 5.41, p = 0.02), but not in the P addition (P+ treatment) groups compared to the P– groups (Figure 2, P+: 35.7%, P–: 25%, χ2 = 0.34, p = 0.56).
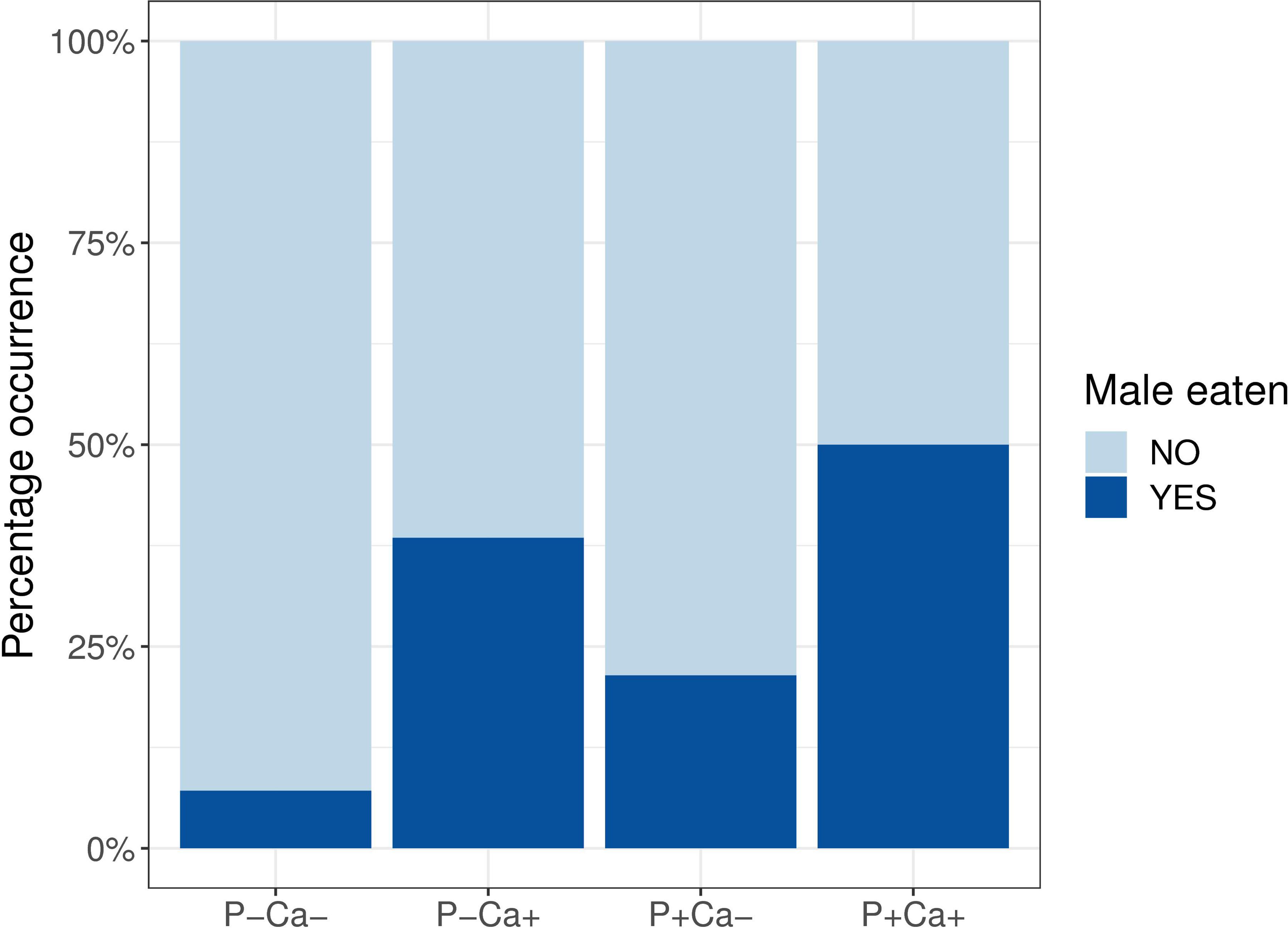
Figure 2. Bar plot showing percentage occurrence of cannibalism by female crickets at the initial phase of the experiment for each food treatment combination. P+/P–: P treatment contrasts; Ca+/Ca–: lime treatment contrasts.
Reproduction
Total number of hatched eggs was best explained by the model incorporating lime addition and occurrence of cannibalism as explaining variables (Table 1, AIC = 698.3). Based on these model parameters, female crickets that consumed their male in the first week of the experiment produced 177 eggs more than females that did not consume their male counterpart (Figures 3A,B and Table 1, intercept = 351 eggs; male eaten = 528 eggs, z = 3.12, p < 0.01). Liming significantly reduced the total number of hatched eggs by 107 eggs (Table 1 and Figures 3A,B, intercept = 351 eggs, Ca+ = 242, z = –3.1, p < 0.05). A model that also included P addition (P+ treatment) received less support (Table 1, AIC = 700.2, relative likelihood = 0.37), and the estimated effect of P addition in this model was minor and not significant, while the modeled effects of liming and cannibalism were highly comparable. Models excluding cannibalistic females show the similar results, with again the best performing model incorporating lime addition as explaining variable (Supplementary Table 4, AIC = 494.9), followed by a model also incorporating P addition (Supplementary Table 4, AIC = 496.9, relative likelihood = 0.37). Liming reduced total number of hatched eggs in non-cannibalistic females by 102 (Supplementary Table 5, intercept = 347, Ca+ = 245, z = –2.3, p < 0.05). P treatment only had a minor, non-significant effect on total hatched eggs in non-cannibalistic females (Supplementary Table 5).
