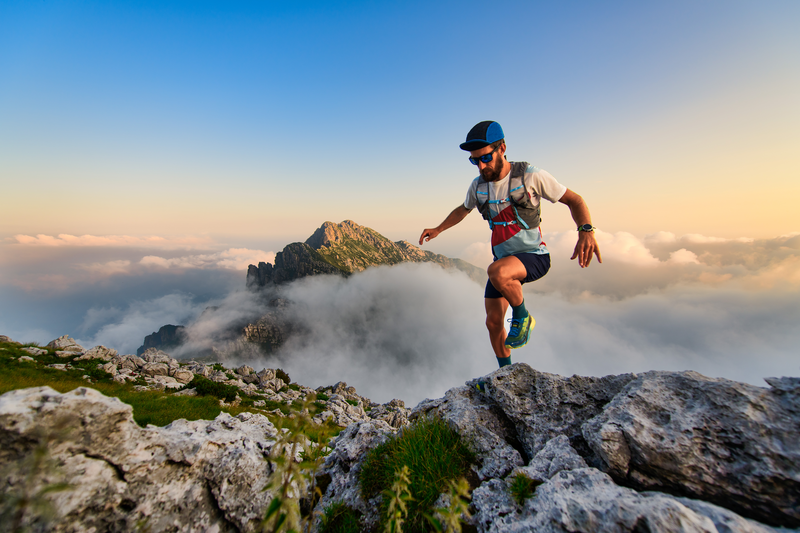
94% of researchers rate our articles as excellent or good
Learn more about the work of our research integrity team to safeguard the quality of each article we publish.
Find out more
BRIEF RESEARCH REPORT article
Front. Ecol. Evol. , 07 June 2021
Sec. Conservation and Restoration Ecology
Volume 9 - 2021 | https://doi.org/10.3389/fevo.2021.658390
This article is part of the Research Topic Modern Conservation: Critical Lessons from Birds of Prey View all 9 articles
Global climate change has advanced the breeding phenology of many avian species. However, raptors’ breeding phenologies may not respond in the same way to the factors that influence passerine breeding dates. We studied reproduction of suburban and rural Red-shouldered Hawks (Buteo lineatus) in southern Ohio, United States, from 1997 to 2020. Mean hatching dates for 786 broods were 24 April [Julian day: 114.1 ± 0.3 d (SE)] for suburban birds and 25 April (Julian day: 114.5 ± 0.4) for rural birds. Egg-laying date averages approximately 33 days before hatching date, or about the third week of March. We used mixed models to test which factors influenced nestling hatching dates from 1997 to 2020. The best model included year, days of snow cover during the pre-laying period (February–March), and mean March temperature, with days of snow cover having the largest effect. Hatching date (in Julian days) was positively related to snow cover and negatively related to air temperature, i.e., young hatched earlier in years with fewer days of snow cover and in warmer years). Young also hatched slightly later as the study progressed. Overall, neither mean hatching date nor any of the weather variables showed a significant trend over the course of the study. Previously published reports indicate that many raptor species do not exhibit advancing hatching dates, and breeding phenologies often reflect local weather conditions. The complexity and diversity of raptor responses to climate change underscore the importance of long-term studies of raptors at multiple locations.
Global climate change has advanced the breeding phenology of many avian species (Root et al., 2003; Thackeray et al., 2010), with subsequent effects on productivity, breeding success, and survival (Dunn and Møller, 2019). Earlier egg-laying by passerines is sometimes explained by the necessity to synchronize hatching of young with the peak abundance of insects, many of which have also advanced their phenology (Both et al., 2009; Thackeray et al., 2010). Failure to adjust breeding dates or insufficient adjustment can result in temporal mismatch of the insect prey and their passerine consumers, and can lead to decreased reproductive success for the passerines (Visser et al., 1998; Dunn, 2019).
Raptors’ breeding phenologies may not respond in the same way to the factors that influence passerine breeding dates (Bretagnolle and Terraube, 2019). Smaller birds such as passerines (and smaller raptors) may be more sensitive to temperature, more able to adjust their physiology, and more likely to exhibit advanced laying dates (Stevenson and Bryant, 2000; Lehikoinen et al., 2011; Bretagnolle and Terraube, 2019). In addition, the amount of time from egg-laying to the period of greatest energy demands of the young is generally longer for larger species; because of this long time period, larger species may be less able to time their breeding to match peak abundance of their prey (Both et al., 2009). Finally, compared to passerines feeding on insects, raptors are typically less dependent on a single prey species or type. Even for raptors that feed exclusively on birds and specialize on juveniles, such as the Eurasian Sparrowhawk (Accipiter nisus), a range of passerine species hatching throughout the spring provides an extended period of suitable prey (Both et al., 2009). Dietary generalist raptors are even less dependent on a particular type of prey, and may be less likely than specialist species to adjust their breeding phenologies (Bretagnolle and Terraube, 2019). In comparison to passerine studies, analyses of raptor breeding phenologies are relatively uncommon; yet shifts in raptor phenologies could have important impacts for the conservation of the individual species and trophic webs (Both et al., 2009; Lehikoinen et al., 2009; Heath et al., 2012).
Investigation of breeding phenology requires a long-term dataset, such as exists for Red-shouldered Hawks (Buteo lineatus) nesting in southern Ohio (Dykstra et al., 2019, 2021). A dietary generalist, the Red-shouldered Hawk inhabits forested areas (e.g., Titus and Mosher, 1981; Bosakowski et al., 1992; Bosakowski and Smith, 1997) and urban/suburban landscapes (Bloom and McCrary, 1996; Dykstra et al., 2000, 2018, 2021) in the eastern half of North America, with a separate subspecies in the far west (Dykstra et al., 2020). In southern Ohio, where, the species is resident year round and has been studied since 1997, birds nesting in urban/suburban areas have reproductive rates equal to those in a nearby natural forested region (Dykstra et al., 2009, 2021). Weather influences reproductive output in both populations: warmer mean temperatures in May (the nestling-rearing period) are associated with higher productivity (young per nesting attempt) and greater nesting success (Dykstra et al., 2021).
The consistent, long-term reproductive data available for these populations allow investigation of whether breeding phenology has advanced over the study period. However, weather conditions during the pre-laying period can also influence breeding phenology of raptors (e.g., Newton, 1986; Lehikoinen et al., 2010, 2013; Kreiderits et al., 2016). The effects of weather variables on breeding phenology are typically explained by indirect effects on prey availability: higher prey availability results in better physiological condition of the female, which leads to an earlier laying date (Newton, 1986; Steenhof et al., 1997; Porro et al., 2020; Bangerter et al., 2021). The specific weather conditions that influence prey availability vary depending on ecoregion, but in temperate or more-northern regions warmer winter or spring weather is generally associated with greater prey abundance and earlier laying dates (Lehikoinen et al., 2013; Bangerter et al., 2021). Conversely, snow cover reduces prey availability, especially for raptors that feed on voles (Microtus spp. and others), which are active out of sight under the snow (Naughton, 2012); greater snow cover has been correlated with delayed breeding by several species of owls (Lehikoinen et al., 2011; Solenen, 2014; Kouba et al., 2020).
We aimed to assess whether breeding phenology of Red-shouldered Hawks in southern Ohio has advanced over the 24-year study period, and also whether weather conditions in the pre-laying period influence breeding dates. We predicted that breeding phenology would have advanced slightly in response to global climate change, warmer air temperatures would be associated with earlier breeding, and greater snow cover would be associated with later breeding.
We studied Red-shouldered Hawks in two study areas in southern Ohio. Our suburban study area included parts of Hamilton, Clermont, Butler, and Warren Counties in southwestern Ohio (SWOH hereafter), primarily suburban areas surrounding the city of Cincinnati, Ohio. Our rural study site included parts of Hocking, Athens, Perry, and Vinton Counties in the area known as the Hocking Hills (HH) region in southeastern Ohio. In SWOH, suburban development varied from densely populated (residential lots approximately 20 m × 35 m) to sparsely populated (>2.5-ha residential lots and undeveloped private land; Dykstra et al., 2000, 2009). Native forest habitat in the SWOH study area includes second-growth oak-hickory (Quercus spp. and Carya spp.) and beech-maple (Fagus grandifolia and Acer saccharum) associations, with sycamores (Plantanus occidentalis) and beech along riparian areas. Most nests in SWOH were located on private land (Dykstra et al., 2003, 2009).
The rural HH study area, heavily forested with sparse human population, included Wayne National Forest, Hocking State Forest, Zaleski State Forest, and associated private lands. Oak-hickory forest predominates, but plantations of white pine (Pinus strobus) and red pine (P. resinosa) are also common. Sycamores, silver maple (Acer saccharinum), beech, and river birch (Betula nigra) are characteristic of lowland riparian areas. Most nests in HH were located on public lands. Nests were found by various techniques that have already been described (Dykstra et al., 2009, 2019). Once a nest was found, we generally revisited the nesting territory in subsequent years to look for a nest. In a few cases, a second clutch of eggs was laid in the same territory in the same year, presumably by the same adults, after the loss of the first clutch; known instances of second clutches were not included in our analyses. It is possible that a few broods resulting from second clutches were included, if we did not detect the loss of the initial clutch, but we believe the incidence of this to be small.
We accessed nests using standard climbing techniques (Pagel and Thorstrom, 2007) when the nestlings were approximately 2–5 weeks old, between May and early July each year from 1997 to 2020 in SWOH and from 1997 to 2016 in HH. We banded the young with US Geological Survey (USGS) bands and colored plastic bands (Haggie Engraving Company, MD, United States) inscribed with individual alpha-numeric codes (Dykstra et al., 2019). We weighed and measured all nestlings (1999–2020) or just the oldest nestling in the brood based on visual assessment of feather development (1997–1998). We measured nestling toepad length and length of the first and second secondaries. Based on our earlier work (Penak et al., 2013), we estimated nestling age (in days) as a function of mean secondary length (MSL, in mm) as:
where 2.3 days was added to account for the mean differences between populations (see Penak et al., 2013). Hatching date (Julian day) of the oldest nestling was back-calculated from age at banding.