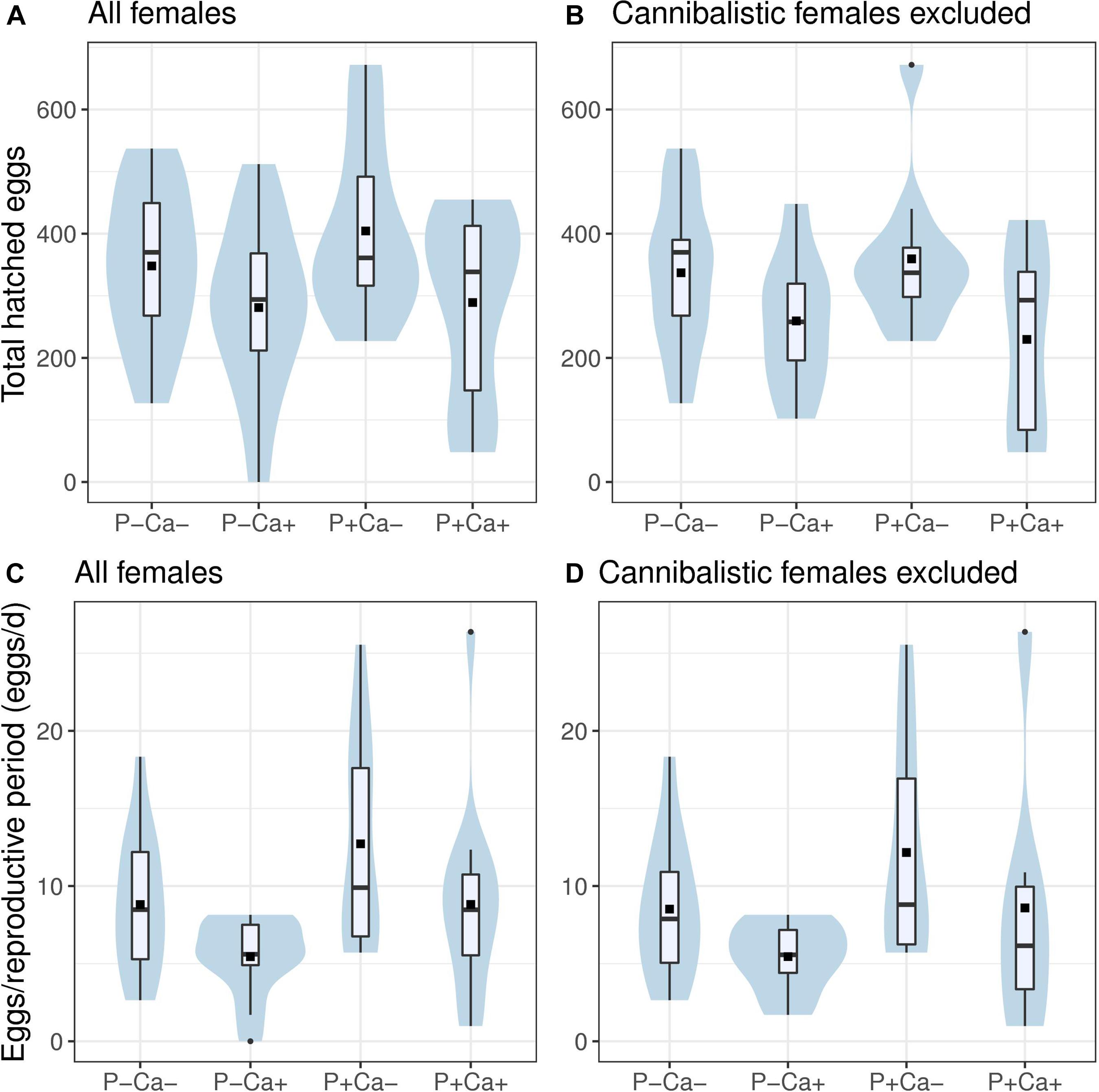
Figure 3. Box-violin plots of Gryllus campestris reproductive success of all individuals (A,C) and all individuals excluding cannibalistic females (B,D). (A,B) Total number of hatched eggs. (B,C) Female daily reproduction [hatched eggs/reproductive period (days)] for different food treatment types. Violins indicate data distribution. Boxes indicate 25, 50, and 75% quantiles, whiskers indicate all data within 1.5*IQR, 1.5 times IQR range, dots represent outliers, squares indicate group mean. P+/P–: P treatment contrasts; Ca+/Ca–: lime treatment contrasts. n = 55 for (A,C), n = 39 for (B,D).
Daily reproduction was best explained by a model incorporating both P and lime addition as explanatory variables (Table 1, AIC = 324.8). Daily reproductive success was significantly increased by P addition (P+: Table 1 and Figure 3C z = 2.62, p < 0.05) and decreased by lime addition (Ca+: Table 1 and Figure 3C, z = 2.61, p < 0.05) by about the same amount (3.3 eggs/day). A model that also included cannibalism received slightly less support (Table 1, AIC = 325.9, rl = 0.56). Cannibalism did not significantly influence daily reproductive success (compare Figures 3C,D), but improved the fit for lime addition as well as the estimated effect of this treatment.). Models excluding cannibalistic females show similar results with the best performing model incorporating P and lime addition as explaining variable (Supplementary Table 4, AIC = 237.4), followed by a model incorporating only P addition as explanatory variable (Supplementary Table 4, AIC = 239.1, relative likelihood = 0.42). Liming reduced daily reproduction in non-cannibalistic females by 3.2 eggs/day, while P addition increased daily reproduction by 3.4 eggs/day in both models (Supplementary Table 5). In these models, both treatment effects were only significant at the 0.1 threshold, probably due to the lower number of replicates and imbalanced grouping (i.e., more individuals in the Ca– groups).
Food Quality
As expected, P+ treatment significantly increased food P and significantly reduced food N:P ratio (Figure 4A and Table 2, +32.9 P, t = 9.22, p < 0.001, –21.9 N:P, i = –10.94, p < 0.001). This reduction in N:P ratio appeared to be less pronounced when lime was added, resulting in a significant interaction between P and lime addition treatments in N:P ratio (Figure 4A and Table 2, –19.4 N:P, t = 2.43, p < 0.05). Food N content was not significantly altered by either of the treatments (Table 2). Food N:P ratio was substantially higher than those reported for terrestrial invertebrates in the treatments without P addition (Elser et al., 2000a), whereas in treatments with P addition, plant tissue N:P closely matched this range (Figure 4A).