Based on prior studies (Huchler et al., 2020), we considered that the weather in the period 3.5–5 weeks prior to egg-laying was most likely to influence laying date (and thus, hatching date, as the length of the incubation period likely did not vary greatly). Because Red-shouldered Hawk courtship in our study area generally begins in February, with egg-laying in mid- to late March, we considered that the weather conditions in March and February were the most likely to be biologically meaningful. In addition to air temperature, we considered snow cover potentially important, as it reduces availability of mammalian prey (Lehikoinen et al., 2011). We obtained weather data from NOAA’s National Centers for Environmental Information, Center for Weather and Climate1. We used data from the weather station at Cincinnati Northern Kentucky International Airport, Covington, Kentucky (39.0444o N, 84.6724o W), for our suburban SWOH study area. For our rural Hocking Hills study area, we primarily used data from the station at Ohio University, Athens, Ohio (39.3224o N, 82.0995o W), supplemented by minimal data from three nearby stations when Athens Ohio University data were unavailable. Weather data were assigned to nests in the respective study areas by year, such that all nests within a year in one study area were associated with the same weather data.
We also obtained North Atlantic Oscillation data from the NOAA website2. We obtained growing degree days data from the CFAES Weather System, Ohio State University3, from a single station that was centrally located between the two study sites (Jackson, Ohio: 39.05194o N, 82.63667o W).
We evaluated several correlated weather-related variables including North Atlantic Oscillation in February and March, the accumulated growing-degree-days (1 January to 31 March), mean February air temperature, mean March air temperature, and mean February–March air temperature, and we selected air temperature as the most intuitive, simple variable. We obtained data for total monthly snowfall and the number of days with snow cover, but these were correlated (r > 0.7) and we considered that the number of days of snow cover would be the better indicator of the potential effects of snow, so we included only days of snow cover in our models. Because we did not know which month’s weather was most important, we ran models with various combinations of the weather variables in February, March, and both months together; however, we did not use the separate months in the same model with the 2-month mean or total due to correlations (see Table 1 for variable definitions).
Our analysis included 786 broods at 278 nesting territories (582 broods at 203 territories in SWOH and 204 broods at 75 territories in HH). We calculated annual mean hatching date for each year in each study area and compared them using a Wilcoxon signed-rank test, and we tested for a temporal trend in annual mean hatching date and annual means of weather variables using simple linear regressions.
We used generalized linear mixed effects models with logit-link function and Poisson distribution to test for effects of weather variables and year on nestling hatching date. We included study area in the models as a fixed effect, and all models included nesting territory as a random effect to account for non-independence of multiple years of data from the same nesting territory. We standardized continuous variables (year, air temperature, snow cover, and snowfall) with a mean of 0 and SD of 1. We then constructed several candidate models (Table 2). Models were estimated using maximum likelihood, with selection based on Akaike information criterion corrected for small sample size (AICc). After model selection, we refit the top model using restricted maximum likelihood (REML) to obtain estimates of effects. Because variables were standardized, the reported parameter estimates reflect effect size.
Table 2. AICc model selection rankings for hatching date (response variable) of Red-shouldered Hawks, in relation to year, temperature, snow cover, and study area.
All analyses were conducted in Systat version 13 (Systat Software, Inc., San Jose, California, United States). Results are presented as mean ± SE, and we considered P < 0.05 to indicate a significant effect.
We determined hatching date for the oldest nestling in each of 786 broods. Average hatching date for all broods over the course of the study was 24 April (Julian day: 114.3 ± 0.3, median date = 113.9, range = 94–153, n = 786 broods). Mean hatching dates were 24 April (Julian day: 114.1 ± 0.3) for suburban birds in southwest Ohio and 25 April (Julian day: 114.5 ± 0.4) for rural birds in Hocking Hills. Annual mean hatching date did not differ between the two areas [Wilcoxon signed rank; Z = –0.26, P = 0.79, n = 20 years (1997–2016)].
The best model included year, the number of days of snow cover during the pre-laying period (February–March), and mean air temperature in March (Tables 2, 3), with nesting territory as a random effect. However, the next two models were also supported (ΔAICc < 2); these models included the same three variables as the top model, with the addition of mean February temperature (second-best model) or study area (third-best model), though neither of these variables were significant. In the best-supported model, the nestling hatching date (in Julian days) was positively related to the number of days of snow cover in February–March, i.e., young hatched earlier in years with fewer days of snow cover (β = 2.08 ± 0.24, t = 8.82, P < 0.001; Figure 1A), and negatively related to the mean March temperature, i.e., young hatched earlier in warmer years (β = –0.75 ± 0.24, t = –3.12, P = 0.001; Figure 1B). Study year positively influenced the hatching date, such that young hatched later as the study progressed (β = 1.22 ± 0.26, t = 4.65, P < 0.001; Table 3). Based on the parameter estimates, snow cover had the largest effect on hatching date.
Table 3. Fixed effects estimated (method: REML) for the best model based on AICc: Hatchdate ∼ year + TempMar + DaysSnowFeb-Mar, with nesting territory as a random effect.
Figure 1. (A) Relationship between mean hatching date and number of days of snow cover in February–March, and (B) relationship between mean hatching date and mean March temperature for Red-shouldered Hawks in southwest Ohio and Hocking Hills study areas in southern Ohio, United States.
Annual mean hatching date did not exhibit a temporal trend over the 24 years of the study (SWOH alone: adjusted R2 = 0.05, P = 0.14, n = 24 years; HH alone: adjusted R2 = 0.0, P = 0.39, n = 20 years; both sites together: adjusted R2 = 0.03, P = 0.21, n = 24 years; Figure 2). Similarly, none of the weather variables tested exhibited a temporal trend over the 24-year period (mean February–March temperatures: SWOH adjusted R2 = 0.0, P = 0.86; HH adjusted R2 = 0.0, P = 0.60) (mean March temperature: SWOH adjusted R2 = 0.0, P = 0.53; HH adjusted R2 = 0.0, P = 0.37) (number of days of snow cover for February–March: SWOH: adjusted R2 = 0.0, P = 0.77; HH: adjusted R2 = 0.0, P = 0.78).
Figure 2. Annual mean hatching date for Red-shouldered Hawks and number of days of snow cover in February–March in southwest Ohio (SWOH) and Hocking Hills (HH) study areas in southern Ohio, United States.
Mean Red-shouldered Hawk hatching dates in southern Ohio did not advance over the 24 years from 1997 through 2020; our consistent methodology, large sample size, and length of study confer confidence in this result. Hatching dates were most influenced by the number of days with snow cover and the air temperature during the pre-laying period, with earlier hatching in years with less snow cover and warmer years. Although climate has warmed overall in Ohio (Frankson et al., 2017), snow cover in February-March and mean March temperature in our study areas showed no trend over the course of our study.
Red-shouldered Hawk hatching dates did not vary greatly in our long-term study; annual mean ranged from Julian day 110 to 121. Likewise, the variation in mean March temperature was moderate, ranging from –0.2oC to 7.7oC, although the variation in the number of days with snow cover was somewhat larger, ranging from 0 to 23 (total for February and March together; Figure 1). Our study areas in southern Ohio occupy the center of the eastern range of the species and reproductive rates here are mid-range to high (Dykstra et al., 2020), with relatively little year-to-year variation (Dykstra et al., 2021). Though climate change is occurring here (Frankson et al., 2017), our results indicated that effects were not detectable in February–March over the time period of 1997 to 2020, though temperatures in April and May did increase during that period in our study area (Dykstra et al., 2021). Despite the fairly modest amount of variation, we documented significant influence of snow cover and air temperature on breeding phenology in a long-term study. We predict that this relationship would be even more striking in areas where variation is greater and/or climate change is more dramatic or affects temperatures during the pre-laying period.
Warmer spring temperatures have been associated with earlier breeding in many raptor species, including Northern Goshawks (Accipiter gentilis) in Finland (Lehikoinen et al., 2013) and Idaho/Utah, United States (Bangerter et al., 2021), Peregrine Falcons (Falco peregrinus) in South Africa (Sumasgutner et al., 2020), Eurasian Kestrels (Falco tinnunculus) in Vienna (Kreiderits et al., 2016), and Common Buzzards (Buteo buteo) in Finland (Lehikoinen et al., 2009), among others (Nielsen and Møller, 2006; Dunn, 2019). Delayed egg-laying of Eurasian Kestrels is associated with more rain in the winter/early spring pre-laying period (Huchler et al., 2020) or in the spring (Constantini et al., 2010; Kreiderits et al., 2016). Delayed egg-laying of Rough-legged Hawks (Buteo lagopus; Terraube et al., 2015), Tengmalm’s Owls (Aegolius funereus; Kouba et al., 2020), Tawny Owls (Strix aluco; Solenen, 2014), and Ural Owls (Strix uralensis; Lehikoinen et al., 2011) is linked to increased winter/spring snow cover, which reduces the prey availability of some species of voles. However, delayed egg-laying of Burrowing Owls (Athene cunicularia) in several populations in the western United States is linked to winter drought conditions, which likely reduce prey abundance (Porro et al., 2020).