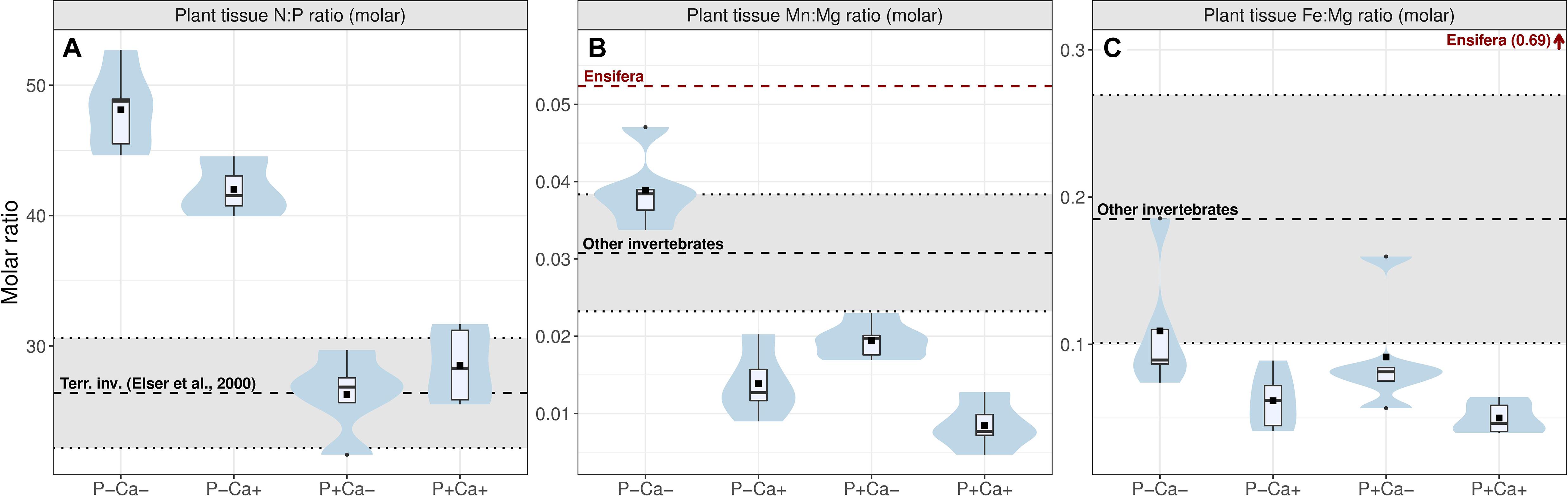
Figure 4. Box-violin plots of treatment effects on plant tissue elemental stoichiometry. (A) N:P molar ratio; (B) Mn:Mg molar ratio; (C) Fe:Mg molar ratio of the Calluna/Molinia food mixture in the experimental fields. Bars represent bootstrapped 95% CI intervals, with group means as horizontal cross-bar. Black dashed horizontal line in A indicate mean tissue N:P ratio of terrestrial invertebrates (Elser et al., 2000a), black dashed horizontal line in (B,C) indicate mean tissue Mn:Mg and Fe:Mg ratio of invertebrates, red dashed horizontal line in (B) indicate Ensifera Mn:Mg ratio. Ensifera Fe:Mg ratio is indicated by an arrow with respective ratio in parentheses (for invertebrate elemental ratios, see Supplementary Table 1). Violins indicate data distribution. Boxes indicate 25, 50, and 75% quantiles, whiskers indicate all data within 1.5*interquantile range, dots represent outliers, squares indicate group mean. P+/P–: P treatment contrasts; Ca+/Ca–: lime treatment contrasts.
Both treatments influenced plant tissue elemental content of the food (Supplementary Figure 2). As expected, food Ca and Mg increased in lime treatments. P addition also increased food Ca and Mg content. Mn decreased strongly in lime treatments and to a lesser extent also in P addition treatment, while for Fe this was also reduced in lime treatments, although this was not significant (Supplementary Figure 2 and Table 2). Together, changes in food Mn, Fe and Mg concentrations resulted in pronounced changes in food Mn:Mg ratio (Figure 4B) and Fe:Mg ratio (Figure 4C), with lime treatment having a stronger or consistent negative effect (Figure 4B and Table 2: –0.023 Mn:Mg:, t = –8.08, p < 0.001; –0.04 Fe:Mg, t = p < 0.01) compared to P treatment (Figure 4B and Table 2: –0.017 Mn:Mg, t = –4.96, p < 0.001, –0.02 Fe:Mg, t = –1.32, p = 0.20). As a result, food Mn:Mg and Fe:Mg ratio declined to levels substantially lower than those observed for Ensifera and other invertebrates (Figures 4B,C).
Comparison of Breeding Experiments
When comparing the results of our hatching experiment with results obtained in a previous feeding experiment, there appears to be a non-linear response in the number of hatched eggs to tissue N:P ratio of the plants fed to the crickets (Figure 5). At N:P molar ratio below 50, the number of hatched eggs varied around 300 for a large range of N:P ratios, but at ratios above 50 (i.e., the Strabrecht “heath” treatment), there was a substantial reduction in the mean number of hatched eggs (107.3). Furthermore, at the lowest treatment N:P ratio of 26.2 (current experiment; P+Ca– treatment), mean number of hatched eggs after 30 days was substantially higher (396.6) compared to treatments with intermediate food N:P ratios (282.2–333).
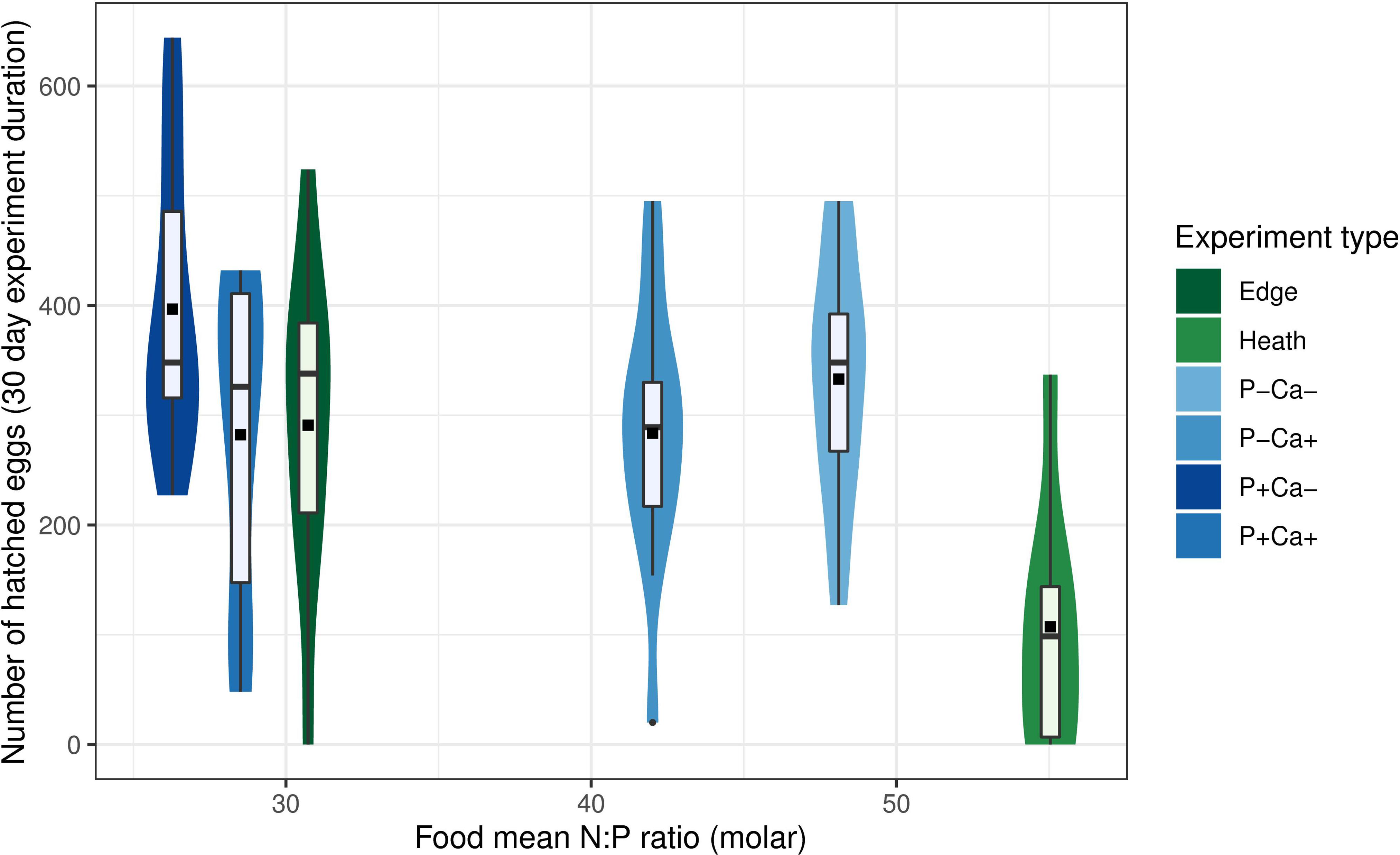
Figure 5. Comparison of reproductive potential of female Gryllus campestris with mean plant tissue N:P ratio under two feeding experiments. Blues: current experiment (by experimental treatment). Greens: experiment performed with plant material collected from Strabrechtse Heide heathland under contrasting nutrient availability (Vogels et al., 2013). Edge: edge between Calluna vulgaris dominated heathland and extensive agricultural field. Heath: Calluna vulgaris dominated dry heathland on mineral poor aeolian sand deposits. For comparability with the Strabrechtse Heide experiment, number of hatched eggs was taken at 30 days experimental duration in the current experiment. Violins indicate data distribution. Boxes indicate 25, 50, and 75% quantiles, whiskers indicate all data within 1.5*interquantile range, dots represent outliers, squares indicate group mean.
Discussion
P Amelioration Effects
P amelioration successfully achieved the aim of removing stoichiometric imbalances, with food N:P ratio decreasing to values that align with the mean ratio of terrestrial invertebrates. Daily reproductive success also improved when crickets were fed food from P addition plots, with female crickets being able to reach their maximal reproductive potential in a shorter timespan. However, higher daily reproduction did not translate in a higher total reproductive success. In allowing crickets to produce eggs until death, those who were fed higher (i.e., less favorable) N:P food were able to eventually catch up with low (i.e., more favorable) N:P food fed crickets, having access to more food over this protracted period. The faster rate of egg production in individuals fed with food from P addition plots may be linked to an increased potential for protein synthesis, as ribosomal RNA is the major pool of cellular P (Elser et al., 1996) and increased allocation to ribosomal RNA is a prerequisite for increased protein synthesis (Elser et al., 2000b). Earlier experiments did however also show a reduced total reproduction in high N:P fed crickets (Vogels et al., 2013). In these earlier experiments, a greater range in the N:P ratio of food tissue was employed with the ratio’s in the highest N:P treatment being very skewed. The smaller differences in food quality in the current experiment likely enabled crickets on high N:P food to catch up. Stoichiometric relationships with growth and fitness are not linear by nature, but rather show an optimum growth at a specific ratio (i.e., the threshold elemental ratio) and growth decreases at both supraoptimal and superoptimal values (Boersma and Elser, 2006). Food N:P ratios at the higher end of the spectrum therefore result in much greater fitness costs than those closer to the threshold elemental ratio of the specific organism.
Liming Effects
Contrary to our expectations, the liming treatment did not improve but rather resulted in a significant reduction in reproductive performance. We also observed increased cannibalistic behavior of female crickets fed with food from limed plots, suggesting that the lower reproductive success was related to a nutrient deficiency induced by liming. For total reproduction, a significant increase in eggs produced under cannibalism partly compensated for the decrease in eggs produced when fed food from limed plots, but even when considering only cannibalistic females (Supplementary Figure 3), there was a clear reduction in total reproduction with liming. Since cannibalism affected total reproduction, but not daily reproduction (for which P addition and subsequent N:P ratio was found to be important), our results suggest that cannibalism was induced due to a deficiency in other (trace) elements rather than a deficiency in CNP macronutrients.
Liming resulted in substantial shifts in food Mn:Mg and Fe:Mg ratios. The skewed ratios arose because plant tissue Mg increased, while Mn and Fe decreased, with Fe decreasing only in the combined P and lime addition treatment. Decreased plant tissue Mn as a result of liming has been reported earlier (Millaleo et al., 2010; Pabian et al., 2012) and could be related to increased root uptake competition by divalent Ca2+ and Mg2+ (Millaleo et al., 2010) or through a reduced bioavailability of Mn2+ resulting from an increased soil pH following liming (Tyler and Olsson, 2001). As a result, plant tissue Mn:Mg and Fe:Mg ratios in the liming treatment diverged strongly from those found in terrestrial invertebrates. Especially for the suborder Ensifera (to which the field cricket also belongs) this mismatch was most pronounced, making micronutrient imbalance a viable hypothesis to explain the observed effects.
Both Mn and Fe deficiencies can be linked to reduced insect performance. In invertebrates, uptake of Mn2+ and Fe2+ is partially regulated through the divalent metal transporter (DMT1) ortholog malvolio (Orgad et al., 1998; Au et al., 2008; Mandilaras et al., 2013). In the DMT1, Fe2+ uptake is inhibited by Ca2+ and Mg2+ (Shawki and Mackenzie, 2010), and inhibition of Mn2+ uptake by Ca2+ has been reported in insects as well (Poteat et al., 2012), suggesting that Ca2+ and Mg2+ inhibit the uptake of Mn2+ and Fe2+ in invertebrates in the same manner. Mn is an essential co-factor in many enzymes, including arginase, an essential enzyme in the urea cycle (Reddy and Campbell, 1969). Reduced activity of arginase due to Mn deficiency would result in reduced N excretion efficiency, ultimately inhibiting amino acid catabolism via build-up of toxic NH4+. Mn deficiency is however rare, and toxic over-exposure to Mn is reported more frequently than Mn deficiency (Ben-Shahar, 2018). Fe is an essential element as well as a strong toxin when freely available. Fe is therefore regulated by the Fe storage and transporting proteins ferritin and transferrin (Locke and Nichol, 1992; Nichol et al., 2002). Transferrin has been identified as the major source of Fe in insect eggs (Geiser and Winzerling, 2012). Mn or Fe deficiency could thus result in reduced egg production, either indirectly through reduced protein synthesis (following disruption of the urea cycle) or directly through reduced Fe transport to developing eggs. Future studies could test the exact nature and applicability of the mechanisms proposed here. Taken together, these results indicate that both Mn and Fe deficiencies can be induced by liming after sod cutting and that these can have cascading effects on reproductive output.