The effects of weather conditions on raptor breeding phenology are often attributed to indirect effects on prey abundance or availability, which influences the physiological condition of the female, which in turn influences laying date (Newton, 1986; Steenhof et al., 1997; Dunn, 2019; Porro et al., 2020; Bangerter et al., 2021). However, other causal relationships have been described; for example, advancing hatching dates of American Kestrels (Falco sparverius) are likely related to changes in the wintering locations of the birds (nearer the breeding sites; Heath et al., 2012). The effects of favorable weather and presumably higher prey populations can also be masked by changes in the proportion of a population that attempts breeding: for Northern Goshawks in the Great Basin (United States) experiencing favorable weather, more pairs occupied territories but average breeding date was later, possibly because more younger birds nested and this cohort typically breeds later than older birds (Bangerter et al., 2021).
For Red-shouldered Hawks in southern Ohio, the influence of snow cover during winter on hatching (and thus laying) date seems likely mediated by prey availability. Although Red-shouldered Hawks are dietary generalists (Strobel and Boal, 2010; Dykstra et al., 2020), they consume a significant amount of mammalian prey (56% by biomass) in the breeding season (Dykstra et al., 2003), and that number is likely higher during winter when reptile and amphibian prey are mostly unavailable at this latitude. As sit-and-wait perch hunters, Red-shouldered Hawks watch for prey (particularly voles, Microtus spp.) moving on the ground; any snow present provides these small mammals with cover, as they often tunnel through snow rather than run on the surface (Naughton, 2012). Thus, although southern Ohio receives little snow (average 57 cm/year, 1997–2020)4, the relationship between snow cover and hatching date in our study area was logical and supported our prediction, and the length of our study allowed detection of the subtle relationship. Associations between snow cover and breeding phenology are more common in subarctic or northern species (e.g., Lehikoinen et al., 2011; Solenen, 2014; Terraube et al., 2015; Kouba et al., 2020); the Red-shouldered Hawk population we studied in southern Ohio may be the southernmost raptor population for which snow cover has been associated with breeding phenology.
Published reports indicate that many raptor species studied did not exhibit advancing hatching dates. Breeding phenologies often reflected local weather conditions such as air temperature and snow depth (Supplementary Table 1).
Dietary preferences may play a role in the likelihood of a bird shifting its breeding phenology; in a meta-analysis, egg-laying dates of tertiary consumers (defined as all predators) advanced more rapidly than those of secondary consumers (Dunn and Møller, 2014; Dunn, 2019). According to the studies we reviewed (Supplementary Table 1), several raptors that have advanced hatching dates consume mainly birds (or insects); fewer of the mammal-eating raptors exhibited shifts. Because within-season variation in small mammal numbers can be less pronounced than seasonal peaks of some insect species (Huchler et al., 2020), we might predict that birds more dependent on insects would be more likely to shift breeding dates (Both et al., 2009), promoting a better match of peak food availability to the time of highest energy requirements of their young. If this tendency is extended up the trophic web, then ornithophagous raptors might also track hatching dates of their avian prey and be more likely to shift phenology than mammal-eating raptors. Indeed, this prediction is supported by several studies of owls that specialize on voles (Supplementary Table 1) and do not exhibit advancing breeding phenology. However, the advancing phenologies for Rough-legged Hawks (Terraube et al., 2015) and Common Buzzards (in Finland; Lehikoinen et al., 2009), which prey mostly on voles, do not fit that pattern. Despite its dependency on voles in Finland (Lehikoinen et al., 2009), the Common Buzzard is overall considered a dietary generalist, consuming mammals, birds (especially juveniles), reptiles and amphibians, and invertebrates; and commonly switching to alternate prey when availability of some species declines (Walls and Kenward, 2020 and references therein). Generalists’ ability to switch to alternate prey is expected to buffer the need to shift breeding phenology (Bretagnolle and Terraube, 2019); this may be the case for the generalist Red-shouldered Hawks we studied, though it was not for the other two Buteos mentioned above. In sum, any influence of trophic level or diet on the likelihood of breeding phenology shifts is not unequivocal, and may be confounded by impacts of other local factors; additional study is needed. Further, avian predator-prey interactions relative to climate change have been little-studied (Bretagnolle and Terraube, 2019; for exceptions, see Nielsen and Møller, 2006; Both et al., 2009), and complex effects may result from climate-induced changes in distribution, phenology, population dynamics, and other factors (Bretagnolle and Terraube. 2019).
Comparing multiple studies of the same species from different locations also highlights the complexity and diversity of raptor responses to climate change. For example, hatching date of Eurasian Sparrowhawks in Finland advanced by 0.18 days/year from 1973 to 2007 (Lehikoinen et al., 2010), but breeding phenology of this species did not advance in Netherlands (Both et al., 2009) or Denmark (Nielsen and Møller, 2006), despite advancing phenologies of their prey. These differences cannot be explained by diet, as sparrowhawk diet at the three locations was similar – primarily birds (Nielsen and Møller, 2006; Both et al., 2009; Lehikoinen et al., 2010 (see also Rytkonen et al., 1998 for diet in Finland)). Across many avian species, there is little consistency in phenology change among populations of the same species or closely related species; instead, factors such as diet and habitat are more important in determining the rate of laying date change (Dunn and Møller, 2014). It is probable that factors other than diet contribute to the likelihood of a phenology shift in raptor species, and that these factors differ among populations and regions.