Cannibalism and Feeding Limitations
The occurrence of cannibalism in the first week of the experiment was an unexpected outcome of the experiment. In nature, cannibalism is rare (T. Tregenza, pers. comm.1), but when males and females were kept within a confined space, females were apparently able to predate on males. Although cannibalism complicated the analysis and interpretation of our results, the higher prevalence of cannibalism in the lime addition treatment provided us with an additional clue to the effect of liming on reproduction. Including cannibalism in our models improved the model fit and revealed a more negative effect of liming on reproduction (as cannibalism enabled females to partly compensate the loss in fecundity due to liming). The results are not confounded by cannibalism, since models excluding cannibalistic females showed the same outcome, albeit with a lower statistical power due to large reductions in the replicates for some treatments.
In our study female crickets were not allowed to actively select food of differing quality. This deprived the females the option to regulate nutrient intake. It is well established that insects are able to complement low intake of specific nutrients by selecting food sources rich in this nutrient. Such regulation in insects and crickets has been extensively reported by several authors for protein and carbohydrates (Waldbauer and Friedman, 1991; Raubenheimer and Simpson, 1993; Berner et al., 2005; Behmer, 2009; Harrison et al., 2014). For P and micronutrients however, there is little to no evidence for intake regulation in insects or crickets (Visanuvimol and Bertram, 2011; Harrison et al., 2014; but see Cease et al., 2016). Not being able to regulate nutrient intake by complementary feeding could explain the higher prevalence of cannibalism in females, suggesting that nutrient deficiencies were most pronounced in liming treatments. In our study design, we did allow for compensatory feeding by providing food pellets of a given quality ad libitum as insects are known compensate for low nutrient content by increasing ingestion rate (Berner et al., 2005). This may explain why total reproduction was not affected by P addition as females fed with lower quality feed were able to compensate by extending the reproductive period and in doing so were able to eat more food.
In the experimental plots used as food source for this experiment, P and lime addition treatments resulted in a significant increase in plant species richness compared to control (Vogels et al., 2020). These were mainly herbaceous and graminoid species typical for Nardus grasslands. In the control, however, species richness was low and vegetation was dominated by Calluna and Molinia. Under field conditions, field crickets will thus have more opportunities to regulate food intake by complementary feeding in the P and Lime treatments. At least for the P addition treatment, this should allow individuals to maximize nutrient intake efficiency. For limed treatments, this depends on the question whether food items rich in specific micronutrients (i.e., Mn and/or Fe rich food sources) are available for the crickets to select. Our result on cannibalism suggests that complementary feeding through a greater reliance on invertebrate food sources may enable the omnivorous field cricket to regulate nutrient intake.
Management Implications
Our results demonstrate that high-impact, large scale management practices such as sod cutting to reduce the effects of chronic increased nitrogen and acidifying deposition include a high risk of unwanted side effects for invertebrate populations. The nature of these unwanted side effects lies in disturbance, habitat homogenization and destruction and in increasing stoichiometric imbalances as shown in this and earlier studies (Härdtle et al., 2006; Härdtle et al., 2009; Vogels et al., 2017). Our study shows that P addition can partially resolve imbalances with respect to N:P ratios, and earlier studies also showed a beneficial effect of P amelioration treatment on floristic diversity and on soil mesofauna (Siepel et al., 2018; Vogels et al., 2020). P addition, however, also increases the risk of renewed grass encroachment under increased nitrogen deposition, and therefore cannot be implemented on a large scale under chronic increased N deposition. Liming has been repeatedly found to improve soil conditions and germination and settlement of characteristic plant species (De Graaf et al., 1998; Dorland et al., 2003, 2004; Kleijn et al., 2008), which has also been demonstrated earlier for the experimental plots in this study (Vogels et al., 2020). Contrary to positive reports of liming on deer (Pabian et al., 2012) and bird populations (Pabian and Brittingham, 2007), our finding of lower reproductive success of the field cricket agrees with previous studies reporting neutral to negative effects of liming on invertebrates (McKie et al., 2006; Korenko et al., 2008; Kappes and Topp, 2014; Michalko et al., 2018; Siepel et al., 2019). This discrepancy is likely caused by the much higher demands for Ca in vertebrates compared to most invertebrate orders (with woodlice, millipedes and snails as the exception). Our results suggest that liming can result in highly skewed elemental ratios which may constrain recovery of invertebrate heathland fauna. We therefore conclude that measures such as large scaled sod cutting to combat increased nitrogen deposition could ultimately result in minor gains accompanied by greater losses for at least the invertebrate diversity that is characteristic of dry heathlands, such as the field cricket. In order to restore desirable biogeochemical conditions, we advise management options such as grazing and regular mowing which do not result in highly skewed ratios for trace elements and macronutrients. Measurements aimed at restoring soil buffer capacity should ideally restore base cation concentrations more gradually and target a broader spectrum of base cations (also Mn and Fe). However, these lower disturbance management options will still constitute a degree of disturbance (e.g., Fottner et al., 2007), limiting the frequency at which such measures can be safely implemented (Maes et al., 2017). At the same time, the impact of these measures on N budgets and soil buffer capacity is lower than high disturbance management options (Härdtle et al., 2006), which means that these management measures have to be repeated more frequently to compensate for the continuous N and acidifying deposition. Taken together, it becomes clear that substantially reducing the annual deposition levels of N in many North Western European heathland regions to levels below the critical load of 10–20 kg⋅ha–1⋅yr–1 is the only option for conservation on the long term (Bobbink and Hettelingh, 2011). We therefore urge policy makers to develop a sound N-emission reduction strategy, since focussing on a N removal strategy will not be sufficient to preserve the biodiversity of oligotrophic nature reserves.
Data Availability Statement
The raw data supporting the conclusions of this article has been made publicly available via the DANS easy repository: https://doi.org/10.17026/dans-zsa-f3y9.