Overall, the relative paucity of studies examining raptor breeding phenology relative to climate change limits our ability to generalize about impacts to this taxa (Bretagnolle and Terraube, 2019). We encourage researchers with appropriate long-term datasets to analyze and report trends or lack of trends. The complexity and diversity of raptor responses to climate change underscore the importance of long-term studies of raptors at multiple locations and highlight the challenges associated with conserving raptor communities in the face of climate change.
The raw data supporting the conclusions of this article will be made available by the authors, without undue reservation.
This research was conducted in compliance with the Guidelines to the Use of Wild Birds in Research. Banding was conducted under US Geological Survey master banding permit No. 23352 and multiple Ohio Wild Animal permits. Researchers do not belong to any institutions and no additional approvals or permits were required for the work.
CD conceived and designed the study. CD, JH, MS, and AW collected the data. CD and JH analyzed the data. CD wrote the initial draft of the manuscript. All authors reviewed and finalized it.
The authors declare that the research was conducted in the absence of any commercial or financial relationships that could be construed as a potential conflict of interest.
We thank Madeline Dykstra, Laura Dykstra, Sandra Stone, Irv Simon, Sara Miller, and Ania Wrona for assistance with fieldwork, Kelly Williams for statistical advice, and the nest landowners for their cooperation in allowing repeated access to the nests. We also thank RAPTOR, Inc., and its volunteers for assistance with information on birds admitted to their facility and for their support. This research was conducted in compliance with the Guidelines to the Use of Wild Birds in Research. Banding was conducted under US Geological Survey master banding permit No. 23352 and multiple Ohio Wild Animal permits.
The Supplementary Material for this article can be found online at: https://www.frontiersin.org/articles/10.3389/fevo.2021.658390/full#supplementary-material
Bangerter, A. B., Heiser, E. R., Carlisle, J. D., and Miller, R. A. (2021). Local weather explains annual variation in Northern Goshawk reproduction in the northern Great Basin, USA. J. Raptor Res. 55, 1–14.
Bloom, P. H., and McCrary, M. D. (1996). “The urban Buteo: Red-shouldered Hawks in southern California,” in Raptors in Human Landscapes: Adaptations to Built and Cultivated Environments, eds D. M. Bird, D. E. Varland, and J. J. Negro (London: Raptor Research Foundation and Academic Press), 31–39.
Bosakowski, T., and Smith, D. G. (1997). Distribution and species richness of a forest raptor community in relation to urbanization. J. Raptor Res. 31, 26–33.
Bosakowski, T., Smith, D. G., and Speiser, R. (1992). Status, nesting density, and macrohabitat selection of Red-shouldered Hawks in northern New Jersey. Wilson Bull. 104, 434–446.
Both, C., van Asch, M., Bijlsma, R. G., van den Burg, A. B., and Visser, M. E. (2009). Climate change and unequal phenological changes across four trophic levels: constraints or adaptations? J. Anim. Ecol. 78, 73–83. doi: 10.1111/j.1365-2656.2008.01458.x
Bretagnolle, V., and Terraube, J. (2019). “Predator-prey interactions and climate change,” in Effects of Climate Change on Birds, Second edition, eds P. O. Dunn and A. P. Møller (Oxford: Oxford University Press), 199–220. doi: 10.1093/oso/9780198824268.003.0015
Constantini, D., Carello, L., and Dell’Omo, G. (2010). North Atlantic Oscillation index and reproductive traits in Mediterranean kestrels. J. Zool. 280, 177–184. doi: 10.1111/j.1469-7998.2009.00649.x
Dunn, P. O. (2019). “Changes in timing of breeding and reproductive success in birds,” in Effects of Climate Change on Birds, 2nd Edn. eds P. O. Dunn and A. P. Møller (Oxford: Oxford University Press), 108–119. doi: 10.1093/oso/9780198824268.003.0009
Dunn, P. O., and Møller, A. P. (2014). Changes in breeding phenology and population size of birds. J. Anim. Ecol. 83, 729–739. doi: 10.1111/1365-2656.12162
Dunn, P. O., and Møller, A. P. (2019). Effects of Climate Change on Birds, 2nd Edn. Oxford: Oxford University Press.
Dykstra, C. R., Bloom, P. H., and McCrary, M. D. (2018). “Red-shouldered Hawk: Adaptable denizen of the suburbs,” in Urban Raptors: Ecology and Conservation of Birds of Prey in Cities, eds C. W. Boal and C. R. Dykstra (Washington, DC: Island Press), 110–125. doi: 10.5822/978-1-61091-841-1_8
Dykstra, C. R., Hays, J. L., and Crocoll, S. T. (2020). “Red-shouldered Hawk (Buteo lineatus), version 1.0,” in Birds of the World, ed. A. F. Poole (Ithaca, NY: Cornell Lab of Ornithology), doi: 10.2173/bow.reshaw.01
Dykstra, C. R., Hays, J. L., Daniel, F. B., and Simon, M. M. (2000). Nest site selection and productivity of suburban Red-shouldered Hawks in southern Ohio. Condor 102, 401–408. doi: 10.1093/condor/102.2.401
Dykstra, C. R., Hays, J. L., and Simon, M. M. (2009). Spatial and temporal variation in reproductive rates of the Red-shouldered Hawk in suburban and rural Ohio. Condor 111, 177–182. doi: 10.1525/cond.2009.080002
Dykstra, C. R., Hays, J. L., Simon, M. M., and Daniel, F. B. (2003). Behavior and prey of nesting Red-shouldered Hawks in southwestern Ohio. J. Raptor Res. 37, 177–187.
Dykstra, C. R., Hays, J. L., Simon, M. M., Wegman, A. R., Dykstra, L. R., and Williams, K. A. (2021). Habitat and weather conditions influence reproductive rates of suburban and rural Red-shouldered Hawks Buteo lineatus. Ibis 163, 623–640. doi: 10.1111/ibi.12877
Dykstra, C. R., Hays, J. L., Simon, M. M., Wegman, A. W., Williams, K. A., and Dykstra, L. R. (2019). Dispersal and survival of Red-shouldered Hawks banded in suburban southern Ohio, 1996–2018. J. Raptor Res. 53, 276–292. doi: 10.3356/jrr-19-15
Frankson, R., Kunkel, K., Champion, S., and Easterling, E. (2017). Ohio state Climate Summary, September 2019. NOAA Technical Report NESDIS 149-OH, National Oceanic and Atmospheric Administration National Centers for Environmental Information. Available online at: https://statesummaries.ncics.org/chapter/oh/#:∼:text=Basedon%20 observations%20through%202018,%20in%20the%20winter%20and%20spring (accessed 31 October 2019).
Heath, J. A., Steenhof, K., and Foster, M. A. (2012). Shorter migration distances associated with higher winter temperatures suggest a mechanism for advancing nesting phenology of American Kestrels Falco sparverius. J. Avian Biol. 43, 376–384. doi: 10.1111/j.1600-048x.2012.05595.x
Huchler, K., Schulze, C. H., Gamauf, A., and Sumasgutner, P. (2020). Shifting breeding phenology in Eurasian Kestrels Falco tinnunculus: effects of weather and urbanization. Front. Ecol. Evol. 8:247. doi: 10.3389/fevo.2020.00247
Kouba, M., Bartos, L., Bartošová, J., Hongisto, K., and Korpimäki, E. (2020). Interactive influences of fluctuations of main food resources and climate change on long-term population decline of Tengmalm’s Owls in the boreal forest. Sci. Rep. 10:20429. doi: 10.1038/s41598-020-77531-y
Kreiderits, A., Gamauf, A., Krenn, H. W., and Sumasgutner, P. (2016). Investigating the influence of local weather conditions and alternative prey composition on the breeding performance of urban Eurasian Kestrels Falco tinnunculus. Bird Study 63, 369–379. doi: 10.1080/00063657.2016.1213791
Lehikoinen, A., Byholm, P., Ranta, E., Saurola, P., Valkama, J., Korpimäki, E., et al. (2009). Reproduction of the common buzzard at its northern range margin under climate change. Oikos 118, 829–836. doi: 10.1111/j.1600-0706.2008.17440.x
Lehikoinen, A., Lindén, A., Byholm, P., Ranta, E., Saurola, P., Valkama, J., et al. (2013). Impact of climate changes and prey abundance on nesting success of a top predator, the goshawk. Oecologia 171, 283–293. doi: 10.1007/s00442-012-2411-z
Lehikoinen, A., Ranta, E., Pietiäinen, H., Byholm, P., Saurola, P., Valkama, J., et al. (2011). The impact of climate and cyclic food abundance on the timing and brood size in four boreal owl species. Oecologia 165, 349–355. doi: 10.1007/s00442-010-1730-1
Lehikoinen, A., Saurola, P., Byholm, P., Lindén, A., and Valkama, J. (2010). Life history events of the Eurasian Sparrowhawk Accipiter nisus in a changing climate. J. Avian Biol. 41, 627–636. doi: 10.1111/j.1600-048x.2010.05080.x
Naughton, D. (2012). The Natural History of Canadian Mammals. Toronto: Canadian Museum of Nature and University of Toronto Press.
Nielsen, J. T., and Møller, A. P. (2006). Effects of food abundance, density and climate change on reproduction in the sparrowhawk Accipiter nisus. Oecologia 149, 505–518. doi: 10.1007/s00442-006-0451-y
Pagel, J. E., and Thorstrom, R. K. (2007). “Accessing nests,” in Raptor Research and Management Techniques, eds D. M. Bird and K. L. Bildstein (Blaine, WA: Hancock House Publishers Ltd), 171–180.