Author Contributions
JV and HS conceived the ideas and designed methodology. JV, MW, and JK collected the data. JV and JK performed the breeding experiment. JV and WV analyzed the data and drafted the manuscript. All authors contributed to the drafts and gave final approval for publication.
Funding
This research was funded by the O+BN program, financed by the Dutch Ministry of Agriculture, Nature and Food Quality and BIJ12 (Grant No. OBN207-DZ).
Conflict of Interest
The authors declare that the research was conducted in the absence of any commercial or financial relationships that could be construed as a potential conflict of interest.
Acknowledgments
We thank Evi Verbaarschot for assistance with field work, Jan-Willem Wolters for laboratory analyses of invertebrate elemental content and Jakob Leidekker from the National Park De Hoge Veluwe for providing access to the park and allowing us to perform experimental research.
Supplementary Material
The Supplementary Material for this article can be found online at: https://www.frontiersin.org/articles/10.3389/fevo.2021.659363/full#supplementary-material
Footnotes
References
Au, C., Benedetto, A., and Aschner, M. (2008). Manganese transport in eukaryotes: the role of DMT1. NeuroToxicology 29, 569–576. doi: 10.1016/j.neuro.2008.04.022
Behmer, S. T. (2009). Insect herbivore nutrient regulation. Annu. Rev. Entomol. 54, 165–187. doi: 10.1146/annurev.ento.54.110807.090537
Ben-Shahar, Y. (2018). The impact of environmental Mn exposure on insect biology. Front. Genet. 9:70. doi: 10.3389/fgene.2018.00070
Berner, D., Blanckenhorn, W. U., and Korner, C. (2005). Grasshoppers cope with low host plant quality by compensatory feeding and food selection: N limitation challenged. Oikos 111, 525–533.
Bobbink, R., and Heil, G. W. (1993). “Atmospheric deposition of sulphur and nitrogen in heathland ecosystems,” in Heathlands: Patterns and Processes in a Changing Environment, eds R. Aerts and G. W. Heil (Dordrecht: Kluwer Academic Publishers), 25–50.
Bobbink, R., and Hettelingh, J. P. (2011). “Review and revision of empirical critical loads and dose-response relationships,” in Proceedings of an Expert Workshop, Noordwijkerhout, 23-25 June 2010, (Bilthoven: Coordination Centre for Effects).
Bobbink, R., Hicks, K., Galloway, J., Spranger, T., Alkemade, R., Ashmore, M., et al. (2010). Global assessment of nitrogen deposition effects on terrestrial plant diversity: a synthesis. Ecol. Appl. 20, 30–59. doi: 10.1890/08-1140.1
Bobbink, R., Hornung, M., and Roelofs, J. G. M. (1998). The effects of air-borne nitrogen pollutants on species diversity in natural and semi-natural European vegetation. J. Ecol. 86, 717–738.
Boersma, M., and Elser, J. J. (2006). Too much of a good thing: on stoichiometrically balanced diets and maximal growth. Ecology 87, 1325–1330.
Borer, E. T., Lind, E. M., Firn, J., Seabloom, E. W., Anderson, T. M., Bakker, E. S., et al. (2019). More salt, please: global patterns, responses and impacts of foliar sodium in grasslands. Ecol. Lett. 22, 1136–1144. doi: 10.1111/ele.13270
Burnham, K. P., and Anderson, D. R. (2002). Model Selection and Multimodel Inference : A Practical Information-Theoretic Approach. New York, NY: Springer.
Cease, A. J., Fay, M., Elser, J. J., and Harrison, J. F. (2016). Dietary phosphate affects food selection, post-ingestive phosphorus fate, and performance of a polyphagous herbivore. J. Exp. Biol. 219, 64–72. doi: 10.1242/jeb.126847
De Graaf, M. C. C., Bobbink, R., Smits, N. A. C., Van Diggelen, R., and Roelofs, J. G. M. (2009). Biodiversity, vegetation gradients and key biogeochemical processes in the heathland landscape. Biol. Conserv. 142, 2191–2201.
De Graaf, M. C. C., Verbeek, P. J. M., Bobbink, R., and Roelofs, J. G. M. (1998). Restoration of species-rich dry heaths: the importance of appropriate soil conditions. Acta Bot. Neerl. 47, 89–111.
Diemont, W. H. (1996). Survival of Dutch Heathlands. “IBN Scientific Contributions 1.”. Wageningen: DLO Institute for Forestry and Nature Research (IBN-DLO).
Dorland, E., Bobbink, R., Messelink, J. H., and Verhoeven, J. T. A. (2003). Soil ammonium accumulation after sod cutting hampers the restoration of degraded wet heathlands. J. Appl. Ecol. 40, 804–814. doi: 10.1046/j.1365-2664.2003.00845.x
Dorland, E., van den Berg, L. J. L., van de Berg, A. J., Vermeer, M. L., Roelofs, J. G. M., and Bobbink, R. (2004). The effects of sod cutting and additional liming on potential net nitrification in heathland soils. Plant Soil 265, 267–277.
Elser, J. J., Dobberfuhl, D. R., MacKay, N. A., and Schampel, J. H. (1996). Organism size, life history, and N:P Stoichiometry: toward a unified view of cellular and ecosystem processes. BioScience 46, 674–684. doi: 10.2307/1312897
Elser, J. J., Fagan, W. F., Denno, R. F., Dobberfuhl, D. R., Folarin, A., Huberty, A., et al. (2000a). Nutritional constraints in terrestrial and freshwater food webs. Nature 408, 578–580.
Elser, J. J., Sterner, R. W., Gorokhova, E., Fagan, W. F., Markow, T. A., Cotner, J. B., et al. (2000b). Biological stoichiometry from genes to ecosystems. Ecol. Lett. 3, 540–550. doi: 10.1046/j.1461-0248.2000.00185.x
Filipiak, M., Kuszewska, K., Asselman, M., Denisow, B., Stawiarz, E., Woyciechowski, M., et al. (2017). Ecological stoichiometry of the honeybee: pollen diversity and adequate species composition are needed to mitigate limitations imposed on the growth and development of bees by pollen quality. PLoS One 12:e0183236. doi: 10.1371/journal.pone.0183236
Fottner, S., Hardtle, W., Niemeyer, M., Niemeyer, T., von Oheimb, G., Meyer, H., et al. (2007). Impact of sheep grazing on nutrient budgets of dry heathlands. Appl. Veg. Sci. 10, 391–398. doi: 10.1111/j.1654-109X.2007.tb00438.x
Geiser, D. L., and Winzerling, J. J. (2012). Insect transferrins: multifunctional proteins. Biochim. Biophys. Acta 1820, 437–451. doi: 10.1016/j.bbagen.2011.07.011
Gjengedal, E., Martinsen, T., and Steinnes, E. (2015). Background levels of some major, trace, and rare earth elements in indigenous plant species growing in Norway and the influence of soil acidification, soil parent material, and seasonal variation on these levels. Environ. Monit. Assess. 187:386. doi: 10.1007/s10661-015-4479-5
Graveland, J. (1998). Effects of acid rain on bird populations. Environ. Rev. 6, 41–54. doi: 10.1139/a98-003
Graveland, J., and vanderWal, R. (1996). Decline in snail abundance due to soil acidification causes eggshell defects in forest passerines. Oecologia 105, 351–360. doi: 10.1007/bf00328738
Graveland, J., Vanderwal, R., Vanbalen, J. H., and Vannoordwijk, A. J. (1994). Poor reproduction in forest passerines from decline of snail abundance on acidified soils. Nature 368, 446–448. doi: 10.1038/368446a0
Haddad, N. M., Haarstad, J., and Tilman, D. (2000). The effects of long-term nitrogen loading on grassland insect communities. Oecologia 124, 73–84. doi: 10.1007/s004420050026
Härdtle, W., Niemeyer, M., Niemeyer, T., Assmann, T., and Fottner, S. (2006). Can management compensate for atmospheric nutrient deposition in heathland ecosystems? J. Appl. Ecol. 43, 759–769. doi: 10.1111/j.1365-2664.2006.01195.x
Härdtle, W., Von Oheimb, G., Gerke, A. K., Niemeyer, M., Niemeyer, T., Assmann, T., et al. (2009). Shifts in N and P budgets of heathland ecosystems: effects of management and atmospheric inputs. Ecosystems 12, 298–310. doi: 10.1007/s10021-008-9223-3
Harrison, S. J., Raubenheimer, D., Simpson, S. J., Godin, J. G. J., and Bertram, S. M. (2014). Towards a synthesis of frameworks in nutritional ecology: interacting effects of protein, carbohydrate and phosphorus on field cricket fitness. Proc. R. Soc. B Biol. Sci. 281:20140539. doi: 10.1098/rspb.2014.0539
Haynes, R. (1982). Effects of liming on phosphate availability in acid soils. Plant Soil 68, 289–308. doi: 10.1007/bf02197935
Ingrisch, S., and Köhler, G. (1998). Die Heuschrecken Mitteleuropas. Magdeburg: Westarp Wissenschaften.
Joern, A., Provin, T., and Behmer, S. T. (2011). Not just the usual suspects: insect herbivore populations and communities are associated with multiple plant nutrients. Ecology 93, 1002–1015. doi: 10.1890/11-1142.1
Jones, L., Stevens, C., Rowe, E. C., Payne, R., Caporn, S. J. M., Evans, C. D., et al. (2017). Can on-site management mitigate nitrogen deposition impacts in non-wooded habitats? Biol. Conserv. 212, 464–475. doi: 10.1016/j.biocon.2016.06.012
Kappes, H., and Topp, W. (2014). Responses of forest snail assemblages to soil acidity buffer system and liming. Ecol. Res. 29, 757–766. doi: 10.1007/s11284-014-1167-9
Kleijn, D., Bekker, R. M., Bobbink, R., De Graaf, M. C. C., and Roelofs, J. G. M. (2008). In search for key biogeochemical factors affecting plant species persistence in heathland and acidic grasslands: a comparison of common and rare species. J. Appl. Ecol. 45, 680–687. doi: 10.1111/j.1365-2664.2007.01444.x
Kleukers, R. M. J. C., van Nieukerken, E. J., Odé, B., Willemse, L. P. M., van Wingerden, W. K. R. E., van Nieukerken, E. J., et al. (1997). De Sprinkhanen en Krekels van Nederland (Orthoptera). Utrecht: KNNV.
Korenko, S., Kula, E., Holec, M., Jarab, M., and Michalkova, V. (2008). Influence of liming on the epigeic spider (Araneae) community of the Krusne hory Mts (Czech Republic). Eur. J. Soil Biol. 44, 559–566. doi: 10.1016/j.ejsobi.2008.08.001
Locke, M., and Nichol, H. (1992). Iron economy in insects - transport, metabolism, and storage. Annu. Rev. Entomol. 37, 195–215.
Maes, D., Decleer, K., De Keersmaeker, L., Van Uytvanck, J., and Louette, G. (2017). Intensified habitat management to mitigate negative effects of nitrogen pollution can be detrimental for faunal diversity: a comment on Jones et al. (2017). Biol. Conserv. 212, 493–494. doi: 10.1016/j.biocon.2017.03.001
Mandilaras, K., Pathmanathan, T., and Missirlis, F. (2013). Iron absorption in Drosophila melanogaster. Nutrients 5, 1622–1647. doi: 10.3390/nu5051622
McKie, B. G., Petrin, Z., and Malmqvist, B. (2006). Mitigation or disturbance? Effects of liming on macroinvertebrate assemblage structure and leaf-litter decomposition in the humic streams of northern Sweden. J. Appl. Ecol. 43, 780–791. doi: 10.1111/j.1365-2664.2006.01196.x
Michalko, R., Kula, E., and Kosulic, O. (2018). Liming alters body size distribution in a community of epigeic spiders in birch forest (Betula pendula Roth). Ann. For. Sci. 75:92. doi: 10.1007/s13595-018-0769-8
Millaleo, R., Reyes- Diaz, M., Ivanov, A. G., Mora, M. L., and Alberdi, M. (2010). Manganese as essential and toxic element for plants: transport, accumulation and resistance mechanisms. J. Soil Sci. Plant Nutr. 10, 470–481.
Nichol, H., Law, J. H., and Winzerling, J. J. (2002). Iron metabolism in insects. Annu. Rev. Entomol. 47, 535–559. doi: 10.1146/annurev.ento.47.091201.145237
Nijssen, M. E., WallisDeVries, M. F., and Siepel, H. (2017). Pathways for the effects of increased nitrogen deposition on fauna. Biol. Conserv. 212, 423–431. doi: 10.1016/j.biocon.2017.02.022
Orgad, S., Nelson, H., Segal, D., and Nelson, N. (1998). Metal ions suppress the abnormal taste behavior of the Drosophila mutant malvolio. J. Exp. Biol. 201(Pt. 1), 115–120.