Penak, B. L., Dykstra, C. R., Miller, S. J., and Bird, D. M. (2013). Using morphometric measurements to estimate age of nestling Red-shouldered Hawks in two eastern populations. Wilson J. Ornithol. 125, 630–637. doi: 10.1676/13-020.1
Porro, C. M., Desmond, M. J., Savidge, J. A., Abadi, F., Cruz-McDonnell, K. K., Davis, J. L., et al. (2020). Burrowing Owl (Athene cunicularia) nest phenology influenced by drought on nonbreeding grounds. Auk 137, 1–17. doi: 10.1093/auk/ukaa008
Root, T. L., Price, J. T., Hall, K. R., Schneider, S. H., Rosenzweig, C., and Pounds, J. A. (2003). Fingerprints of global warming on wild animals and plants. Nature 421, 57–60. doi: 10.1038/nature01333
Rytkonen, S., Kuokkanen, P., Hukkanen, M., and Huhtala, K. (1998). Prey selection by sparrowhawks Accipiter nisus and characteristics of vulnerable prey. Ornis Fenn. 75, 77–87.
Solenen, T. (2014). Timing of breeding in rural and urban Tawny Owls Strix aluco in southern Finland: effects of vole abundance and winter weather. J. Ornithol. 155, 27–36. doi: 10.1007/s10336-013-0983-y
Steenhof, K., Kochert, M. N., and McDonald, T. L. (1997). Interactive effects of prey and weather on Golden Eagle reproduction. J. Anim. Ecol. 66, 350–362. doi: 10.2307/5981
Stevenson, I. R., and Bryant, D. M. (2000). Climate change and constraints on breeding. Nature 406, 366–367. doi: 10.1038/35019151
Strobel, B. N., and Boal, C. W. (2010). Regional variations in diet of breeding Red-shouldered Hawks. Wilson J. Ornithol. 122, 68–74. doi: 10.1676/09-071.1
Sumasgutner, P., Jenkins, A., Amar, A., and Altwegg, R. (2020). Nest boxes buffer the effects of climate on breeding performance in an African urban raptor. PLoS One 15:e0234503. doi: 10.1371/journal.pone.0234503
Terraube, J., Villers, A., Ruffino, L., Iso-Iivari, L., Henttonen, H., Oksanen, T., et al. (2015). Coping with fast climate change in northern ecosystems: mechanisms underlying the population-level response of a specialist avian predator. Ecography 38, 690–699. doi: 10.1111/ecog.01024
Thackeray, S. J., Sparks, T. H., Frederiksen, M., Burthe, S., Bacon, P. J., Bell, J. R., et al. (2010). Trophic-level asynchrony in rates of phenological change for marine, freshwater, and terrestrial environments. Glob. Chang. Biol. 16, 3304–3313. doi: 10.1111/j.1365-2486.2010.02165.x
Titus, K., and Mosher, J. A. (1981). Nest-site habitat selected by woodland hawks in the central Appalachians. Auk 98, 270–281.
Visser, M. E., van Noordwijk, A. J., Tinbergen, J. M., and Lessells, C. M. (1998). Warmer springs lead to mistimed reproduction in Great Tits (Parus major). Proc. R. Soc. Lond. B 265, 1867–1870. doi: 10.1098/rspb.1998.0514
Keywords: breeding phenology, Buteo lineatus, climate change, hatching date, raptor, Red-shouldered Hawk, snow cover, temperature
Citation: Dykstra CR, Hays JL, Simon MM and Wegman AR (2021) Breeding Phenology of Red-Shouldered Hawks (Buteo lineatus) Is Related to Snow Cover and Air Temperature During the Pre-Laying Period. Front. Ecol. Evol. 9:658390. doi: 10.3389/fevo.2021.658390
Received: 25 January 2021; Accepted: 03 May 2021;
Published: 07 June 2021.
Edited by:
Keith L. Bildstein, Independent Researcher, Blandon, FL, United StatesReviewed by:
Richard Patrick Reading, Butterfly Pavilion, United StatesCopyright © 2021 Dykstra, Hays, Simon and Wegman. This is an open-access article distributed under the terms of the Creative Commons Attribution License (CC BY). The use, distribution or reproduction in other forums is permitted, provided the original author(s) and the copyright owner(s) are credited and that the original publication in this journal is cited, in accordance with accepted academic practice. No use, distribution or reproduction is permitted which does not comply with these terms.
*Correspondence: Cheryl R. Dykstra, Y2hlcnlsZHlrc3RyYUBnbWFpbC5jb20=
Disclaimer: All claims expressed in this article are solely those of the authors and do not necessarily represent those of their affiliated organizations, or those of the publisher, the editors and the reviewers. Any product that may be evaluated in this article or claim that may be made by its manufacturer is not guaranteed or endorsed by the publisher.
Research integrity at Frontiers
Learn more about the work of our research integrity team to safeguard the quality of each article we publish.