Pabian, S. E., and Brittingham, M. C. (2007). Terrestrial liming benefits birds in an acidified forest in the northeast. Ecol. Appl. 17, 2184–2194. doi: 10.1890/07-0394.1
Pabian, S. E., Ermer, N. M., Tzilkowski, W. M., and Brittingham, M. C. (2012). Effects of liming on forage availability and nutrient content in a forest impacted by acid rain. PLoS One 7:e39755. doi: 10.1371/journal.pone.0039755
Penuelas, J., Janssens, I. A., Ciais, P., Obersteiner, M., and Sardans, J. (2020). Anthropogenic global shifts in biospheric N and P concentrations and ratios and their impacts on biodiversity, ecosystem productivity, food security, and human health. Glob. Change Biol. 26, 1962–1985. doi: 10.1111/gcb.14981
Poteat, M. D., Díaz-Jaramillo, M., and Buchwalter, D. B. (2012). Divalent metal (Ca, Cd, Mn, Zn) uptake and interactions in the aquatic insect Hydropsyche sparna. J. Exp. Biol. 215, 1575–1583. doi: 10.1242/jeb.063412
Raubenheimer, D., and Simpson, S. J. (1993). The geometry of compensatory feeding in the locust.. Behav. 45, 953–964. doi: 10.1006/anbe.1993.1114
Reddy, S. R. R., and Campbell, J. W. (1969). Arginine metabolism in insects: properties of insect fat body arginase. Comp. Biochem. Physiol. 28, 515–534. doi: 10.1016/0010-406X(69)92087-8
Roem, W. J., and Berendse, F. (2000). Soil acidity and nutrient supply ratio as possible factors determining changes in plant species diversity in grassland and heathland communities. Biol. Conserv. 92, 151–161. doi: 10.1016/s0006-3207(99)00049-x
Roem, W. J., Klees, H., and Berendse, F. (2002). Effects of nutrient addition and acidification on plant species diversity and seed germination in heathland. J. Appl. Ecol. 39, 937–948.
Shawki, A., and Mackenzie, B. (2010). Interaction of calcium with the human divalent metal-ion transporter-1. Biochem. Biophys. Res. Commun. 393, 471–475. doi: 10.1016/j.bbrc.2010.02.025
Siepel, H., Bobbink, R., van de Riet, B. P., van den Burg, A. B., and Jongejans, E. (2019). Long-term effects of liming on soil physico-chemical properties and micro-arthropod communities in Scotch pine forest. Biol. Fertil. Soils 55, 675–683. doi: 10.1007/s00374-019-01378-3
Siepel, H., Vogels, J., Bobbink, R., Bijlsma, R.-J., Jongejans, E., de Waal, R., et al. (2018). Continuous and cumulative acidification and N deposition induce P limitation of the micro-arthropod soil fauna of mineral-poor dry heathlands. Soil Biol. Biochem. 119, 128–134. doi: 10.1016/j.soilbio.2018.01.025
Sterner, R. W., and Elser, J. J. (2002). Ecological Stoichiometry: The Biology of Elements from Molecules to the Biosphere. New Jersey, NJ: Princeton University Press.
Stevens, C. J., Dise, N. B., and Gowing, D. J. (2009). Regional trends in soil acidification and exchangeable metal concentrations in relation to acid deposition rates. Environ. Pollut. 157, 313–319. doi: 10.1016/j.envpol.2008.06.033
Stevens, C. J., Duprè, C., Dorland, E., Gaudnik, C., Gowing, D. J. G., Bleeker, A., et al. (2010). Nitrogen deposition threatens species richness of grasslands across Europe. Environ. Pollut. 158, 2940–2945. doi: 10.1016/j.envpol.2010.06.006
Tian, D., and Niu, S. (2015). A global analysis of soil acidification caused by nitrogen addition. Environ. Res. Lett. 10:024019. doi: 10.1088/1748-9326/10/2/024019
Tyler, G., and Olsson, T. (2001). Plant uptake of major and minor mineral elements as influenced by soil acidity and liming. Plant Soil 230, 307–321. doi: 10.1023/A:1010314400976
Visanuvimol, L., and Bertram, S. M. (2011). How dietary phosphorus availability during development influences condition and life history traits of the cricket, Acheta domesticus. J. Insect Sci. 11:63.
Vogels, J. J., Verberk, W. C. E. P., Lamers, L. P. M., and Siepel, H. (2017). Can changes in soil biochemistry and plant stoichiometry explain loss of animal diversity of heathlands? Biol. Conserv. 212, 432–447. doi: 10.1016/j.biocon.2016.08.039
Vogels, J. J., Webb, N. R., and Siepel, H. H. (2013). “Impact of changed plant stoichiometric quality on heathland fauna composition,” in Economy and Ecology of Heathlands, eds W. H. Diemont, W. J. M. Heijman, H. H. Siepel, and N. R. Webb (Zeist: KNNV publishing).
Vogels, J. J., Weijters, M. J., Bobbink, R., Bijlsma, R.-J., Lamers, L. P. M., Verberk, W. C. E. P., et al. (2020). Barriers to restoration: soil acidity and phosphorus limitation constrain recovery of heathland plant communities after sod cutting. Appl. Veg. Sci. 23, 94–106. doi: 10.1111/avsc.12471
Waldbauer, G. P., and Friedman, S. (1991). Self-selection of optimal diets by insects. Annu. Rev. Entomol. 36, 43–63.
Keywords: acidification, restoration management, nitrogen deposition, elemental ecology, invertebrate diversity, insect decline, sod-cutting, ecological stoichiometry
Citation: Vogels JJ, Verberk WCEP, Kuper JT, Weijters MJ, Bobbink R and Siepel H (2021) How to Restore Invertebrate Diversity of Degraded Heathlands? A Case Study on the Reproductive Performance of the Field Cricket Gryllus campestris (L.). Front. Ecol. Evol. 9:659363. doi: 10.3389/fevo.2021.659363
Received: 27 January 2021; Accepted: 22 April 2021;
Published: 19 May 2021.
Edited by:
Anita Diaz, Bournemouth University, United KingdomReviewed by:
Tom Tregenza, University of Exeter, United KingdomPiotr Sewerniak, Nicolaus Copernicus University in Toruń, Poland
Copyright © 2021 Vogels, Verberk, Kuper, Weijters, Bobbink and Siepel. This is an open-access article distributed under the terms of the Creative Commons Attribution License (CC BY). The use, distribution or reproduction in other forums is permitted, provided the original author(s) and the copyright owner(s) are credited and that the original publication in this journal is cited, in accordance with accepted academic practice. No use, distribution or reproduction is permitted which does not comply with these terms.
*Correspondence: Joost J. Vogels, j.vogels@science.ru.nl